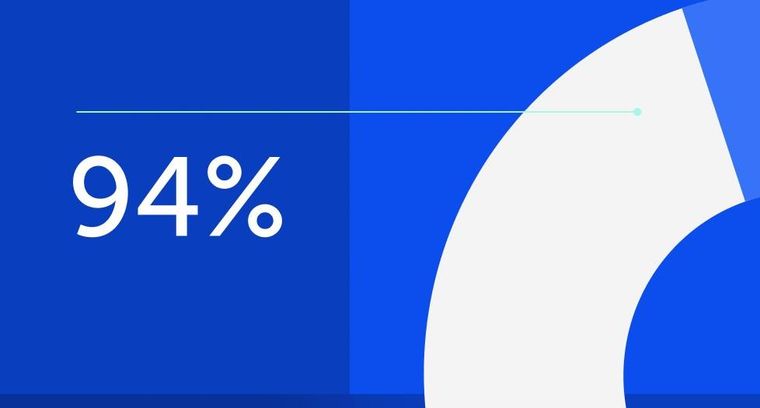
94% of researchers rate our articles as excellent or good
Learn more about the work of our research integrity team to safeguard the quality of each article we publish.
Find out more
EDITORIAL article
Front. Synaptic Neurosci., 13 September 2023
Volume 15 - 2023 | https://doi.org/10.3389/fnsyn.2023.1270701
This article is part of the Research TopicNew Insights Into Synaptic Plasticity in Fear ConditioningView all 5 articles
Editorial on the Research Topic
New insights into synaptic plasticity in fear conditioning
The fear conditioning task in rodents serves as a valuable tool for studying learning and memory, as well as enhancing our understanding of post-traumatic stress disorder (PTSD) and other stress-related disorders. By utilizing controlled experimental paradigms, traumatic experiences can be mimicked in rodents, allowing investigation of underlying neurobiological mechanisms. During the fear conditioning task, animals learn to associate a neutral stimulus, such as a tone or a context, with an aversive, unconditioned stimulus, most often a mild foot shock. Then, the previously neutral stimulus becomes conditioned stimulus, and when the animals are re-exposed to it, they show fear response. The fear response is most commonly measured as the percentage of time spent freezing, which is defined as the time the animal spends motionless, except for cardiac and respiratory movement.
While several brain regions are involved in processing and storing of these associative episodic-like memories, the spatial attributes rely primarily on the hippocampus, while emotional valence depends mostly on the amygdala (Maren, 2001, 2008; Josselyn, 2010; Izquierdo et al., 2016). Understanding how these two hub regions are regulated, and function in coordination with other brain regions, and how stress hormones and synaptic mechanisms influence brain circuits involved in fear and emotional processing, may potentially bring new insights for treating maladaptive memories. The present Research Topic encompasses diverse studies examining brain regions recruited for fear and emotional processing on molecular, circuit and behavioral level.
Hippocampal formation and entorhinal cortex show a high degree of interconnectivity and functional interaction between the hippocampus and MEC is crucial for spatial memory processing (Hafting et al., 2005; Sanders et al., 2015). Hartner and Schrader's article explores the effects of stress hormones, norepinephrine (NE), and glucocorticoids on inhibitory signaling in the Layer 2 of medial entorhinal cortex (MEC_LII) circuitry. The authors show the ability of NE to increase the frequency and amplitude of inhibitory inputs to the MEC, an effect that is cell-specific, mediated by the activation α1 adrenergic receptors, and modulated by co-administration of corticosteroids. This study sheds light on how stress influences underlying mechanisms of fear memory formation by demonstrating the ability of stress hormones to alter inhibitory synaptic inputs within the MEC-LII.
