- 1Department of Otolaryngology, First Affiliated Hospital of Dalian Medical University, Dalian, China
- 2National Joint Engineering Laboratory, Stem Cell Clinical Research Center, Regenerative Medicine Center, First Affiliated Hospital of Dalian Medical University, Dalian, China
The GAIP interacting protein c terminus (GIPC) genes encode a small family of proteins characterized by centrally located PDZ domains. GIPC3 encodes a 312 amino acid protein. Variants of human GIPC3 are associated with non-syndromic hearing loss. GIPC3 is one of over a hundred different genes with variants causing human deafness. Screening for variants of GIPC3 is essential for early detection of hearing loss in children and eventually treatment of deafness. Accordingly, this paper assesses the status of research developments on the role of GIPC3 in hereditary deafness and the effects of pathogenic variants on the auditory system.
1. Introduction
Deafness is a major global public health issue and a common human disorder. According to the World Hearing Report, which was released by the World Health Organization (WHO), more than 1.5 billion people have varying degrees of hearing loss worldwide (Chadha et al., 2021; World Health Organization, 2021). Among these conditions of various degrees, sensorineural hearing loss (SNHL) is the most common congenital sensory disorder in children. The incidence of SNHL is one to two per thousand live births (van Beeck Calkoen et al., 2019), of which 70% are non-syndromic hearing loss (NSHL) (Sindura and Banerjee, 2019). Hereditary deafness is largely caused by gene mutations. More than 6,000 mutations in more than 150 genes have been causally linked to this condition, and GJB2, SLC26A4, TMC1, OTOF, and CDH23 mutations account for the majority of these mutations. As research has progressed, it has been discovered that mutations in GIPC3 play a significant role in the pathogenesis of various types of SNHL and are strongly associated with NSHL (Sloan-Heggen et al., 2016; Azaiez et al., 2018). However, many of the functions and pathogenic mechanisms of GIPC3 are still not fully elucidated. This review concludes the research developments on the involvement the GIPC3 gene in hereditary deafness and its effects on the auditory system.
2. Overview of the GIPC3 gene
2.1. Structure and function of the GIPC3 gene
GIPC3 is a member of the GIPC family. The GAIP interacting protein, c terminus (GIPC), which interacts with the G Alpha-interacting protein (GAIP), is involved in the G protein-coupled signaling complex that constitutes the regulation of vesicle transport (De Vries et al., 1998). Saitoh et al. (2002) isolated GIPC3 cDNAs from human fetal lung and discovered that GIPC3 encodes a protein consisting of 312 amino acids with a central PDZ structural domain. Additionally, it shared 59.9% of its total amino acids with GIPC1, 55.3% of its total amino acids with GIPC2. These findings indicate that GIPC3 is highly correlated with GIPC1 and GIPC2. The GIPC3 gene comprises six exons. It is located on chromosome 19 at region 19p13.3 and is associated with the thromboxane A2 receptor (TBXA2R) gene (Katoh, 2002). All GIPC family members contain the highly conserved GIPC homology 1 (GH1), GH2, and central PDZ domains, which interact with protein binding through their structural domains (Katoh, 2013).
