- 1Department of Veterinary Public Health and Preventive Medicine, Faculty of Veterinary Medicine, University of Ibadan, Ibadan, Nigeria
- 2Federal College of Animal Health and Production Technology, Ibadan, Nigeria
- 3Department of Veterinary Medicine, Faculty of Veterinary Medicine, University of Ibadan, Ibadan, Nigeria
Introduction: Glyphosate is a non-targeted organophosphate insecticide whose solubility and mobility in hydrophilic solvents enable its rapid leaching into the soil and subsequent contamination of ground and surface water and possible build-up in the aquatic food chain. Based on the public health importance of glyphosate in fish through consumption, it is crucial to determine the current residue concentration in culture Clarias gariepinus species. The aim of the present study is to evaluate glyphosate’s residue concentrations and its metabolites in cultured African Catfish offered for sale in selected markets in Ibadan.
Methods: A total of twenty-five (25) adult Clarias gariepinus (300 ± 50 g) were sourced from five (5) selected active fish markets (Ojoo, Iwo road, Eleyele, Challenge, and Apata) within the Ibadan metropolis. The collected fish tissue samples (liver, kidney, and spleen) were prepared for glyphosate residue concentration analysis using Liquid Chromatography (LC).
Results: The results showed that glyphosate residues were recorded in all the seventy-five (75) fish tissue samples obtained from the selected fish markets in the Ibadan metropolis and all residue concentrations were above both the recommended Acceptable Daily Intake (ADI) of 1.0 mg/kg (1 × 10−3 mg/L) and Maximum Residue Limits (MRL) of 0.01 mg/kg (1 × 10−5 mg/L). Isopropylamine has the highest residue concentration followed by N-Phosphonomethyl and Aminomethylphosphonic Acid (AMPA), while N-Acetyl Glyphosate has the least residue concentration across the sampled markets.
Discussion: The presence of residues of glyphosate and its metabolites in ready-to-eat fish calls for holistic, systematic, and effective risk management strategies towards monitoring pesticide/herbicide usage in aquaculture production and ensuring the provision of wholesome fish and fish products for the consumers.
Introduction
Fish farming is a popular industry in Nigeria with increased demand for nutritious animal protein. Catfish farming is practiced by around 80% of fish farmers in Nigeria, and it is the most popular variety of fish employed in aquaculture. As a result, the usage of agrochemicals for fish growth and sustainability has increased. On farms, various agrochemicals such as Formalin, Copper Sulphate, Malachite green, and Potassium Permanganate among others have been abused and/or misused (Adeyemo et al., 2011; Alarape et al., 2013). The adverse side effects of glyphosate and aminomethyl phosphonic acid, or metabolites, on soil, water quality, plant, animal, and human health have been studied extensively in recent years due to glyphosate’s extensive use and accumulation in the environment and food products (Battaglin et al., 2014; Séralini et al., 2014). In 2015, the World Health Organization (WHO) categorized the glyphosate herbicide as possibly human carcinogenic based on their most recent findings on its potential chronic negative effects (EFSA, 2015; Guyton et al., 2015; IARC, 2015).
According to the Rodriguez-Gil et al. (2017) report, a typical and maximum application of glyphosate and runoff rates from the soil resulted in estimated concentrations of 0.21–0.99 mg POEA L−1 surface water, which were responsible for the estimated impairment of 21%–43% of a wide range of aquatic species. Sandrini et al. (2013) found that pure glyphosate suppressed acetylcholinesterase activity in brown mussels (Perna perna) and several fish species at low concentrations (1–676 mg L−1) (Menéndez-Helman et al., 2012; Sandrini et al., 2013). These findings are consistent with the effects of glyphosate on terrestrial animals. In zebrafish embryos exposed to Roundup® at higher concentrations (50 mg L−1), developmental (forebrain, midbrain, and eye) damage was seen. Glyphosate was reported to have damaged the zebrafish primary motoneurons at a low concentration of 0.01 mg L−1 resulting in erratic movements at a young age (Roy et al., 2016; Zhang et al., 2017a). The metabolism in many tissues was impacted by the prolonged exposure of goldfish (Carassius auratus) to glyphosate (34 mg L−1), which resulted in an excess of oxidative stress and severe kidney damage (Li et al., 2017). The interactions between fish and their diseases are also impacted by glyphosate, in addition to the direct effects on aquatic creatures mentioned above.
Herbicides made of glyphosate may pollute the soil in and surrounding treated regions. Because of its delayed breakdown by soil microbes due to its adsorption to clay and organic matter, which causes its accumulation in soils over time, glyphosate was initially not thought to be an issue for both ground and surface water (Sihtmäe et al., 2013; Banks et al., 2014; Sviridov et al., 2015; Travaglia et al., 2015; Cassigneul et al., 2016; Okada et al., 2016; Sidoli et al., 2016). By multiple earlier observations, glyphosate and metabolites, its breakdown product, may linger for more than a year in soils with a high clay concentration, but swiftly wash out of sandy soils (Bergström et al., 2011; Okada et al., 2016; Sidoli et al., 2016). Despite its attachment to clay and organic matter, glyphosate and metabolites are degraded in part by soil pH (Zhang et al., 2015b), and after a significant amount of rain, some of the chemicals dissolve in groundwater (Maqueda et al., 2017). The soil particles carrying glyphosate and metabolites are also transported into surface water by rain and erosive processes, where they can either persist in the particulate phase or dissolve (Maqueda et al., 2017).
While contaminated particles might settle and mix with the bottom sediment, dissolved glyphosate and metabolites in surface water can sorb down to the bottom (Maqueda et al., 2017). Due to its widespread acceptance, glyphosate and its metabolites are currently present in large quantities in a range of natural waterways and sediments. Glyphosate biodegrades much more slowly in sediment than in water (Grandcoin et al., 2017; Maqueda et al., 2017; Poiger et al., 2017). In the United States, where glyphosate-resistant crops are produced on genetically engineered plants, glyphosate and metabolites are commonly found in soil, surface water, and groundwater (Battaglin et al., 2014). Glyphosate levels in the river and stream water have been found to range from 2 to 430 g L−1 (Coupe et al., 2011; Mahler et al., 2017). Additionally, it has been found in the spring snowmelt, air, and rain during the planting and growing season (Chang et al., 2011). When glyphosate is used, it eventually winds up in seawater, where it is extremely persistent (Mercurio et al., 2014).
