- Division of System Neurophysiology, National Institute for Physiological Sciences and Department of Physiological Sciences, Graduate University for Advanced Studies, Myodaiji, Okazaki, Japan
Applying high-frequency stimulation (HFS) to deep brain structure, known as deep brain stimulation (DBS), has now been recognized an effective therapeutic option for a wide range of neurological and psychiatric disorders. DBS targeting the basal ganglia thalamo-cortical loop, especially the internal segment of the globus pallidus (GPi), subthalamic nucleus (STN) and thalamus, has been widely employed as a successful surgical therapy for movement disorders, such as Parkinson’s disease, dystonia and tremor. However, the neurophysiological mechanism underling the action of DBS remains unclear and is still under debate: does DBS inhibit or excite local neuronal elements? In this review, we will examine this question and propose the alternative interpretation: DBS dissociates inputs and outputs, resulting in disruption of abnormal signal transmission.
Introduction
Applying high-frequency electrical stimulation (HFS) to a specific target in subcortical structures, known as deep brain stimulation (DBS), was introduced as a surgical treatment for movement disorders in early 1990s (Benabid et al., 1991, 1994; Siegfried and Lippitz, 1994a,b; Limousin et al., 1995). Since then, DBS has been widely accepted as an effective therapeutic option. DBS targeting the ventral thalamus dramatically alleviates essential and resting tremor (Benabid et al., 1991, 1996; Siegfried and Lippitz, 1994b; Koller et al., 1997; Rehncrona et al., 2003). DBS targeting the subthalamic nucleus (STN) and the internal segment of the globus pallidus (GPi) has been largely used for treatment of Parkinson’s disease, and GPi-DBS has marked effects on improvement of dystonic symptoms (Limousin et al., 1995; Deep-Brain Stimulation for Parkinson’s Disease Study Group, 2001; Coubes et al., 2004; Wichmann and Delong, 2006; Kringelbach et al., 2007; Ostrem and Starr, 2008; Vitek, 2008; Vidailhet et al., 2013). However, the exact mechanism of the effectiveness remains to be elucidated.
Since DBS gives rise to similar effects to those of lesions, it was originally considered to inhibit local neuronal elements. In fact, neuronal firings of neighboring neurons were inhibited by STN- or GPi-DBS (Boraud et al., 1996; Dostrovsky et al., 2000; Wu et al., 2001; Filali et al., 2004; Lafreniere-Roula et al., 2010). On the other hand, recent studies have emphasized activation of neuronal elements. Actually, STN-DBS increased activity of GPi neurons through the excitatory STN-GPi projections (Hashimoto et al., 2003; Galati et al., 2006; Reese et al., 2011), and GPi-DBS reduced activity of thalamic neurons through the inhibitory GPi-thalamic projections (Anderson et al., 2003; Pralong et al., 2003; Montgomery, 2006). In addition, recent studies reported multi-phasic responses consisting of excitation and inhibition in GPi neurons during GPi-DBS (Bar-Gad et al., 2004; Erez et al., 2009; McCairn and Turner, 2009; Leblois et al., 2010). In this article, we critically review recent studies, and discuss the possible mechanism of effectiveness of DBS.
Deep Brain Stimulation (DBS) Inhibits Local Neuronal Elements
Both DBS and lesion were found to produce similar benefits on alleviation of symptoms. For example, STN-DBS has similar effects on Parkinsonian motor signs (Benazzouz et al., 1993; Benabid et al., 1994; Limousin et al., 1995) to the STN-lesion (Bergman et al., 1990; Aziz et al., 1991; Levy et al., 2001) and blockade of synaptic transmission from the STN to the GPi (Graham et al., 1990; Brotchie et al., 1991). Thus, DBS was originally assumed to inhibit local neuronal elements. Actually, the most common effect of STN- or GPi-HFS on neighboring neurons was reduction of the firing rates.
Distinct suppression of neuronal activity was recorded during STN-DBS around the stimulating sites in Parkinsonian patients during stereotactic surgery (Filali et al., 2004; Welter et al., 2004). Similar results were also obtained in animal models, such as Parkinsonian monkeys (Meissner et al., 2005; Moran et al., 2011) and rats (Tai et al., 2003; Shi et al., 2006). Stimulus artifacts hinder detection of spikes during 2–3 ms after stimulus pulses and some spikes may be obscured when neuronal activities are recorded nearby the stimulating electrodes. Recent studies enabled detection of spikes just after stimulus pulses by removal of stimulus artifacts using the template subtraction method (Wichmann, 2000; Hashimoto et al., 2002) and confirmed that STN-DBS decreased firing of neighboring neurons (Meissner et al., 2005; Moran et al., 2011). Although STN-HFS much decreased neuronal firing around the stimulation site, complete cessation of STN firing was observed in a limited number of neurons. STN-HFS at 140 Hz reduced mean firing rate of STN neurons by 77% in Parkinsonian patients, and among them, 71% of STN neurons exhibited residual neuronal activity, while only 29% of STN neurons exhibited total inhibition (Welter et al., 2004). Similar results were also observed in Parkinsonian monkeys (Meissner et al., 2005), and Parkinsonian and normal rats (Tai et al., 2003). Decreased abnormal oscillatory activity in the STN was also observed during STN-DBS in Parkinsonian monkeys (Meissner et al., 2005). Inhibitory effects sometimes outlasted the stimulus period (Tai et al., 2003; Filali et al., 2004; Welter et al., 2004).
Inhibitory effects of GPi-DBS on firing of the neighboring neurons were also reported (Boraud et al., 1996; Dostrovsky et al., 2000; Wu et al., 2001; McCairn and Turner, 2009). Complete inhibition of local neuronal firing was more commonly induced by GPi-DBS than by STN-DBS (Figure 1A). GPi-HFS at 100 Hz induced complete inhibition of 76% of neighboring neurons in normal monkeys (Chiken and Nambu, 2013), and the inhibition outlasted the stimulus period, sometimes over 100 ms after the end of stimulation. Similar post-train inhibition was also observed in Parkinsonian patients (Lafreniere-Roula et al., 2010).
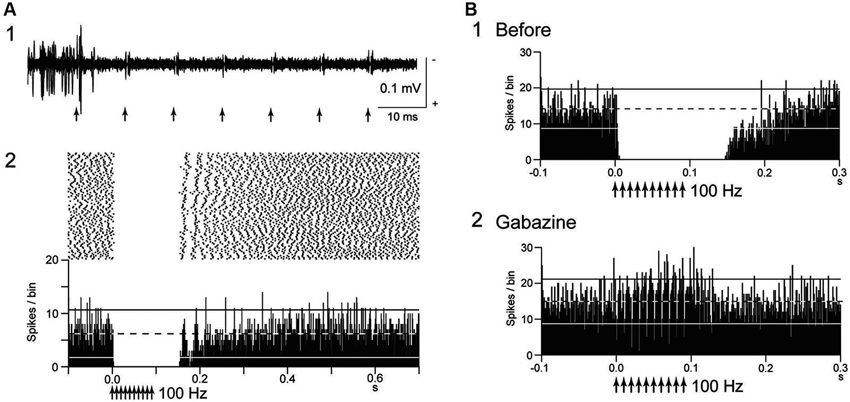
Figure 1. Deep brain stimulation (DBS) inhibits local neuronal firing. (A) Responses of an internal pallidal (GPi) neuron to local GPi repetitive high-frequency stimulation (HFS; 30 μA, 100 Hz, 10 pulses) in a normal monkey. Raw traces of spike discharges after removing the stimulus artifacts by the template subtraction method (1) and raster and peristimulus time histogram (PSTHs; 100 trials; binwidth, 1 ms) (2) are shown. Arrows indicate the timing of local stimulation. Spontaneous discharges of the GPi neuron were completely inhibited by the stimulation. (B) Effect of local injection of gabazine (GABAA receptor antagonist) in the vicinity of the recorded GPi neuron on inhibition of spontaneous activity induced by GPi-HFS. The inhibition was abolished after gabazine injection. Modified from Chiken and Nambu (2013).