2.2. Factors interacting with GIPC3
The GAIP interacting protein c terminus are widely involved in signal transduction, receptor and adhesion molecule transport, cell migration, and neurotransmitter release through protein interactions. They are also connected to numerous physiological and pathological processes in cells (Booth et al., 2002; Varsano et al., 2006; Yi et al., 2007; Prahst et al., 2008). The GH2 domain of GIPC directly interacts with the adaptor-binding domain 1 (ABD1) motif of myosin VI (MYO6) to oligomerize MYO6 and form the GIPC-MYO6 complex (Yu et al., 2009). Shang’s research analyzed the co-localization of Plexin D1 with GIPC1 and MYO6. It proved GIPC-mediated endocytosis and intracellular transport are important aspects of signal regulation of these receptors. Deafness caused by these mutations is therefore likely due to loss of the anchoring function of the GIPC3-MYO6 complex in stereocilia (Shang et al., 2017). The GIPC-MYO6 complex has been suggested to translocate early endosomes away from the plasma membrane through the cortical actin network and plays a pivotal role in the trafficking of transmembrane proteins on endocytic vesicles (Spudich et al., 2007; de Jonge et al., 2019). Myo6 is specifically expressed in mouse inner ear cochlear sensory hair cells, demonstrating that it is essential for hair cell formation and maintenance of their structural integrity (Avraham et al., 1995). Furthermore, it is required for effective Ca2+-dependent exocytosis in mature inner hair cell ribbon synapses (Roux et al., 2009). Myo6 and Gipc3 both have comparable localization and functions in mouse cochleae (Avraham et al., 1995; Charizopoulou et al., 2011). Therefore, this complex may be involved in signal transduction in the inner ear and may regulate Ca2+-dependent exocytosis at inner hair cell ribbon synapses (Roux et al., 2009). However, the exact mechanisms involved in this process are unclear. In addition, adaptor protein containing a PH domain and leucine zipper motif 1 (APPL1), which is a cytoplasmic signaling regulator, binds to the PDZ structural domain of GIPC3 protein and is crucial for GIPC3-mediated cell viability (Ding et al., 2017).
2.3. Localized expression study of GIPC3
By using Northern Blot analysis on human tissues, Saitoh et al. (2002) discovered that GIPC3 mRNA was almost ubiquitously expressed in normal adult and normal fetal tissues. GIPC3 mRNA was expressed at relatively higher level in the jejunum, followed by the lymph nodes, parietal lobes in brain, fetal spleen, and fetal thymus. However, GIPC3 expression was not detected in the human ear at that time. As research progressed, Charizopoulou et al. (2011) used immunofluorescence staining to show that Gipc3 was exclusively localized in hair cells and spiral ganglion neurons (SGNs) of the mouse cochlea. In particular, distinct punctate staining was observed at the base of inner hair cells (IHCs) and outer hair cells (OHCs), around the nucleus, and throughout the apical region of the hair cells. These findings also laid the foundation for the subsequent finding that GIPC3 mutations trigger hearing loss in human and mouse. Abundant staining was also detected in the cytoplasm of vestibular hair cells. However, there was no research in potential role of GIPC3 in genetic vestibular disorders.
3. Effects of GIPC3 mutations on the auditory system
3.1. Several common GIPC3 mutations that trigger hereditary non-syndromic deafness
GIPC3 is commonly expressed in adult tissues, and GIPC3 mutations have an impact on several auditory system components. In humans, GIPC3 mutations can lead to NSHL, a disorder in which hearing loss is caused by damage to the inner ear, the auditory nerve, or the auditory center of the brain. NSHL manifests only as abnormalities of the auditory system without lesions in other organs and systems. It demonstrates significant locus and allelic heterogeneity and is inherited in a variety of ways (Gao et al., 2017). For 80% of NSHL cases, the mode of inheritance is autosomal recessive. To better understand the specific locus of the GIPC3 mutations that cause NSHL, Chen et al. (1997) applied gene sequencing to map a new locus of autosomal recessive non-syndromic hearing loss (ARNSHL), and it mapped to DFNB15 on chromosome 19p. In a Dutch ARNSHL family, Charizopoulou et al. (2011) identified a nucleotide substitution in exon 6 that localized to DFNB95 on chromosome 19p by GIPC3 mutation analysis. Through sequencing analysis, a missense mutation in exon 5 located at DFNB15 on chromosome 19p was identified in an Indian ARNSHL family. Six missense mutations and one shift mutation in GIPC3, located at DFNB72 on chromosome 19p, were found in seven Pakistani families with varying degrees of sensorineural deafness (Ain et al., 2007; Rehman et al., 2011). In summary, GIPC3 mutations have been shown to underlie NSHL associated with DFNB15, DFNB72 and DFNB95. In NSHL, biallelic, nonsense, and shift mutations were also observed in the GIPC3 gene (Chen et al., 1997; Ain et al., 2007; Charizopoulou et al., 2011; Rehman et al., 2011; Diaz-Horta et al., 2012; Sirmaci et al., 2012; Ramzan et al., 2013; Petrova et al., 2021), and more GIPC3 mutation loci are gradually being reported as research progresses (Siddiqi et al., 2014; Ammar-Khodja et al., 2015; Asgharzade et al., 2018; Khan et al., 2019; Bitarafan et al., 2020; Kannan-Sundhari et al., 2020; Zhou et al., 2020; Table 1).
