- 1Electrical Engineering Department, Universitas Sumatera Utara, Medan, North Sumatra, Indonesia
- 2Wigwe University, Isiokpo, Nigeria
The scarcity of marine life caused by shallow waters and pollution has pushed fishermen to venture up to 10 km offshore, where clearer waters offer richer fish resources, but at much higher fuel costs. This situation has caused increased unemployment, so many fishermen have changed professions. This is exacerbated by seasonal variations and resource limitations. This research introduces a fish sensor network (FSN), which is designed to equip floating fish houses (FADs) with network-connected fish sensors. The proposed network allows fishermen to find locations with high fish populations, thereby reducing fuel costs and increasing fishing efficiency. This article presents preliminary findings, identifies potential challenges including natural factors such as wind, waves, currents, corrosion, and radio propagation, as well as man-made obstacles such as traffic density and physical measurements at the research site in Belawan, Indonesia. Additionally, this paper briefly discusses the energy availability that poses further challenges.
1 Introduction
The fish sensor network (FSN) represents a revolutionary wireless sensor network application that is designed to detect fish underwater and efficiently to share their locations. This chapter delves into the application of FSN in Belawan city, Indonesia, shedding light on its potential to transform the local fishing industry. Approximately 90% of Indonesian population engaged in fishing livelihoods, grapples with widespread poverty among fishermen, largely attributed to the unpredictable nature of their occupation (Latief et al., 2020). In Belawan specifically, this socioeconomic challenge is exacerbated by the geographical features of shallow coastal contours, spanning depths of 2–7 m and extending up to 7 km offshore. The turbid water profiles prevalent in these shallow waters, as illustrated in Figure 1, sourced from Google Earth, further complicate fishing endeavors.
Within the confines of the Belawan area, certain sections are subject to governmental regulations governing port traffic, which restrict fishermen’s access to nearby deeper waters. Moreover, the shallow regions, characterized by depths less than 7 m, often exhibit turbid conditions, resulting in a scarcity of marine biota. The situation is worsened by pollution levels that have contributed to a decline in fish populations over time.
In pursuit of higher incomes, fishermen are compelled to venture up to 10 km offshore to areas boasting clearer water contours and richer fish resources. However, reaching these promising fishing grounds entails considerable expenses, with eight-person capacity boats consuming 10–20 L of diesel for a single round trip. At a price of IDR 6,800 per liter for biodiesel, the fuel costs alone range from approximately IDR 68,000 to IDR 136,000 per trip, excluding expenditures on food and supplies.
The declining fish populations can be attributed to various factors, including high mortality rates resulting from indiscriminate fishing practices such as trawling. This has led to a significant reduction in fish population, with some species experiencing a decline of up to 33%, and others showing a 3% decrease from normal levels (Atmaja and Nugroho, 2019). Such trends underscore the multifaceted challenges facing the sustainability of fish resources in the region.
As a consequence of dwindling catches, fishermen are confronted with escalating exploitation costs and labor burdens, precipitating the closure of numerous small-scale fishing operations. This, in turn, has contributed to rising unemployment rates and prompted many fishermen to seek alternative livelihoods, often straying far from their areas of expertise. Over the past decade, there has been a staggering 25% reduction in the number of active fishermen (Suherman et al., 2020), with a significant portion falling below the poverty line.
While government initiatives aimed at empowering fishermen economically have been introduced, the involvement of the community, including academia, remains paramount in addressing both internal and external challenges. Fishermen’s poverty is further compounded by natural obstacles such as seasonal variations, fluctuating income sources, pricing discrepancies in the fish market, limited access to capital, and the constrained capacity of the local fish processing industry. Additionally, external competition from fishermen equipped with advanced catch processing technology adds to the plight of traditional fishermen (Latief et al., 2020).
The modernization of fishing methods holds promise not only in uplifting marginalized traditional fishermen but also in potentially bolstering catch volumes and enhancing fishermen’s awareness of sustainable maritime resource management practices. Technologies such as fish finder technology (Haag et al., 2019) present valuable tools for assisting fishermen in identifying fish potential in the vast expanse of the sea. However, the widespread adoption of such technologies remains hampered by cost constraints and operational limitations, thereby limiting their accessibility to the fishing community.
This paper presents natural and man-made factors that may influence the fish sensor implementation in increasing fish catchment. Natural factors such as wind, waves, currents, corrosion, and radio propagation are potential drifting sensor functions, while man-made factors such as traffics and physical security influence the sensor physically.
