- 1Department of Clinical and Cognitive Neuroscience, Central Institute of Mental Health, Medical Faculty Mannheim, Heidelberg University, Mannheim, Germany
- 2Small Animal Clinic, Department of Veterinary Clinical Sciences, University of Gießen, Gießen, Germany
- 3Section of Biomagnetism, Department of Neurology, University Hospital Heidelberg, Heidelberg, Germany
Objectives: In tinnitus, several brain regions seem to be structurally altered, including the medial partition of Heschl's gyrus (mHG), the site of the primary auditory cortex. The mHG is smaller in tinnitus patients than in healthy controls. The corpus callosum (CC) is the main interhemispheric commissure of the brain connecting the auditory areas of the left and the right hemisphere. Here, we investigate whether tinnitus status is associated with CC volume. Methods: The midsagittal cross-sectional area of the CC was examined in tinnitus patients and healthy controls in which an examination of the mHG had been carried out earlier. The CC was extracted and segmented into subregions which were defined according to the most common CC morphometry schemes introduced by Witelson (1989) and Hofer and Frahm (2006). Results: For both CC segmentation schemes, the CC posterior midbody was smaller in male patients than in male healthy controls and the isthmus, the anterior midbody, and the genou were larger in female patients than in female controls. With CC size normalized relative to mHG volume, the normalized CC splenium was larger in male patients than male controls and the normalized CC splenium, the isthmus and the genou were larger in female patients than female controls. Normalized CC segment size expresses callosal interconnectivity relative to auditory cortex volume. Conclusion: It may be argued that the predominant function of the CC is excitatory. The stronger callosal interconnectivity in tinnitus patients, compared to healthy controls, may facilitate the emergence and maintenance of a positive feedback loop between tinnitus generators located in the two hemispheres.
Introduction
Tinnitus is an auditory phantom sensation that is experienced in the absence of adequate acoustic stimulation. Affected individuals often describe it as kind of ringing, hissing, or buzzing, most often continuous, sometimes intermittent. Tinnitus affects a considerable proportion of the general population, with a minority experiencing considerable distress (Hazell, 1990; Feldmann, 1998; Baguley and McFerran, 2002; Lockwood et al., 2002; Eggermont and Roberts, 2004; Shargorodsky et al., 2010). Often, but not always, tinnitus is associated with a certain measure of hearing loss mostly of the upper part of the range of hearing (Henry et al., 1999). Hearing loss represents the most prevalent risk factor for tinnitus (Sindhusake et al., 2003; Moller, 2007).
The pathophysiological mechanisms underlying tinnitus are largely unknown. The disorder is characterized by hyperexcitability resulting from a reduction of inhibitory processes and gain increase at various stages of the afferent auditory pathway including the auditory cortex (Salvi et al., 2000; Syka, 2002; Diesch et al., 2004; Eggermont and Roberts, 2004; Parra and Pearlmutter, 2007; Sun et al., 2009; Norena, 2011). Maladaptive plastic changes of the functional organization of the auditory system and the auditory cortex in particular seem to be implicated (Mühlnickel et al., 1998; Wienbruch et al., 2006). Several brain regions of tinnitus patients appear to be structurally altered, among them the thalamus and the nucleus accumbens (Mühlau et al., 2006), the inferior colliculus and the hippocampus (Landgrebe et al., 2009), and the medial partition of Heschl's gyrus (mHG), the anatomical site of the primary auditory cortex (Schneider et al., 2009).
Schneider et al. (2009) found smaller gray matter volumes of the medial partition of mHG in tinnitus patients than in healthy controls. Compared to hearing-impaired controls without tinnitus, patients with bilateral tinnitus showed mHG volume reduction in both hemispheres. Patients with unilateral tinnitus also showed mHG volume reduction. However, in these patients the reduction occurred almost exclusively on the side ipsilateral to the affected ear. To make sense of these volumetric reduction and lateralization effects the interconnection via the corpus callosum (CC) of the auditory cortices of the left and the right hemisphere may have to be considered.
Audition assumes a special status with regard to callosal connection. While in vision and somatosensation only the midline zone features callosal fiber connections, in audition callosal projections are distributed across the full extent of the auditory cortex (Bamiou et al., 2007). The CC fully connects homotopic and heterotopic auditory cortical areas of the two hemispheres. The callosal input to either hemisphere is characterized by a particular balance of excitatory and inhibitory processes. At the single unit level, the callosal fibers originating from cortical pyramidal cells are neurochemically excitatory. However, as they may terminate on inhibitory interneurons of the contralateral side, callosal projections are both excitatory and inhibitory functionally (Bloom and Hynd, 2005; Karayannis et al., 2007; Tang et al., 2007).
The CC is smaller in smaller brains (Jancke et al., 1997). More specifically, a smaller auditory cortex presumably gives rise to a smaller number of callosal fibers crossing to the contralateral side. In tinnitus, as long as the callosal volume reduction were proportional to the volume reduction of the auditory cortex, nothing would be expected to change. However, if the reduction in the number of callosal fibers were proportionally larger than the reduction of auditory cortex volume, the transcallosal influence would be weakened. In this case, if the default transcallosal influence were mainly inhibitory, the reduction in interhemispheric inhibition might be hypothesized to facilitate the emergence and maintenance of tinnitus. Conversely, if the reduction in the number of callosal fibers were proportionally smaller than the reduction of auditory cortex volume or if reduction of auditory cortex volume was accompanied by an increase of the number of callosal fibers, the transcallosal influence would be amplified. If the default transcallosal influence were largely excitatory, the increase in interhemispheric excitation likely would facilitate the development and persistence of tinnitus.
Apart from cortical volume, hemisphere asymmetry of cortical volume may also be important. On the basis of findings in rats, Galaburda et al. (1990) and Rosen et al. (1989) have proposed the existence of an inverse relationship between callosal connectivity and the magnitude of cortical hemisphere asymmetry. In humans, this inverse relationship has been confirmed by Aboitiz et al. (1992b,c), and Dorion et al. (2000), and with some caveats relating to the influence of gender and handedness, Luders et al. (2003). Luders et al. reported an inverse relationship between midsagittal callosal area and perisylvian asymmetries for males, but not for females. In the sample studied by Schneider et al. (2009), the mHG ipsilateral to the ear affected by tinnitus was smaller and the interhemispheric mHG asymmetry index was larger in patients with unilateral tinnitus than in healthy controls. Given the relationship between hemisphere asymmetry and CC size, it may be hypothesized that the Schneider et al. hemisphere asymmetry index is correlated with CC size, with gender eventually modulating the relationship. Furthermore, under the assumption that the default transcallosal influence were predominantly inhibitory, reduced CC size would mean reduced inhibition and potentially facilitate the development and maintenance of tinnitus.
Materials and Methods
Study Participants
In the current study, the midsagittal cross-sectional surface area of the CC was determined for T1-weighted structural MR images (Siemens TRIO, 3 T, MPRAGE, matrix 256 × 256 mm2, 176 sagittal slices, slice thickness 1 mm, TR 1930 ms, TE 4.38 ms) obtained from 105 subjects. Of these, 99 had been acquired in a prior volumetric study of the medial part (mHG) of Heschl's gyrus (Schneider et al., 2009)1. Six additional structural scans were available for the present study. Thus, CC volumetric scores were determined for 105 subjects and cross-correlations between CC and mHG volumetric scores for 99 subjects. Given the composition of the sample, the distribution of subjects across tinnitus status, musicality, and sex was almost the same as in the Schneider et al. (2009) study. As in Schneider et al. (2009), tinnitus patients were accepted to the study if they presented with chronic tonal or quasi-tonal tinnitus with a tinnitus frequency above 1 kHz. Tinnitus was considered chronic if its onset dated back 6 months or more. Individuals with noisiform or pulsatile tinnitus, Ménière's disease, otosclerosis, chronic headache, neurological disorders such as brain tumors, and individuals being treated for mental disorders were excluded from the study. Tinnitus was defined as chronic if it had lasted for more than 6 months. Controls did not present with tinnitus. Participants were assigned to the musician group if they worked as professional musicians, earned a score of at least 25 on the Advanced Measures of Music Audiation (AMMA) test, a standardized test of musicality which is independent of musical expertise (Gordon, 1989, 1998), or both. There were 25 musicians with tinnitus, 10 of them female, 38 non-musicians with tinnitus, 10 of them female, 31 musicians (13 female) without tinnitus, and 11 non-musicians (7 female) without tinnitus (Table 1). Pure-tone audiograms were obtained for 12 tonal frequencies between 125 Hz and 15 kHz to document the extent of hearing loss (Figure 1).
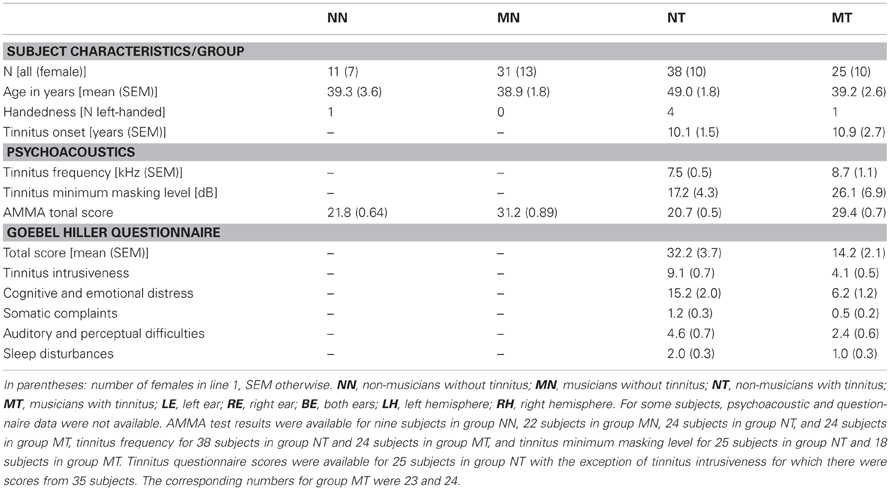
Table 1. Subject characteristics, psychoacoustic test results, and TQ tinnitus questionnaire scores.
