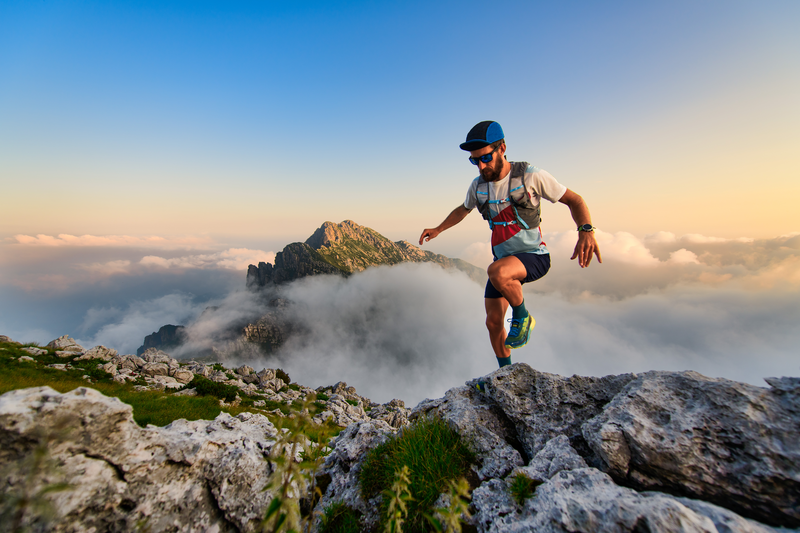
94% of researchers rate our articles as excellent or good
Learn more about the work of our research integrity team to safeguard the quality of each article we publish.
Find out more
BRIEF RESEARCH REPORT article
Front. Toxicol. , 02 October 2024
Sec. Regulatory Toxicology
Volume 6 - 2024 | https://doi.org/10.3389/ftox.2024.1438890
This article is part of the Research Topic Emerging topics on chemical safety assessment View all 8 articles
Neonicotinoids are the most widely used class of insecticides in the United States (U.S.). and the world. Consistent with their high use and persistence, neonicotinoids are often found contaminating drinking water and food. They are also detected in human urine, breast milk, amniotic and cerebrospinal fluids, as well as the brains of treated rodents. Neonicotinoids were once thought to pose little neurotoxic risk to humans, but a growing body of research challenges that assumption. In this study we provide the first comprehensive assessment of unpublished rodent developmental neurotoxicity (DNT) studies on five neonicotinoids that were submitted to the U.S. Environmental Protection Agency (EPA) by neonicotinoid manufacturers. Groups of female rats were administered three different doses of a neonicotinoid during pregnancy and lactation, and their offspring subjected to various neurological tests and brain measurements. We identified nicotine-like effects such as reduced brain size, indicative of neuronal cell loss. Statistically significant shrinkage of brain tissue was observed in high-dose offspring for five neonicotinoids: acetamiprid, clothianidin, imidacloprid, thiacloprid, and thiamethoxam. Two brain regions reduced in the rodent studies–the corpus callosum and caudate-putamen–tend to be smaller in people diagnosed with attention-deficit hyperactivity disorder (ADHD), and in children of mothers who smoked during pregnancy, suggesting a possible link between perinatal neonicotinoid exposure and ADHD. A decreased auditory startle reflex was reported for acetamiprid at all doses and was statistically significant in the mid- and high-dose offspring, and for clothianidin in juvenile high-dose females. No mid- or low-dose brain morphometric data were submitted for acetamiprid, imidacloprid, or thiacloprid. Thiamethoxam mid- and low-dose brain morphometric data were provided to EPA upon request. Only partial mid-dose brain morphometry data were submitted for clothianidin, but no low-dose data. Yet despite this lack of data, EPA concluded that only the high-dose brain morphometric effects were treatment-related–setting the mid-dose as the study’s No Observed Adverse Effect Level (NOAEL) or failing to find a definitive NOAEL for acetamiprid, clothianidin, imidacloprid, thiacloprid and thiamethoxam. We found numerous deficiencies in EPA’s regulatory oversight and data analyses. EPA dismissed statistically significant adverse effects, accepted substandard DNT studies despite lack of valid positive control data, and allowed neonicotinoid registrants to unduly influence agency decision-making. We conclude that perinatal exposure to neonicotinoids and their metabolites induces adverse, nicotine-like neurotoxic effects in rodent bioassays, and that the exposure limits set by EPA for human exposure are either not protective or not supported by available neurotoxicity data. We propose regulatory changes to empower EPA to better protect public health from developmental neurotoxins like neonicotinoids.
Most major classes of insecticides act by disrupting the nervous system through pathways that are conserved across invertebrate and vertebrate species (U.S. EPA, 2024b). For instance, both the organophosphate (OP) and the carbamate classes of insecticides are designed to disrupt cholinergic nerve function (Soltaninejad and Shadnia, 2014). Similarly, a newer class of insecticides, the neonicotinoids (neonics), act as cholinergic receptor agonists by binding to nicotinic acetylcholine receptors (nAChRs), which results in the opening of calcium and other cation channels. By this mechanism the neonicotinoid pesticides exert their lethal effect on invertebrates (Tomizawa and Casida, 2003).
Neonics are now the most widely used insecticides in the US and globally with over three-quarters of neonicotinoids used as seed treatments, coated onto seeds of crops before dispersal (see Figure 1) (Douglas and Tooker, 2015; Douglas et al., 2024). Neonicotinoid seed coatings have dramatically expanded the amount of farmland treated with insecticides: at least 150 million acres in 2012 (Steeger, 2014), six times the amount of land treated with the top ten insecticides combined in 2001 (U.S. EPA, 2024b). Non-agricultural uses of neonics include lawns and gardens, parks and playgrounds, indoor bed bug treatment, and flea and tick treatments for pets.
Figure 1. U.S. Geological survey data on neonicotinoid use (Wieben, 2021). Seed coating uses included up through 2014; Kynetec, the firm that supplies USGS with usage data, stopped reporting the amounts of neonicotinoids in seed coatings in 2015.
With such widespread use, neonics routinely contaminate: waterways and tap water (Goulson, 2013; Klarich Wong et al., 2019; Millemann et al., 2020; Aggarwal, 2021); foods including fruits, vegetables and baby foods (Craddock et al., 2019; Zhang et al., 2019; USDA, 2022); and even human breast milk (Chen et al., 2020). Based on these food and water monitoring reports, it seems likely that a child growing up today may have been exposed to neonic pesticides during fetal development from in utero exposure, in infancy from contaminated breast milk and formula reconstituted with neonic-contaminated tap water, and into childhood from consuming contaminated drinking water and baby foods. Programs that conduct pesticide food and water monitoring, as well as biomonitoring, should continue and be expanded.
Given the potential for people to be regularly exposed to neonicotinoids, including during vulnerable periods of early life development, it is important to ensure that risk evaluations and regulatory approval of these neurotoxic insecticides meet (and hopefully exceed) the legal protections required by federal pesticide law.
The rodent Developmental Neurotoxicity Study (DNT) is one of several studies EPA uses to determine whether a pesticide poses a particular risk when exposures take place during early development of the brain and nervous system. This is because fetal and early infant life is when the mammalian brain and nervous system is being built. Neurotransmitters and their receptors help coordinate the process; they promote cell replication, initiate differentiation into different cell types, trigger then terminate formation of axons and synapses, regulate cell death and promote cell migration to specific brain regions to form the final architecture of the brain (England et al., 2017; Loser et al., 2021). If this complex and fragile developmental process is disrupted by xenobiotics, there is little opportunity for repair, and the damage can be permanent (Rice and Barone, 2000). The DNT study is known as a “guideline” study because it follows standardized Test Guidelines (U.S. EPA, 1998; OECD, 2007) to provide regulatory agencies with information needed to determine dose-response values and exposure limits.
Generally speaking, EPA sets maximum limits for acute (one-time) and chronic (lifetime) exposure by first deriving a no observed adverse effect level (NOAEL) from one or more guideline animal studies conducted with a pesticide. To set the human exposure limit, EPA divides the NOAEL by an uncertainty factor that is normally 100 (10 for interspecies extrapolation from a rodent study, and 10 for intraspecies differences across the human population) (U.S. EPA, 2002c). However, neither factor accounts for the greater susceptibility to pesticidal harm when exposure occurs in utero or in early life.
The Food Quality Protection Act (FQPA) of 1996 mandated that EPA consider available information concerning “the special susceptibility of infants and children,” including “neurological differences between infants and children and adults, and effects of in utero exposure to pesticide chemicals,” to ensure a “reasonable certainty” that “no harm” will result “from aggregate exposure” to a pesticide, including “all anticipated dietary and all other exposures for which there is reliable information” (FQPA, 1996).
The FQPA child protective factor is one way EPA can ensure a margin of protection–by reducing allowable exposure by a factor of 10 to account for the greater susceptibility of the young to developmental toxicants (EPA, 2002a). FQPA puts the burden of proof squarely on EPA to ensure that all uses of a pesticide meet the “reasonable certainty of no harm” standard for the general population and for every age group of children, including aggregate exposures from food, drinking water, and all household uses such as flea treatments for pets. The FQPA also mandates cumulative assessment of pesticides that share a common mechanism of toxicity. By law, EPA can modify or eliminate the FQPA 10X safety factor “only if, on the basis of reliable data, such margin will be safe for infants and children.” (FQPA, 1996). Unfortunately, EPA has too often reduced or removed this important child-protection factor from its pesticide assessments, including for the neonicotinoids (Naidenko, 2020).