While growing genetically modified crops is prohibited in Europe, unlike the United States, glyphosate has been found in several water sources (although at lower concentrations than in the United States) with very low concentrations (0.1–2.5 g L−1) surface water samples in Germany (Skark et al., 1998), northeastern Spain (Sanchis et al., 2012), Hungary (Mörtl et al., 2013), France (Villeneuve et al., 2011), and Switzerland (Poiger et al., 2017). In addition to runoff from agricultural land, Grandcoin et al. (2017) and Hanke et al. (2010) suggest that urban runoff is another source of glyphosate for streams and rivers. According to Rosenbom et al. (2010), numerous Northern European nations have outlawed the use of glyphosate on paved surfaces because it increases runoff from impermeable and linked paved surfaces. Yet glyphosate and metabolites were discovered in samples of sewage and stormwater runoffs, outputs from wastewater treatment plants, and even packaged bottled water (Birch et al., 2011; Grandcoin et al., 2017). According to reports, glyphosate and metabolites are typically found in drinking water, however at very low amounts that are below the recommended daily intake (ADI) that was established in 1997 (WHO, 2005).
Although, Bastos et al. (2020) reprted that there were no published works that have evaluated the toxicological effects of glyphosate in aquatic mammals and birds which demonstrated lack of knowledge on the risk of exposure of aquatic animal in the environments contaminated by glyphosate. But in contrary, Shehata et al. (2014), treported that glyphosate residues could be found in the liver, spleen, lung, intestine, heart, muscles, kidney, and animal feed. This is because glyphosate is applied at higher rates and more frequently than before. Eighty-nine percent (89%) of the corn crop and 94% of the soybean crop in the United States were herbicide-tolerant varieties, and glyphosate was likely sprayed on most of them. Additionally, a considerable portion of the feed used for animals comes from genetically modified crops. According to research, 57% of maize and 85% of soybeans are used annually in livestock diets worldwide (McNaughton et al., 2011). In none of the aforementioned research was it discovered that feeding animals glyphosate-sprayed crops during cultivation decreased their output. In the findings of Muhammad et al. (2021), they concluded that a relatively high concentration of technical grade glyphosate is needed to induce significant changes in fish, however, it was stated that the bodyweight index is the most sensitive toxicity parameter in that at 25 mg/L of glyphosate, there was reduction in the fish body weight. Negative correlations between the glyphosate concentration and toxicity parameters such as specific growth rate (SGR), hepato-somatic index (HIS), and gonado-somatic index (GSI) were observed (Muhammad et al., 2021). Ruminants are major consumers of genetically modified (GE) crops, and as bacterial protein and end products of microbial fermentation make up a sizable amount of their metabolizable nutrients, they serve as models for research on the impact of pesticide residues on gut microbes. Similar to this, although microbial proteins are not consumed, bacterial fermentation activity in the hindguts of non-ruminant animals is significant for some nutrients. Recently, glyphosate exposure toxicity has been reported to cause several changes such as haematologic and biochemical processes in tissues (Modesto and Martinez, 2010), genotoxicity (Guilherme et al., 2012; de Brito Rodrigues et al., 2019), histopathological damage and immunotoxicity (Antunes et al., 2017; Ma et al., 2019), or cardiotoxicity (Gaur and Bhargava, 2019) in fish.
This present study was designed to evaluate the residue concentrations of glyphosate and its metabolites in cultured African Catfish offered for sale in selected markets in Ibadan.
Materials and methods
Sample collection
Five adults Clarias gariepinus samples (300 ± 50 g) each were sourced from five selected active cultured fish markets (Ojoo, Iwo road, Eleyele, Challenge, and Apata) within the Ibadan metropolis. A total number of twenty-five adult Clarias gariepinus were transported to the Fish and Wildlife Unit Laboratory, Department of Veterinary Public Health and Preventive Medicine, Faculty of Veterinary Medicine, University of Ibadan.
Each fish was pithed before sacrifice. Organs (muscle, liver, and kidney) were then harvested through dissection of the fish and separately put inside the sterile sample nylons, sealed, and kept under the ice. The collected samples were then taken to the International Institute of Tropical Agriculture (I.I.T.A) for glyphosate residue concentration analysis.
Solid-phase extraction (SPE) and quantification
The SPE was carried out based on the procedures described by Delmonico et al. (2014). The digestion tubes were washed with water, later rinsed with distilled water, and finally rinsed using 0.5% HCl in ultra-pure water (purest scientific water) and allowed to dry. The fish tissue samples were homogenized and stored in Pyrex tubes in a viscous form. 0.5 mL of homogenized samples were poured into each of the digestion tubes using a precision pipette. About 20 mL of the ammomethyl-phosphonic-nitric acids mixture in the ratio 5:2:5 was then dispensed into each of the digestion tubes containing the samples. The mix is then digested for two and half hours on a digestion block at 25°C and covered with a condenser.
At the end of the digestion process, the digests were brought out and allowed to cool to room temperature (25°C). Each cooled digest was made up to 25 mL volumes with ultra-pure water, covered with paraffin paper, swirled to mix properly, tightly covered, and shaken on a mechanical shaker for 10 min. They were then centrifuged for 5 min at 5,000 revolutions per minute (rpm). The resultant supernatants were analyzed using Reverse Phase LC (Agilent Technologies, Santa Clara, CA, United States) with C18, 5 µm 120 Â, 4.6 × 250 mm column as stationary phase and methanol/H2O (90:10) as mobile phase at a flow rate of 1 mL/min for 30 min.
Method validation
The SPE-LC method’s specificity, linearity, sensitivity, accuracy, precision, and resistance to matrix effects were all validated.
Data analysis
Data obtained were calculated by the interphase software with LC. The results were expressed in frequency, percentages, and variance (descriptive statistics) on SPSS Statistics Version 26.
