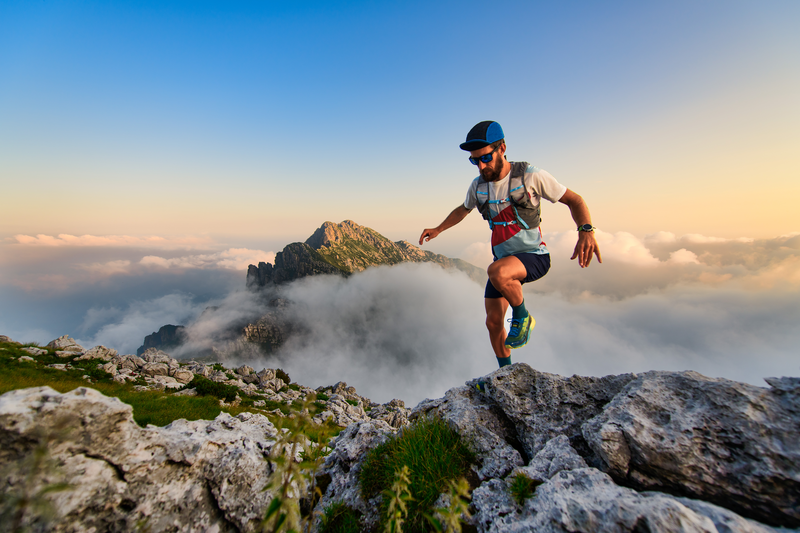
94% of researchers rate our articles as excellent or good
Learn more about the work of our research integrity team to safeguard the quality of each article we publish.
Find out more
REVIEW article
Front. Toxicol. , 17 May 2023
Sec. Developmental and Reproductive Toxicology
Volume 5 - 2023 | https://doi.org/10.3389/ftox.2023.1189303
This article is part of the Research Topic European Partnership on the Assessment of Risks from Chemicals (PARC): Focus on New Approach Methodologies (NAMs) in Risk Assessment View all 10 articles
Current test strategies to identify thyroid hormone (TH) system disruptors are inadequate for conducting robust chemical risk assessment required for regulation. The tests rely heavily on histopathological changes in rodent thyroid glands or measuring changes in systemic TH levels, but they lack specific new approach methodologies (NAMs) that can adequately detect TH-mediated effects. Such alternative test methods are needed to infer a causal relationship between molecular initiating events and adverse outcomes such as perturbed brain development. Although some NAMs that are relevant for TH system disruption are available–and are currently in the process of regulatory validation–there is still a need to develop more extensive alternative test batteries to cover the range of potential key events along the causal pathway between initial chemical disruption and adverse outcomes in humans. This project, funded under the Partnership for the Assessment of Risk from Chemicals (PARC) initiative, aims to facilitate the development of NAMs that are specific for TH system disruption by characterizing in vivo mechanisms of action that can be targeted by in embryo/in vitro/in silico/in chemico testing strategies. We will develop and improve human-relevant in vitro test systems to capture effects on important areas of the TH system. Furthermore, we will elaborate on important species differences in TH system disruption by incorporating non-mammalian vertebrate test species alongside classical laboratory rat species and human-derived in vitro assays.
Within current European regulatory testing frameworks, chemical compounds that can cause adverse effects in vivo through thyroid hormone (TH) system disruption are primarily assessed in laboratory rodents. Assessments typically focus on gross effects on the thyroid gland itself, including changes in weight or histopathology, or by measuring levels of circulating THs (ECHA/EFSA, 2018). Although these measurements are informative, they are inadequate when it comes to identifying the broad suite of potential TH system disruptors (Noyes et al., 2019; Couderq et al., 2020; Kortenkamp et al., 2020). For instance, it is recognized that many potential TH system disruptors can act via a number of different mechanisms that are not detected by analyzing the thyroid gland itself, nor by measuring systemic levels of the THs triiodothyronine (T3) and thyroxine (T4) (Noyes et al., 2019; Gilbert et al., 2020). This is because changes in, for instance, deiodinase activity or membrane transport of THs, will not necessarily affect circulating levels of TH, but could still have a large impact on TH availability and action in target tissues (Landers and Richard, 2017; Gilbert et al., 2020; Köhrle and Frädrich, 2022). Lastly, TH-mediated adverse effects in target tissues peripheral to the thyroid gland are not addressed by current test methods, including TH-mediated developmental neurotoxicity.
By early 2023, more than 22,000 unique industrial chemicals were registered under the European REACH regulation (Registration, Evaluation, Authorisation and restriction of Chemicals) (Directorate-General for Environment, 2023). The REACH standard information requirements are based on the tonnage level of combined import and production per registrant, but the same chemical can be imported or produced by several different registrants. Based on today’s information requirements, this means that in vivo tests that aim to determine whether chemicals possess TH system disrupting properties in mammals are not performed for chemicals at the 1–10 tonnes/year level (per registrant). From 10 tonnes and above, endpoints and biomarkers such as TH concentrations and thyroid histopathology are included in some rodent tests but, as already discussed, these are insufficient in identifying all TH system disruptors. Although pesticides and biocides (under the plant protection products or biocidal products regulations) are subject to much stronger testing requirements despite low tonnage levels, the tests, biomarkers, and endpoints are the same as for REACH registered chemicals. There is thus a pressing need to establish additional measurable endpoints or biomarkers for testing purposes including new approach methods.
Decades of research into the hypothalamic-pituitary-thyroid (HPT) axis, and the biological functions of the THs, has identified a complex network of endocrine regulation and highlighted multiple entry points for possible disruption by chemical substances. These include TH synthesis, transport and receptor binding, as well as local tissue uptake and metabolism (Figure 1) (OECD, 2014; Noyes et al., 2019; Gilbert et al., 2020). The potential molecular initiating events (MIEs) for TH system disruptors can be tested for by various new approach methodologies (NAMs), including in silico approaches, in chemico and in vitro assays as well as information from chemical exposure for hazard assessment (European Chemicals Agency, 2016). Indeed, a number of newly developed NAMs have already shown great promise for future implementation in international test guideline programmes and hence are currently in the process of regulatory validation within the framework of the Organization for Economic Co-operation and Development (OECD) (Joint Research Centre, 2023). However, even with these new additions to a broader testing battery of methods relevant for assessing TH system disruption, not all critical steps between a MIE and an adverse outcome (AO) in adverse outcome pathways (AOPs) are covered.