As part of implementing the FQPA, the EPA has required pesticide manufacturers (called “registrants”) to conduct a rodent test following a guideline specifically for DNT (U.S. EPA, 1998; OECD, 2007). The guideline method specifies that groups of female rats are fed differing doses of the test substance during pregnancy and lactation to assess potential effects on the neurological development through adulthood (postnatal day 60 or later) of offspring exposed in utero and in mother’s milk. The DNT guideline test includes neuropathology assessments, neurobehavioral endpoints, and body weight and other parameters common to other toxicity studies. There are four test methods for behavior: a functional observation battery (FOB); an open-field locomotor test to measure motor activity; an auditory startle test that measures the reflexive response to intense acoustic stimuli; and some tests for learning and memory such as a water maze test and a passive avoidance test. Developmental landmarks are recorded, including the ability to roll and reflexes for surface righting, time of eye opening and pupil constriction reflexes, and vaginal patency in females and preputial separation in males. Post-mortem observations include brain weight and brain histology to evaluate potential neuropathology. At postnatal day (PND) 11 and study termination, morphometric analysis to assess structural development of the brain is performed on various brain regions, such as structures within the neocortex, hippocampus and cerebellum, as well as subcortical regions like caudate-putamen and corpus callosum (U.S. EPA, 1998). The DNT study can be used to establish an acceptable exposure threshold for an acute (single) exposure, known as the acute reference dose, because “there is a presumption that effects during development may result from a single exposure” (U.S. EPA, 2002c).
Here we evaluate EPA’s DNT Data Evaluation Records (DERs), comprehensive evaluations prepared by EPA staff, for five unpublished DNT studies submitted to EPA by neonicotinoid manufacturers.
Although EPA produced a summary data report for dinotefuran, we did not include it in our analysis because it did not record any significant adverse effects. EPA noted that there were no adverse effects on litter number or offspring viability at the high dose, and that there were no deficiencies with the study; it was classified by EPA as “acceptable” (U.S. EPA, 2013).
Below and summarized in Table 1, we report evidence of brain tissue thinning in at least some of the offspring in the high dose treatment group of DNT studies with all five of the neonics we analyzed–acetamiprid, clothianidin, imidacloprid, thiacloprid, and thiamethoxam. The DNT guideline only requires that brain morphometric data be submitted to EPA for the mid and low dose groups if there is pathology in the high dose group. Unfortunately, other than for thiamethoxam, EPA received either no data or only partial data from each registrant for the mid and low dose groups. Even lacking brain morphometric data for mid and low doses, EPA presumed that the effect was only at the high dose. Other endpoints where EPA reported statistically significant adverse effects are discussed, and identified in the Table as “yes.” Where no statistically significant effects were identified, the space is left blank in the Table.
Table 1. Summary of significant adverse findings identified by EPA Data Evaluation Records (DERs) of Registrant-sponsored Developmental Neurotoxicity (DNT) studies for neonicotinoid pesticides.
In a 2003 DNT study sponsored by Nippon Soda (Nemec, 2003), acetamiprid was administered via gavage to pregnant/lactating rats at doses of 0, 2.5, 10 or 45 mg/kg/day from gestation day 6 (GD6) through PND21. The study was first assessed in 2004, then revisited in 2007 and 2008 in response to the sponsor’s objections (U.S. EPA, 2008). EPA reviewers were unable to conclude whether or not acetamiprid affected learning or memory due to high variability in the test results, and effects on motor activity were uncertain due to problems with the control data: namely, that the normal developmental pattern for locomotion and motor activity was not seen in male control animals, and that the level of motor activity in control males seemed high. Brain morphometric data were only provided for the control and high dose animals. At PND72, the length of the ventral limb of the dentate hilus of the hippocampal formation was reduced by 15% in both male and female offspring, a statistically significant finding in the females (U.S. EPA, 2008).
The agency identified a dose-responsive decrease in the maximum auditory startle response in male offspring of all dosage groups at both timepoints (PND20/PND60): low-dose 15%/10%; mid-dose 27%/40%; and high-dose 42%/53% (U.S. EPA, 2008). The EPA’s statistical analysis identified the mid-dose as a significant effect level when data from male and female pups from PND20 and 60 were combined. The registrant contested EPA’s conclusions in a rebuttal report, arguing that the mid-dose was a no-effect level based on statistical analyses by two consulting groups (U.S. EPA, 2008). EPA statisticians rejected the consultants’ analyses due to inappropriate use of models and statistical errors (U.S. EPA, 2008). The European Food Safety Authority (EFSA et al., 2024) likewise rejected the pesticide industry’s statistical interpretation and set a no-effect level at the low dose of 2.5 mg/kg/day (EFSA, 2015). With the standard 100X uncertainty factor and the 10X FQPA child protective factor, the maximum acute exposure level regarded as safe for infants and children, known as the acute population, adjusted dose (aPAD), would be 2.5/1,000 = 0.0025 mg/kg/day.
In 2008, the EPA without explanation overruled its own statisticians’ conclusions and raised the offspring NOAEL to the mid-dose of 10 mg/kg/day in accordance with the registrant’s request (U.S. EPA, 2008). In 2017, EPA removed the FQPA child protective factor (reduced from 10X to 1X) in part based on the DNT study (U.S. EPA, 2017). These two changes together increased the aPAD by 40-fold to 0.1 mg/kg/day.
In Table 2, we show how these different aPADs result in radically different risk pictures (see Table 2 footnote). Based on EPA’s upperbound estimates of acute dietary exposure to acetamiprid, infants and children are exposed to 64%–87% of EPA’s official aPAD–where anything under 100% is considered acceptable (U.S. EPA, 2017). In contrast, that same exposure level exceeds a protective aPAD of 0.0025 mg/kg/day by a substantial 25–35-fold. Details on how EPA calculates dietary risk is in the 2017 acetamiprid draft human health risk assessment (U.S. EPA, 2017). In brief, dietary exposure is calculated as the combined exposure from both food residues and drinking water sources. Drinking water levels are predicted for both surface and groundwater sources using models. Food exposure is predicted using models populated with food consumption data from the US Department of Agriculture’s survey of “What We Eat in America.” Age-adjusted body weights and ingestion factors come from EPA’s Exposure Factors Handbook (U.S. EPA, 2024a).
The more protective aPAD we propose for acetamiprid, 0.0025 mg/kg/day, is similar to what the European Food Safety Authority (EFSA) is now proposing. EFSA is recommending that the acceptable daily intake (ADI) of acetamiprid be lowered from 0.025 down to 0.005 mg/kg per day, to be more protective of potential developmental neurotoxicity risks (EFSA et al., 2024). EFSA’s 84-page report supporting the recommendation includes the results of a systematic review of public literature discussing evidence of acetamiprid and DNT effects from both in vitro and in vivo studies, including ones cited in this manuscript. We refer readers to that report for further details.
In a DNT study sponsored by Takeda Chemical Industries in 2000, female rats were fed clothianidin in the diet from GD0 to PND22 at doses of 0, 13, 43 and 142 mg/kg/day during gestation (Hoberman, 2000).
EPA flagged several serious study deficiencies that, to our knowledge, were never remedied. The study sponsor failed to provide EPA with the brain morphometric data for the low dose group. At the mid-dose, morphometric data were provided for females but not for males. For the mid dose females the brain morphometry data was provided to EPA only as a mean of both brain hemispheres, instead of separately (U.S. EPA, 2005). EPA noted all this in its list of study deficiencies and requested that any additional morphometric measurements should be submitted to EPA. Additional study deficiencies noted by EPA included no mention of any test results for pupillary function such as constriction and response to light.
Of the 17 brain measurements taken at PND12 and termination, among the high dose animals, statistically significant differences were reported for 6 measurements in females (2 increased, and 4 decreased) and 3 measurements in males (two increased, and 1 decreased). At termination, the 4 reported differences were all decreases (3 in females, 1 in males), suggesting that by about 3 months of age (PND83-87) the neurodevelopmental effects of clothianidin may include a thinning of brain tissues.
For the mid dose brain morphometric data for females, there were no statistically significant effects on brain measurements (U.S. EPA, 2005). It remains unknown whether the conclusion may have been different had the registrant submitted data for the individual hemispheres, and if the male data had also been submitted. The study remains classified as deficient for lacking this information.
Despite this, EPA set the offspring neurotoxicity NOAEL at the mid-dose based on high-dose effects, including decreased motor activity (number and duration of movements) in male offspring, decreased auditory startle response in female offspring and, at termination (PND83-87), a 5% thinning of the hippocampal gyrus in both sexes and a 6% reduction in caudate putamen thickness in females (U.S. EPA, 2005).
In a 2001 DNT study conducted by Bayer (Sheets, 2001), imidacloprid was fed to three groups of pregnant/lactating Wistar rats from GD0 to PND21. Doses during gestation were 0, 8, 19 and 55 mg/kg/day. After weaning, offspring were given untreated feed and evaluated until 75 days of age.
