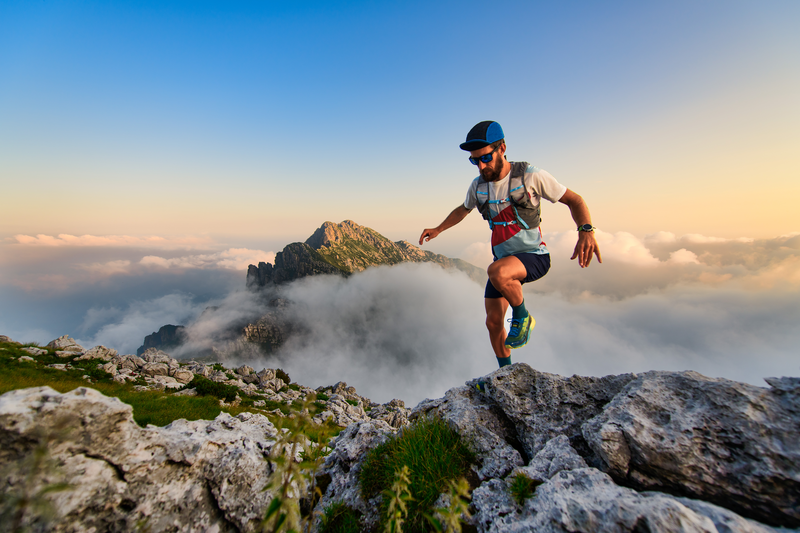
95% of researchers rate our articles as excellent or good
Learn more about the work of our research integrity team to safeguard the quality of each article we publish.
Find out more
REVIEW article
Front. Toxicol. , 20 June 2022
Sec. Regulatory Toxicology
Volume 4 - 2022 | https://doi.org/10.3389/ftox.2022.880818
Cytochrome P450 (CYP) enzymes play a key role in the metabolism of both xenobiotics and endogenous chemicals, and the activity of some CYP isoforms are susceptible to induction and/or inhibition by certain chemicals. As CYP induction/inhibition can bring about significant alterations in the level of in vivo exposure to CYP substrates and metabolites, CYP induction/inhibition data is needed for regulatory chemical toxicity hazard assessment. On the basis of available human in vivo pharmaceutical data, a draft Organisation for Economic Co-operation and Development Test Guideline (TG) for an in vitro CYP HepaRG test method that is capable of detecting the induction of four human CYPs (CYP1A1/1A2, 2B6, and 3A4), has been developed and validated for a set of pharmaceutical proficiency chemicals. However to support TG adoption, further validation data was requested to demonstrate the ability of the test method to also accurately detect CYP induction mediated by industrial and pesticidal chemicals, together with an indication on regulatory uses of the test method. As part of “GOLIATH”, a European Union Horizon-2020 funded research project on metabolic disrupting chemical testing approaches, work is underway to generate supplemental validated data for an additional set of chemicals with sufficient diversity to allow for the approval of the guideline. Here we report on the process of proficiency chemical selection based on a targeted literature review, the selection criteria and considerations required for acceptance of proficiency chemical selection for OECD TG development (i.e. structural diversity, range of activity, relevant chemical sectors, global restrictions etc). The following 13 proposed proficiency chemicals were reviewed and selected as a suitable set for use in the additional validation experiments: tebuconazole, benfuracarb, atrazine, cypermethrin, chlorpyrifos, perfluorooctanoic acid, bisphenol A, N,N-diethyl-m-toluamide, benzo-[a]-pyrene, fludioxonil, malathion, triclosan, and caffeine. Illustrations of applications of the test method in relation to endocrine disruption and non-genotoxic carcinogenicity are provided.
The application of in vitro test method tools for chemical hazard assessment in relation to human health protection is often limited due to insufficient understanding of chemical metabolism, bioactivation or deactivation, and bioavailability.
The liver is a main site of Phase I and Phase II metabolism of endogenous and exogenous substances including nutrients, drugs and chemicals, but many other tissues (such as but not limited to the gut, kidney, placenta) in the body do also have metabolism competency. Phase I metabolism encompasses the biochemical reactions that introduce reactive and polar groups into xenobiotic compounds by oxidation, reduction, or hydrolytic reactions. The major Phase I modifications are catalysed by a large family of Cytochrome P450 (CYP) enzymes (Lewis 2002), and in the first steps, CYPs may transform a xenobiotic into a harmless metabolite (detoxification) that can be easily eliminated via Phase II, or, vice versa, a non-toxic parent chemical may be transformed (metabolically bioactivated) into a toxic metabolite.
CYP induction, that is transcriptional activation/upregulation in CYP gene expression and protein levels, is first triggered by the binding of a chemical to specific nuclear receptors, these constitute the molecular initiation event (MIE). The Aryl hydrocarbon Receptor (AhR), is primarily responsible for the CYP1A and 1B family, the Pregnane X Receptor (PXR) for the CYP3A family and the Constitutive Androstane Receptor (CAR) for the CYP 2B family (Waxman 1999), and the Peroxisome Proliferator Activated receptors (PPARs) for the CYP4A family. These receptors also play major roles in regulating many physiological functions, including hormone and lipid regulation (Kliewer, Lehmann et al., 1999; Jacobs and Lewis 2002).
The CYP1A, 1B, 3A and 2E subfamilies are responsible for the bioactivation of the majority of xenobiotics. For example, with repeated exposure, many chemical carcinogens are bioactivated by CYP1A, indeed such chemicals selectively induce this family, thus exacerbating their carcinogenicity (Ioannides and Lewis 2004; Rendic and Guengerich 2021). Pharmaceuticals, nutrients and some industrial chemicals are mostly activated by CYP3A4, and dietary nutrients/contaminants by CYP1A2, 2E1, and 3A4 (Jacobs and Lewis 2002; Jacobs, Nolan et al., 2005; Hakkola, Hukkanen et al., 2020; Rendic and Guengerich 2021 and references therein). Endogenous substances that are usually components of physiological processes, are mainly activated by CYP1A, 1B1, and 3A enzymes (Rendic and Guengerich 2021). The latter recent review analysing the catalytic activity of CYP families in relation to catalytic bioactivation showed predominant participation of CYP3A4, 1A2, and 1A1, followed by CYP2E1 and 1B1. CYPs 2C9, 2D6, 2A6, 2C19, and 2B6 also having significant participation.
Phase II metabolism often involves the further conjugation of the metabolite with polar molecules, such as sulphate, amino acids, glutathione or glucuronic acid, facilitated by various transferases, generating metabolites that are more soluble to facilitate elimination.
Phase I CYP enzyme induction therefore plays a pivotal initial role in the metabolism of both xenobiotics and endogenous chemicals and constitutes a sensitive biomarker for metabolic competence of in vitro test systems. Chemically mediated induction and/or inhibition of CYPs can lead to marked changes in CYP substrate and metabolite concentrations, and in vitro CYP induction and inhibition data are currently commonly used to predict potential CYP mediated clinical drug interactions for pharmaceuticals (EMA 2012; US FDA, 2020) and are compiled in pharmaceutical CYP induction/inhibition databases (e.g. SIMCYP (Marsousi, Desmeules et al., 2018)).
Thus CYP induction and inhibition data are also needed for a wide number of human health endpoints both for pharmaceutical therapeutic and chemical hazard assessment purposes, for instance ranging from hormone and fatty acid metabolism (Kliewer, Lehmann et al., 1999), hepatotoxicity, and steatosis (Massart, Begriche et al., 2022) to, inflammatory responses (Rubin, Janefeldt et al., 2015), and non-genotoxic carcinogenicity (Ioannides and Lewis 2004; Jacobs, Colacci et al., 2016).
Furthermore, the greater inclusion of CYP induction/inhibition data into chemical hazard assessment will facilitate the shift from regulatory reliance on animal in vivo testing to New Approach Methodologies (NAMs) that refer to and include a battery of relevant in vitro and in silico tools. While the incorporation of metabolic capacity into in vitro genotoxicity testing has been routinely conducted for several decades, in contrast, although discussed a lot, it has not progressed very quickly for other human health endpoints. Illustrations of how the tests could be combined within the OECD conceptual frameworks and Integrated Approaches to Testing and Assessment (IATA) have been developed (Jacobs, Laws et al., 2013; Jacobs, Colacci et al., 2020).
Whilst many chemicals are metabolized by CYPs, enzyme induction data are the focus of the HepaRG CYP enzyme induction test method (Bernasconi, Pelkonen et al., 2019). The need for human CYP induction/inhibition test data to improve the predictive accuracy of in vitro test methods and in silico tools for chemical toxicological hazard assessment, was established by the OECD Test Guideline Programme over 14 years ago, particularly in relation to endocrine disruption (Jacobs, Janssens et al., 2008; OECD 2008). OECD member countries recommended that the human CYP enzyme induction test method was the optimum metabolism test method to take forward for OECD Test Guideline (TG) purposes, and on this basis, validation activities were later initiated and completed for a test method for an in vitro human hepatocyte cell line CYP enzyme induction assay. The assay is capable of detecting an induction of the enzymatic activity of four CYP isoforms (CYP1A1/1A2, 2B6, and 3A4) (Bernasconi, Pelkonen et al., 2019), and was developed and validated using pharmaceutical chemicals. These CYP enzymes are commonly involved in metabolizing drugs and environmental toxicants (Esteves, Rueff et al., 2021), and in producing pharmacokinetic (PK) interactions of medicines (EMA 2012; Ooka, Lynch et al., 2020; US FDA, 2020).
The test method utilizes the metabolic capacity of differentiated cryopreserved immortalized human HepaRG cells coupled with analytical liquid chromatography and mass spectrometry (LC-MS) to quantify the induction of the CYP enzymes based on model substrate conversion (Bernasconi, Pelkonen et al., 2019). To improve human relevance, the study was designed on the basis of human CYP induction evidence, rather than the more plentiful in vivo rodent data. There was sufficient relevant human CYP induction data to allow for an assessment of the human translation potential of the test method, available only for pharmaceuticals (JRC TSAR, 2009). At that time there were no human in vivo data available for other regulatory sector classes (not least because there are ethical issues with respect to pesticide and contaminant testing in controlled human studies). In 2019, a draft TG was submitted for review to the OECD that was based upon the successfully performed and peer reviewed validation data generated using pharmaceutical chemicals. Review feedback was received from the OECD Working Group of National Coordinators of the TG Programme (WNT) that an essential requirement for the approval of the draft TG would be the provision of supporting validation data generated with additional proficiency chemicals representative of chemicals used in other relevant sectors, including industrial chemicals and pesticides. This was because some members of the WNT did not consider that the chemical applicability domain of pharmaceuticals tested in the original validation, gave sufficient coverage of the industrial chemical applicability domain that this test method is intended to be applied to. Due to the lack of primary human data for non-pharmaceuticals, the WNT accepted a compromise proposal on how to utilise the wider chemical metabolism data in the scientific literature, using metabolism data generated from relevant human cell lines, for example. This data was utilised to generate a chemical selection list, to supplement the reference/proficiency chemical list in the original HepaRG CYP enzyme induction test method validation, and the review and selection process is described in this paper. This list of suitable proficiency chemicals is the basis for augmenting the chemical applicability domain of the test method. This additional validation data is being generated on the HepaRG CYP enzyme induction test method, within “GOLIATH”, a European Union Horizon-2020 funded research project on metabolic disrupting chemical testing approaches.
Here we provide details on the selected chemicals, the method and supporting data used for selecting the chemicals, and an overview of intended regulatory applications of the test method.
The chemical and structural diversity of the proficiency chemicals used for (pre)validation needs to address both the chemical applicability domain of the chemical Universe for which the test method is intended to predict endpoint-specific toxicity, but also be structurally relevant for the biological role of the endpoint. In addition, in many cases, where known, natural and endogenous ligands should be included in the chemical selection (Waxman 1999; Jacobs and Lewis 2002) as these are the ligands that the anthropogenic chemicals of concern are mimicking.
A targeted reiterative but not systematic literature search was carried out to identify an initial selection pool of candidate proficiency chemicals for which human CYP modulation data was available for CYP1A1/1A2, CYP2B6, and CYP3A4, and that belonged to OECD TG Programme relevant chemical classes (including industrial chemicals, pesticides, and food additives). Data sources from both human cell line in vitro and human in vivo studies relating to specified CYP induction and directly related receptors were sourced and critically evaluated, but due to the general scarcity of in vivo human studies carried out with non-pharmaceutical chemicals, only human in vitro data was available for most chemicals. Support that this would be an acceptable approach to take was first established within the OECD WNT, given the scarcity of human in vivo data. In some cases, it was appropriate to use rodent data for weight of evidence support.
On this regulatory acceptable basis, between 2017 and 2021, Scopus and pubmed search engines were queried with respect to human relevant data, cytochrome P450, and chemicals, including pesticides/bacteriocides and excluding pharmaceuticals as these are already addressed in the draft TG. The results were filtered and prioritised for human relevant data including human relevant cell lines, e.g. HepaRG, HepG2, and the relevant CYPs in the draft validated TG, together with relevant references within the papers. These were critically reviewed and double checked by the authors and then external regulatory experts for the OECD TG Programme (WNT).
