- 1SenzaGen AB, Lund, Sweden
- 2RISE Research Institutes of Sweden AB, Borås, Sweden
Biocompatibility testing of medical devices is governed by the ISO 10993 series of standards and includes evaluation of skin sensitization potential of the final product. A majority of all medical devices are tested using in vivo methods, largely due to the lack of in vitro methods validated within the applicability domain of solid materials. The GARDskin method for assessment of chemical skin sensitizers is a validated method included in the OECD Test Guideline 442E, based on evaluation of transcriptional patterns of an endpoint-specific genomic biomarker signature in a dendritic cell-like cell, following test chemical exposure. The current study aimed to evaluate the applicability of GARDskin for the purpose of testing solid materials by incorporation of extraction procedures described in ISO 10993-12:2021, as well as to demonstrate the functionality of the proposed protocols, by testing of custom-made materials spiked with sensitizing agents. It was shown that GARDskin is compatible with both polar and non-polar extraction vehicles frequently used for the purpose of medical device biological testing. Further, exploring three different material types spiked with up to four different sensitizing agents, as well as three unspiked control materials and commercial reference products, it was shown that the method correctly classified all evaluated test materials. Taken together, the data presented suggest that GARDskin may constitute a valid alternative to in vivo experimentation for the purpose of skin sensitization assessment of medical devices.
Introduction
Medical device toxicology is currently being redefined, transitioning from a process that largely relies on the results of animal testing, to one which is increasingly focused on the use of in vitro and in chemico methods for evaluation of the biological safety of devices (Kerecman Myers et al., 2017). The biological evaluation of medical devices is governed by the ISO 10993 series of standards, where the framework is described in ISO 10993-1: 2018. (ISO 10993-1, 2018). The biological endpoints (i.e., adverse effects) related to skin exposure that have to be evaluated for all medical devices in direct or indirect contact with the body include skin irritation (ISO 10993-23, 2021) and skin sensitization (ISO 10993-10, 2021). An in vitro test method to assess skin irritation of medical devices has been validated and included in ISO 10993-23 in 2021 and is now the preferred choice for irritation testing and is increasingly accepted on many markets worldwide. (De Jong et al., 2018). However, an in vitro test method to assess skin sensitization potential of medical devices or materials has not been sufficiently qualified. Rather, such testing is conventionally performed in vivo, primarily using the Guinea Pig Maximization Test (GPMT) (Magnusson and Kligman, 1969) or the Buehler Occluded Patch Test (Buehler, 1965).
The immunobiological mechanisms leading to skin sensitization are today well understood and summarized as an Adverse Outcome Pathway (AOP) (OECD, 2014). To date, several mechanistically based new approach methodologies (NAM) for in vitro assessment of chemical skin sensitizers have been validated and regulatory accepted for use within certain applicability domains. These methods address the first three of the four key events (KE) of the AOP and are described in OECD Test Guidelines (TG) 442C, 442D and 442E, respectively (OECD, 2022b; OECD, 2023a; OECD, 2023b). There is now an interest in qualifying these methods for the assessment of skin sensitization of medical devices, where the framework for such qualification has been outlined in a recent guidance document (ISO/TS 11796, 2023).
Several next-generation NAM:s are emerging, with the potential of both broadening and sharpening the applicability of in vitro methods. One such method is the Genomic Allergen Rapid Detection (GARD) assay for assessment of chemical skin sensitizers (GARDskin) (OECD, 2023b), which was regulatory accepted and included in the OECD TG 442E in 2022. The GARDskin method evaluates the transcriptional patterns of an endpoint-specific genomic biomarker signature (GPS) (Johansson et al., 2011; OECD, 2022a) in a dendritic cell-like cell line exposed to test chemicals, which allows for machine-learning assisted classifications based on high-dimensional gene expression data, monitoring a wide range of immunobiological mechanisms associated with the AOP for skin sensitization.
Of importance, all available OECD in vitro methods for skin sensitization were developed and validated for assessment of neat chemicals. For these methods to be qualified for assessment of complex and dilute mixtures, metals, and solid materials, additional developments and validating exercises are required. In the case of the biological evaluation of medical devices, all testing of solid materials is to be performed on extracts containing a mixture of chemicals extracted from the material, by the use of both a polar and non-polar solvent (i.e., extraction vehicles), in order to capture clinically relevant leachables (ISO 10993-12, 2021). Thus, any in vitro test system utilized for such purposes must be both compatible with such solvents, at sufficient concentrations, and be sensitive enough in order to detect the presence of skin sensitizing agents in a complex and dilute formulation.
Here, the progress in adapting the conventional GARDskin method to comply with requirements imposed by the ISO 10993 series of standards is presented. The assay’s compatibility with polar and non-polar solvents has been evaluated. Presented data also demonstrate the functionality of ISO 10993-12:2021 protocols for extraction of materials frequently used in medical devices, spiked with weak, moderate and strong sensitizers and the ability of the cellular system to accurately detect the presence of extracted chemicals from the materials.