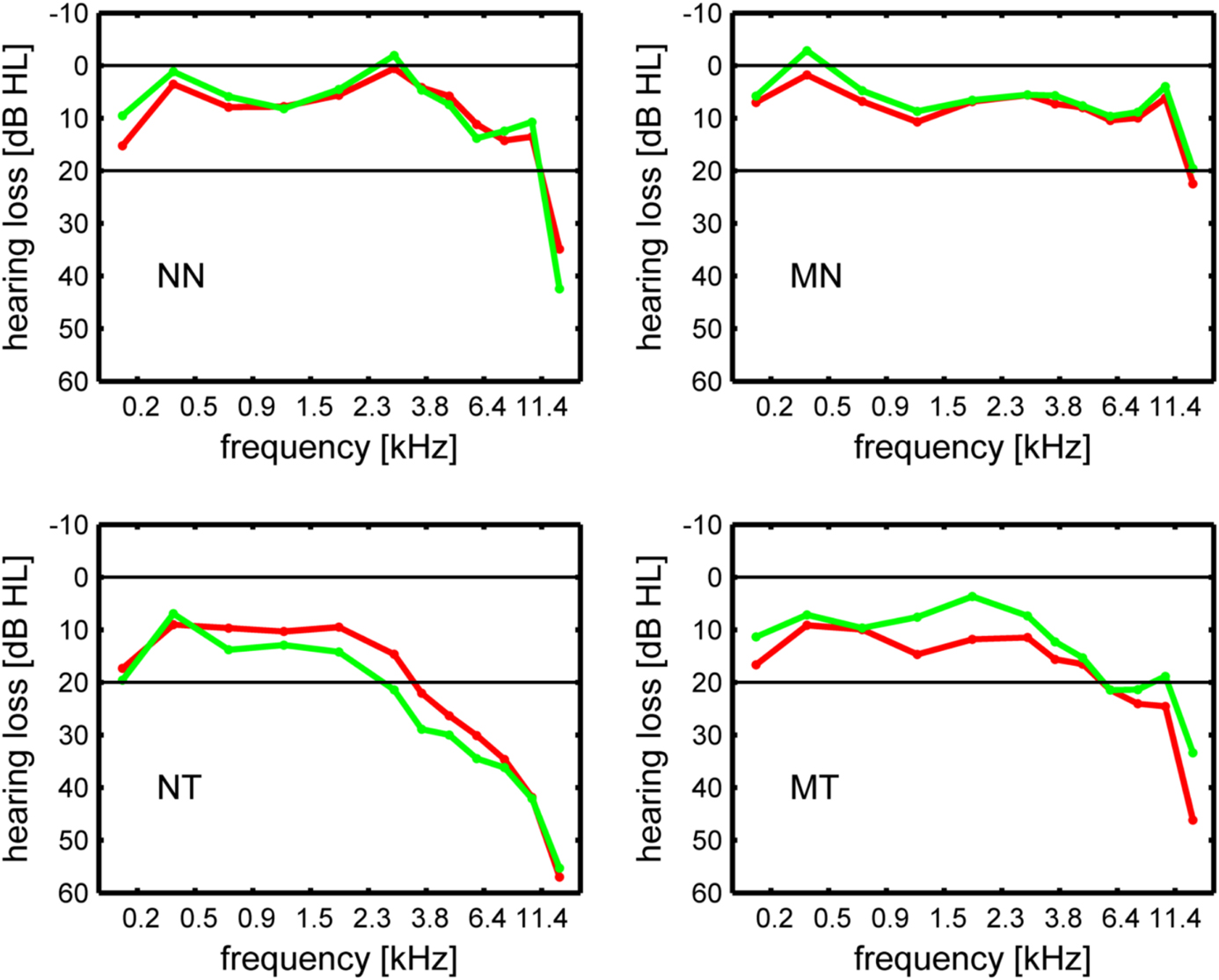
Figure 1. Hearing loss in dB HL in the left ear (green line) and the right ear (red line). NN, non-musicians without tinnitus; MN, musicians without tinnitus; NT, non-musicians with tinnitus; MT, musicians with tinnitus.
Summary indices of hearing loss were determined by computing averages across frequencies, i.e., across right and left ear low frequency (125 Hz–746 Hz) and high frequency (1.183 kHz–15 kHz) hearing loss scores. For patients, hearing loss at the tinnitus frequency was computed both for the left and the right ear by interpolating between the respective hearing loss values that were closest to the tinnitus frequency on the frequency axis. The equivalent tonal frequency and the minimum masking level of the tinnitus were determined using the methods described in Schneider et al. (2009). There was no significant difference between musicians with tinnitus and non-musicians with tinnitus with regard to the tinnitus frequency (t(60) = 1.13, n.s.) or the tinnitus minimum masking level (t(41) = 1.14, n.s.). Patients were also asked to complete the German version of the Tinnitus Questionnaire (TQ, Hallam et al., 1988; Hallam, 1996) published by Goebel and Hiller (Goebel and Hiller, 1994, 1998). Musicians with tinnitus attained significantly lower scores than non-musicians with tinnitus on the TQ (t(46) = 4.16, p < 0.0005) and its subscales (tinnitus intrusiveness: t(57) = 5.23, p < 0.0005, cognitive and emotional distress: t(46) = 3.78, p < 0.0005, somatic complaints: t(46) = 2.12, p < 0.05, auditory and perceptual difficulties: t(46) = 2.33, p < 0.025, sleep disturbances: t(46) = 2.41, p < 0.02).
Corpus Callosum Segmentation
Morphological analysis of the CC was done in analogy to the procedure described by Luders et al. (2003). The midline sagittal cross-sectional surface area of the CC was reconstructed from the T1-weighted MRI data with the software program MRIcro (Ver. 1.40). Midsagittal sections were identified relative to the interhemispheric fissure in sagittal, horizontal, and coronal views. They were confirmed by the presence of the cerebral falx. The original sagittal slices were reorientated to maximize apparent extent of the CC in the sagittal view. Using MRIcro's free rotation function, the whole brain was resliced such that the longitudinal fissure ran parallel to the sagittal slices. A tissue intensity contrast-based region-of-interest (ROI) comprising the CC was defined in the slice that included the longitudinal fissure and the two neighboring slices to the left and the right using the MRIcro “image intensity defined 3D ROI” function. Tissue intensity thresholds were manually adjusted on an individual basis to optimally capture and extract the CC. If the CC and neighboring structures like the fornix did not differ in intensity or else were not segregated by an intensity-marked boundary, the automatic segmentation was corrected manually. The CC surface area image thus delineated was extracted from each of the three selected slices and saved for further processing. The segmentation was performed blindly, i.e., without knowledge on the side of the segmenter of the participant group the brain originated from.
Using procedures written in matlab and functions of the matlab image processing toolbox, the extracted CC images were segmented into subregions defined by the segmentation schemes put forward by Witelson (1989) and Hofer and Frahm (2006). The CC segmentation proposed by Witelson (1989) is the one most frequently used. As the Witelson scheme has been derived from non-human primates, the human diffusion tensor imaging-derived scheme proposed by Hofer and Frahm (2006) was used as an alternative. Figure 2 illustrates the 2 segmentation schemes. The Witelson scheme employs four coronal subdivisions located at, from anterior to posterior, 1/3, 1/2, 2/3, and 4/5 of the total length of the CC. These subdivisions section the CC into five partitions representing the genu, the anterior part of the midbody, the posterior part of the midbody, the isthmus, and the splenium. The Hofer and Frahm scheme places the subdivisions somewhat differently, at 1/6, 1/2, 2/3, and 3/4. Relative to the Witelson scheme, the Hofer and Frahm scheme reduces segments 1 and 4 in size, increases segments 2 and 5, and leaves segment 3 unchanged.