EPA identified two major treatment-related neurodevelopmental effects (U.S. EPA, 2002b). First, the thickness of the caudate/putamen brain region was reduced by 5.4% in high-dose female pups at PND11 and by 2% at study termination (PND70), described by EPA as a “persistent change” in this structure. Second, motor activity was reduced in high-dose male and female pups at PND17, and in female pups at PND21 (U.S. EPA, 2002b). Though not statistically significant, EPA regarded the reduced motor activity as treatment-related and adverse due to its consistency in both sexes and magnitude (31%–38%). In a separate review of the same study, the California Department of Pesticide Regulation found a significant 27% reduction in the thickness of the corpus callosum in high-dose females at PND11 (Cal, 2006). The corpus callosum effects were not identified or reported by EPA.
Bayer did not comply with an EPA directive to supply caudate/putamen morphometric measurements for low- or mid-dose female animals (U.S. EPA 2002b), as required by both EPA and OECD Test Guidelines (U.S. EPA, 1998, OECD, 2007). Despite not having adequate data to assess harmful effects on the caudate/putamen, corpus callosum or other brain structures at the low- and mid-dose, in 2002 EPA set the offspring NOAEL at the mid-dose (U.S. EPA, 2002b).
In a 2001 DNT study sponsored by Bayer Corporation (Hoberman, 2001), female rats were administered thiacloprid in the diet from GD0 to PND22 at 0, 4.4, 25.6 and 40.8 mg/kg/day during gestation. Brain weight and neuropathology were assessed at PND12 and PND68-79. A number of brain regions were adversely affected in male offspring at the high dose, including statistically significant 4% reductions in the corpus striatum, a region that encompasses the caudate-putamen, at both PND12 and termination; a 14% reduction in the corpus callosum at PND12; and a 5% reduction in the dentate gyrus at termination. EPA noted that “a definitive NOAEL was not established for these findings” given the lack of data for the mid- and low-doses (U.S. EPA, 2003a).
In tests of passive avoidance and behavior retention, females showed significantly poorer performance at the mid-dose and high-dose treatments compared with controls (U.S. EPA, 2003a). EPA identified “suggestive effects” on motor activity and auditory startle reflex in both the mid- and high-dose groups that were not statistically significant.
Sexual maturation was statistically significantly delayed by an average of a half to a full day in the mid and high dose male pups (as measured by preputial separation), and in the high dose female pups (as measured by vaginal patency) (U.S. EPA, 2003a). These are measurements of hormone-dependent developmental landmarks of sexual maturity that occur at the time of puberty in both rats and humans.
EPA’s documented concerns with the positive control data that was submitted with the study were substantial: “Most of the positive control studies are unacceptable for use with the current study. … None of the studies demonstrated the laboratory’s ability to detect major functional neurotoxic endpoints using the observational methods used in the current study.” (U.S. EPA, 2003a). EPA's list of study deficiencies was a page long, with the lack of acceptable positive control data listed last. Other study deficiencies identified by EPA included: inadequate description of the methods used to evaluate functional behavior; motor activity never habituated, with no explanation provided; the termination of the study with final brain pathology data was over an 11-day period, with no explanation for this wide range of ages at study termination; brain measurements were made bilaterally but only reported as the mean value of both hemispheres; although treatment-related alterations in brain morphology were reported for the high-dose, the brain morphometry at the mid and low dose levels were required but were not received.
The study was judged “unacceptable” due to numerous serious deficiencies, including failure to supply brain morphometry, for low- and mid-dose groups. Because of this, EPA could not set a definitive NOAEL for offspring and arbitrarily applied a 3X “database uncertainty factor” in calculating the effect concentration (U.S. EPA, 2003b).
In a 2003 DNT study sponsored by Syngenta Crop Protection Inc. (Brammer, 2003), thiamethoxam was administered in the diet to female rats from GD7 to PND22 at doses of 0, 4, 35 and 300 mg/kg/day during gestation. Brain morphometry was conducted on high-dose animals sacrificed at PND12 and at study termination on PND63. Upon request by EPA, Syngenta submitted mid- and low-dose brain morphometric data, which were analyzed in a separate DER in 2007 (U.S. EPA, 2007).
Thiamethoxam reduced brain weight significantly at termination in high-dose males and females as well as mid-dose females. Of the 14 brain regions/parameters that were analyzed in the male offspring at termination, 12 of the high-dose parameters were significantly reduced (by 5%–20%) compared with control animals (U.S. EPA, 2007). At the mid-dose, 9 of the parameters were reduced in size compared with controls, 6 of the regions were reduced by 2%–13%, and 3 were statistically significant reductions. Among low-dose male offspring at termination, 6 of 12 regions were reduced in size (by 5%–15%), and 2 were statistically significant (U.S. EPA, 2007).
The most consistently affected brain regions across sexes and doses were the dorsal cortex, the thalamus, and the corpus callosum–the latter’s thickness reduced by 20% and 16% in high-dose males at termination and females at PND12, respectively (U.S. EPA, 2007). Significant changes in the male thalamus at termination included reduced height (high-dose), reduced width (mid- and high-dose), and decreased overall width of the thalamus/cortex (all doses). The thalamus width of females was significantly reduced in all dosage groups at PND12. The dorsal cortex thickness of males at termination was significantly reduced by 11%–15% in all dosage groups in one set of level 3 specimens, and by 6%–11% in high-dose males for three other sets of specimens (levels 3, 4 and 5).
Age at sexual maturation in male offspring (measured as preputial separation) was delayed across all thiamethoxam treatment groups, by an average of a half-day at the low dose and an average of 1.5 days at the high dose (U.S. EPA, 2007). The delay was statistically significant in the low (p < 0.05) and high dose group (p < 0.01), compared with control animals. EPA notes in its report that the study did not monitor or report on other developmental landmarks such as tooth eruption and ear pinna unfolding.
Despite the treatment-related effects in offspring of all dosage groups and both sexes, including reduced brain weight in mid-dose females, EPA concluded that only effects at the high dose were treatment-related and set the study offspring NOAEL at the mid-dose, 35 mg/kg/day (U.S. EPA, 2020).
Our review of the EPA data reports for rodent DNT studies consistently found a significant reduction in brain tissue in high-dose offspring for five neonicotinoids: acetamiprid, clothianidin, imidacloprid, thiacloprid, and thiamethoxam.
Additionally, reported effects of acetamiprid include reduced auditory startle reflex at all doses, with statistical significance in the mid- and high-dose groups. The clothianidin DNT also reported reduced auditory startle reflex in high-dose juvenile females. Decreased motor activity was observed for clothianidin (high-dose males) and imidacloprid (high-dose in both sexes). The thiamethoxam DNT recorded delayed sexual maturation in male offspring across all doses that was statistically significant at the low and high dose. Thiacloprid was associated with poor behavior retention in mid- and high-dose females, and with delayed sexual maturation in the mid and high dose male pups, and in the high dose female pups (See Table 1).
Because the study sponsor failed to submit to EPA the required brain morphometric data for mid- or low-dose groups for acetamiprid, imidacloprid, or thiacloprid, a true NOAEL for the morphometric brain effects cannot be determined. Thiamethoxam’s mid- and low-dose data were supplied to the EPA upon request. For clothianidin only the female mid-dose data were given to EPA, but not male mid-dose or the low dose for either sex. Despite these data gaps, EPA designated the mid dose (for which in most cases it had no data) as the NOAEL for all five neonic pesticides. In addition to the obvious problems with determining a NOAEL without supportive data, in some cases this determination was contrary to the recommendations of the scientist that reviewed the data (acetamiprid) or was made despite a lengthy list of concerns regarding study deficiencies (thiacloprid).
The precise mechanisms of the effects we identified are unclear, and it is beyond the scope of our study to explore them in detail (the regulatory DNT studies are intended only to identify endpoints associated with developmental neurotoxicity and to quantify potential differences in life-stage susceptibility, not investigate mode of action.) However, some insights might be gleaned from the extensive body of research on nicotine, a well-established developmental neurotoxin (Slotkin, 2008; England et al., 2017; Castro et al., 2023), based on their extensive similarities.
Neonicotinoids are similar in structure to nicotine, and like it are agonists of nicotinic acetylcholine receptors (nAChRs) (Kimura-Kuroda et al., 2012). Neonicotinoids penetrate the blood-brain barrier (Hirano et al., 2021; Katić et al., 2021) and access the fetal brain (Burke et al., 2018) in animal models. They are detected in human cerebrospinal fluid (Laubscher et al., 2022; Li et al., 2022), pass through the human placenta (Zhang et al., 2022), and are found in the breast milk of lactating women (Zhang et al., 2023). Fetal exposure to nicotine via maternal smoking has long been established (Luck et al., 1985).