2 The imperative for a fish sensor network
A comprehensive understanding of the echo signal’s components from a fish finder, encompassing factors such as frequency shift, bandwidth, amplitude, emission, and processing speed, plays a pivotal role in determining the accuracy and reliability of fish detection. While fish finders present a promising solution for assisting fishermen in pinpointing fish locations, the overarching challenge lies in their prohibitive cost, rendering them inaccessible to many fishermen. Compounding this issue is their standalone nature, which hampers seamless information sharing among fishing communities (Perea-Munoz et al., 2022).
The integration of fish finders equipped with a global positioning system (GPS) has emerged as a game-changer in the fishing industry, offering tangible benefits such as enhanced catch yields and reduced fish search costs. Research conducted in (Tombak and Graha, 2016) underscores the potential economic dividends, indicating potential fuel cost savings of up to 16.67% and a substantial increase in annual income amounting to IDR 160,536,000.
Operating within the frequency range of 15 kHz–455 kHz, fish finder devices emit low-frequency sound impulses directed towards the seabed, with echoes subsequently visualized in various forms, including object size, composition, symbols, and seabed depth. The efficacy of fish finders hinges on factors such as frequency and signal strength, with research in (Yasim et al., 2021) demonstrating a notable increase in effectiveness over time, as depicted in Figure 2. Over the course of 3 months, there was a progressive improvement, with a 24% increase in the first month, 27% in the second month, and 32% in the third month. This underscores the importance of continuous skill development among fishermen in utilizing fish finder technology, with proficiency directly correlating to enhanced catch rates.
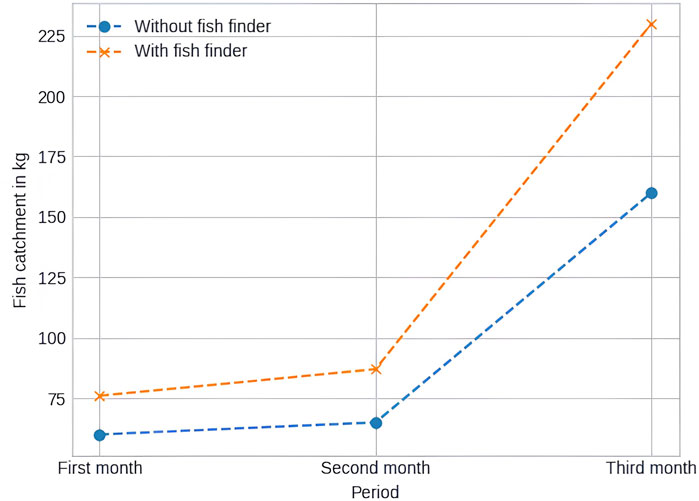
Figure 2. Increase in catch with fish finder (Yasim et al., 2021).
In their quest to identify prime fishing spots, fishermen often rely on fish aggregating devices (FADs) or fish houses strategically positioned throughout the ocean. These structures, ranging from permanent installations such as old tires marked with floating buoys and foliage to simpler variations utilizing GPS coordinates, serve as beacons for fish congregations. While FADs offer promising prospects for boosting catch yields, their efficacy is contingent upon factors such as placement and maintenance as illustrated in Figure 3. To prevent drift and ensure stability, FADs must be anchored securely, with synthetic ropes emerging as a durable option, as suggested by research conducted in (Thahir et al., 2019).
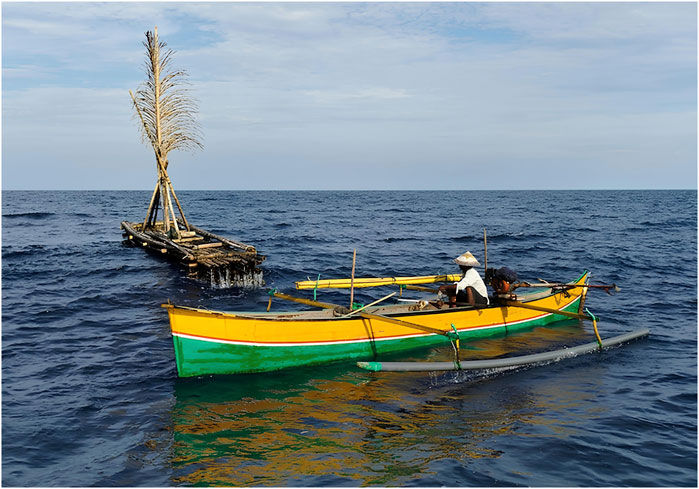
Figure 3. Fish aggregating device (Cressey, 2014).