Ethical consideration
The University of Ibadan Animal Care and Use in Research Committee accepted the research protocol, which was given the number UI-ACUREC/019-0220/6.
Results
The residue concentrations of glyphosate its metabolites as detected in fish organs and muscles from different fish markets are shown in Supplementary Figures S1–S15. Isopropylamine has the highest residue concentration followed by N-Phosphonomethyl and Aminomethylphosphonic Acid (AMPA), while N-Acetyl Glyphosate has the least residue concentration in fish organs and muscles obtained from Ojoo fish market (Supplementary Figures S1–S3).
From the ANOVA result obtained in Table 1, there is significance difference (p-value less than 0.05) among the fish organs and muscles across the glyphosate and its metabolites.
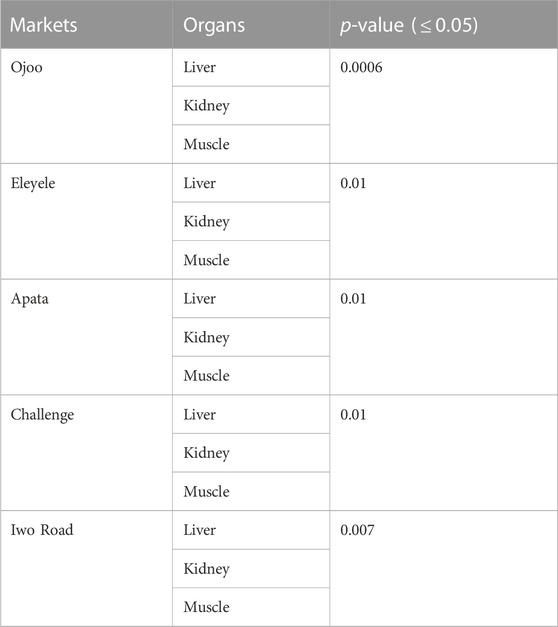
TABLE 1. Comparison of glyphosate and its metabolites’ residue concentrations in organs and muscles of cultured African Catfish offered for sales at different fish markets in Ibadan.
The average residue concentrations of glyphosate and its intermediates as detected in the fish organs and muscles samples (liver, kidney, and muscle) were shown in Figures 1–5. Isopropylamine has the highest statistically significant residue concentration (p-value ≤0.05) followed by N-Phosphonomethyl and Aminomethylphosphonic Acid (AMPA), while N-Acetyl Glyphosate has the least residue concentration in fish organs and muscles obtained across all the fish market (Figures 1–5). Muscle had the highest residue concentration of both glyphosate and its metabolites across all the fish markets. Glyphosate and its metabolites residue concentration detected in Kidney were lower than those detected in muscle and higher than the detected concentrations in the liver samples across all the fish markets (Figures 1–5).
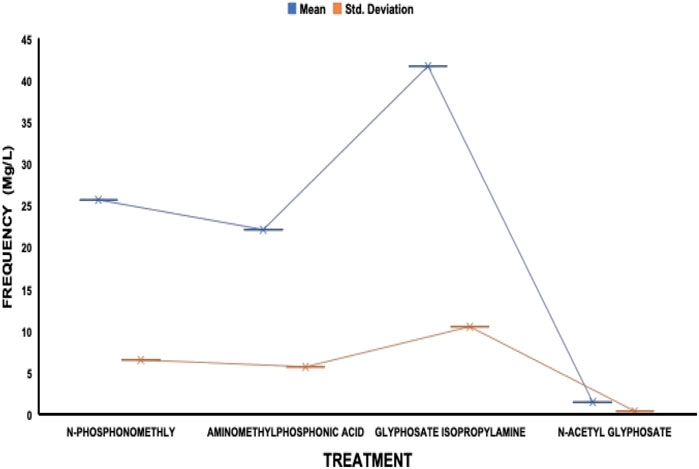
FIGURE 1. Frequency of glyphosate and its metabolites’ residue concentrations present in the Catfish organs and muscles offered for sale at Ojoo fish market. The error bars of box plots represent mean and standard deviation.
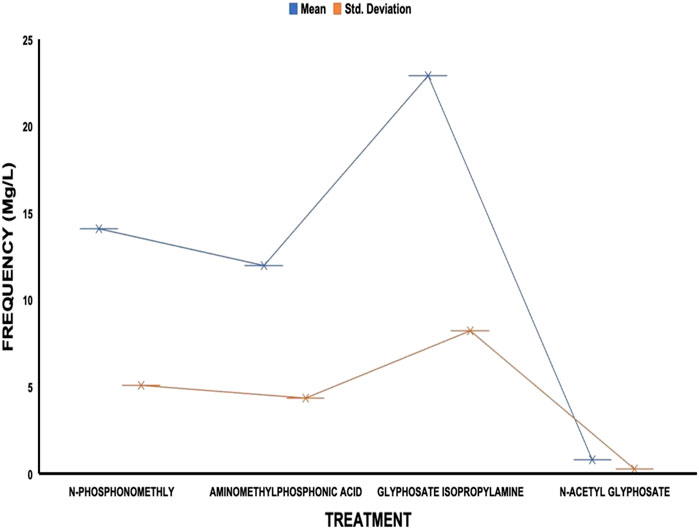
FIGURE 2. Frequency of glyphosate and its metabolites’ residue concentrations present in the Catfish organs and muscles offered for sale at Iwo road fish market. The error bars of box plots represent mean and standard deviation.
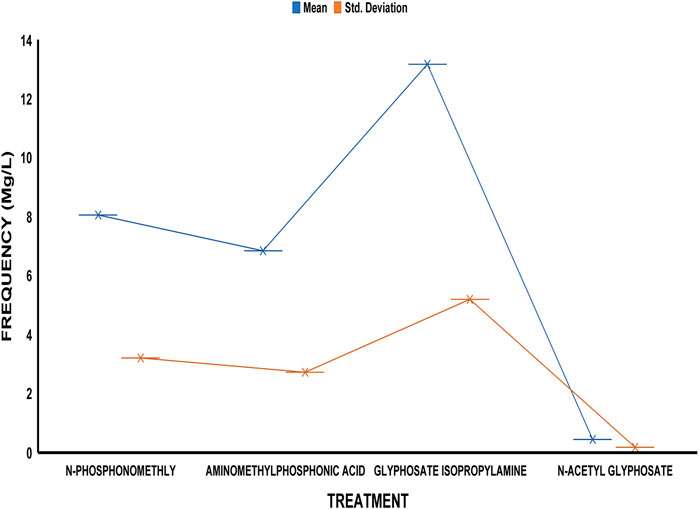
FIGURE 3. Frequency of glyphosate and its metabolites’ residue concentrations present in the Catfish organs and muscles offered for sale at Eleyele fish market. The error bars of box plots represent mean and standard deviation.