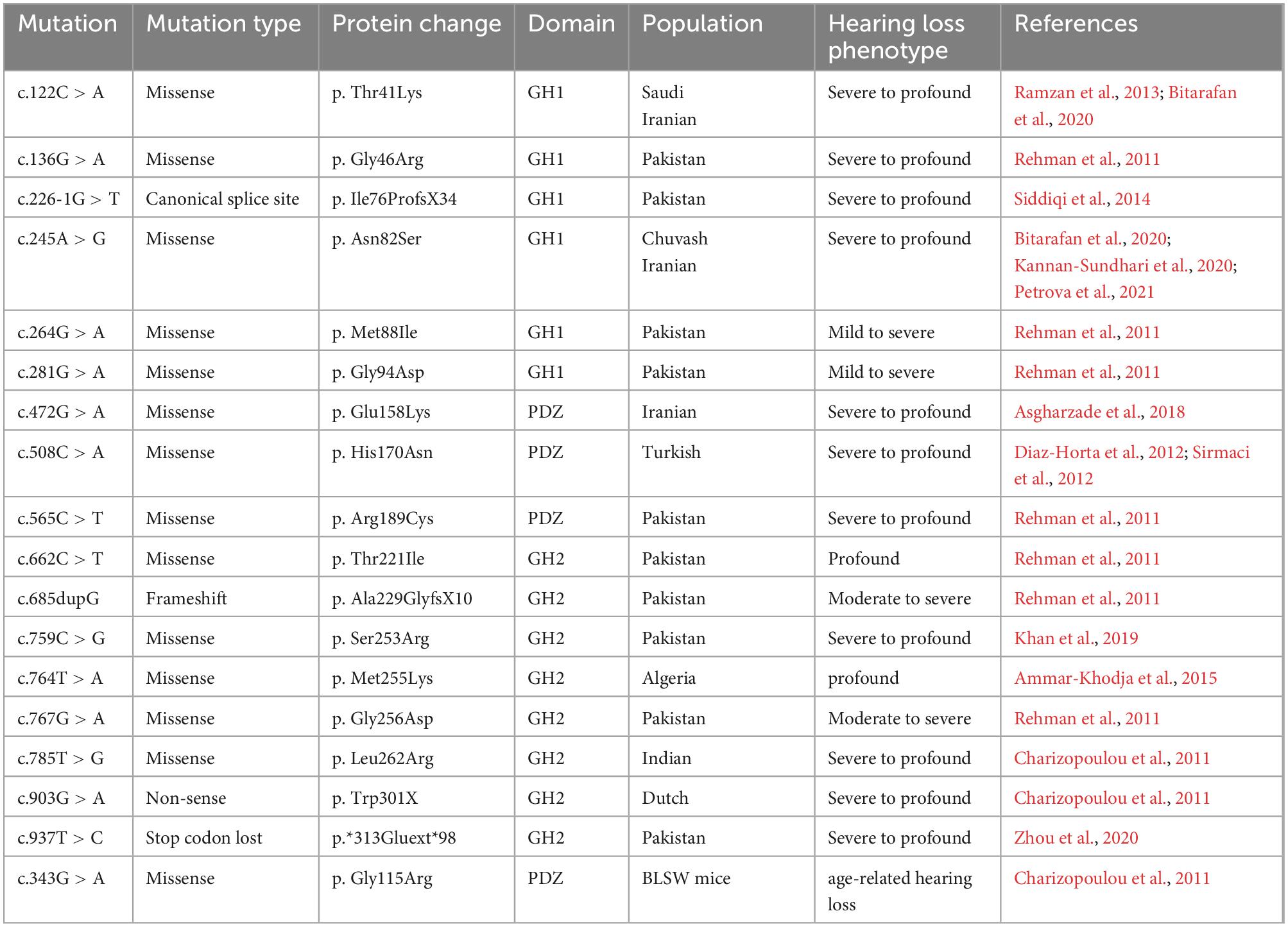
Table 1. Summaries GIPC3 mutations associated with autosomal recessive non-syndromic hearing impairment.