To the contrary, multiphasic responses consisting of the excitation and inhibition during GPi-HFS were recently observed in GPi neurons of Parkinsonian monkeys (Bar-Gad et al., 2004; Erez et al., 2009; McCairn and Turner, 2009) and dystonic hamsters (Leblois et al., 2010). The discrepant results may be due to differences in stimulus parameters used in these experiments: larger axons are easily activated by electrical stimulation than smaller ones (Ranck, 1975), and continuous repetitive stimulation might cause failure of postsynaptic events due to receptor desensitization and/or transmitter depletion (Wang and Kaczmarek, 1998; Zucker and Regehr, 2002). Such multiphasic responses may normalize abnormal firings, such as bursting and oscillatory activity in Parkinson’s disease and dystonia as described below.
Mechanism of Inhibition
Several possible mechanisms account for the inhibitory responses have been proposed, including depolarization-block and inactivation of voltage-gated currents (Beurrier et al., 2001; Shin et al., 2007). However, these are less probable, because both single-pulse and low-frequency stimulation in the GPi evoked intense short latency inhibition in neighboring neurons (Dostrovsky et al., 2000; Dostrovsky and Lozano, 2002; Chiken and Nambu, 2013). Another possible mechanism is that the inhibition is caused by activation of GABAergic afferents in the stimulated nucleus (Boraud et al., 1996; Dostrovsky et al., 2000; Dostrovsky and Lozano, 2002; Meissner et al., 2005; Johnson et al., 2008; Liu et al., 2008; Deniau et al., 2010). A recent study confirmed that inhibitory responses induced by GPi-HFS were mediated by GABAA and GABAB receptors (Chiken and Nambu, 2013; Figure 1B). GABAergic inhibition is strong and inhibits even directly evoked spikes by GPi stimulation, which is characterized constant- and short latency (Figures 2A, B; Chiken and Nambu, 2013).
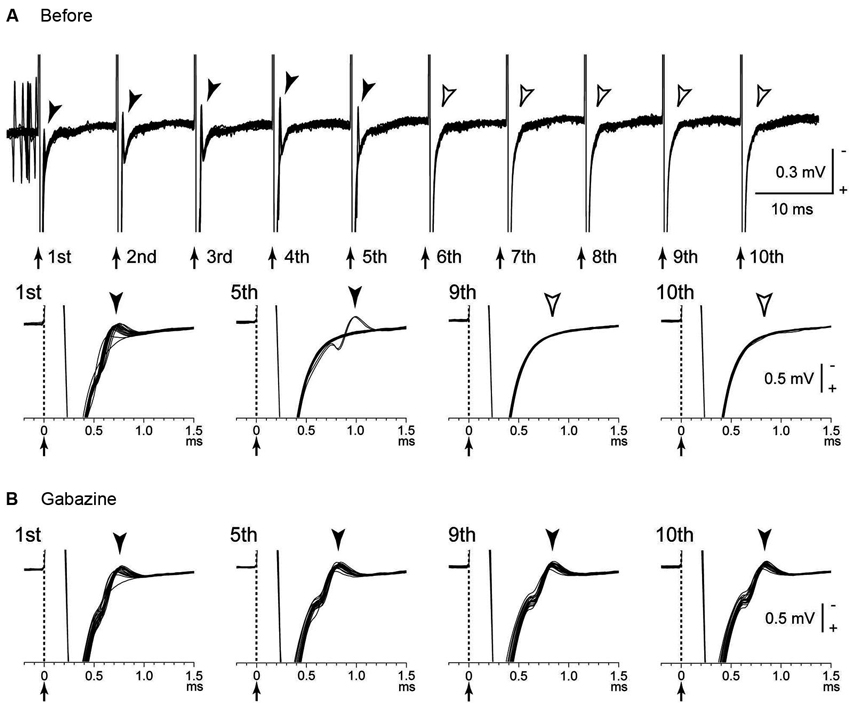
Figure 2. Directly evoked spikes of GPi neurons were inhibited during GPi-HFS. (A) Raw traces showing directly evoked spikes of a GPi neuron by stimulus pulses during GPi-HFS (40 μA, 100 Hz, 10 pulses) in a normal monkey. Traces with long (top) and short (bottom) time scales are shown. Arrows with dotted lines indicate the timing of local stimulation (time 0 in the bottom traces). Filled arrowheads indicate directly evoked spikes. GPi-HFS failed to evoke spikes (open arrowheads; from 6th to 10th stimuli). (B) Effects of local gabazine injection on the inhibition of direct evoked GPi responses. Gabazine injection decreased failure rate, and each stimulus successfully evoked spikes (5th, 9th, and 10th stimuli). Modified from Chiken and Nambu (2013).
The GPi receives excitatory glutamatergic inputs from the STN as well as inhibitory GABAergic inputs from the striatum and GPe (Smith et al., 1994; Shink and Smith, 1995). Afferent axon terminals from the STN are also activated by the stimulation, but the glutamatergic excitation is probably overwhelmed because of predominance of GABAergic inputs in the GPi (Shink and Smith, 1995). On the other hand, many GPe neurons exhibited complex responses composed of both excitation and inhibition during GPe-HFS (Chiken and Nambu, 2013). The density of GPe terminals on GPi neurons is higher than those on GPe neurons (Shink and Smith, 1995), and the balance between GABAergic and glutamatergic inputs may explain the different effects between GPe-HFS and GPi-HFS. Similarly, STN-HFS stimulated both glutamatergic and GABAergic afferents and generated both excitatory and inhibitory post-synaptic potentials (EPSPs and IPSPs) in the STN neurons (Lee et al., 2004). Thus, HFS activates afferent axons in the stimulated nucleus, and the effects vary depending on the composition of the inhibitory and excitatory axon terminals.