In the validated test method, overall CYP enzymatic activity is quantified in an in vitro human hepatocyte cell line (cryopreserved HPR116 differentiated HepaRG cells) before and after pretreatment with test chemicals, by measuring the rate of metabolic conversion of substrates that are selective for CYP1A1/1A2 (phenacetin to acetaminophen), CYP2B6 (bupropion to hydroxybupropion), and CYP3A4 (midazolam to 1′-hydroxymidazolam) using a LC-MS analytical technique (Bernasconi, Pelkonen et al., 2019). In such cell systems, the overall effect on enzyme activity is dependent on the extent to which a chemical up or downregulates not only CYP mRNA/protein levels, but also functional enzymatic activity. Data evaluated in the literature search therefore included in vitro mRNA/protein quantity and enzyme activity data generated using human cells, as well as enzyme activity data generated in human liver microsomes (HLMs) and recombinant enzyme preparations (REPS). CYP1A1/1A2 gene expression is well understood and extensively documented to be induced by activation of the AhR (e.g. Bock 2014; Vogel, Van Winkle et al., 2020), and activation of CAR and PXR has been shown to induce CYP2B6 and CYP3A4 (Tolson and Wang 2010; Wang, Ong et al., 2012). Data showing activation of these receptors was taken to indicate likely mRNA/protein upregulation of the respective CYPs. Preliminary assessment as to whether a candidate chemical is likely to induce, inhibit, or have no effect on a specific CYP in the test method was made on the basis of enzyme activity data from human cell systems with innate CYP expression, when this data was available. Where this data was not available, estimations were made based on available mRNA/protein quantity data and enzyme activity data from non-cell preparations, when possible. In the latter case, estimations were considered to be of lower reliability as compared to cases where cell-based enzymatic activity data was available, and insufficient data were considered to be available to estimate the effect of a chemical in the test method if overall the available good quality data was considered to be contradictory. In the design of validation experiments intended for applications beyond classification and prioritisation purposes, it is good practice to include proficiency chemicals that are expected to produce a potency range from negative to low, moderate, and strong effects, and to generate concentration-response information, as this is of greater utility for IATA approaches (Jacobs, Ezendam et al., 2022). Thus, for chemicals for which cell-based enzymatic activity data showed them to be an inducer, the magnitude of any observed CYP enzyme induction was categorized as low (≤3 fold), moderate (>3 to 4.5 fold), or strong (>4.5 fold), and this information was used in the chemical selection considerations. When cell-based enzymatic data was absent (not tested), the magnitude of an expected effect was categorized as uncertain.
As the conversion of phenacetin to acetaminophen is catalyzed by both CYP1A1 and 1A2 (Kcat = 0.84 and 2.2 min−1, respectively) (Huang, Deshmukh et al., 2012), production of acetaminophen is used as an overall marker of both CYP1A1 and 1A2 activity in the CYP enzyme induction assay; and CYP1A1/1A2 activity predictions were thus made based on the cumulative available data for both CYP1A1 and 1A2. Formation of 1′-hydroxymidazolam is widely used as a selective marker of CYP3A4 activity. The latter reaction is catalyzed to a significant extent by both CYP3A4 and 3A5 (Vmax = 35 and 72 nmol/min/nmol CYP, and Km = 5 and 14 μM, respectively) (Williams, Ring et al., 2002), but the HepaRG cell line contains two CYP3A5*3 alleles, which are known to be null due to expressed RNA instability (Jackson, Li et al., 2016). It is expected that CYP3A4/5 activity in HepaRG cells is predominantly attributable to CYP3A4 activity. Hydroxybupropion formation, on the other hand, has been shown to be catalyzed nearly exclusively by CYP2B6 (Faucette, Hawke et al., 2000; Hesse, Venkatakrishnan et al., 2000), with other CYP isoforms (including CYP2C19 and 3A4) being involved to only a negligible extent (Faucette, Hawke et al., 2001; Sager, Price et al., 2016).
As it is anticipated that on becoming an OECD TG, the test method will be widely utilised internationally, and will fall under the Mutual Acceptance of Data agreement for the OECD member country regulatory jurisdictions, it is important that proficiency chemicals should be associated with the minimum possible transport, supply and usage restrictions, and accommodate national and international limitations on use. Chemicals that are excessively expensive to procure or that have restricted availability in OECD member countries were therefore avoided when possible. Examples of chemicals with restricted availabilities include all chemicals listed under the Stockholm Convention on Persistent Organic Pollutants (POPs) (http://chm.pops.int/TheConvention/ThePOPs/TheNewPOPs/tabid/2511/Default.aspx, accessed 10 December 2021), and certain classes of controlled substances, such as drugs with abuse potential for example anabolic steroids and cannabinols. Chemical mixtures containing undefined or variable chemical constituents, including (stereo)isomers and racemic mixtures were also avoided when possible, due to the potential for between batch variability. Data and data sources that were considered to be of inadequate quality, due to lack of information regarding the successful establishment of the assay(s), or poor reproducibility (Taxvig 2020; Franzosa, Bonzo et al., 2021), were not utilised.
From the candidate pool of chemicals, a proposed set of proficiency validation chemicals was selected to enable adequate coverage of structural diversity but also a representative selection of chemicals from relevant sectors (including industrial chemicals, pesticides, and food additives) that was practically possible, on the basis of publicly available scientific literature. Importantly, to be able to fully evaluate the functioning and reliability of the test method, the proposed proficiency chemicals were also chosen to try to ensure the inclusion of a sufficient number of negative chemicals (a minimum of 25% of total tested), with the range of positive chemicals that would adequately probe the ability of the test method to detect individual induction of each of the four measured CYPs (CYP1A1/1A2, CYP2B6, and CYP3A4).
Using a targeted literature search and the selection criteria detailed in the methodology section, an initial pool of potential proficiency candidates consisting of a total of 23 chemicals were identified, and a tabular listing of these chemicals which includes summaries of available CYP activity data and chemical structure images are provided in Table 1, Parts A and B. From these candidates, on the basis of the review exercise, the following 13 chemicals were selected to augment the current proficiency chemical list, consisting of pharmaceuticals and to be proposed as additional proficiency chemicals for further validation experiments: tebuconazole, benfuracarb, atrazine, cypermethrin, chlorpyrifos, perfluorooctanoic acid (PFOA), bisphenol A (BPA), N,N-diethyl-m-toluamide (DEET), benzo-[a]-pyrene (B [a]P), fludioxonil, malathion, triclosan and caffeine (See Table 1 Part A). These 13 chemicals have a diverse range of structures and molecular weights and include representative examples of industrial chemicals, pesticides, and food and cosmetics additives. Excepting caffeine, all the chemicals display a degree of lipophilicity (experimental log P ranging from 1.97 to 7.75). Any potential solubility issues will be evaluated and addressed by solubility and cytotoxicity assessment that are part of the planned validation augmentation study design. These data will be reported following completion of the planned experiments.
TABLE 1. Part A = Proposed Set of Additional Industrial, Pesticidal, and Food Additive Proficiency Chemicals to Use in Further CYP Induction Validation Experiments. Part B = Initial Candidate Selection Pool Chemicals that were Evaluated but Not Selected. The magnitude of any observed CYP enzyme induction was categorized as low (≤3 fold), moderate (>3 to 4.5 fold), and or strong (>4.5 fold), when data from cell-based enzymatic activity assays was available. When cell-based enzymatic data was absent (not tested), the magnitude of an expected effect was categorized as uncertain. Data on induction of mRNA or protein was not used to estimate magnitudes of effect.
The selected set contains the following number of expected enzyme inducers: 7 x CYP1A1/1A2 (four low, two strong, one uncertain), 6 x CYP2B6 (two low, two moderate, two uncertain), and 8 x CYP3A4 (four low, two moderate, one strong, one uncertain). These will allow for a sufficient evaluation of the ability of the assay to accurately measure the activity of all four CYP isoforms that are covered by this assay. Additionally, the selected set also contains two expected inhibitors for CYP1A1, CYP2B6, and CYP3A4, which will allow also for an evaluation of the performance of the test method in detecting CYP enzyme inhibitors. For validation experiments, whilst it is considered good practice to include a proficiency chemical set that contains at least 25% of negative chemicals, unfortunately it was not possible to fully meet this criterion for this augmented chemical set, as sufficient data regarding no CYP activity was only available for two of the 23 candidate pool chemicals. Therefore, the augmentation chemical set contains two chemicals that are expected to have no effect on CYP1A1/1A2 activity, but no additional non pharmaceutical chemicals that are expected to have no effect on CYP2B6 or CYP3A4 activity. Following the additional chemical augmentation validation confirmatory testing, the relative potencies of the chemicals in Table 2 will be consolidated.
TABLE 2. Set of Proficiency Pharmaceutical Chemicals that have been Evaluated in Experiments Carried out by Bernasconi, Pelkonen et al., 2019 to Validate the In Vitro CYP Induction Assay in Primary Human Hepatocytes and HepaRG cells.
Validation data for the test method has previously been generated for the 12 pharmaceutical proficiency chemicals shown in Table 2, and in silico chemical space evaluations are reported to demonstrate that the structures of the latter pharmaceuticals are representative of EU REACH (https://echa.europa.eu/regulations/reach/legislation), Drugbank, and Tox21 listed chemicals (Bernasconi, Pelkonen et al., 2019). Overall, the total set of 25 proficiency chemicals (including the 12 pharmaceuticals and the proposed 13 additional industrial, pesticidal, and food additive chemicals) is considered to be sufficiently diverse and representative of OECD TG Programme relevant chemical classes to facilitate draft TG approval at the OECD.
PK, including absorption, distribution, metabolism, and excretion (ADME) play a key role in determining in vivo exposure to a parent chemical and its metabolites after dosing, and PK data is used extensively in the design and interpretation of toxicological assessments of test chemicals. Currently, toxicokinetic ADME data for single and repeated dose in vivo studies (OECD 2010) is commonly generated and used in Europe for high tonnage industrial chemicals and for pesticides and worldwide for pharmaceuticals (ICH S3A, 1994). Relevant data from several in vitro ADME assays, including, for example, Caco-2 cell permeability assays measuring absorption potential; transporter protein substrate/inhibition assays which provide data on distribution, excretion, and PK interactions; S9 and microsome addition and in vitro metabolism assay data can contribute on a weight of evidence basis to the toxicological hazard assessment. However, when the data is generated according to an accepted OECD TG as part of the Mutual Acceptance of Data agreement, this data can be submitted without additional testing to many OECD regulatory jurisdictions. The process of validation is intended to establish the relevance, reproducibility and reliability of a test method for a specific regulatory purpose (OECD 2005) and gives much greater confidence in the reliability of the test data generated.
That there is an urgent need to develop new OECD TGs for these in vitro test method is well established at the OECD (Jacobs, Janssens et al., 2008; OECD 2008; Jacobs, Laws et al., 2013; Bernasconi, Pelkonen et al., 2019), as data from these assays could be used alongside standard in vivo PK data in a complimentary fashion to aid toxicological assessments. Importantly, several quantitative in vitro to in vivo extrapolation (QIVIVE) and in silico physiology-based pharmacokinetic (PBPK) models have been shown to be capable of accurately predicting in vivo PK parameters from in vitro data (OECD, 2021; Tsaioun, Blaauboer et al., 2016), and it is hoped that in the future QIVIVE and PBPK approaches could replace certain types of in vivo PK data, which would allow for a significant reduction in animal use.
With regards to metabolism, the availability of additional validated in vitro assays capable of generating human relevant metabolic profiling and CYP induction/inhibition data would be particularly valuable. Animal relevant metabolic profile data from non-human in vivo studies is often available. However, to date, there are no adopted TGs available for in vitro test method that produce human metabolic profile data, which hampers efforts focused on determining the relevance of animal metabolite data to humans. Moreover, for results from in vitro toxicity assays to accurately predict potential in vivo toxicity, it is essential that the concentrations of parent chemical/metabolites that are tested in vitro are representative of in vivo levels, and, for this reason, metabolic transformation steps are included in many in vitro toxicity assays, including all of the OECD TG in vitro genotoxicity test method. At the moment, there are, however, a number of in vitro OECD TGs that lack appropriate (pre-)incubation steps to account for in vivo metabolism, including, for example, all of the Level 2 in vitro mechanistic human cell based test method specified in the current OECD Endocrine Disruptor Guidance Document 150 (OECD 2018). Moreover, for many chemicals, there are significant differences in metabolism between rats and humans, and only rat S9 microsomes are used to produce metabolic transformation in the OECD genotoxicity TGs. In a recent European Food Safety Authority (EFSA) scientific panel opinion paper relating to the toxicological testing of pesticides, the use of in vitro human metabolite data is recommended to identify any potential human relevant metabolites that had not been adequately tested in non-human toxicological studies (EFSA 2021).
CYP induction data can indicate whether and to what extent a chemical is likely to undergo CYP-mediated metabolism, and results showing significant CYP induction could be used as an indicator that (pre-)incubation steps to account for in vivo metabolism should be included in any toxicity assays lacking metabolic competence. In relevant situations, CYP induction and inhibition data could facilitate the selection of optimal in vivo test chemical doses for human and other animal studies, and also indicate the possible involvement of CYP metabolism/metabolites in adverse or PK effects. Perturbations in the levels of endogenous chemicals that are metabolised by CYPs are associated with several adverse effects, and CYP induction data could also be used to support the contribution of CYP-mediated mechanisms in adverse outcome pathways and IATAs as shown in Figure 1B. Furthermore, the performance of a number of QIVIVE/PBPK models and PK databases such as MetaPath (Kolanczyk, Schmieder et al., 2012) would be substantially expanded by the incorporation of available CYP induction data. It would be particularly beneficial to generate CYP data for food chemical classes for example, as in Europe, little mammalian in vivo data is available for these.
FIGURE 1. Selected illustrations of regulatory applications of in vitro metabolism test method/systems falling within the OECD TG Programme. panel (A) Introduction of metabolism in vitro testing at Level 2 of the OECD Conceptual Framework for Testing and Assessment of Endocrine Disrupting Chemicals (EDCs).