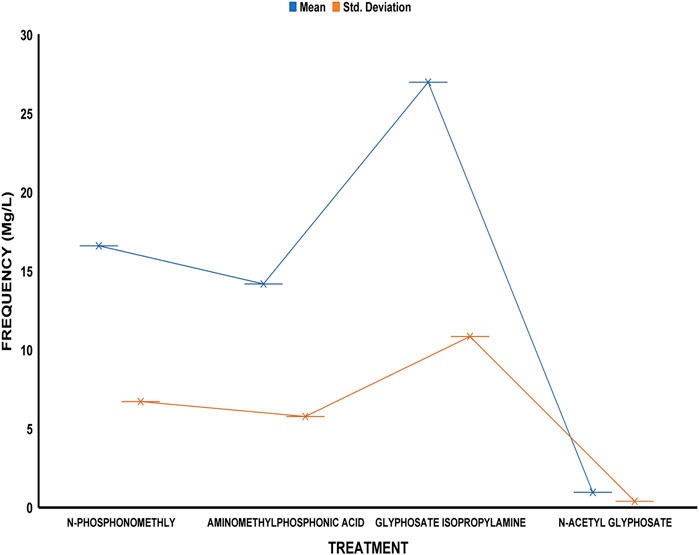
FIGURE 4. Frequency of glyphosate and its metabolites’ residue concentrations present in the Catfish organs and muscles offered for sale at Apata fish market. The error bars of box plots represent mean and standard deviation.
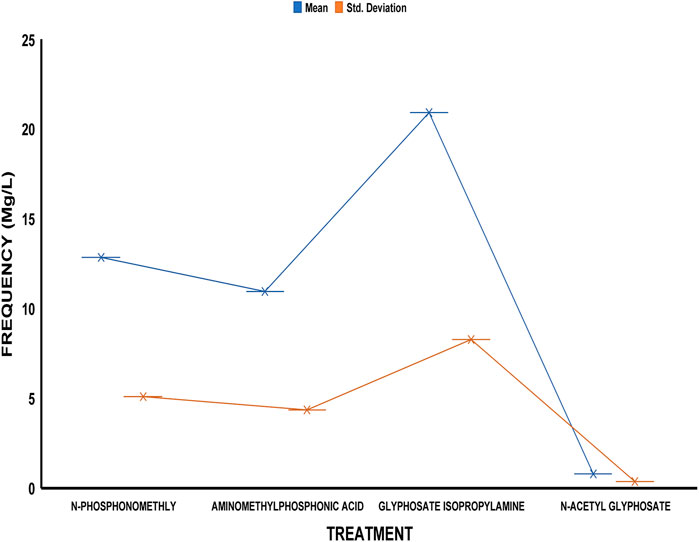
FIGURE 5. Frequency of glyphosate and its metabolites’ residue concentrations present in the Catfish organs and muscles offered for sale at Challenge fish market. The error bars of box plots represent mean and standard deviation.
Isopropylamine has the highest residue concentration followed by N-Phosphonomethyl and Aminomethylphosphonic Acid (AMPA), while N-Acetyl Glyphosate has the least residue concentration in fish tissues obtained across all the fish market (Figures 1–5).
Discussion
Large quantities of glyphosate-based herbicides are applied to crops two to three times per season to get rid of weeds that grow back after herbicides application. Glyphosate residues can remain stable in foods for years, irrespective of the method of food preservation. The health of both humans and animals may be impacted by glyphosate, according to several earlier research. In this study, glyphosate residues were recorded in all the fish samples obtained from the selected fish markets in the Ibadan metropolis which is in agreement with the reports of Kruger et al. (2013). The detection of glyphosate residues in the fish organs and muscles in this study confirmed the use of several glyphosate-based herbicides (Force-Up, Vinash, Para force, and Round-Up) on the fish farms (fish as a non-target organism) in Nigeria (Alarape et al., 2021), use of banned pesticides like pyrethroids, organophosphates, carbamates, and organochlorine in agricultural products in Bangladesh (Jallow et al., 2017) and Ghana (Essumang et al., 2009). Although glyphosate-based herbicides were not used directly on fish, environmental contamination may be a possible source of the herbicide residues found in fish in the research location where such herbicides are used on farms close to fish farms. In addition to demonstrating that glyphosate and its metabolites were present in the fish sold for human consumption, it also demonstrated a definite pattern regarding the degree of chemical contamination in the fish farms’ water supplies. This is supported by a 2009 investigation by Essumang et al. (2009) that found variable concentrations of organochlorine (OC) and organophosphorus (OP) pesticides in the lagoons of Etsii, Fosu, Korle, and Chemu in Ghana.
Glyphosate and several of its metabolites (AMPA, N-acetyl glyphosate, and N-acetyl AMPA) have an acceptable daily intake (ADI) for people of 1.0 mg/kg (1 × 10−3 mg/L) (FAO, 2004; FAO, 2011). The findings of this investigation showed that the residue of glyphosate and its metabolites in the fish samples exceeded recognized safety thresholds. The environmentally relevant concentration of glyphosate is about 0.4 mg/L (Glusczak et al., 2007). Despite this, studies based on actual water runoffs from fields freshly applied with glyphosate formulation have demonstrated glyphosate concentrations as high as 5.2 mg/L (Edwards et al., 1980), whilst simulated studies using sand as a matrix showed water runoffs containing as high as 17 mg/L glyphosate (Fu, 2020). According to Vicini et al. (2021), the permitted concentration in drinking water in Europe is less than 0.1 mg/L, but the maximum residue limit (MRL) as suggested by WHO and FAO [Codex, Alimentarius Commission (2009)] was 0.01 mg/kg (1 × 10−5 mg/L) (EPA, 2015). In this study, all fish samples obtained from the fish markets in Ibadan had glyphosate and its metabolites residue concentration above the recommended MRL. Glyphosate Isopropylamine had the highest residue concentration in all the fish samples analyzed while N-Acetyl Glyphosate had the least residue concentration (Figures 1–5). The highest glyphosate and its metabolites’ residue concentration was recorded throughout the fish muscle samples, followed by the fish kidney while the liver had the least residue concentrations when compared with both muscle and kidney (Supplementary Figures S1–S15).