FIGURE 1. The thyroid hormone (TH) system and tests to assess chemical effects that may lead to altered TH-signaling and adverse effects. The TH system has many components and molecules that can be targeted by environmental chemicals in one and/or all tissues. Targets can be grouped into classes according to the type of assay that can be used to test for potential effects of chemicals, e.g., by developing enzyme activity assays where each assay tests the activity of one enzyme, TPO, DIO1, DIO2, DEHAL1 etc. In vivo, each interaction may lead to altered TH-signaling with potential adverse effects on development and physiology. Abbreviations: DEHAL1: iodothyrosine dehalogenase, DIO: deiodinase, HPT: hypothalamic-pituitary-thyroid, MCT: monocarboxylate transporter, NIS: Sodium/Iodide Symporter, OATPs: organic-anion-transporting polypeptides, rT3: reverse T3, RXR: retinoid X receptor, SULTs: sulfotransferases, T2: 3,5-diiodo-L-thyronine, T3: triiodothyronine, T4: thyroxine, TBG: thyroxine-binding globulin, Tg: thyroglobulin, TH: thyroid hormone, TPO: thyroperoxidase, TR: thyroid receptor, TRE: thyroid response element, TRH: thyrotropin releasing hormone, TSH: thyroid stimulating hormone, TSHR: thyroid stimulating hormone receptor, TTR: transthyretin, UGTs: Uridine 5′-diphospho-glucuronosyltransferases.
A major challenge in developing new NAMs for TH system disruption is the lack of fundamental biological knowledge of key aspects of TH-mediated developmental processes. Thus, current efforts aimed at improving our capacity to safeguard human and ecological health from TH system disrupting chemicals should include efforts to better understand the complex biology of toxicological effects in vivo. And these efforts should leverage the knowledge we already possess to devise a broad NAM-based screening battery capable of identifying key events (KEs) of TH-mediated causal pathways. In this project, that we are performing under the European Union (EU)-funded Partnership for the Assessment of Risk from Chemicals (PARC, 2023) programme (Marx-Stoelting et al., 2023; PARC), we aim to provide both novel biological knowledge of how THs may regulate development and how TH system disruptors can perturb these processes, in order to establish and develop NAMs to be incorporated in regulatory decision making for the identification of TH system disruptors.
THs are involved in the differentiation, growth, and function of virtually all vertebrate tissues and organs (Yen, 2001). They play distinct roles throughout life and exert specific actions in a spatiotemporal manner through both systemic and local regulation of TH signaling. This is achieved by a fine-tuned regulation of an intricate network of TH synthesis, feedback mechanisms, serum distribution, membrane transporters, metabolizing enzymes, receptors and more (Zoeller et al., 2007; Ortiga-Carvalho et al., 2016). The different components all work in concert to deliver THs to target cells where they regulate gene expression through interactions with nuclear TH receptors (TRs) or through non-genomic mechanisms (Ortiga-Carvalho et al., 2016; Taylor and Heyland, 2022).
TH action is critical for the development of the central nervous system where THs help regulate crucial events such as neuronal differentiation, migration, synaptogenesis and myelination. Deficiency in THs during these events can thus have profound and irreversible effects on the developing brain. Worryingly, many environmental chemicals have been shown to act as TH system disruptors and can thus potentially affect brain development with significant consequences for life-long cognition (Gilbert et al., 2020; Marty et al., 2022). In this context, it is also important to consider the regulatory networks that finetune TH action in complex organisms. For instance, THs can act directly on the developing liver and control processes such as lipid metabolism, but the liver itself may also modify systemic TH regulation. Deciphering these mechanisms of effects and function will be critical if we are to faithfully capture potential TH system disrupting chemicals by use of alternative test method batteries.
The developing mammalian fetus does not synthesize THs during early stages of gestation and is therefore dependent on maternal supply at critical stages of neuronal development (Figure 2). In humans, the fetal thyroid gland starts producing THs by the second trimester, but maternal supply continues to be important throughout gestation until birth (Morreale de Escobar et al., 2004). Consequently, fetal brain development is dependent on maternal thyroid function, something that is evident from numerous epidemiological studies linking low maternal T4 levels to child neurodevelopmental effects (Henrichs et al., 2010; Román et al., 2013; Ghassabian et al., 2014; Modesto et al., 2015; Gyllenberg et al., 2016; Korevaar et al., 2016). From the second trimester of pregnancy, a correct iodine supply from the mother to the fetus is also essential, so that the fetus can produce its own THs (De La Vieja and Santisteban, 2018). Therefore, amongst other things, the presence of membrane transporters of both iodide and THs in the placenta throughout pregnancy is essential to maintain the correct balance of mother-fetus TH levels.
FIGURE 2. The thyroid hormone system and fetal development. Maternal (A) and fetal (B) TH levels during human pregnancy. In this period the requirement for iodine in the mother’s diet increases considerably, as does the net demand for TH production by the maternal thyroid gland. Before the onset of fetal thyroid function the fetus is critically dependent on TH transferred from the maternal to the fetal circulation. Timing of ontogeny (C), function and autoregulation (D) of the fetal thyroid gland during pregnancy. (E) Maternal TH fully supply fetal requirements until fetal TH secretion begin at around 15 weeks of gestation after which fetal TH levels come from both the maternal and the fetal thyroid gland. (F) Timing of some TH mediated neurodevelopmental processes in the fetal brain. Early neuronal proliferation and migration is dependent on maternal TH in the first trimester of pregnancy. Also in the first trimester, inactivating type 3 deiodinase (DIO3) enzyme expression drops and development of the thyroid gland begins. In the second and third trimesters, the brain continues to develop, now increasingly relying on T4 produced by both the fetus and the mother. As the fetal hypothalamic-pituitary axis develops, a surge in thyroid-stimulating hormone (TSH) secretion occurs, initiating fetal TH production, expression of the activating enzyme deiodinase type 2 (DIO2), and increasing thyroid hormone nuclear receptors occupancy by T3. DIO: deiodinase, hCG: human chorionic gonadotropin, T3: triiodothyronine, T4: thyroxine, TBG: thyroxine-binding globulin, TH: thyroid hormone, TRH: thyrotropin releasing hormone, TSH: thyroid stimulating hormone, (Burrow et al., 1994; Howdeshell, 2002; López-Márquez et al., 2021).
