- 1Department of Pharmacology and Toxicology National Institute of Pharmaceutical Education and Research, Raebareli (NIPER-R), Transit Campus, Raebareli, India
- 2Department of Neuro-Oncology The University of Texas, MD Anderson Cancer Center, Houston, TX, United States
Microplastics (MPs), small pieces of plastic (∼5 mm), are released into the environment not only as a result of the decomposition of large-sized plastics but also from day-to-day use of plastic products. Chronic exposure to MPs has been attributed to harmful effects on aquatic organisms and rodents. Effects include gastrointestinal toxicity, hepatotoxicity, neurotoxicity, and reproductive and developmental toxicities. Exposure to MPs may also potentially affect human health. Herein, we reviewed the impact of MPs on male and female reproductive systems and the associated mechanisms involved in the reproductive and developmental toxicities of MPs. We performed a literature search in Google Scholar and PubMed using the following keywords: MPs and reproductive toxicity; MPs and developmental studies; MPs and infertility; MPs and aquatics; and MPs and rodents. Evidence of MPs accumulation has been reported in many organs of humans and experimental models. The harmful effects of MPs have been manifested in male and female reproductive systems of mammalian and aquatic animals, including developmental effects on gametes, embryos, and their offspring. This review describes various signaling pathways involved in MPs-associated male and female reproductive and developmental toxicities.
Introduction
Plastics are frequently used in day-to-day life due to their low cost, ease of handling, transportation, production process, and widespread applications. The production of plastics has been increasing continuously for the last 60 years. Many of these are broken down into small plastics called microplastics (MPs) (Avio et al., 2017). Mechanical stress, sunlight, and an oxidizing atmosphere decompose large plastics into MPs, typically 5 mm in diameter. Numerous samples from the environment, including rivers, Antarctic snow, and biogas plants, have been collected to identify various types of MPs such as polypropylene (PP), polyethylene (PE), polystyrene (PS), and polyethylene terephthalate (PT) (Dumichen et al., 2017; Aves et al., 2022). The generation of MPs is not only limited to their degradative by-products but also emerged from clothing microfibres (Hernandez et al., 2017; Galvao et al., 2020), indoor dust (Zhang et al., 2020), cosmetics (facial scrubs) (Napper et al., 2015), tap water (Tong et al., 2020), and seafood (MPs accumulation) (Leung et al., 2021).
MPs affect the normal functioning of the organisms and may cause several organ-specific toxicities such as neuronal, digestive, reproductive, and developmental toxicity (Yin et al., 2021). Compromised sperm quality in men and infertility problems in women have been reported among plastic industry workers. (Jelnes, 1988; Hougaard et al., 2009). Indeed, micro- and nano-particles of plastics may pose more risk to the reproductive system. Various studies have been conducted on animals in order to understand the effect of MPs on male and female fertility (Hou B. et al., 2021; Haddadi et al., 2022; Wei et al., 2022). Moreover, MPs may also affect the growth of offspring when the mother is exposed for a longer duration, suggesting the detrimental effects of MPs on development and growth (Luo et al., 2019; Wang et al., 2019; Hu et al., 2021). Therefore, further research studies are required to understand the in-depth biological effects of MPs on the reproductive and development process, as they can affect future generations.
Reproductive toxic effects of MPs exposure
Reproductive toxicity is defined as exposure to any substance that interferes with the normal functioning of male and female reproductive organs, causing the loss of fertility (United Nations Economic Commission for Europe (UNECE), 2011). Continuous exposure to environmental toxicants and pollutants such as MPs can compromise the fertility of males and females (Wei et al., 2022). It has been reported that MPs induce reproductive toxicity in various organisms, including rodents (An et al., 2021; Hou B. et al., 2021) and aquatic species such as oysters (Sussarellu et al., 2016), cladocerans (Jaikumar et al., 2019), Caenorhabditis elegans (Chen et al., 2022), and zebrafish (Danio rerio) (Qiang and Cheng, 2021). In this section, we have covered the effect of MPs on structural, functional, and hormonal changes in male and female reproductive organs (Figure 1).
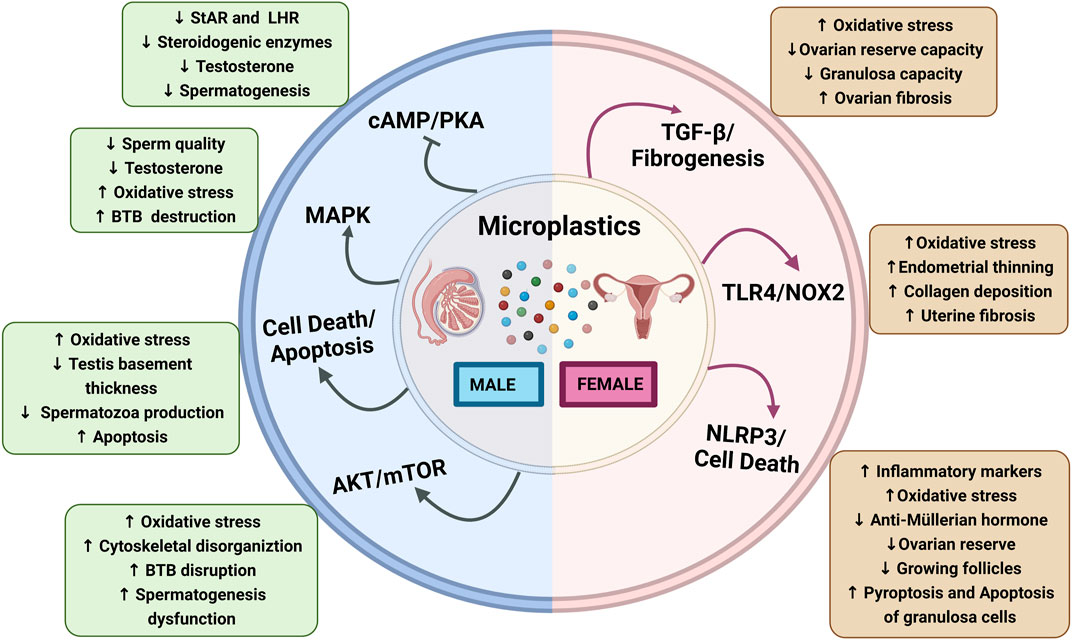
FIGURE 1. Schematic diagram showing microplastics affecting male and female reproductive functions: Microplastics affecting male reproductive functions via the activation of different signaling pathways, Akt/mTOR, apoptosis, MAPK, and inhibition of cAMP/PK3 that results in increased oxidative stress, BTB disruption, and spermatogenesis dysfunction and decrease in steroidogenic enzymes and LHR downregulation. Microplastics affecting the female reproductive functions altered by the activation of NLRP3, TLR4/NOX2, and TGF-β/fibrogenesis that results in increased oxidative stress, endometrial thinning, collagen deposition, inflammatory markers, and pyroptosis and apoptosis of granulosa cells while ovarian reserve, growing follicles, granulosa cells capacity, and anti-müllerian hormone levels are decreased. Abbreviations: cAMP, cyclic adenosine monophosphate; AKT, Ak strain transforming; BTB, blood–testes barrier; LHR, luteinizing hormone receptor; MAPK, mitogen-activated protein kinase; mTOR, mammalian target of rapamycin; NOX-2, NADPH oxidase-2; NLRP3, nod-like receptor family pyrin domain containing 3; PKA, protein kinase A; StAR, steroidogenic acute regulatory protein; TGF-β, transforming growth factor-β; TLR4, toll-like receptor-4.