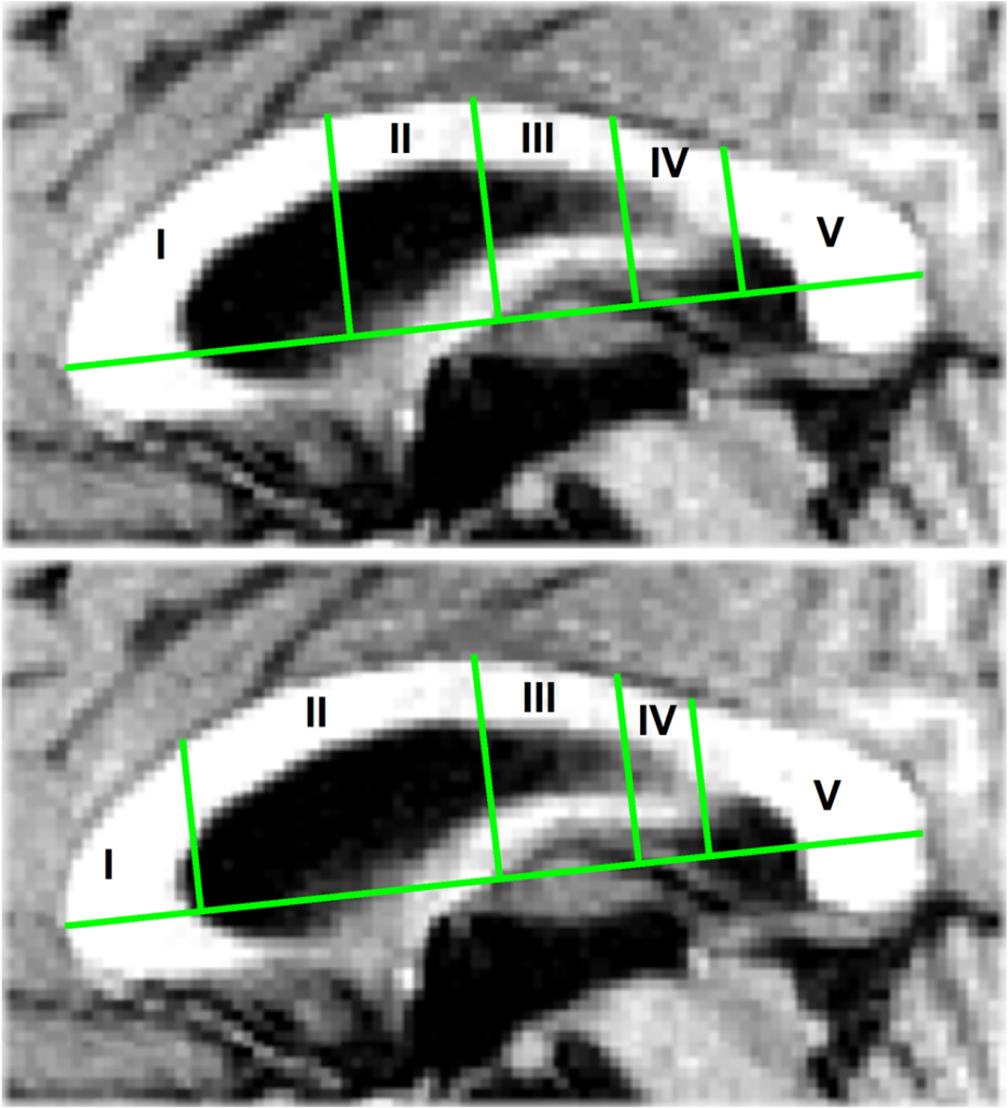
Figure 2. (Top) The Witelson (1989) segmentation of the corpus callosum with four coronal subdivisions at, from anterior to posterior, 1/3, 1/2, 2/3, and 4/5 of the corpus callosum total length. (Bottom) The Hofer and Frahm (2006) segmentation with subdivisions at 1/6, 1/2, 2/3, and 3/4 of the total length.
Area measurements for the entire CC surface area and the surface area of each of the segments were obtained in mm2 and pooled across the three sagittal slices selected. For a subset of 10 brains both intra- and inter-rater reliability coefficients were determined. For the entire CC surface area the intra-rater reliability was r = 0.97 and the inter-rater reliability r = 0.98. Intra-rater reliability ranged from 0.94 and inter-rater reliability from 0.96 to 0.99 for the Witelson and the Hofer and Frahm segments.
Data Analysis
The Witelson CC segment surface area scores and, separately, the Hofer and Frahm scores were submitted to a multivariate analysis of covariance (SPSS procedures GLM and MANOVA) with the CC segment scores as dependent variables, musicality and tinnitus status as grouping factors, and with whole intracranial brain size as a covariate. Intracranial brain size was determined using the Brainsuite software package (Shattuck and Leahy, 2002). The whole brain size covariate was used to normalize the CC segment scores. Simple effects analyses were run with sex as parameter. In analogy to Schneider et al. (2009), the analysis was rerun with age, handedness, and the four summary hearing loss indices as additional covariates. Because univariate analysis, but not multivariate analysis, offers the possibility to localize effects to particular CC segments, both multivariate and univariate contrasts were considered.
In the Schneider et al. (2009) study, the gray matter volumes of the mHG of both hemispheres were computed. The mHG was partitioned in eight successive cross-sectional 3 mm-slices orientated perpendicularly to the major axis of the respective individual mHG. For each of the eight homologous segment pairs, an index of volumetric (a) symmetry was computed in terms of the standardized difference between the right and the left hemisphere volume: δS = (VRH−VLH)/(VRH+VLH). This index assumes positive values if the right hemisphere volume is larger than the left hemisphere volume and negative values if the left hemisphere volume is larger than the right hemisphere volume. For the present study, bilateral compound mHG segment volumes comprising homologous mHG segment pairs were computed and submitted to multivariate analysis of covariance with whole brain size as a covariate. The maximum of the absolute volumetric asymmetry, max(abs(δS)), was calculated and the segment index score of the segment where the asymmetry attained its maximum was determined.
Presumably, any putative alterations of CC size in tinnitus would be closely related to the alterations in the auditory cortex. However, a smaller CC in tinnitus patients, compared with healthy controls, could represent an epiphenomenon of the smaller auditory cortex rather than a structural factor that might be related to tinnitus in its own right. To disentangle these alternatives, normalized CC segment surface scores, i.e., CC segment scores divided by the total bilateral compound mHG volume to the power of 2/3, i.e., normalized CCsurface = CCsurface/mHGvolume(2/3), were computed and submitted to analysis of variance. For tinnitus patients, correlations were computed between the normalized CC segment surface scores and the Goebel and Hiller (1994, 1998) tinnitus questionnaire total score as well as the “tinnitus intrusiveness” subscale score.
When they were computed across all study participants, Schneider et al. (2009) found significant negative correlations between three of the four summary indices of hearing loss and left and right hemisphere mHG volume. These correlations were recomputed for the present sample, both without and with age as a covariate. In addition, (partial) correlations were computed between the hearing loss indices and total CC surface area, the surface area of the anterior CC (Witelson/Hofer and Frahm segments I and II), and the surface area of the posterior CC (Witelson/Hofer and Frahm segments III, IV, and V) surface area. Again in correspondence with Schneider et al. (2009), in order to more closely examine the relationship between CC surface area and hearing loss, the partial correlations of these CC surface area indices and the hearing loss indices were also determined separately for the four groups of subjects. In the tinnitus groups, the partial correlations with left and right ear hearing loss estimated for the tinnitus frequency was also determined.
The maximum absolute mHG segment asymmetry and its segmental location were also submitted to analyses of variance with tinnitus status and musicality as grouping factors and sex as the parameter in simple effects analyses. To assess the hypothesis of a negative correlation between hemispheric asymmetry and CC size, partial correlations, with whole brain size as a covariate, between the maximum absolute volumetric asymmetry score and the CC segment surface area scores were computed.
Results
Table 2 summarizes the corpus callosum (CC) segmentation results. Tinnitus status did not show a significant multivariate effect on CC segment size (Witelson: T2(5, 96) = 0.11, n.s.; Hofer and Frahm: T2(5, 96) = 0.10, n.s.). However, there were significant univariate effects. For both segmentation schemes the third segment was significantly smaller in tinnitus patients than in healthy controls (Witelson/Hofer and Frahm segment III: F(1,100) = 5.56, p < 0.02). For musicality results differed between the segmentation schemes both with regard to multivariate (Witelson: T2(5, 96) = 0.09, n.s.; Hofer and Frahm: T2(5, 96) = 0.13, p < 0.05) and univariate effects (Witelson segment II: F(1, 100) = 5.98, p < 0.02; Hofer segment I: F(1, 100) = 5.48, p < 0.025; Hofer segment II: F(1, 100) = 5.35, p < 0.025; Hofer segment IV: F(1, 100) = 5.81, p < 0.02). The Witelson segment II and the Hofer segments I and IV were smaller in musicians than non-musicians. The Hofer segment II was larger in musicians than in non-musicians. There were no other significant multivariate or univariate effects.
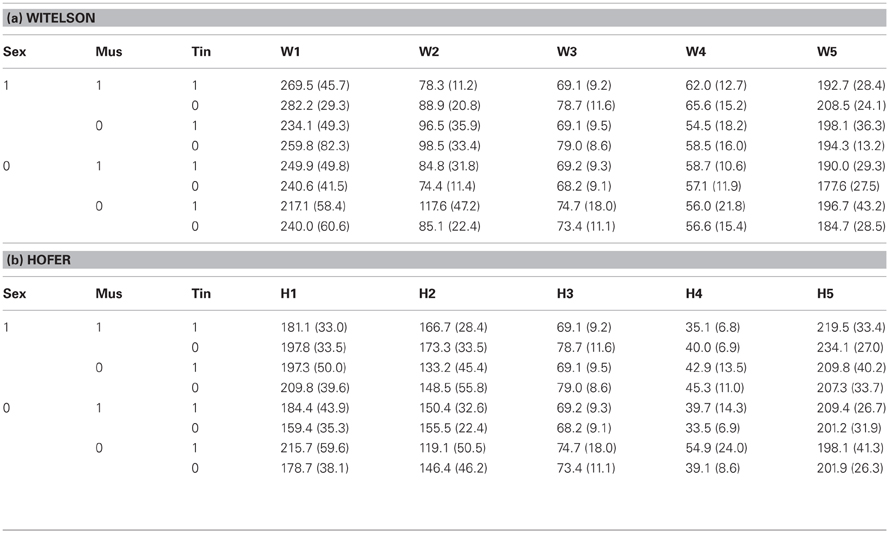
Table 2. Corpus callosum segment sagittal surface area in mm2 (standard deviation in parentheses) according to (a) Witelson (1989) and (b) Hofer and Frahm (2006) subdivided by sex (1: male, 0: female), musicality (1: musician, 0: non-musician), and tinnitus status (1: with tinnitus, 0: without tinnitus).