During pregnancy, both total and free T4 levels increase during the first trimester to reach a steady state that is maintained for the remainder of the gestational period. Although these absolute changes to TH levels are relatively small, there is a net loss of THs and an increase in hormone production by the thyroid gland. This is because there is a net increase in plasma volume as the fetus grows, so that more TH must be produced to keep steady state conditions, but also due to an increase in renal excretion of iodine that potentially reduces available iodine in the thyroid gland. Furthermore, during the first trimester, human chorionic gonadotropin (hCG) stimulates TH production in the thyroid gland and the presence of estrogen causes an increase in thyroxine-binding globulin (TBG). Later in gestation there are also changes to peripheral TH metabolism (Howdeshell, 2002; Morreale de Escobar et al., 2004). Combined, all these factors increase the net demand for TH synthesis in the thyroid gland; demands that can be met by a healthy and robust TH system, but not necessarily by a compromised TH system.
Current chemical legislation in the EU mandate the testing of potential endocrine disrupting properties for pesticides, biocides and REACH-regulated chemicals produced at high tonnage levels. These assessments typically include evaluation of estrogenic, androgenic, thyroid, and steroidogenesis (EATS) modalities using various test assays and animal toxicity studies in a tiered process (ECHA/EFSA, 2018; OECD, 2018). Of the five tiers, the first two can be performed animal-free, whereas the last three tiers require animal testing. In many ways, the EATS modalities have, to a large extent, defined the types of assays that are used to test for endocrine disruption. As already discussed, a challenge with respect to the thyroid (T) modality is that current OECD test methods remain inadequate when it comes to identifying TH system disruption and its adverse effects on the developing organism (Gilbert et al., 2020; Kortenkamp et al., 2020); not only because we do not have an adequate battery of NAMs to capture all potential MIEs and KEs, and lack sensitive in vivo tests for TH-mediated adversity, but also because current regulation requires in vivo evidence to support indicative NAMs data. Notably, the REACH §44 (1) risk concept mentions explicitly structural similarity and the relevance of transformation products, thus pointing to in silico (e.g., read-across, ligand-receptor docking) and in chemico (compound transformation to active or inactive metabolites) approaches to support compound evaluation, but the regulatory frameworks still have a way to go in incorporating non-animal test data for chemical regulation.
TH system disruption has been identified as one of the priority areas for AOP and integrated approaches for testing and assessment (IATA) development within PARC. In this context, there is close interaction with the work on AOP development for endocrine and metabolic disruption (PARC Task 5.3.2) and the development of IATAs for endocrine disruption (PARC Task 6.1.1). All experimental data on TH system disruption that are generated in PARC are being mapped to the AOP network, either for improving or expanding existing AOPs, or for the development of new AOPs. The PARC endocrine disruption AOP and IATA development processes are closely aligned to ensure that the methods that are considered for inclusion in the IATAs are supported by KE descriptions of well-developed AOPs. The development of TH system disruption IATAs for both human and environmental health has been initiated, and since these IATAs are based on the conservation of pathways across vertebrates, envisioned to share a number of early KE, the work on assay development and cross-species comparison and extrapolation will be highly relevant to support these activities.
At time of writing this report, there are around 30 AOP pages for TH system disruption already available in the AOP-Wiki, together including 50 linear AOPs. A limited number of AOPs have been developed to the level where they have been endorsed by the OECD Working Party on Hazard Assessment and the Working Group of National Coordinators of the Test Guidelines Programme (WPHA/WNT). This includes the AOP initiated by inhibition of thyroperoxidase (TPO) and leading to adverse neurodevelopmental outcomes in mammals (Crofton et al., 2019) and the AOP leading from inhibition of the Na+/I- symporter (NIS) to learning and memory impairment in mammals (Rolaki et al., 2019). Five AOPs linking TPO and deiodinase inhibition to impaired swim bladder inflation in fish have recently been endorsed (Vergauwen et al., 2022a; Vergauwen et al., 2022b; Vergauwen et al., 2022c; Vergauwen et al., 2022d; Vergauwen et al., 2022e). A multitude of other AOPs describing TH system disruption with applicability to different taxa are in varying stages of development. When combining these AOPs in one large AOP network, a cross-species AOP network emerges (Knapen et al., 2018; Noyes et al., 2019; Knapen et al., 2020).
As a first step in using this AOP network to support cross-species extrapolation and sharing of knowledge between human and environmental health, the taxonomic domain of applicability of the MIEs and the AOs in the network was investigated in the context of altered TH levels. This effort, which was part of the Horizon 2020 ERGO project (Holbech et al., 2020), was based on both an analysis of target conservation (for the MIEs) using the US Environmental Protection Agency’s Sequence Alignment to Predict Across Species Susceptibility (SeqAPASS) tool, and empirical evidence from the literature for the MIEs and AOs. While most AOPs typically are initially developed for one species or taxonomic group, evidence was found that multiple molecular initiating events and adverse outcomes are in fact applicable across different vertebrate taxa. This analysis provides a basis for developing case studies to investigate responses to TH system disrupting chemicals across species, and the development of approaches for AOP network-based cross-species extrapolation.
Despite previous activities at the OECD level and in various EU initiatives on the identification, development and evaluation of in vitro methods addressing the thyroid hormone system (OECD, 2014), we still lack key knowledge about the many MIEs and downstream KEs that can lead to TH-mediated AOs. To address this challenge, this specific project under the PARC programme, aims to provide new mechanistic knowledge that will facilitate NAM development (Figure 3). Specifically, we will address key characteristics of TH system disruption such as TH system regulation and specific points of vulnerability to chemical disruption. This will include direct effects on the developing brain by diminished TH levels, but also effects mediated through other targets such as the liver. Thus, direct versus indirect perturbation of the TH axis by exogenous chemical substances will be scrutinized by use of sophisticated NAMs that build on, for instance, human stem cell-based in vitro assays. We will develop and mature in vitro and in silico tests to capture effects on key components of the TH system, such as TH transport across physiologic barriers. Importantly, we will include the characterization of evolutionary conservation of the TH system axis between rats and humans, but also mammalian and non-mammalian vertebrates (e.g., fish and amphibians) and conserved elements of the thyroid-like system that exist in some invertebrate phyla (e.g., mollusks) to allow for additional NAMs that can inform on human health hazards. The project will focus on the following three areas and align activities with the AOP framework to fill essential data gaps necessary for improved risk assessment regimens for TH system disruptors.