MPs-induced male reproductive toxicity
MPs-induced reproductive dysfunctions have been observed in the aquatic species, which is attributed to the MPs accumulation in reproductive tissues (Issac and Kandasubramanian, 2021; Qiang and Cheng, 2021; Cormier et al., 2022; Liu Y. et al., 2022). Toxicity evaluation of PE-MPs (<400 µm) in freshwater hydra at concentrations of 0.01, 0.02, 0.04, and 0.08 g/ml for 3–96 h have reported morphological (clubbed and disintegrated tentacles) and reproductive (hydranth numbers) changes; however, these changes are non-lethal (Murphy and Quinn, 2018). Furthermore, Qiang et al. have investigated the testicular toxicity of PS-MPs in zebrafish, and exposure to 100 and 1,000 μg/L of MPs has shown increased testicular apoptosis (Qiang and Cheng, 2021). Moreover, the effect of MPs on testis has not been limited to zebrafish but has also gained attention in other organisms. Earthworms (Eisenia andrei) exposed to nano-plastics (>100 nm) for 21 days have been shown to reduce male reproductive functions and deformities in sperm cells, such as reduced mature bundle, damaged plasma membranes, and reduced density of sperm and viability of coelomocytes (Kwak and An, 2021). In addition, chronic exposure to PS-MPs (10 µm) for 28 days exhibits a significant decrease in testosterone, spermatogenic cells, and disrupted blood–testis barrier (BTB) integrity in Balb/c mice (Jin et al., 2021). Some dose-response studies have been performed to evaluate the effects of MPs on the male reproductive organs (Park et al., 2020; Ijaz et al., 2021). PS-MPs have been assessed at the concentration of 2, 20, 200, and 2,000 μg/L in SD rats, and the lowest observed adverse effect level (LOAEL) showed at a dose of 20 μg/L, while the highest concentration (2,000 μg/L) has been reported maximum toxicity (Ijaz et al., 2021). Furthermore, at the highest concentration, PS-MPs have been reported to decrease sperm counts, motility, and viability and also reduce the follicle-stimulating hormone (FSH), luteinizing hormone (LH), and testicular and plasma testosterone levels (Ijaz et al., 2021). MPs (4 and 10 µm) exposure to male Balb/c mice for 28 days have disrupted the BTB and caused testicular inflammation via the downregulation of BTB-linked proteins (tight junction protein zonula occludens-1, occludin, basal ectoplasmic specializations (ES) protein, N-cadherin and β-catenin, and gap junction protein CX43) in the testis (Wei et al., 2021).
The steroidogenic enzymes P450scc, P450c17, 3β-HSD, and 17β-HSD are involved in testosterone synthesis in Leydig’s cells (Sun et al., 2019). Jin et al. reported that administration of 100 and 1,000 μg/L of PS-MPs (0.5, 4, and 10 μm) in mice with drinking water for 180 days had shown a significant decrease in steroidogenic enzymes and steroidogenic acute regulatory protein (StAR) levels (Jin et al., 2022). MPs may also cause morphological changes in sperm, such as absent and small head and acrosome loss (Jin et al., 2022). A repeated oral toxicity study for 28 days in SD rats has shown that PP-MPs induce lesions in the testis and epididymis at 25 mg/kg/day (Jin et al., 2022). A recent study has reported that exposure to PS-MPs (1%–10% crushed PS disposable plates for 90 days) showed a remarkable decrease in epididymal sperm count, motility, and serum testosterone level in male albino Wistar rats (Ilechukwu et al., 2022). Based on the above mentioned findings from diverse model systems, it is pertinent that MPs exposure adversely affects the male reproductive system (Figure 1). However, more evidence is required to validate the mechanism of MPs toxicity on male fertility and reproductive health. Hence, it is suggested that future mechanistic investigations are urgently needed to understand the MPs-associated reproductive toxicities.
MPs-induced female reproductive toxicity
The harmful effects of MPs have not been limited to the male reproductive system (D'Angelo and Meccariello, 2021) but have also been shown to adversely affect the female reproductive system (Wei et al., 2022). Although, as compared to male reproductive studies, a limited number of studies are conducted to evaluate the harmful effects on the female reproductive system. MPs-associated female reproductive toxicity has been studied in several species, including zebrafish (Qiang and Cheng, 2021), oysters (Sussarellu et al., 2016), zooplankton (copepods) (Cole et al., 2015), medaka fishes (Yan et al., 2020; Li et al., 2022), mice (Liu Z. et al., 2022; Wei et al., 2022), and rats (An et al., 2021). Here, we have summarized the available studies on MPs exposure in female reproductive cells/organs.
Copepods, a zooplankton species, were subjected to PS-beads (20 μm, 75 MPs/ml) and cultured algae (250 µgCL−1) for 24 h and have shown a significant reduction in ingestion, fecundity, and survival rate, though no change in laying out eggs were observed (Cole et al., 2015). PS-MPs (2 and 6 µm) exposure (0.023 mg/L) to oysters for 2 months has reported a decrease in diameter and number of oocytes (Sussarellu et al., 2016). The combined effect of MPs with heavy metals in medaka fishes (Oryzias melastigma) has been shown to perturb similar changes such as irregular oocytes, partly adhesion, and empty follicle in the ovaries of female medaka (Yan et al., 2020). Moreover, PS-MPs (5 µm) exposure (0.1 mg/day) for 24–26 days by oral gavage has perturbs folliculogenesis such as disrupted follicles maturation, differentiation, and increased number of atretic and cyst follicles in Wistar rats (Haddadi et al., 2022). In addition, estrous cycle disruption due to exposure to MPs has also been reported in female rats (Haddadi et al., 2022). PS-MPs (5 µm) led to a significant decrease in the duration of the fourth estrus cycle and reduced the duration of the metestrus phase compared to control rats (Haddadi et al., 2022). Further, the continuous exposure to PS-MPs (30 mg/kg) for 35 days impaired the follicles development, quality, and maturation of oocytes in the ovaries of mice (Liu Z. et al., 2022). Furthermore, the accumulation of MPs (0.5 µm) in granulosa cells of rats has been reported to interfere with the normal functioning, growth, and differentiation of oocytes and lead to female reproductive toxicity via apoptosis, pyroptosis, and fibrosis (An et al., 2021; Hou J. et al., 2021). Therefore, extensive exposure to MPs aggravates various toxicities such as decreased diameter and number of oocytes, decreased or empty follicles, inflamed ovaries, and reduced ovarian reserve (Yan et al., 2020; Hou J. et al., 2021; Liu Z. et al., 2022).