The construction of stationary FADs, as illustrated in Figure 4, presents a potential avenue for enhancing durability and longevity. However, compared to traditional FADs, the efficacy of synthetic ropes is only 50%, albeit offering long-term resilience. Building upon prior research (Suherman et al., 2022), the proposition of a fish sensor network emerges as a viable solution to mitigate losses resulting from futile fishing expeditions. By providing real-time insights into fish locations, a fish sensor network has the potential to revolutionize fishing practices, empowering fishermen with actionable data to optimize their catch efficiency and economic returns.
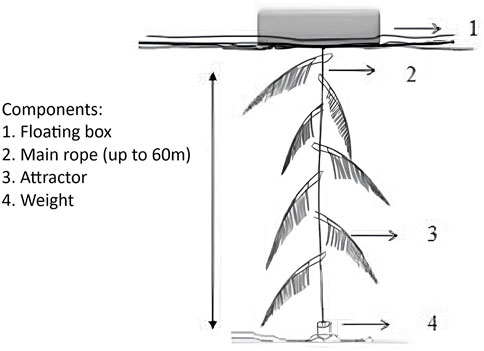
Figure 4. Stationary FAD (Thahir et al., 2019).
3 Configuration of the fish sensor network
The Fish Sensor Network (FSN) stands as a progressive extension of the wireless sensor network (WSN) paradigm pioneered by this research initiative. FSN comprises an array of fish sensors strategically dispersed across fishing domains, meticulously positioned to surveil both fish targets and the seabed surface with precision and efficacy. These sensors operate by capturing acoustic signals, subjecting them to rigorous analysis, and rendering them into actionable visualizations. Under optimal conditions, sensors can be deployed both above and below the sea surface, as delineated in Figure 5A, meticulously conceptualized and designed by the research team. Equipped with an array of cutting-edge features, including Global Positioning System (GPS) modules, communication links, energy harvesters such as solar cells and wind turbines, energy storage solutions such as robust batteries, radio transceivers, and antennas (Wang et al., 2022), these sensors are engineered to withstand the corrosive properties of marine environments, strong currents, and maritime traffic.
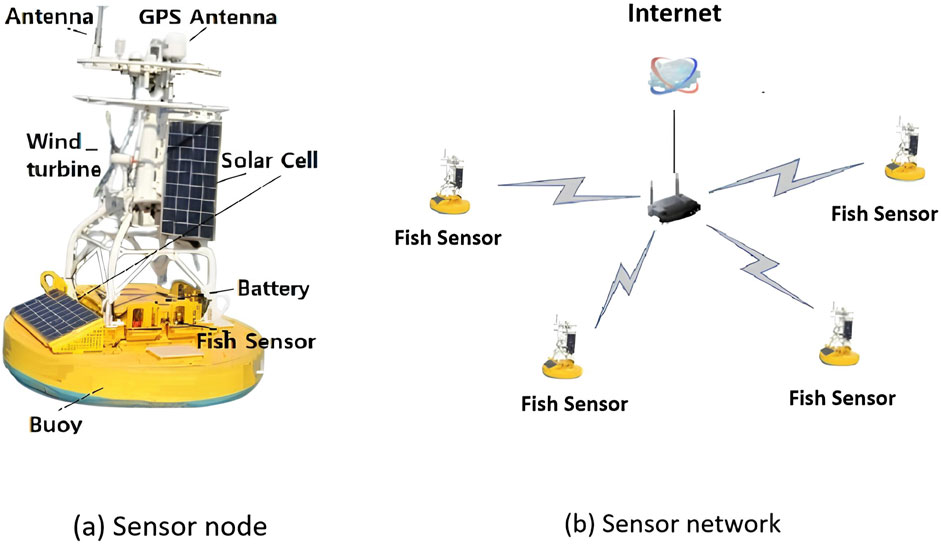
Figure 5. FSN configuration: (A) Sensor node consists of sensors, power source and radio communication; (B) The sensor network consists of many interconnected sensor nodes.
Moreover, FSN’s network architecture encompasses various operational modes, including centralized configurations exemplified in Figure 5, where radio signal transmitters such as WiFi, WiMAX, or cellular networks serve as vital conduits, bridging sensors with centralized servers acting as repositories and distributors of invaluable data to the wider community.
The incorporation of buoys into the sensor data infrastructure facilitates the deployment of floating sensors, while anchoring mechanisms ensure steadfastness and prevent undesired drift. In scenarios where FSN operates in a multihop mode, sensors are endowed with the requisite functionalities to collect, forward, route, and transmit data, enabling seamless relay of information from disparate sensors to the designated sink node (Wang et al., 2022).
At the heart of FSN lies the fish sensor, equipped with an array of sophisticated detection mechanisms aimed at uncovering underwater topographies and potential fish aggregating sites, thereby covering expansive areas with unparalleled precision and efficiency. Figure 6 illustrates various options for fish detection, ranging from acoustic sensors affixed to bait boats, precision radar systems deployed via specially designed explorer vessels, to portable fish finders integrated as sensor nodes.