The results of these DNT studies contribute to the growing evidence that neonicotinoids exert adverse, nicotine-like effects on the developing mammalian brain (Cal, 2006; Kimura-Kuroda et al., 2012). The reported dimensions of certain brain regions were nearly all smaller in adult offspring exposed perinatally to neonicotinoids, while overall brain weight declined in response to thiamethoxam. Reduced volume of the developing brain is a sensitive indicator of neuronal cell loss from exposure to developmental neurotoxicants (Kaufmann and Gröters, 2006). These findings are consistent with studies showing reduced neurogenesis and increased neuronal cell death in the hippocampus of neonatal mice exposed to either imidacloprid or acetamiprid (Nakayama et al., 2019), and decreased neurogenesis in mouse embryos following prenatal exposure to acetamiprid (Kagawa and Nagao, 2018).
Imaging studies have shown that fetal brain exposure to nicotine via maternal smoking during pregnancy also reduces human brain volume and the dimensions of certain brain regions (Anblagan et al., 2013; England et al., 2017), likewise via neuronal cell damage and death (Slotkin, 2008). And while maternal smoking involves perinatal exposure to many bioactive compounds in tobacco smoke that suppress overall fetal growth, animal models involving exposure to nicotine alone demonstrate nicotine-specific, cholinergic effects on fetal brain development at very early stages of development, even when subsequent birth weight is normal (England et al., 2017). Importantly, reduced brain dimensions in the rat DNT studies persisted in adult offspring (PND 63-87). Perinatal nicotine exposure likewise can cause changes in the trajectory of brain development that persist into maturity (Slotkin, 2008).
These similarities in the effects of neonicotinoids and nicotine on mammalian brain size beg the question of whether they may also trigger similar neurobehavioral outcomes.
As discussed above, the reduced brain dimensions in the DNT rat studies were accompanied by functional nervous system deficits: decreased auditory startle reflex, decreased motor activity, and impaired learning, suggesting a possible link between brain effects and neurobehavioral outcomes. Interestingly, auditory processing defects are also effects of in utero nicotine exposure (Dwyer et al., 2008).
The brain structures most consistently reduced across rodent DNT studies were the corpus callosum and the caudate-putamen. The corpus callosum is a bundle of nerve fibers that connects the right and left hemispheres and processes sensory, motor and high-level cognitive signals (Goldstein et al., 2024). The caudate-putamen is part of the dorsal striatum, which is primarily involved in control over conscious motor movements and executive functions (Young et al., 2024). The neonicotinoid-induced reduction of these structures in rodent studies suggests a possible link to attention-deficit hyperactivity disorder (ADHD) in humans, for several reasons. First, imaging studies seeking neuroanatomical correlates of ADHD have found that people with clinically diagnosed ADHD tend to have smaller corpus callosa (Hynd et al., 1991; Giedd et al., 1994; Baumgardner et al., 1996; Semrud-Clikeman and Bledsoe, 2011; Yu et al., 2023), and in some studies reduced volume of the caudate-putamen as well (Valera et al., 2007; Emond et al., 2009). While these studies did not investigate potential causal factors, others have found a decrease in corpus callosum thickness in children born to mothers who smoked during pregnancy—suggesting a potential link with nicotine—in some cases accompanied by lack of coordination during information and auditory process (Bublitz and Stroud, 2012). Two additional studies find the corpus callosum reduction only in female (Paus et al., 2008) or male (Björnholm et al., 2020) children of maternal smokers. Finally, others have identified smoking during pregnancy as a risk factor for ADHD in their children, irrespective of possible anatomical anomalies of the brain (Milberger, et al., 1996; Milberger et al., 1998; Dong et al., 2018; Huang et al., 2018). That prenatal exposure to tobacco smoke (in humans) and neonicotinoids (in rats) both induce shrinkage of structures whose smaller size appears to be characteristic of ADHD, and that people having a mother who smoked during pregnancy is independently associated with ADHD, at least suggests the possibility that prenatal exposure to neonicotinoids in humans may increase risk of this disorder as well. While this hypothesis is largely correlational, it finds support in the common effects exerted by neonicotinoids and nicotine on mammalian brain development discussed above.
Of course, one must also consider exposure, and the fact that neonicotinoids show considerably less affinity for mammalian nAChRs than nicotine (Tomizawa and Casida, 2003). However, two neonicotinoids break down to form nicotinoid metabolites (desnitro-imidacloprid and descyano-thiacloprid) that have equal or greater potency as agonists of nAChRs in mammals relative to nicotine (Tomizawa and Casida, 2003). Imidacloprid is degraded to its desnitro form in the environment, in treated plants, and in the mammalian liver (Cal, 2006; Loser et al., 2021). Desnitro-imidacloprid is found in human urine (Wang et al., 2020) and in drinking water (Klarich et al., 2017). A preliminary risk assessment of dietary exposure to desnitro-imidacloprid in food concluded that internal levels could be high enough to activate nAChRs, and would even be more likely to desensitize these same receptors–with desensitization occurring at around 17 nM, roughly 10-fold lower than activating levels (Loser et al., 2021). This resembles the capacity of nicotine to desensitize rat nAChRs at the low, non-activating concentration of 10 nM (Paradiso and Steinbach, 2003). Neonicotinoid desensitization of nAChRs could be as problematic as activation, disrupting normal neuronal function and neurodevelopment (Loser et al., 2021) with potential effects on the operation of neural networks involved in memory and learning processes (Ochoa et al., 1989).
Because these metabolites of imidacloprid and thiacloprid have nicotine-like potency, one might expect to see neurodevelopmental impacts of exposure to their parent chemicals at low exposure levels. While we have not exhaustively reviewed the literature, two relevant studies conducted at doses near or below acute regulatory thresholds for human exposure stand out. Babeľová et al. (2017) orally exposed female mice to 0.03 mg/kg/day thiacloprid on days 1–3 of pregnancy, and found the isolated day 4 blastocytes exhibited significantly decreased cell numbers versus controls, cell loss that could ramify into neuronal cell deficits in the brain of developing fetuses. Saito et al. (2023) orally administered imidacloprid at 0.01 mg/kg/day or nicotine (0.1 mg/kg/day) to maternal mice from embryonic day 11 to 4 weeks after birth, and found that both imidacloprid and nicotine impaired certain aspects of learning and memory in male pups subjected to a water maze test.
Industry and EPA scientists who support in vitro approaches to assess DNT (discussed below) have argued that brain morphometry is unreliable because it is prone to “technical artifact” (Jackson et al., 2024). Yet when properly performed, morphometric analysis of brains can supply valuable data for regulators and is associated with less variability than body weight (Crofton, et al., 2001), a commonly used endpoint. The full suite of DNT test methods have been extensively validated; can provide reliable, relevant and reproducible data; and represent the best available science for assessing DNT potential in humans (Makris et al., 2009). However, improvements are needed. An analysis of 69 pesticide DNT study results submitted to EPA found that among the neurobehavioral tests, cognitive function and the FOB were used the least to determine a LOAEL, suggesting that within the guideline test they are not sufficiently sensitive endpoints (Vorhees and Makris, 2015). Locomotor activity and auditory startle were used most frequently for setting a LOAEL. Vorhees and Williams (2024) recommended updates to the DNT with additional requirements for more sensitive tests of learning and memory, while also noting that additional guidance may be helpful to improve the rigor of testing and reporting of results.
Deficiencies in DNT study data that do arise are often attributable to poor performance. An EPA review of positive control studies (studies undertaken with positive control chemicals known to disrupt neurological development) from labs that perform DNT studies found very troubling deficiencies; for instance, only three of the 16 demonstrated proficiency in testing for all DNT endpoints (Crofton et al., 2004). For 4 of the 5 DNT studies reviewed here (excepting clothianidin), adequate positive control data had either not been received or fully evaluated by EPA at the time the DERs were written, potentially compromising the integrity and reliability of the test results. Indeed, for thiacloprid EPA noted that: “None of the [positive control] studies demonstrated the laboratory’s ability to detect major functional neurotoxic endpoints using the observational methods used in the current study.” (U.S. EPA, 2003a).
Poor scientific practices can also be perpetuated by deficient regulatory oversight. For instance, EPA flagged the failure to submit brain morphometry for mid- and/or low-dose animals as a study deficiency, but then went ahead and set a LOAEL at the high-dose and NOAEL at the mid-dose for acetamiprid, clothianidin, and imidacloprid with the presumption–in the absence of complete data–that there would be no adverse effects on the brain at the mid- and low-doses. We believe that such determinations should be based on data, not speculation. Other unremedied deficiencies identified by EPA included inadequate assessment of motor activity, learning and memory (acetamiprid), no reporting of criteria for scoring errors in the water maze tests (clothianidin and thiacloprid) and failure to report how functional observation assessments are conducted (clothianidin and thiacloprid). EPA thus accepts studies that it deems deficient and that may well miss important adverse neurological effects, and registrants face no consequences for failing to supply missing or inadequate data.
It is our opinion that the quality of rodent DNT and other regulatory toxicology studies would improve considerably if EPA were to reject seriously deficient studies, enforce requests for additional data, and cancel or refrain from approving or re-approving pesticides when reliable data are lacking.