Overall, these studies suggest that MPs are associated with negative effects on the female reproductive system (Figure 1).
MPs-induced developmental toxicity
Developmental toxicity is defined as any reversible or irreversible functional or structural alteration caused by environmental insult, diet, and toxic chemicals or physical factors that affect organisms’ normal growth, differentiation, development, or behaviour (Hougaard, 2021). In this section, we have discussed the development and growth effects such as fetal growth, deformities, and death in offspring whose parents are exposed to MPs for an extended period. A recent study investigated four generations (F0, F1, F2, and F3) of developmental effects in Daphnia magna with 21 days of exposure to MPs and reported a significantly reduced population growth rate and reproduction (Martins and Guilhermino, 2018). Furthermore, there is a slow recovery up to F3 generation, which accounts for the developmental toxicity in the Daphnia magna population (Martins and Guilhermino, 2018). Moreover, a transgenerational study of PS-MPs in the marine medaka (Oryzias melastigma) has reported a delayed incubation time and gonads maturation, hatching rate, and body length of offspring at the dose of 20 and 200 mg/L (Wang et al., 2019). Findings of aquatic studies have also been translated into rodents. The results of 90 days of repeated exposure (0.125, 0.5, 2 mg/day) to PE-MPs (40–48 µm) have shown a significant reduction in the number of live births/dam, sex ratio, and pups’ body weight in the Institute of Cancer Research (ICR) mice (Park et al., 2020). In addition, MPs have disrupted maternal-fetal connection in allogenic pregnant mice, as evident by increased embryo resorption rate and decreased number and diameter of uterine arterioles (Hu et al., 2021). This finding indicates that MPs may pose a threat to fetus development. Several studies have reported the developmental and reproductive toxicities of MPs in different species, summarized in Table 1. However, more studies are required to decipher the mechanisms of MP-associated developmental toxicity and how MPs could cross the placental barrier and impact growth in utero and postnatal stages.
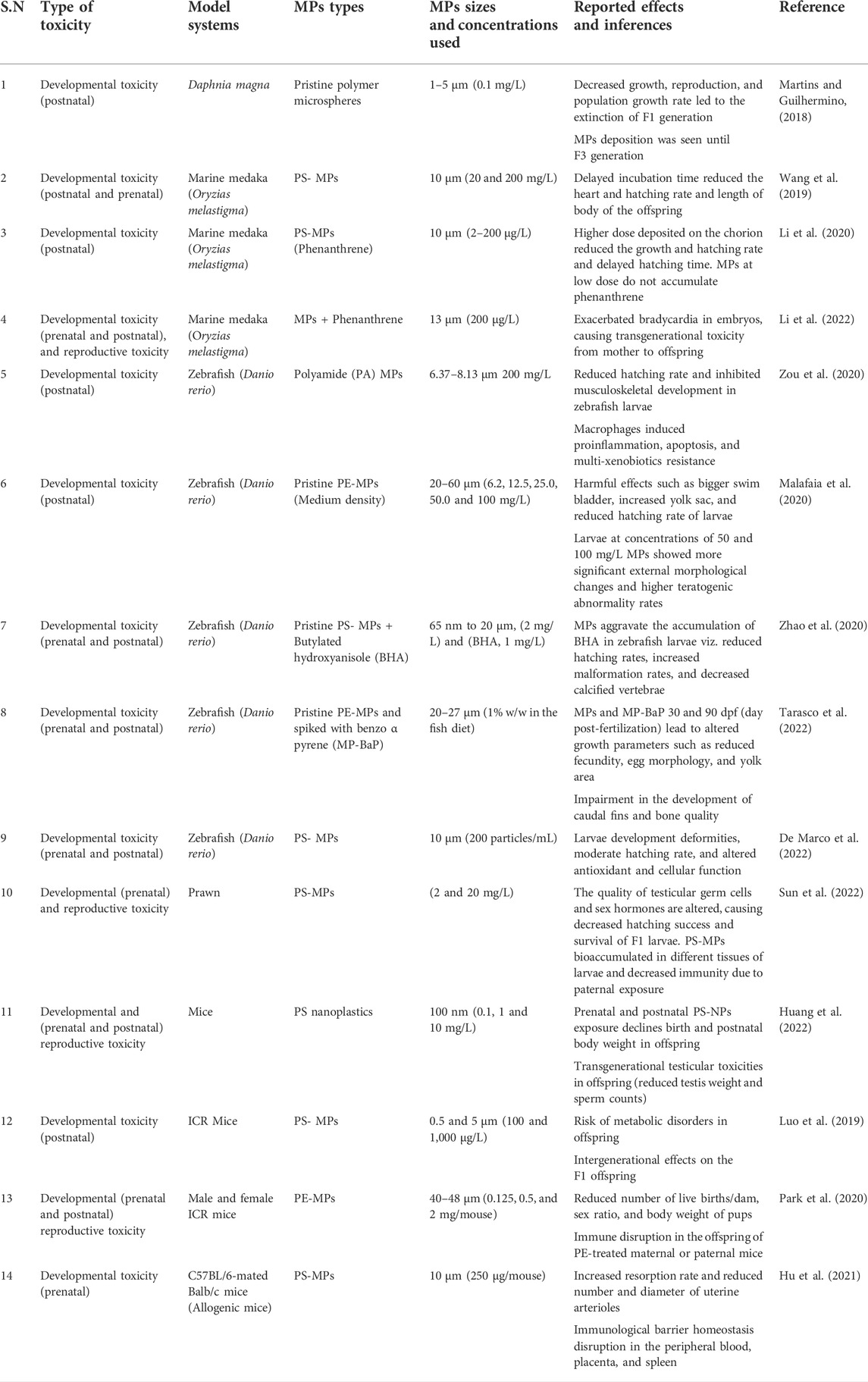
TABLE 1. Summary of experimental studies showing developmental and harmful effects of MPs in different species and their offspring.
Potential signaling pathways involved in MPs-induced male and female reproductive toxicity
So far, MPs and their effects on reproductive and developmental organs have been reported in different model systems, primarily in laboratory experiments. MPs-induced reproductive toxicity, dysfunctions, and impairments in fertility are associated with many signaling pathways (Figure 1).
Oxidative stress and MAPK signaling pathway
Oxidative stress is a primary mediator in male and female reproductive dysfunctions (Khan et al., 2011; Agarwal et al., 2014; Ahmad et al., 2017). Excessive reactive oxygen species (ROS) generation creates an imbalance between oxidant and antioxidant status, leading to lipid peroxidation, DNA damage, and protein breakdown (Ahmad et al., 2017; Yu et al., 2018). Several experimental studies have confirmed that microplastics cause ROS production, which increases oxidative stress in gonads (An et al., 2021; Kim et al., 2021; Wei et al., 2021). In addition, mitogen-activated protein kinases (MAPK) pathways are activated through different stimuli, viz. chemical agents and UV-induced damage, cytokines, and oxidative stress (Stramucci et al., 2018). Xie et al. reported that MPs exposure activated MAPK signaling via oxidative stress in the mouse testis (Xie et al., 2020). Moreover, PS-MPs exposure for 6 weeks induces ROS generation and increases the phosphorylation of p38 and JNK MAPK in the testis of Balb/c mice (Xie et al., 2020).