Moreover, CYP induction and inhibition data are currently commonly used to predict potential CYP mediated clinical PK drug interactions for pharmaceuticals (EMA 2012; US FDA, 2020) and the availability and use of a validated and adopted OECD CYP induction test method, that will fall under the Mutual Acceptance of Data agreement, will therefore also be valuable for drug discovery and regulatory application for pharmaceutical CYP drug interaction evaluations.
Relevant in vitro metabolism data would greatly benefit several specific IATAs, including the developmental neurotoxicity (Bal-Price, Crofton et al., 2015) and non-genotoxic carcinogenicity (Jacobs, Colacci et al., 2020) IATAs currently under development, and the OECD Conceptual Framework for Endocrine Disruptors (updated OECD 2018). With respect to the latter, as proposed in 2013 (Jacobs, Laws et al., 2013), in vitro metabolism test method can be added at level 2 of the Endocrine Disruptor Conceptual Framework as shown in Figure 1A,B below, but will also have great utility in informing all levels of the Conceptual Framework. This will begin to accommodate the additional in vitro assay flexibility needs for the regulatory identification of endocrine disruptors (Solecki, Kortenkamp et al., 2017), by filling the metabolism translational gap between in vitro level 2 assays and the WHO definition of endocrine disruptor, as “an exogenous substance or mixture that alters the function(s) of the endocrine system and consequently causes adverse effects in an intact organism, or its progeny, or (sub) populations”. Quoting from the consensus paper ‘a) Alterations of the function of the endocrine system may arise from interaction with hormone receptors, changes in circulating levels of the hormone, and from the impact of chemical(s) on hormone synthesis, transport, metabolism and other factors’. c) The term “intact organism” is understood to mean that the effect would occur in vivo, either observable in a test animal system, epidemiologically or clinically. However, it does not necessarily mean that the adverse effect has to be demonstrated in an intact test animal, but may be shown in adequately validated alternative test systems predictive of adverse effects in humans and/or wildlife‘ (Solecki, Kortenkamp et al., 2017).
In addition to mediating detoxification, CAR, PXR and AhR have been implicated in the regulation of a broader range of physiological functions (Kretschmer and Baldwin 2005; Wang and Tompkins 2008; Yi, Fashe et al., 2020), where dysregulation can lead to adverse effects (Hakkola, Bernasconi et al., 2018) and receptor and CYP induction have well documented roles for instance in inflammation (Christmas, 2015; Rubin, Janefeldt et al., 2015), cholestasis, steatosis (Gomez-Lechon, Jover et al., 2009), hepatotoxicity (Woolbright and Jaeschke 2015), carcinogenesis (De Mattia, Cecchin et al., 2016; Pondugula, Pavek et al., 2016; Fucic, Guszak et al., 2017), and thyroid disruption (OECD 2006; OECD 2014). Thus, in these cases, induction of specified CYP enzymes may serve as a biomarker for key events associated with adverse health effects. In addition, the use of CYP induction data, in combination with assays that can address foetal and early life chemical exposure mediated via the placenta, such as the hPlacentox human placental JEG-3 cell line model (Rat, Olivier et al., 2017; Olivier, Wakx et al., 2021) will assist regulatory in vitro tool development, reducing metabolism uncertainties and improving the evidence base to address ‘disruption of the programming role of hormones during prenatal and postnatal development [that] can cause adverse effects that do not become evident until later in life’ (Solecki, Kortenkamp et al., 2017). CYP3A induction increases by approximately 2-fold during pregnancy, and has been shown to be mediated by cortisol plasma concentrations during pregnancy (Sachar, Kelly et al., 2019).
CYP induction testing is a critical initial testing aspect in IATAs such as that for non-genotoxic carcinogenicity (Figure 1B).
The HepaRG CYP enzyme induction test method as validated thus far can address CYP1A, 3A4 and 2B6, as primary targets. Looking forward, assays for additional CYP isoforms will be needed for specific IATAs, as identified in Figure 1B, and for metabolism evaluations for chemicals that are metabolised by other inducible CYPs. For instance, an assay for CYP2E1 is being assessed within the OECD non-genotoxic carcinogenicity IATA expert group. Furthermore, among the xenobiotic-metabolizing CYPs, CYP2D6 results in a large contribution of genetic variation to the interindividual variation in CYP enzyme activity (Ingelman-Sundberg, Sim et al., 2007), and an FDA guideline on in vitro drug interaction testing of pharmaceuticals recommends also evaluating induction of CYP2C8, 2C9, 2C19 (US FDA, 2020). Thus, while inclusion of additional CYP enzymes (such as CYP2D6) and contribution of nuclear receptors such as the glucocorticoid receptor (GR) which induces CYP2D6 (Farooq, Kelly et al., 2016), and PPARs (which induce CYP4A, Kliewer, Lehmann et al., 1999, Waxman, 1999) would be useful to explore in the near future, the scope of this study was to augment the chemical applicability domain of the validated draft TG without de novo validation of additional targets.
It is noted that GR is functional in the HepaRG cell line (Hart, Li et al., 2010; Farooq, Kelly et al., 2016; Sachar, Kelly et al., 2019) and thus subsequent additional testing with glucocorticoids (such as prednisone, prednisolone, cortisone, corticosterone, dexamethasone, betamethasone, triamcinolone, 6-methylprednisolone, 21-deoxy cortisol, deflazacort and hydrocortisol) could be run in the assay to develop further model applications, to ascertain glucocorticoid activity via GR, especially when running the CYP HepaRG assay in relation to in vitro GR transactivation and placental models, or for inclusion of such information in a metabolic disruption IATA.
Moving beyond molecular initiating events, to subsequent key events, there will need to be specific considerations for the direct and indirect CYP activity in relation to inflammation for example, for carcinogenicity.
Following successful adoption and application of the HepaRG CYP enzyme induction test method, it would be useful to develop complementary components of in vitro human metabolism systems into OECD TGs, including induction/inhibition assays for additional CYP isoforms, and assays for Phase II metabolism, metabolite profiling, and metabolic transformation pre-incubation steps (as discussed above). The use of human microsomes and/or S9 mixes, or cryopreserved primary human hepatocytes (PHH) have utility in research and drug discovery, but for chemical hazard assessment purposes, cryopreserved PHH with very wide ranging variability in responses, had reproducibility issues in a validation exercise (Bernasconi, Pelkonen et al., 2019), and following OECD peer review, were considered too variable for TG development. There are also (unknown) viral transmission concerns with the use of primary human tissues in routine chemical testing.
The HepaRG CYP enzyme induction test method was agreed by the OECD member countries to be the best (longer term) option to take forward for regulatory use in 2008 (Jacobs, Janssens et al., 2008; OECD 2008; Jacobs, Laws et al., 2013), and following successful validation with pharmaceuticals (Bernasconi, Pelkonen et al., 2019) it is the most ready and reliable system available, and superior in validation performance to cryopreserved hepatocytes. A variety of additional non validated in vitro human liver on a chip and 3D liver organoid systems are currently in development, but the complexity, between batch variability, and lack of validation data for these models (Telles-Silva, Pacheco et al., 2022) mean that they are not ready for test guideline development at present. However available literature evidence indicates that HepaRG cells are well suited to all of these applications, as mRNA for many key proteins involved in xenobiotic metabolism are expressed in differentiated HepaRG cells, including xenobiotic sensing nuclear receptors (AhR, PXR, CAR, and PPAR-α), CYPs (CYP1A1, 1A2, 2A6, 2B6, 2C8, 2C9, 2C19, 2D6, 2E1, 3A4, 3A5, 3A7), other Phase I metabolic enzymes [including various isoforms of alcohol dehydrogenase, aldehyde dehydrogenase, and flavin-containing monooxygenase], and Phase II metabolic enzymes [including various isoforms of glutathione S-transferase, UDP-glucuronosyltransferase, N-acetyltransferase, and sulfotransferase] (Aninat, Piton et al., 2006; Guillouzo, Corlu et al., 2007; Josse, Aninat et al., 2008; Antherieu, Chesne et al., 2010; Hart, Li et al., 2010). Enzymatic activity for CYP1A1/1A2, 2B6, 2C8, 2C9, 2C19, 2D6, 2E1, and 3A4 has also been confirmed to be present (Aninat, Piton et al., 2006; Josse, Aninat et al., 2008; Antherieu, Chesne et al., 2010; Lubberstedt, Muller-Vieira et al., 2011). Furthermore, chemical-induced induction and inhibition of CYP1A1/1A2, 2A6, 2B6, 2C8, 2C9, 2C19, 2D6, 3A4 has been demonstrated in HepaRG cells (Aninat, Piton et al., 2006; Josse, Aninat et al., 2008; Kanebratt and Andersson 2008; Turpeinen, Tolonen et al., 2009; Antherieu, Chesne et al., 2010; Yajima, Uno et al., 2014). The metabolic profile data generated for several chemicals in HepaRG cells have also been shown to be equivalent to that produced using PHH, including aflatoxin B1 and acetaminophen (Aninat, Piton et al., 2006) but without the inherent biological variability observed with different batches of PHH. Intrinsic clearance values generated for a large number of reference drugs in HepaRG cells have also been shown to be equivalent to the values generated in PHH (Lubberstedt, Muller-Vieira et al., 2011; Zanelli, Caradonna et al., 2012). In the future a potentially promising approach to explore would therefore be to develop the HepaRG cell system into an all-in-one system/assay providing all the desired abovementioned in vitro metabolic functionalities.
For now however, it is really important to first address the outstanding steps required to enable the successful adoption of the HepaRG CYP enzyme induction test method as a TG, and the work described herein provides the essential concrete chemical selection and applications step required to enable international TG progress within an immediate timeframe. Following the planned additional validation experiments with this candidate chemical selection list there will be an evidence basis upon which to refine the chemical list for use as additional proficiency chemicals for the HepaRG CYP enzyme induction test method, and to do any further analyses that may be warranted.
Overall, it is apparent that the availability of CYP induction data would significantly aid the toxicological assessment of chemicals, and our ongoing work to augment the chemical applicability domain as an extension to the validation of the CYP enzyme induction HepaRG test method is a requirement for the (near) future approval of this test method, at the OECD.
Here we have also given some examples of immediate applications of the assay for the OECD Conceptual Framework for Endocrine Disruptors, and for the OECD IATA for non-genotoxic carcinogens. There will also be necessary applications to other complex human health IATAs, including that for thyroid disruption (OECD, 2014), and metabolic disruption (Legler, Zalko et al., 2020).
MNJ conceived, instigated and developed the project, discussion and acquired funding. BK expanded the original database, which was then supplemented by EB, both of whom also contributed to the discussion. All authors contributed to manuscript drafting, revision, and read and approved the submitted version.
This project has received funding from the European Union’s Horizon 2020 research and innovation programme under grant agreement No. 825489.
This output reflects the views only of the author(s), and the European Union cannot be held responsible for any use which may be made of the information contained therein.
The authors declare that the research was conducted in the absence of any commercial or financial relationships that could be construed as a potential conflict of interest.
All claims expressed in this article are solely those of the authors and do not necessarily represent those of their affiliated organizations, or those of the publisher, the editors and the reviewers. Any product that may be evaluated in this article, or claim that may be made by its manufacturer, is not guaranteed or endorsed by the publisher.
The authors gratefully acknowledge the support received from Camilla Bernasconi, Sandra Coecke, European Commission Joint Research Centre, Italy and the constructive comments from Betty Hakkert, RIVM, The Netherlands, and Knud Ladegaard Pedersen, The Danish Environmental Protection Agency, Denmark on the selection of non-pharmaceutical chemicals and screening the Danish QSAR database. Many thanks also to Christophe Chesné, Biopredic International, for useful comments on the draft manuscript.
AChE, Acetylcholinesterase; ADME, Absorption, Distribution, Metabolism, and Excretion; AhR, Aryl Hydrocarbon Receptor; B[a]P, Benzo-[a]-Pyrene; BPA, Bisphenol A; CAR, Constitutive Androstane Receptor; CYP, Cytochrome P450; DEET, N,N-Diethyl-m-Toluamide; DHMB, 2,3-Dihydroxy-4-Methoxybenzaldehyde; EDCs, Endocrine Disrupting Chemicals; EFSA, European Food Safety Authority; EROD, Ethoxyresorufin-O-Deethylase; HLM, Human Liver Microsomes; IATA, Integrated Approach to Testing and Assessment; ICH, International Conference on Harmonisation of Technical Requirements for Registration of Pharmaceuticals for Human Use; LC-MS, Liquid Chromatography-Mass Spectrometry; LOAEL, Lowest Observed Adverse Effect Level; MIE, Molecular Initiation Event; NAM, New Approach Methodologies; OECD, Organisation for Economic Cooperation and Development; PBPK, Physiology-Based Pharmacokinetic; PFOA, Perfluorooctanoic Acid; PHH, Primary Human Hepatocytes; PK, Pharmacokinetics; POPs, Persistent Organic Pollutants; PPARs, Peroxisome Proliferator Activated Receptors; PXR, Pregnane X Receptor; QIVIVE, Quantitative In Vitro to In Vivo Extrapolation; REPS, Recombinant Enzyme Preparations; TBBPA, Tetra Brominated Bisphenol A; TG, OECD Test Guideline; WNT, OECD Working Group of National Coordinators of the Test Guideline Programme.