Deep Brain Stimulation (DBS) Excites Local Neuronal Elements
It is rational that local stimulation excites local neuronal elements. Actually, directly evoked spikes, which are characterized by short- and constant latency, are induced in GPi neurons by GPi-HFS (Johnson and McIntyre, 2008; McCairn and Turner, 2009). Such excitation may propagate through efferent projections. Thalamic activity was reduced during GPi-HFS through inhibitory GPi-thalamic projections in Parkinsonian monkeys (Anderson et al., 2003) and dystonia patients (Pralong et al., 2003; Montgomery, 2006). GPi activity was increased during STN-DBS through excitatory STN-GPi projections (Hashimoto et al., 2003; Galati et al., 2006; Reese et al., 2011). STN-DBS increased both glutamate and GABA levels in the substantia nigra pars reticulata (SNr) of normal rats in microdialysis studies (Windels et al., 2000; see also Windels et al., 2005). An intraoperative microdialysis study revealed that STN-DBS produced significant increase in extracellular concentration of cyclic guanosine monophosphate (cGMP) in the GPi (Stefani et al., 2005). Functional magnetic resonance imaging (MRI) and positron emission tomography (PET) studies in humans indicated that efferent outputs from the stimulated nucleus are excited during DBS (Jech et al., 2001; Hershey et al., 2003; Boertien et al., 2011). Changes of the firing rates and patterns of target nuclei may normalize abnormal firings, such as bursting and oscillatory activity, which are observes in the cortico-basal ganglia loop of Parkinson’s disease and dystonia (Anderson et al., 2003; Hashimoto et al., 2003; Hammond et al., 2007; Johnson et al., 2008; Vitek, 2008; Deniau et al., 2010).
According to the modeling study (McIntyre et al., 2004), subthreshold HFS suppressed intrinsic firings in the cell bodies, while suprathreshold HFS generated efferent outputs at the stimulus frequency in the axon without representative activation of the cell bodies. Thus, although stimulation may fail to activate cell bodies of GPi neurons due to strong GABAergic inhibition, it can still excite the efferent axons and provide inhibitory inputs to the thalamus at the stimulus frequency.
DBS also antidromically excites afferent axons. Actually, antidromic activation of GPi neurons induced by STN-DBS was observed in Parkinsonian monkeys (Moran et al., 2011), and antidromic activation of thatamic (Vop) neurons induced by GPi-DBS was observed in Parkinsonian patients (Montgomery, 2006). Low intensity STN-HFS induced GABAergic inhibition in the SNr through antidromic activation of GPe neurons projecting to both the STN and SNr (Maurice et al., 2003; see also Moran et al., 2011), whereas higher intensity stimulation induced glutamatergic excitation in the SNr through activation of STN-SNr projections. STN-HFS also activated motor cortical neurons antidromically and suppressed abnormal low frequency synchronization including beta band oscillation in Parkinsonian rats (Li et al., 2007, 2012; Degos et al., 2013). Recent development of optogenetics has enabled selective stimulation of afferent inputs or efferent outputs, and contribute to analyzing the mechanism of effectiveness of DBS. A recent study has shown that selective stimulation of cortico-STN afferent axons can robustly ameliorate symptoms in Parkinsonian rats without activation of STN efferent axons (Gradinaru et al., 2009), suggesting that therapeutic effects of STN-DBS may be exclusively accounted for activation of cortico-STN afferent axons.
It is also probable that STN-DBS induces dopamine release through STN-SNc projections. STN-DBS induced dopamine release by activation of nigrostriatal dopaminergic neurons in rats (Meissner et al., 2003) and pigs (Shon et al., 2010), however it did not increase dopamine level of the striatum in human patients (Abosch et al., 2003; Hilker et al., 2003). DBS may also affect neurons whose axons pass nearby the stimulating site. A model-based study showed that clinically effective STN-DBS also activated the lenticular fasciculus, which is composed of GPi-thalamic fibers, in addition to STN neurons temselves (Miocinovic et al., 2006). Actually, STN-DBS induced direct excitation of GPi neurons through activation of the lenticular fasciculus (Moran et al., 2011).
Participation of non-neuronal glial tissues should also be considered as one of possible mechanisms of DBS effectiveness. DBS induced glutamate and adenosine triphosphate (ATP) release from astrocytes (Fellin et al., 2006; Tawfik et al., 2010). A recent study revealed that HFS applied to the thalamus induced abrupt increase in extracellular ATP and adenosine (Bekar et al., 2008). Adenosine activation of A1 receptors depressed excitatory transmission in the thalamus, and alleviated tremor in a mouse model. Thus, it is possible that ATP and glutamate are released from astrocytes triggered by DBS and modulate neuronal activity in the stimulated nucleus (Vedam-Mai et al., 2012; Jantz and Watanabe, 2013).
Deep Brain Stimulation (DBS) Disrupts Neuronal Transmission
The striatum and STN are input stations of the basal ganglia and receive inputs from a wide area of the cerebral cortex (Mink, 1996; Nambu et al., 2002). The information is processed through the hyperdirect, direct, and indirect pathways and reaches the GPi/SNr, the output station of the basal ganglia (Figure 3A). During voluntary movements, neuronal signals originating in the cortex are considered to be transmitted through these pathways, reach the GPi/SNr and control movements (Mink, 1996; Nambu et al., 2002). Signal transmission through the direct pathway reduces GPi activity and facilitates movements by disinhibiting the thalamus, whereas the hyperdirect and indirect pathways increase GPi activity and suppress movements (Nambu et al., 2002; Nambu, 2007; Kravitz et al., 2010; Sano et al., 2013).
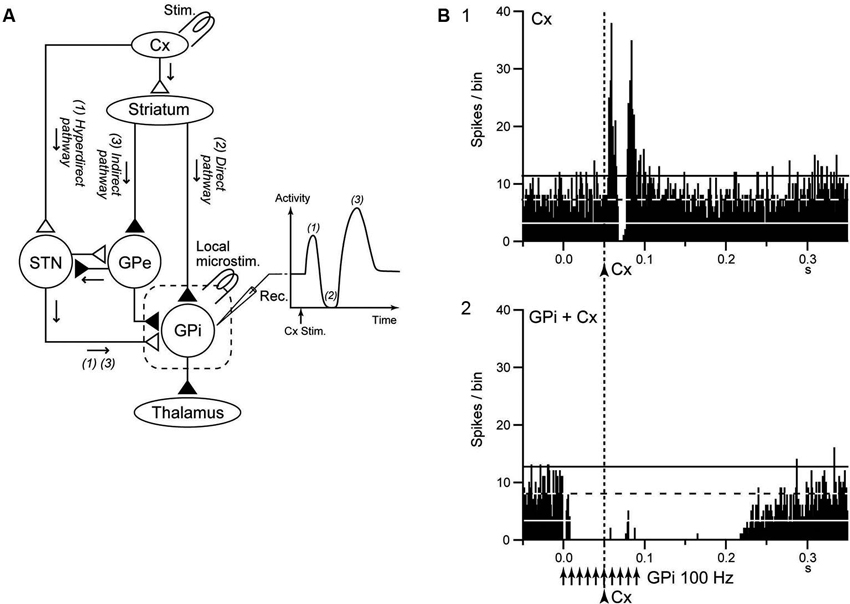
Figure 3. GPi-DBS disrupts information flow through the GPi. (A) Schematic diagram showing the cortico-basal ganglia pathway and stimulating (Stim.) and recording (Rec.) sites in the electrophysiological experiments (left), along with a typical response pattern (right) in the (GPi) to cortical stimulation (Cx Stim.) with early excitation, inhibition, and late excitation, which are mediated by the (1) cortico-subthalamo (STN)-GPi hyperdirect, (2) striato-GPi direct, and (3) striato-external pallido (GPe)-STN-GPi indirect pathways, respectively. (B) Effects of local GPi-HFS on cortically evoked responses of a GPi neuron in a normal monkey. PSTH (100 trials) in response to the single pulse stimulation (arrowhead with dotted line) of the primary motor cortex (Cx) (1) and PSTH in response to Cx stimulation (arrowhead with dotted line) during GPi-HFS (arrows) (2) are shown. Cortical stimulation was applied 50 ms after the initiation of GPi-HFS. The cortically evoked responses were entirely inhibited during GPi-HFS. Modified from Chiken and Nambu (2013).