Nuclear factor erythroid 2-related factor 2(Nrf2) is a critical transcription factor and acts as an antioxidant which is negatively regulated by Kelch-like ECH-associated protein 1 (Keap-1) (Kovac et al., 2015). Li et al. have shown that PS-MPs increase oxidative stress, activate the p38 MAPK, and deplete the nuclear Nrf2 pathway, which leads to poor quantity and quality of sperms and compromised BTB integrity (Li et al., 2021). The integrity of the BTB junction is regulated via N-clathrin during internalization, while selective infiltration is regulated by occludin through tight connections of BTB (Mruk and Cheng, 2010; Lie et al., 2013). Interestingly, PS-MPs have been reported to damage BTB and significantly decrease the expressions of connexin-43, claudin, and N-cadherin in rats (Li et al., 2021). In a nutshell, the MAPK-Nrf2 pathway and oxidative stress-associated mechanism seem to be involved in MPs-induced reproductive dysfunctions (Figure 1). However, more investigations are needed to identify the precise role of this signaling in MP-induced reproductive adverse effects.
Akt and mTOR signaling pathway
Mammalian target of rapamycin (mTOR) plays a vital role in the cellular processes by providing energy and cytoskeletal structure. mTOR forms two complexes, mTORC1 and mTORC2, and exerts opposite physiological functions by binding with raptor and rictor (Jesus et al., 2017; Wang and Zhang, 2019). Moreover, it plays a crucial role in the maintenance and process of spermatogenesis (Jesus et al., 2017). The ribosomal protein S6 (rpS6), a downstream gene of mTORC1, has been reported to disorganize the F-actin, resulting in leaky BTB via the rpS6-Akt-MMP-9 signaling pathway (Mok et al., 2014; Mok et al., 2015). Also, rictor helps to develop F-actin organization and maintains the BTB integrity via protein kinase C alpha (PKC-α) and gap junctions (Mok et al., 2013). A recent study has shown that PS-MPs trigger ROS-mediated imbalance of mTORC1 and mTORC2 signaling, which alter the expression of actin-related protein 3 (Arp3) and epidermal growth factor receptor pathway substrate 8 (Eps8) actin-binding proteins, eventually disrupting BTB integrity and spermatogenesis (Wei et al., 2021). The current research is still in the infancy phase and requires more studies to validate the role of Akt and mTOR signaling pathways in MPs-associated reproductive toxicity.
Inflammasome (NLRP3) and fibrotic signaling pathways
NLRP3, NOD-like receptor protein 3, is a multi-protein that acts as the defense mechanism against microorganisms, endogenous damage, and toxic stimuli but is also involved in male and female infertility (de Rivero Vaccari, 2020; Sano et al., 2022). The activation of NLRP3 triggers an apoptotic and inflammatory response by converting pro-caspase-1 to caspase-1 and pro-interleukin-1 (pro-IL-1β) and pro-interleukin-18 (pro-IL-18) into interleukin-1β (IL-1β) and interleukin-18 (IL-18), respectively (Charan et al., 2022). A recent study has ascertained that PS-MPs trigger the NLRP3/caspase-1 signaling pathway by oxidative stress, leading to decreased ovarian reserve in rats (Hou J. et al., 2021). However, limited findings are reported on NLRP3-mediated biological effects of MPs.
Fibrosis is a process of development of connective tissue as a repairing response to injury and affects organ structure and function, including the reproductive organs (Amargant et al., 2020). A recent study has reported that PS-MPs exposure causes fibrosis in ovaries via activation of toll-like receptor-4/NADPH oxidase-2 (TLR4/NOX2) signaling (Wu et al., 2022). This study has also reported an increase in oxidative stress, which consequently leads to activation of NOTCH and transforming growth factor-β (TGF-β)-mediated fibrosis in the endometrial epithelial cells and uterus (Wu et al., 2022). Furthermore, PS-MPs have elevated the expression of Wnt/β-catenin, alpha-smooth muscle actin (α-SMA), TGF-β, and fibronectin in ovarian granulosa cells, thereby leading to ovarian fibrosis (An et al., 2021). Therefore, inflammatory and fibrotic signaling might be involved in the MPs-induced reproductive toxicity, particularly in females (Figure 1).
Cell death and apoptotic signaling pathways
Apoptosis pathways are the most explored in MPs-induced male and female reproductive toxicity (Qiang and Cheng, 2021; Liu Z. et al., 2022). Qiang et al. have reported that MPs induced the caspase-dependent apoptosis in zebrafish testis, which is mediated via the upregulation of several caspases and p53 (Qiang and Cheng, 2021). Furthermore, PS-MP exposure for 35 days leads to perturbed mitochondrial membrane potential and increased inflammatory and apoptotic markers (caspase-3 and Bax) that result in ovarian inflammation and poor quality of oocytes in mice (Liu Z. et al., 2022). Hence, these studies suggest that both (intrinsic and extrinsic) apoptotic pathways are involved in MPs-induced cell death and apoptosis in reproductive organs (Figure 1).
Steroidogenic and endocrine signaling pathways
Testosterone is crucial in spermatogenesis and is secreted by Leydig cells and regulated by LH signaling (Ramaswamy and Weinbauer, 2014). LH binds to its receptor (LHR) at the Leydig cell membrane, which, in turn, increases cAMP and other downstream pathways such as protein kinase A (PKA), StAR, and steroid synthases (Tremblay, 2015). Of note, Jin et al. have reported that chronic exposure to PS-MPs reduce testosterone, LH, and FSH contents in rat serum and downregulate the expression of StAR via inhibiting the AC/cAMP/PKA pathway in vitro (Jin et al., 2022). However, the effects of MPs on steroidogenic and endocrine signaling pathways are meager.
Conclusion
MPs have been shown to accumulate not only in various organs in experimental models but also in human organs such as blood, lymph, placenta, meconium, and lungs (Segovia-Mendoza et al., 2020; Amato-Lourenco et al., 2021; Braun et al., 2021; Cobanoglu et al., 2021; Ragusa et al., 2021; Jenner et al., 2022). However, MPs accumulation is not identified in the reproductive organs of humans. Assessment of MPs-associated adverse effects in humans is challenging. The concentration and size of MPs and the duration of exposure used in experimental models might be quite low and/or high compared to human exposure to MPs. However, marine organisms can be one of the indirect sources of MPs accumulation in humans from seafood and other packaged food materials. Thus, more regulations and awareness are required to curb the generation of MPs in industries and landfill sites.