Abass, K., Lämsä, V., Reponen, P., Küblbeck, J., Honkakoski, P., Mattila, S., et al. (2012). Characterization of Human Cytochrome P450 Induction by Pesticides. Toxicology 294 (1), 17–26. doi:10.1016/j.tox.2012.01.010
Abass, K., and Pelkonen, O. (2013). The Inhibition of Major Human Hepatic Cytochrome P450 Enzymes by 18 Pesticides: Comparison of the N-In-One and Single Substrate Approaches. Toxicol. Vitro 27 (5), 1584–1588. doi:10.1016/j.tiv.2012.05.003
Abass, K., Reponen, P., Mattila, S., Rautio, A., and Pelkonen, O. (2014). Human Variation and CYP Enzyme Contribution in Benfuracarb Metabolism in Human In Vitro Hepatic Models. Toxicol. Lett. 224 (2), 300–309. doi:10.1016/j.toxlet.2013.08.023
Abass, K., Turpeinen, M., and Pelkonen, O. (2009). An Evaluation of the Cytochrome P450 Inhibition Potential of Selected Pesticides in Human Hepatic Microsomes. J. Environ. Sci. Health, Part B 44 (6), 553–563. doi:10.1080/03601230902997766
Abe, T., Takahashi, M., Kano, M., Amaike, Y., Ishii, C., Maeda, K., et al. (2017). Activation of Nuclear Receptor CAR by an Environmental Pollutant Perfluorooctanoic Acid. Arch. Toxicol. 91 (6), 2365–2374. doi:10.1007/s00204-016-1888-3
Allmyr, M., Panagiotidis, G., Sparve, E., Diczfalusy, U., and Sandborgh-Englund, G. (2009). Human Exposure to Triclosan via Toothpaste Does Not Change CYP3A4 Activity or Plasma Concentrations of Thyroid Hormones. Basic & Clin. Pharmacol. Toxicol. 105 (5), 339–344. doi:10.1111/j.1742-7843.2009.00455.x
Andersson, T., RÖhss, K., Bredberg, E., and Hassan-Alin, M. (2001). Pharmacokinetics and Pharmacodynamics of Esomeprazole, the S-Isomer of Omeprazole. Aliment. Pharmacol. Ther. 15 (10), 1563–1569. doi:10.1046/j.1365-2036.2001.01087.x
Aninat, C., Piton, A., Glaise, D., Le Charpentier, T., Langouët, S., Morel, F., et al. (2006). Expression of Cytochromes P450, Conjugating Enzymes and Nuclear Receptors in Human Hepatoma HepaRG Cells. Drug Metab. Dispos. 34 (1), 75–83. doi:10.1124/dmd.105.006759
Anthérieu, S., Chesné, C., Li, R., Camus, S., Lahoz, A., Picazo, L., et al. (2010). Stable Expression, Activity, and Inducibility of Cytochromes P450 in Differentiated HepaRG Cells. Drug Metab. Dispos. 38 (3), 516–525. doi:10.1124/dmd.109.030197
Asimus, S., Elsherbiny, D., Hai, T. N., Jansson, B., Huong, N. V., Petzold, M. G., et al. (2007). Artemisinin Antimalarials Moderately Affect Cytochrome P450 Enzyme Activity in Healthy Subjects. Fundam. Clin. Pharmacol. 21 (3), 307–316. doi:10.1111/j.1472-8206.2007.00471.x
Agency for Toxic Substances and Disease Registry (2017). Division of Toxicology and Human Health Sciences. Atlanta, GA: Environmental Toxicology Branch.
Backman, J. T., Granfors, M. T., and Neuvonen, P. J. (2006). Rifampicin Is Only a Weak Inducer of CYP1A2-Mediated Presystemic and Systemic Metabolism: Studies with Tizanidine and Caffeine. Eur. J. Clin. Pharmacol. 62 (6), 451–461. doi:10.1007/s00228-006-0127-x
Bal-Price, A., Crofton, K. M., Leist, M., Allen, S., Arand, M., Buetler, T., et al. (2015). International STakeholder NETwork (ISTNET): Creating a Developmental Neurotoxicity (DNT) Testing Road Map for Regulatory Purposes. Arch. Toxicol. 89 (2), 269–287. doi:10.1007/s00204-015-1464-2
Barditchcrovo, P., Trapnell, C., Ette, E., Zacur, H., Coresh, J., Rocco, L., et al. (1999). The Effects of Rifampin and Rifabutin on the Pharmacokinetics and Pharmacodynamics of a Combination Oral Contraceptive. Clin. Pharmacol. Ther. 65 (4), 428–438. doi:10.1016/s0009-9236(99)70138-4
Begas, E., Kouvaras, E., Tsakalof, A., Papakosta, S., and Asprodini, E. K. (2007). In Vivo evaluation of CYP1A2, CYP2A6, NAT-2 and Xanthine Oxidase Activities in a Greek Population Sample by the RP-HPLC Monitoring of Caffeine Metabolic Ratios. Biomed. Chromatogr. 21 (2), 190–200. doi:10.1002/bmc.736
Behr, A.-C., Kwiatkowski, A., Ståhlman, M., Schmidt, F. F., Luckert, C., Braeuning, A., et al. (2020a). Impairment of Bile Acid Metabolism by Perfluorooctanoic Acid (PFOA) and Perfluorooctanesulfonic Acid (PFOS) in Human HepaRG Hepatoma Cells. Arch. Toxicol. 94 (5), 1673–1686. doi:10.1007/s00204-020-02732-3
Behr, A.-C., Plinsch, C., Braeuning, A., and Buhrke, T. (2020b). Activation of Human Nuclear Receptors by Perfluoroalkylated Substances (PFAS). Toxicol. Vitro 62, 104700. doi:10.1016/j.tiv.2019.104700
Berger, B., Bachmann, F., Duthaler, U., Krähenbühl, S., and Haschke, M. (2018). Cytochrome P450 Enzymes Involved in Metoprolol Metabolism and Use of Metoprolol as a CYP2D6 Phenotyping Probe Drug. Front. Pharmacol. 9, 774. doi:10.3389/fphar.2018.00774
Bernasconi, C., Pelkonen, O., Andersson, T. B., Strickland, J., Wilk-Zasadna, I., Asturiol, D., et al. (2019). Validation of In Vitro Methods for Human Cytochrome P450 Enzyme Induction: Outcome of a Multi-Laboratory Study. Toxicol. Vitro 60, 212–228. doi:10.1016/j.tiv.2019.05.019
Birkett, D., Miners, J., and Attwood, J. (1983). Evidence for a Dual Action of Sulphinpyrazone on Drug Metabolism in Man: Theophylline-Sulphinpyrazone Interaction. Br. J. Clin. Pharmacol. 15 (5), 567–569. doi:10.1111/j.1365-2125.1983.tb02093.x
Bjork, J. A., Butenhoff, J. L., and Wallace, K. B. (2011). Multiplicity of Nuclear Receptor Activation by PFOA and PFOS in Primary Human and Rodent Hepatocytes. Toxicology 288 (1-3), 8–17. doi:10.1016/j.tox.2011.06.012
Bock, K. W. (2014). Homeostatic Control of Xeno- and Endobiotics in the Drug-Metabolizing Enzyme System. Biochem. Pharmacol. 90 (1), 1–6. doi:10.1016/j.bcp.2014.04.009
Braeuning, A., Mentz, A., Schmidt, F. F., Albaum, S. P., Planatscher, H., Kalinowski, J., et al. (2020). RNA-Protein Correlation of Liver Toxicity Markers in HepaRG Cells. EXCLI J. 19, 135–153. doi:10.17179/excli2019-2005
Caboni, P., Sherer, T. B., Zhang, N., Taylor, G., Na, H. M., Greenamyre, J. T., et al. (2004). Rotenone, Deguelin, Their Metabolites, and the Rat Model of Parkinson's Disease. Chem. Res. Toxicol. 17 (11), 1540–1548. doi:10.1021/tx049867r
Christmas, P. (2015). Role of Cytochrome P450s in Inflammation. Adv. Pharmacol. 74, 163–192. doi:10.1016/bs.apha.2015.03.005
Corrales, J., Kristofco, L. A., Steele, W. B., Yates, B. S., Breed, C. S., Williams, E. S., et al. (2015). Global Assessment of Bisphenol A in the Environment: Review and Analysis of its Occurrence and Bioaccumulation. Dose Response 13 (3), 1559325815598308. doi:10.1177/1559325815598308
Crawford, P., Chadwick, D., Martin, C., Tjia, J., Back, D., and Orme, M. (1990). The Interaction of Phenytoin and Carbamazepine with Combined Oral Contraceptive Steroids. Br. J. Clin. Pharmacol. 30 (6), 892–896. doi:10.1111/j.1365-2125.1990.tb05457.x
D'Agostino, J., Zhang, H., Kenaan, C., and Hollenberg, P. F. (2015). Mechanism-Based Inactivation of Human Cytochrome P450 2B6 by Chlorpyrifos. Chem. Res. Toxicol. 28 (7), 1484–1495. doi:10.1021/acs.chemrestox.5b00156
Das, P. C., Cao, Y., Rose, R. L., Cherrington, N., and Hodgson, E. (2008a). Enzyme Induction and Cytotoxicity in Human Hepatocytes by Chlorpyrifos and N,N-Diethyl-M-Toluamide (DEET). Drug Metabol. Drug Interact. 23 (3-4), 237–260. doi:10.1515/dmdi.2008.23.3-4.237
Das, P. C., Streit, T. M., Cao, Y., Rose, R. L., Cherrington, N., Ross, M. K., et al. (2008b). Pyrethroids: Cytotoxicity and Induction of CYP Isoforms in Human Hepatocytes. Drug Metabol. Drug Interact. 23 (3-4), 211–236. doi:10.1515/dmdi.2008.23.3-4.211
Das, P. C., Cao, Y., Cherrington, N., Hodgson, E., and Rose, R. L. (2006). Fipronil Induces CYP Isoforms and Cytotoxicity in Human Hepatocytes. Chemico-Biological Interact. 164 (3), 200–214. doi:10.1016/j.cbi.2006.09.013
De Mattia, E., Cecchin, E., Roncato, R., and Toffoli, G. (2016). Pregnane X Receptor, Constitutive Androstane Receptor and Hepatocyte Nuclear Factors as Emerging Players in Cancer Precision Medicine. Pharmacogenomics 17 (14), 1547–1571. doi:10.2217/pgs-2016-0095
De Wilde, A. R., Heyndrickx, A., and Carton, D. (1986). A Case of Fatal Rotenone Poisoning in a Child. J. Forensic Sci. 31 (4), 1492–1498. doi:10.1520/jfs11931j
Delescluse, C., Ledirac, N., de Sousa, G., Pralavorio, M., Lesca, P., and Rahmani, R. (1998). Cytotoxic Effects and Induction of Cytochromes P450 1A1/2 by Insecticides, in Hepatic or Epidermal Cells: Binding Capability to the Ah Receptor. Toxicol. Lett. 96-97, 33–39. doi:10.1016/s0378-4274(98)00047-2
Diconsiglio, E., Meneguz, A., and Testai, E. (2005). Organophosphorothionate Pesticides Inhibit the Bioactivation of Imipramine by Human Hepatic Cytochrome P450s. Toxicol. Appl. Pharmacol. 205 (3), 237–246. doi:10.1016/j.taap.2004.10.009
Dingemanse, J., and van Giersbergen, P. L. M. (2004). Clinical Pharmacology of Bosentan, a Dual Endothelin Receptor Antagonist. Clin. Pharmacokinet. 43 (15), 1089–1115. doi:10.2165/00003088-200443150-00003
EFSA (2011). Conclusion on the Peer Review of the Pesticide Risk Assessment of the Active Substance Prochloraz. Online, EFSA. 9 (7), 2323.
EFSA (2021). Scientific Opinion of the Scientific Panel on Plant Protection Products and Their Residues (PPR Panel) on Tesing and Interpretation of Comparative In Vitro Metabolism Studies. EFSA J. 19 (12), e06970.
EFSA (2019). Statement on the Available Outcomes of the Human Health Assessment in the Context of the Pesticides Peer Review of the Active Substance Chlorpyrifos-Methyl. EFSA J. 17 (8), e05810. doi:10.2903/j.efsa.2019.5810
Elsherbiny, D. A., Asimus, S. A., Karlsson, M. O., Ashton, M., and Simonsson, U. S. H. (2008). A Model Based Assessment of the CYP2B6 and CYP2C19 Inductive Properties by Artemisinin Antimalarials: Implications for Combination Regimens. J. Pharmacokinet. Pharmacodyn. 35 (2), 203–217. doi:10.1007/s10928-008-9084-6
Ema, (2012). Guideline on the Investigation of Drug Interactions - Revision 1. London, United Kingdom: European Medicines Agency. 59.
Emoto, C., Murase, S., Sawada, Y., Jones, B. C., and Iwasaki, K. (2003). In Vitro Inhibitory Effect of 1-Aminobenzotriazole on Drug Oxidations Catalyzed by Human Cytochrome P450 Enzymes: A Comparison with SKF-525A and Ketoconazole. Drug Metabolism Pharmacokinet. 18 (5), 287–295. doi:10.2133/dmpk.18.287
Esteves, F., Rueff, J., and Kranendonk, M. (2021). The Central Role of Cytochrome P450 in Xenobiotic Metabolism-A Brief Review on a Fascinating Enzyme Family. JoX 11 (3), 94–114. doi:10.3390/jox11030007
Faniband, M., Ekman, E., Littorin, M., Maxe, M., Larsson, E., and Lindh, C. H. (2019). Biomarkers of Exposure to Pyrimethanil After Controlled Human Experiments. J. Anal. Toxicol. 43 (4), 277–283. doi:10.1093/jat/bky091
Farooq, M., Kelly, E. J., and Unadkat, J. D. (2016). CYP2D6 Is Inducible by Endogenous and Exogenous Corticosteroids. Drug Metabolism Dispos. 44 (5), 750–757. doi:10.1124/dmd.115.069229
Faucette, S. R., Hawke, R. L., Lecluyse, E. L., Shord, S. S., Yan, B., Laethem, R. M., et al. (2000). Validation of Bupropion Hydroxylation as a Selective Marker of Human Cytochrome P450 2B6 Catalytic Activity. Drug Metab. Dispos. 28 (10), 1222–1230.