In the simple effect analyses, the multivariate effect of tinnitus status onto CC segment size was significant in males (Witelson: T2(5, 96) = 0.12, p < 0.05; Hofer and Frahm: T2(5, 96) = 0.12, p < 0.05), but not in females (Witelson: T2(5, 96) = 0.08, n.s.; Hofer and Frahm: T2(5, 96) = 0.09, n.s.). There were no significant multivariate effects of musicality and the musicality-by-tinnitus status interaction in either group. As to univariate tests, CC segment III was significantly smaller in male tinnitus patients than male healthy controls (Witelson, Hofer and Frahm: F(1, 100) = 10.00, p < 0.002). In males there were no significant univariate effects of musicality and of the musicality-by-tinnitus status interaction. In female tinnitus patients, the segments Witelson II and Hofer and Frahm I and IV were significantly larger than in female healthy controls (Witelson II: F(1, 100) = 5.48, p < 0.025; Hofer and Frahm I: F(1, 100) = 6.26, p < 0.02; Hofer and Frahm IV: F(1, 100) = 7.60, p < 0.01). In females, the same segments were smaller in musicians than non-musicians (Witelson II: F(1, 100) = 5.81, p < 0.02; Hofer and Frahm I: F(1, 100) = 5.52, p < 0.025; Hofer and Frahm IV: F(1, 100) = 6.51, p < 0.02).
These results were essentially preserved in the extended covariate analysis with intracranial brain size, age, handedness, and the four summary hearing loss indices as covariates both in males (tinnitus status: Witelson: T2(5, 79) = 0.15, p < 0.05; Hofer and Frahm: T2(5, 79) = 0.14, p < 0.06; Witelson/Hofer and Frahm segment III: F(1, 83) = 8.56, p < 0.005) and females (tinnitus status: segment Witelson II: F(1, 83) = 3.82, p < 0.06; segment Hofer and Frahm I: F(1, 83) = 4.59, p < 0.05; segment Hofer and Frahm IV: F(1, 83) = 6.65, p < 0.02; musicality: segment Witelson II: F(1, 83) = 5.98, p < 0.02; segment Hofer and Frahm I: F(1, 83) = 5.92, p < 0.02; segment Hofer and Frahm IV: F(1, 83) = 6.81, p < 0.02).
There were significant multivariate and univariate effects both of tinnitus status and musicality on bilateral compound mHG segment volume (tinnitus status: T2(8, 86) = 0.23, p < 0.02, F(1, 93) = 10.5–13.1, p < 0.002–0.0005; musicality: T2(8, 86) = 0.65, p < 0.0005, F(1, 93) = 24.7–48.6, p < 0.0005). There were no significant effects of the interaction. All eight bilateral compound mHG segments were smaller in tinnitus patients than in healthy controls and larger in musicians than in non-musicians. In the simple effects analyses, the multivariate effects of tinnitus status and the interaction of tinnitus status and musicality were not significant either for males or females. However, in the univariate analysis all eight bilateral compound mHG segments were significantly smaller in male tinnitus patients than in male healthy controls (F(1, 93) = 4.6–8.3, p < 0.05–0.005). For all bilateral compound mHG segments, the difference went into the same direction in females, but without attaining significance (F(1, 93) = 0.9–3.6, p < 0.40–0.07). For musicality, multivariate and univariate effects were significant in males and in females. In both groups, each of the segments was significantly larger in musicians than in non-musicians (males: T2(8, 86) = 0.33, p < 0.001, F(1, 93) = 4.1–24.1, p < 0.05–0.0005; females: T2(8, 86) = 0.21, p < 0.05, F(1, 93) = 7.6–12.9, p < 0.01–0.001).
Normalized CC segment size showed significant multivariate effects of tinnitus status (Witelson: T2(5, 90) = 0.17, p < 0.02; Hofer and Frahm: T2(5, 90) = 0.16, p < 0.02). In the univariate analysis, the Witelson segments II (F(1, 94) = 5.9, p < 0.02) and V (F(1, 94) = 10.8, p < 0.001) and the Hofer and Frahm segments I (F(1, 94) = 8.1, p < 0.005), IV (F(1, 94) = 5.9, p < 0.02), and V (F(1, 94) = 8.5, p < 0.005) were significant. Musicality also featured significant multi- and univariate effects (Witelson: T2(5, 90) = 0.51, p < 0.0005, F(1, 94) = 13.9–45.4, p < 0.0005–0.00005; Hofer: T2(5, 90) = 0.54, p < 0.0005, F(1, 94) = 9.5–45.4, p < 0.005–0.00005), with each of the segments individually attaining significance. Normalized CC segment size was larger for tinnitus patients than for healthy controls and larger for non-musicians than for musicians (Figure 3). There were no significant multi- or univariate effects of the tinnitus status-by-musicality interaction.
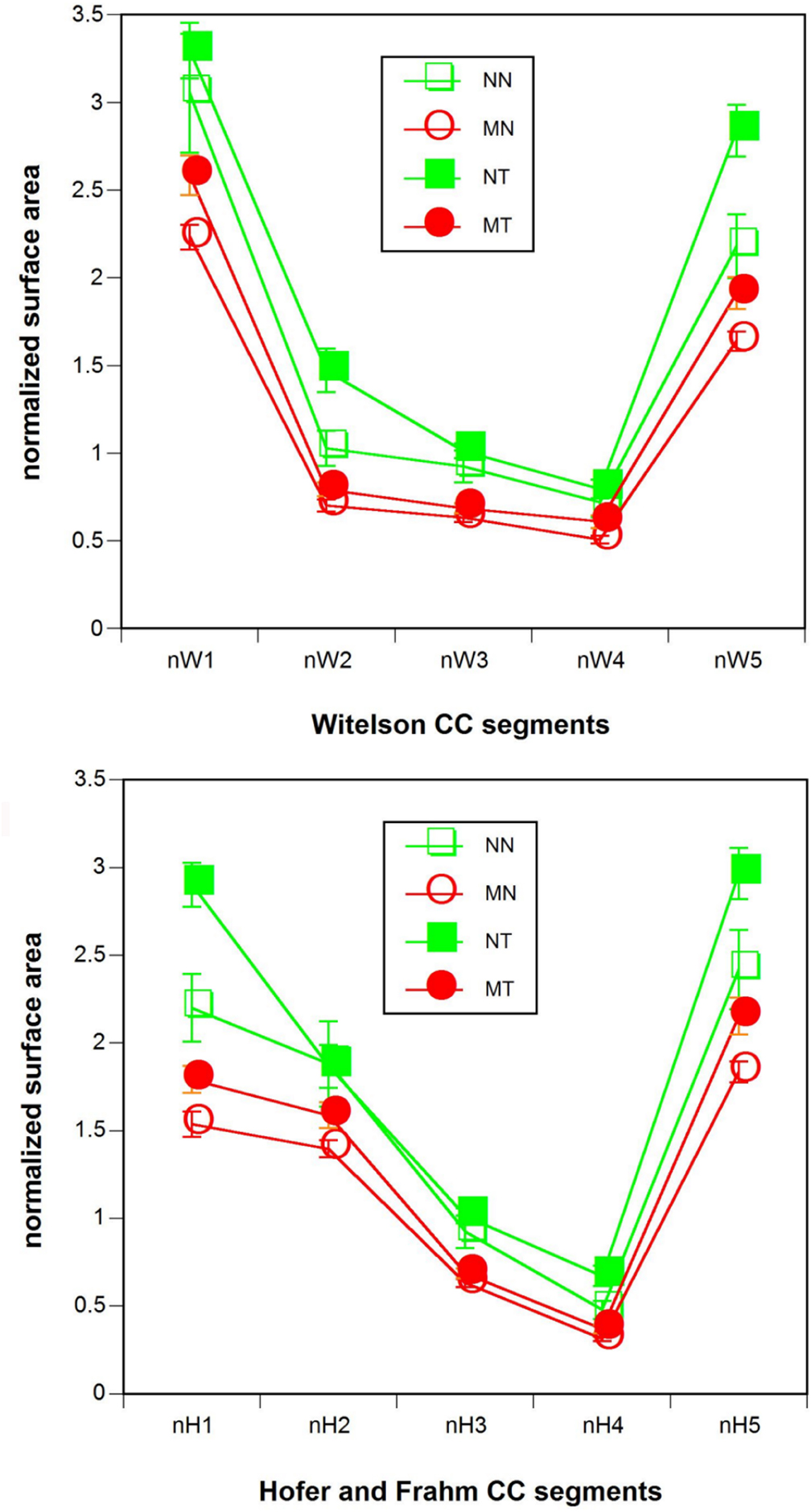
Figure 3. Normalized (top) Witelson (1989) and (bottom) Hofer and Frahm (2006) CC segment size. Red: musicians. Green: non-musicians. Filled symbols: tinnitus patients. Open symbols: healthy controls. NN, non-musicians without tinnitus; MN, musicians without tinnitus; NT, non-musicians with tinnitus; MT, musicians with tinnitus.