The toxic effects of MPs are mainly studied in rodents and aquatic experimental models, but the implication of these findings to the human population is still debatable. MPs might trigger their adverse effects via oxidative stress, apoptosis, inflammatory and fibrotic response, and altering hormonal balance. Other than these findings, the mechanism(s) of MPs toxicities remain largely unknown. The current understanding of MPs-associated reproductive toxicity is limited and is a nascent area of research, which needs future mechanistically focused investigation to understand the harmful effects of MPs on male and female reproductive organs, including the risk of developmental effects.
Author contributions
SpK and SbK conceptualized the idea of review, critically checked the manuscript, and did all the necessary corrections. ID collected all the works of literature and wrote and formatted the manuscript.
Conflict of interest
The authors declare that the research was conducted in the absence of any commercial or financial relationships that could be construed as a potential conflict of interest.
Publisher’s note
All claims expressed in this article are solely those of the authors and do not necessarily represent those of their affiliated organizations, or those of the publisher, the editors, and the reviewers. Any product that may be evaluated in this article, or claim that may be made by its manufacturer, is not guaranteed or endorsed by the publisher.
References
Agarwal, A., Virk, G., Ong, C., and Du Plessis, S. S. (2014). Effect of oxidative stress on male reproduction. World J. Mens. Health 32 (1), 1–17. doi:10.5534/wjmh.2014.32.1.1
Ahmad, G., Almasry, M., Dhillon, A. S., Abuayyash, M. M., Kothandaraman, N., and Cakar, Z. (2017). Overview and sources of reactive oxygen species (ROS) in the reproductive system, in Oxidative stress in human reproduction (Cham: Springer), 1–16.
Amargant, F., Manuel, S. L., Tu, Q., Parkes, W. S., Rivas, F., Zhou, L. T., et al. (2020). Ovarian stiffness increases with age in the mammalian ovary and depends on collagen and hyaluronan matrices. Aging Cell 19 (11), e13259. doi:10.1111/acel.13259
Amato-Lourenco, L. F., Carvalho-Oliveira, R., Junior, G. R., Dos Santos Galvao, L., Ando, R. A., and Mauad, T. (2021). Presence of airborne microplastics in human lung tissue. J. Hazard. Mat. 416, 126124. doi:10.1016/j.jhazmat.2021.126124
An, R., Wang, X., Yang, L., Zhang, J., Wang, N., Xu, F., et al. (2021). Polystyrene microplastics cause granulosa cells apoptosis and fibrosis in ovary through oxidative stress in rats. Toxicology 449, 152665. doi:10.1016/j.tox.2020.152665
Aves, A., Revell, L., Gaw, S., Ruffell, H., Schuddeboom, A., Wotherspoon, N., et al. (2022). First evidence of microplastics in Antarctic snow. Cryosphere 16, 2127–2145. doi:10.5194/tc-16-2127-2022
Avio, C. G., Gorbi, S., and Regoli, F. (2017). Plastics and microplastics in the oceans: from emerging pollutants to emerged threat. Mar. Environ. Res. 128, 2–11. doi:10.1016/j.marenvres.2016.05.012
Braun, T., Ehrlich, L., Henrich, W., Koeppel, S., Lomako, I., Schwabl, P., et al. (2021). Detection of microplastic in human placenta and meconium in a clinical setting. Pharmaceutics 13 (7), 921. doi:10.3390/pharmaceutics13070921
Charan, H. V., Dwivedi, D. K., Khan, S., and Jena, G. (2022). Mechanisms of NLRP3 inflammasome-mediated hepatic stellate cell activation: therapeutic potential for liver fibrosis. Genes Dis. doi:10.1016/j.gendis.2021.12.006
Chen, H., Yang, Y., Wang, C., Hua, X., Li, H., Xie, D., et al. (2022). Reproductive toxicity of UV-photodegraded polystyrene microplastics induced by DNA damage-dependent cell apoptosis in Caenorhabditis elegans. Sci. Total Environ. 811, 152350. doi:10.1016/j.scitotenv.2021.152350
Cobanoglu, H., Belivermis, M., Sikdokur, E., Kilic, O., and Cayir, A. (2021). Genotoxic and cytotoxic effects of polyethylene microplastics on human peripheral blood lymphocytes. Chemosphere 272, 129805. doi:10.1016/j.chemosphere.2021.129805
Cole, M., Lindeque, P., Fileman, E., Halsband, C., and Galloway, T. S. (2015). The impact of polystyrene microplastics on feeding, function and fecundity in the marine copepod Calanus helgolandicus. Environ. Sci. Technol. 49 (2), 1130–1137. doi:10.1021/es504525u
Cormier, B., Cachot, J., Blanc, M., Cabar, M., Clérandeau, C., Dubocq, F., et al. (2022). Environmental microplastics disrupt swimming activity in acute exposure in Danio rerio larvae and reduce growth and reproduction success in chronic exposure in D. rerio and Oryzias melastigma. Environ. Pollut. 308, 119721. doi:10.1016/j.envpol.2022.119721
D'Angelo, S., and Meccariello, R. (2021). Microplastics: a threat for male fertility. Int. J. Environ. Res. Public Health 18 (5), 2392. doi:10.3390/ijerph18052392
De Marco, G., Conti, G. O., Giannetto, A., Cappello, T., Galati, M., Iaria, C., et al. (2022). Embryotoxicity of polystyrene microplastics in zebrafish Danio rerio. Environ. Res. 208, 112552. doi:10.1016/j.envres.2021.112552
de Rivero Vaccari, J. P. (2020). The inflammasome in reproductive biology: a promising target for novel therapies. Front. Endocrinol. 11, 8. doi:10.3389/fendo.2020.00008
Dumichen, E., Eisentraut, P., Bannick, C. G., Barthel, A. K., Senz, R., and Braun, U. (2017). Fast identification of microplastics in complex environmental samples by a thermal degradation method. Chemosphere 174, 572–584. doi:10.1016/j.chemosphere.2017.02.010
Galvao, A., Aleixo, M., De Pablo, H., Lopes, C., and Raimundo, J. (2020). Microplastics in wastewater: microfiber emissions from common household laundry. Environ. Sci. Pollut. Res. Int. 27 (21), 26643–26649. doi:10.1007/s11356-020-08765-6
Haddadi, A., Kessabi, K., Boughammoura, S., Rhouma, M. B., Mlouka, R., Banni, M., et al. (2022). Exposure to microplastics leads to a defective ovarian function and change in cytoskeleton protein expression in rat. Environ. Sci. Pollut. Res. Int. 29, 34594–34606. doi:10.1007/s11356-021-18218-3