Faucette, S. R., Hawke, R. L., Shord, S. S., Lecluyse, E. L., and Lindley, C. M. (2001). Evaluation of the Contribution of Cytochrome P450 3A4 to Human Liver Microsomal Bupropion Hydroxylation. Drug Metab. Dispos. 29 (8), 1123–1129.
Fellay, J., Marzolini, C., Decosterd, L., Golay, K. P., Baumann, P., Buclin, T., et al. (2005). Variations of CYP3A Activity Induced by Antiretroviral Treatment in HIV-1 Infected Patients. Eur. J. Clin. Pharmacol. 60 (12), 865–873. doi:10.1007/s00228-004-0855-8
Ferland, S., Côté, J., Ratelle, M., Thuot, R., and Bouchard, M. (2015). Detailed Urinary Excretion Time Courses of Biomarkers of Exposure to Permethrin and Estimated Exposure in Workers of a Corn Production Farm in Quebec, Canada. Annhyg. 59 (9), 1152–1167. doi:10.1093/annhyg/mev059
Franco, M. E., Sutherland, G. E., Fernandez-Luna, M. T., and Lavado, R. (2020). Altered Expression and Activity of Phase I and II Biotransformation Enzymes in Human Liver Cells by Perfluorooctanoate (PFOA) and Perfluorooctane Sulfonate (PFOS). Toxicology 430, 152339. doi:10.1016/j.tox.2019.152339
Franzosa, J. A., Bonzo, J. A., Jack, J., Baker, N. C., Kothiya, P., Witek, R. P., et al. (2021). High-Throughput Toxicogenomic Screening of Chemicals in the Environment Using Metabolically Competent Hepatic Cell Cultures. npj Syst. Biol. Appl. 7 (1), 7. doi:10.1038/s41540-020-00166-2
Fucic, A., Guszak, V., and Mantovani, A. (2017). Transplacental Exposure to Environmental Carcinogens: Association with Childhood Cancer Risks and the Role of Modulating Factors. Reprod. Toxicol. 72, 182–190. doi:10.1016/j.reprotox.2017.06.044
Fustinoni, S., Mercadante, R., Polledri, E., Rubino, F. M., Mandic-Rajcevic, S., Vianello, G., et al. (2014). Biological Monitoring of Exposure to Tebuconazole in Winegrowers. J. Expo. Sci. Environ. Epidemiol. 24 (6), 643–649. doi:10.1038/jes.2014.14
Garfitt, S. J., Jones, K., Mason, H. J., and Cocker, J. (2002). Development of a Urinary Biomarker for Exposure to the Organophosphate Propetamphos: Data from an Oral and Dermal Human Volunteer Study. Biomarkers 7 (2), 113–122. doi:10.1080/13547500110112775
Germer, S., Piersma, A. H., van der Ven, L., Kamyschnikow, A., Fery, Y., Schmitz, H. J., et al. (2006). Subacute Effects of the Brominated Flame Retardants Hexabromocyclododecane and Tetrabromobisphenol A on Hepatic Cytochrome P450 Levels in Rats. Toxicology 218 (2), 229–236. doi:10.1016/j.tox.2005.10.019
Gillum, J. G., Sesler, J. M., Bruzzese, V. L., Israel, D. S., and Polk, R. E. (1996). Induction of Theophylline Clearance by Rifampin and Rifabutin in Healthy Male Volunteers. Antimicrob. Agents Chemother. 40 (8), 1866–1869. doi:10.1128/aac.40.8.1866
Goedtke, L., John, A., Lampen, A., Seidel, A., Braeuning, A., and Hessel-Pras, S. (2021). Mixture Effects of Food-Relevant Polycyclic Aromatic Hydrocarbons on the Activation of Nuclear Receptors and Gene Expression, Benzo[a]pyrene Metabolite Profile and DNA Damage in HepaRG Cells. Food Chem. Toxicol. 147, 111884. doi:10.1016/j.fct.2020.111884
Gomez-Lechon, M., Jover, R., and Donato, M. (2009). Cytochrome P450 and Steatosis. Cdm 10 (7), 692–699. doi:10.2174/138920009789895543
Gramec Skledar, D., Tomašič, T., Carino, A., Distrutti, E., Fiorucci, S., and Peterlin Mašič, L. (2016). New Brominated Flame Retardants and Their Metabolites as Activators of the Pregnane X Receptor. Toxicol. Lett. 259, 116–123. doi:10.1016/j.toxlet.2016.08.005
Guillouzo, A., Corlu, A., Aninat, C., Glaise, D., Morel, F., and Guguen-Guillouzo, C. (2007). The Human Hepatoma HepaRG Cells: A Highly Differentiated Model for Studies of Liver Metabolism and Toxicity of Xenobiotics. Chemico-Biological Interact. 168 (1), 66–73. doi:10.1016/j.cbi.2006.12.003
Hakkola, J., Bernasconi, C., Coecke, S., Richert, L., Andersson, T. B., and Pelkonen, O. (2018). Cytochrome P450 Induction and Xeno-Sensing Receptors Pregnane X Receptor, Constitutive Androstane Receptor, Aryl Hydrocarbon Receptor and Peroxisome Proliferator-Activated Receptor α at the Crossroads of Toxicokinetics and Toxicodynamics. Basic Clin. Pharmacol. Toxicol. 123 (Suppl. 5), 42–50. doi:10.1111/bcpt.13004
Hakkola, J., Hukkanen, J., Turpeinen, M., and Pelkonen, O. (2020). Inhibition and Induction of CYP Enzymes in Humans: An Update. Arch. Toxicol. 94 (11), 3671–3722. doi:10.1007/s00204-020-02936-7
Hart, S. N., Li, Y., Nakamoto, K., Subileau, E.-a., Steen, D., and Zhong, X.-b. (2010). A Comparison of Whole Genome Gene Expression Profiles of HepaRG Cells and HepG2 Cells to Primary Human Hepatocytes and Human Liver Tissues. Drug Metab. Dispos. 38 (6), 988–994. doi:10.1124/dmd.109.031831
Härtter, S., Nordmark, A., Rose, D.-M., Bertilsson, L., Tybring, G., and Laine, K. (2003). Effects of Caffeine Intake on the Pharmacokinetics of Melatonin, a Probe Drug for CYP1A2 Activity. Br. J. Clin. Pharmacol. 56 (6), 679–682. doi:10.1046/j.1365-2125.2003.01933.x
Herman, D., Locatelli, I., Grabnar, I., Peternel, P., Stegnar, M., Lainščak, M., et al. (2006). The Influence of Co-Treatment with Carbamazepine, Amiodarone and Statins on Warfarin Metabolism and Maintenance Dose. Eur. J. Clin. Pharmacol. 62 (4), 291–296. doi:10.1007/s00228-006-0104-4
Hesse, L. M., Venkatakrishnan, K., Court, M. H., von Moltke, L. L., Duan, S. X., Shader, R. I., et al. (2000). CYP2B6 Mediates the In Vitro Hydroxylation of Bupropion: Potential Drug Interactions with Other Antidepressants. Drug Metab. Dispos. 28 (10), 1176–1183.
Hodgson, E., and Rose, R. L. (2007). Human Metabolic Interactions of Environmental Chemicals. J. Biochem. Mol. Toxicol. 21 (4), 182–186. doi:10.1002/jbt.20175
Horita, Y., and Doi, N. (2014). Comparative Study of the Effects of Antituberculosis Drugs and Antiretroviral Drugs on Cytochrome P450 3A4 and P-Glycoprotein. Antimicrob. Agents Chemother. 58 (6), 3168–3176. doi:10.1128/aac.02278-13
Hu, Q., and Hartmann, R. W. (2014). The Renaissance of CYP17 Inhibitors for the Treatment of Prostate Cancer. Cancer Drug Des. Discov. 1, 319–356. doi:10.1016/b978-0-12-396521-9.00011-5
Huang, Q., Deshmukh, R. S., Ericksen, S. S., Tu, Y., and Szklarz, G. D. (2012). Preferred Binding Orientations of Phenacetin in CYP1A1 and CYP1A2 Are Associated with Isoform-Selective Metabolism. Drug Metab. Dispos. 40 (12), 2324–2331. doi:10.1124/dmd.112.047308
ICH S3A (1994). Note for Guidance on Toxicokinetics: The Assessment of Systemic Exposure in Toxicity Studies. Geneva, Switzerland: European Medicines Agency. Available at: https://database.ich.org/sites/default/files/S3A_Guideline.pdf.
Ingelman-Sundberg, M., Sim, S. C., Gomez, A., and Rodriguez-Antona, C. (2007). Influence of Cytochrome P450 Polymorphisms on Drug Therapies: Pharmacogenetic, Pharmacoepigenetic and Clinical Aspects. Pharmacol. Ther. 116, 496–526. doi:10.1016/j.pharmthera.2007.09.004
Ioannides, C., and V. Lewis, D. (2004). Cytochromes P450 in the Bioactivation of Chemicals. Ctmc 4 (16), 1767–1788. doi:10.2174/1568026043387188
Jackson, J. P., Li, L., Chamberlain, E. D., Wang, H., and Ferguson, S. S. (2016). Contextualizing Hepatocyte Functionality of Cryopreserved HepaRG Cell Cultures. Drug Metabolism Dispos. 44 (9), 1463–1479. doi:10.1124/dmd.116.069831
Jacobs, M., Ezendam, J., Hakkert, B., and Oelgeschlaeger, M. (2022). Potential of Concentration-Response Data to Broaden Regulatory Application of In Vitro Test Guidelines. Altex 39 (2), 315–321. doi:10.14573/altex.2107091
Jacobs, M., Janssens, W., Bernauer, U., Brandon, E., Coecke, S., Combes, R., et al. (2008). The Use of Metabolising Systems for In Vitro Testing of Endocrine Disruptors. Cdm 9 (8), 796–826. doi:10.2174/138920008786049294
Jacobs, M., Laws, S. C., Willett, K., Schmieder, P., Odum, J., and Bovee, T. F. (2013). In Vitro Metabolism and Bioavailability Tests for Endocrine Active Substances: What Is Needed Next for Regulatory Purposes? Altex 30 (3), 331–351. doi:10.14573/altex.2013.3.331
Jacobs, M. N., Colacci, A., Louekari, K., Luijten, M., Hakkert, B. C., Paparella, M., et al. (2016). International Regulatory Needs for Development of an IATA for Non-Genotoxic Carcinogenic Chemical Substances. Altex 33 (4), 359–392. doi:10.14573/altex.1601201
Jacobs, M. N., Colacci, A., Corvi, R., Vaccari, M., Aguila, M. C., Corvaro, M., et al. (2020). Chemical Carcinogen Safety Testing: OECD Expert Group International Consensus on the Development of an Integrated Approach for the Testing and Assessment of Chemical Non-Genotoxic Carcinogens. Arch. Toxicol. 94 (8), 2899–2923. doi:10.1007/s00204-020-02784-5
Jacobs, M. N., Nolan, G. T., and Hood, S. R. (2005). Lignans, Bacteriocides and Organochlorine Compounds Activate the Human Pregnane X Receptor (PXR). Toxicol. Appl. Pharmacol. 209 (2), 123–133. doi:10.1016/j.taap.2005.03.015
Jacobs*, M. N., and Lewis, D. F. V. (2002). Steroid Hormone Receptors and Dietary Ligands: A Selected Review. Proc. Nutr. Soc. 61 (1), 105–122. doi:10.1079/pns2001140
Jennen, D. G. J., Magkoufopoulou, C., Ketelslegers, H. B., van Herwijnen, M. H. M., Kleinjans, J. C. S., and van Delft, J. H. M. (2010). Comparison of HepG2 and HepaRG by Whole-Genome Gene Expression Analysis for the Purpose of Chemical Hazard Identification. Toxicol. Sci. 115 (1), 66–79. doi:10.1093/toxsci/kfq026
Ji, P., Damle, B., Xie, J., Unger, S. E., Grasela, D. M., and Kaul, S. (2008). Pharmacokinetic Interaction Between Efavirenz and Carbamazepine After Multiple-Dose Administration in Healthy Subjects. J. Clin. Pharmacol. 48 (8), 948–956. doi:10.1177/0091270008319792
Jossé, R., Aninat, C., Glaise, D., Dumont, J., Fessard, V., Morel, F., et al. (2008). Long-Term Functional Stability of Human HepaRG Hepatocytes and Use for Chronic Toxicity and Genotoxicity Studies. Drug Metab. Dispos. 36 (6), 1111–1118. doi:10.1124/dmd.107.019901
Josse, R., Sharanek, A., Savary, C. C., and Guillouzo, A. (2014). Impact of Isomalathion on Malathion Cytotoxicity and Genotoxicity in Human HepaRG Cells. Chemico-Biological Interact. 209, 68–76. doi:10.1016/j.cbi.2013.12.002
Jrc Tsar, (2009). Cytochrome P450 (CYP) Enzyme Induction in Vitro Method Using Cryopreserved Differentiated Human HepaRG™ Cells (Test Method Number: TM2009-14). Retrieved from https://tsar.jrc.ec.europa.eu/test-method/tm2009-14.