In simple effects analyses with sex as parameter the multivariate effects of musicality and, with the exception of the Hofer and Frahm segment II, all univariate effects of musicality were preserved both for males (Witelson: T2(5, 90) = 0.20, p < 0.005, F(1, 94) = 5.5–17.7, p < 0.05–0.0005; Hofer: T2(5, 90) = 0.21, p < 0.005, F(1, 94) = 3.3–17.7, p < 0.08–0.0005) and females (Witelson: T2(5, 90) = 0.17, p < 0.02, F(1, 94) = 5.5–15.1, p < 0.025–0.0005; Hofer: T2(5, 90) = 0.16, p < 0.02, F(1, 94) = 2.8–15.1, p < 0.10–0.0005). However, the multivariate effects of tinnitus status were not preserved in the simple effects analysis either for males or females. Of the tinnitus status univariate effects only a few survived both for males (Witelson V: F(1, 94) = 4.2, p < 0.05; Hofer and Frahm segment V: F(1, 94) = 5.6, p < 0.02) and females (Witelson segment II: F(1, 94) = 7.3, p < 0.01; Witelson segment V: F(1, 94) = 4.1, p < 0.05; Hofer and Frahm segment I: F(1, 94) = 6.5, p < 0.02; Hofer and Frahm segment IV: F(1, 94) = 7.8, p < 0.01).
The correlations of normalized CC segment size with the total score of the Goebel and Hiller (1994, 1998) tinnitus questionnaire were positive throughout (r = 0.12– 0.47) and attained significance at the p < 0.05 level or better in the Witelson segments I, III, IV, and V and the Hofer and Frahm segments II, III, and V. For tinnitus intrusiveness the range of correlations was r = 0.22–0.46, with the Witelson segments I, III, IV, and V and the Hofer and Frahm segments I, II, III, and V being significant, again at p < 0.05 or better. The correlations with ‘normalized total CC size were r = 0.36, df = 46, p < 0.02 (Goebel and Hiller total score) and r = 0.43, df = 46, p < 0.001 (Goebel and Hiller intrusiveness score). By comparison, the total bilateral compound mHG volume was correlated negatively with the Goebel and Hiller total score (r = −0.44, df = 46, p < 0.002) and the Goebel and Hiller intrusiveness score (r = −0.48, df = 46, p < 0.0005) (Figure 4).
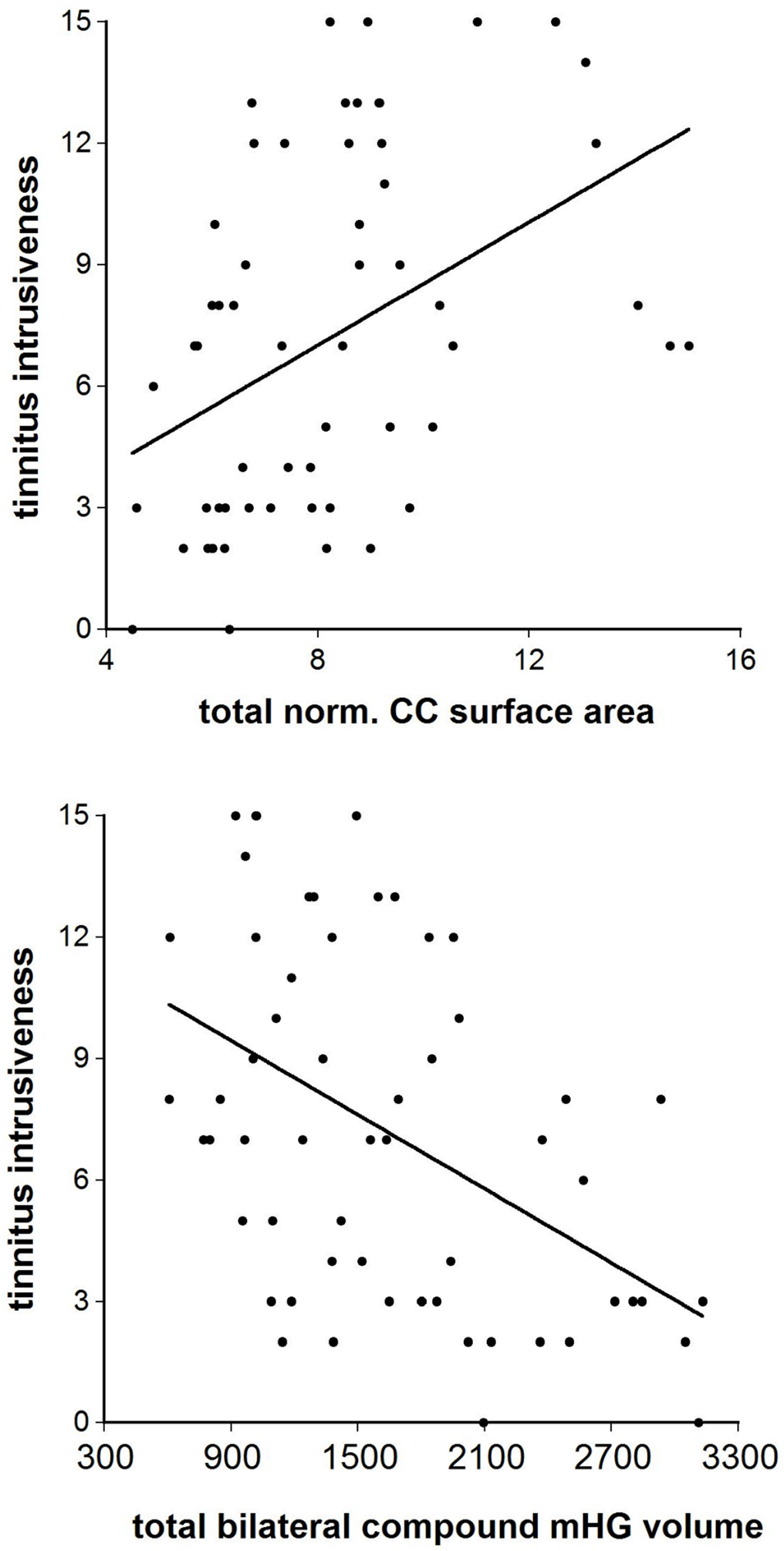
Figure 4. The correlation between the Goebel and Hiller (1994, 1998) tinnitus questionnaire “tinnitus intrusiveness” subscale and (top) total normalized corpus callosum midsagittal surface area and (bottom) total bilateral compound mHG volume.
Several of the correlations of bilateral compound, left hemisphere, and right hemisphere mHG volume with left and right ear low and high frequency hearing loss were statistically significant (Table 3, lines 1–3). The mHG volume indices were also significantly correlated with age, but the relationship of mHG volume and hearing loss was not completely accounted for by the age confound. When age was partialled out, the significance of the correlations of high frequency hearing loss in the left ear with bilateral compound and with right hemisphere mHG volume was preserved. By comparison, all correlations and partial correlations of total, anterior, and posterior CC surface area with the hearing loss indices were close to zero and statistically not significant (Table 3, lines 4–6). This picture did not change when the correlations and partial correlations were computed separately for males and females. The groupwise examination of the relationship of CC surface area and hearing loss resulted in a range of partial correlation coefficients, again with age as the covariate, of (−0.61 to −0.02) for non-musicians without tinnitus, of (−0.01–0.18) for musicians without tinnitus, and of (−0.13–0.19) for non-musicians with tinnitus, none of them statistically significant. However, for musicians with tinnitus the 12 partial correlation coefficients computed ranged from rpart = 0.01 to rpart = 0.66, 8 of them attained statistical significance, and 3 of them—the correlations between right ear high frequency hearing loss and anterior CC surface area (rpart = 0.58, p < 0.004), left ear hearing loss at the tinnitus frequency and anterior CC surface area (rpart = 0.66, p < 0.001), and left ear hearing loss at the tinnitus frequency and total CC surface area (rpart = 0.66, p < 0.001)—survived Bonferroni-Holmes correction. When male and female musicians with tinnitus were considered separately, the correlations of left ear hearing loss at the tinnitus frequency with total CC surface area (rpart = 0.73, p < 0.003) and anterior CC surface area (rpart = 0.76, p < 0.002) in males and of left ear high frequency loss with total CC surface area (rpart = 0.87, p < 0.005) and right ear low frequency loss with anterior CC surface area (rpart = 0.93, p < 0.001) in females survived Bonferroni-Holmes correction.
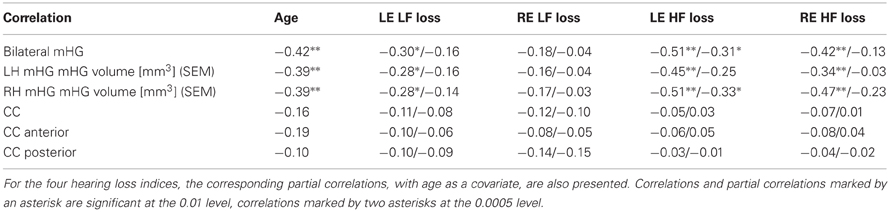
Table 3. Correlations of total bilateral mHG volume, total mHG volume of the left (LH) and the right hemisphere (RH), total corpus callosum (CC) volume, anterior CC surface area, and posterior CC surface area with chronological age, left ear low frequency loss (LE LF loss), right ear low frequency loss (RE LF loss), left ear high frequency loss (LE HF loss), and right ear high frequency loss (RE HF loss).