Innovations abound in the realm of fish sensor networks, building upon previous endeavors that often referred to underwater acoustic sensor networks in a more general sense (Liu et al., 2021). While prior research predominantly focused on deep-water deployments (Menandro et al., 2022), endeavors in estuarine environments leaned heavily on bathymetric data (Fassoni-Andrade et al., 2021), with transducers submerged in water. Furthermore, studies leveraging fish finders primarily explored standalone applications (Chandrasekhar, 2022), often employing a single vessel, whereas our preceding research (Suherman et al., 2022) blazes a trail by seamlessly integrating fish finders into a cohesive network, extending tangible benefits to fishermen at large. Given the efficacy of fish aggregating devices (FADs) (Yasim et al., 2021) and lights (Geraci et al., 2021) in enhancing fish capture, future sensor node designs may amalgamate elements from both components, further augmenting the efficacy and versatility of FSN.
4 FSN challenges
4.1 Wave current and wind speed
Navigating the intricate maritime environment of Belawan port, situated on the Sumatra island of Indonesia and serving as a vital link between Sumatra and Malacca through a 12 km sailing line with a width of 100 m, poses formidable challenges. The port’s water depth, plunging to depths of up to −10 MLWS (Mean Low Water Springs), is subject to the dynamic interplay of two influential rivers: the Belawan and Deli rivers. Consequently, the wave and current patterns within the vicinity are characterized by complexity and volatility, rendering them inherently unpredictable.
Official records maintained by the local government offer insights into the fluctuating nature of these hydrological phenomena. During the wet season, current velocities fluctuate within the range of 0.04367 m/s to 0.4608 m/s, whereas in the dry season, these speeds exhibit a notable decrease, approximately halving to values ranging from 0.0229 m/s to 0.2744 m/s (Suherman et al., 2022). Figure 7 provides a visual representation of typical wave current patterns observed in the region.
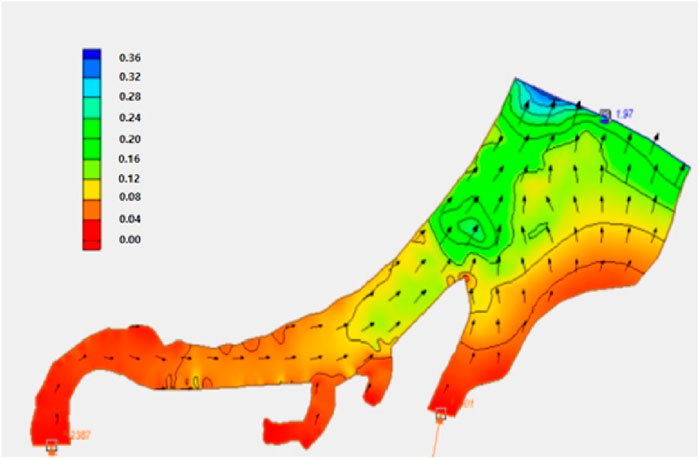
Figure 7. Sample wave current in Belawan port (Suherman et al., 2022).
The inherent instability of wave currents presents a significant challenge for the installation and maintenance of sensor nodes within the Belawan port vicinity. Without meticulous attention to installation protocols and robust anchoring mechanisms, sensor displacement becomes a distinct possibility, compromising data integrity and operational efficacy. As such, mitigating the impact of wave currents emerges as a paramount consideration in the design and deployment of the FSN infrastructure within this dynamic maritime environment.
4.2 Atmospheric corrosion rate
Conversely, the prevailing wind patterns originating from the Northwest of Medan and flowing towards the North exhibit velocities ranging from 3 to 15 knots. Concurrently, tidal levels oscillate within the range of 0.5–0.8 m, accompanied by ambient temperatures peaking between 24 and 32.2°C. The atmospheric humidity hovers between 68% and 91%, fostering conditions conducive to the proliferation of humid and moisture-laden air masses (Suherman et al., 2022).
This meteorological milieu engenders a corrosive environment, particularly pronounced in beach areas surrounding the Belawan port and within the expansive confines of the port itself, sprawling across an impressive expanse of 127,518 square meters. The deployment of conventional hard metal materials in infrastructure development and container placement within the port precinct renders them susceptible to corrosive degradation induced by prolonged exposure to the harsh climatic conditions.