There is considerable momentum at EPA’s Office of Pesticide Programs to replace DNT rodent studies with new approach methodologies (NAMs) involving in vitro cell-based assays and in silico computational models (Crofton et al., 2014). The rationales most often cited are the time and expense of animal testing, and the laudable goal of reducing animal suffering (Crofton et al., 2014; Zaveri et al., 2019). However thus far, there is no adequate alternative to in vivo DNT studies (Vandenberg, and Zoeller, 2019). The OECD recently reviewed the DNT in vitro battery of tests (called the DNT IVB), warning that, “Several gaps in coverage of neurodevelopment processes and cell types have been acknowledged, including assays for neuroectodermal formation, peripheral nervous system specific processes, astrocyte differentiation and maturation, the blood-brain and placental barriers, microglia regulation of neuronal growth and connectivity, neuronal subtype specification, and axon myelination…. Also, the current DNT IVB does not fully account for sex or human genetic diversity that may influence susceptibility to chemical-induced developmental neurotoxicity (i.e., gene × environment interaction). These factors may result in lower sensitivity and specificity.” (OECD, 2023).
The European Partnership for the Assessment of Risks from Chemicals (PARC), which includes authors from 22 government agencies and academic institutions, published an article in Frontiers in Toxicology in April 2024 concluding that the current DNT NAMs have too many gaps to be used in risk assessment at this time (Tal et al., 2024). PARC particularly identified functional gaps, including tests of cognitive and neurobehavioral outcomes, cell processes within whole organisms, and learning and memory. The PARC report notes that these gaps will remain even with the future-planned DNT NAMs tests, unless additional whole animal tests are included using zebrafish.
Instead of investing in updating the rodent DNT tests to improve the quality, rigor, and sensitivity to detect complex neurodevelopmental effects such as learning, memory and behavior, EPA has placed its confidence in the DNT NAMs tests. EPA is so confident in NAMs that it is relying on a lack of bioactivity in NAMs tests as evidence of lack of DNT, leading to less-protective risk estimates for several organophosphate pesticides (U.S. EPA, 2023; U.S. EPA, 2024c). This misuse of NAMs is strongly opposed by health scientists and regulators alike (Children’s Health Protection Advisory Committee, 2021; Birnbaum, et al., 2024; Khan, 2024; Lam et al., 2024; Newell-Price, 2024).
EPA should make DNT studies a core requirement for registration of every pesticide, as its own scientists recommended in 1999 (U.S. EPA, 1999). This would reverse a disturbing trend of DNT study waivers that EPA has granted and even celebrated in recent years (Craig et al., 2019; Lerner, 2021).
Given the clear evidence of neonicotinoids’ mammalian neurotoxicity, EPA should reduce the acute and chronic reference doses (exposure limits) for each of them by a factor of at least 10 to account for the special sensitivity of the developing nervous system, as mandated by the FQPA.
Because neonicotinoids and their metabolites share a common mechanism of toxicity with nicotine, EPA should conduct a cumulative assessment of these insecticides, as mandated by another provision of the FQPA. This could be accomplished by assigning each neonicotinoid and major metabolite a relative potency factor that accounts for the greater toxicity of certain metabolites.
The FQPA authorizes EPA to eliminate a 10X child protective factor only if it has reliable information to find reasonable certainty of no harm to children without that protection. Given the gaps in coverage and the lack of validation with DNT NAMs, the risks to human and environmental health, and scientific uncertainties are far too great for EPA to rely on negative results (no bioactivity results) from NAMs tests. Instead, EPA could follow a recommendation of its Children’s Health Protection Advisory Committee, and employ NAMs results only to indicate or upgrade concern for a hazard, but not to conclude absence of hazard or to reduce the margin of protection afforded by the FQPA 10X child protective factor (CHPAC, 2021).
The rodent studies reviewed here provide valuable insights into the developmental neurotoxicity of five neonicotinoids, revealing similarities to the effects of nicotine, which is known to disrupt mammalian neurological development. Early-life exposure to each neonicotinoid reduced the dimensions of various brain regions, signifying neuronal cell death and reduced neurogenesis. Shrinkage of the brain regions most consistently affected across studies–the corpus callosum and caudate-putamen–suggests a possible role in the genesis of attention-deficit hyperactivity disorder (ADHD). The studies also demonstrated reduced auditory startle response and suggested adverse effects on learning and memory.
Further research is needed into the developmental neurotoxicity of neonicotinoids, and in particular metabolites equipotent to nicotine, especially given the ubiquitous use of and exposure to these compounds and the potential for life-long impairment. The conduct and oversight of regulatory DNT studies on neonicotinoids and other pesticides must be improved so they can provide higher-quality data. Well-conducted rodent studies of sufficient statistical power and strict adherence to required animal welfare protections remain critical for assessing xenobiotic disruption of complex neurodevelopmental processes. While new approach methodologies (NAMs) may contribute valuable insights into the cellular and molecular mechanisms of such adverse effects, they are not currently capable of replacing in vivo assessments.
Neonicotinoid insecticides are widely used, environmentally persistent, and are detected in drinking water, foods, human urine, breast milk, amniotic and cerebrospinal fluids, and the brains of treated rodents. Here we provide the first comprehensive assessment of unpublished rodent developmental neurotoxicity (DNT) studies on five neonicotinoids sponsored by neonicotinoid manufacturers. Statistically significant shrinkage of brain tissue was observed in high-dose offspring for five neonicotinoids: acetamiprid, clothianidin, imidacloprid, thiacloprid, and thiamethoxam. A decreased auditory startle reflex was reported for acetamiprid at all doses and was statistically significant in the mid- and high-dose offspring, and for clothianidin in juvenile high-dose females.
The original contributions presented in the study are included in the article/supplementary material, further inquiries can be directed to the corresponding author.
JS: Conceptualization, Formal Analysis, Investigation, Methodology, Validation, Visualization, Writing–original draft, Writing–review and editing. ND: Conceptualization, Formal Analysis, Investigation, Methodology, Validation, Writing–original draft, Writing–review and editing. WF: Conceptualization, Formal Analysis, Investigation, Methodology, Validation, Visualization, Writing–original draft, Writing–review and editing.
The author(s) declare that no financial support was received for the research, authorship, and/or publication of this article.
The authors declare that the research was conducted in the absence of any commercial or financial relationships that could be construed as a potential conflict of interest.
The handling editor LV declared a past co-authorship with the author JS.
All claims expressed in this article are solely those of the authors and do not necessarily represent those of their affiliated organizations, or those of the publisher, the editors and the reviewers. Any product that may be evaluated in this article, or claim that may be made by its manufacturer, is not guaranteed or endorsed by the publisher.
Aggarwal, V. (2021). Legal agricultural use determination for imidacloprid detections in California. California environmental protection agency department of pesticide regulation. Available at: https://www.cdpr.ca.gov/docs/emon/grndwtr/imidacloprid/imidacloprid_lau.pdf (Accessed May 7, 2024).
Anblagan, D., Jones, N. W., Costigan, C., Parker, A. J. J., Allcock, K., Aleong, R., et al. (2013). Maternal smoking during pregnancy and fetal organ growth: a magnetic resonance imaging study. PLoS ONE 8, e67223. doi:10.1371/journal.pone.0067223
Babeľová, J., Šefčíková, Z., Čikoš, Š., Špirková, A., Kovaříková, V., Koppel, J., et al. (2017). Exposure to neonicotinoid insecticides induces embryotoxicity in mice and rabbits. Toxicology 392, 71–80. doi:10.1016/j.tox.2017.10.011
Baumgardner, T. L., Singer, H. S., Denckla, M. B., Rubin, M. A., Abrams, M. T., Colli, M. J., et al. (1996). Corpus callosum morphology in children with Tourette syndrome and attention deficit hyperactivity disorder. Neurology 47, 477–482. doi:10.1212/WNL.47.2.477
Birnbaum, L. S., Eskenazi, B., Hertz-Picciotto, I., Hyland, C., Joglekar, R., Ritz, B., et al. (2024). Comment submitted to the US EPA by Project TENDR, The Arc of the US et al. - On EPA’s Proposed Interim Registration Decisions for Several Organophosphate Pesticides: Acephate; Malathion; Dimethoate. Available at: https://www.regulations.gov/comment/EPA-HQ-OPP-2008-0915-0227 (Accessed August 23, 2024).
Björnholm, L., Nikkinen, J., Kiviniemi, V., Niemelä, S., Drakesmith, M., Evans, J. C., et al. (2020). Prenatal exposure to maternal cigarette smoking and structural properties of the human corpus callosum. NeuroImage 209, 116477. doi:10.1016/j.neuroimage.2019.116477
Brammer, A. (2003). Thiamethoxam: developmental neurotoxicity study in rats. Study number rr0936. MRID 46028202. Cent. Toxicol. Lab. Alderley Park, Macclesfield, Ches. UK. Unpubl.
Bublitz, M. H., and Stroud, L. R. (2012). Maternal smoking during pregnancy and offspring brain structure and function: review and agenda for future research. Nicotine and Tob. Res. 14, 388–397. doi:10.1093/ntr/ntr191
Burke, A. P., Niibori, Y., Terayama, H., Ito, M., Pidgeon, C., Arsenault, J., et al. (2018). Mammalian susceptibility to a neonicotinoid insecticide after fetal and early postnatal exposure. Sci. Rep. 8, 16639. doi:10.1038/s41598-018-35129-5
Cal, E. P. A. (2006). Imidacloprid. Risk characterization document: dietary and drinking water exposure. California: California Environmental Protection Agency (Cal EPA), Department of Pesticide Regulation. Available at: https://www.cdpr.ca.gov/docs/risk/rcd/imidacloprid.pdf (Accessed August 25, 2024).