3.2. Effect of Gipc3 mutations on mechanical conduction in the inner ear
Mechanical conduction and electrical signal conduction coexist in the inner ear, and the integrity of both is critical to sound perception. Each part of the cochlea is involved in this process. Mechanical conduction in the peripheral auditory system depends on the movement of hair cells and their stereocilia. Sound transmission into the inner ear causes vibrations in the basilar membrane, which causes shearing movements in the reticular layer of the organ of Corti and the tectorial membrane. This progress causes the bending of the OHC stereocilia and opening of the ion channels. This induces the amplification of sound-evoked vibrations that reach the cochlea and are transmitted to the IHC. These effects further give rise to neurotransmitter release, generating electrical signals that pass from the SGNs to the superior olivary nucleus complex. Then, the signals are transmitted to the lateral thalamus and inferior colliculus. Finally, they are passed through the medial geniculate afferent fibers, terminating in the auditory cortex of the brain to generate auditory sensation (Ren, 2005).
Charizopoulou et al. (2011) identified that the progressive sensorineural deafness (age-related hearing loss 5, ahl5) and audiogenic seizures (juvenile audiogenic monogenic seizure 1, jams1) that develop in Black Swiss (BLSW) mice were associated with 343G > A mutation in the PDZ structural domain of Gipc3. They also discovered that, in contrast to C3HeB/FeJ mice, BLSW mice prematurely exhibited OHC and IHC stereocilia bundle defects, such as stereocilia sparseness, stereocilia disorder, and impaired maturation. Additionally, with age progression, there was marked degeneration of the organ of Corti, a loss of SGNs, and reduced efficiency in OHC function, as revealed by distortion product otoacoustic emissions (DPOAEs) and lower levels of Gipc3 protein expression in afferent neurons of hair cells and SGNs. Thus, Gipc3 is necessary for postnatal stereocilia maturation and the long-term survival of hair cells and SGNs. When Gipc3 is mutated, it causes mechanical conduction disorders in the inner ear (Figure 1).