Asede et al. focus on the role of ErbB4, a receptor for neuregulin that is exclusively expressed in GABAergic neurons of the central nervous system, in intercalated cell clusters (ITCs) of the amygdala. As the amygdala plays a crucial role in fear conditioning (LeDoux, 2000, 2007; Krabbe et al., 2018), understanding the synaptic mechanisms involved in its circuitry is essential. This study focused on the medial paracapsular ITC (mpITC) and its thalamic inputs. The authors provide insights into how the deletion of ErbB4 differentially affects inhibitory and excitatory circuits, thus disturbing the excitation-inhibition balance in the amygdala and how lack of ErbB4 compromises LTP of the thalamo-mpITC synapses, contributing to further understanding of the functional role ErbB4 has during fear conditioning.
Ferrara et al. scrutinize the two procedurally similar but distinct processes: memory extinction and memory interference during reconsolidation following fear conditioning. Extinction involves reducing fear responses through numerous repeated exposures to either conditioned stimulus (CS) or conditioning context on its own, without the previously predictive unconditioned stimulus, such as mild foot shock. This leads to the formation of a new, inhibitory memory (Quirk and Mueller, 2008; Pape and Pare, 2010). In contrast, interference during reconsolidation requires only a few CS presentations and modifies the original fear memory (Nader and Hardt, 2009; Lee et al., 2017). This review examines the behavioral and neurobiological mechanisms underlying these two processes, providing valuable insights into the boundary conditions of post-conditioning cue exposure.
Liu et al. investigate the neural circuitry involved in assigning emotional valence to stimuli. The central amygdala (CeA) and the ventral tegmental area (VTA) are key regions involved in emotional processing and assignment of valence (Namburi et al., 2016; Tye, 2018). The authors for the first time identified neural populations that project to both the CeA and the VTA in the posterior bed nucleus of the stria terminalis (pBNST), pedunculopontine tegmental nucleus (PPTg), and the anterior part of the basomedial amygdala (BMA). The study shows that the positive valence is induced by activating pBNST neurons, while PPTg activation produces a negative valence, and valence processing is not influenced by BMA activation. These findings contribute to our understanding of the neural mechanisms underlying emotional regulation and point to possible important targets for mood disorder treatment.
The aim of this Research Topic is to offer New Insights into Synaptic Plasticity in Fear Conditioning. While previous studies on fear conditioning have mostly focused on examining the triad of brain regions crucial for fear conditioning -hippocampus, amygdala, and medial prefrontal cortex-, the articles in this Research Topic broaden the scope by investigating circuits and molecular mechanisms of other brain regions that play important role in fear conditioning and emotion processing. Additionally, this Research Topic is putting a spotlight on the inhibitory transmission, and the inhibition/excitation balance. The inhibitory transmission has been historically understudied. Albeit interneurons are fewer in number in areas crucial for fear conditioning they are not passive gain regulators but exert their robust inhibition via intricate network of connections on the excitatory projection neurons, thus shaping the network activity (Lucas and Clem, 2018). Hence, with this Research Topic we hope to highlight the complex interplay between brain circuits involved in fear and emotional processing and provide a better understanding of the neurobiological mechanisms that potentially support stress-related disorders.
ACr: Writing—original draft. ACi: Writing—review and editing. AY: Writing—original draft.
The authors declare that the research was conducted in the absence of any commercial or financial relationships that could be construed as a potential conflict of interest.
All claims expressed in this article are solely those of the authors and do not necessarily represent those of their affiliated organizations, or those of the publisher, the editors and the reviewers. Any product that may be evaluated in this article, or claim that may be made by its manufacturer, is not guaranteed or endorsed by the publisher.