The distribution of the segmental location of the mHG asymmetry maximum is displayed in Figure 5. The most frequent segmental location of the maximum asymmetry differed between tinnitus patients and controls (F(1, 95) = 10.30, p < 0.002). In tinnitus patients, the asymmetry maximum was located most frequently in the medial segment S1. In healthy controls, the most frequent location of this maximum was a lateral one, in segment S7 (males) or S8 (females). The effect was statistically marginal for males (F(1, 95) = 3.15, p < 0.08), but significant for females (F(1, 95) = 7.14, p < 0.01). Musicality and the musicality-by-tinnitus status interaction were not significant.
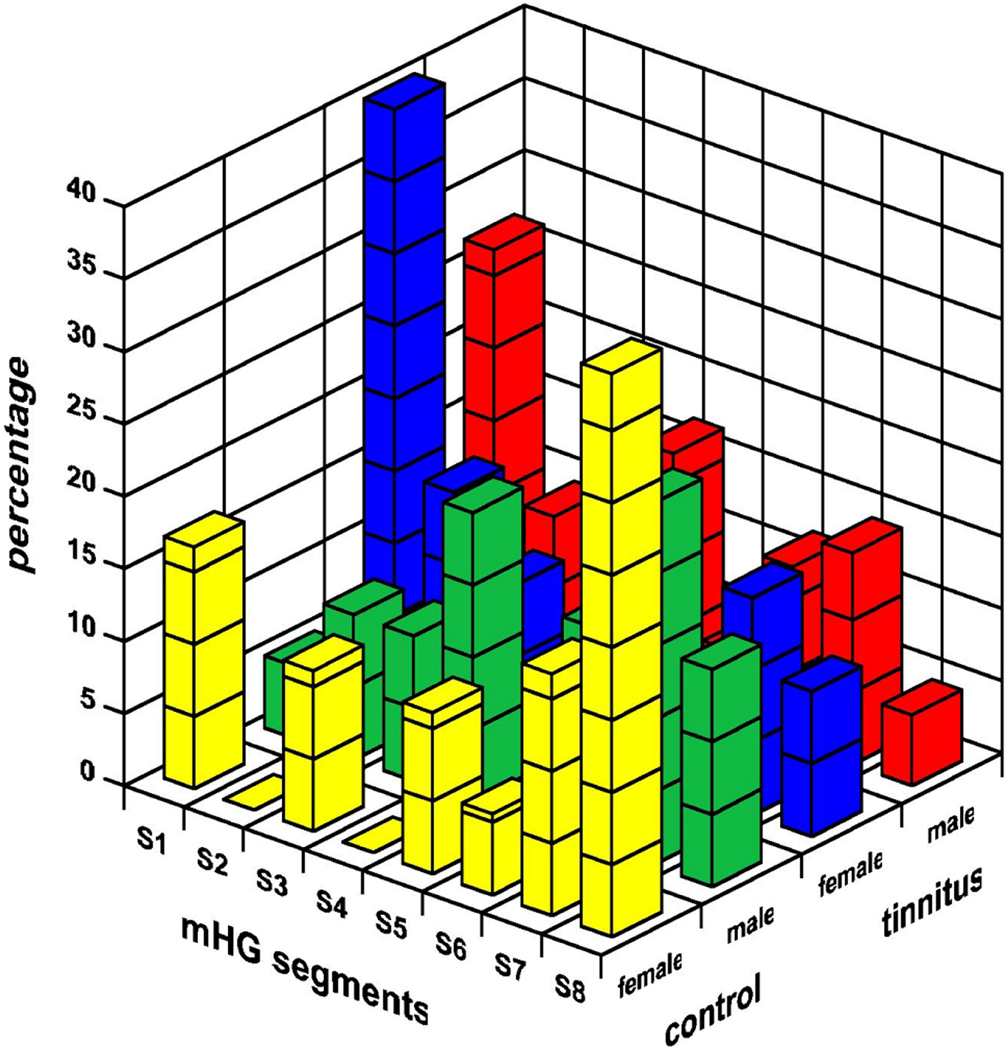
Figure 5. The segmental location of the mHG asymmetry maximum in male and female tinnitus patients and healthy controls. The mHG segments are numbered from most medial (S1) to most lateral (S8).
Because of the difference between tinnitus patients and controls in the segmental location of the mHG asymmetry maximum, maximum medial (segments 1–4) and maximum lateral (segments 5–8) segment asymmetry were determined separately. The maximum medial mHG segment asymmetry was larger for tinnitus patients than healthy controls (F(1, 95) = 7.74, p < 0.01). Simple effect analyses showed that this contrast was significant for males (F(1, 95) = 8.21, p < 0.005), but not for females (F(1, 95) = 1.68, n.s). Musicality and the musicality-by-tinnitus status interaction were not significant. There were no significant effects of tinnitus status, musicality, or their interaction on maximum lateral mHG segment asymmetry.
However, for the maximum medial mHG segment asymmetry, none of the partial correlations with CC segment size, with whole brain size as a covariate, was statistically significant. Significant partial correlations were obtained for male musicians between maximum lateral mHG segment asymmetry and the size of the third Witelson/the third Hofer and Frahm CC segment (rpart = −0.43, df = 29, p < 0.02) and the second Hofer and Frahm CC segment (rpart = −0.38, df = 29, p < 0.05). With tinnitus status as an additional covariate, these correlations were hardly affected (third Witelson/third Hofer and Frahm CC segment: rpart = −0.40, df = 28, p < 0.05; second Hofer and Frahm CC segment: rpart = −0.37, df = 28, p < 0.05).
Discussion
The present study revealed effects of tinnitus status on CC midsagittal segment surface area that were dependent on sex and, for females, on the segmentation scheme used, Witelson (1989) or Hofer and Frahm (2006). In males, when the size of the entire brain was taken into account, the posterior midbody of the CC, i.e., the Witelson and Hofer and Frahm segment III, was smaller in tinnitus patients than in healthy controls. In female tinnitus patients, the Witelson segment II and the Hofer and Frahm segments I and IV were larger than they were in female healthy controls. The results were not appreciably altered when age, handedness, and indices of hearing loss were added as covariates. This pattern of findings does not lend itself to a straightforward interpretation. Fibers interconnecting the primary and association auditory cortical fields of both sides have been located in the posterior midbody, corresponding to the Witelson/Hofer and Frahm segment III (Aboitiz et al., 1992a; Chance et al., 2006), but also in the isthmus, corresponding to the Witelson/Hofer and Frahm segment IV (Cipolloni and Pandya, 1985; Witelson, 1989; Aboitiz et al., 1992a; Hofer and Frahm, 2006), and in the splenium, corresponding to the Witelson/Hofer and Frahm segment V (Sugishita et al., 1995; Pollmann et al., 2002; Fujimoto et al., 2006). The role in females of the Witelson segment II which carries premotor and supplementary motor fibers (Witelson, 1989; Hofer and Frahm, 2006) and of the Hofer and Frahm segment I which carries prefrontal fibers (Hofer and Frahm, 2006) suggests that attentional factors may be involved.
It seems difficult to make sense of the difference between males and females in the effect of tinnitus on CC segment size, decrease of the posterior midbody in males and increase of the isthmus and the genou in females. As the relationship of CC surface area to hearing loss was small and statistically insignificant, both for the sample as a whole and for males and females taken separately, the difference cannot be accounted for in terms of hearing loss or the effect of hearing loss on CC size varying between the sexes. Tinnitus might eventually be facilitated by CC size decrease, if CC function were predominantly inhibitory, and by CC size increase, if its function were predominantly excitatory. There is not much reason to assume that the CC functions in a predominantly inhibitory fashion in males and a predominantly excitatory fashion in females. While some researchers have claimed that the female CC, if scaled relative to whole brain size, is larger and thus facilitates transfer of information between the hemispheres (Oka et al., 1999; Mitchell et al., 2003), others have not found a difference (Bishop and Wahlsten, 1997; Luders et al., 2006; Zarei et al., 2006). Thus, there is little reason to speculate on the possibility of males and females differing with regard to the relation of tinnitus to callosal structure and function. Most probably then, CC segment surface area, even if related to whole brain size, does not provide a structural measure that lends itself to a functional interpretation.
When it came to the analysis of normalized CC segment surface area with normalization relative to the size of the auditory cortex, the pattern of results was more homogeneous and lent itself to a more straightforward interpretation. Both tinnitus status and musicality were significant in the multivariate analysis. In the univariate analysis, the Witelson segments II and V and the Hofer and Frahm segments I, II, and V were significantly larger in tinnitus patients than they were in healthy controls. The direction of the effect was the same in the remaining segments, although without attaining significance. Furthermore, all normalized Witelson and Hofer and Frahm segments were significantly larger in non-musicians than in musicians. The simple effects analyses largely reproduced the main analysis. In males, the Witelson and the Hofer and Frahm segment V and in females, the Witelson segments II and V and the Hofer and Frahm segments I and IV were significantly larger in patients than in healthy controls. Both for males and females the difference went into the same direction for the segments where the effect fell short of significance. Within the tinnitus group, subjective ratings of tinnitus intrusiveness were correlated positively with the normalized size of the majority of both Witelson and Hofer and Frahm segments and negatively with total bilateral compound mHG volume. The effect of tinnitus on Witelson segment V and the Hofer and Frahm segments IV and V is to be expected, as these segments carry fibers projecting from primary and association auditory cortex.