FIGURE 3. The project: New approach methodologies (NAMs) for improved human health risk assessment. Building upon the adverse outcome pathway (AOP)-framework the project develops NAMs relating to specific molecular initiating events (MIE) and key events (KE) for thyroid hormone system disruption. Some of these relations are shown. The project also includes a number of activities to translate and/or validate assays, including leveraging data from zebrafish embryos to inform on human health and in vitro to in vivo translation.
We will address considerable knowledge gaps about how TH system disruptors act in the developing organism. It still remains poorly understood how different MIEs cause effects throughout the organism. This is partly because THs exert specific spatiotemporal functions during development; functions that are not well characterized. Another uncertainty relates to how chemical exposure, TH signaling, and developmental processes interact both systemically and locally. It is recognized that disrupted hormone concentrations at the systemic level can cause varying effects locally, also for TH system disruption, as recently exemplified in rats exposed to the perfluorinated compound PFOS (Davidsen et al., 2022). This means that we need to carefully consider the mechanisms or effects that we are targeting with NAMs when designing non-animal test methods for predictive toxicology purposes. We will leverage in vivo rat toxicity studies with multi-organ RNA-seq approaches (e.g., brain, liver, thyroid gland, heart) to decipher modes of action and characterize relationships between different MIEs, their downstream KEs and ultimately AOs. This will provide knowledge needed to pinpoint future NAMs that are necessary to cover more completely the various MIEs, pathways and effects that TH system disruption may have on the intact organism.
For the in vitro assessment of TH system disruptors, additional focus will be on the liver. This is an important area to consider because a large proportion of chemical compounds may cause TH system disruption through effects in the liver (extrathyroidal mode of action), including per- and polyfluoroalkyl substances (PFAS), flame retardants, pesticides and food additives. With attention on the developing liver, a human-relevant stem cell-based in vitro model will be used to assess TH-mediated liver toxicity relevant for the early phases of development. Multipotent human skin-derived precursor cells (hSKPs) will be differentiated towards “hepatic progenitor cells” (hSKP-HPC), mimicking human liver embryogenesis (De Kock et al., 2009; Snykers et al., 2009). With this assay we aim to develop a method to characterize effects of chemicals on the developing liver, TH-signaling and its downstream effects (e.g., lipid metabolism), but also how chemicals may change the TH system in the liver (including deiodination and sulfation, nuclear receptor activation, TH distribution and transmembrane transport) and their downstream consequences for the organism. To achieve this, we will for instance use transcriptomics approaches to enable comparisons with effects observed in in vivo toxicity studies to scrutinize potential similarities and differences between rats and humans. Finally, through synergistic activities across PARC projects, physiological-based kinetics (PBK) modelling will further aid in the quantitative understanding of causal relationships leading to adverse effect outcomes.
In addition to the in vitro assays, we will develop new quantitative structure–activity relationship (QSAR) models for in silico prediction of chemical substances’ potential TH disrupting properties. They will be developed to expand on the existing publicly available battery of endpoints relevant for TH system disruption (see e.g., www.qsar.food.dtu.dk). QSAR models that are already finalized or under development by the PARC partner include TPO, NIS, TRs, deiodinases (DIOs), pregnane X receptor (PXR), constitutive androstane receptor (CAR), and aryl hydrocarbon receptor (AhR). This project will contribute with models for TTR binding (expansion/remodeling with other technologies of previously published model (Zhang et al., 2015)) and, to our knowledge, the first models for monocarboxylate transporter 8 (MCT8) and iodothyrosine dehalogenase (DEHAL1) activity, and possibly more. Generation of additional experimental data to challenge and expand the predictive capacity of certain QSAR models is also planned as collaborative activities across the PARC partnership.
To address some of the complexities of the TH system, we will explore the use of a 3D cell culture to model the complex process of TH synthesis and develop assays to test for potential chemical interference with TH transport across biological barriers. We will explore a thyroid follicle model (human or animal) for its inherent characteristics, ability for TH synthesis and its predictive capacity for TH system disruption by comparison with results from various NAMs, e.g., covering NIS and TPO activities. We will develop in vitro bioassays to study the inhibition of TH transport across physiological barriers with a focus on the blood-cerebrospinal fluid-barrier. This is crucial since TH action depends on TH being actively transported into both cells, the fetus and the brain. To this end, an induced pluripotent stem cell (iPSC)-based choroid plexus organoid model capable of excreting a cerebrospinal (CSF)-like fluid (Pellegrini et al., 2020) will be developed and used to study TH transport across the blood-cerebrospinal fluid-barrier and to identify new TH transmembrane transporters. Then, relevant transporters will be selected to expand the battery of stable TH transmembrane transporter-overexpressing cell lines, where MCT8 currently exist and organic-anion-transporting polypeptides 1C1 (OATP1C1) and organic anion transporter 4 (OAT4) are under development. These cell lines can be used to screen compounds for their capacity to inhibit TH transmembrane transporters that not only play a role in TH transport across the blood-cerebrospinal fluid-barrier, but also across the placenta and the blood-brain-barrier.
To further facilitate the implementation of more NAMs for human-relevant chemical risk assessment, we will assess the benefit of including non-mammalian test species such as fish embryos and invertebrates in IATAs tailored for human and ecological health parameters. Fish embryos are not protected under the current EU legislation for the use of laboratory animals until the free-feeding stage, which corresponds to 5 days post fertilization (dpf) for zebrafish kept at 28°C (European Commission, 2020). This represents an opportunity for reducing animal testing. The TH system is highly conserved across vertebrate taxa and using data from, for instance, fish to inform on potential risks to human health would greatly facilitate the transition away from high reliance on mammalian animal toxicity testing. Development of the TH system in zebrafish covers the earliest phases of embryonic development that rely on maternally transferred THs, but also the development of the entire HPT axis, which parallels human development. During the first 5 dpf the thyroid and HPT-axis develops into a fully functional organ and, importantly, is susceptible to TPO inhibition (Opitz et al., 2011; Stinckens et al., 2016; Persani and Marelli, 2017; Vergauwen et al., 2018; Walter et al., 2019). Furthermore, elements of a vertebrate-like TH signaling pathway (that is; TRs, retinoid X receptor (RXR) and enzymes involved in vertebrate TH synthesis) have been identified in some invertebrate phyla (Taylor and Heyland, 2017) including mollusks, and both TRs and TH (of endogenous and/or exogenous origin) appear to regulate aspects of larval metamorphosis in some mollusk species (Morthorst et al., 2023). Although the exact mechanisms are largely unknown, disruption of TH signaling and metamorphosis in invertebrates may inform on potential effects in vertebrates.