The corrosive impact of salt-laden water precipitation poses a significant challenge, necessitating meticulous consideration in the design and deployment of FSN infrastructure. To mitigate the deleterious effects of atmospheric corrosion, it becomes imperative to integrate predictive models such as ASTM G-1 and ASTM G-50 standards into FSN sensor development endeavors. These standards offer invaluable insights into the anticipated lifespan of installed materials, with a clear correlation between corrosive rates and material longevity. Figure 8 provides a comprehensive depiction of corrosive rates ranging from 0.114 to 1.370 mm per year (mpy), exhibiting notable spatial variability across distinct geographical areas within the maritime domain. By incorporating such predictive models, FSN sensor nodes can be engineered to withstand the corrosive onslaught of the maritime environment, ensuring prolonged operational efficacy and data integrity amidst challenging atmospheric conditions.
4.3 Radio propagation losses
The radio propagation dynamics encountered by the FSN undergo a significant transformation when traversing over the expansive seascape. The saline-laden air prevalent in maritime environments exerts a pronounced attenuation effect on radio signals, precipitating rapid signal degradation. Furthermore, the water surface serves as a potent medium for the reflection and diffraction of radio signals, exacerbating signal losses and impairing transmission reliability.
During the rainy season, the attenuation of radio signals is exacerbated, with precipitation acting as a potent impediment to signal propagation. Consequently, meticulous consideration must be afforded to the radio link budget for FSN deployments, with a keen awareness of the variable atmospheric conditions prevailing throughout the operational environment. Extensive research endeavors have sought to elucidate the intricate interplay of radio propagation phenomena over marine expanses, consistently highlighting the adverse impact of signal distraction and reflection by the saline water surface on signal fidelity and strength.
Figure 9 offers a glimpse into the empirical manifestation of radio signal degradation over sea environments, as demonstrated in a study conducted in (Pinem et al., 2020). The depicted propagation characteristics underscore the formidable challenges posed by maritime radio propagation dynamics, necessitating adaptive strategies and robust engineering solutions to mitigate signal losses and ensure reliable data transmission within the context of FSN deployments.
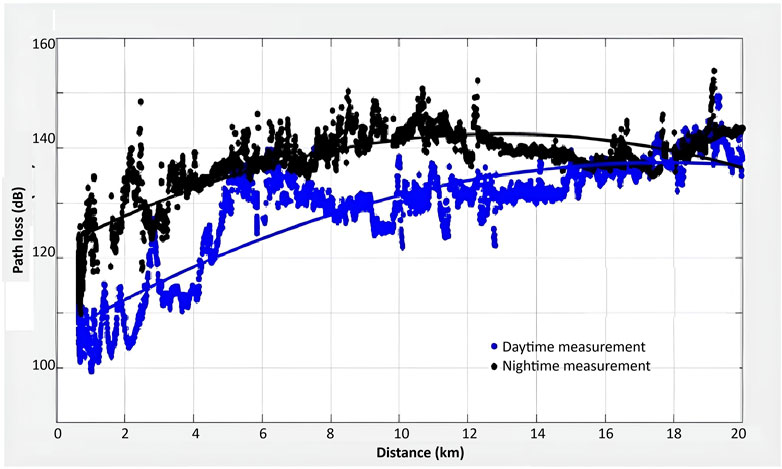
Figure 9. Propagation characteristics over the sea (Pinem et al., 2020).
4.4 Traffic density
The bustling maritime thoroughfare of Belawan Pelindo one serves as a veritable nexus of shipping activity, as documented in its comprehensive annual report (Geraci et al., 2021). Over the course of the first 6 months of 2020, a staggering total of 435 sizable vessels traversed the port, accompanied by a cargo payload comprising 531,551 TEUs (Twenty-foot Equivalent Units) of containers, marking a notable 5% increase from the previous year’s record. These maritime behemoths collectively transported an impressive aggregate cargo volume of 3,150,594 tons, encompassing an eclectic array of commodities ranging from papers and cements to sugar.
As the year progressed, the average international traffic density soared to 54.92 Box/Ship/Hour, underscoring the relentless tempo of commercial maritime operations. Meanwhile, the bustling domestic terminal recorded a commendable throughput rate of 47.66 B/S/H, indicative of the vibrant domestic trade dynamics underpinning the port’s operations. It's worth noting that these figures do not account for the myriad fishing vessels that ply the waters of Belawan port, numbering in the hundreds and further complicating the already congested maritime landscape.
Such prodigious maritime traffic poses a formidable challenge in identifying safe and strategically viable positions for the deployment of FSN sensors. The sheer volume and diversity of vessels navigating the port waters necessitate meticulous planning and coordination to safeguard against potential collisions and interference with maritime traffic. Consequently, the task of securing optimal sensor locations within this dynamic maritime ecosystem demands a nuanced understanding of traffic patterns and operational imperatives, underscoring the exigency for adaptive strategies and collaborative engagement with relevant stakeholders to ensure the seamless integration of FSN infrastructure amidst bustling maritime activity.