Materials and methods
Test materials and controls
The spiked test materials was produced by the Research Institutes of Sweden (RISE). Four different sensitizing agents were used as spikes during production: 2-Aminophenol, Propyl gallate, Cinnamic aldehyde and Phenyl benzoate. All chemicals were purchased from Sigma-Aldrich (St. Louis, MO, US). Additional details of each sensitizing agent are provided in Table 1. The rationale for selection of sensitizing agents was based on the following selection criteria. Firstly, the chemicals should represent a range of sensitizing potencies, including weak, moderate and strong sensitizers. Secondly, the chemicals should have available reference data from historical assessment using the conventional GARDskin method. Lastly, the chemicals should, if possible, have been used in similar work (Coleman et al., 2015; Pellevoisin et al., 2021) in order to facilitate direct comparisons of results. The sensitizing agents were introduced in silicone (MED-2000, NuSil Technology, Carpinteria, CA) and thermoplastic polyurethane (TPU, Coim Laripur® LPR 7560 EG, Telko, Sweden) at a final concentration of 10%, similar to the materials described and used in similar work (Coleman et al., 2015; 2018). In addition, commercially available materials, approved for the medical device market, were included in the study as negative reference materials. For this purpose, a silicone tube (EC60001), a TPU tube (Estane 58,277) and a polyvinyl chloride (PVC) tube (RB3 NDG), were all acquired from Medizintechnik Promedt GmbH (Tornesch, Germany). The vehicles used for extraction were Super Refined Olive Oil-LQ-(MH) (CAS 8001-25-0) (Croda, East Yorkshire, UK), Sesame oil (CAS 8008-74-0) (Sigma Aldrich, St, Louis, MO) and 0.9% saline (G-Biosciences, St. Louis, MO) supplemented with 1% Penicillin-Streptomycin Solution (PEST) (100x stock solution, 10,000 units/mL Penicillin, 10,000 μg/mL Streptomycin) (Biowest, Riverside, MO). The positive control, p-Phenylenediamine (PPD) (CAS No. 106-50-3) (Sigma Aldrich, St. Louis, MO) was freshly prepared as 1,000x stock solutions in DMSO and further diluted in respective extraction vehicles prior to in-well cellular exposures, as further described below.
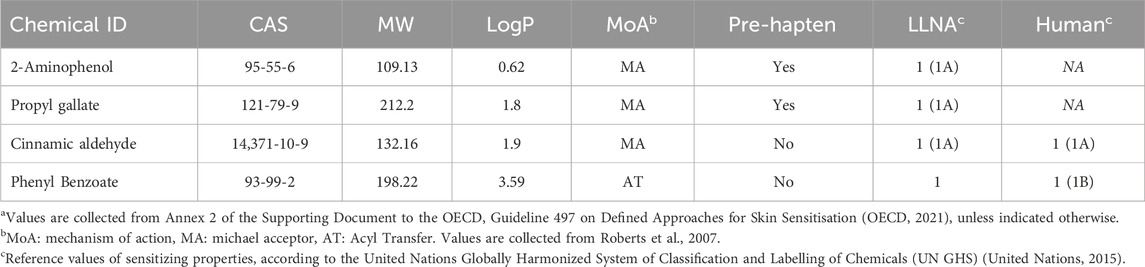
TABLE 1. Details of sensitizing agents used for production of custom materialsa.
Production of silicone-based test materials
To facilitate dispersion in silicone films the sensitizing agents were first dissolved in tetrahydrofuran (THF) (CAS No. 109-99-9) (Sigma-Aldrich) at 1 g/mL. Of each solution with sensitizing agent 500 µL was added to 4.7 g of Silicone, MED-2000 (USP class VI Medical Grade), to obtain a final pre-cured concentration of sensitizer of 9.6% (w/w). The reference silicone, used as negative control material, was impregnated with 500 µL THF without any sensitizing agent added. Uncured silicone samples were mixed using a Speed-Mixer DAC150 FV at 3,500 rpm for 2 min. Silicone mixtures were dispensed into a metal mold (110 × 110 × 2 mm) clamped between two Teflon sheets under pressure and cured at ambient temperature and humidity under pressure (1 kPa) for 72 h. During curing, acetic acid is released resulting in a final concentration of sensitizing agent in the silicone film of 10% (w/w). Due to the volatility of THF, the vehicle is not expected to remain in the silicone film after curing. Following curation, high quality materials and good dispersions of sensitizing agents were ensured by visual inspection and characterized with Attenuated Total Reflection Fourier Transform Infrared Spectroscopy (ATR-FTIR), using a Nicolet 6700 FT-IR Spectrometer (Thermo Scientific, Waltham, MA, US) (data not shown). All test materials were stored at 4°C.
Production of TPU-based test materials
TPU pellets (USP Class VI Medical Grade) were cryomilled in a Retsch ZM200 Ultra Centrifugal Mill (Retsch, Dusseldorf, Germany) to obtain a TPU powder. The TPU powder was thoroughly mixed with skin sensitizing agents at 10% (w/w) using a spatula. The reference TPU film, used as negative control material, was prepared using identical TPU without any impregnating additions. The prepared mixtures were dispensed into a metal mold (110 × 110 × 2 mm) clamped between two Teflon sheets under pressure, using a Fontijne LabEcon 300 hydraulic laboratory press (Fontinje, Rotterdam, Netherlands) and was cured in the press at 180°C, pre-heated for 2 min at the almost closed position before pressing at 50 kN for 1 min and then cooled to 45°C at maintained press force. Following curation, high quality materials were confirmed by visual inspection and ATR-FTIR, as described above (data not shown). All test materials were stored at 4°C.
Test material extractions
The test material was prepared according to ISO 10993-12 (ISO 10993-12, 2021). In short, 0.2 g of the material was incubated, with rotation, at 37°C (±1°C) for 72 h (±2 h) in 1 mL of 0.9% saline supplemented with 1% PEST, 1 mL Super Refined Olive Oil-LQ-(MH) or 1 mL Sesame oil in borosilicate glass vials with caps of Teflon (C405-1) (Thermo Scientific, Waltham, MA). The test material was extracted in three replicates in each vehicle. After the extraction, the test material extracts were used for cell exposure experiments the same day. Vehicle controls (saline, olive oil and sesame oil) were incubated at identical conditions as the test material and were included in each GARD experiment.
