- 1Department of Neurology, Center for Translational Neuro- and Behavioral Sciences (C-TNBS), Essen University Hospital, University of Duisburg-Essen, Essen, Germany
- 2School of Physiology, Pharmacology and Neuroscience, University of Bristol, Bristol, United Kingdom
The role of the cerebellum in emotional control has gained increasing interest, with studies showing it is involved in fear learning and memory in both humans and rodents. This review will focus on the contributions of the cerebellum to the extinction of learned fear responses. Extinction of fearful memories is critical for adaptive behaviour, and is clinically relevant to anxiety disorders such as post-traumatic stress disorder, in which deficits in extinction processes are thought to occur. We present evidence that supports cerebellar involvement in fear extinction, from rodent studies that investigate molecular mechanisms and functional connectivity with other brain regions of the known fear extinction network, to fMRI studies in humans. This evidence is considered in relation to the theoretical framework that the cerebellum is involved in the formation and updating of internal models of the inner and outer world by detecting errors between predicted and actual outcomes. In the case of fear conditioning, these internal models are thought to predict the occurrence of an aversive unconditioned stimulus (US), and when the aversive US is unexpectedly omitted during extinction learning the cerebellum uses prediction errors to update the internal model. Differences between human and rodent studies are highlighted to help inform future work.
Introduction
Learned fear is a key survival response, but successfully extinguishing fear when the threat is no longer present is equally important for behavioural adaptation. It is thought that an inability to extinguish fearful memories underlies anxiety disorders such as post-traumatic stress disorder (Milad and Quirk, 2012). All animals (including humans) have a variety of defensive responses to a threat. While humans can be interviewed about their emotional feeling of fear towards a threat, defensive behaviours such as flight/freezing are often used as a proxy for the fear state in rodents and, as a result, caution is needed in such an assignment. Under experimental conditions, defensive responses can be elicited using fear conditioning paradigms which provide a model in both humans and other species to study “fear” over time. Defensive responses can be triggered not only by the adverse stimulus itself, but also by an initially neutral stimulus predicting the occurrence of an adverse event. Pavlovian fear conditioning paradigms use this characteristic to study behavioural and physiological mechanisms involved in fear acquisition and extinction. During fear acquisition, an unconditioned stimulus (US), inducing innate fear, is repeatedly paired with a conditioned neutral stimulus (CS) to elicit fearful behavioural responses, and so the CS becomes the CS+. To investigate extinction of a fearful memory, the experimenter can observe the conditioned responses when the US is no longer paired with the CS. As extinction training progresses, CS+ related behavioural conditioned responses gradually decrease due to the unexpected omission of the aversive US. Over repeated presentations of the CS alone the omission of the aversive US leads to prediction of the absence of the US, termed extinction learning. This can be compared over time with a CS of similar intensity that has not been paired, termed a CS−.
The contribution of the cerebellum to the emotional network has been described since the late 1930s (Clark, 1939; Zanchetti and Zoccolini, 1954; Berman et al., 1974), however, more recently, it has gained increasing interest in relation to conditioned fear behaviour (Sacchetti et al., 2002; Frontera et al., 2020; Vaaga et al., 2020; Lawrenson et al., 2022). Previous studies have found that the cerebellum is involved in the extinction of learned motor responses (Ernst et al., 2017), raising the possibility that the cerebellum may be supporting a similar role in the extinction of learned emotional responses. This review focuses on evidence for such a role in conditioned fear extinction, from rodent studies that investigate cerebellar functional connectivity with other brain regions and underlying molecular mechanisms, to fMRI studies in humans.
The neurocircuitry underpinning the cerebellum in adaptive fear behaviours
The neurocircuitry and regional activation underpinning conditioned fear learning and memory has been mapped extensively in rodents (Apps and Strata, 2015; Tovote et al., 2015) and humans (Fullana et al., 2016; Greco and Liberzon, 2016). The cerebellum has been found to play a role in motor (Koutsikou et al., 2014), autonomic (Supple and Leaton, 1990), emotional and cognitive (Timmann et al., 2010; Apps and Strata, 2015; Guell et al., 2018) functions relating to conditioned fear learning (Leaton, 2003; Strata, 2015). Broadly speaking the cerebellum is widely thought to act as, or is part of, an internal predictive system, implicated in associative appetitive and fear learning processes driven by prediction error corresponding to the discrepancy between predicted and actual outcomes (Popa and Ebner, 2019). Even though its role is not fully understood, a summary of its functional and structural connectivity with vital components of the limbic system have previously been highlighted by Apps and Strata (2015). The cerebellum is connected directly with the periaqueductal grey (Teune et al., 2000; Koutsikou et al., 2014; Frontera et al., 2020; Vaaga et al., 2020; Lawrenson et al., 2022), the ventral tegmental area (VTA; Watabe-Uchida et al., 2012; Carta et al., 2019; Pisano et al., 2021), the thalamus (Watabe-Uchida et al., 2012; Apps and Strata, 2015; Gornati et al., 2018; Pisano et al., 2021) and hypothalamus (Dietrichs and Haines, 1989; Apps and Strata, 2015) as well as indirectly with other cortical and subcortical structures such as the amygdala (Jung et al., 2022), the anterior cingulate cortex, hippocampus and the striatum (Moreno-Rius, 2018).
The cerebellar-periaqueductal grey pathway and fear extinction: rodent studies
In recent years, there has been a focus on the function of reciprocal connections between the cerebellum and the ventrolateral region of the periaqueductal grey (vlPAG; Whiteside and Snider, 1953; Teune et al., 2000; Koutsikou et al., 2014). The vlPAG is known to play a role in defensive behaviours including freezing (Koutsikou et al., 2014; Tovote et al., 2016) and recent histological studies, have shown in mice that glutamatergic projection neurons from the medial cerebellar nuclei (MCN) make direct connections with GABAergic, glutamatergic and dopaminergic neurons in the vlPAG (see Figure 1; Frontera et al., 2020; Vaaga et al., 2020).