4.5 Enhancing physical security measures
The dataset provided by the Indonesia Statistics Body (Rahmadysah et al., 2022) paints a stark picture of the prevalence of criminal activities spanning a spectrum from violent crimes like murder to more mundane offenses such as gambling, with incidents cataloged at regular intervals averaging every three to 4 years. Detailed in Table 1, the statistics freshly released in 2021 underscore the multifaceted nature of criminal occurrences, with theft emerging as the foremost criminal malaise, accounting for a substantial 44%–56% of total reported criminal incidents.
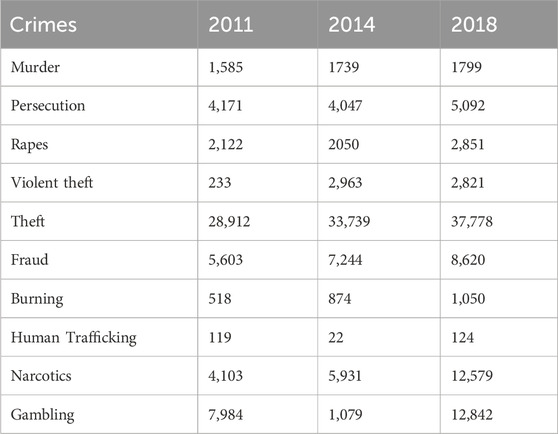
Table 1. Criminal spread in term of number of area (Tim Redaksi, 2021).
Given the remote locales often inhabited by sensors within the context of FSN deployments, the specter of theft looms ominously, posing a tangible threat to the integrity and functionality of sensor assets. To forestall the prospect of sensor pilferage, the implementation of targeted physical security measures assumes paramount importance. Chief among these strategies is the imperative to render sensors inconspicuous through meticulous camouflage techniques. However, the efficacy of conventional sensor designs, as depicted in Figure 1, may be compromised by inherent limitations in camouflage applicability. Consequently, the adoption of smaller, more discreet sensor installations, alongside the deployment of underwater sensor configurations, presents a viable solution to circumvent the challenges posed by theft and ensure the sustained operability and efficacy of FSN infrastructure in remote and vulnerable environments.
5 Case study: power generation enhancement
A pivotal element underpinning the seamless operation of FSN resides in the robustness and reliability of the energy source. Facilitating the dissemination of critical information regarding fish presence in FADs to aid fishermen in Belawan necessitates the uninterrupted functionality of fish sensors. The energy demand for sustaining operations remains relatively modest, typically ranging from one to 5 W to power both the fish sensors and ancillary radio links. Given the remote offshore locations of sensor deployments spanning distances of 5–10 km, the sustainability of the energy source assumes paramount importance in ensuring uninterrupted system operation.
However, the perennial challenge lies in the finite energy reservoirs afforded by conventional battery power sources. While solar cells (Oliveira-Pinto and Stokkermans, 2020), wind turbines (Cottura et al., 2021), and ocean turbines (Xiao et al., 2021) emerge as viable alternatives, their susceptibility to theft poses a significant impediment to widespread adoption. Consequently, the quest for alternative energy harvesting methodologies gains traction, with a particular emphasis on concealing energy harvesting infrastructure within weathered floating structures to obfuscate the system’s presence and mitigate theft risks.
Innovative energy harvesting solutions, such as pendulum-based generators championed by researchers (Hao et al., 2020; Xiao et al., 2021), offer promising avenues for augmenting energy autonomy within FSN deployments. However, existing systems suffer from notable drawbacks, including cumbersome size, exorbitant installation costs, and vulnerability to theft. The integration of compact generators concealed within the confines of FAD boxes mitigates visibility concerns, albeit with potential trade-offs in energy output efficiency.
Furthermore, the adoption of wave motion-based generators presents an intriguing proposition, albeit with inherent challenges stemming from the comparatively slower rotation induced by wave motion as opposed to wave impact or wind gusts. Despite potential energy output limitations, such harvesting devices may suffice for meeting the energy demands of low-power sensors. To ensure the sustained viability of the energy source, the deployment of miniaturized energy generators harnessing pendulum motion or submerged underwater generators emerges as compelling strategies worthy of exploration and refinement.