GARDskin medical device protocol
The protocols of the conventional GARDskin assay have been previously published (EURL ECVAM, 2021) and summarized in OECD test guideline (OECD, 2023b). In short, the GARDskin utilizes the human myeloid leukemia SenzaCell cell line (ATCC Depository PTA-123875) as an in vitro model for dendritic cells (DC), thus monitoring the immunological activation of DC in response to xenobiotics following test item exposure.
Initial exposure experiments are performed in a titrated range of test item concentrations in order to evaluate its cytotoxic properties. Based on the dose-response relationship of exposure concentrations and cell viability, test item-specific GARD input concentrations are established at low-to non-toxic levels, which is used for downstream testing. Specifically, any test item exhibiting cytotoxic properties are assayed at the concentration yielding ∼90% relative cell viability, referred to as the Rv90 concentration. Correspondingly, non-cytotoxic test items are assayed at the top concentration of the explored titration range, which for neat chemicals is set at a default value of 500 µM.
Following repeated exposure experiments at the GARD input concentration, RNA is isolated from exposed cell cultures in three independent experiments. Here, independent experiments refer to experiments separated in time, with freshly and separately prepared cell cultures, samples and reagents, thus giving rise to biological replicate samples. The gene expression profile, i.e., mRNA levels of the genes in the GARDskin GPS (Johansson et al., 2011), is quantified using NanoString nCounter technology (Geiss et al., 2008) and the skin sensitizing hazard property of the test item is predicted using the GARDskin prediction model, based on a Support Vector Machine (SVM) (Cortes and Vapnik, 1995) prediction algorithm, appropriately trained and frozen during assay development (Forreryd et al., 2016). The prediction algorithm assigns individual samples with decision values (DV), the signs of which are evaluated for hazard classification; any test item with a positive mean DV is classified as a skin sensitizer. Specific details of the complete analysis pipeline, including a background on the identification and immunological relevance of genomic biomarkers of the GPS, data handling, normalization procedures and an in-depth description of the prediction algorithm, are publicly available elsewhere (OECD, 2022a).
The term test item is used here to describe the entity of investigation in any GARDskin assay. However, the GARDskin assay was designed for, and validated for, assessment of neat monoconstituent chemical compounds. The following protocol adaptations were introduced in order to successfully assess sensitizing hazard of chemicals extracted from solid materials, while also complying with relevant ISO 10993 standards.
Firstly, the assay compatibility of a selected set of extraction vehicles, as described in sections above, was evaluated. To prepare for assessment of dilute extracted chemicals in complex formulations, extraction vehicle exposure concentrations were sought to be maximized, while maintaining steady state cell cultures in terms of cell viability and a non-detectable impact on GARDskin classifications. For exposures of oils to cell culture-wells, oil were added to the top of the cell medium, without additional attempts to mix the oil-water phases.
Secondly, when evaluating cytotoxic properties of test material extracts, the top concentration in the titration range was set to correspond to the optimized concentration of the respective extraction vehicles. Subsequent establishment of test material extract-specific GARD input concentrations was done by application of identical decision criteria as used within the conventional GARDskin assay. Of note, however, when testing test material extracts, as opposed to neat chemicals, deviating input concentrations are accepted in downstream repeated exposures giving rise to triplicate RNA samples. This protocol design was introduced to take into account two additional sources of variability; Firstly, test materials cannot be assumed to be 100% homogenous. Depending on the specific parts of the test materials being prepared for extraction, different compositions of test material extracts may be achieved. Secondly, as also considered and described in ISO 10993-12:2021, extraction procedures cannot be assumed to be 100% reproducible, as varying degrees of extraction efficiency may be expected.
Thirdly, the positive control of the conventional GARDskin assay (PPD) was administered in the same extraction vehicles used for administration of test items, at identical exposure conditions and vehicle concentrations. Similarly, the blank extraction vehicles, at identical exposure conditions, were considered negative controls.
Lastly, the prediction model was adapted to mirror the dual testing of polar and non-polar solvents. A test material with a positive mean DV from either, or both, of the polar and non-polar test material extracts is classified as a skin sensitizer.
Thus, the conventional GARDskin assay protocols with the adaptations listed above forms the protocols henceforth referred to as the GARDskin Medical Device protocols, which were used to generate all data in this study. A list of key experimental steps and technical components comparing the conventional GARDskin method with the GARDskin Medical Device protocols is presented in Table 2.