Chiken and Nambu (2013) recently examined responses of GPi neurons evoked by motor cortical stimulation during GPi-HFS in normal monkeys. In that study, both cortically evoked responses and spontaneous discharges were completely inhibited during GPi-HFS by strong GABAergic inhibition (Figure 3B), suggesting that GPi-HFS blocks information flow through the GPi. Since abnormal cortically evoked responses (Chiken et al., 2008; Kita and Kita, 2011; Nishibayashi et al., 2011) and abnormal bursts and oscillatory activity (Wichmann et al., 1994; Bergman et al., 1998; Starr et al., 2005; Brown, 2007; Chiken et al., 2008; Nishibayashi et al., 2011; Tachibana et al., 2011) were observed in GPi neurons in Parkinson’s disease and dystonia, signal transmission of such abnormal activities to the thalamus and motor cortex would be responsible for motor symptoms. Thus, disruption of the abnormal information flow could suppress expression of motor symptoms. This mechanism may explain the paradox that GPi-DBS produces similar therapeutic effects to lesions of the GPi: both GPi-DBS and GPi-lesion interrupt abnormal information flow through the GPi.
STN-DBS may also interrupt neurotransmission of abnormal signals. Maurice et al. (2003) examined the effects of STN-DBS on cortically evoked responses of SNr neurons in normal rats. Cortically evoked early and late excitation was totally abolished during high intensity STN-HFS, and much reduced during low intensity STN-DBS, while cortically evoked inhibition was preserved (Figure 4A), suggesting that information flow through the trans-STN pathway was blocked by STN-DBS without interrupting other pathways. The response patterns of SNr neurons during STN-DBS are similar to those of GPi neurons during STN blockade by muscimol in normal monkeys (Nambu et al., 2000; Figure 4B). Thus it is rational that STN-DBS has similar effect to lesion or silencing of the STN. In Parkinson’s disease, due to the loss of dopaminergic modulation, the information flow through the striato-GPi direct pathway is weakened, whereas the information flow through the striato-GPe indirect pathway is facilitated. Both STN-DBS and STN lesioning may alter the balance of inhibitory inputs through the direct pathway and excitatory inputs through the hyperdirect and indirect pathways to the GPi by disrupting information flow through the STN, and effectively alleviate bradykinesia seen in Parkinson’s disease. Similar idea, a functional disconnection of the stimulated elements, has also proposed by other groups (Anderson et al., 2006; Deniau et al., 2010; Moran et al., 2011).
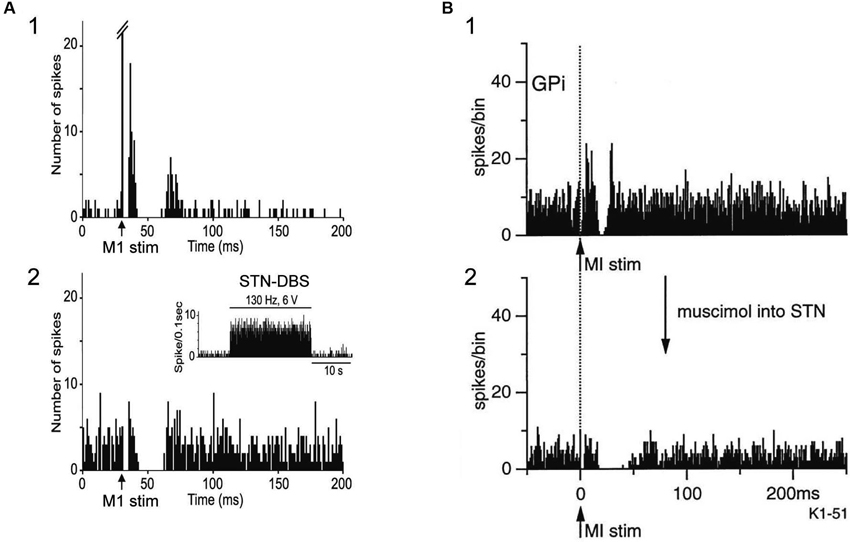
Figure 4. Both STN-DBS and STN blocking disrupt information flow through the STN. (A) Effects of local STN-DBS on cortically evoked responses of a substantia nigra pars reticulata (SNr) neuron in a normal rat. PSTH (50 trials) in response to the single pulse stimulation of the Cx (arrow) (1) and PSTH in response to Cx stimulation during STN-DBS (2) are shown. The cortically evoked early and late excitation was abolished during STN-DBS, while cortically evoked inhibition was preserved. Modified from Maurice et al. (2003). (B) Effects of STN blocking on cortically evoked responses of a GPi neuron in a normal monkey. PSTH (100 trials) in response to the single pulse stimulation of the Cx (arrow with dotted line) (1) and PSTH in response to Cx stimulation after blocking STN activity by muscimol (GABAA receptor agonist) injection into the STN (2) are shown. The cortically evoked early and late excitation was abolished after injection of muscimol into the STN, while cortically evoked inhibition was preserved. Modified from Nambu et al. (2000). Note that the pattern of cortically evoked responses of a SNr neuron during STN-DBS is similar to that of a GPi neuron after STN blocking.
Conclusion
DBS has variety of effects on neurons in the stimulated nucleus of the cortico-basal ganglia loop, though transmitter release, orthodromic activation of efferent axons, antidromic activation of afferent axons, and direct stimulation of passing axons nearby the stimulating electrode. The effects vary depending on the neural composition of the stimulated nucleus, and the effects extend much wider than originally expected. However, a common mechanism would underlie the effectiveness of DBS: DBS dissociates inputs and outputs in the stimulated nucleus and disrupts abnormal information flow through the cortico-basal ganglia loop (Figure 5). The mechanism may explain the paradox that DBS produces similar therapeutic effects to lesions or silencing of the nucleus.
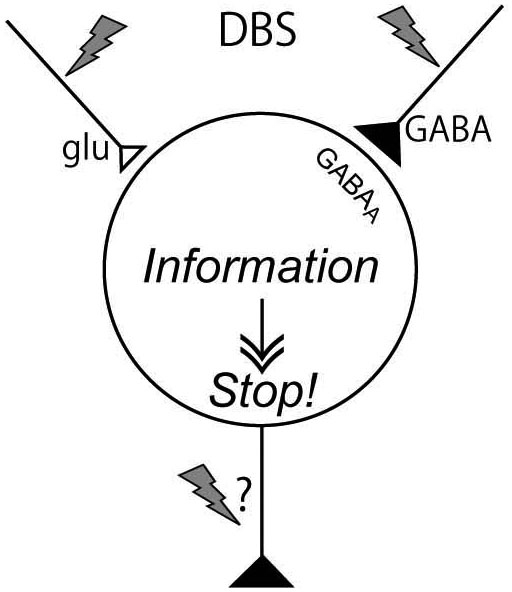
Figure 5. Mechanism underling effectiveness of deep brain stimulation (DBS). DBS activates axon terminals in the stimulated nucleus and induces release of large amount of neurotransmitters, such as GABA and glutamate, and dissociates inputs and outputs in the stimulated nucleus, resulting in disruption of abnormal information flow through the cortico-basal ganglia loop.