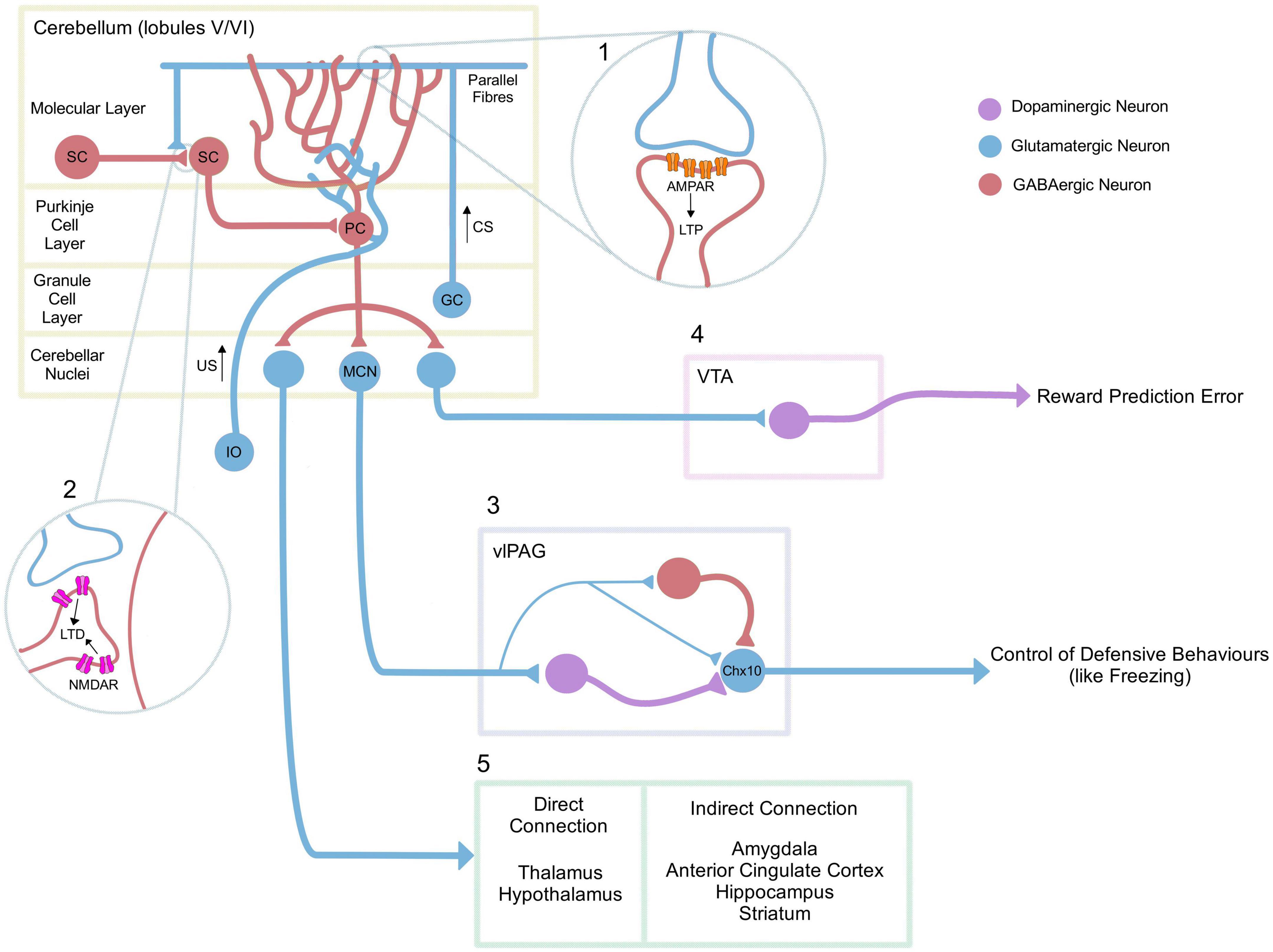
Figure 1. Neurocircuitry underlying adaptive cerebellar fear processes. In classical pavlovian conditioning, the inferior olive (IO) signals the US, while the mossy fibre-parallel fibre pathway (MF-PF) signals the unconditional stimulus. (1) During the acquisition of a fear memory, there is an upregulation of AMPA receptors at the PF-PC synapse, resulting in post-synaptic long-term potentiation (LTP; Sacchetti et al., 2004; Zhu et al., 2007), as well as an increase of GABAergic signalling onto Purkinje cells from molecular layer interneurons/stellate cells (SC) (Scelfo et al., 2008). Various cerebellar lobules in rodents have been associated with fear learning, but the underlying mechanisms relating to LTP have focused primarily on lobules V/VI. (2) In contrast, NMDAR-mediated long-term depression (LTD) at SC-SC synapses has been associated with extinction (Dubois and Liu, 2021). (3) The medial cerebellar nuclei (MCN) have also been directly associated with fear extinction (Frontera et al., 2020), via projections to dopaminergic, GABAergic and glutamatergic neurons in the vlPAG, Vaaga et al. (2020). MCN projections are capable of modulating glutamatergic neurons that express the transcription factor Chx10 in the vlPAG (Vaaga et al., 2020) which have previously been associated with freezing behaviour through their projection to the magnocellular reticular nucleus. This in turn is directly connected to motor neurons of the spinal cord (Tovote et al., 2016). (4) The cerebellar nuclei also project to the VTA; an area associated with the enhancement of fear extinction learning (Carta et al., 2019). (5) Finally, the cerebellum also projects either directly (thalamus, hypothalamus) or indirectly (amygdala, anterior cingulate cortex, hippocampus, striatum) to multiple other areas that could be related to the fear network (Apps and Strata, 2015). PC: Purkinje Cell, MCN: Medial Cerebellar Nuclei, SC: Stellate Cell, GC: Granule Cell, CS: Conditioned Stimulus, US: Unconditioned Stimulus, VTA: Ventral Tegmental Area, vlPAG: VentroLateral PeriAqueductal Grey.