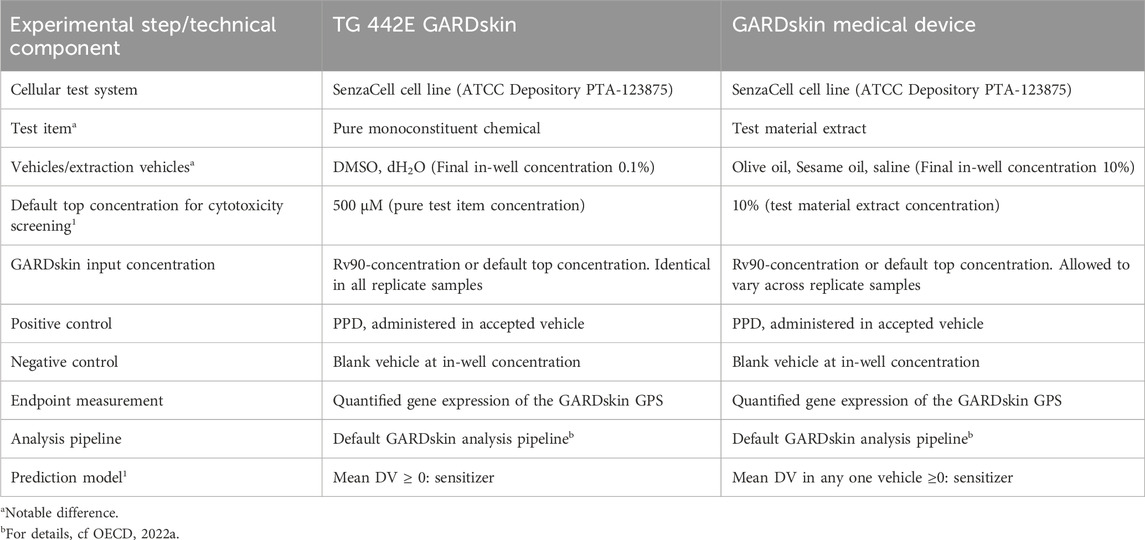
TABLE 2. Comparison of key experimental steps and technical components of the OECD TG442E GARDskin method and the GARDskin Medical Device protocols.
Results
Compatibility of extraction vehicles with the test system
The successful applicability of GARDskin to assess skin sensitizing potential of extracted chemicals from solid materials is dependent on the test system’s compatibility with both polar and non-polar vehicles used for extraction. To this end, the compatibility of saline, olive oil and sesame oil with the test system was evaluated, with results summarized in Figure 1.
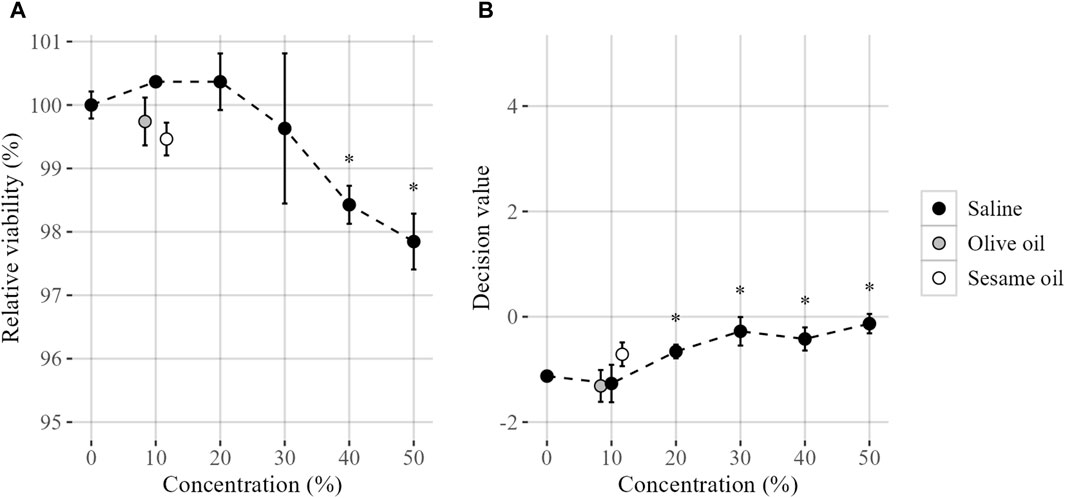
FIGURE 1. Compatibility of extraction vehicles on steady state cell cultures, evaluated by (A) the relative viability and (B) GARDskin Decision Values. Mean values (n = 3) are presented by plotted datapoints, and standard deviation are represented by error bars. Statistical significance (Student’s t-test, p < 0.05) of deviating values compared with unstimulated control are indicated by an asterisk (*). The figures are produced with a jitter function for increased visibility. Tested concentrations were 0, 10, 20, 30, 40% and 50% (saline) and 0% and 10% (olive and sesame oil).
Firstly, a range of in-well saline concentrations were evaluated. The saline was well tolerated by the SenzaCell cell line, with a non-detectable impact of relative cell viability, as compared to unstimulated cells, using up to 30% saline in-well. However, further increase of the saline concentration results in a small, but statistically significant, decrease in relative cell viability.
The impact of saline on the GARDskin prediction algorithm output, i.e., the DV:s generated by the SVM classifier, was also investigated. While negative classifications are generated throughout the titration range, a statistically significant impact is detectable on saline concentrations of 20% and above, compared to unstimulated cells. Thus, it was concluded that a top concentration of 10% saline is compatible with the test system, with maintained steady-state cell cultures.
The compatibility with the non-polar vehicles olive oil and sesame oil was then evaluated, guided by the results of the saline evaluation. It was confirmed that both olive and sesame oil were tolerated by the test system at the proposed concentration (10%), again by monitoring the impact of relative cell viability compared to unstimulated cells, as well as the potential impact on baseline GARDskin DV:s.
Following these findings, the GARD skin Medical Device protocols adopted a procedure in which all test item exposures are administrated with an in-well extraction vehicle concentration of 10%. Any dilutions of test material extracts and controls required in order to mitigate cytotoxicity are done using the corresponding blank vehicle, so that all cell exposures, irrespective of dilution factor of the test item, are performed with an identical and non-detectable background signal from the extraction vehicle.