Kanebratt, K., Diczfalusy, U., Bäckström, T., Sparve, E., Bredberg, E., Böttiger, Y., et al. (2008). Cytochrome P450 Induction by Rifampicin in Healthy Subjects: Determination Using the Karolinska Cocktail and the Endogenous CYP3A4 Marker 4β-Hydroxycholesterol. Clin. Pharmacol. Ther. 84 (5), 589–594. doi:10.1038/clpt.2008.132
Kanebratt, K. P., and Andersson, T. B. (2008). HepaRG Cells as an In Vitro Model for Evaluation of Cytochrome P450 Induction in Humans. Drug Metab. Dispos. 36 (1), 137–145. doi:10.1124/dmd.107.017418
Ketter, T. A., Jenkins, J. B., Schroeder, D. H., Pazzaglia, P. J., Marangell, L. B., George, M. S., et al. (1995). Carbamazepine but Not Valproate Induces Bupropion Metabolism. J. Clin. Psychopharmacol. 15 (5), 327–333. doi:10.1097/00004714-199510000-00004
Kim, H. J., Choi, M. S., Rehman, S. U., Ji, Y. S., Yu, J. S., Nakamura, K., et al. (2019). Determination of Urinary Caffeine Metabolites as Biomarkers for Drug Metabolic Enzyme Activities. Nutrients 11 (8), 1947. doi:10.3390/nu11081947
Kliewer, S. A., Lehmann, J. M., Milburn, M. V., and Willson, T. M. (1999). The PPARs and PXRs: Nuclear Xenobiotic Receptors That Define Novel Hormone Signaling Pathways. Recent Prog. Horm. Res. 54, 345–348.
Knebel, C., Heise, T., Zanger, U. M., Lampen, A., Marx-Stoelting, P., and Braeuning, A. (2019). The Azole Fungicide Tebuconazole Affects Human CYP1A1 and CYP1A2 Expression by an Aryl Hydrocarbon Receptor-Dependent Pathway. Food Chem. Toxicol. 123, 481–491. doi:10.1016/j.fct.2018.11.039
Knebel, C., Neeb, J., Zahn, E., Schmidt, F., Carazo, A., Holas, O., et al. (2018). Unexpected Effects of Propiconazole, Tebuconazole, and Their Mixture on the Receptors CAR and PXR in Human Liver Cells. Toxicol. Sci. 163 (1), 170–181. doi:10.1093/toxsci/kfy026
Kolanczyk, R. C., Schmieder, P., Jones, W. J., Mekenyan, O. G., Chapkanov, A., Temelkov, S., et al. (2012). MetaPath: An Electronic Knowledge Base for Collating, Exchanging and Analyzing Case Studies of Xenobiotic Metabolism. Regul. Toxicol. Pharmacol. 63 (1), 84–96. doi:10.1016/j.yrtph.2012.02.013
Korashy, H. M., Shayeganpour, A., Brocks, D. R., and El-Kadi, A. O. S. (2007). Induction of Cytochrome P450 1A1 by Ketoconazole and Itraconazole but Not Fluconazole in Murine and Human Hepatoma Cell Lines. Toxicol. Sci. 97 (1), 32–43. doi:10.1093/toxsci/kfm012
Kot, M., and Daniel, W. A. (2008a). Caffeine as a Marker Substrate for Testing Cytochrome P450 Activity in Human and Rat. Pharmacol. Rep. 60 (6), 789–797.
Kot, M., and Daniel, W. A. (2008b). The Relative Contribution of Human Cytochrome P450 Isoforms to the Four Caffeine Oxidation Pathways: An In Vitro Comparative Study with cDNA-Expressed P450s Including CYP2C Isoforms. Biochem. Pharmacol. 76 (4), 543–551. doi:10.1016/j.bcp.2008.05.025
Kretschmer, X. C., and Baldwin, W. S. (2005). CAR and PXR: Xenosensors of Endocrine Disrupters? Chemico-Biological Interact. 155 (3), 111–128. doi:10.1016/j.cbi.2005.06.003
Kuzbari, O., Peterson, C. M., Franklin, M. R., Hathaway, L. B., Johnstone, E. B., Hammoud, A. O., et al. (2013). Comparative Analysis of Human CYP3A4 and Rat CYP3A1 Induction and Relevant Gene Expression by Bisphenol A and Diethylstilbestrol: Implications for Toxicity Testing Paradigms. Reprod. Toxicol. 37, 24–30. doi:10.1016/j.reprotox.2013.01.005
Lasch, A., Marx-Stoelting, P., Braeuning, A., and Lichtenstein, D. (2021). More Than Additive Effects on Liver Triglyceride Accumulation by Combinations of Steatotic and Non-Steatotic Pesticides in HepaRG Cells. Arch. Toxicol. 95 (4), 1397–1411. doi:10.1007/s00204-021-02997-2
Lawrie, R. D., Mitchell, R. D., Dhammi, A., Wallace, A., Hodgson, E., and Roe, R. M. (2020). Role of Long Non-Coding RNA in DEET- and Fipronil-Mediated Alteration of Transcripts Associated with Phase I and Phase II Xenobiotic Metabolism in Human Primary Hepatocytes. Pesticide Biochem. Physiology 167, 104607. doi:10.1016/j.pestbp.2020.104607
Legler, J., Zalko, D., Jourdan, F., Jacobs, M., Fromenty, B., Balaguer, P., et al. (2020). The GOLIATH Project: Towards an Internationally Harmonised Approach for Testing Metabolism Disrupting Compounds. Int. J. Mol. Sci. 21 (10), 3480. doi:10.3390/ijms21103480
Lemaire, G., de Sousa, G., and Rahmani, R. (2004). A PXR Reporter Gene Assay in a Stable Cell Culture System: CYP3A4 and CYP2B6 Induction by Pesticides. Biochem. Pharmacol. 68 (12), 2347–2358. doi:10.1016/j.bcp.2004.07.041
Lemaire, G., Mnif, W., Pascussi, J.-M., Pillon, A., Rabenoelina, F., Fenet, H., et al. (2006). Identification of New Human Pregnane X Receptor Ligands Among Pesticides Using a Stable Reporter Cell System. Toxicol. Sci. 91 (2), 501–509. doi:10.1093/toxsci/kfj173
Lewis, D. V. F. (2002). Guide to Cytochromes P450 Structure and Function. London, United Kingdom: Taylor & Francis. ISBN 9780748408979, pp 240.
Lin, J. H. (2006). CYP Induction-Mediated Drug Interactions: In Vitro Assessment and Clinical Implications. Pharm. Res. 23 (6), 1089–1116. doi:10.1007/s11095-006-0277-7
Loboz, K., Gross, A., Williams, K., Liauw, W., Day, R., Blievernicht, J., et al. (2006). Cytochrome P450 2B6 Activity as Measured by Bupropion Hydroxylation: Effect of Induction by Rifampin and Ethnicity. Clin. Pharmacol. Ther. 80 (1), 75–84. doi:10.1016/j.clpt.2006.03.010
López-Cortés, L. F., Ruiz-Valderas, R., Viciana, P., Alarcón-González, A., Gómez-Mateos, J., León-Jimenez, E., et al. (2002). Pharmacokinetic Interactions Between Efavirenz and Rifampicin in HIV-Infected Patients with Tuberculosis. Clin. Pharmacokinet. 41 (9), 681–690. doi:10.2165/00003088-200241090-00004
Lübberstedt, M., Müller-Vieira, U., Mayer, M., Biemel, K. M., Knöspel, F., Knobeloch, D., et al. (2011). HepaRG Human Hepatic Cell Line Utility as a Surrogate for Primary Human Hepatocytes in Drug Metabolism Assessment In Vitro. J. Pharmacol. Toxicol. Methods 63 (1), 59–68. doi:10.1016/j.vascn.2010.04.013
Lucas, R. A., Gilfillan, D. J., and Bergstrom, R. F. (1998). A Pharmacokinetic Interaction Between Carbamazepine and Olanzapine: Observations on Possible Mechanism. Eur. J. Clin. Pharmacol. 54 (8), 639–643. doi:10.1007/s002280050527
Luckert, C., Ehlers, A., Buhrke, T., Seidel, A., Lampen, A., and Hessel, S. (2013). Polycyclic Aromatic Hydrocarbons Stimulate Human CYP3A4 Promoter Activity via PXR. Toxicol. Lett. 222 (2), 180–188. doi:10.1016/j.toxlet.2013.06.243
Marsousi, N., Desmeules, J. A., Rudaz, S., and Daali, Y. (2018). Prediction of Drug-Drug Interactions Using Physiologically-Based Pharmacokinetic Models of CYP450 Modulators Included in Simcyp Software. Biopharm. Drug Dispos. 39 (1), 3–17. doi:10.1002/bdd.2107
Massart, J., Begriche, K., Hartman, J. H., and Fromenty, B. (2022). Role of Mitochondrial Cytochrome P450 2E1 in Healthy and Diseased Liver. Cells 11 (2). doi:10.3390/cells11020288
Medjakovic, S., Zoechling, A., Gerster, P., Ivanova, M. M., Teng, Y., Klinge, C. M., et al. (2014). Effect of Nonpersistent Pesticides on Estrogen Receptor, Androgen Receptor, and Aryl Hydrocarbon Receptor. Environ. Toxicol. 29 (10), 1201–1216. doi:10.1002/tox.21852
Miller, M., Cosgriff, J., Kwong, T., and Morken, D. A. (1984). Influence of Phenytoin on Theophylline Clearance. Clin. Pharmacol. Ther. 35 (5), 666–669. doi:10.1038/clpt.1984.92
Mitchell, R. D., Dhammi, A., WallaceHodgson, A. E., and Roe, E. R. M. (2016). Impact of Environmental Chemicals on the Transcriptome of Primary Human Hepatocytes: Potential for Health Effects. J. Biochem. Mol. Toxicol. 30 (8), 375–395. doi:10.1002/jbt.21801
Moreland, T., Park, B., and Rylance, G. (1982). Microsomal Enzyme Induction in Children: The Influence of Carbamazepine Treatment on Antipyrine Kinetics, 6 Beta-Hydroxycortisol Excretion and Plasma Gamma-Glutamyltranspeptidase Activity. Br. J. Clin. Pharmacol. 14 (6), 861–865. doi:10.1111/j.1365-2125.1982.tb02050.x
Mouly, S., Lown, K. S., Kornhauser, D., Joseph, J. L., Fiske, W. D., Benedek, I. H., et al. (2002). Hepatic but Not Intestinal CYP3A4 Displays Dose-Dependent Induction by Efavirenz in Humans*. Clin. Pharmacol. Ther. 72 (1), 1–9. doi:10.1067/mcp.2002.124519
National Research Council (Us), (1994). Subcommittee to Review Permethrin Toxicity from Military Uniforms. Washington, DC: The National Academies Press. doi:10.17226/9274
Ngaimisi, E., Mugusi, S., Minzi, O. M., Sasi, P., Riedel, K.-D., Suda, A., et al. (2010). Long-Term Efavirenz Autoinduction and its Effect on Plasma Exposure in HIV Patients. Clin. Pharmacol. Ther. 88 (5), 676–684. doi:10.1038/clpt.2010.172
Niwa, T., Tsutsui, M., Kishimoto, K., Yabusaki, Y., Ishibashi, F., and Katagiri, M. (2000). Inhibition of Drug-Metabolizing Enzyme Activity in Human Hepatic Cytochrome P450s by Bisphenol A. Biol. Pharm. Bull. 23 (4), 498–501. doi:10.1248/bpb.23.498
Novotna, A., Korhonova, M., Bartonkova, I., Soshilov, A. A., Denison, M. S., Bogdanova, K., et al. (2014a). Enantiospecific Effects of Ketoconazole on Aryl Hydrocarbon Receptor. PLoS One 9 (7), e101832. doi:10.1371/journal.pone.0101832
Novotná, A., Krasulová, K., Bartoňková, I., Korhoňová, M., Bachleda, P., Anzenbacher, P., et al. (2014b). Dual Effects of Ketoconazole Cis-Enantiomers on CYP3A4 in Human Hepatocytes and HepG2 Cells. PLoS One 9 (10), e111286. doi:10.1371/journal.pone.0111286
OECD (2020a). Case Study on the Use of Integrated Approaches for Testing and Assessment for Identification and Characterisation of Parkinsonian Hazard Liability of Deguelin by an AOP-Based Testing and Read-Across Approach. Paris, France: ENV/JM/MONO(2020)22. Series on Testing and Assessment. Available at: https://www.oecd.org/officialdocuments/publicdisplaydocumentpdf/?cote=ENV/JM/MONO(2020)22&docLanguage=en.
OECD (2008). Detailed Review Paper on the Use of Metabolising Systems for in Vitro Testing of Endocrine Disruptors, OECD Series on Testing and Assessment, No. 97. Paris: OECD Publishing. Available at: https://www.oecd.org/officialdocuments/publicdisplaydocumentpdf/?cote=env/jm/mono(2008)24&doclanguage=en.
OECD (2006). Detailed Review Paper on Thyroid Hormone Disruption Assays OECD Series on Testing and Assessment, No. 57. Paris: OECD Publishing. Available at: https://www.oecd.org/env/ehs/testing/37235405.pdf.
OECD (2005). Guidance Document on the Validation and International Acceptance of New or Updated Test Methods for Hazard Assessment. Series on Testing and Assessment No. 34. Available at: https://www.oecd.org/officialdocuments/publicdisplaydocumentpdf/?cote=ENV/JM/MONO(2005)14&doclanguage=en.
OECD (2021). Guidance Document on the Characterisation, Validation and Reporting of Physiologically Based Kinetic (PBK) Models for Regulatory Purposes. OECD Series on Testing and Assessment No. 331. Available at: https://www.oecd.org/chemicalsafety/risk-assessment/guidance-document-on-the-characterisation-validation-and-reporting-of-physiologically-based-kinetic-models-for-regulatory-purposes.pdf.