The findings of Akan et al. (2019) are in contrast to the high glyphosate residue concentrations reported in this study, where the concentrations of herbicide residues recorded were below both the WHO and FAO maximum residue limit (MRL) of 0.01 mg/kg (1 × 10−5 mg/L) and the acceptable daily intake value (ADI) of 0.006 mg/kg (6 × 10−6 mg/L), which is considered safe for consumption as of the time of their present research work. The difference in the findings may be due to differences in vegetation, weather, and rates of usage of herbicides in the Northern part of Nigeria. When animals are fed crops cultivated with glyphosate or indirectly exposed to glyphosate, it is not anticipated that animal products, except kidney and liver due to their physiological functions, will contain appreciable residues of glyphosate. This is because glyphosate has a high water solubility (10.5 g/L), a low octanol-water partition constant (log POW = 3.2), and is quickly excreted through the kidney (Bus, 2015). This assertion is in contrast to the findings in this study because glyphosate residues were detected in the muscles of the fish sample with the highest concentrations.
Conclusion
The increased use of herbicides in agricultural sectors is a result of the world’s rising food demand, but their residues in agricultural products, particularly fish, are raising serious health issues for consumers and public health officials because they are linked to food safety. The presence of a high residue concentration of glyphosate and its metabolites in the muscles of collected fish samples calls for direct and targeted regulations on its use in food animal-based farms.
Despite the advantages of using glyphosate-based herbicides, the results of this study and many others show that these chemicals can have negative effects on the aquatic environment, human health, and even the global food-chain cycle (Abdulkareem et al., 2014). Through the ingestion of feed and contact with the usage of polluted water, glyphosate residue may come into contact with humans and animals. Singh et al. (2020) claim that the extensive use of glyphosate has caused ecosystems to become contaminated, which is negatively affecting microorganisms, plants, animals, and humans. Therefore, this study has established the presence of glyphosate-based herbicide and its metabolites in cultured Clarias gariepinus (African Catfish) offered for sale in fish markets within the Ibadan metropolis.
Data availability statement
The original contributions presented in the study are included in the article/Supplementary Material, further inquiries can be directed to the corresponding author.
Ethics statement
The animal study was approved by the Animal Care and Use Research Ethics Committee, University of Ibadan. The study was conducted in accordance with the local legislation and institutional requirements.
Author contributions
SAA, OKA, and AFF: conceptualization. AAA, ROA, and OAI: data collection. SAA and ROA: formal analysis. SAA, OKA, and AFF: supervision. SAA, AFF, OAI, AAA, and ROA: manuscript preparation and revision. All authors contributed to the article and approved the submitted version.
Conflict of interest
The authors declare that the research was conducted in the absence of any commercial or financial relationships that could be construed as a potential conflict of interest.
The reviewer EO declared a shared affiliation with the authors SAA, OAI, ROA, and OKA to the handling editor at the time of review.
Publisher’s note
All claims expressed in this article are solely those of the authors and do not necessarily represent those of their affiliated organizations, or those of the publisher, the editors and the reviewers. Any product that may be evaluated in this article, or claim that may be made by its manufacturer, is not guaranteed or endorsed by the publisher.
Supplementary material
The Supplementary Material for this article can be found online at: https://www.frontiersin.org/articles/10.3389/ftox.2023.1250137/full#supplementary-material
References
Abdulkareem, S. I., Lawal, N. O., and Moyebi, O. D. (2014). Effect of lethal and sub-lethal concentrations of glyphosate on some biochemical parameters and growth responses of African catfish (Clarias gariepinus). Egypt. Acad. J. Biol. Sci. 6 (2), 47–54. doi:10.21608/eajbsz.2014.13486
Adeyemo, O. K., Alarape, S. A., and Emikpe, B. O. (2011). Reprotoxic effect of malachite green on african catfish Clarias gariepinus (burchell 1822). J. Fish. Aquatic Sci. 6 (5), 563–570. doi:10.3923/jfas.2011.563.570
Akan, J. C., Mohammed, Z., and Jafiya, L. (2019). Organochlorine pesticide residues in fish samples from alau dam, borno state, north eastern Nigeria. J. Environ. Anal. Toxicol. 3, 171. doi:10.4172/2161-0525.1000171
Alarape, S. A., Adebiyi, O. E., and Adeyemo, O. K. (2021). Histopathological effects and micronucleus assay of glyphosate-based herbicides on cultured african catfish (Clarias gariepinus, burchell 1822).