Hafting, T., Fyhn, M., Molden, S., Moser, M. B., and Moser, E. I. (2005). Microstructure of a spatial map in the entorhinal cortex. Nature. 436, 801–806. doi: 10.1038/nature03721
Izquierdo, I., Furini, C. R., and Myskiw, J. C. (2016). Fear memory. Physiol. Rev. 96, 695–750. doi: 10.1152/physrev.00018.2015
Josselyn, S. A. (2010). Continuing the search for the engram: examining the mechanism of fear memories. J. Psychiat. Neurosci. 35, 221–228. doi: 10.1503/jpn.100015
Krabbe, S., Gründemann, J., and Lüthi, A. (2018). Amygdala inhibitory circuits regulate associative fear conditioning. Biol. Psychiatry. 83, 800–809. doi: 10.1016/j.biopsych.2017.10.006
LeDoux, J. E. (2000). Emotion circuits in the brain. Annu. Rev. Neurosci. 23, 155–184. doi: 10.1146/annurev.neuro.23.1.155
Lee, J. L. C., Nader, K., and Schiller, D. (2017). An update on memory reconsolidation updating. Trends Cogn. Sci. 21, 531–545. doi: 10.1016/j.tics.2017.04.006
Lucas, E. K., and Clem, R. L. (2018). GABAergic interneurons: The orchestra or the conductor in fear learning and memory? Brain Res. Bull. 141:13–19. doi: 10.1016/j.brainresbull.2017.11.016
Maren, S. (2001). Neurobiology of pavlovian fear conditioning. Annu. Rev. Neurosci. 24:897–931. doi: 10.1146/annurev.neuro.24.1.897
Maren, S. (2008). Pavlovian fear conditioning as a behavioral assay for hippocampus and amygdala function: cautions and caveats. Eur. J. Neurosci. 28, 1661–1666. doi: 10.1111/j.1460-9568.2008.06485.x
Nader, K., and Hardt, O. (2009). A single standard for memory: the case for reconsolidation. Nat. Rev. Neurosci. 10, 224–234. doi: 10.1038/nrn2590
Namburi, P., Al-Hasani, R., Calhoon, G. G., Bruchas, M. R., and Tye, K. M. (2016). Architectural representation of valence in the limbic system. Neuropsychopharmacol. 41, 1697–1715. doi: 10.1038/npp.2015.358
Pape, H. C., and Pare, D. (2010). Plastic synaptic networks of the amygdala for the acquisition, expression, and extinction of conditioned fear. Physiol. Rev. 90, 419–63. doi: 10.1152/physrev.00037.2009
Quirk, G. J., and Mueller, D. (2008). Neural mechanisms of extinction learning and retrieval. Neuropsychopharmacol. 33, 56–72. doi: 10.1038/sj.npp.1301555
Sanders, H., Rennó-Costa, C., Idiart, M., and Lisman, J. (2015). Grid cells and place cells: an integrated view of their navigational and memory function. Trend. Neurosci. 38, 763–775. doi: 10.1016/j.tins.2015.10.004
Keywords: fear conditioning, memory extinction, hippocampal formation/hippocampus, amygdala, ventral tegmental area (VTA)
Citation: Crestani AP, Cicvaric A and Yiu AP (2023) Editorial: New insights into synaptic plasticity in fear conditioning. Front. Synaptic Neurosci. 15:1270701. doi: 10.3389/fnsyn.2023.1270701
Received: 01 August 2023; Accepted: 30 August 2023;
Published: 13 September 2023.
Edited by:
Clive R. Bramham, University of Bergen, NorwayReviewed by:
José M. Delgado-García, Universidad Pablo de Olavide, SpainCopyright © 2023 Crestani, Cicvaric and Yiu. This is an open-access article distributed under the terms of the Creative Commons Attribution License (CC BY). The use, distribution or reproduction in other forums is permitted, provided the original author(s) and the copyright owner(s) are credited and that the original publication in this journal is cited, in accordance with accepted academic practice. No use, distribution or reproduction is permitted which does not comply with these terms.
*Correspondence: Ana P. Crestani, cGF1bGEuY3Jlc3RhbmlAZ21haWwuY29t; Ana Cicvaric, YW5hLmNpY3ZhcmljQGVpbnN0ZWlubWVkLmVkdQ==
Disclaimer: All claims expressed in this article are solely those of the authors and do not necessarily represent those of their affiliated organizations, or those of the publisher, the editors and the reviewers. Any product that may be evaluated in this article or claim that may be made by its manufacturer is not guaranteed or endorsed by the publisher.
Research integrity at Frontiers
Learn more about the work of our research integrity team to safeguard the quality of each article we publish.