As normalized CC segments represent a ratio of absolute CC segment size to mHG size, the effect of larger normalized CC segments in tinnitus could be the result of larger absolute CC segment size, smaller mHG size, or both. In males with tinnitus, absolute CC segment size was smaller or equal and in females with tinnitus, it was larger or equal to what it was in controls. In males, smaller mHG size seems to be the predominant factor, while in females both factors seem to be involved. However, the tinnitus-related increase of normalized CC segment size in males shows that the reduction in the absolute size of CC segments was not proportionate to the mHG size reduction. Thus, both in male and female tinnitus patients certain segments of the CC are disproportionately large relative to the auditory cortex and may, therefore, be hypothesized to provide an enhanced connection between the hemispheres, possibly including a wider spatial range of convergent and divergent fiber projections.
The surface area of the midsagittal CC may mirror the number of axons interconnecting the two hemispheres (Aboitiz et al., 1992a) or a decrease in fiber density resulting from an increase in the diameter of or the distance between fibers (LaMantia and Rakic, 1990). Be that as it may, in either case the callosal transmission of information, of functionally excitatory and inhibitory influences, would be facilitated. Functionally, callosal projections are both excitatory and inhibitory (Bloom and Hynd, 2005; Karayannis et al., 2007; Tang et al., 2007). Findings in patients that underwent callosotomy as a treatment of otherwise intractable epilepsy suggest that the excitatory influence is the dominant one. Section of the CC disrupts the spread of seizures between the hemispheres (Ojemann, 1987). Although cases of a disruption of seizure inhibition by callosotomy have also been reported (Spencer et al., 1984; Ferbert et al., 1992; Netz et al., 1995), this effect is not as profound as the disruption of the interhemispheric spread of seizures (Bloom and Hynd, 2005). Assuming that it is hypersynchronous firing of neurons in the auditory cortex that represents the neurophysiological correlate of tinnitus (Eggermont and Roberts, 2004; Eggermont, 2007) and that the default transcallosal influence is mainly excitatory, the CC may well facilitate the development and maintenance of tinnitus. The synchronous activity of a population of single units in one hemisphere may be facilitated by the excitatory transcallosal inputs it receives and may in turn facilitate the emergence of one or more descendant synchronized populations via its callosal projection onto the auditory cortex of the respective contralateral hemisphere. And this maladaptive facilitatory function would necessarily be enhanced in subjects whose CC were disproportionally large and thus provided a particularly strong interhemispheric connection with a wider spatial range of convergent and divergent projections. Even if a putative tinnitus generator was originally restricted to one hemisphere, the establishment over time of such a positive feedback loop may facilitate the emergence of one or more mirror generators on the respective contralateral side. While most patients (85.0%) included in the dataset of the Oregon tinnitus archive (http://www.tinnitusarchive.org/) did not remember any kind of change in perceived tinnitus localization since the onset of their tinnitus, it is fitting that the by far most frequent kind of change (10.9%) was “Started on one side, now on both.” This percentage is not small given that the majority of answers indicated a bilateral tinnitus location (“both ears”: 63.0%, “fills head”: 11.4%). Unfortunately, there is little information on whether and how the location of the hearing impairment and the duration of the tinnitus since its onset and the interaction of these factors affect the distribution of perceived tinnitus location.
The anterior CC carries premotor, supplementary motor, and prefrontal fiber projections (Witelson, 1989; Hofer and Frahm, 2006). As a result of training and practice, it is larger in musicians than in non-musicians (Schlaug et al., 1995, 2009). The finding in the present study of positive correlations in both male and female musicians with tinnitus between some of the indicators of hearing loss and anterior CC surface area may be taken to suggest that, in musicians, hearing loss may lead to an increase in anterior CC size. A potentially related positive correlation, between postero-medial left hemisphere mHG volume and right ear hearing loss, was found in musicians with tinnitus by Schneider et al. (2009). These findings invite some more speculative considerations. In the present study, while musicians with tinnitus did not differ in the tinnitus minimum masking level from non-musicians without tinnitus, they earned significantly lower scores on the tinnitus questionnaire and its subscales. This may indicate that musical training and practice may serve a protective function. The hypothetical mechanism underlying a putative protective function may relate to the fact that the playing of an instrument generates regularly patterned and correlated reafferent input streams to the auditory and the somatosensory system. There are multiple somatosensory entries into the auditory system that are capable of modulating auditory cortex activity (Schroeder et al., 2001; Lakatos et al., 2007). Increase of auditory cortex size and enhancement of fiber projections through the anterior CC may represent components of a protective auditory-motor-somatosensory-auditory loop.
The second hypothesis investigated, that tinnitus patients, on the average, exhibit a larger degree of interhemispheric mHG asymmetry than healthy controls and that mHG asymmetry is negatively correlated with the size of the posterior CC segments that transmit auditory information, was rejected by the data of this study. The segmental location of the maximum mHG asymmetry differed between tinnitus patients and healthy controls. For patients, the location of the mHG asymmetry maximum was extremely medial, for controls, it was extremely lateral. For males, but not females, the maximum medial asymmetry was larger for patients than for controls. However, it was the lateral, not the medial maximum asymmetry that was correlated negatively with the surface area of the posterior midbody segment of the CC, and this correlation was hardly at all affected by tinnitus status.
Conflict of Interest Statement
The authors declare that the research was conducted in the absence of any commercial or financial relationships that could be construed as a potential conflict of interest.
Acknowledgments
Parts of this research were supported by a grant of the Deutsche Forschungsgemeinschaft to E. Diesch and A. Rupp (Di497/3-2). We would like to thank the reviewers of an earlier version of this paper for their insightful and constructive comments. Please address correspondence to Eugen Diesch. (e-mail:ZXVnZW4uZGllc2NoQHppLW1hbm5oZWltLmRl).
Footnotes
- ^The data of seven of the 106 subjects that were examined in the Schneider et al. (2009) study could not be retrieved from backup media.
References
Aboitiz, F., Scheibel, A. B., Fisher, R. S., and Zaidel, E. (1992a). Fiber composition of the human corpus callosum. Brain Res. 598, 143–153.
Aboitiz, F., Scheibel, A. B., Fisher, R. S., and Zaidel, E. (1992b). Individual differences in brain asymmetries and fiber composition in the human corpus callosum. Brain Res. 598, 154–161.
Aboitiz, F., Scheibel, A. B., and Zaidel, E. (1992c). Morphometry of the Sylvian fissure and the corpus callosum, with emphasis on sex differences. Brain 115(Pt 5), 1521–1541.
Baguley, D. M., and McFerran, D. J. (2002). Current perspectives on tinnitus. Arch. Dis. Child 86, 141–143.
Bamiou, D. E., Sisodiya, S., Musiek, F. E., and Luxon, L. M. (2007). The role of the interhemispheric pathway in hearing. Brain Res. Rev. 56, 170–182.
Bishop, K. M., and Wahlsten, D. (1997). Sex differences in the human corpus callosum: myth or reality? Neurosci. Biobehav. Rev. 21, 581–601.
Bloom, J. S., and Hynd, G. W. (2005). The role of the corpus callosum in interhemispheric transfer of information: excitation or inhibition? Neuropsychol. Rev. 15, 59–71.
Chance, S. A., Casanova, M. F., Switala, A. E., and Crow, T. J. (2006). Minicolumnar structure in Heschl's gyrus and planum temporale: asymmetries in relation to sex and callosal fiber number. Neuroscience 143, 1041–1050.
Cipolloni, P. B., and Pandya, D. N. (1985). Topography and trajectories of commissural fibers of the superior temporal region in the rhesus monkey. Exp. Brain Res. 57, 381–389.
Diesch, E., Struve, M., Rupp, A., Ritter, S., Hulse, M., and Flor, H. (2004). Enhancement of steady-state auditory evoked magnetic fields in tinnitus. Eur. J. Neurosci. 19, 1093–1104.
Dorion, A. A., Chantome, M., Hasboun, D., Zouaoui, A., Marsault, C., Capron, C., and Duyme, M. (2000). Hemispheric asymmetry and corpus callosum morphometry: a magnetic resonance imaging study. Neurosci. Res. 36, 9–13.
Eggermont, J. J. (2007). Correlated neural activity as the driving force for functional changes in auditory cortex. Hear. Res. 229, 69–80.
Eggermont, J. J., and Roberts, L. E. (2004). The neuroscience of tinnitus. Trends Neurosci. 27, 676–682.
Feldmann, H. (1998). Tinnitus: Grundlagen einer rationalen Diagnostk und Therapie, 2. Auflage. Stuttgart, New York, NY: Thieme.
Ferbert, A., Priori, A., Rothwell, J. C., Day, B. L., Colebatch, J. G., and Marsden, C. D. (1992). Interhemispheric inhibition of the human motor cortex. J. Physiol. 453, 525–546.