Alarape, S. A., Ajani, F., Adeyemo, O. K., and Shobiye, J. O. (2013). Effect of copper sulphate on spawning success in african catfish (Clarias gariepinus, burchell 1822). J. Fish. Aquatic Sci. 8 (6), 714–720. doi:10.3923/jfas.2013.714.720
Antunes, A. M., Rocha, T. L., Pires, F. S., de Freitas, M. A., Leite, V. R. M. C., Arana, S., et al. (2017). Gender-specific histopathological response in guppies Poecilia reticulata exposed to glyphosate or its metabolite Aminomethylphosphonic acid. J. Appl. Toxicol. 37, 1098–1107. doi:10.1002/jat.3461
Banks, M. L., Kennedy, A. C., Kremer, R. J., and Eivazi, F. (2014). Soil microbial community response to surfactants and herbicides in two soils. Appl. Soil Ecol. 74, 12–20. doi:10.1016/j.apsoil.2013.08.018
Bastos, G. B., Cardoso, G. P., dos Santos, S. D., de Melo e Silva, N. C., Alves de Lima, A., Antonio, B. D. A., et al. (2020). Ecotoxicology of glyphosate-based herbicides on aquatic environment. IntechOpen. doi:10.5772/intechopen.85157
Battaglin, W. A., Meyer, M. T., Kuivila, K. M., and Dietze, J. E. (2014). Glyphosate and its degradation product AMPA occur frequently and widely in U.S. soils, surface water, groundwater, and precipitation. J. Am. Water Resour. Assoc. 50, 275–290. doi:10.1111/jawr.12159
Bergström, L., Börjesson, E., and Stenström, J. (2011). Laboratory and lysimeter studies of glyphosate and aminomethyl phosphonic acid in a sand and a clay soil. J. Environ. Qual. 40, 98–108. doi:10.2134/jeq2010.0179
Birch, H., Mikkelson, P. S., Jenson, J. K., and Lützhøft, H. C. (2011). Micropollutants in stormwater runoff and combined sewer overflow in the Copenhagen area, Denmark. Water Sci. Technol. 64, 485–493. doi:10.2166/wst.2011.687
Bus, J. S. (2015). Analysis of Moms across America report suggesting bioaccumulation of glyphosate in U.S. mother's breast milk: implausibility based on inconsistency with available body of glyphosate animal toxicokinetic, human biomonitoring, and physico-chemical data. Regul. Toxicol. Pharmacol. 73, 758–764. doi:10.1016/j.yrtph.2015.10.022
Cassigneul, A., Benoit, P., Bergheaud, V., Dumeny, V., Etiévant, V., Goubard, Y., et al. (2016). Fate of glyphosate and degradates in cover crop residues and underlying soil: a laboratory study. Sci. Tot. Environ. 545–546, 582–590. doi:10.1016/j.scitotenv.2015.12.052
Chang, F. C., Simcik, M. F., and Capel, P. (2011). Occurrence and fate of the herbicide glyphosate and its degradate aminomethylphosphonic acid in the atmosphere. Environ. Toxicol. Chem. 30, 548–555. doi:10.1002/etc.431
Codex Alimentarius Commission (2009). “Pesticide residue in food and feed,” in Extraneous maximum residue limits (Westport, Connecticut: Greenwood Publishing group).
Coupe, R. H., Kalkhoff, S. K., Capel, P. D., and Gregoire, C. (2011). Fate and transport of glyphosate and aminomethylphosphonic acid in surface waters of agricultural basins. Pest Manag. Sci. 68, 16–30. doi:10.1002/ps.2212
de Brito Rodrigues, L., Costa, G. G., Thá, E. L., da Silva, L. R., de Oliveira, R., Leme, D. M., et al. (2019). Impact of the glyphosate-based commercial herbicide, its components and its metabolite AMPA on non-target aquatic organisms. Mut. Res.-Genet. Toxicol. Environ. Mut. 842, 94–101. doi:10.1016/j.mrgentox.2019.05.002
Delmonico, E. L., Bertozzi, J., de Souza, N. E., and Oliveira, C. C. (2014). Determinação de glifosato e ácido aminometilfosfônico para erifcar a qualidade da água de abastecimento público utilizando EFS e CLAE. Acta. Sci. Technol. 36, 513–519. doi:10.4025/actascitechnol.v36i3.22406
Edwards, W. M., Triplett, G. B., and Kramer, R. M. (1980). A watershed study of glyphosate transport in runoff. J. Environ. Qual. 9, 661–665. doi:10.2134/jeq1980.00472425000900040024x
EFSA (2015). Peer review of the pesticide risk assessment of the potential endocrine disrupting properties of glyphosate. EFSA J. 13 (11), e04979. doi:10.2903/j.efsa.2017.4979
EPA (2015). Electronic code of federal regulations, title 40: protection of environment. PART 180-tolerances and exemptions for pesticide chemical residues in food. Subpart C-specific tolerances. Washington, DC: Environmental Protection Agency. Available at: http://www.ecfr.gov/cgi-bin/text-idx?tpl=/ecfrbrowse/Title40/40cfr180_main_02.tpl (Accessed date September 29, 2021).
Essumang, D. K., Togoh, G. K., and Chokky, L. (2009). Pesticide residues in the water and fish (lagoon tilapia) samples from lagoons in Ghana. Bull. Chem. Soc. Ethiop. 23 (1), 19–27. doi:10.4314/bcse.v23i1.21294
FAO (2004). Pesticide residues in food: toxicological evaluations; international programme on chemical safety. Rome, Italy: World Health Organization Food and Agriculture Organization.
FAO (2011). Pesticide residues in food: toxicological evaluations; international programme on chemical safety. Geneva, Switzerland: World Health Organization Food and Agriculture Organization, 373–385. doi:10.1016/j.bbrc.2019.04.074
Fu, W. (2020). A model experiment to investigate the possibility of glyphosate contamination in st. Kitts and nevis. Master’s Thesis. Orlando, FL, USA: University of Central Florida.