Targeted inhibition or excitation using Designer Receptors Exclusively Activated by Designer Drugs (DREADDs) of the MCN-vlPAG pathway in mice demonstrated that this pathway is involved in extinction of a conditioned response (Frontera et al., 2020). Frontera et al. (2020) showed that inhibition of the MCN-vlPAG pathway during extinction resulted in an increase in freezing behaviour that persisted after the manipulation and during an extinction recall test. When the pathway was activated there was also a smaller, slower, and less pronounced increase of freezing behaviour in comparison to controls which was abolished in extinction recall testing (Frontera et al., 2020). It is unclear why both inhibition and excitation of the pathway using DREADDs resulted in an effect in a similar direction. Temporally targeted optogenetic stimulation of the MCN-vlPAG pathway during delivery of the CS+ tone offset caused a large impairment of conditioned freezing during all the extinction sessions, but did not change extinction recall testing suggesting this modulation was affecting the expression of fear behaviour, but not the memory of extinction itself. Likewise, optogenetic stimulation of the pathway during the pairing of the US foot-shock with the conditioned tone resulted in decreased freezing levels overall during extinction. These data suggest the pathway is involved in supporting the process of extinction or the expression of fear behaviour, but it is unclear whether it contributes to extinction memory.
Further evidence that the cerebellum modulates vlPAG function was demonstrated following pharmacological inactivation of the MCN during consolidation (Lawrenson et al., 2022). Lawrenson et al. (2022) recorded temporally precise single unit activity in the vlPAG in response to the onset and offset of conditioned auditory cue during extinction. Modulation of MCN output during consolidation disrupted the temporal precision at tone offset during early extinction, which was associated with an increase in overall vlPAG unit responsiveness (as measured by response area). This manipulation also resulted in an increase in the duration of freezing epochs in comparison to controls during early extinction, suggesting inhibition of the MCN during fear consolidation affects how freezing behaviour is expressed during recall of the fear memory.
Other experiments targeting the MCN-vlPAG pathway have demonstrated that its modulation during acquisition of the fear memory affects fear-related freezing behaviour during extinction, but does not test extinction directly (Frontera et al., 2020; Lawrenson et al., 2022). These experiments agree that inhibition of the MCN-vlPAG pathway strengthens acquisition of the fear memory, resulting in increased levels of freezing during extinction in comparison with controls, while excitation of the pathway reduces acquisition of the fear memory resulting in decreased freezing during recall of the fear memory and extinction.
Together these experiments (Frontera et al., 2020; Lawrenson et al., 2022) provide evidence that the role of the cerebellum via its interactions with the vlPAG is to support fear acquisition, fear memory and the reduction of a cued fear memory to benefit extinction. It is currently unclear whether this pathway contributes to extinction recall and memory of extinguished fear.
Molecular and physiological mechanisms underlying fear extinction in the cerebellum
In studies using the eyeblink reflex (where a CS tone is paired with an US air puff to the eye), Medina et al. (2002), showed that a disinhibition of the inferior olive prevented extinction of the conditioned response suggesting that both the US during acquisition and lack of US during extinction are encoded by the climbing fibre system, which could in part arise via inhibitory input from the cerebellar nuclei. They suggested that the difference between acquisition versus extinction is reflected by climbing fibre signalling relative to background levels of neuronal activity. It is possible that a similar mechanism with a bidirectional modulation of climbing fibres contributes to adaptive regulation of emotional control in the cerebellum.
A number of studies have also investigated the molecular mechanisms underlying fear learning and memory relating to Long-Term Potentiation (LTP) (Sacchetti et al., 2004; Zhu et al., 2007), but very few have investigated extinction. Following fear acquisition in mice, there is an increase in GABAergic signalling from molecular layer interneurons (MLIs) onto Purkinje cells (Scelfo et al., 2008) and neighbouring MLIs in cerebellar lobules V/VI (Dubois and Liu, 2021). This increase in GABAergic signalling between MLIs was abolished in an NMDA-dependent manner during fear extinction, as shown by the deletion of the GluN2D NMDA receptor subunit. Importantly this deletion did not affect acquisition or consolidation but did impair fear extinction (Dubois and Liu, 2021). Blocking GABAergic signalling specifically in granule cells, however, has been shown to cause an increase in general anxiety in mice, especially in females, which also showed decreased interest in social interactions with other female mice, and impaired maternal behaviour (Rudolph et al., 2020). However, this raises the question of whether GABAergic signalling is involved in extinction processes or if it is affecting the general emotional state of the animal.
Overall, current research is lacking a cohesive and integrative understanding of the molecular and physiological mechanisms of cerebellar fear extinction which are needed to support and better understand behavioural findings from rodent and human studies.
The role of the cerebellum in fear extinction: evidence from human studies
In humans, the cerebellum has been shown to be involved in the acquisition of learned fear (Casey et al., 1994; Ploghaus et al., 1999; Knight et al., 2005; Carlsson et al., 2006; Carlson et al., 2011; Cacciaglia et al., 2013; Michelle Welman et al., 2018), but less is known about its role in fear extinction (Lange et al., 2015). The fMRI studies that report activation in the cerebellum during fear extinction are shown in Table 1. Most sites of activation are in posterolateral regions (Linnman et al., 2012; Faul et al., 2020; Graner et al., 2020; Labrenz et al., 2022), while other studies report activation in the vermis (Kattoor et al., 2014; Utz et al., 2015). These inconsistencies are likely due to differences in experimental design (US type, location and saliency, reinforcement frequency, inclusion of changes of context) and fMRI analysis. For example, Kattoor et al. (2014) were able to detect both posterolateral and vermal differential sites of activation in early, but not late extinction using a visceral US and geometric shapes as conditioned visual stimuli. In comparison, another study also using geometric shapes as the CS, but paired with an electrical stimulation as the US, found only vermal activation in extinction (Utz et al., 2015). A virtual reality study reported activity in lobule VI during late extinction only when CSs (corresponding to different male avatars) were located nearby, and not when presented further away (Faul et al., 2020). Another study using visceral pain as the US reported CS + related activity in early extinction in posterolateral lobules VI, Crus I and Crus II. The CS + related activity was similar to activation during acquisition, and could therefore represent activity related to a remaining fear response (Labrenz et al., 2022).