OECD (2014). New Scoping Document on in Vitro and Ex Vivo Assays for the Identification of Modulators of Thyroid Hormone Signalling, OECD Series on Testing and Assessment, No. 207. Paris: OECD Publishing. doi:10.1787/9789264274716-en
OECD (2020b). No. 321 Case Study on the Use of Integrated Approaches for Testing and Assessment for Systemic Toxicity Arising from Cosmetic Exposure to Caffeine, Series on Testing and Assessment. Paris, France: OECD Publishing. Available at: https://www.oecd.org/officialdocuments/publicdisplaydocumentpdf/?cote=ENV/JM/MONO(2020)17&docLanguage=en.
OECD (2018). Revised Guidance Document 150 on Standardised Test Guidelines for Evaluating Chemicals for Endocrine Disruption, OECD Series on Testing and Assessment, No. 150. Paris: OECD Publishing. doi:10.1787/9789264304741-en
OECD (2010). Test No. 417: Toxicokinetics, OECD Guidelines for the Testing of Chemicals, Section 4. Paris: OECD Publishing. doi:10.1787/9789264070882-en
Oerlemans, A., Verscheijden, L. F. M., Mol, J. G. J., Vermeulen, R. C. H., Westerhout, J., Roeleveld, N., et al. (2019). Toxicokinetics of a Urinary Metabolite of Tebuconazole Following Controlled Oral and Dermal Administration in Human Volunteers. Arch. Toxicol. 93 (9), 2545–2553. doi:10.1007/s00204-019-02523-5
Ohnhaus, E. E., and Park, B. K. (1979). Measurement of Urinary 6-?-Hydroxycortisol Excretion as an In Vivo Parameter in the Clinical Assessment of the Microsomal Enzyme-Inducing Capacity of Antipyrine, Phenobarbitone and Rifampicin. Eur. J. Clin. Pharmacol. 15 (2), 139–145. doi:10.1007/bf00609878
Olivier, E., Wakx, A., Fouyet, S., Dutot, M., and Rat, P. (2021). JEG-3 Placental Cells in Toxicology Studies: A Promising Tool to Reveal Pregnancy Disorders. Anat. Cell Biol. 54 (1), 83–92. doi:10.5115/acb.20.234
Ooka, M., Lynch, C., and Xia, M. (2020). Application of In Vitro Metabolism Activation in High-Throughput Screening. Int. J. Mol. Sci. 21 (21), 8182. doi:10.3390/ijms21218182
Oscarson, M., Zanger, U., Rifki, O., Klein, K., Eichelbaum, M., and Meyer, U. (2006). Transcriptional Profiling of Genes Induced in the Livers of Patients Treated with Carbamazepine. Clin. Pharmacol. Ther. 80 (5), 440–456. doi:10.1016/j.clpt.2006.08.013
Parker, A. C., Pritchard, P., Preston, T., and Choonara, I. (1998). Induction of CYP1A2 Activity by Carbamazepine in Children Using the Caffeine Breath Test. Br. J. Clin. Pharmacol. 45 (2), 176–178. doi:10.1046/j.1365-2125.1998.00684.x
Paul, K. B., Thompson, J. T., Simmons, S. O., Vanden Heuvel, J. P., and Crofton, K. M. (2013). Evidence for Triclosan-Induced Activation of Human and Rodent Xenobiotic Nuclear Receptors. Toxicol. Vitro 27 (7), 2049–2060. doi:10.1016/j.tiv.2013.07.008
Perucca, E., Hedges, A., Makki, K. A., Ruprah, M., Wilson, J. F., and Richens, A. (20041984). A Comparative Study of the Relative Enzyme Inducing Properties of Anticonvulsant Drugs in Epileptic Patients. Br. J. Clin. Pharmacol. 58 (7), S854–S863. doi:10.1111/j.1365-2125.2004.02311.x
Peyre, L., Rouimi, P., de Sousa, G., Héliès-Toussaint, C., Carré, B., Barcellini, S., et al. (2014). Comparative Study of Bisphenol A and its Analogue Bisphenol S on Human Hepatic Cells: A Focus on Their Potential Involvement in Nonalcoholic Fatty Liver Disease. Food Chem. Toxicol. 70, 9–18. doi:10.1016/j.fct.2014.04.011
Pichard, L., Fabre, I., Fabre, G., Domergue, J., Saint Aubert, B., Mourad, G., et al. (1990). Cyclosporin A Drug Interactions. Screening for Inducers and Inhibitors of Cytochrome P-450 (Cyclosporin A Oxidase) in Primary Cultures of Human Hepatocytes and in Liver Microsomes. Drug Metab. Dispos. 18 (5), 595–606.
Pondugula, S. R., Pavek, P., and Mani, S. (2016). Pregnane X Receptor and Cancer: Context-Specificity Is Key. Nucl. Recept. Res. 3, 101198. doi:10.11131/2016/101198
Rat, P., Olivier, E., Tanter, C., Wakx, A., and Dutot, M. (2017). A Fast and Reproducible Cell- and 96-well Plate-Based Method for the Evaluation of P2X7 Receptor Activation Using YO-PRO-1 Fluorescent Dye. J. Biol. Methods 4 (1), e64. doi:10.14440/jbm.2017.136
Ratelle, M., Côté, J., and Bouchard, M. (2015). Toxicokinetics of Permethrin Biomarkers of Exposure in Orally Exposed Volunteers. Toxicol. Lett. 232 (2), 369–375. doi:10.1016/j.toxlet.2014.12.003
Reed, G. A., Peterson, K. S., Smith, H. J., Gray, J. C., Sullivan, D. K., Mayo, M. S., et al. (2005). A Phase I Study of Indole-3-Carbinol in Women: Tolerability and Effects. Cancer Epidemiol. Biomarkers Prev. 14 (8), 1953–1960. doi:10.1158/1055-9965.epi-05-0121
Rendic, S. P., and Guengerich, F. P. (2021). Human Family 1-4 Cytochrome P450 Enzymes Involved in the Metabolic Activation of Xenobiotic and Physiological Chemicals: an Update. Arch. Toxicol. 95 (2), 395–472. doi:10.1007/s00204-020-02971-4
Robertson, S. M., Maldarelli, F., Natarajan, V., Formentini, E., Alfaro, R. M., and Penzak, S. R. (2008). Efavirenz Induces CYP2B6-Mediated Hydroxylation of Bupropion in Healthy Subjects. J. Acquir Immune Defic. Syndr. 49 (5), 513–519. doi:10.1097/qai.0b013e318183a425
Robson, R., Miners, J., Wing, L., and Birkett, D. (1984). Theophylline-Rifampicin Interaction: Non-Selective Induction of Theophylline Metabolic Pathways. Br. J. Clin. Pharmacol. 18 (3), 445–448. doi:10.1111/j.1365-2125.1984.tb02487.x
Rost, K. L., Fuhr, U., Thomsen, T., Zaigler, M., Brockmöller, J., Bohnemeier, H., et al. (1999). Omeprazole Weakly Inhibits CYP1A2 Activity in Man. Int. J. Clin. Pharmacol. Ther. 37 (11), 567–574.
Rost, K. L., Brösicke, H., Heinemeyer, G., and Roots, I. (1994). Specific and Dose-Dependent Enzyme Induction by Omeprazole in Human Beings. Hepatology 20 (5), 1204–1212. doi:10.1002/hep.1840200516
Rubin, K., Janefeldt, A., Andersson, L., Berke, Z., Grime, K., and Andersson, T. B. (2015). HepaRG Cells as Human-Relevant In Vitro Model to Study the Effects of Inflammatory Stimuli on Cytochrome P450 Isoenzymes. Drug Metab. Dispos. 43 (1), 119–125. doi:10.1124/dmd.114.059246
Sachar, M., Kelly, E. J., and Unadkat, J. D. (2019). Mechanisms of CYP3A Induction During Pregnancy: Studies in HepaRG Cells. Aaps J. 21 (3), 45. doi:10.1208/s12248-019-0316-z
Sager, J. E., Price, L. S. L., and Isoherranen, N. (2016). Stereoselective Metabolism of Bupropion to OH-Bupropion, Threohydrobupropion, Erythrohydrobupropion, and 4'-OH-Bupropion In Vitro. Drug Metabolism Dispos. 44 (10), 1709–1719. doi:10.1124/dmd.116.072363
Sarich, T., Kalhorn, T., Magee, S., al-Sayegh, F., Adams, S., Slattery, J., et al. (1997). The Effect of Omeprazole Pretreatment on Acetaminophen Metabolism in Rapid and Slow Metabolizers of S-Mephenytoin*. Clin. Pharmacol. Ther. 62 (1), 21–28. doi:10.1016/s0009-9236(97)90148-x
Schauer, U. M. D., Völkel, W., and Dekant, W. (2006). Toxicokinetics of Tetrabromobisphenol A in Humans and Rats After Oral Administration. Toxicol. Sci. 91 (1), 49–58. doi:10.1093/toxsci/kfj132
Schmidt, F. F., Lichtenstein, D., Planatscher, H., Mentz, A., Kalinowski, J., Steinhilber, A. E., et al. (2021). Pesticide Mixture Effects on Liver Protein Abundance in HepaRG Cells. Toxicology 458, 152839. doi:10.1016/j.tox.2021.152839
Selim, S., Hartnagel, R. E., Osimitz, T. G., Gabriel, K. L., and Schoenig, G. P. (1995). Absorption, Metabolism, and Excretion of N,N-Diethyl-M-Toluamide Following Dermal Application to Human Volunteers. Toxicol. Sci. 25 (1), 95–100. doi:10.1093/toxsci/25.1.95
Sergent, T., Dupont, I., Jassogne, C., Ribonnet, L., van der Heiden, E., Scippo, M.-L., et al. (2009). CYP1A1 Induction and CYP3A4 Inhibition by the Fungicide Imazalil in the Human Intestinal Caco-2 Cells-Comparison with Other Conazole Pesticides. Toxicol. Lett. 184 (3), 159–168. doi:10.1016/j.toxlet.2008.11.009
Shimada, T., and Guengerich, F. P. (2006). Inhibition of Human Cytochrome P450 1A1-, 1A2-, and 1B1-Mediated Activation of Procarcinogens to Genotoxic Metabolites by Polycyclic Aromatic Hydrocarbons. Chem. Res. Toxicol. 19 (2), 288–294. doi:10.1021/tx050291v
Simonsson, U., Jansson, B., Hai, T. N., Huong, D. X., Tybring, G., and Ashton, M. (2003). Artemisinin Autoinduction Is Caused by Involvement of Cytochrome P450 2B6 but Not 2C9. Clin. Pharmacol. Ther. 74 (1), 32–43. doi:10.1016/s0009-9236(03)00092-4
Slattery, J. T., Kalhorn, T. F., McDonald, G. B., Lambert, K., Buckner, C. D., Bensinger, W. I., et al. (1996). Conditioning Regimen-Dependent Disposition of Cyclophosphamide and Hydroxycyclophosphamide in Human Marrow Transplantation Patients. Jco 14 (5), 1484–1494. doi:10.1200/jco.1996.14.5.1484
Solecki, R., Kortenkamp, A., Bergman, Å., Chahoud, I., Degen, G. H., Dietrich, D., et al. (2017). Scientific Principles for the Identification of Endocrine-Disrupting Chemicals: A Consensus Statement. Arch. Toxicol. 91 (2), 1001–1006. doi:10.1007/s00204-016-1866-9
Spratlen, M. J., Perera, F. P., Lederman, S. A., Robinson, M., Kannan, K., Herbstman, J., et al. (2020). The Association Between Perfluoroalkyl Substances and Lipids in Cord Blood. J. Clin. Endocrinol. Metab. 105 (1), 43–54. doi:10.1210/clinem/dgz024
Staiger, C., Schlicht, F., Walter, E., Gundert-Remy, U., Hildebrandt, R., de Vries, J., et al. (1983). Effect of Single and Multiple Doses of Sulphinpyrazone on Antipyrine Metabolism and Urinary Excretion of 6-Beta-Hydroxycortisol. Eur. J. Clin. Pharmacol. 25 (6), 797–801. doi:10.1007/bf00542523
Svensson, U. S. H., Ashton, M., Hai, T. N., Bertilsson, L., Huong, D. X., Van Huong, N., et al. (1998). Artemisinin Induces Omeprazole Metabolism in Human Beings*. Clin. Pharmacol. Ther. 64 (2), 160–167. doi:10.1016/s0009-9236(98)90149-7
Szychowski, K. A., Wnuk, A., Kajta, M., and Wójtowicz, A. K. (2016). Triclosan Activates Aryl Hydrocarbon Receptor (AhR)-Dependent Apoptosis and Affects Cyp1a1 and Cyp1b1 Expression in Mouse Neocortical Neurons. Environ. Res. 151, 106–114. doi:10.1016/j.envres.2016.07.019
Tassaneeyakul, W., Birkett, D. J., Veronese, M. E., McManus, M. E., Tukey, R. H., Quattrochi, L. C., et al. (1993). Specificity of Substrate and Inhibitor Probes for Human Cytochromes P450 1A1 and 1A2. J. Pharmacol. Exp. Ther. 265 (1), 401–407.
Taxvig, C. (2020). Advancing the Application of Alternative Tests Methods in Chemical Risk Assessment by Incorporating Metabolism into in Vitro Assays (In-Vita). Copenhagen, Denmark: Pesticide Research, National Food Institute, Technical University of Denmark.