Gaur, H., and Bhargava, A. (2019). Glyphosate induces toxicity and modulates calcium and NO signaling in zebrafish embryos. Biochem. Bioph. Res. Comm. 513, 1070–1075. doi:10.1016/j.bbrc.2019.04.074
Glusczak, L., dos Santos Miron, D., Moraes, B. S., Simões, R. R., Schetinger, M. R. C., Morsch, V. M., et al. (2007). Acute effects of glyphosate herbicide on metabolic and enzymatic parameters of silver catfish (Rhamdia quelen). Biochem. Physiol. Part C Pharmacol. Toxicol. Endocrinol. 146, 519–524. doi:10.1016/j.cbpc.2007.06.004
Grandcoin, A., Piel, S., and Baurès, E. (2017). AminoMethylPhosphonic acid (AMPA) in natural waters: its sources, behavior and environmental fate. Water Res. 117, 187–197. doi:10.1016/j.watres.2017.03.055
Guilherme, S., Santos, M., Barroso, C., Gaivão, I., and Mário, P. (2012). Differential genotoxicity of Roundup® formulation and its constituents in blood cells of fish (Anguilla anguilla): considerations on chemical interactions and DNA damaging mechanisms. Ecotoxicology 21, 1381–1390. doi:10.1007/s10646-012-0892-5
Guyton, K. Z., Loomis, D., Grosse, Y., El Ghissassi, F., Benbrahim-Tallaa, L., Guha, N., et al. (2015). Carcinogenicity of tetrachlorvinphos, parathion, malathion, diazinon, and glyphosate. Lancet Oncol. 16 (5), 490–491. doi:10.1016/S1470-2045(15)70134-8
Hanke, I., Wittmer, I., Bischofberger, S., Stamm, S., and Singer, H. (2010). Relevance of urban glyphosate use for surface water quality. Chemosphere 81, 422–429. doi:10.1016/j.chemosphere.2010.06.067
IARC (2015). Evaluation of five organophosphate insecticides and herbicides. Lyon, France: World Health Organization, International Agency for Research on Cancer. http://www.iarc.fr/en/media-centre/iarcnews/pdf/MonographVolume112.pdf (Accessed date September 29, 2021).
Jallow, M. F. A., Awadh, D. G., Albaho, M. S., Devi, V. Y., and Thomas, B. M. (2017). Pesticide Knowledge and safety practices among farm workers in Kuwait: results of a survey. Int. J. Environ. Res. Publ. Health 14, 340. doi:10.3390/ijerph14040340
Krüger, M., Shehata, A. A., Schrödl, W., and Rodloff, A. (2013). Glyphosate suppresses the antagonistic effect of Enterococcus spp. on Clostridium botulinum. Anaerobe 20, 74–78. doi:10.1016/j.anaerobe.2013.01.005
Li, M. H., Ruan, L. Y., Zhou, J. W., Fu, Y. H., Jiang, L., Zhao, H., et al. (2017). Metabolic profiling of goldfish (Carassius auratis) after long-term glyphosate-based herbicide exposure. Aquat. Toxicol. 188, 159–169. doi:10.1016/j.aquatox.2017.05.004
Ma, J., Zhu, J., Wang, W., Ruan, P., Rajeshkumar, S., and Li, X. (2019). Biochemical and molecular impacts of glyphosate-based herbicide on the gills of common carp. Environm. Pollut. 252, 1288–1300. doi:10.1016/j.envpol.2019.06.040
Mahler, B. J., Van Metre, P. C., Burley, T. E., Loftin, K. A., Meyer, M. T., and Nowell, L. H. (2017). Similarities and differences in occurrence and temporal fluctuations in glyphosate and atrazine in small Midwestern streams (USA) during the 2013 growing season. Sci. Total Environ. 579, 149–158. doi:10.1016/j.scitotenv.2016.10.236
Maqueda, C., Undabeytia, T., Villaverde, J., and Morillo, E. (2017). Behaviour of glyphosate in a reservoir and the surrounding agricultural soils. Sci. Total Environ. 593–594, 787–795. doi:10.1016/j.scitotenv.2017.03.202
McNaughton, J., Roberts, M., Rice, D., Smith, B., Hinds, M., Delaney, B., et al. (2011). Evaluation of broiler performance and carcass yields when fed diets containing corn grain from transgenic stacked-trait product DAS-Ø15Ø7-1xDAS-59122-7xMON-ØØ81Ø-6xMON-ØØ6Ø3-6. J. Appl. Poult. Res. 20, 542–553. doi:10.3382/japr.2011-00367
Menéndez-Helman, R. J., Ferreyroa, G. V., dos Santos Alfonso, M., and Salibrán, A. (2012). Glyphosate as an acetylcholinesterase inhibitor in Cnesterodon decemmaculatus. Contam. Toxicol. 88, 6–9. doi:10.1007/s00128-011-0423-8
Mercurio, P., Flores, F., Mueller, J. F., Carter, S., and Negri, A. P. (2014). Glyphosate persistence in seawater. Mar. Pollut. Bull. 85, 385–390. doi:10.1016/j.marpolbul.2014.01.021
Modesto, K. A., and Martinez, C. B. R. (2010). Roundup causes oxidative stress in liver and inhibits acetylcholinesterase in muscle and brain of the fish Prochilodus lineatus. Chemosphere 78, 294–299. doi:10.1016/j.chemosphere.2009.10.047
Mörtl, M., Németh, G., Juracsek, J., Darvas, B., Kamp, L., Rubio, F., et al. (2013). Determination of glyphosate residues in Hungarian water samples by immunoassay. Microchem. J. 107, 143–151. doi:10.1016/j.microc.2012.05.021
Muhammad, U. A., Yasid, N. A., Daud, H. M., and Shukor, M. Y. (2021). Glyphosate herbicide induces changes in the growth pattern and somatic indices of crossbred red Tilapia (O. niloticus × O. mossambicus). Anim. (Basel) 11 (5), 1209. doi:10.3390/ani11051209
Okada, E., Luis Costa, J., and Bedmar, F. (2016). Adsorption and mobility of glyphosate in different soils under no-till and conventional tillage. Geoderma 263, 78–85. doi:10.1016/j.geoderma.2015.09.009
Poiger, T., Buerge, I. J., Bächli, A., Müller, M. D., and Balmer, M. E. (2017). Occurrence of the herbicide glyphosate and its metabolite AMPA in surface waters in Switzerland determined with on-line solid phase extraction LC-MS/MS. Environ. Sci. Poll. Res. 24, 1588–1596. doi:10.1007/s11356-016-7835-2
Rodriguez-Gil, J. L., Prosser, R., Poirier, D., Lissemore, L., Thompson, D., Hanson, M., et al. (2017). Aquatic hazard assessment of Mon 0818, a commercial mixture of alkylamine ethoxylates commonly used in glyphosate-containing herbicide formulations. Part 1: species sensitivity distribution from laboratory acute exposures. Environ. Toxicol. Chem. 36, 501–511. doi:10.1002/etc.3559
Rosenbom, A. E., Brüsch, W., Juhler, R. K., Ernstsen, V., Gudmundsson, L., Kjær, J., et al. (2010). The Danish pesticide leaching assessment programme monitoring results may 1999–june 2009. Copenhagen, Denmark: Geological Survey of Denmark and Green land Ministry of Climate and Energy and Faculty of Agricultural Sciences.