6 Conclusion
This paper has outlined some challenges of the fish sensor networks (FSN) implementation to assist fishermen in increasing their catchments. Natural challenges include wind, waves, currents, corrosion, and radio propagation. Man-made obstacles such as traffic density and physical security, may drift sensor physically. Sensor requires the ability to detect, to quantify fishes under the sea, and to send the information through radio link. Wave and current require serious attention for FSN installation with robust anchoring mechanisms to avoid sensor displacement. Sensor may be drifted due to wind, wave and current, so that weigthing is necessary to ensure fixed location. The corrosive influence of salt-laden water precipitation necessitates FSN material considerations. Sensor installation should consider traffics and physical measure to ensure continous services. Radio links should have sufficient signal budget due to sea surface losses. The water surface is a potent medium for the radio signal reflection and diffraction. The rainy season worsens the attenuation of radio signals. Power requirement may consider the wave harvesting approaches since the appearance of energy source installation such as solar panel construction, reduces the physical security of sensor.
Author contributions
Suherman: Data curation, Formal Analysis, Methodology, Writing–original draft, Writing–review and editing. MA-A: Funding acquisition, Supervision, Validation, Writing–review and editing.
Funding
The author(s) declare that financial support was received for the research, authorship, and/or publication of this article. This research has been funded by Universitas Sumatera Utara, Indonesia through the Talenta Research Schema.
Conflict of interest
The authors declare that the research was conducted in the absence of any commercial or financial relationships that could be construed as a potential conflict of interest.
Publisher’s note
All claims expressed in this article are solely those of the authors and do not necessarily represent those of their affiliated organizations, or those of the publisher, the editors and the reviewers. Any product that may be evaluated in this article, or claim that may be made by its manufacturer, is not guaranteed or endorsed by the publisher.
References
Atmaja, S. B., and Nugroho, D. (2019). Efforts to build and recover small Pelagic fish stock in Java Sea/Upaya membangun kembali dan pemulihan stok ikan Pelagis kecil di laut Jawa. J. Penelit. Perikan. Indones. 25 (3), 179–189. doi:10.15578/jppi.25.3.2019.179-189
Chandrasekhar, V. (2022). ICT applications for developing entrepreneurship in fisheries. ICAR-Central Institute of Fisheries Technology.
Cottura, L., Caradonna, R., Ghigo, A., Novo, R., Bracco, G., and Mattiazzo, G. (2021). Dynamic modeling of an offshore floating wind turbine for application in the Mediterranean Sea. Energies 14 (1), 248. doi:10.3390/en14010248
Cressey, D. (2014). Use of ‘fish aggregating devices’ could be unsustainable. Nature. doi:10.1038/nature.2014.14593
Fassoni-Andrade, A. C., Durand, F., Moreira, D., Azevedo, A., dos Santos, V. F., Funi, C., et al. (2021). Comprehensive bathymetry and intertidal topography of the Amazon estuary. Earth Syst. Sci. Data Discuss. 2021, 2275–2291. doi:10.5194/essd-13-2275-2021
Geraci, M. L., Colloca, F., Di Maio, F., Falsone, F., Fiorentino, F., Sardo, G., et al. (2021). How is artificial lighting affecting the catches in deep water rose shrimp trawl fishery of the Central Mediterranean Sea? Ocean and Coast. Manag. 215, 105970. doi:10.1016/j.ocecoaman.2021.105970
Haag, M. A., Lambert, J. C., Waddell, J. C., and Crampton, W. G. (2019). A new and improved electric fish finder with resources for printed circuit board fabrication. Neotropical Ichthyol. 17, e190099. doi:10.1590/1982-0224-20190099
Hao, C., He, J., Zhang, Z., Yuan, Y., Chou, X., and Xue, C. (2020). A pendulum hybrid generator for water wave energy harvesting and hydrophone-based wireless sensing. AIP Adv. 10 (12). doi:10.1063/5.0036220
Latief, M., Sultan, S., and Suryanto, S. (2020). “Understanding poverty of Indonesia maritime society in five point zero (5.0) era,” in Proceedings of the 1st hasanuddin international conference on social and political sciences, 21–22.
Liu, Y., Wang, H., Cai, L., Shen, X., and Zhao, R. (2021). Fundamentals and advancements of topology discovery in underwater acoustic sensor networks: a review. IEEE Sensors J. 21 (19), 21159–21174. doi:10.1109/jsen.2021.3104533
Menandro, P. S., Bastos, A. C., Misiuk, B., and Brown, C. J. (2022). Applying a multi-method framework to analyze the multispectral acoustic response of the seafloor. Front. Remote Sens. 3, 860282. doi:10.3389/frsen.2022.860282
Oliveira-Pinto, S., and Stokkermans, J. (2020). Assessment of the potential of different floating solar technologies–Overview and analysis of different case studies. Energy Convers. Manag. 211, 112747. doi:10.1016/j.enconman.2020.112747
Perea-Munoz, J. M., Miles, A., and Bayle-Sempere, J. T. (2022). Sharing goals by timely communication improves fishermen’s satisfaction with marine protected areas: a case study in the Mediterranean. Ambio 51 (6), 1520–1534. doi:10.1007/s13280-021-01683-y
Pinem, M., Syafruddin, N., Rambe, A. H., Suherman, , and Zulfin, M. (2020). “Characterization of path loss for radio wave propagation over the sea in 4G network,” in 2020 4rd international conference on electrical, telecommunication and computer engineering (ELTICOM), 72–76.