We will establish new assays in zebrafish to determine the function of iodide and TH transporters. We will apply genomic and proteomic approaches to zebrafish toxicity studies to decipher the modes of action and characterize the relationships between the different MIEs and their key downstream events. These molecular analyses will be correlated to behavioral assays using zebrafish larvae to assess how potential TH system disruption affects brain development and alters behavior, for instance tail coiling to assess early motor innervation (Saint-Amant and Drapeau, 1998; de Oliveira et al., 2021), locomotor response to changes in light condition to assess the integration of the central nervous system with the peripheral nervous system and sensory organs (Berg et al., 2018), anxiety-like behaviors to assess serotonergic, dopaminergic, and adrenergic system function (Markin et al., 2021), and a habituation assay in response to auditory and light stimuli to assess non-associative learning (Roberts et al., 2013). This integration will link MIEs and KEs with AOs at the level of an intact organism. Finally, functional and proteomics data from zebrafish will be compared to data from human-based in vitro assays to validate cross-species extrapolation capacity.
To further investigate the potential of using data from e.g., fish to inform on potential risks to human health, zebrafish embryo assay data will be used in cross-species extrapolation case studies. We will collect TH system disruption data from different vertebrate taxa (i.e., fish, amphibians and mammals), both from the literature as well as data becoming available in PARC (see work described above), over the next years. This work will use the AOP framework, and specifically the TH system disruption cross-species AOP network and the associated evaluation of the taxonomic domain of applicability, to anchor observations across species to common toxicological pathways. This will aid the development of strategies for cross-species extrapolation, and sharing knowledge between human and environmental health evaluation.
As we move towards greater reliance on NAMs for chemical risk assessment, we need to close existing knowledge gaps to ensure adequate test and non-test evaluation of TH system disrupting properties. By focusing on in vivo rodent toxicogenomics, advanced stem cell-based in vitro bioassays, transporter and zebrafish embryo investigations, concomitant with cross-species and in silico studies, this project, under the PARC framework, will close important gaps in knowledge and available tests necessary to facilitate further 3R approaches for a testing framework capable of adequately identifying TH system disruptors. In other words, this project aims to improve human health risk assessment of TH system disrupting chemicals to better safeguard human health and the environment.
LR and TS wrote the first draft of the manuscript. LR, TS and ADV made graphics. All authors contributed to conception, design of the study, and wrote sections of the manuscript. All authors contributed to manuscript revision, read, and approved the submitted version.
This project is part of the Partnership for the Assessment of Risk from Chemicals (PARC), funding by the European Union’s Horizon Europe research and innovation programme under Grant Agreement No 101057014. Additional co-funders are acknowledged, including the Danish Environmental Protection Agency (DK EPA), the German Federal Institute for Risk Assessment (BfR), the University of Antwerp Research Fund (project ID 44602), the Spanish Government (Grant Number: MCIN/AEI/10.13039/501100011033/PID 2021-125948OB-I00/FEDER, UE), and Chair Mireille Aerens for the Development of Alternative Methods, Vrije Universiteit Brussel.
The authors declare that the research was conducted in the absence of any commercial or financial relationships that could be construed as a potential conflict of interest.
All claims expressed in this article are solely those of the authors and do not necessarily represent those of their affiliated organizations, or those of the publisher, the editors and the reviewers. Any product that may be evaluated in this article, or claim that may be made by its manufacturer, is not guaranteed or endorsed by the publisher.
Andersson, N., Arena, M., Auteri, D., Barmaz, S., Grignard, E., Kienzler, A., et al. (2018). Guidance for the identification of endocrine disruptors in the context of Regulations (EU) No 528/2012 and (EC) No 1107/2009. EFSA J. 16 (6), 053111–e6135. doi:10.2903/j.efsa.2018.5311
Arnesdotter, E., Spinu, N., James, F., Ebbrell, D., MarkCronin, T. D., Vanhaecke, T., et al. (2021). Derivation, characterisation and analysis of an adverse outcome pathway network for human hepatotoxicity. Toxicology 459, 152856. doi:10.1016/j.tox.2021.152856
Berg, E. M., Rebecka Björnfors, E., Pallucchi, I., Picton, L. D., and El Manira, A. (2018). Principles governing locomotion in vertebrates: Lessons from zebrafish. Front. Neural Circuits 12, 73. doi:10.3389/fncir.2018.00073
Burrow, G. N., Fisher, D. A., and Larsen, P. R. (1994). Maternal and fetal thyroid function. N. Engl. J. Med. 331 (16), 1072–1078. doi:10.1056/NEJM199410203311608
Couderq, S., Leemans, M., and Jean, B. F. (2020). Testing for thyroid hormone disruptors, a review of non-mammalian in vivo models. Mol. Cell. Endocrinol. 508, 110779. doi:10.1016/j.mce.2020.110779
Crofton, K. M., Gilbert, M. E., Friedman, K. P., Demeneix, B., Marty, M. S., and Zoeller, R. T. (2019). Inhibition of thyroperoxidase and subsequent adverse neurodevelopmental outcomes in mammals” AOPWiki. Retrieved June 1, 2022 Available at: https://aopwiki.org/aops/42.