Hernandez, E., Nowack, B., and Mitrano, D. M. (2017). Polyester textiles as a source of microplastics from households: a mechanistic study to understand microfiber release during washing. Environ. Sci. Technol. 51 (12), 7036–7046. doi:10.1021/acs.est.7b01750
Hou, B., Wang, F., Liu, T., and Wang, Z. (2021). Reproductive toxicity of polystyrene microplastics: In vivo experimental study on testicular toxicity in mice. J. Hazard. Mat. 405, 124028. doi:10.1016/j.jhazmat.2020.124028
Hou, J., Lei, Z., Cui, L., Hou, Y., Yang, L., An, R., et al. (2021). Polystyrene microplastics lead to pyroptosis and apoptosis of ovarian granulosa cells via NLRP3/Caspase-1 signaling pathway in rats. Ecotoxicol. Environ. Saf. 212, 112012. doi:10.1016/j.ecoenv.2021.112012
Hougaard, K. S., Hannerz, H., Feveile, H., and Bonde, J. P. (2009). Increased incidence of infertility treatment among women working in the plastics industry. Reprod. Toxicol. 27 (2), 186–189. doi:10.1016/j.reprotox.2009.01.003
Hougaard, K. S. (2021). Next generation reproductive and developmental Toxicology: crosstalk into the future. Front. Toxicol. 3, 652571. doi:10.3389/ftox.2021.652571
Hu, J., Qin, X., Zhang, J., Zhu, Y., Zeng, W., Lin, Y., et al. (2021). Polystyrene microplastics disturb maternal-fetal immune balance and cause reproductive toxicity in pregnant mice. Reprod. Toxicol. 106, 42–50. doi:10.1016/j.reprotox.2021.10.002
Huang, T., Zhang, W., Lin, T., Liu, S., Sun, Z., Liu, F., et al. (2022). Maternal exposure to polystyrene nanoplastics during gestation and lactation induces hepatic and testicular toxicity in male mouse offspring. Food Chem. Toxicol. 160, 112803. doi:10.1016/j.fct.2021.112803
Ijaz, M. U., Shahzadi, S., Samad, A., Ehsan, N., Ahmed, H., Tahir, A., et al. (2021). Dose-dependent effect of polystyrene microplastics on the testicular tissues of the male sprague dawley rats. Dose. Response. 19 (2), 15593258211019882. doi:10.1177/15593258211019882
Ilechukwu, I., Ehigiator, B., Ben, I., Okonkwo, C., Olorunfemi, O., Modo, E. U., et al. (2022). Chronic toxic effects of polystyrene microplastics on reproductive parameters of male rats. Environ. Anal. Health Toxicol. 37 (2), e2022015–e2022010. doi:10.5620/eaht.2022015
Issac, M. N., and Kandasubramanian, B. (2021). Effect of microplastics in water and aquatic systems. Environ. Sci. Pollut. Res. Int. 28 (16), 19544–19562. doi:10.1007/s11356-021-13184-2
Jaikumar, G., Brun, N. R., Vijver, M. G., and Bosker, T. (2019). Reproductive toxicity of primary and secondary microplastics to three cladocerans during chronic exposure. Environ. Pollut. 249, 638–646. doi:10.1016/j.envpol.2019.03.085
Jelnes, J. E. (1988). Semen quality in workers producing reinforced plastic. Reprod. Toxicol. 2 (3-4), 209–212. doi:10.1016/0890-6238(88)90024-x
Jenner, L. C., Rotchell, J. M., Bennett, R. T., Cowen, M., Tentzeris, V., and Sadofsky, L. R. (2022). Detection of microplastics in human lung tissue using μFTIR spectroscopy. Sci. Total Environ. 831, 154907. doi:10.1016/j.scitotenv.2022.154907
Jesus, T. T., Oliveira, P. F., Sousa, M., Cheng, C. Y., and Alves, M. G. (2017). Mammalian target of rapamycin (mTOR): a central regulator of male fertility? Crit. Rev. Biochem. Mol. Biol. 52 (3), 235–253. doi:10.1080/10409238.2017.1279120
Jin, H., Ma, T., Sha, X., Liu, Z., Zhou, Y., Meng, X., et al. (2021). Polystyrene microplastics induced male reproductive toxicity in mice. J. Hazard. Mat. 401, 123430. doi:10.1016/j.jhazmat.2020.123430
Jin, H., Yan, M., Pan, C., Liu, Z., Sha, X., Jiang, C., et al. (2022). Chronic exposure to polystyrene microplastics induced male reproductive toxicity and decreased testosterone levels via the LH-mediated LHR/cAMP/PKA/StAR pathway. Part. Fibre Toxicol. 19 (1), 13–17. doi:10.1186/s12989-022-00453-2
Khan, S., Ahmad, T., Parekh, C. V., Trivedi, P. P., Kushwaha, S., and Jena, G. (2011). Investigation on sodium valproate induced germ cell damage, oxidative stress and genotoxicity in male Swiss mice. Reprod. Toxicol. 32 (4), 385–394. doi:10.1016/j.reprotox.2011.09.007
Kim, J. H., Yu, Y. B., and Choi, J. H. (2021). Toxic effects on bioaccumulation, hematological parameters, oxidative stress, immune responses and neurotoxicity in fish exposed to microplastics: A review. J. Hazard. Mat. 413, 125423. doi:10.1016/j.jhazmat.2021.125423
Kovac, S., Angelova, P. R., Holmstrom, K. M., Zhang, Y., Dinkova-Kostova, A. T., and Abramov, A. Y. (2015). Nrf2 regulates ROS production by mitochondria and NADPH oxidase. Biochim. Biophys. Acta 1850 (4), 794–801. doi:10.1016/j.bbagen.2014.11.021
Kwak, J. I., and An, Y. J. (2021). Microplastic digestion generates fragmented nanoplastics in soils and damages earthworm spermatogenesis and coelomocyte viability. J. Hazard. Mat. 402, 124034. doi:10.1016/j.jhazmat.2020.124034
Leung, M. M., Ho, Y. W., Lee, C. H., Wang, Y., Hu, M., Kwok, K. W. H., et al. (2021). Improved Raman spectroscopy-based approach to assess microplastics in seafood. Environ. Pollut. 289, 117648. doi:10.1016/j.envpol.2021.117648
Li, S., Wang, Q., Yu, H., Yang, L., Sun, Y., Xu, N., et al. (2021). Polystyrene microplastics induce blood-testis barrier disruption regulated by the MAPK-Nrf2 signaling pathway in rats. Environ. Sci. Pollut. Res. Int. 28 (35), 47921–47931. doi:10.1007/s11356-021-13911-9
Li, Y., Wang, J., Yang, G., Lu, L., Zheng, Y., Zhang, Q., et al. (2020). Low level of polystyrene microplastics decreases early developmental toxicity of phenanthrene on marine medaka (Oryzias melastigma). J. Hazard. Mat. 385, 121586. doi:10.1016/j.jhazmat.2019.121586
Li, Y., Yang, G., Wang, J., Lu, L., Li, X., Zheng, Y., et al. (2022). Microplastics increase the accumulation of phenanthrene in the ovaries of marine medaka (Oryzias melastigma) and its transgenerational toxicity. J. Hazard. Mat. 424, 127754. doi:10.1016/j.jhazmat.2021.127754