Conflict of Interest Statement
The authors declare that the research was conducted in the absence of any commercial or financial relationships that could be construed as a potential conflict of interest.
Acknowledgments
This work was supported by a Grant-in-Aid for Scientific Research (A) 21240039, the Core Research for Evolutional Science and Technology, Strategic Japanese-German Cooperative Programme, and Brain Machine Interface Development under the Strategic Research Program for Brain Sciences to Atsushi Nambu, and a Grant-in-Aid for Scientific Research (C) 25430021 to Satomi Chiken. We thank Kana Miyamoto, Shigeki Sato, Hitomi Isogai, and Keiko Matsuzawa for technical assistance.
References
Abosch, A., Kapur, S., Lang, A. E., Hussey, D., Sime, E., Miyasaki, J., et al. (2003). Stimulation of the subthalamic nucleus in Parkinson’s disease does not produce striatal dopamine release. Neurosurgery 53, 1095–1105. doi: 10.1227/01.neu.0000088662.69419.1b
Anderson, T. R., Hu, B., Iremonger, K., and Kiss, Z. H. (2006). Selective attenuation of afferent synaptic transmission as a mechanism of thalamic deep brain stimulation-induced tremor arrest. J. Neurosci. 26, 841–850. doi: 10.1523/jneurosci.3523-05.2006
Anderson, M. E., Postupna, N., and Ruffo, M. (2003). Effects of high-frequency stimulation in the internal globus pallidus on the activity of thalamic neurons in the awake monkey. J. Neurophysiol. 89, 1150–1160. doi: 10.1152/jn.00475.2002
Aziz, T. Z., Peggs, D., Sambrook, M. A., and Crossman, A. R. (1991). Lesion of the subthalamic nucleus for the alleviation of 1-methyl-4-phenyl-1,2,3,6-tetrahydropyridine (MPTP)-induced parkinsonism in the primate. Mov. Disord. 6, 288–292. doi: 10.1002/mds.870060404
Bar-Gad, I., Elias, S., Vaadia, E., and Bergman, H. (2004). Complex locking rather than complete cessation of neuronal activity in the globus pallidus of a 1-methyl-4-phenyl-1,2,3,6-tetrahydropyridine-treated primate in response to pallidal microstimulation. J. Neurosci. 24, 7410–7419. doi: 10.1523/jneurosci.1691-04.2004
Bekar, L., Libionka, W., Tian, G. F., Xu, Q., Torres, A., Wang, X., et al. (2008). Adenosine is crucial for deep brain stimulation-mediated attenuation of tremor. Nat. Med. 14, 75–80. doi: 10.1038/nm1693
Benabid, A. L., Pollak, P., Gao, D., Hoffmann, D., Limousin, P., Gay, E., et al. (1996). Chronic electrical stimulation of the ventralis intermedius nucleus of the thalamus as a treatment of movement disorders. J. Neurosurg. 84, 203–214. doi: 10.3171/jns.1996.84.2.0203
Benabid, A. L., Pollak, P., Gervason, C., Hoffmann, D., Gao, D. M., Hommel, M., et al. (1991). Long-term suppression of tremor by chronic stimulation of the ventral intermediate thalamic nucleus. Lancet 337, 403–406. doi: 10.1016/0140-6736(91)91175-t
Benabid, A. L., Pollak, P., Gross, C., Hoffmann, D., Benazzouz, A., Gao, D. M., et al. (1994). Acute and long-term effects of subthalamic nucleus stimulation in Parkinson’s disease. Stereotact. Funct. Neurosurg. 62, 76–84. doi: 10.1159/000098600
Benazzouz, A., Gross, C., Féger, J., Boraud, T., and Bioulac, B. (1993). Reversal of rigidity and improvement in motor performance by subthalamic high-frequency stimulation in MPTP-treated monkeys. Eur. J. Neurosci. 5, 382–389. doi: 10.1111/j.1460-9568.1993.tb00505.x
Bergman, H., Feingold, A., Nini, A., Raz, A., Slovin, H., Abeles, M., et al. (1998). Physiological aspects of information processing in the basal ganglia of normal and parkinsonian primates. Trends Neurosci. 21, 32–38. doi: 10.1016/S0166-2236(97)01151-X
Bergman, H., Wichmann, T., and DeLong, M. R. (1990). Reversal of experimental parkinsonism by lesions of the subthalamic nucleus. Science 249, 1436–1438. doi: 10.1126/science.2402638
Beurrier, C., Bioulac, B., Audin, J., and Hammond, C. (2001). High-frequency stimulation produces a transient blockade of voltage-gated currents in subthalamic neurons. J. Neurophysiol. 85, 1351–1356.
Boertien, T., Zrinzo, L., Kahan, J., Jahanshahi, M., Hariz, M., Mancini, L., et al. (2011). Functional imaging of subthalamic nucleus deep brain stimulation in Parkinson’s disease. Mov. Disord. 26, 1835–1843. doi: 10.1002/mds.23788
Boraud, T., Bezard, E., Bioulac, B., and Gross, C. (1996). High frequency stimulation of the internal globus pallidus (GPi) simultaneously improves parkinsonian symptoms and reduces the firing frequency of GPi neurons in the MPTP-treated monkey. Neurosci. Lett. 215, 17–20. doi: 10.1016/s0304-3940(96)12943-8
Brotchie, J. M., Mitchell, I. J., Sambrook, M. A., and Crossman, A. R. (1991). Alleviation of parkinsonism by antagonism of excitatory amino acid transmission in the medial segment of the globus pallidus in rat and primate. Mov. Disord. 6, 133–138. doi: 10.1002/mds.870060208
Brown, P. (2007). Abnormal oscillatory synchronisation in the motor system leads to impaired movement. Curr. Opin. Neurobiol. 17, 656–664. doi: 10.1016/j.conb.2007.12.001
Chiken, S., and Nambu, A. (2013). High-frequency pallidal stimulation disrupts information flow through the pallidum by GABAergic inhibition. J. Neurosci. 33, 2268–2280. doi: 10.1523/jneurosci.4144-11.2013
Chiken, S., Shashidharan, P., and Nambu, A. (2008). Cortically-evoked long-lasting inhibition of pallidal neurons in a transgenic mouse model of dystonia. J. Neurosci. 28, 13967–13977. doi: 10.1523/jneurosci.3834-08.2008
Coubes, P., Cif, L., El Fertit, H., Hemm, S., Vayssiere, N., Serrat, S., et al. (2004). Electrical stimulation of the globus pallidus internus in patients with primary generalized dystonia: long-term results. J. Neurosurg. 101, 189–194. doi: 10.3171/jns.2004.101.2.0189
Deep-Brain Stimulation for Parkinson’s Disease Study Group. (2001). Deep-brain stimulation of the subthalamic nucleus or the pars interna of the globus pallidus in Parkinson’s disease. N. Engl. J. Med. 345, 956–963. doi: 10.1056/nejmoa000827
Degos, B., Deniau, J. M., Chavez, M., and Maurice, N. (2013). Subthalamic nucleus high-frequency stimulation restores altered electrophysiological properties of cortical neurons in parkinsonian rat. PLoS One 8:e83608. doi: 10.1371/journal.pone.0083608
Deniau, J. M., Degos, B., Bosch, C., and Maurice, N. (2010). Deep brain stimulation mechanisms: beyond the concept of local functional inhibition. Eur. J. Neurosci. 32, 1080–1091. doi: 10.1111/j.1460-9568.2010.07413.x
Dostrovsky, J. O., Levy, R., Wu, J. P., Hutchison, W. D., Tasker, R. R., and Lozano, A. M. (2000). Microstimulation-induced inhibition of neuronal firing in human globus pallidus. J. Neurophysiol. 84, 570–574.