Davidsen, N., Ramhøj, L., Kugathas, I., Poulsen, R., Evrard, B., Thomas, A., et al. (2022). PFOS-induced thyroid hormone system disrupted rats display organ-specific changes in their transcriptomes. Environ. Pollut. 305, 119340. doi:10.1016/j.envpol.2022.119340
De Kock, J., Vanhaecke, T., Biernaskie, J., Vera, R., and Snykers, S. (2009). Characterization and hepatic differentiation of skin-derived precursors from adult foreskin by sequential exposure to hepatogenic cytokines and growth factors reflecting liver development. Toxicol. Vitro 23 (8), 1522–1527. doi:10.1016/j.tiv.2009.08.014
De La Vieja, A., and Santisteban, P. (2018). Role of iodide metabolism in physiology and cancer. Endocrine-Related Cancer 25 (4), R225–R245. doi:10.1530/ERC-17-0515
De Oliveira, A. A. S., Brigante, T. A. V., and Oliveira., D. P. (2021). Tail coiling assay in zebrafish (Danio rerio) embryos: Stage of development, promising positive control candidates, and selection of an appropriate organic solvent for screening of developmental neurotoxicity (DNT). WaterSwitzerl. 13 (2), 119. doi:10.3390/w13020119
Directorate-General for Environment (2023). Reach. Retrieved April 27, 2023 Available at: https://ec.europa.eu/environment/chemicals/reach/reach_en.htm.
ECHA (2023). REACH registration statistics. Available at: https://echa.europa.eu/documents/10162/2741157/registration_statistics_en.pdf/58c2d7bd-2173-4cb9-eb3b-a6bc14a6754b?t=1649160655122.
ECHA/EFSA. 2018. “ECHA (European chemicals agency) and EFSA (European food safety authority) with the technical support of the joint research Centre (JRC).
European Chemicals Agency (2016). New approach methodologies in regulatory science: Proceedings of a scientific workshop. Helsinki: ECHA, 19–20. April 2016.
European Commission (2020). COMMISSION IMPLEMENTING DECISION (EU) 2020/569 of 16 april 2020 a common format and information content for the submission of the information to Be by member states pursuant to directive 2010/63/EU of the European parliament and of the council on the protection of animals used for scientific purposes and repealing commission decision 2012/707/EU. Official Journal of the European Union.
Ghassabian, A., El Marroun, H., Peeters, R. P., Jaddoe, V. W., Hofman, A., Verhulst, F. C., et al. (2014). Downstream effects of maternal hypothyroxinemia in early pregnancy: Nonverbal IQ and brain morphology in school-age children. J. Clin. Endocrinol. Metabolism 99 (7), 2383–2390. doi:10.1210/jc.2013-4281
Gilbert, M. E., O’Shaughnessy, K. L., and Axelstad, M. (2020). Regulation of thyroid-disrupting chemicals to protect the developing brain. Endocrinology 161 (10), bqaa106–17. doi:10.1210/endocr/bqaa106
Gyllenberg, D., Sourander, A., Marja Surcel, H., Hinkka-Yli-Salomäki, S., McKeague, I. W., and Brown, A. S. (2016). Hypothyroxinemia during gestation and offspring schizophrenia in a national birth cohort. Biol. Psychiatry 79 (12), 962–970. doi:10.1016/j.biopsych.2015.06.014
Henrichs, J., Bongers-Schokking, J. J., Schenk, J. J., Ghassabian, A., Schmidt, H. G., Visser, T. J., et al. (2010). Maternal thyroid function during early pregnancy and cognitive functioning in early childhood: The generation R study. J. Clin. Endocrinol. Metabolism 95 (9), 4227–4234. doi:10.1210/jc.2010-0415
Herin, F., Boutet-Robinet, E., Levant, A., Dulaurent, S., Manika, M., Galatry-Bouju, F., et al. 2011. Thyroid function tests in persons with occupational exposure to fipronil. Thyroid 21(7):701–706. doi:10.1089/thy.2010.0449
Holbech, H., Matthiessen, P., Hansen, M., Schüürmann, G., Knapen, D., Reuver, M., et al. (2020). Ergo: Breaking down the wall between human health and environmental testing of endocrine disrupters. Int. J. Mol. Sci. 21 (8), 2954. doi:10.3390/ijms21082954
Howdeshell, K. L. (2002). A model of the development of the brain as a construct of the thyroid system. Environ. Health Perspect. 110, 337–348. doi:10.1289/ehp.02110s3337
Joint Research Centre 2023. “EU-NETVAL (European union network of laboratories for the validation of alternative methods).” Retrieved April 27, 2023 Available at: https://joint-research-centre.ec.europa.eu/eu-reference-laboratory-alternatives-animal-testing-eurl-ecvam/alternative-methods-toxicity-testing/eu-netval-european-union-network-laboratories-validation-alternative-methods_en.