In a more recent fMRI study Ernst et al. (2019) did not find differential CS activation during fear extinction, but did find activation in the US window corresponding to the omission of the US during extinction in left Crus I. Activation was much stronger for repeated unexpected omissions of the US in acquisition, where partial reinforcement allowed the analysis of fMRI responses during CS + no-US windows. As the aversive stimulus is predicted but not received, this activity is hypothesized to be related to prediction error processing which may drive fear extinction learning. During unexpected omissions in acquisition, a differential pattern of activation was again found in left Crus I, but also right Crus I and bilateral lobule VI, Crus II and vermis (Ernst et al., 2019). These observations are to some extent in accordance with another study (Yágüez et al., 2005) where a 50% reinforced phase was followed by a 100% reinforced learning phase. In the 50% reinforced phase, activity during the US window in unreinforced trials resulted in a small but significant cluster in left Crus I. While Yágüez et al. (2005) argue that this activity represents US anticipation without influence of the US presentation, it could instead be interpreted as prediction error related activity due to an unexpected omission of an aversive stimulus.
Different parts of the cerebellum have been reported to match both the unexpected presentation or omission of the US (Ploghaus et al., 2000). In both cases fMRI signals increased indicating an unsigned prediction error, however, activation in response to the unexpected US presentation is likely related to both the stimulus itself and prediction error. This study demonstrated cerebellar activity during the unexpected omission of the US in the initial extinction trial, with mean activities cantered bilaterally around lobule VI. Prediction error related activity during extinction was also found in lobule VI and Crus I on a trend level in a recent study, which used a deep learning model to estimate prediction error values for an fMRI analysis with parametric modulation (Batsikadze et al., 2022). In essence, human fMRI research typically implicates the posterolateral lobules in fear extinction, while the vermis may also play a role, and US window activations suggest an involvement in prediction error processing.
The absence of an expected aversive US results in a better-than-expected outcome, which may be internally perceived and treated as a rewarding event. Consequently, fear extinction learning may be a form of reinforcement learning (Kalisch et al., 2019). In support of this claim, a recent study in mice documented direct excitatory projections from the cerebellar nuclei to the VTA (Carta et al., 2019); a region implicated in the reward circuit (Carta et al., 2019). Dopaminergic (DA) neurons in the VTA are activated following an unexpected US omission during fear extinction (Luo et al., 2018; Salinas-Hernández et al., 2018), specifically during early trials when the prediction error is the highest (Salinas-Hernández et al., 2018). Furthermore, inhibition or excitation of VTA DA neurons at the time of US omission during extinction was, respectively, linked with impairment and enhancement of fear extinction learning. These findings might indicate a role for the cerebellum in reward and reward prediction error processing driving fear extinction.
Until now the role of the human cerebellum in fear extinction has almost exclusively been studied with fMRI in healthy participants. Valuable insights might also be found in patients with cerebellar disease. For example, five patients with lesions of the cerebellar vermis demonstrated a lack of fear conditioned bradycardia, even though no differences were found with matched controls regarding skin conduction responses (Maschke et al., 2002). Additionally, non-invasive brain stimulation could provide a method for altering cerebellar activity during fear extinction (Klaus and Schutter, 2022), and test fMRI observations. For instance, transcranial direct current stimulation (tDCS) applied over the right dorsolateral prefrontal cortex before and during extinction was found to change connectivity in the anterior lobe of the cerebellum and lobule VI (Ganho-Ávila et al., 2022). However, likely due to the highly convoluted cerebellar cortex the effects of cerebellar tDCS have been difficult to reproduce in cerebellar-dependent learning paradigms, such as eyeblink conditioning and reach adaptation (Kimpel et al., 2020). Attention instead has moved to the possibility of using other non-invasive stimulation techniques such as transcranial focused ultrasound neuromodulation to activate the cerebellum. This method has shown over the last years promising results in reaching deep brain structures in humans and mice by enabling higher spatial resolution modulation (di Biase et al., 2019). Combining fMRI and EEG can also provide increased temporal resolution to study the cerebellum and its role in the timing of cues in the fear network (Warbrick, 2022).
Linking rodent and human cerebellar research in fear extinction
There are a number of important differences between rodent and human cerebellar research regarding fear extinction that currently make it difficult to directly compare results across species. Rodent studies have primarily used males, whereas human studies involve both sexes. Indeed, growing evidence indicates that anxiety disorders are prevalent in females (McLean et al., 2011) and that sex hormones have an effect on fear extinction processes (Graham and Milad, 2013; Merz et al., 2018), making it central to consider both sexes. In rodents, the US signal is most commonly presented in the form of an electrical shock while in humans the US signals are more diverse, including the use of sounds (scream or loud noise), visceral pain or olfactory cues (rotten egg smell), as well as electrical shocks. Furthermore, it is likely that in rodents the US is perceived as a real threat, while in humans it is perceived as an artificial experiment. In the experiments described above, the CS in rodents is an auditory signal (which can be variable in frequency, duration and tone type). In humans the CS’s used are predominantly visual, while auditory cues such as tones can also be used. This variability in US and CS signals between rodents and humans, as well as across studies within the same species, needs to be addressed in order to make studies more translatable and reproducible. Behavioural measures of defensive state in rodents (e.g., freezing) are not directly comparable with autonomic measures of fear in humans (e.g., skin conductance responses). Moreover, rodent research typically involves targeted manipulation of specific cerebellar regions (e.g., cerebellar vermis and MCN) or neuronal types, that allows investigation of distinct neural pathways, but lacks a comprehensive overview of all potential cerebellar areas and projections involved. In humans, fMRI allows for observation of the whole cerebellar cortex during fear extinction, however, focused imaging of the human cerebellar nuclei and its outputs is lacking. Often the strongest fMRI signal is observed in the lateral hemispheres, while in rodents the focus has been on the vermis and MCN meaning a direct comparison is not possible. To date, rodent fMRI studies during fear learning have excluded the cerebellum (Brydges et al., 2013; Harris et al., 2015), and so its future inclusion is essential to better understand the cerebellar role in fear extinction.