GARDskin predictions of test materials and controls
In the absence of readily available medical device-associated materials with sensitizing properties, the functionality of the extraction procedures and the ability of the GARDskin prediction model to accurately detect the presence of sensitizing agents in obtained extracts was demonstrated using customized test materials. First, a silicone material (MED-2000) was spiked with 2-aminophenol, propyl gallate, cinnamic aldehyde and phenyl benzoate and assayed along with an unspiked negative control material. Similarly, a TPU material was spiked with 2-aminophenol and cinnamic aldehyde and assayed along with an unspiked negative control material. Lastly, these customized test materials were complemented with commercially available counterparts, i.e., tubes made out of silicone and TPU, as well as a PVC material. All eleven test materials were subjected to extraction procedures in adherence with ISO standards, using saline and olive oil as polar and non-polar vehicles, respectively. In addition, all but the customized TPU materials were extracted using sesame oil as a complimentary polar vehicle.
All resulting test items (i.e., extracts) were assayed according to the GARDskin Medical Device protocols, as established above. In an initial step, the cytotoxic properties of each test item were evaluated. As defined, all non-cytotoxic test items were assayed at the default top concentration of 10%. Any test item inducing cytotoxicity was diluted in the respective extraction vehicle, to the concentration inducing 90% relative cell viability (defined as the Rv90 concentration), before being administered to in-well cell exposures. All final test item concentrations used for downstream analysis are summarized in Table 3.
Following cell exposures, the conventional GARDskin protocols were followed. Total RNA was isolated from exposed cell cultures and the gene expression levels of the GARDskin GPS were quantified using NanoString instrumentation. Lastly, the GARDskin prediction model was employed to classify each test material extract, with results being summarized in Figure 2. It was concluded that all spiked customized materials were accurately classified as sensitizers, while unspiked customized materials, as well as all commercially available products, were accurately classified as non-sensitizers. Of importance, all classifications of spiked materials as sensitizers match those of the corresponding GARDskin classifications of the pure sensitizing agents, all of which have been assayed previously (2-aminophenol; Forreryd et al., 2016; Forreryd et al., 2023a; Johansson et al., 2017. Propyl gallate; Johansson et al., 2017; Johansson et al., 2019. Phenyl benzoate; Johansson et al., 2017. Cinnamic aldehyde; Johansson et al., 2019). Of further note, all sensitizing agents were accurately classified in all test material extracts, irrespective of the extraction vehicle being used. However, MED-2000 spiked with phenyl benzoate was assigned with DV:s of notably smaller magnitude when extracted with olive oil. A confirmed explanation as to why this is the case is not available, however, it can be hypothesized that the sensitizing agent was more successfully extracted using saline or sesame oil.
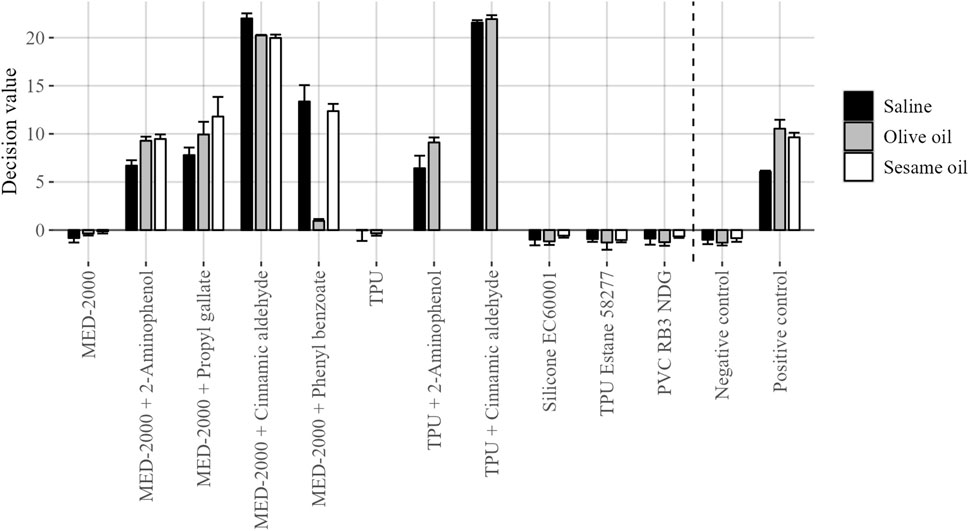
FIGURE 2. GARDskin classification results of investigated test material extracts. Plotted data corresponds to mean decision values (n = 3), with standard deviation represented by error bars.
Taken together, the adapted GARDskin Medical Device prediction model, which is based on evaluation of both a polar and non-polar extract, would have classified each spiked material as a sensitizer, irrespective of if olive oil or sesame oil were used.
Discussion
Skin sensitization is, alongside the endpoints of irritation and cytotoxicity, one of the three biological endpoints that must be evaluated for all medical devices (ISO 10993-1, 2018). In the cases of irritation (ISO 10993-23, 2021) and cytotoxicity (ISO 10993-5, 2009), validated in vitro methods have been qualified and are well established in routine use. However, skin sensitization testing of medical device products and materials are in practice predominantly being done by the use of in vivo experimentation. Nonetheless, the ISO 10993 series of standards explicitly encourages the use of in vitro methods, provided such methods are sufficiently validated, as defined by the regulatory context. To this end, ongoing efforts aim to qualify existing and emerging NAM:s for skin sensitization to the applicability domain of medical devices.