Fujimoto, C., Ito, K., Iwasaki, S., Nakao, K., and Sugasawa, M. (2006). Reversible impairment of auditory callosal pathway in 5-fluorouracil-induced leukoencephalopathy: parallel changes in function and imaging. Otol. Neurotol. 27, 716–719.
Galaburda, A. M., Rosen, G. D., and Sherman, G. F. (1990). Individual variability in cortical organization: its relationship to brain laterality and implications to function. Neuropsychologia 28, 529–546.
Goebel, G., and Hiller, W. (1994). The tinnitus questionnaire. A standard instrument for grading the degree of tinnitus. Results of a multicenter study with the tinnitus questionnaire. HNO 42, 166–172.
Hallam, R. S., Jakes, S. C., and Hinchcliffe, R. (1988). Cognitive variables in tinnitus annoyance. Br. J. Clin. Psychol. 27(Pt 3), 213–222.
Hazell, J. (1990). Tinnitus and disability with ageing: adaptation and management. Acta Otolaryngol. Suppl. 476, 202–208.
Henry, J. A., Meikle, M., and Gilbert, A. (1999). “Audiometric correlates of tinnitus pitch: insights from the Tinnitus Data Registry,” in Proceedings of the Sixth International Tinnitus Seminar, ed J. W. P. Hazell (London: The Tinnitus and Hyperacusis Centre), 51–57.
Hofer, S., and Frahm, J. (2006). Topography of the human corpus callosum revisited–comprehensive fiber tractography using diffusion tensor magnetic resonance imaging. Neuroimage 32, 989–994.
Jancke, L., Staiger, J. F., Schlaug, G., Huang, Y., and Steinmetz, H. (1997). The relationship between corpus callosum size and forebrain volume. Cereb. Cortex 7, 48–56.
Karayannis, T., Huerta-Ocampo, I., and Capogna, M. (2007). GABAergic and pyramidal neurons of deep cortical layers directly receive and differently integrate callosal input. Cereb. Cortex 17, 1213–1226.
Lakatos, P., Chen, C. M., O'Connell, M. N., Mills, A., and Schroeder, C. E. (2007). Neuronal oscillations and multisensory interaction in primary auditory cortex. Neuron 53, 279–292.
LaMantia, A. S., and Rakic, P. (1990). Cytological and quantitative characteristics of four cerebral commissures in the rhesus monkey. J. Comp Neurol. 291, 520–537.
Landgrebe, M., Langguth, B., Rosengarth, K., Braun, S., Koch, A., Kleinjung, T., May, A., De Ridder, R. D., and Hajak, G. (2009). Structural brain changes in tinnitus: grey matter decrease in auditory and non-auditory brain areas. Neuroimage 46, 213–218.
Luders, E., Narr, K. L., Zaidel, E., Thompson, P. M., and Toga, A. W. (2006). Gender effects on callosal thickness in scaled and unscaled space. Neuroreport 17, 1103–1106.
Luders, E., Rex, D. E., Narr, K. L., Woods, R. P., Jancke, L., Thompson, P. M., Mazziotta, J. C., and Toga, A. W. (2003). Relationships between sulcal asymmetries and corpus callosum size: gender and handedness effects. Cereb. Cortex 13, 1084–1093.
Mitchell, T. N., Free, S. L., Merschhemke, M., Lemieux, L., Sisodiya, S. M., and Shorvon, S. D. (2003). Reliable callosal measurement: population normative data confirm sex-related differences. AJNR Am. J. Neuroradiol. 24, 410–418.
Mühlau, M., Rauschecker, J. P., Oestreicher, E., Gaser, C., Röttinger, M., Wohlschläger, A. M., Simon, F., Etgen, T., Conrad, B., and Sander, D. (2006). Structural brain changes in tinnitus. Cereb. Cortex 16, 1283–1288.
Mühlnickel, W., Elbert, T., Taub, E., and Flor, H. (1998). Reorganization of auditory cortex in tinnitus. Proc. Natl. Acad. Sci. U.S.A. 95, 10340–10343.
Netz, J., Ziemann, U., and Homberg, V. (1995). Hemispheric asymmetry of transcallosal inhibition in man. Exp. Brain Res. 104, 527–533.
Norena, A. J. (2011). An integrative model of tinnitus based on a central gain controlling neural sensitivity. Neurosci. Biobehav. Rev. 35, 1089–1109.
Ojemann, G. A. (1987). Surgical therapy for medically intractable epilepsy. J. Neurosurg. 66, 489–499.
Oka, S., Miyamoto, O., Janjua, N. A., Honjo-Fujiwara, N., Ohkawa, M., Nagao, S., Kondo, H., Minami, T., Toyoshima, T., and Itano, T. (1999). Re-evaluation of sexual dimorphism in human corpus callosum. Neuroreport 10, 937–940.
Parra, L. C., and Pearlmutter, B. A. (2007). Illusory percepts from auditory adaptation. J. Acoust. Soc. Am. 121, 1632–1641.
Pollmann, S., Maertens, M., Von Cramon, D. Y., Lepsien, J., and Hugdahl, K. (2002). Dichotic listening in patients with splenial and nonsplenial callosal lesions. Neuropsychology 16, 56–64.
Rosen, G. D., Sherman, G. F., and Galaburda, A. M. (1989). Interhemispheric connections differ between symmetrical and asymmetrical brain regions. Neuroscience 33, 525–533.
Salvi, R. J., Wang, J., and Ding, D. (2000). Auditory plasticity and hyperactivity following cochlear damage. Hear. Res. 147, 261–274.
Schlaug, G., Forgeard, M., Zhu, L., Norton, A., Norton, A., and Winner, E. (2009). Training-induced neuroplasticity in young children. Ann. N.Y. Acad. Sci. 1169, 205–208.
Schlaug, G., Jancke, L., Huang, Y., and Steinmetz, H. (1995). In vivo evidence of structural brain asymmetry in musicians. Science 267, 699–701.
Schneider, P., Andermann, M., Wengenroth, M., Goebel, R., Flor, H., Rupp, A., and Diesch, E. (2009). Reduced volume of Heschl's gyrus in tinnitus. Neuroimage 45, 927–939.
Schroeder, C. E., Lindsley, R. W., Specht, C., Marcovici, A., Smiley, J. F., and Javitt, D. C. (2001). Somatosensory input to auditory association cortex in the macaque monkey. J. Neurophysiol. 85, 1322–1327.
Shargorodsky, J., Curhan, G. C., and Farwell, W. R. (2010). Prevalence and characteristics of tinnitus among US adults. Am. J. Med. 123, 711–718.
Shattuck, D. W., and Leahy, R. M. (2002). BrainSuite: an automated cortical surface identification tool. Med. Image Anal. 6, 129–142.
Sindhusake, D., Golding, M., Newall, P., Rubin, G., Jakobsen, K., and Mitchell, P. (2003). Risk factors for tinnitus in a population of older adults: the blue mountains hearing study. Ear Hear. 24, 501–507.
Spencer, S. S., Spencer, D. D., Glaser, G. H., Williamson, P. D., and Mattson, R. H. (1984). More intense focal seizure types after callosal section: the role of inhibition. Ann. Neurol. 16, 686–693.
Sugishita, M., Otomo, K., Yamazaki, K., Shimizu, H., Yoshioka, M., and Shinohara, A. (1995). Dichotic listening in patients with partial section of the corpus callosum. Brain 118(Pt 2), 417–427.
Sun, W., Lu, J., Stolzberg, D., Gray, L., Deng, A., Lobarinas, E., and Salvi, R. J. (2009). Salicylate increases the gain of the central auditory system. Neuroscience 159, 325–334.
Syka, J. (2002). Plastic changes in the central auditory system after hearing loss, restoration of function, and during learning. Physiol. Rev. 82, 601–636.
Tang, J., Xiao, Z., and Suga, N. (2007). Bilateral cortical interaction: modulation of delay-tuned neurons in the contralateral auditory cortex. J. Neurosci. 27, 8405–8413.
Wienbruch, C., Paul, I., Weisz, N., Elbert, T., and Roberts, L. E. (2006). Frequency organization of the 40-Hz auditory steady-state response in normal hearing and in tinnitus. Neuroimage 33, 180–194.
Witelson, S. F. (1989). Hand and sex differences in the isthmus and genu of the human corpus callosum. A postmortem morphological study. Brain 112(Pt 3), 799–835.
Keywords: tinnitus, auditory cortex, corpus callosum, MRI volumetry
Citation: Diesch E, Schummer V, Kramer M and Rupp A (2012) Structural changes of the corpus callosum in tinnitus. Front. Syst. Neurosci. 6:17. doi: 10.3389/fnsys.2012.00017
Received: 27 December 2011; Accepted: 05 March 2012;
Published online: 26 March 2012.
Edited by:
Jos J. Eggermont, University of Calgary, CanadaReviewed by:
David C. Lyon, University of California, USAFatima T. Husain, University of Illinois at Urbana-Champaign, USA
Copyright: © 2012 Diesch, Schummer, Kramer and Rupp. This is an open-access article distributed under the terms of the Creative Commons Attribution Non Commercial License, which permits non-commercial use, distribution, and reproduction in other forums, provided the original authors and source are credited.
*Correspondence: Eugen Diesch, Department of Clinical and Cognitive Neuroscience, Central Institute of Mental Health, Medical Faculty Mannheim, Heidelberg University, Square J5, D-68159 Mannheim, Germany. e-mail:ZXVnZW4uZGllc2NoQHppLW1hbm5oZWltLmRl