Castro, E. M., Lotfipour, S., and Leslie, F. M. (2023). Nicotine on the developing brain. Pharmacol. Res. 190, 106716. doi:10.1016/j.phrs.2023.106716
Chen, D., Liu, Z., Barrett, H., Han, J., Lv, B., Li, Y., et al. (2020). Nationwide biomonitoring of neonicotinoid insecticides in breast milk and health risk assessment to nursing infants in the Chinese population. J. Agric. Food Chem. 68, 13906–13915. doi:10.1021/acs.jafc.0c05769
Children’s Health Protection Advisory Committee (CHPAC) (2021). Report to the U.S. EPA - protecting children’s health under amended TSCA: chemical prioritization. Available at: https://www.regulations.gov/document/EPA-HQ-OA-2022-0574-0011 (Accessed August 23, 2024).
Craddock, H. A., Huang, D., Turner, P. C., Quirós-Alcalá, L., and Payne-Sturges, D. C. (2019). Trends in neonicotinoid pesticide residues in food and water in the United States, 1999–2015. Environ. Health 18, 7. doi:10.1186/s12940-018-0441-7
Craig, E., Lowe, K., Akerman, G., Dawson, J., May, B., Reaves, E., et al. (2019). Reducing the need for animal testing while increasing efficiency in a pesticide regulatory setting: lessons from the EPA Office of pesticide Programs’ hazard and science policy council. Regul. Toxicol. Pharmacol. 108, 104481. doi:10.1016/j.yrtph.2019.104481
Crofton, K., Fritsche, E., Ylikomi, T., and Bal-Price, A. (2014). International STakeholder NETwork (ISTNET) for creating a developmental neurotoxicity testing (DNT) roadmap for regulatory purposes. ALTEX 31, 223–224. doi:10.14573/altex.1402121
Crofton, K., Makris, S. L., Sette, W. F., Mendez, E., and Raffaele, K. C. (2004). A qualitative retrospective analysis of positive control data in developmental neurotoxicity studies. Neurotoxicology Teratol. 26, 345–352. doi:10.1016/j.ntt.2004.02.007
Crofton, K. M., Makris, S. L., Sette, W. F., Mendez, E., and Raffaele, K. C. (2001). Developmental neurotoxicity testing guidelines: variability in morphometric assessments of neuropathology. Abstract #539. Toxicol. a Suppl. Toxicol. Sci. 113. Available at: https://www.toxicology.org/pubs/docs/Tox/2001Tox.pdf.
Dong, T., Hu, W., Zhou, X., Lin, H., Lan, L., Hang, B., et al. (2018). Prenatal exposure to maternal smoking during pregnancy and attention-deficit/hyperactivity disorder in offspring: a meta-analysis. Reprod. Toxicol. 76, 63–70. doi:10.1016/j.reprotox.2017.12.010
Douglas, M., Krupke, C., and Tooker, J. (2024). Comment submitted to the US EPA - requirements applicable to treated seed (EPA–HQ–OPP–2023–0420). Available at: https://www.regulations.gov/comment/EPA-HQ-OPP-2023-0420-0246 (Accessed May 7, 2024).
Douglas, M. R., and Tooker, J. F. (2015). Large-scale deployment of seed treatments has driven rapid increase in use of neonicotinoid insecticides and preemptive pest management in U.S. Field crops. Environ. Sci. Technol. 49, 5088–5097. doi:10.1021/es506141g
Dwyer, J. B., Broide, R. S., and Leslie, F. M. (2008). Nicotine and brain development. Birth Defects Res. Pt C 84, 30–44. doi:10.1002/bdrc.20118
EFSA (2015). Scientific panel on plant protection products and their residues: minutes of the meeting of the working group on developmental neurotoxicity (DNT) of acetamiprid and imidacloprid. Eur. Food Saf. Auth. (EFSA). Available at: https://www.efsa.europa.eu/sites/default/files/wgs/pesticides/wgDNTacetamipridimidacloprid.pdf (Accessed May 7, 2024).
EFSA Hernandez Jerez, A., Coja, T., Paparella, M., Price, A., Henri, J., et al. (2024). Statement on the toxicological properties and maximum residue levels of acetamiprid and its metabolites. EFS2 22, e8759. doi:10.2903/j.efsa.2024.8759
Emond, V., Joyal, C., and Poissant, H. (2009). Structural and functional neuroanatomy of attention-deficit hyperactivity disorder (ADHD). L’Encéphale 35, 107–114. doi:10.1016/j.encep.2008.01.005
England, L. J., Aagaard, K., Bloch, M., Conway, K., Cosgrove, K., Grana, R., et al. (2017). Developmental toxicity of nicotine: a transdisciplinary synthesis and implications for emerging tobacco products. Neurosci. and Biobehav. Rev. 72, 176–189. doi:10.1016/j.neubiorev.2016.11.013
FQPA (1996). Public law 104 - 170 - food quality protection act of 1996. Available at: https://www.govinfo.gov/app/details/PLAW-104publ170/summary.
Giedd, J. N., Castellanos, F. X., Casey, B. J., Kozuch, P., King, A. C., Hamburger, S. D., et al. (1994). Quantitative morphology of the corpus callosum in attention deficit hyperactivity disorder. AJP 151, 665–669. doi:10.1176/ajp.151.5.665
Goldstein, A., Covington, B. P., Mahabadi, N., and Mesfin, F. B. (2024). “Neuroanatomy, corpus callosum,” in StatPearls, treasure island (FL) (United States: StatPearls Publishing). Available at: http://www.ncbi.nlm.nih.gov/books/NBK448209/(Accessed May 7, 2024).
Goulson, D. (2013). REVIEW: an overview of the environmental risks posed by neonicotinoid insecticides. J. Appl. Ecol. 50, 977–987. doi:10.1111/1365-2664.12111
Hirano, T., Miyata, Y., Kubo, S., Ohno, S., Onaru, K., Maeda, M., et al. (2021). Aging-related changes in the sensitivity of behavioral effects of the neonicotinoid pesticide clothianidin in male mice. Toxicol. Lett. 342, 95–103. doi:10.1016/j.toxlet.2021.02.010
Hoberman, A. M. (2000). Developmental neurotoxicity study of TI 435 (clothianidin) administered orally via the diet to CRL:CD@ presumed pregnant rats. Horsham, PA: Argus Research Laboratories, Inc.
Hoberman, A. M. (2001). Oral (diet) developmental neurotoxicity study of YRC 2894 (thiacloprid) in CRL:CD®(SD)IGS BR VAF/PLUS®. Horsham, PA: Argus Research Laboratories, Inc.
Huang, L., Wang, Y., Zhang, L., Zheng, Z., Zhu, T., Qu, Y., et al. (2018). Maternal smoking and attention-deficit/hyperactivity disorder in offspring: a meta-analysis. Pediatrics 141, e20172465. doi:10.1542/peds.2017-2465
Hynd, G. W., Semrud-Clikeman, M., Lorys, A. R., Novey, E. S., Eliopulos, D., and Lyytinen, H. (1991). Corpus callosum morphology in attention deficit-hyperactivity disorder: morphometric analysis of MRI. J. Learn Disabil. 24, 141–146. doi:10.1177/002221949102400302
Jackson, B., Minnema, L., Wu, Y., Schlosser, C., Hofstra, A., Carstens, K., et al. (2024). Utility of developmental neurotoxicity in vitro battery to address regulatory challenges. 1141903 bytes. Sci. Inventory. doi:10.23645/EPACOMPTOX.25541578
Kagawa, N., and Nagao, T. (2018). Neurodevelopmental toxicity in the mouse neocortex following prenatal exposure to acetamiprid. J Appl. Toxicol. 38, 1521–1528. doi:10.1002/jat.3692
Katić, A., Kašuba, V., Kopjar, N., Lovaković, B. T., Marjanović Čermak, A. M., Mendaš, G., et al. (2021). Effects of low-level imidacloprid oral exposure on cholinesterase activity, oxidative stress responses, and primary DNA damage in the blood and brain of male Wistar rats. Chemico-Biological Interact. 338, 109287. doi:10.1016/j.cbi.2020.109287
Kaufmann, W., and Gröters, S. (2006). Developmental neuropathology in DNT-studies—a sensitive tool for the detection and characterization of developmental neurotoxicants. Reprod. Toxicol. 22, 196–213. doi:10.1016/j.reprotox.2006.04.021
Khan, F. (2024). Comment submitted to the US EPA by public health – Seattle and king county (PHSKC) - on EPA’s proposed interim registration decision for acephate. Available at: https://www.regulations.gov/comment/EPA-HQ-OPP-2008-0915-0305 (Accessed August 23, 2024).