Knapen, D., Angrish, M. M., Fortin, M. C., Katsiadaki, I., Leonard, M., Margiotta-Casaluci, L., et al. (2018). Adverse outcome pathway networks I: Development and applications. Environ. Toxicol. Chem. 37 (6), 1723–1733. doi:10.1002/etc.4125
Knapen, D., Stinckens, E., Cavallin, J. E., Ankley, G. T., Holbech, H., Villeneuve, D. L., et al. (2020). Toward an AOP network-based tiered testing strategy for the assessment of thyroid hormone disruption. Environ. Sci. Technol. 54 (14), 8491–8499. doi:10.1021/acs.est.9b07205
Köhrle, J., and Frädrich, C. (2022). Deiodinases control local cellular and systemic thyroid hormone availability. Free Radic. Biol. Med. 193, 59–79. doi:10.1016/j.freeradbiomed.2022.09.024
Korevaar, T. I. M., Ryan, M., Medici, M., Chaker, L., Vincent, W. V., Jaddoe, Y. B., et al. (2016). Association of maternal thyroid function during early pregnancy with offspring iq and brain morphology in childhood: A population-based prospective cohort study. Lancet Diabetes Endocrinol. 4 (1), 35–43. doi:10.1016/S2213-8587(15)00327-7
Kortenkamp, A., Axelstad, M., Gustaf Bornehag, C., Cenijn, P., Christiansen, S., Lindberg, J., et al. 2020. “Removing critical gaps in chemical test methods by developing new assays for the identification of thyroid hormone system-disrupting chemicals–The athena project.” Int. J. Mol. Sci. 21, 3123(9). doi:10.3390/ijms21093123
Landers, K., and Richard, K. (2017). Traversing barriers – how thyroid hormones pass placental, blood-brain and blood-cerebrospinal fluid barriers. Mol. Cell. Endocrinol. 458, 22–28. doi:10.1016/j.mce.2017.01.041
López-Márquez, A., Carrasco-López, C., Fernández-Méndez, C., and Santisteban, P. (2021). Unraveling the complex interplay between transcription factors and signaling molecules in thyroid differentiation and function, from embryos to adults. Front. Endocrinol. 12, 654569–654618. doi:10.3389/fendo.2021.654569
Markin, P. A., Alex Brito, , , Moskaleva, N. E., Tagliaro, F., Vadim, V. T., Savitskii, M. V., et al. (2021). Short- and medium-term exposures of diazepam induce metabolomic alterations associated with the serotonergic, dopaminergic, adrenergic and aspartic acid neurotransmitter systems in zebrafish (Danio rerio) embryos/larvae. Comp. Biochem. Physiology - Part D Genomics Proteomics 38, 100816. doi:10.1016/j.cbd.2021.100816
Marty, M. S., Sauer, U. G., Charlton, A., Ghaffari, R., Guignard, D., Hallmark, N., et al. (2022). Towards a science-based testing strategy to identify maternal thyroid hormone imbalance and neurodevelopmental effects in the progeny–Part III: How is substance-mediated thyroid hormone imbalance in pregnant/lactating rats or their progeny related to neurodevelopmental effects? Crit. Rev. Toxicol. 52 (7), 546–617. doi:10.1080/10408444.2022.2130166
Marx-Stoelting, P., Rivière, G., Luijten, M., Aiello-Holden, K., Bandow, N., Baken, K., et al. (2023). A walk in the PARC: Developing and implementing 21st century chemical risk assessment in Europe. Archives Toxicol. 97, 893–908. doi:10.1007/s00204-022-03435-7
Modesto, T., Tiemeier, H., Peeters, R. P., Jaddoe, V. V., Hofman, A., Verhulst, F. C., et al. (2015). Maternal mild thyroid hormone insufficiency in early pregnancy and attention-deficit/hyperactivity disorder symptoms in children. JAMA Pediatr. 169 (9), 838–845. doi:10.1001/jamapediatrics.2015.0498
Morreale de Escobar, G., María Jesús., O., and Escobar del Rey, F. (2004). Role of thyroid hormone during early brain development. Eur. J. Endocrinol./Eur. Fed. Endocr. c. 151, U25–U37. doi:10.1530/eje.0.151u025
Morthorst, J. E., Holbech, H., De Crozé, N., Matthiessen, P., and LeBlanc, G. A. (2023). Thyroid-like hormone signaling in invertebrates and its potential role in initial screening of thyroid hormone system disrupting chemicals. Integr. Environ. Assess. Manag. 19 (1), 63–82. doi:10.1002/ieam.4632
Noyes, P. D., Patience, B., Haselman, J. T., Gilbert, M. E., Hornung, M. W., Barone, S., et al. (2019). Evaluating chemicals for thyroid disruption: Opportunities and challenges with in vitro testing and adverse outcome pathway approaches. Environ. Health Perspect. 127 (9), 95001. doi:10.1289/EHP5297
OECD (2014). New scoping document on in vitro and ex vivo assays for the identification of modulators of thyroid hormone signaling. OECD Environment. Health and Safety Publications Series on Testing and Assessment No. 207. ENV/JM/MONO(23).
OECD (2018). Revised guidance document 150 on standardised test guidelines for evaluating chemicals for endocrine disruption. OECD Ser. Test. Assess. (286). doi:10.1787/9789264304741-en
Opitz, R., Maquet, E., Zoenen, M., Dadhich, R., and Costagliola, S. (2011). TSH receptor function is required for normal thyroid differentiation in zebrafish. Mol. Endocrinol. 25 (9), 1579–1599. doi:10.1210/me.2011-0046
Ortiga-Carvalho, T. M., Chiamolera, M. I., Pazos-Moura, C. C., and Wondisford, F. E. (2016). Hypothalamus-pituitary-thyroid Axis. Compr. Physiol. 6 (3), 1387–1428. doi:10.1002/cphy.c150027
PARC (2023). Partnership for the assessment of risks from chemicals. Retrieved March 7, 2023 Available at: https://www.eu-parc.eu/.
Pellegrini, L., Bonfio, C., Chadwick, J., Begum, F., Skehel, M., and Madeline, A. (2020). Human CNS barrier-forming organoids with cerebrospinal fluid production. Science 369 (6500), eaaz5626. doi:10.1126/science.aaz5626
Persani, L., and Marelli, F. (2017). How zebrafish research has helped in understanding thyroid diseases. F1000Res. 6, 2137. doi:10.12688/f1000research.12142.1
Roberts, A. C., Bill, B. R., and Glanzman, D. L. (2013). Learning and memory in zebrafish larvae In Frontiers in Neural Circuits (Issue JUL). doi:10.3389/fncir.2013.00126
Rolaki, A., Pistollato, F., and Munn, S. (2019). Adverse outcome pathway on inhibition of Na+/I-symporter (NIS) leads to learning and memory impairment. OECD series on adverse outcome pathways No. 14. Paris: OECD Publishing. doi:10.1787/7ca86a34-en
Román, G. C., Ghassabian, A., Bongers-Schokking, J. J., Vincent, W. V. J., Hofman, A., De Rijke, Y. B., et al. (2013). Association of gestational maternal hypothyroxinemia and increased autism risk. Ann. Neurology 74 (5), 733–742. doi:10.1002/ana.23976