Discussion
In summary, there is a range of anatomical, physiological, behavioural, and imaging evidence that the cerebellum plays a role in the extinction of conditioned defensive states in rodents and humans. Taking into consideration the multiple connections of the cerebellum with the fear network (Apps and Strata, 2015; Tovote et al., 2015) and the role of the cerebellum in fear learning and extinction processing (Frontera et al., 2020; Lawrenson et al., 2022), its overall contribution is likely to coordinate different aspects of fear circuitry in order to facilitate the temporally appropriate adaptive responses of the organism towards changes in the environment. There are a number of key unknowns highlighted in this review. In particular, current research is lacking a basic understanding of the molecular and physiological mechanisms underlying cerebellar fear extinction processes. In rodent and human studies, further fear extinction experiments are also needed to better understand the role of cerebellar projections with other key regions in the fear network such as the VTA in reward/safety signalling. It is also unclear how different cerebellar regions in both rodents and humans contribute to the emotional, motor, sensory and autonomic components of fear extinction, and how this changes with task specificity. Do individual differences in the way the cerebellum processes fear learning and extinction contribute to anxiety disorders? Given the prevalence of anxiety disorders in women, it is also unknown whether there are sex differences relating to cerebellar function. Furthermore, the question remains, how can we better link human and rodent studies to make data more comparable so that rodent studies are more clinically relevant?
Author contributions
FS-S, VS, EN, and AD collaborated equally on the preparation of the manuscript. FS-S wrote the sections on molecular studies in rodents and created Figure 1, while also contributing to the discussion. VS focused on other rodent-related sections and helped with the introduction. EN wrote the portions on human fMRI studies, created the table, and contributed to the discussion. AD wrote other human-related parts of the review and contributed to the introduction. CL supervised review, provided the correction, and revisions. DT and RA gave feedback and proofreading. All authors contributed to the design and conceptualization of the review and approved the final submitted version.
Funding
This work has received funding from the European Union’s Horizon 2020 Research and Innovation Programme under the Marie Skłodowska-Curie grant agreement No. 956414. This work was also supported by a grant from the German Research Foundation (DFG; project number: 316,803,389—SFB 1280) to DT (subproject A05), and the UKRI Medical Research Council (MRC; project number: MR/T019484/1).
Conflict of interest
The authors declare that the research was conducted in the absence of any commercial or financial relationships that could be construed as a potential conflict of interest.
Publisher’s note
All claims expressed in this article are solely those of the authors and do not necessarily represent those of their affiliated organizations, or those of the publisher, the editors and the reviewers. Any product that may be evaluated in this article, or claim that may be made by its manufacturer, is not guaranteed or endorsed by the publisher.
Abbreviations
CS, conditioned stimulus; LTP, long-term potentiation; MCN, medial cerebellar nuclei; MLIs, molecular layer interneurons; US, unconditioned stimulus; VTA, ventral tegmental area; vlPAG, Ventrolateral Periaqueductal Grey.
References
Apps, R., and Strata, P. (2015). Neuronal circuits for fear and anxiety — the missing link. Nat. Rev. Neurosci. 16, 642–642. doi: 10.1038/nrn4028
Batsikadze, G., Diekmann, N., Ernst, T. M., Klein, M., Maderwald, S., Deuschl, C., et al. (2022). The cerebellum contributes to context-effects during fear extinction learning: A 7T fMRI study. Neuroimage 253:119080. doi: 10.1016/j.neuroimage.2022.119080
Berman, A. J., Berman, D., and Prescott, J. W. (1974). “The effect of cerebellar lesions on emotional behavior in the rhesus monkey,” in The cerebellum, epilepsy, and behavior, eds I. S. Cooper, M. Riklan, and R. S. Snider (Boston, MA: Springer). doi: 10.1007/978-1-4613-4508-4_12
Brydges, N. M., Whalley, H. C., Jansen, M. A., Merrifield, G. D., Wood, E. R., Lawrie, S. M., et al. (2013). Imaging conditioned fear circuitry using awake rodent fMRI. PLoS One 8:e54197. doi: 10.1371/journal.pone.0054197
Cacciaglia, R., Nees, F., Pohlack, S. T., Ruttorf, M., Winkelmann, T., Witt, S. H., et al. (2013). A risk variant for alcoholism in the NMDA receptor affects amygdala activity during fear conditioning in humans. Biol. Psychol. 94, 74–81. doi: 10.1016/j.biopsycho.2013.05.006