Dostrovsky, J. O., and Lozano, A. M. (2002). Mechanisms of deep brain stimulation. Mov. Disord. 17, S63–S68. doi: 10.1002/mds.10143
Erez, Y., Czitron, H., McCairn, K., Belelovsky, K., and Bar-Gad, I. (2009). Short-term depression of synaptic transmission during stimulation in the globus pallidus of 1-methyl-4-phenyl-1,2,3,6-tetrahydropyridine-treated primates. J. Neurosci. 29, 7797–7802. doi: 10.1523/jneurosci.0401-09.2009
Fellin, T., Pascual, O., and Haydon, P. G. (2006). Astrocytes coordinate synaptic networks: balanced excitation and inhibition. Physiology (Bethesda) 21, 208–215. doi: 10.1152/physiol.00161.2005
Filali, M., Hutchison, W. D., Palter, V. N., Lozano, A. M., and Dostrovsky, J. O. (2004). Stimulation-induced inhibition of neuronal firing in human subthalamic nucleus. Exp. Brain Res. 156, 274–281. doi: 10.1007/s00221-003-1784-y
Galati, S., Mazzone, P., Fedele, E., Pisani, A., Peppe, A., Pierantozzi, M., et al. (2006). Biochemical and electrophysiological changes of substantia nigra pars reticulata driven by subthalamic stimulation in patients with Parkinson’s disease. Eur. J. Neurosci. 23, 2923–2928. doi: 10.1111/j.1460-9568.2006.04816.x
Gradinaru, V., Mogri, M., Thompson, K. R., Henderson, J. M., and Deisseroth, K. (2009). Optical deconstruction of parkinsonian neural circuitry. Science 324, 354–359. doi: 10.1126/science.1167093
Graham, W. C., Robertson, R. G., Sambrook, M. A., and Crossman, A. R. (1990). Injection of excitatory amino acid antagonists into the medial pallidal segment of a 1-methyl-4-phenyl-1,2,3,6-tetrahydropyridine (MPTP) treated primate reverses motor symptoms of parkinsonism. Life Sci. 47, PL91–PL97. doi: 10.1016/0024-3205(90)90376-3
Hammond, C., Bergman, H., and Brown, P. (2007). Pathological synchronization in Parkinson’s disease: networks, models and treatments. Trends Neurosci. 30, 357–364. doi: 10.1016/j.tins.2007.05.004
Hashimoto, T., Elder, C. M., Okun, M. S., Patrick, S. K., and Vitek, J. L. (2003). Stimulation of the subthalamic nucleus changes the firing pattern of pallidal neurons. J. Neurosci. 23, 1916–1923.
Hashimoto, T., Elder, C. M., and Vitek, J. L. (2002). A template subtraction method for stimulus artifact removal in high-frequency deep brain stimulation. J. Neurosci. Methods 113, 181–186. doi: 10.1016/s0165-0270(01)00491-5
Hershey, T., Revilla, F. J., Wernle, A. R., McGee-Minnich, L., Antenor, J. V., Videen, T. O., et al. (2003). Cortical and subcortical blood flow effects of subthalamic nucleus stimulation in PD. Neurology 61, 816–821. doi: 10.1212/01.wnl.0000083991.81859.73
Hilker, R., Voges, J., Ghaemi, M., Lehrke, R., Rudolf, J., Koulousakis, A., et al. (2003). Deep brain stimulation of the subthalamic nucleus does not increase the striatal dopamine concentration in parkinsonian humans. Mov. Disord. 18, 41–48. doi: 10.1002/mds.10297
Jantz, J. J., and Watanabe, M. (2013). Pallidal deep brain stimulation modulates afferent fibers, efferent fibers and glia. J. Neurosci. 33, 9873–9875. doi: 10.1523/jneurosci.1471-13.2013
Jech, R., Urgosík, D., Tintera, J., Nebuzelský, A., Krásenský, J., Liscák, R., et al. (2001). Functional magnetic resonance imaging during deep brain stimulation: a pilot study in four patients with Parkinson’s disease. Mov. Disord. 16, 1126–1132. doi: 10.1002/mds.1217
Johnson, M. D., and McIntyre, C. C. (2008). Quantifying the neural elements activated and inhibited by globus pallidus deep brain stimulation. J. Neurophysiol. 100, 2549–2563. doi: 10.1152/jn.90372.2008
Johnson, M. D., Miocinovic, S., McIntyre, C. C., and Vitek, J. L. (2008). Mechanisms and targets of deep brain stimulation in movement disorders. Neurotherapeutics 5, 294–308. doi: 10.1016/j.nurt.2008.01.010
Kita, H., and Kita, T. (2011). Cortical stimulation evokes abnormal responses in the dopamine-depleted rat basal ganglia. J. Neurosci. 31, 10311–10322. doi: 10.1523/jneurosci.0915-11.2011
Koller, W., Pahwa, R., Busenbark, K., Hubble, J., Wilkinson, S., Lang, A., et al. (1997). High-frequency unilateral thalamic stimulation in the treatment of essential and parkinsonian tremor. Ann. Neurol. 42, 292–299. doi: 10.1002/ana.410420304
Kravitz, A. V., Freeze, B. S., Parker, P. R. L., Kay, K., Thwin, M. T., Deisseroth, K., et al. (2010). Regulation of parkinsonian motor behaviours by optogenetic control of basal ganglia circuitry. Nature 466, 622–626. doi: 10.1038/nature09159
Kringelbach, M. L., Jenkinson, N., Owen, S. L., and Aziz, T. Z. (2007). Translational principles of deep brain stimulation. Nat. Rev. Neurosci. 8, 623–635. doi: 10.1038/nrn2196
Lafreniere-Roula, M., Kim, E., Hutchison, W. D., Lozano, A. M., Hodaie, M., and Dostrovsky, J. O. (2010). High-frequency microstimulation in human globus pallidus and substantia nigra. Exp. Brain Res. 205, 251–261. doi: 10.1007/s00221-010-2362-8
Leblois, A., Reese, R., Labarre, D., Hamann, M., Richter, A., Boraud, T., et al. (2010). Deep brain stimulation changes basal ganglia output nuclei firing pattern in the dystonic hamster. Neurobiol. Dis. 38, 288–298. doi: 10.1016/j.nbd.2010.01.020
Lee, K. H., Chang, S. Y., Roberts, D. W., and Kim, U. (2004). Neurotransmitter release from high-frequency stimulation of the subthalamic nucleus. J. Neurosurg. 101, 511–517. doi: 10.3171/jns.2004.101.3.0511
Levy, R., Lang, A. E., Dostrovsky, J. O., Pahapill, P., Romas, J., Saint-Cyr, J., et al. (2001). Lidocaine and muscimol microinjections in subthalamic nucleus reverse Parkinsonian symptoms. Brain 124, 2105–2118. doi: 10.1093/brain/124.10.2105
Li, S., Arbuthnott, G. W., Jutras, M. J., Goldberg, J. A., and Jaeger, D. (2007). Resonant antidromic cortical circuit activation as a consequence of high-frequency subthalamic deep-brain stimulation. J. Neurophysiol. 98, 3525–3537. doi: 10.1152/jn.00808.2007
Li, Q., Ke, Y., Chan, D. C., Qian, Z. M., Yung, K. K., Ko, H., et al. (2012). Therapeutic deep brain stimulation in Parkinsonian rats directly influences motor cortex. Neuron 76, 1030–1041. doi: 10.1016/j.neuron.2012.09.032
Limousin, P., Pollak, P., Benazzouz, A., Hoffmann, D., Le Bas, J. F., Broussolle, E., et al. (1995). Effect of parkinsonian signs and symptoms of bilateral subthalamic nucleus stimulation. Lancet 345, 91–95. doi: 10.1016/s0140-6736(95)90062-4
Liu, Y., Postupna, N., Falkenberg, J., and Anderson, M. E. (2008). High frequency deep brain stimulation: what are the therapeutic mechanisms? Neurosci. Biobehav. Rev. 32, 343–351. doi: 10.1016/j.neubiorev.2006.10.007
Maurice, N., Thierry, A. M., Glowinski, J., and Deniau, J. M. (2003). Spontaneous and evoked activity of substantia nigra pars reticulata neurons during high-frequency stimulation of the subthalamic nucleus. J. Neurosci. 23, 9929–9936.