Kimura-Kuroda, J., Komuta, Y., Kuroda, Y., Hayashi, M., and Kawano, H. (2012). Nicotine-like effects of the neonicotinoid insecticides acetamiprid and imidacloprid on cerebellar neurons from neonatal rats. PLoS ONE 7, e32432. doi:10.1371/journal.pone.0032432
Klarich, K. L., Pflug, N. C., DeWald, E. M., Hladik, M. L., Kolpin, D. W., Cwiertny, D. M., et al. (2017). Occurrence of neonicotinoid insecticides in finished drinking water and fate during drinking water treatment Environ. Sci. Technol. Lett. 4 (5), 168–173. doi:10.1021/acs.estlett.7b00081
Klarich Wong, K. L., Webb, D. T., Nagorzanski, M. R., Kolpin, D. W., Hladik, M. L., Cwiertny, D. M., et al. (2019). Chlorinated byproducts of neonicotinoids and their metabolites: an unrecognized human exposure potential? Environ. Sci. Technol. Lett. 6, 98–105. doi:10.1021/acs.estlett.8b00706
Lam, J., Chhun, D., Arellano, A., and Magdale, J. (2024). Comment submitted to the US EPA by academic researchers from the department of public health at the California state university, east bay - on EPA’s proposed interim registration decision for acephate. Available at: https://www.regulations.gov/comment/EPA-HQ-OPP-2008-0915-0275 (Accessed August 23, 2024).
Laubscher, B., Diezi, M., Renella, R., Mitchell, E. A. D., Aebi, A., Mulot, M., et al. (2022). Multiple neonicotinoids in children’s cerebro-spinal fluid, plasma, and urine. Environ. Health 21, 10. doi:10.1186/s12940-021-00821-z
Lerner, S. (2021). The department of yes: how pesticide companies corrupted the EPA and poisoned America. Intercept. Available at: https://theintercept.com/2021/06/30/epa-pesticides-exposure-opp/(Accessed October 23, 2021).
Li, A. J., Si, M., Yin, R., Qiu, R., Li, H., Yao, F., et al. (2022). Detection of neonicotinoid insecticides and their metabolites in human cerebrospinal fluid. Environ. Health Perspect. 130, 127702. doi:10.1289/EHP11374
Loser, D., Grillberger, K., Hinojosa, M. G., Blum, J., Haufe, Y., Danker, T., et al. (2021). Acute effects of the imidacloprid metabolite desnitro-imidacloprid on human nACh receptors relevant for neuronal signaling. Arch. Toxicol. 95, 3695–3716. doi:10.1007/s00204-021-03168-z
Luck, W., Nau, H., Hansen, R., and Steldinger, R. (1985). Extent of nicotine and cotinine transfer to the human fetus, placenta and amniotic fluid of smoking mothers. Dev. Pharmacol. Ther. 8, 384–395. doi:10.1159/000457063
Makris, S. L., Raffaele, K., Allen, S., Bowers, W. J., Hass, U., Alleva, E., et al. (2009). A retrospective performance assessment of the developmental neurotoxicity study in support of OECD test guideline 426. Environ. Health Perspect. 117, 17–25. doi:10.1289/ehp.11447
Milberger, S., Biederman, J., Faraone, S. V., Chen, L., and Jones, J. (1996). Is maternal smoking during pregnancy a risk factor for attention deficit hyperactivity disorder in children? AJP 153, 1138–1142. doi:10.1176/ajp.153.9.1138
Milberger, S., Biederman, J., Faraone, S. V., and Jones, J. (1998). Further evidence of an association between maternal smoking during pregnancy and attention deficit hyperactivity disorder: findings from a high-risk sample of siblings. J. Clin. Child Psychol. 27, 352–358. doi:10.1207/s15374424jccp2703_11
Millemann, D. R., Genievich, H., Reilly, E., Rush, A., Goodrow, S., and Procopio, N. A. (2020). A review of neonicotinoid insecticides and occurrence in New Jersey surface water and groundwater. New Jersey Divison of Science and Research. Bureau Environ. Assess. Available at: https://dspace.njstatelib.org/server/api/core/bitstreams/ee97ec9b-7927-44bd-9612-f1bc8df62950/content (Accessed May 7, 2024).
Naidenko, O. V. (2020). Application of the Food Quality Protection Act children's health safety factor in the U.S. EPA pesticide risk assessments. Environ. Health 19 (1), 16. doi:10.1186/s12940-020-0571-6
Nakayama, A., Yoshida, M., Kagawa, N., and Nagao, T. (2019). The neonicotinoids acetamiprid and imidacloprid impair neurogenesis and alter the microglial profile in the hippocampal dentate gyrus of mouse neonates. J Appl. Toxicol. 39, 877–887. doi:10.1002/jat.3776
Nemec, M. (2003). An oral developmental neurotoxicity study in rats. Ashland, Ohio: WIL Research Laboratories, Inc. Laboratory Project ID WIL-21193. MRID 46255619. Unpublished.
Newell-Price, J. (2024). Comment submitted to the US EPA by the endocrine society - on EPA’s proposed interim registration decision for acephate. Available at: https://www.regulations.gov/comment/EPA-HQ-OPP-2008-0915-0188 (Accessed August 23, 2024).
Ochoa, E. L. M., Chattopadhyay, A., and McNamee, M. G. (1989). Desensitization of the nicotinic acetylcholine receptor: molecular mechanisms and effect of modulators. Cell. Mol. Neurobiol. 9, 141–178. doi:10.1007/BF00713026
OECD (2007). Test No. 426: developmental neurotoxicity study. Available at: https://www.oecd-ilibrary.org/content/publication/9789264067394-en.
OECD (2023). Initial recommendations on evaluation of data from the developmental neurotoxicity (DNT) in-vitro testing battery. Available at: https://one.oecd.org/document/ENV/CBC/MONO(2023)13/en/pdf (Accessed May 7, 2024).
Paradiso, K. G., and Steinbach, J. H. (2003). Nicotine is highly effective at producing desensitization of rat alpha4beta2 neuronal nicotinic receptors. J. Physiology 553, 857–871. doi:10.1113/jphysiol.2003.053447
Paus, T., Nawazkhan, I., Leonard, G., Perron, M., Pike, G. B., Pitiot, A., et al. (2008). Corpus callosum in adolescent offspring exposed prenatally to maternal cigarette smoking. NeuroImage 40, 435–441. doi:10.1016/j.neuroimage.2007.10.066
Rice, D., and Barone, S. (2000). Critical periods of vulnerability for the developing nervous system: evidence from humans and animal models. Environ. Health Perspect. 108, 511–533. doi:10.1289/ehp.00108s3511
Saito, H., Furukawa, Y., Sasaki, T., Kitajima, S., Kanno, J., and Tanemura, K. (2023). Behavioral effects of adult male mice induced by low-level acetamiprid, imidacloprid, and nicotine exposure in early-life. Front. Neurosci. 17, 1239808. doi:10.3389/fnins.2023.1239808
Semrud-Clikeman, M., and Bledsoe, J. (2011). Updates on attention-deficit/hyperactivity disorder and learning disorders. Curr. Psychiatry Rep. 13, 364–373. doi:10.1007/s11920-011-0211-5
Sheets, L. P. (2001). A developmental neurotoxicity screening study with technical grade imidacloprid in wistar rats. Bayer corporation, agriculture division, toxicology, 17745 south metcalf ave. Stilwell, Kans. Laboratory report number 110245. MRID 45537501. Unpublished.
Slotkin, T. A. (2008). If nicotine is a developmental neurotoxicant in animal studies, dare we recommend nicotine replacement therapy in pregnant women and adolescents? Neurotoxicology Teratol. 30, 1–19. doi:10.1016/j.ntt.2007.09.002
Soltaninejad, K., and Shadnia, S. (2014). “History of the use and epidemiology of organophosphorus poisoning,” in Basic and clinical toxicology of organophosphorus compounds. Editors M. Balali-Mood, and M. Abdollahi (London: Springer London), 25–43. doi:10.1007/978-1-4471-5625-3_2
Steeger, T. (2014). Bee health in the USA and the debate about neonicotinoids. Plant Health Aust. Available at: https://www.planthealthaustralia.com.au/wp-content/uploads/2014/05/Thomas-Steeger.pdf (Accessed May 7, 2024).
Tal, T., Myhre, O., Fritsche, E., Rüegg, J., Craenen, K., Aiello-Holden, K., et al. (2024). New approach methods to assess developmental and adult neurotoxicity for regulatory use: a PARC work package 5 project. Front. Toxicol. 6, 1359507. doi:10.3389/ftox.2024.1359507
Tomizawa, M., and Casida, J. E. (2003). Selective toxicity of neonicotinoids attributable to specificity of insect and mammalian nicotinic receptors. Annu. Rev. Entomol. 48, 339–364. doi:10.1146/annurev.ento.48.091801.112731
USDA (2022). Pesticide data program database search. Available at: https://apps.ams.usda.gov/pdp (Accessed May 7, 2024).
U.S. EPA (1998). Health effects test guidelines OPPTS 870.6300: developmental neurotoxicity study. Available at: https://www.regulations.gov/document/EPA-HQ-OPPT-2009-0156-0042 (Accessed May 7, 2024).
U.S. EPA (1999). Draft toxicology data requirements for assessing risks of pesticide exposure to children’s health: report of the Toxicology Working Group of the 10X Task Force. Available at: https://archive.epa.gov/scipoly/sap/meetings/web/pdf/10xtx428.pdf (Accessed April 25, 2024).