Carlson, J. M., Greenberg, T., Rubin, D., and Mujica-Parodi, L. R. (2011). Feeling anxious: Anticipatory amygdalo-insular response predicts the feeling of anxious anticipation. Soc. Cogn. Affect. Neurosci. 6, 74–81. doi: 10.1093/scan/nsq017
Carlsson, K., Andersson, J., Petrovic, P., Petersson, K. M., Öhman, A., and Ingvar, M. (2006). Predictability modulates the affective and sensory-discriminative neural processing of pain. Neuroimage 32, 1804–1814. doi: 10.1016/j.neuroimage.2006.05.027
Carta, I., Chen, C. H., Schott, A. L., Dorizan, S., and Khodakhah, K. (2019). Cerebellar modulation of the reward circuitry and social behavior. Science 363:eaav0581. doi: 10.1126/science.aav0581
Casey, K. L., Minoshima, S., Berger, K. L., Koeppe, R. A., Morrow, T. J., and Frey, K. A. (1994). Positron emission tomographic analysis of cerebral structures activated specifically by repetitive noxious heat stimuli. J. Neurophysiol. 71, 802–807. doi: 10.1152/jn.1994.71.2.802
Clark, S. L. (1939). Responses following electrical stimulation of the cerebellar cortex in the normal cat. J. Neurophysiol. 2, 19–35. doi: 10.1152/jn.1939.2.1.19
di Biase, L., Falato, E., and Di Lazzaro, V. (2019). Transcranial focused ultrasound (tFUS) and transcranial unfocused ultrasound (tUS) neuromodulation: From theoretical principles to stimulation practices. Front. Neurol. 10:549. doi: 10.3389/fneur.2019.00549
Dietrichs, E., and Haines, D. E. (1989). Interconnections between hypothalamus and cerebellum. Anat. Embryol. 179, 207–220. doi: 10.1007/BF00326585
Dubois, C. J., and Liu, S. J. (2021). GluN2D NMDA receptors gate fear extinction learning and interneuron plasticity. Front. Synaptic Neurosci. 13:681068. doi: 10.3389/fnsyn.2021.681068
Ernst, T. M., Brol, A. E., Gratz, M., Ritter, C., Bingel, U., Schlamann, M., et al. (2019). The cerebellum is involved in processing of predictions and prediction errors in a fear conditioning paradigm. Elife 8:e46831. doi: 10.7554/eLife.46831
Ernst, T. M., Thürling, M., Müller, S., Kahl, F., Maderwald, S., Schlamann, M., et al. (2017). Modulation of 7 T fMRI signal in the cerebellar cortex and nuclei during acquisition, extinction, and reacquisition of conditioned eyeblink responses. Hum. Brain Mapp. 38, 3957–3974. doi: 10.1002/hbm.23641
Faul, L., Stjepanovic, D., Stivers, J. M., Stewart, G. W., Graner, J. L., Morey, R. A., et al. (2020). Proximal threats promote enhanced acquisition and persistence of reactive fear-learning circuits. Proc. Natl. Acad. Sci. U.S.A. 117, 16678–16689. doi: 10.1073/pnas.2004258117
Frontera, J. L., Baba Aissa, H., Sala, R. W., Mailhes-Hamon, C., Georgescu, I. A., Léna, C., et al. (2020). Bidirectional control of fear memories by cerebellar neurons projecting to the ventrolateral periaqueductal grey. Nat. Commun. 11:5207. doi: 10.1038/s41467-020-18953-0
Fullana, M. A., Harrison, B. J., Soriano-Mas, C., Vervliet, B., Cardoner, N., Àvila-Parcet, A., et al. (2016). Neural signatures of human fear conditioning: An updated and extended meta-analysis of fMRI studies. Mol. Psychiatry 21, 500–508. doi: 10.1038/mp.2015.88
Ganho-Ávila, A., Guiomar, R., Valério, D., Gonçalves, ÓF., and Almeida, J. (2022). Offline tDCS modulates prefrontal-cortical–subcortical-cerebellar fear pathways in delayed fear extinction. Exp. Brain Res. 240, 221–235. doi: 10.1007/s00221-021-06248-9
Gornati, S. V., Schäfer, C. B., Eelkman Rooda, O. H. J., Nigg, A. L., De Zeeuw, C. I., and Hoebeek, F. E. (2018). Differentiating cerebellar impact on thalamic nuclei. Cell Rep. 23, 2690–2704. doi: 10.1016/j.celrep.2018.04.098
Graham, B. M., and Milad, M. R. (2013). Blockade of estrogen by hormonal contraceptives impairs fear extinction in female rats and women. Biol. Psychiatry 73, 371–378. doi: 10.1016/j.biopsych.2012.09.018
Graner, J. L., Stjepanović, D., and LaBar, K. S. (2020). Extinction learning alters the neural representation of conditioned fear. Cogn. Affect. Behav. Neurosci. 20, 983–997. doi: 10.3758/s13415-020-00814-4
Greco, J. A., and Liberzon, I. (2016). Neuroimaging of fear-associated learning. Neuropsychopharmacology 41, 320–334. doi: 10.1038/npp.2015.255
Guell, X., Gabrieli, J. D. E., and Schmahmann, J. D. (2018). Triple representation of language, working memory, social and emotion processing in the cerebellum: Convergent evidence from task and seed-based resting-state fMRI analyses in a single large cohort. Neuroimage 172, 437–449. doi: 10.1016/j.neuroimage.2018.01.082
Harris, A. P., Lennen, R. J., Marshall, I., Jansen, M. A., Pernet, C. R., Brydges, N. M., et al. (2015). Imaging learned fear circuitry in awake mice using fMRI. Eur. J. Neurosci. 42, 2125–2134. doi: 10.1111/ejn.12939
Jung, S. J., Vlasov, K., D’Ambra, A. F., Parigi, A., Baya, M., Frez, E. P., et al. (2022). Novel Cerebello-amygdala connections provide missing link between cerebellum and limbic system. Front. Syst. Neurosci. 16:879634. doi: 10.3389/fnsys.2022.879634
Kalisch, R., Gerlicher, A. M. V., and Duvarci, S. (2019). A Dopaminergic Basis for Fear Extinction. Trends Cogn. Sci. 23, 274–277. doi: 10.1016/j.tics.2019.01.013