Lie, P. P., Cheng, C. Y., and Mruk, D. D. (2013). Signalling pathways regulating the blood-testis barrier. Int. J. Biochem. Cell Biol. 45 (3), 621–625. doi:10.1016/j.biocel.2012.12.009
Liu, Y., Zhang, J., Zhao, H., Cai, J., Sultan, Y., Fang, H., et al. (2022). Effects of polyvinyl chloride microplastics on reproduction, oxidative stress and reproduction and detoxification-related genes in Daphnia magna. Comp. Biochem. Physiol. C. Toxicol. Pharmacol. 254, 109269. doi:10.1016/j.cbpc.2022.109269
Liu, Z., Zhuan, Q., Zhang, L., Meng, L., Fu, X., and Hou, Y. (2022). Polystyrene microplastics induced female reproductive toxicity in mice. J. Hazard. Mat. 424, 127629. doi:10.1016/j.jhazmat.2021.127629
Luo, T., Zhang, Y., Wang, C. Y., Wang, X. Y., Zhou, J. J., Shen, M. L., et al. (2019). Maternal exposure to different sizes of polystyrene microplastics during gestation causes metabolic disorders in their offspring. Environ. Pollut. 255, 113122. doi:10.1016/j.envpol.2019.113122
Malafaia, G., de Souza, A. M., Pereira, A. C., Goncalves, S., da Costa Araujo, A. P., Ribeiro, R. X., et al. (2020). Developmental toxicity in zebrafish exposed to polyethylene microplastics under static and semi-static aquatic systems. Sci. Total Environ. 700, 134867. doi:10.1016/j.scitotenv.2019.134867
Martins, A., and Guilhermino, L. (2018). Transgenerational effects and recovery of microplastics exposure in model populations of the freshwater cladoceran Daphnia magna Straus. Sci. Total Environ. 631, 421–428. doi:10.1016/j.scitotenv.2018.03.054
Mok, K. W., Chen, H. Q., Lee, W. M., and Cheng, C. Y. (2015). rpS6 regulates blood-testis barrier dynamics through arp3-mediated actin microfilament organization in rat sertoli cells. An in vitro study. Endocrinology 156 (5), 1900–1913. doi:10.1210/en.2014-1791
Mok, K. W., Mruk, D. D., and Cheng, C. Y. (2014). rpS6 regulates blood-testis barrier dynamics through Akt-mediated effects on MMP-9. J. Cell Sci. 127 (22), 4870–4882. doi:10.1242/jcs.152231
Mok, K. W., Mruk, D. D., Lee, W. M., and Cheng, C. Y. (2013). Rictor/mTORC2 regulates blood-testis barrier dynamics via its effects on gap junction communications and actin filament network. FASEB J. 27 (3), 1137–1152. doi:10.1096/fj.12-212977
Mruk, D. D., and Cheng, C. Y. (2010). Tight junctions in the testis: new perspectives. Philos. Trans. R. Soc. Lond. B Biol. Sci. 365 (1546), 1621–1635. doi:10.1098/rstb.2010.0010
Murphy, F., and Quinn, B. (2018). The effects of microplastic on freshwater Hydra attenuata feeding, morphology & reproduction. Environ. Pollut. 234, 487–494. doi:10.1016/j.envpol.2017.11.029
Napper, I. E., Bakir, A., Rowland, S. J., and Thompson, R. C. (2015). Characterisation, quantity and sorptive properties of microplastics extracted from cosmetics. Mar. Pollut. Bull. 99 (1-2), 178–185. doi:10.1016/j.marpolbul.2015.07.029
Park, E. J., Han, J. S., Park, E. J., Seong, E., Lee, G. H., Kim, D. W., et al. (2020). Repeated-oral dose toxicity of polyethylene microplastics and the possible implications on reproduction and development of the next generation. Toxicol. Lett. 324, 75–85. doi:10.1016/j.toxlet.2020.01.008
Qiang, L., and Cheng, J. (2021). Exposure to polystyrene microplastics impairs gonads of zebrafish (Danio rerio). Chemosphere 263, 128161. doi:10.1016/j.chemosphere.2020.128161
Ragusa, A., Svelato, A., Santacroce, C., Catalano, P., Notarstefano, V., Carnevali, O., et al. (2021). Plasticenta: first evidence of microplastics in human placenta. Environ. Int. 146, 106274. doi:10.1016/j.envint.2020.106274
Ramaswamy, S., and Weinbauer, G. F. (2014). Endocrine control of spermatogenesis: role of FSH and LH/testosterone. Spermatogenesis 4 (2), e996025. doi:10.1080/21565562.2014.996025
Sano, M., Komiyama, H., Shinoda, R., Ozawa, R., Watanabe, H., Karasawa, T., et al. (2022). NLRP3 inflammasome is involved in testicular inflammation induced by lipopolysaccharide in mice. Am. J. Reprod. Immunol. 87 (4), e13527. doi:10.1111/aji.13527
Segovia-Mendoza, M., Nava-Castro, K. E., Palacios-Arreola, M. I., Garay-Canales, C., and Morales-Montor, J. (2020). How microplastic components influence the immune system and impact on children health: focus on cancer. Birth Defects Res. 112 (17), 1341–1361. doi:10.1002/bdr2.1779
Stramucci, L., Pranteda, A., and Bossi, G. (2018). Insights of crosstalk between p53 protein and the MKK3/MKK6/p38 MAPK signaling pathway in cancer. Cancers 10 (5), 131. doi:10.3390/cancers10050131
Sun, J., Wang, D., Lin, J., Liu, Y., Xu, L., Lv, R., et al. (2019). Icariin protects mouse Leydig cell testosterone synthesis from the adverse effects of di (2-ethylhexyl) phthalate. Toxicol. Appl. Pharmacol. 378, 114612. doi:10.1016/j.taap.2019.114612
Sun, S., Jin, Y., Luo, P., and Shi, X. (2022). Polystyrene microplastics induced male reproductive toxicity and transgenerational effects in freshwater prawn. Sci. Total Environ. 842, 156820. doi:10.1016/j.scitotenv.2022.156820
Sussarellu, R., Suquet, M., Thomas, Y., Lambert, C., Fabioux, C., Pernet, M. E. J., et al. (2016). Oyster reproduction is affected by exposure to polystyrene microplastics. Proc. Natl. Acad. Sci. U. S. A. 113 (9), 2430–2435. doi:10.1073/pnas.1519019113
Tarasco, M., Gavaia, P. J., Bensimon-Brito, A., Cordelières, F. P., Santos, T., Martins, G., et al. (2022). Effects of pristine or contaminated polyethylene microplastics on zebrafish development. Chemosphere 303, 135198. doi:10.1016/j.chemosphere.2022.135198
Tong, H., Jiang, Q., Hu, X., and Zhong, X. (2020). Occurrence and identification of microplastics in tap water from China. Chemosphere 252, 126493. doi:10.1016/j.chemosphere.2020.126493
Tremblay, J. J. (2015). Molecular regulation of steroidogenesis in endocrine Leydig cells. Steroids 103, 3–10. doi:10.1016/j.steroids.2015.08.001
United Nations Economic Commission for Europe (UNECE) (2011). “Globally harmonized system of classification and labelling ofChemicals (GHS),” in Part 3. Health and environmental hazards (United Nations, New York/Geneva: Reproductive Toxicity). Chapter 3.7.