Roques, B. B., Lacroix, M. Z., Puel, S., Gayrard, V., Picard-Hagen, N., Jouanin, I., et al. (2012). CYP450-Dependent biotransformation of the insecticide fipronil into fipronil sulfone can mediate fipronil-induced thyroid disruption in rats. Toxicol. Sci. 127 (1), 29–41. doi:10.1093/toxsci/kfs094
Saint-Amant, L., and Drapeau, P. (1998). Time course of the development of motor behaviors in the zebrafish embryo. J. Neurobiol. 37 (4), 622–632. doi:10.1002/(SICI)1097-4695(199812)37:4<622::AID-NEU10>3.0.CO;2-S
Snykers, S., Tom, H., De Rop, E., Vinken, M., Fraczek, J., De Kock, J., et al. (2009). Role of epigenetics in liver-specific gene transcription, hepatocyte differentiation and stem cell reprogrammation. J. Hepatology 51 (1), 187–211. doi:10.1016/j.jhep.2009.03.009
Stinckens, E., Vergauwen, L., Schroeder, A. L., Maho, W., Blackwell, B. R., Witters, H., et al. (2016). Impaired anterior swim bladder inflation following exposure to the thyroid peroxidase inhibitor 2-mercaptobenzothiazole Part II: Zebrafish. Aquat. Toxicol. 173, 204–217. doi:10.1016/j.aquatox.2015.12.023
Taylor, E., and Heyland, A. (2022). Evolution of non-genomic nuclear receptor function. Mol. Cell. Endocrinol. 539, 111468. doi:10.1016/j.mce.2021.111468
Taylor, E., and Heyland, A. (2017). Evolution of thyroid hormone signaling in animals: Non-genomic and genomic modes of action. Mol. Cell. Endocrinol. 459, 14–20. doi:10.1016/j.mce.2017.05.019
Vergauwen, L., Cavallin, J. E., Ankley, G. T., Bars, C., Gabriëls, I. J., Michiels, E. D. G., et al. (2018). Gene transcription ontogeny of hypothalamic-pituitary-thyroid Axis development in early-life stage fathead minnow and zebrafish. General Comp. Endocrinol. 266, 87–100. doi:10.1016/j.ygcen.2018.05.001
Vergauwen, L., Stinckens, E., Villeneuve, D. L., and Knapen, D. (2022a). Deiodinase 1 inhibition leading to increased mortality via reduced anterior swim bladder inflation. OECD series on adverse outcome pathways No. 25. Paris: OECD Publishing. doi:10.1787/9f651c16-en
Vergauwen, L., Stinckens, E., Villeneuve, D. L., and Knapen, D. (2022b). Deiodinase 1 inhibition leading to increased mortality via reduced posterior swim bladder inflation. OECD series on adverse outcome pathways No. 24. Paris: OECD Publishing. doi:10.1787/9cb525e9-en
Vergauwen, L., Stinckens, E., Villeneuve, D. L., and Knapen, D. (2022c). OECD series on adverse outcome pathways No. 23. Paris: OECD Publishing. doi:10.1787/b1bf0bea-enDeiodinase 2 inhibition leading to increased mortality via reduced anterior swim bladder inflation
Vergauwen, L., Stinckens, E., Villeneuve, D. L., and Knapen, D. (2022d). Deiodinase 2 inhibition leading to increased mortality via reduced posterior swim bladder inflation. OECD series on adverse outcome pathways No. 22. Paris: OECD Publishing. doi:10.1787/dc406014-en
Vergauwen, L., Stinckens, E., Villeneuve, D. L., and Knapen, D. (2022e). Thyroperoxidase inhibition leading to increased mortality via reduced anterior swim bladder inflation. OECD series on adverse outcome pathways No. 26. Paris: OECD Publishing. doi:10.1787/447c5cba-en
Walter, K. M., Miller, G. W., Chen, X., Yaghoobi, B., Puschner, B., and Pamela, J. (2019). Effects of thyroid hormone disruption on the ontogenetic expression of thyroid hormone signaling genes in developing zebrafish (Danio rerio). General Comp. Endocrinol. 272, 20–32. doi:10.1016/j.ygcen.2018.11.007
Yen, P. M. (2001). Physiological and molecular basis of thyroid hormone action. Physiol. Rev. 81 (3), 1097–1142.
Zhang, J., Kamstra, J. H., Ghorbanzadeh, M., Weiss, J. M., Hamers, T., and Patrik, L. (2015). In silico approach to identify potential thyroid hormone disruptors among currently known dust contaminants and their metabolites. Environ. Sci. Technol. 49 (16), 10099–10107. doi:10.1021/acs.est.5b01742
Keywords: PARC, endocrine disruption, thyroid disruption, non-animal test methods, regulatory toxicology, adverse outcome pathways, chemicals
Citation: Ramhøj L, Axelstad M, Baert Y, Cañas-Portilla AI, Chalmel F, Dahmen L, De La Vieja A, Evrard B, Haigis A-C, Hamers T, Heikamp K, Holbech H, Iglesias-Hernandez P, Knapen D, Marchandise L, Morthorst JE, Nikolov NG, Nissen ACVE, Oelgeschlaeger M, Renko K, Rogiers V, Schüürmann G, Stinckens E, Stub MH, Torres-Ruiz M, Van Duursen M, Vanhaecke T, Vergauwen L, Wedebye EB and Svingen T (2023) New approach methods to improve human health risk assessment of thyroid hormone system disruption–a PARC project. Front. Toxicol. 5:1189303. doi: 10.3389/ftox.2023.1189303
Received: 18 March 2023; Accepted: 05 May 2023;
Published: 17 May 2023.
Edited by:
Rosaria Meccariello, University of Naples Parthenope, ItalyCopyright © 2023 Ramhøj, Axelstad, Baert, Cañas-Portilla, Chalmel, Dahmen, De La Vieja, Evrard, Haigis, Hamers, Heikamp, Holbech, Iglesias-Hernandez, Knapen, Marchandise, Morthorst, Nikolov, Nissen, Oelgeschlaeger, Renko, Rogiers, Schüürmann, Stinckens, Stub, Torres-Ruiz, Van Duursen, Vanhaecke, Vergauwen, Wedebye and Svingen. This is an open-access article distributed under the terms of the Creative Commons Attribution License (CC BY). The use, distribution or reproduction in other forums is permitted, provided the original author(s) and the copyright owner(s) are credited and that the original publication in this journal is cited, in accordance with accepted academic practice. No use, distribution or reproduction is permitted which does not comply with these terms.
*Correspondence: Louise Ramhøj, bG91cmFtQGZvb2QuZHR1LmRr
†Present address:Gerrit Schüürmann, Institute of Organic Chemistry Technical University Bergakademie Freiberg, Leipziger, Germany
Disclaimer: All claims expressed in this article are solely those of the authors and do not necessarily represent those of their affiliated organizations, or those of the publisher, the editors and the reviewers. Any product that may be evaluated in this article or claim that may be made by its manufacturer is not guaranteed or endorsed by the publisher.
Research integrity at Frontiers
Learn more about the work of our research integrity team to safeguard the quality of each article we publish.