Telles-Silva, K. A., Pacheco, L., Komatsu, S., Chianca, F., Caires-Júnior, L. C., Araujo, B. H. S., et al. (2022). Applied Hepatic Bioengineering: Modeling the Human Liver Using Organoid and Liver-On-A-Chip Technologies. Front. Bioeng. Biotechnol. 10, 845360. doi:10.3389/fbioe.2022.845360
The Danish Centre on Endocrine Disrupters, (2020). Prochloraz - Evaluation Report. Retrieved from https://edlists.org/sites/edlists.org/files/media/document/Prochloraz.pdf.
Thorn, C. F., Aklillu, E., McDonagh, E. M., Klein, T. E., and Altman, R. B. (2012). PharmGKB Summary. Pharmacogenet Genomics 22 (5), 389–395. doi:10.1097/fpc.0b013e3283505d5e
Tolson, A. H., and Wang, H. (2010). Regulation of Drug-Metabolizing Enzymes by Xenobiotic Receptors: PXR and CAR. Adv. Drug Deliv. Rev. 62 (13), 1238–1249. doi:10.1016/j.addr.2010.08.006
Tsaioun, K., Blaauboer, B. J., and Hartung, T. (2016). Evidence-Based Absorption, Distribution, Metabolism, Excretion (ADME) and its Interplay with Alternative Toxicity Methods. ALTEX 33 (4), 343–358. doi:10.14573/altex.1610101
Turpeinen, M., Tolonen, A., Chesne, C., Guillouzo, A., Uusitalo, J., and Pelkonen, O. (2009). Functional Expression, Inhibition and Induction of CYP Enzymes in HepaRG Cells. Toxicol. Vitro 23 (4), 748–753. doi:10.1016/j.tiv.2009.03.008
US FDA, (2020). In Vitro Drug Interaction Studies - Cytochrome P450 Enzyme- and Transporter-Mediated Drug Interactions Guidance for Industry. Rockville, MD: Center for Drug Evaluation and Research, United States Food and Drug Administration. 46.
Usmani, K. A., Cho, T. M., Rose, R. L., and Hodgson, E. (2006). Inhibition of the Human Liver Microsomal and Human Cytochrome P450 1A2 and 3A4 Metabolism of Estradiol by Deployment-Related and Other Chemicals. Drug Metab. Dispos. 34 (9), 1606–1614. doi:10.1124/dmd.106.010439
van Giersbergen, P. L. M., Halabi, A., and Dingemanse, J. (2002a). Single- and Multiple-Dose Pharmacokinetics of Bosentan and its Interaction with Ketoconazole. Br. J. Clin. Pharmacol. 53 (6), 589–595. doi:10.1046/j.1365-2125.2002.01608.x
Vangiersbergen, P., Treiber, A., Clozel, M., Bodin, F., and Dingemanse, J. (2002b). In Vivo and In Vitro Studies Exploring the Pharmacokinetic Interaction Between Bosentan, a Dual Endothelin Receptor Antagonist, and Glyburide. Clin. Pharmacol. Ther. 71 (4), 253–262. doi:10.1067/mcp.2002.122473
Vaynshteyn, D., and Jeong, H. (2012). Caffeine Induces CYP1A2 Expression in Rat Hepatocytes but Not in Human Hepatocytes. Dml 6 (2), 116–119. doi:10.2174/1872312811206020116
Vinggaard, A. M., Hass, U., Dalgaard, M., Andersen, H. R., Bonefeld-jorgensen, E., Christiansen, S., et al. (2006). Prochloraz: An Imidazole Fungicide with Multiple Mechanisms of Action. Int. J. Androl. 29 (1), 186–192. doi:10.1111/j.1365-2605.2005.00604.x
Vlach, M., Quesnot, N., Dubois-Pot-Schneider, H., Ribault, C., Verres, Y., Petitjean, K., et al. (2019). Cytochrome P450 1A1/2, 2B6 and 3A4 HepaRG Cell-Based Biosensors to Monitor Hepatocyte Differentiation, Drug Metabolism and Toxicity. Sensors (Basel) 19 (10), 2245. doi:10.3390/s19102245
Vogel, C. F. A., Van Winkle, L. S., Esser, C., and Haarmann-Stemmann, T. (2020). The Aryl Hydrocarbon Receptor as a Target of Environmental Stressors - Implications for Pollution Mediated Stress and Inflammatory Responses. Redox Biol. 34, 101530. doi:10.1016/j.redox.2020.101530
Vrzal, R., Zenata, O., Doricakova, A., and Dvorak, Z. (2015). Environmental Pollutants Parathion, Paraquat and Bisphenol A Show Distinct Effects Towards Nuclear Receptors-Mediated Induction of Xenobiotics-Metabolizing Cytochromes P450 in Human Hepatocytes. Toxicol. Lett. 238 (1), 43–53. doi:10.1016/j.toxlet.2015.07.008
Walsky, R. L., Astuccio, A. V., and Obach, R. S. (2006). Evaluation of 227 Drugs for In Vitro Inhibition of Cytochrome P450 2B6. J. Clin. Pharmacol. 46 (12), 1426–1438. doi:10.1177/0091270006293753
Walter, E., Staiger, C., Vries, J., Weber, E., Bitzer, W., Degott, M., et al. (1982). Enhanced Drug Metabolism After Sulfinpyrazone Treatment in Patients Aged 50 to 60 Years. Klin. Wochenschr 60 (22), 1409–1413. doi:10.1007/bf01716246
Wanchana, S., Yamashita, F., and Hashida, M. (2003). QSAR Analysis of the Inhibition of Recombinant CYP 3A4 Activity by Structurally Diverse Compounds Using a Genetic Algorithm-Combined Partial Least Squares Method. Pharm. Res. 20 (9), 1401–1408. doi:10.1023/a:1025702009611
Wang, H., and Tompkins, L. (2008). CYP2B6: New Insights into a Historically Overlooked Cytochrome P450 Isozyme. Cdm 9 (7), 598–610. doi:10.2174/138920008785821710
Wang, Y.-M., Ong, S. S., Chai, S. C., and Chen, T. (2012). Role of CAR and PXR in Xenobiotic Sensing and Metabolism. Expert Opin. Drug Metabolism Toxicol. 8 (7), 803–817. doi:10.1517/17425255.2012.685237
Waxman, D. J. (1999). P450 Gene Induction by Structurally Diverse Xenochemicals: Central Role of Nuclear Receptors CAR, PXR, and PPAR. Archives Biochem. Biophysics 369 (1), 11–23. doi:10.1006/abbi.1999.1351
Weber, C., Banken, L., Birnboeck, H., and Schulz, R. (1999a). Effect of the Endothelin-Receptor Antagonist Bosentan on the Pharmacokinetics and Pharmacodynamics of Warfarin. J. Clin. Pharmacol. 39 (8), 847–854. doi:10.1177/00912709922008380
Weber, C., Schmitt, R., Birnboeck, H., Hopfgartner, G., Eggers, H., Meyer, J., et al. (1999b). Multiple-dose Pharmacokinetics, Safety, and Tolerability of Bosentan, an Endothelin Receptor Antagonist, in Healthy Male Volunteers. J. Clin. Pharmacol. 39 (7), 703–714. doi:10.1177/00912709922008344
Werk, E. E., Macgee, J., and Sholiton, L. J. (1964). Effect of Diphenylhydantoin on Cortisol Metabolism in Man *. J. Clin. Invest. 43, 1824–1835. doi:10.1172/jci105056
Wietholtz, H., Zysset, T., Kreiten, K., Kohl, D., Buchsel, R., and Matern, S. (1989). Effect of Phenytoin, Carbamazepine, and Valproic Acid on Caffeine Metabolism. Eur. J. Clin. Pharmacol. 36 (4), 401–406. doi:10.1007/bf00558303
Wietholtz, H., Zysset, T., Marschall, H.-U., Generet, K., and Matern, S. (1995). The Influence of Rifampin Treatment on Caffeine Clearance in Healthy Man. J. Hepatology 22 (1), 78–81. doi:10.1016/0168-8278(95)80263-0
Williams, J. A., Ring, B. J., Cantrell, V. E., Jones, D. R., Eckstein, J., Ruterbories, K., et al. (2002). Comparative Metabolic Capabilities of CYP3A4, CYP3A5, and CYP3A7. Drug Metab. Dispos. 30 (8), 883–891. doi:10.1124/dmd.30.8.883
Williams, M. L., Wainer, I. W., Embree, L., Barnett, M., Granvil, C. L., and Ducharme, M. P. (1999). Enantioselective Induction of Cyclophosphamide Metabolism by Phenytoin. Chirality 11 (7), 569–574. doi:10.1002/(sici)1520-636x(1999)11:7<569::aid-chir9>3.0.co;2-r
Wing, L., Miners, J., and Lillywhite, K. (1985). Verapamil Disposition-Effects of Sulphinpyrazone and Cimetidine. Br. J. Clin. Pharmacol. 19 (3), 385–391. doi:10.1111/j.1365-2125.1985.tb02658.x
Woolbright, B. L., and Jaeschke, H. (2015). Xenobiotic and Endobiotic Mediated Interactions Between the Cytochrome P450 System and the Inflammatory Response in the Liver. Adv. Pharmacol. 74, 131–161. doi:10.1016/bs.apha.2015.04.001
Yajima, K., Uno, Y., Murayama, N., Uehara, S., Shimizu, M., Nakamura, C., et al. (2014). Evaluation of 23 Lots of Commercially Available Cryopreserved Hepatocytes for Induction Assays of Human Cytochromes P450. Drug Metab. Dispos. 42 (5), 867–871. doi:10.1124/dmd.113.056804
Yamreudeewong, W., DeBisschop, M., Martin, L. G., and Lower, D. L. (2003). Potentially Significant Drug Interactions of Class III Antiarrhythmic Drugs. Drug Saf. 26 (6), 421–438. doi:10.2165/00002018-200326060-00004
Yang, D., Wang, X., Chen, Y.-t., Deng, R., and Yan, B. (2009). Pyrethroid Insecticides: Isoform-Dependent Hydrolysis, Induction of Cytochrome P450 3A4 and Evidence on the Involvement of the Pregnane X Receptor. Toxicol. Appl. Pharmacol. 237 (1), 49–58. doi:10.1016/j.taap.2009.02.012
Yi, M., Fashe, M., Arakawa, S., Moore, R., Sueyoshi, T., and Negishi, M. (2020). Nuclear Receptor CAR-Erα Signaling Regulates the Estrogen Sulfotransferase Gene in the Liver. Sci. Rep. 10 (1), 5001. doi:10.1038/s41598-020-61767-9
Yim, S. K., Kim, K., Chun, S., Oh, T., Jung, W., Jung, K., et al. (2020). Screening of Human CYP1A2 and CYP3A4 Inhibitors from Seaweed In Silico and In Vitro. Mar. Drugs 18 (12), 603. doi:10.3390/md18120603
Yueh, M.-F., He, F., Chen, C., Vu, C., Tripathi, A., Knight, R., et al. (2020). Triclosan Leads to Dysregulation of the Metabolic Regulator FGF21 Exacerbating High Fat Diet-Induced Nonalcoholic Fatty Liver Disease. Proc. Natl. Acad. Sci. U.S.A. 117 (49), 31259–31266. doi:10.1073/pnas.2017129117
Zanelli, U., Caradonna, N. P., Hallifax, D., Turlizzi, E., and Houston, J. B. (2012). Comparison of Cryopreserved HepaRG Cells with Cryopreserved Human Hepatocytes for Prediction of Clearance for 26 Drugs. Drug Metab. Dispos. 40 (1), 104–110. doi:10.1124/dmd.111.042309
Zhou, X., Pant, S., Nemunaitis, J., Craig Lockhart, A., Falchook, G., Bauer, T. M., et al. (2018). Effects of Rifampin, Itraconazole and Esomeprazole on the Pharmacokinetics of Alisertib, an Investigational Aurora a Kinase Inhibitor in Patients with Advanced Malignancies. Invest New Drugs 36 (2), 248–258. doi:10.1007/s10637-017-0499-z
Keywords: CYP, P450, validation, test guideline, HepaRG, metabolism
Citation: Jacobs MN, Kubickova B and Boshoff E (2022) Candidate Proficiency Test Chemicals to Address Industrial Chemical Applicability Domains for in vitro Human Cytochrome P450 Enzyme Induction. Front. Toxicol. 4:880818. doi: 10.3389/ftox.2022.880818
Received: 21 February 2022; Accepted: 25 April 2022;
Published: 20 June 2022.
Edited by:
Rex FitzGerald, Swiss Centre for Applied Human Toxicology (SCAHT), SwitzerlandReviewed by:
Olavi R. Pelkonen, University of Oulu, FinlandCopyright © 2022 Jacobs, Kubickova and Boshoff. This is an open-access article distributed under the terms of the Creative Commons Attribution License (CC BY). The use, distribution or reproduction in other forums is permitted, provided the original author(s) and the copyright owner(s) are credited and that the original publication in this journal is cited, in accordance with accepted academic practice. No use, distribution or reproduction is permitted which does not comply with these terms.
*Correspondence: Miriam Naomi Jacobs, TWlyaWFtLkphY29ic0BwaGUuZ292LnVr
†ORCID: Miriam Naomi Jacobs, https://orcid.org/0000-0002-4858-0118; Barbara Kubickova, https://orcid.org/0000-0002-5044-7180
Disclaimer: All claims expressed in this article are solely those of the authors and do not necessarily represent those of their affiliated organizations, or those of the publisher, the editors and the reviewers. Any product that may be evaluated in this article or claim that may be made by its manufacturer is not guaranteed or endorsed by the publisher.
Research integrity at Frontiers
Learn more about the work of our research integrity team to safeguard the quality of each article we publish.