Wang, J., Li, Y., Lu, L., Zheng, M., Zhang, X., Tian, H., et al. (2019). Polystyrene microplastics cause tissue damages, sex-specific reproductive disruption and transgenerational effects in marine medaka (Oryzias melastigma). Environ. Pollut. 254, 113024. doi:10.1016/j.envpol.2019.113024
Wang, Y., and Zhang, H. (2019). Regulation of autophagy by mTOR signaling pathway. Adv. Exp. Med. Biol. 1206, 67–83. doi:10.1007/978-981-15-0602-4_3
Wei, Y., Zhou, Y., Long, C., Wu, H., Hong, Y., Fu, Y., et al. (2021). Polystyrene microplastics disrupt the blood-testis barrier integrity through ROS-Mediated imbalance of mTORC1 and mTORC2. Environ. Pollut. 289, 117904. doi:10.1016/j.envpol.2021.117904
Wei, Z., Wang, Y., Wang, S., Xie, J., Han, Q., and Chen, M. (2022). Comparing the effects of polystyrene microplastics exposure on reproduction and fertility in male and female mice. Toxicology 465, 153059. doi:10.1016/j.tox.2021.153059
Wu, H., Xu, T., Chen, T., Liu, J., and Xu, S. (2022). Oxidative stress mediated by the TLR4/NOX2 signalling axis is involved in polystyrene microplastic-induced uterine fibrosis in mice. Sci. Total Environ. 838 (2), 155825. doi:10.1016/j.scitotenv.2022.155825
Xie, X. M., Deng, T., Duan, J. F., Xie, J., Yuan, J. L., and Chen, M. Q. (2020). Exposure to polystyrene microplastics causes reproductive toxicity through oxidative stress and activation of the p38 MAPK signaling pathway. Ecotoxicol. Environ. Saf. 190, 110133. doi:10.1016/j.ecoenv.2019.110133
Yan, W., Hamid, N., Deng, S., Jia, P. P., and Pei, D. S. (2020). Individual and combined toxicogenetic effects of microplastics and heavy metals (Cd, Pb, and Zn) perturb gut microbiota homeostasis and gonadal development in marine medaka (Oryzias melastigma). J. Hazard. Mat. 397, 122795. doi:10.1016/j.jhazmat.2020.122795
Yin, K., Wang, Y., Zhao, H. J., Wang, D. X., Guo, M. H., Mu, M. Y., et al. (2021). A comparative review of microplastics and nanoplastics: toxicity hazards on digestive, reproductive and nervous system. Sci. Total Environ. 774, 145758. doi:10.1016/j.scitotenv.2021.145758
Yu, P., Liu, Z., Wu, D., Chen, M., Lv, W., and Zhao, Y. (2018). Accumulation of polystyrene microplastics in juvenile Eriocheir sinensis and oxidative stress effects in the liver. Aquat. Toxicol. 200, 28–36. doi:10.1016/j.aquatox.2018.04.015
Zhang, J., Wang, L., and Kannan, K. (2020). Microplastics in house dust from 12 countries and associated human exposure. Environ. Int. 134, 105314. doi:10.1016/j.envint.2019.105314
Zhao, H-J., Xu, J-K., Yan, Z-H., Ren, H-Q., and Zhang, Y. (2020). Microplastics enhance the developmental toxicity of synthetic phenolic antioxidants by disturbing the thyroid function and metabolism in developing zebrafish. Environ. Int. 140, 105750. doi:10.1016/j.envint.2020.105750
Zou, W., Xia, M., Jiang, K., Cao, Z., Zhang, X., and Hu, X. (2020). Photo-oxidative degradation mitigated the developmental toxicity of polyamide microplastics to zebrafish larvae by modulating macrophage-triggered proinflammatory responses and apoptosis. Environ. Sci. Technol. 54 (21), 13888–13898. doi:10.1021/acs.est.0c05399
Glossary
AC Adenylyl cyclase
Akt Ak strain transforming
Arp3 Actin-related protein 3
αSMA Alpha-smooth muscle actin
Bax B-cell lymphoma 2–associated X protein
BTB Blood–testes barrier
3β-HSD 3-beta hydroxysteroid dehydrogenase
17β-HSD 17β-hydroxysteroid dehydrogenases
cAMP Cyclic adenosine monophosphate
DNA Deoxyribonucleic acid
FSH Follicle-stimulating hormone
ICR Institute of Cancer Research
JNK c-Jun N-terminal kinase
LH Luteinizing hormone
LHR Luteinizing hormone receptor
MPs Microplastics
p38 MAPK p38 mitogen-activated protein kinase
MMP 9 Matrix metallopeptidase 9
mTOR Mammalian target of rapamycin
mTORC1 mTOR complex 1
mTORC2 mTOR complex 2
NOX 2 NADPH oxidase-2
Nrf2 Nuclear factor erythroid 2-related factor 2
NLRP3 Nod-like receptor family pyrin domain containing 3
P450scc Cholesterol side-chain cleavage enzyme
PE Polyethylene
PET Polyethylene terephthalate
PP Polypropylene
PS Polystyrene
PKA Protein kinase A
ROS Reactive oxygen species
SD Sprague Dawley
rpS6 Ribosomal protein S6
StAR Steroidogenic acute regulatory protein
TGF β Transforming growth factor-β
TLR4 Toll-like receptor 4
UV Ultraviolet
Wnt Wingless-related integration site.
Keywords: BTB-blood–testis barrier, MPs-microplastics, developmental toxicity, signaling, reproductive toxicity
Citation: Dubey I, Khan S and Kushwaha S (2022) Developmental and reproductive toxic effects of exposure to microplastics: A review of associated signaling pathways. Front.Toxicol. 4:901798. doi: 10.3389/ftox.2022.901798
Received: 22 March 2022; Accepted: 03 August 2022;
Published: 31 August 2022.
Edited by:
Terje Svingen, Technical University of Denmark, DenmarkReviewed by:
Yunpeng Hou, China Agricultural University, ChinaMonica Kam Draskau, Technical University of Denmark, Denmark
Copyright © 2022 Dubey, Khan and Kushwaha. This is an open-access article distributed under the terms of the Creative Commons Attribution License (CC BY). The use, distribution or reproduction in other forums is permitted, provided the original author(s) and the copyright owner(s) are credited and that the original publication in this journal is cited, in accordance with accepted academic practice. No use, distribution or reproduction is permitted which does not comply with these terms.
*Correspondence: Sapana Kushwaha, sapna.pharm@gmail.com