Rahmadysah, A., Ramadhan, M. C., and Zulyadi, R. (2022). Penegakan Hukum Tindak Pidana Pencurian dengan Kekerasan di Wilayah Hukum Kepolisian Sektor Medan Labuhan. Sci. (JEHSS) 5 (2), 1565–1575. doi:10.34007/jehss.v5i2.1429
Suherman, , Rambe, A. H., Haris, N., Syafrinal, , and Andoni, S. (2022). “Considerations on Fish sensor network installation,” in 2022 6th international conference on electrical, telecommunication and computer engineering (ELTICOM), 102–105.
Suherman, A., Santosa, M. A., Wijayanto, D., Sunoko, R., and Juwana, S. (2020). The eradication of IUU fishing in Indonesia for fisheries resources sustainability by the Task Force 115. Aquac. Aquarium, Conservation and Legislation 13 (5), 2522–2537. doi:10.14710/ijfst.16.3.154-164
Thahir, M. A., Baskoro, M. S., and Gazali, M. (2019). Catchment comparisons between rafia rope rumpon and traditional rumpon in West Aceh/Perbandingan Hasil Tangkapan Pada Rumpon Tali Rafia Dan Rumpon Tradisional di Perairan Aceh Barat. J. Ilmu Dan. Teknol. Kelaut. Trop. 11 (2), 369–376. doi:10.29244/jitkt.v11i2.25031
Tim Redaksi (2021). Banyaknya peristiwa kejahatan/pelanggaran yang dilaporkan menurut jenis kejahatan/pelanggaran 2019-2021. Badan Pus. Stat. Sumat. Utara 34 (493), 1.
Tombak, G. B., and Graha, P. (2016). Study on GPS and fish finder effectifity in increasing fish catchment/Studi kelayakan penerapan teknologi GPS dan fish finder untuk meningkatkan hasil tangkapan ikan. Search Inform. Sci. Entrepreneur, Appl. Art, Res. Humanism 15, 55–60.
Wang, X., Shi, Y., Yang, P., Tao, X., Li, S., Lei, R., et al. (2022). Fish-wearable data snooping platform for underwater energy harvesting and fish behavior monitoring. Small 18 (10), 2107232. doi:10.1002/smll.202107232
Xiao, H., Liu, Z., Zhang, R., Kelham, A., Xu, X., and Wang, X. (2021). Study of a novel rotational speed amplified dual turbine wheel wave energy converter. Appl. Energy 301, 117423. doi:10.1016/j.apenergy.2021.117423
Yasim, A., Sidartawan, R., Widityo, P. G., and Kusnadi, R. F. (2021). Fish finder usage in increasing fish ctachment in Desa Puger Wetan Jember/Penggunaan Fish Finder sebagai Upaya Peningkatan Hasil Tangkapan Nelayan Tradisional di Desa Puger Wetan Kabupaten Jember. J. Ilm. Teknol. Marit. 15 (2), 53–60. doi:10.29122/jurnalwave.v15i02.4749
Keywords: wireless sensor network, fish sensor network, sensor, energy sources, fishermen
Citation: Suherman and Al-Akaidi M (2024) Fish sensor network: WSN application for fishermen. Front. Internet. Things 3:1428157. doi: 10.3389/friot.2024.1428157
Received: 05 May 2024; Accepted: 23 October 2024;
Published: 08 November 2024.
Edited by:
Nitin Auluck, Indian Institute of Technology Ropar, IndiaReviewed by:
Sudeepta Mishra, Indian Institute of Technology Ropar, IndiaCopyright © 2024 Suherman and Al-Akaidi. This is an open-access article distributed under the terms of the Creative Commons Attribution License (CC BY). The use, distribution or reproduction in other forums is permitted, provided the original author(s) and the copyright owner(s) are credited and that the original publication in this journal is cited, in accordance with accepted academic practice. No use, distribution or reproduction is permitted which does not comply with these terms.
*Correspondence: Marwan Al-Akaidi, mmalakaidi@gmail.com