U.S. EPA (2002a). Determination of the appropriate FQPA safety factor(s) in assessing pesticide tolerances. Washington, DC: Office of Pesticide Programs, U.S. Environmental Protection Agency. Available at: https://www.epa.gov/pesticide-science-and-assessing-pesticide-risks/determination-appropriate-fqpa-safety-factors (Accessed September 17, 2024).
U.S. EPA (2002b). EPA data evaluation record, imidacloprid developmental neurotoxicity study- rat. Oppts 870.6300. Mrid 45537501. Prepared by oak ridge national laboratory. Sheets, lp, A developmental neurotoxicity screening study with technical grade imidacloprid in wistar rats, bayer corporation, mrid 45537501 (sept. 14, 2001). Available at: https://biologicaldiversity.org/programs/environmental_health/pdfs/Imidacloprid-2002-DNT-DER.pdf (Accessed May 10, 2024).
U.S. EPA (2002c). A review of the reference dose and reference concentration processes. Available at: https://www.epa.gov/sites/default/files/2014-12/documents/rfd-final.pdf (Accessed May 11, 2024).
U.S. EPA (2003a). Data evaluation record for oral (diet) developmental neurotoxicity study of YRC 2894 (thiacloprid) in CRL:CD®(SD)IGS BR VAF/PLUS. Available at: https://biologicaldiversity.org/programs/environmental_health/pdfs/Thiacloprid-2003-DNT-DER.pdf (Accessed May 10, 2024).
U.S. EPA (2003b). Thiacloprid in/on pome fruits and cotton. Health Eff. Div. (HED) Risk Assess. Available at: https://www3.epa.gov/pesticides/chem_search/cleared_reviews/csr_PC-014019_23-Jul-03_a.pdf (Accessed May 11, 2024).
U.S. EPA (2005). Data Evaluation Record for Developmental neurotoxicity study of TI 435 (clothianidin) administered orally via the diet to CRL:CD presumed pregnant rats. TXR# 0050321. MRID 45422804. Available at: https://biologicaldiversity.org/programs/environmental_health/pdfs/Clothianidin-2002-DNT-DER.pdf (Accessed May 10, 2024).
U.S. EPA (2007). Data evaluation record for thiamethoxam: developmental neurotoxicity study in rats. TXR# 0054533. (MRID nos. 46028201, original DER 46028202, updated with requested brain data 47034201). Available at: https://biologicaldiversity.org/programs/environmental_health/pdfs/Thiamethoxam-2007-DNT-DER.pdf (Accessed May 10, 2024).
U.S. EPA (2008). Data evaluation record for acetamiprid: an oral developmental neurotoxicity study in rats. TXR# 0052563. (MRID 46255619, registrant rebuttal MRID 46779201 dated 3/2006 and MRID 47181101 dated 4/2007). Available at: https://biologicaldiversity.org/programs/environmental_health/pdfs/Acetamiprid-2004-DNT-DER.pdf (Accessed May 10, 2024).
U.S. EPA (2013). Data evaluation record for oral (diet) developmental neurotoxicity study of MTI-446 (dinotefuran) in crl:CD(SD) rats. MRID 48291601. Available at: https://www.biologicaldiversity.org/programs/environmental_health/pdfs/2013-dinotefuran-DNT-DER.pdf (Accessed May 17, 2024).
U.S. EPA (2017). Acetamiprid. Draft human health risk assessment for registration review. Document ID EPA-HQ-OPP-2012-0329-0025. Available at: https://www.regulations.gov/document/EPA-HQ-OPP-2012-0329-0025 (Accessed September 17, 2024).
U.S. EPA (2020). Data evaluation record (supplemental) for thiamethoxam: developmental neurotoxicity study in rats. TXR# 0058141. (MRID nos. 46028201, original DER 46028202, updated with requested brain data 47034201). Available at: https://biologicaldiversity.org/programs/environmental_health/pdfs/Thiamethoxam-2020-DNT-Supplemental-DER.pdf (Accessed May 10, 2024).
U.S. EPA (2023). Evaluation of the developmental neurotoxicity potential of acephate/methamidophos to inform the FQPA safety factor. Available at: https://www.regulations.gov/document/EPA-HQ-OPP-2008-0915-0057 (Accessed May 7, 2024).
U.S. EPA (2024a). About the exposure factors Handbook. Available at: https://www.epa.gov/expobox/about-exposure-factors-handbook (Accessed August 23, 2024).
U.S. EPA (2024b). Causal analysis/diagnosis decision information system (CADDIS): insecticides. Available at: https://www.epa.gov/caddis/insecticides (Accessed May 7, 2024).
U.S. EPA (2024c). Evaluation of the developmental neurotoxicity potential of malathion/malaoxon to inform the FQPA safety factor. Available at: https://www.regulations.gov/document/EPA-HQ-OPP-2009-0317-0156 (Accessed May 7, 2024).
Valera, E. M., Faraone, S. V., Murray, K. E., and Seidman, L. J. (2007). Meta-analysis of structural imaging findings in attention-deficit/hyperactivity disorder. Biol. Psychiatry 61, 1361–1369. doi:10.1016/j.biopsych.2006.06.011
Vandenberg, L. N., and Zoeller, R. T. (2019). Thinking through the EPA’s commitment to eliminate the use of mammals in toxicity testing. Environ. Health News. Available at: https://www.ehn.org/epa-lab-animals-chemical-testing-2640450647.html (Accessed May 7, 2024).
Vorhees, C. V., and Makris, S. L. (2015). Assessment of learning, memory, and attention in developmental neurotoxicity regulatory studies: synthesis, commentary, and recommendations. Neurotoxicology Teratol. 52, 109–115. doi:10.1016/j.ntt.2015.10.004
Vorhees, C. V., and Williams, M. T. (2024). Tests for learning and memory in rodent regulatory studies. Curr. Res. Toxicol. 6, 100151. doi:10.1016/j.crtox.2024.100151
Wang, A., Mahai, G., Wan, Y., Yang, Z., He, Z., Xu, S., et al. (2020). Assessment of imidacloprid related exposure using imidacloprid-olefin and desnitro-imidacloprid: neonicotinoid insecticides in human urine in Wuhan, China. Environ. Int. 141, 105785. doi:10.1016/j.envint.2020.105785
Wieben, C. M. (2021). Estimated annual agricultural pesticide use by major crop or crop group for States of the conterminous United States, 1992–2019. doi:10.5066/P900FZ6Y
Young, C. B., Reddy, V., and Sonne, J. (2024). “Neuroanatomy, basal ganglia,” in StatPearls, treasure island (FL) China, (StatPearls Publishing). Available at: http://www.ncbi.nlm.nih.gov/books/NBK537141/(Accessed May 7, 2024).
Yu, M., Gao, X., Niu, X., Zhang, M., Yang, Z., Han, S., et al. (2023). Meta-analysis of structural and functional alterations of brain in patients with attention-deficit/hyperactivity disorder. Front. Psychiatry 13, 1070142. doi:10.3389/fpsyt.2022.1070142
Zaveri, M., Padilla, M., and Pesier, J. (2019). E.P.A. Says it will drastically reduce animal testing. Available at: https://www.nytimes.com/2019/09/10/climate/epa-animal-testing.html (Accessed May 7, 2024).
Zhang, H., Bai, X., Zhang, T., Song, S., Zhu, H., Lu, S., et al. (2022). Neonicotinoid insecticides and their metabolites can pass through the human placenta unimpeded. Environ. Sci. Technol. 56, 17143–17152. doi:10.1021/acs.est.2c06091
Zhang, Q., Lu, Z., Chang, C.-H., Yu, C., Wang, X., and Lu, C. (2019). Dietary risk of neonicotinoid insecticides through fruit and vegetable consumption in school-age children. Environ. Int. 126, 672–681. doi:10.1016/j.envint.2019.02.051
Keywords: pesticide, neurotoxic, DNT, neonicotinoid, EPA-environmental protection agency, neurodevelopment, developmental, brain
Citation: Sass JB, Donley N and Freese W (2024) Neonicotinoid pesticides: evidence of developmental neurotoxicity from regulatory rodent studies. Front. Toxicol. 6:1438890. doi: 10.3389/ftox.2024.1438890
Received: 26 May 2024; Accepted: 05 September 2024;
Published: 02 October 2024.
Edited by:
Laura N. Vandenberg, University of Massachusetts Amherst, United StatesReviewed by:
Steeve Herve Thany, Université d’Orléans, FranceCopyright © 2024 Sass, Donley and Freese. This is an open-access article distributed under the terms of the Creative Commons Attribution License (CC BY). The use, distribution or reproduction in other forums is permitted, provided the original author(s) and the copyright owner(s) are credited and that the original publication in this journal is cited, in accordance with accepted academic practice. No use, distribution or reproduction is permitted which does not comply with these terms.
*Correspondence: Jennifer Beth Sass, anNhc3NAbnJkYy5vcmc=
Disclaimer: All claims expressed in this article are solely those of the authors and do not necessarily represent those of their affiliated organizations, or those of the publisher, the editors and the reviewers. Any product that may be evaluated in this article or claim that may be made by its manufacturer is not guaranteed or endorsed by the publisher.
Research integrity at Frontiers
Learn more about the work of our research integrity team to safeguard the quality of each article we publish.