Kattoor, J., Thürling, M., Gizewski, E. R., Forsting, M., Timmann, D., and Elsenbruch, S. (2014). Cerebellar contributions to different phases of visceral aversive extinction learning. Cerebellum 13, 1–8. doi: 10.1007/s12311-013-0512-9
Kimpel, O., Hulst, T., Batsikadze, G., Ernst, T. M., Nitsche, M. A., Timmann, D., et al. (2020). Long-term effects of cerebellar anodal transcranial direct current stimulation (tDCS) on the acquisition and extinction of conditioned eyeblink responses. Sci. Rep. 10:22434. doi: 10.1038/s41598-020-80023-8
Klaus, J., and Schutter, D. J. L. G. (2022). Non-invasive brain stimulation of the cerebellum in emotion. Adv. Exp. Med. Biol. 1378, 109–121. doi: 10.1007/978-3-030-99550-8_8
Knight, D. C., Nguyen, H. T., and Bandettini, P. A. (2005). The role of the human amygdala in the production of conditioned fear responses. Neuroimage 26, 1193–1200. doi: 10.1016/j.neuroimage.2005.03.020
Koutsikou, S., Crook, J. J., Earl, E. V., Leith, J. L., Watson, T. C., Lumb, B. M., et al. (2014). Neural substrates underlying fear-evoked freezing: The periaqueductal grey-cerebellar link. J. Physiol. 592, 2197–2213. doi: 10.1113/jphysiol.2013.268714
Labrenz, F., Spisák, T., Ernst, T. M., Gomes, C. A., Quick, H. H., Axmacher, N., et al. (2022). Temporal dynamics of fMRI signal changes during conditioned interoceptive pain-related fear and safety acquisition and extinction. Behav. Brain Res. 427:113868. doi: 10.1016/j.bbr.2022.113868
Lange, I., Kasanova, Z., Goossens, L., Leibold, N., De Zeeuw, C. I., van Amelsvoort, T., et al. (2015). The anatomy of fear learning in the cerebellum: A systematic meta-analysis. Neurosci. Biobehav. Rev. 59, 83–91. doi: 10.1016/j.neubiorev.2015.09.019
Lawrenson, C., Paci, E., Pickford, J., Drake, R. A. R., Lumb, B. M., and Apps, R. (2022). Cerebellar modulation of memory encoding in the periaqueductal grey and fear behaviour. Elife 11:e76278. doi: 10.7554/eLife.76278
Linnman, C., Zeidan, M. A., Furtak, S. C., Pitman, R. K., Quirk, G. J., and Milad, M. R. (2012). Resting amygdala and medial prefrontal metabolism predicts functional activation of the fear extinction circuit. Am. J. Psychiatry 169, 415–423. doi: 10.1176/appi.ajp.2011.10121780
Lonsdorf, T. B., Menz, M. M., Andreatta, M., Fullana, M. A., Golkar, A., Haaker, J., et al. (2017). Don’t fear ‘fear conditioning’: Methodological considerations for the design and analysis of studies on human fear acquisition, extinction, and return of fear. Neurosci. Biobehav. Rev. 77, 247–285. doi: 10.1016/j.neubiorev.2017.02.026
Luo, R., Uematsu, A., Weitemier, A., Aquili, L., Koivumaa, J., McHugh, T. J., et al. (2018). A dopaminergic switch for fear to safety transitions. Nat. Commun. 9:2483. doi: 10.1038/s41467-018-04784-7
Maschke, M., Schugens, M., Kindsvater, K., Drepper, J., Kolb, F. P., Diener, H. C., et al. (2002). Fear conditioned changes of heart rate in patients with medial cerebellar lesions. J. Neurol. Neurosurg. Psychiatry 72, 116–118. doi: 10.1136/jnnp.72.1.116
McLean, C. P., Asnaani, A., Litz, B. T., and Hofmann, S. G. (2011). Gender differences in anxiety disorders: Prevalence, course of illness, comorbidity and burden of illness. J. Psychiatr. Res. 45, 1027–1035. doi: 10.1016/j.jpsychires.2011.03.006
Medina, J. F., Nores, W. L., and Mauk, M. D. (2002). Inhibition of climbing fibres is a signal for the extinction of conditioned eyelid responses. Nature 416, 330–333. doi: 10.1038/416330a
Merz, C. J., Kinner, V. L., and Wolf, O. T. (2018). Let’s talk about sex …differences in human fear conditioning. Curr. Opin. Behav. Sci. 23, 7–12. doi: 10.1016/j.cobeha.2018.01.021
Michelle Welman, F. H. S., Smit, A. E., Jongen, J. L. M., Tibboel, D., van der Geest, J. N., and Holstege, J. C. (2018). Pain experience is somatotopically organized and overlaps with pain anticipation in the human cerebellum. Cerebellum 17, 447–460. doi: 10.1007/s12311-018-0930-9
Milad, M. R., and Quirk, G. J. (2012). Fear extinction as a model for translational neuroscience: Ten years of progress. Annu. Rev. Psychol. 63, 129–151. doi: 10.1146/annurev.psych.121208.131631
Moreno-Rius, J. (2018). The cerebellum in fear and anxiety-related disorders. Prog. Neuro-Psychopharmacol. Biol. Psychiatry 85, 23–32. doi: 10.1016/j.pnpbp.2018.04.002
Pisano, T. J., Dhanerawala, Z. M., Kislin, M., Bakshinskaya, D., Engel, E. A., Hansen, E. J., et al. (2021). Homologous organization of cerebellar pathways to sensory, motor, and associative forebrain. Cell Rep. 36:109721. doi: 10.1016/j.celrep.2021.109721
Ploghaus, A., Tracey, I., Clare, S., Gati, J. S., Rawlins, J. N. P., and Matthews, P. M. (2000). Learning about pain: The neural substrate of the prediction error for aversive events. Proc. Natl. Acad. Sci. U.S.A. 97, 9281–9286. doi: 10.1073/pnas.160266497