In the current study, obtained data demonstrate that GARDskin (OECD, 2023b) can, with few minor adaptations to the conventional protocol, be applied to accurately predict the sensitizing potential of medical device polar and non-polar extracts, prepared according to established extraction procedures described in ISO 10993-12. Furthermore, it was shown that the method is sufficiently sensitive to accurately detect extracted chemicals in such complex mixtures, from two custom materials, spiked with weak, moderate and strong chemical skin sensitizers. Here, custom-made spiked materials were employed in the absence of readily available medical devices with known and well-characterized sensitizing properties. Indeed, all spiked materials, being silicone-based or TPU-based, respectively, were classified as sensitizing hazards by the GARDskin method, based on response-signals originating from both polar and non-polar extracts. Of further note, both sesame oil and olive oil, which are commonly used non-polar extraction vehicles for skin sensitization testing of medical devices, were demonstrated to work with the assay. While all test material extracts produced from spiked materials were individually classified as positive by the GARDskin prediction model, this also shows that the adapted GARDskin Medical Device prediction model classified all spiked materials as positive, in line with the expectation, irrespective of which non-polar vehicle was considered by the prediction model. Lastly, the specificity of the method was further explored by testing commercially available silicone and TPU products, as well as a PVC product, using identical protocols. Here, all commercial materials were classified as non-sensitizers.
To the best of our knowledge, this is the first time an OECD TG 442E method for skin sensitization has been shown to be compatible with ISO 10993 established extraction procedures and vehicles. However, recent work investigates the response in both DPRA (OECD TG 442C) and LuSens (OECD TG 44D) when testing a set of commercially available medical devices (Svobodová et al., 2021). While the specificity from such testing is encouraging, a systematic evaluation of positive response signals from testing of expected positive control materials is lacking. In addition, examples of similar work include the use of non-validated methods and experimental setups (McKim et al., 2012; Coleman et al., 2015; Pellevoisin et al., 2021). The lack of validated in vitro methods explored for their applicability for testing of medical devices may, at least in part, be attributed to the difficulties typically associated with applying oil-based test material extracts to submerged cell cultures. Indeed, in work by Svobodova et al., the organic solvent used for extractions were DMSO (LuSens) or excluded altogether (DPRA). In the present study, oil-based extracts were added to the surface of cell culture-wells, without any attempts to further mix the oil-water phases. Nonetheless, it was concluded that positive signals were obtained also from such oil-exposed samples. This may partially be explained by the low limit of detection of GARDskin, which has been demonstrated to be able to detect sensitizing agents at concentrations of only a few ppm (Gradin et al., 2021). In addition, GARDskin has been shown to be widely applicable for hydrophobic substances (Forreryd et al., 2023a), which together with herein presented data may suggest that oil-water phase separation may not necessarily constitute a hurdle. However, it must be considered that these conclusions are, so far, based on a small set of sensitizing agents, all of which are at least in part soluble in water, with LogP-values ranging up to 3.59. Further testing of a wider chemical domain is warranted, in order to explore if these conclusions hold true also for even more hydrophobic substances.
Even though classifications of all materials were successful and concordant across the different solvents, a notable observation was made for MED-2000 spiked with phenyl benzoate, for which the olive oil generated DV:s with significantly lower magnitude, compared to both sesame oil and saline. No apparent explanation as to why is readily available. Indeed, olive oil and sesame oil have a similar composition (Burnett et al., 2017) and therefore also their extraction efficiencies may be assumed to be comparable. Nonetheless, potential hypothesized explanations may include differential extraction efficiencies, unique solvent components with quenching effects, or deviating responsiveness in exposed cell cultures. Repeated testing and chemical characterization of the extracts may be warranted to reach firm conclusions.
It may be noted that the term ‘medical device’ may encompass a wide range of products, with varying physical and physiochemical properties. While the herein presented work is specifically aimed to address issues arising when testing solid materials, a number of medical devices, such as readily available complex mixtures and substance-based devices, are not expected to be advantageously tested using extraction protocols. In such instances, it is important to consider the applicability domain of each test method, to ensure appropriate testing of specific products. To this end, the ability of GARDskin to accurately detect skin sensitizing properties in complex samples, such as mixtures and formulations (Corvaro et al., 2023), UVCBs (Forreryd et al., 2023a) and metals (Forreryd et al., 2023b) have been demonstrated. In addition, applicability has been characterized across a wide chemical domain (OECD, 2023b). As such, it is expected that GARD skin may be widely applied to address the testing needs of a wide range of medical devices with varying properties.
Moving forward, the acceptance of NAM:s for the use of testing of skin sensitizing properties of medical devices will ultimately depend on regulating bodies, which in turn will depend on both the regulatory context and the geographical location of the relevant market. In order to harmonize all efforts to reach acceptance across all markets, experts within the ISO technical committee (TC) 194 recently published a technical specification describing the validating qualification steps foreseen to be required (ISO/TS 11796, 2023). This guidance may be viewed as complementary to guidance provided by the OECD for testing of chemicals (c.f. OECD Guidance Document No. 1 and No. 34). Using the definitions provided in ISO/TS 11796, the herein presented work may be regarded as a ‘feasibility study’, detailing all required amendments to the conventional GARDskin protocols, as well as demonstrating functionality. As such, a ‘pre-validation study’, comprising testing of a set of negative reference material extracts (polar and non-polar) spiked with a known concentration of a chemical skin sensitizer, as well as a ‘validation study’, comprising additional testing of a smaller set of test items in an inter-laboratory exercise, are expected to follow.
In conclusion, we here show how the conventional protocols of the GARDskin method for assessment of chemical skin sensitizers can be adapted to comply with relevant ISO 10993 standards for testing of solid materials. We demonstrate the functionality of the amended protocols by accurate skin sensitizing hazard classifications of custom produced solid materials, spiked with sensitizing agents. Lastly, we confirm the specificity of the proposed method by accurate non-hazard classifications following testing of commercially available solid materials. The herein presented results constitute an important step towards the replacement of in vivo testing of medical devices.
Data availability statement
The raw data supporting the conclusion of this article will be made available by the authors, without undue reservation.