McCairn, K. W., and Turner, R. S. (2009). Deep brain stimulation of the globus pallidus internus in the parkinsonian primate: local entrainment and suppression of low-frequency oscillations. J. Neurophysiol. 101, 1941–1960. doi: 10.1152/jn.91092.2008
McIntyre, C. C., Savasta, M., Walter, B. L., and Vitek, J. L. (2004). How does deep brain stimulation work? Present understanding and future questions. J. Clin. Neurophysiol. 21, 40–50. doi: 10.1097/00004691-200401000-00006
Meissner, W., Harnack, D., Reese, R., Paul, G., Reum, T., Ansorge, M., et al. (2003). High-frequency stimulation of the subthalamic nucleus enhances striatal dopamine release and metabolism in rats. J. Neurochem. 85, 601–609. doi: 10.1046/j.1471-4159.2003.01665.x
Meissner, W., Leblois, A., Hansel, D., Bioulac, B., Gross, C. E., Benazzouz, A., et al. (2005). Subthalamic high frequency stimulation resets subthalamic firing and reduces abnormal oscillations. Brain 128, 2372–2382. doi: 10.1093/brain/awh616
Mink, J. W. (1996). The basal ganglia: focused selection and inhibition of competing motor programs. Prog. Neurobiol. 50, 381–425. doi: 10.1016/s0301-0082(96)00042-1
Miocinovic, S., Parent, M., Butson, C. R., Hahn, P. J., Russo, G. S., Vitek, J. L., et al. (2006). Computational analysis of subthalamic nucleus and lenticular fasciculus activation during therapeutic deep brain stimulation. J. Neurophysiol. 96, 1569–1580. doi: 10.1152/jn.00305.2006
Montgomery, E. B. Jr. (2006). Effects of GPi stimulation on human thalamic neuronal activity. Clin. Neurophysiol. 117, 2691–2702. doi: 10.1016/j.clinph.2006.08.011
Moran, A., Stein, E., Tischler, H., Belelovsky, K., and Bar-Gad, I. (2011). Dynamic stereotypic responses of Basal Ganglia neurons to subthalamic nucleus high-frequency stimulation in the parkinsonian primate. Front. Syst. Neurosci. 5:21. doi: 10.3389/fnsys.2011.00021
Nambu, A. (2007). Globus pallidus internal segment. Prog. Brain. Res. 160, 135–150. doi: 10.1016/s0079-6123(06)60008-3
Nambu, A., Tokuno, H., and Takada, M. (2002). Functional significance of the cortico-subthalamo-pallidal ‘hyperdirect’ pathway. Neurosci. Res. 43, 111–117. doi: 10.1016/s0168-0102(02)00027-5
Nambu, A., Tokuno, H., Hamada, I., Kita, H., Imanishi, M., Akazawa, T., et al. (2000). Excitatory cortical inputs to pallidal neurons via the subthalamic nucleus in the monkey. J. Neurophysiol. 84, 289–300.
Nishibayashi, H., Ogura, M., Kakishita, K., Tanaka, S., Tachibana, Y., Nambu, A., et al. (2011). Cortically evoked responses of human pallidal neurons recorded during stereotactic neurosurgery. Mov. Disord. 26, 469–476. doi: 10.1002/mds.23502
Ostrem, J. L., and Starr, P. A. (2008). Treatment of dystonia with deep brain stimulation. Neurotherapeutics 5, 320–330. doi: 10.1016/j.nurt.2008.01.002
Pralong, E., Debatisse, D., Maeder, M., Vingerhoets, F., Ghika, J., and Villemure, J. G. (2003). Effect of deep brain stimulation of GPI on neuronal activity of the thalamic nucleus ventralis oralis in a dystonic patient. Neurophysiol. Clin. 33, 169–173. doi: 10.1016/j.neucli.2003.07.001
Ranck, J. B. Jr. (1975). Which elements are excited in electrical stimulation of mammalian central nervous system: a review. Brain Res. 98, 417–440. doi: 10.1016/0006-8993(75)90364-9
Reese, R., Leblois, A., Steigerwald, F., Potter-Nerger, M., Herzog, J., Mehdorn, H. M., et al. (2011). Subthalamic deep brain stimulation increases pallidal firing rate and regularity. Exp. Neurol. 229, 517–521. doi: 10.1016/j.expneurol.2011.01.020
Rehncrona, S., Johnels, B., Widner, H., Törnqvist, A. L., Hariz, M., and Sydow, O. (2003). Long-term efficacy of thalamic deep brain stimulation for tremor: double-blind assessments. Mov. Disord. 18, 163–170. doi: 10.1002/mds.10309
Sano, H., Chiken, S., Hikida, T., Kobayashi, K., and Nambu, A. (2013). Signals through the striatopallidal indirect pathway stop movements by phasic excitation in the substantia nigra. J. Neurosci. 33, 7583–7594. doi: 10.1523/jneurosci.4932-12.2013
Shi, L. H., Luo, F., Woodward, D. J., and Chang, J. Y. (2006). Basal ganglia neural responses during behaviorally effective deep brain stimulation of the subthalamic nucleus in rats performing a treadmill locomotion test. Synapse 59, 445–457. doi: 10.1002/syn.20261
Shin, D. S., Samoilova, M., Cotic, M., Zhang, L., Brotchie, J. M., and Carlen, P. L. (2007). High frequency stimulation or elevated K+ depresses neuronal activity in the rat entopeduncular nucleus. Neuroscience 149, 68–86. doi: 10.1016/j.neuroscience.2007.06.055
Shink, E., and Smith, Y. (1995). Differential synaptic innervation of neurons in the internal and external segments of the globus pallidus by the GABA- and glutamate-containing terminals in the squirrel monkey. J. Comp. Neurol. 358, 119–141. doi: 10.1002/cne.903580108
Shon, Y. M., Lee, K. H., Goerss, S. J., Kim, I. Y., Kimble, C., Van Gompel, J. J., et al. (2010). High frequency stimulation of the subthalamic nucleus evokes striatal dopamine release in a large animal model of human DBS neurosurgery. Neurosci. Lett. 475, 136–140. doi: 10.1016/j.neulet.2010.03.060
Siegfried, J., and Lippitz, B. (1994a). Bilateral chronic electrostimulation of ventroposterolateral pallidum: a new therapeutic approach for alleviating all parkinsonian symptoms. Neurosurgery 35, 1126–1130. doi: 10.1227/00006123-199412000-00016