Ploghaus, A., Tracey, I., Gati, J. S., Clare, S., Menon, R. S., Matthews, P. M., et al. (1999). Dissociating pain from its anticipation in the human brain. Science 284, 1979–1981. doi: 10.1126/science.284.5422.1979
Popa, L. S., and Ebner, T. J. (2019). Cerebellum, predictions and errors. Front. Cell. Neurosci. 12:524. doi: 10.3389/fncel.2018.00524
Rudolph, S., Guo, C., Pashkovski, S. L., Osorno, T., Gillis, W. F., Krauss, J. M., et al. (2020). Cerebellum-specific deletion of the GABAA receptor δ subunit leads to sex-specific disruption of behavior. Cell Rep. 33:108338. doi: 10.1016/j.celrep.2020.108338
Sacchetti, B., Baldi, E., Ambrogi Lorenzini, C., and Bucherelli, C. (2002). Cerebellar role in fear-conditioning consolidation. Proc. Natl. Acad. Sci. U.S.A. 99, 8406–8411. doi: 10.1073/pnas.112660399
Sacchetti, B., Scelfo, B., Tempia, F., and Strata, P. (2004). Long-term synaptic changes induced in the cerebellar cortex by fear conditioning. Neuron 42, 973–982. doi: 10.1016/j.neuron.2004.05.012
Salinas-Hernández, X. I., Vogel, P., Betz, S., Kalisch, R., Sigurdsson, T., and Duvarci, S. (2018). Dopamine neurons drive fear extinction learning by signaling the omission of expected aversive outcomes. Elife 7:e38818. doi: 10.7554/eLife.38818
Scelfo, B., Sacchetti, B., and Strata, P. (2008). Learning-related long-term potentiation of inhibitory synapses in the cerebellar cortex. Proc. Natl. Acad. Sci. U.S.A. 105, 769–774. doi: 10.1073/pnas.0706342105
Supple, W. F., and Leaton, R. N. (1990). Cerebellar vermis: Essential for classically conditioned bradycardia in the rat. Brain Res. 509, 17–23. doi: 10.1016/0006-8993(90)90303-S
Teune, T. M., Van Der Burg, J., Van Der Moer, J., Voogd, J., and Ruigrok, T. J. H. (2000). Topography of cerebellar nuclear projections to the brain stem in the rat. Prog. Brain Res. 124, 141–172. doi: 10.1016/s0079-6123(00)24014-4
Timmann, D., Drepper, J., Frings, M., Maschke, M., Richter, S., Gerwig, M., et al. (2010). The human cerebellum contributes to motor, emotional and cognitive associative learning. A review. Cortex 46, 845–857. doi: 10.1016/j.cortex.2009.06.009
Tovote, P., Esposito, M. S., Botta, P., Chaudun, F., Fadok, J. P., Markovic, M., et al. (2016). Midbrain circuits for defensive behaviour. Nature 534, 206–212. doi: 10.1038/nature17996
Tovote, P., Fadok, J. P., and Lüthi, A. (2015). Neuronal circuits for fear and anxiety. Nat. Rev. Neurosci. 16, 317–331. doi: 10.1038/nrn3945
Utz, A., Thürling, M., Ernst, T. M., Hermann, A., Stark, R., Wolf, O. T., et al. (2015). Cerebellar vermis contributes to the extinction of conditioned fear. Neurosci. Lett. 604, 173–177. doi: 10.1016/j.neulet.2015.07.026
Vaaga, C. E., Brown, S. T., and Raman, I. M. (2020). Cerebellar modulation of synaptic input to freezing-related neurons in the periaqueductal gray. Elife 9:e54302. doi: 10.7554/eLife.54302
Warbrick, T. (2022). Simultaneous EEG-fMRI: What have we learned and what does the future hold?. Sensors 22:2262. doi: 10.3390/s22062262
Watabe-Uchida, M., Zhu, L., Ogawa, S. K., Vamanrao, A., and Uchida, N. (2012). Whole-brain mapping of direct inputs to midbrain dopamine neurons. Neuron 74, 858–873. doi: 10.1016/j.neuron.2012.03.017
Whiteside, J. A., and Snider, R. S. (1953). Relation of cerebellum to upper brain stem. J. Neurophysiol. 16, 397–413. doi: 10.1152/jn.1953.16.4.397
Yágüez, L., Coen, S., Gregory, L. J., Amaro, E., Altman, C., Brammer, M. J., et al. (2005). Brain response to visceral aversive conditioning: A functional magnetic resonance imaging study. Gastroenterology 128, 1819–1829. doi: 10.1053/j.gastro.2005.02.068
Zanchetti, A., and Zoccolini, A. (1954). Autonomic hypothalamic outbursts elicited by cerebellar stimulation. J. Neurophysiol. 17, 475–483. doi: 10.1152/jn.1954.17.5.475
Keywords: cerebellum, fear extinction, fMRI, prediction error, fear behaviour, cerebro-cerebellar circuits, electrophysiology
Citation: Doubliez A, Nio E, Senovilla-Sanz F, Spatharioti V, Apps R, Timmann D and Lawrenson CL (2023) The cerebellum and fear extinction: evidence from rodent and human studies. Front. Syst. Neurosci. 17:1166166. doi: 10.3389/fnsys.2023.1166166
Received: 14 February 2023; Accepted: 27 March 2023;
Published: 21 April 2023.
Edited by:
James Joseph Chrobak, University of Connecticut, United StatesReviewed by:
Marta Miquel, Universitat Jaume I, SpainCopyright © 2023 Doubliez, Nio, Senovilla-Sanz, Spatharioti, Apps, Timmann and Lawrenson. This is an open-access article distributed under the terms of the Creative Commons Attribution License (CC BY). The use, distribution or reproduction in other forums is permitted, provided the original author(s) and the copyright owner(s) are credited and that the original publication in this journal is cited, in accordance with accepted academic practice. No use, distribution or reproduction is permitted which does not comply with these terms.
*Correspondence: Charlotte L. Lawrenson, cHljbGxAYnJpc3RvbC5hYy51aw==
†These authors have contributed equally to this work and share first authorship