Author contributions
R-MJ: Conceptualization, Project administration, Writing–review and editing. OL: Methodology, Writing–review and editing. AJ: Methodology, Writing–review and editing. MB: Methodology, Writing–review and editing. EP: Conceptualization, Project administration, Writing–review and editing. HJ: Conceptualization, Supervision, Writing–original draft, Resources, Writing–review and editing.
Funding
The author(s) declare that no financial support was received for the research, authorship, and/or publication of this article. The reported study was funded in its entirety by SenzaGen AB.
Acknowledgments
The authors wish to acknowledge contributions by and thank Dr. Robin Gradin for preparations of figures and Henrik Petersen for assistance in production of custom materials.
Conflict of interest
R-MJ, OL, AJ, and HJ were employees of SenzaGen AB, the method developer of GARDskin.
The remaining authors declare that the research was conducted in the absence of any commercial or financial relationships that could be construed as a potential conflict of interest.
Publisher’s note
All claims expressed in this article are solely those of the authors and do not necessarily represent those of their affiliated organizations, or those of the publisher, the editors and the reviewers. Any product that may be evaluated in this article, or claim that may be made by its manufacturer, is not guaranteed or endorsed by the publisher.
References
Buehler, E. V. (1965). Delayed contact hypersensitivity in the Guinea pig. Archives Dermatology 91, 171–177. doi:10.1001/archderm.1965.01600080079017
Burnett, C. L., Fiume, M. M., Bergfeld, W. F., Belsito, D. V., Hill, R. A., Klaassen, C. D., et al. (2017). Safety assessment of plant-derived fatty acid oils. Int. J. Toxicol. 36 (3), 51S–129S. doi:10.1177/1091581817740569
Coleman, K. P., Grailer, T. P., McNamara, L. R., Rollins, B. L., Christiano, N. J., Kandárová, H., et al. (2018). Preparation of irritant polymer samples for an in vitro round robin study. Toxicol Vitro 50, 401–406. doi:10.1016/j.tiv.2018.01.018
Coleman, K. P., McNamara, L. R., Grailer, T. P., Willoughby Sr, J. A., Keller, D. J., Patel, P., et al. (2015). Evaluation of an in vitro human dermal sensitization test for use with medical device extracts. Appl. Vitro Toxicol. 1 (2), 118–130. doi:10.1089/aivt.2015.0007
Cortes, C., and Vapnik, V. (1995). Support-vector networks. Mach. Learn. 20 (3), 273–297. doi:10.1007/bf00994018
Corvaro, M., Henriquez, J., Settivari, R., Mattson, U. T., Forreryd, A., Gradin, R., et al. (2023). GARD™skin and GARD™potency: a proof-of-concept study to investigate the applicability domain for agrochemical formulations.
De Jong, W. H., Hoffmann, S., Lee, M., Kandárová, H., Pellevoisin, C., Haishima, Y., et al. (2018). Round robin study to evaluate the reconstructed human epidermis (RhE) model as an in vitro skin irritation test for detection of irritant activity in medical device extracts. Toxicol Vitro 50, 439–449. doi:10.1016/j.tiv.2018.01.001
EURL ECVAM (2021). GARDskin assay protocol. Available at: https://tsar.jrc.ec.europa.eu/system/files/Published/GARDskin%20Assay%20Protocol%20TSAR.pdf.
Forreryd, A., Gradin, R., Humfrey, C., Sweet, L., and Johansson, H. (2023a). Exploration of the GARD™skin applicability domain: indirectly acting haptens, hydrophobic substances and UVCBs. ALTEX 40 (1), 53–60. doi:10.14573/altex.2201281
Forreryd, A., Gradin, R., Larne, O., Rajapakse, N., Deag, E., and Johansson, H. (2023b). The GARD™skin assay: investigation of the applicability domain for metals. ALTEX 40 (3), 425–438. doi:10.14573/altex.2203021
Forreryd, A., Zeller, K. S., Lindberg, T., Johansson, H., and Lindstedt, M. (2016). From genome-wide arrays to tailor-made biomarker readout - progress towards routine analysis of skin sensitizing chemicals with GARD. Toxicol Vitro 37, 178–188. doi:10.1016/j.tiv.2016.09.013
Geiss, G. K., Bumgarner, R. E., Birditt, B., Dahl, T., Dowidar, N., Dunaway, D. L., et al. (2008). Direct multiplexed measurement of gene expression with color-coded probe pairs. Nat. Biotechnol. 26 (3), 317–325. doi:10.1038/nbt1385
ISO 10993-1 (2018). Biological evaluation of medical devices — Part 1: evaluation and testing within a risk management process. Available at: https://www.iso.org/standard/68936.html.
ISO 10993-10 (2021). Biological evaluation of medical devices — Part 10: tests for skin sensitization. Available at: https://www.iso.org/standard/75279.html.
ISO 10993-12 (2021). Biological evaluation of medical devices — Part 12: sample preparation and reference materials. Available at: https://www.iso.org/standard/75769.html.
ISO 10993-23 (2021). Biological evaluation of medical devices — Part 23: tests for irritation. Available at: https://www.iso.org/standard/74151.html.
ISO 10993-5 (2009). Biological evaluation of medical devices — Part 5: tests for in vitro cytotoxicity. Available at: https://www.iso.org/standard/36406.html.
ISO/TS 11796 (2023). Biological evaluation of medical devices — requirements for interlaboratory studies to demonstrate the applicability of validated in vitro methods to assess the skin sensitization of medical devices. Available at: https://www.iso.org/standard/83916.html.