Roy, N. M., Carneiro, B., and Och, J. (2016). Glyphosate induces neurotoxicity in zebrafish. Environ. Toxicol. Pharmacol. 42, 45–54. doi:10.1016/j.etap.2016.01.003
Sanchis, J., Kantiani, L., Llorca, M., Rubio, F., Ginebreda, A., Fraile, J., et al. (2012). Determination of glyphosate in groundwater samples using an ultrasensitive immunoassay and confirmation by on-line solid-phase extraction followed by liquid chromatography coupled to tandem mass spectrometry. Anal. Bioanal. Chem. 402, 2335–2345. doi:10.1007/s00216-011-5541-y
Sandrini, J. Z., Rola, R. C., Lopes, F. M., Buffon, H. F., Freitas, H. F., Freita, M. M., et al. (2013). Effects of glyphosate on cholinesterase activity of the mussel Perna perna and the fish Danio rerio and Jenynsia multidentata: in vitro studies. Aquat. Toxicol. 130-131, 171–173. doi:10.1016/j.aquatox.2013.01.006
Séralini, G. E., Clair, E., Mesnage, R., Gress, S., Defarge, N., Malatesta, M., et al. (2014). Republished study: long-termtoxicity of a roundup herbicide and a Roundup-tolerant genetically modified maize. Environ. Sci. Eur. 26, 14. doi:10.1186/s12302-014-0014-5
Shehata, A. A., Schrodi, W., Schledorn, P., and Krueger, M. (2014). Distribution of glyphosate in chicken organs and its reduction by humic acid supplementation. J. Poult. Sci. 51, 333–337. doi:10.2141/jpsa.0130169
Sidoli, P., Baran, N., and Angulo-Jaramillo, R. (2016). Glyphosate and AMPA adsorption in soils: laboratory experiments and pedotransfer rules. Environ. Sci. Pollut. Res. 23, 5733–5742. doi:10.1007/s11356-015-5796-5
Sihtmäe, M., Blinova, I., Kunnis-Beres, K., Kanarbik, L., Heinlaan, M., and Kahru, A. (2013). Ecotoxicological effects of different glyphosate formulations. Appl. Soil Ecol. 72, 215–224. doi:10.1016/j.apsoil.2013.07.005
Singh, S., Kumar, V., Gill, J. P. K., Dalta, S., Singh, S., Dhaka, V., et al. (2020). Herbicide glyphosate: toxicity and microbial degradation. Int. J. Environ. Res. Public Health 17, 7519. doi:10.3390/ijerph17207519
Skark, C., Zullei-Seibert, N., Schottler, U., and Schlett, C. (1998). The occurrence of glyphosate in surface water. Int. J. Environ. Anal. Chem. 70, 93–104. doi:10.1080/03067319808032607
Sviridov, A. V., Shushkova, T. V., Ermakova, I. T., Ivanova, E. V., Epiktetov, D. O., and Leontievsky, A. A. (2015). Microbial degradation of glyphosate herbicides (review). Appl. Biochem. Microbiol. 51, 183–190. doi:10.7868/s0555109915020221
Travaglia, C., Masciarelli, O., Fortuna, J., Marchetti, G., Cardozo, P., Lucero, M., et al. (2015). Towards sustainable maize production: glyphosate detoxification by Azospirillum sp. and Pseudomonas sp. Crop. Prot. 77, 102–109. doi:10.1016/j.cropro.2015.07.003
Vicini, J. L., Jensen, P. K., Young, B. M., and Swarthout, J. T. (2021). Residues of glyphosate in food and dietary exposure. Compr. Rev. Food Sci. Food Saf. 20 (5), 5226–5257. doi:10.1111/1541-4337.12822
Villeneuve, A., Larroudé, S., and Humbert, J. F. (2011). “Herbicide contamination of freshwater ecosystems: impact on microbial communities,” in Pesticides - formulations. Editor M. Stoytcheva (Fate: Effects), 285–312.
WHO (2005). Glyphosate and AMPA in drinking-water: 2005. Available at: http://www.who.int/water_sanitation_health/dwq/chemicals/glyphosateampa290605.pdf (Accessed date September 17, 2021).
Zhang, C., Hu, X., Luo, J., Wu, Z., Wang, L., Li, B., et al. (2015b). Degradation dynamics of glyphosate in different types of citrus orchard soils in China. Molecules 20, 1161–1175. doi:10.3390/molecules20011161
Keywords: glyphosate, Clarias gariepinus, liquid chromatography, residue, metabolites, herbicides
Citation: Alarape SA, Fagbohun AF, Ipadeola OA, Adeigbo AA, Adesola RO and Adeyemo OK (2023) Assessment of glyphosate and its metabolites’ residue concentrations in cultured African Catfish offered for sale in selected markets in Ibadan, Oyo State, Nigeria. Front. Toxicol. 5:1250137. doi: 10.3389/ftox.2023.1250137
Received: 29 June 2023; Accepted: 03 October 2023;
Published: 02 November 2023.
Edited by:
Azubuike Chukwuka, National Environmental Standards and Regulations Enforcement Agency (NESREA), NigeriaReviewed by:
Nosakhare Osazee Erhunmwunse, University of Benin, NigeriaPramita Sharma, University of Burdwan, India
Emmanuel Omogbemi, University of Ibadan, Nigeria
Copyright © 2023 Alarape, Fagbohun, Ipadeola, Adeigbo, Adesola and Adeyemo. This is an open-access article distributed under the terms of the Creative Commons Attribution License (CC BY). The use, distribution or reproduction in other forums is permitted, provided the original author(s) and the copyright owner(s) are credited and that the original publication in this journal is cited, in accordance with accepted academic practice. No use, distribution or reproduction is permitted which does not comply with these terms.
*Correspondence: Selim Adewale Alarape, bGluazJzYXNAeWFob28uY28udWs=