Siegfried, J., and Lippitz, B. (1994b). Chronic electrical stimulation of the VL-VPL complex and of the pallidum in the treatment of movement disorders: personal experience since 1982. Stereotact. Funct. Neurosurg. 62, 71–75. doi: 10.1159/000098599
Smith, Y., Wichmann, T., and DeLong, M. R. (1994). Synaptic innervation of neurones in the internal pallidal segment by the subthalamic nucleus and the external pallidum in monkeys. J. Comp. Neurol. 343, 297–318. doi: 10.1002/cne.903430209
Starr, P. A., Rau, G. M., Davis, V., Marks, W. J. Jr., Ostrem, J. L., Simmons, D., et al. (2005). Spontaneous pallidal neuronal activity in human dystonia: comparison with Parkinson’s disease and normal macaque. J. Neurophysiol. 93, 3165–3176. doi: 10.1152/jn.00971.2004
Stefani, A., Fedele, E., Galati, S., Pepicelli, O., Frasca, S., Pierantozzi, M., et al. (2005). Subthalamic stimulation activates internal pallidus: evidence from cGMP microdialysis in PD patients. Ann. Neurol. 57, 448–452. doi: 10.1002/ana.20402
Tachibana, Y., Iwamuro, H., Kita, H., Takada, M., and Nambu, A. (2011). Subthalamo-pallidal interactions underlying parkinsonian neuronal oscillations in the primate basal ganglia. Eur. J. Neurosci. 34, 1470–1484. doi: 10.1111/j.1460-9568.2011.07865.x
Tai, C. H., Boraud, T., Bezard, E., Bioulac, B., Gross, C., and Benazzouz, A. (2003). Electrophysiological and metabolic evidence that high-frequency stimulation of the subthalamic nucleus bridles neuronal activity in the subthalamic nucleus and the substantia nigra reticulata. FASEB J. 17, 1820–1830. doi: 10.1096/fj.03-0163com
Tawfik, V. L., Chang, S. Y., Hitti, F. L., Roberts, D. W., Leiter, J. C., Jovanovic, S., et al. (2010). Deep brain stimulation results in local glutamate and adenosine release: investigation into the role of astrocytes. Neurosurgery 67, 367–375. doi: 10.1227/01.neu.0000371988.73620.4c
Vedam-Mai, V., van Battum, E. Y., Kamphuis, W., Feenstra, M. G., Denys, D., Reynolds, B. A., et al. (2012). Deep brain stimulation and the role of astrocytes. Mol. Psychiatry 17, 124–131. doi: 10.1038/mp.2011.61
Vidailhet, M., Jutras, M. F., Roze, E., and Grabli, D. (2013). Deep brain stimulation for dystonia. Handb. Clin. Neurol. 116, 167–187. doi: 10.1016/B978-0-444-53497-2.00014-0
Vitek, J. L. (2008). Deep brain stimulation: how does it work? Cleve. Clin. J. Med. 75(Suppl. 2), S59–S65. doi: 10.3949/ccjm.75.suppl_2.s59
Wang, L. Y., and Kaczmarek, L. K. (1998). High-frequency firing helps replenish the readily releasable pool of synaptic vesicles. Nature 394, 384–388. doi: 10.1038/28645
Welter, M. L., Houeto, J. L., Bonnet, A. M., Bejjani, P. B., Mesnage, V., Dormont, D., et al. (2004). Effects of high-frequency stimulation on subthalamic neuronal activity in parkinsonian patients. Arch. Neurol. 61, 89–96. doi: 10.1001/archneur.61.1.89
Wichmann, T. (2000). A digital averaging method for removal of stimulus artifacts in neurophysiologic experiments. J. Neurosci. Methods 98, 57–62. doi: 10.1016/s0165-0270(00)00190-4
Wichmann, T., Bergman, H., and DeLong, M. R. (1994). The primate subthalamic nucleus. III. Changes in motor behavior and neuronal activity in the internal pallidum induced by subthalamic inactivation in the MPTP model of parkinsonism. J. Neurophysiol. 72, 521–530.
Wichmann, T., and Delong, M. R. (2006). Deep brain stimulation for neurologic and neuropsychiatric disorders. Neuron 52, 197–204. doi: 10.1016/j.neuron.2006.09.022
Windels, F., Bruet, N., Poupard, A., Urbain, N., Chouvet, G., Feuerstein, C., et al. (2000). Effects of high frequency stimulation of subthalamic nucleus on extracellular glutamate and GABA in substantia nigra and globus pallidus in the normal rat. Eur. J. Neurosci. 12, 4141–4146. doi: 10.1046/j.1460-9568.2000.00296.x
Windels, F., Carcenac, C., Poupard, A., and Savasta, M. (2005). Pallidal origin of GABA release within the substantia nigra pars reticulata during high-frequency stimulation of the subthalamic nucleus. J. Neurosci. 25, 5079–5086. doi: 10.1523/jneurosci.0360-05.2005
Wu, Y. R., Levy, R., Ashby, P., Tasker, R. R., and Dostrovsky, J. O. (2001). Does stimulation of the GPi control dyskinesia by activating inhibitory axons? Mov. Disord. 16, 208–216. doi: 10.1002/mds.1046
Keywords: deep brain stimulation, basal ganglia, subthalamic nucleus, globus pallidus, cortico-basal ganglia loop, electrophysiology
Citation: Chiken S and Nambu A (2014) Disrupting neuronal transmission: mechanism of DBS? Front. Syst. Neurosci. 8:33. doi: 10.3389/fnsys.2014.00033
Received: 26 August 2013; Accepted: 19 February 2014;
Published online: 14 March 2014.
Edited by:
Ahmed A. Moustafa, University of Western Sydney, AustraliaReviewed by:
Alessandro Stefani, University of Rome, ItalyRobert S. Turner, University of Pittsburgh, USA
Copyright © 2014 Chiken and Nambu. This is an open-access article distributed under the terms of the Creative Commons Attribution License (CC BY). The use, distribution or reproduction in other forums is permitted, provided the original author(s) or licensor are credited and that the original publication in this journal is cited, in accordance with accepted academic practice. No use, distribution or reproduction is permitted which does not comply with these terms.
*Correspondence: Atsushi Nambu, Division of System Neurophysiology, National Institute for Physiological Sciences and Department of Physiological Sciences, Graduate University for Advanced Studies, 38 Nishigonaka, Myodaiji, Okazaki 444-8585, Japan e-mail: nambu@nips.ac.jp