Johansson, H., Gradin, R., Forreryd, A., Agemark, M., Zeller, K., Johansson, A., et al. (2017). Evaluation of the GARD assay in a blind Cosmetics Europe study. ALTEX 34 (4), 515–523. doi:10.14573/altex.1701121
Johansson, H., Gradin, R., Johansson, A., Adriaens, E., Edwards, A., Zuckerstätter, V., et al. (2019). Validation of the GARD™skin assay for assessment of chemical skin sensitizers: ring trial results of predictive performance and reproducibility. Toxicol. Sci. 170 (2), 374–381. doi:10.1093/toxsci/kfz108
Johansson, H., Lindstedt, M., Albrekt, A. S., and Borrebaeck, C. A. (2011). A genomic biomarker signature can predict skin sensitizers using a cell-based in vitro alternative to animal tests. BMC Genomics 12, 399. doi:10.1186/1471-2164-12-399
Kerecman Myers, D., Goldberg, A. M., Poth, A., Wolf, M. F., Carraway, J., McKim, J., et al. (2017). From in vivo to in vitro: the medical device testing paradigm shift. ALTEX 34 (4), 479–500. doi:10.14573/altex.1608081
Magnusson, B., and Kligman, A. M. (1969). The identification of contact allergens by animal assay. The Guinea pig maximization test. J. Investigative Dermatology 52, 268–276. doi:10.1038/jid.1969.42
McKim, J. M., Keller, D. J., and Gorski, J. R. (2012). An in vitro method for detecting chemical sensitization using human reconstructed skin models and its applicability to cosmetic, pharmaceutical, and medical device safety testing. Cutan. Ocul. Toxicol. 31 (4), 292–305. doi:10.3109/15569527.2012.667031
OECD (2014). “The adverse Outcome Pathway for skin sensitisation initiated by covalent binding to proteins,” in OECD series on testing and assessment (Paris: OECD Publishing). doi:10.1787/9789264221444-en
OECD (2021). Series on testing and assessment No. 336: Annex 2 of the supporting document to the guideline (GL) on defined Approaches (DAs) for skin sensitisation. Available at: http://www.oecd.org/env/testguidelines.
OECD (2022a). Supporting document to the genomic allergen Rapid detection test method for skin sensitisation (GARD™skin), described in test guideline 442E. OECD series on testing and assessment, No. 357. Paris: OECD Publishing.
OECD (2022b). “Test No. 442D,” in Vitroskin sensitisation: ARE-Nrf2 luciferase test method. OECD guidelines for the testing of chemicals, section 4 (Paris: OECD Publishing). doi:10.1787/9789264229822-en
OECD (2023a). “Test No. 442C,” in Chemico skin sensitisation: assays addressing the adverse Outcome Pathway key event on covalent binding to proteins (Paris: OECD Publishing). doi:10.1787/9789264229709-en
OECD (2023b). “Test No. 442E,” in Vitroskin sensitisation: in vitro skin sensitisation assays addressing the key event on activation of dendritic cells on the adverse Outcome Pathway for skin sensitisation (Paris: OECD Publishing). doi:10.1787/9789264264359-en
Pellevoisin, C., Cottrez, F., Johansson, J., Pedersen, E., Coleman, K., and Groux, H. (2021). Pre-validation of SENS-IS assay for in vitro skin sensitization of medical devices. Toxicol Vitro 71, 105068. doi:10.1016/j.tiv.2020.105068
Roberts, D. W., Patlewicz, G., Kern, P. S., Gerberick, F., Kimber, I., Dearman, R. J., et al. (2007). Mechanistic applicability domain classification of a local lymph node assay dataset for skin sensitization. Chem. Res. Toxicol. 20 (7), 1019–1030. doi:10.1021/tx700024w
Svobodová, L., Rucki, M., Vlkova, A., Kejlova, K., Jírová, D., Dvorakova, M., et al. (2021). Sensitization potential of medical devices detected by in vitro and in vivo methods. ALTEX 38 (3), 419–430. doi:10.14573/altex.2008142
Keywords: gard, skin sensitization, in vitro, NAMs, medical device, ISO 10993, biocompatibility
Citation: Jenvert R-M, Larne O, Johansson A, Berglin M, Pedersen E and Johansson H (2024) Evaluation of the applicability of GARDskin to predict skin sensitizers in extracts from medical device materials. Front. Toxicol. 6:1320367. doi: 10.3389/ftox.2024.1320367
Received: 12 October 2023; Accepted: 26 February 2024;
Published: 12 March 2024.
Edited by:
Montse Mitjans Arnal, Universitat de Barcelona, SpainReviewed by:
Emanuela Corsini, University of Milan, ItalyCynthia Ryan, University of Alabama at Birmingham, United States
Xiao Huang, International Flavors and Fragrances, United States
Copyright © 2024 Jenvert, Larne, Johansson, Berglin, Pedersen and Johansson. This is an open-access article distributed under the terms of the Creative Commons Attribution License (CC BY). The use, distribution or reproduction in other forums is permitted, provided the original author(s) and the copyright owner(s) are credited and that the original publication in this journal is cited, in accordance with accepted academic practice. No use, distribution or reproduction is permitted which does not comply with these terms.
*Correspondence: Henrik Johansson, aGVucmlrLmpvaGFuc3NvbkBzZW56YWdlbi5jb20=
†Present addresses: Rose-Marie Jenvert, Veranex Sweden AB, Gothenburg, Sweden
Angelica Johansson, Department of Immunotechnology, Lund University, Lund, Sweden
Emma Pedersen, Mölnlycke Healthcare AB, Gothenburg, Sweden