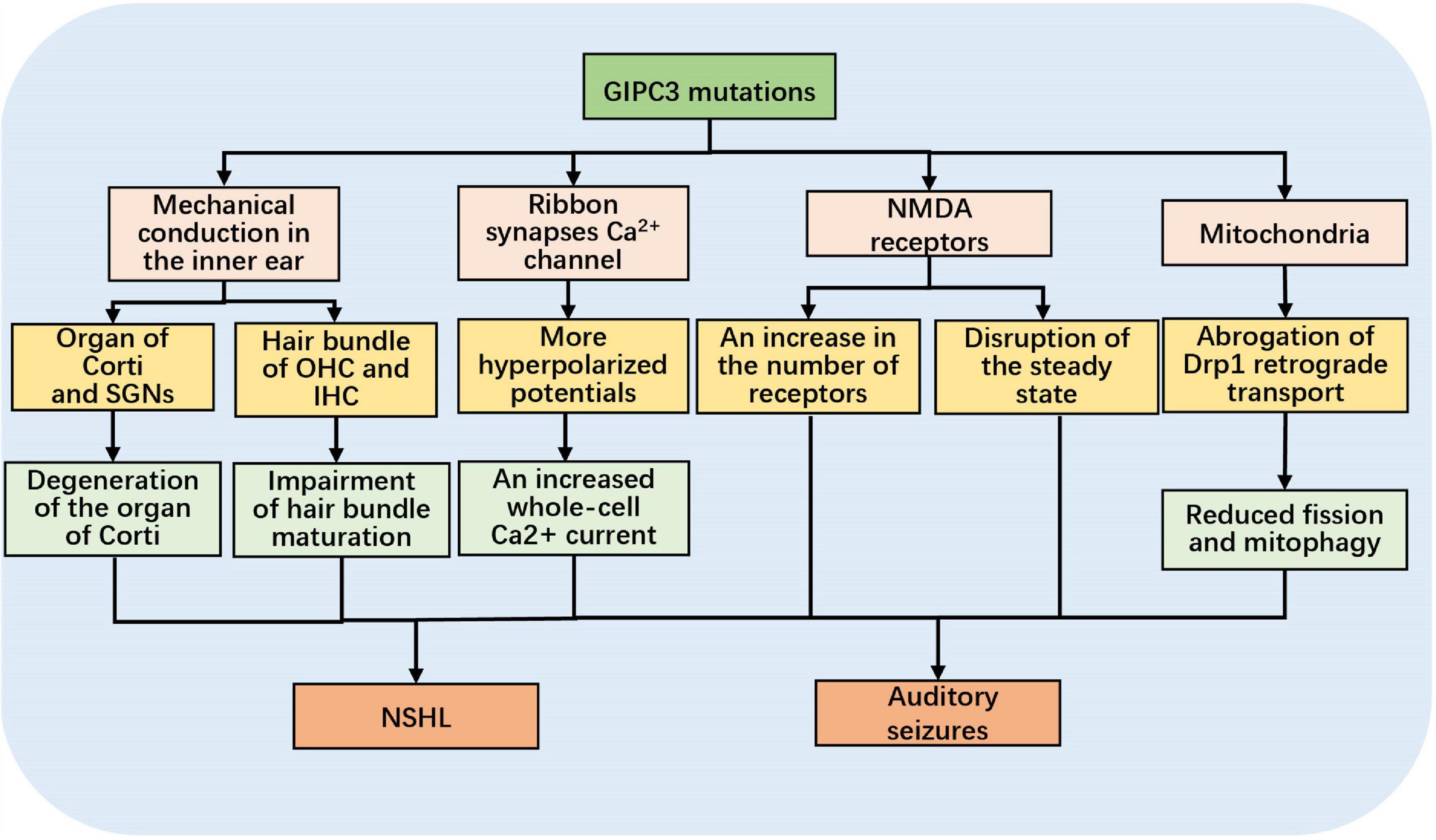
Figure 1. Schematic diagram of the mechanisms of hearing loss and acoustic seizures triggered by GIPC3 mutations.
3.3. Effect of Gipc3 mutations on Ca2+ influx and spiral ganglion cells
Sound signals are converted into electrical signals in hair cells, which are transmitted via auditory nerve fibers to spiral ganglion cells and then to all levels of centers. The process of mechanical-electrical conversion in hair cells is achieved via synapses. The ribbon synapse serves as the critical location for exocytosis; the neurotransmitter glutamate is released in response to mechanical stimuli. In contrast, transmitter release from cochlear hair cells is triggered by Ca2+ influx through the dihydropyridine-sensitive L-type voltage-gated calcium channel (VGCC) (Fuchs et al., 2003; Frank et al., 2009). Inner hair cell ribbon synapses contain a host of active zones (AZs), and each AZ houses multiple Ca2+ channels. Ohn et al. (2016) found that the number of Ca2+ channels varied among AZs and that larger, more complicated AZs had more Ca2+ channels, creating a modiolar-pillar gradient at the inner hair cell ribbon synapses. On the side near the SGNs, the larger AZ has more Ca2+ channels and is able to associate with SGNs with lower spontaneous firing frequencies and higher auditory nerve firing thresholds. Conversely, on the side away from the SGNs, AZs can be associated with SGNs with higher spontaneous firing frequencies and lower auditory nerve firing thresholds. However, the voltage dependence of Ca2+ influx varied with AZ location, showing a gradient opposite to that of maximal Ca2+ influx. In the IHC of BLSW mice, disruption of Gipc3 reversed the modiolar-pillar gradient of maximal AZ Ca2+ influx, shifting the activation voltage of Ca2+ channels to a more hyperpolarized potential. This, in turn, caused an increase in IHC whole-cell Ca2+ influx and increased the proportion of SGNs with high spontaneous discharge rates (Figure 1). For this reason, Gipc3 mutations may therefore affect the hyperpolarization potential of Ca2+ channels and alter AZ gradients.
3.4. Association between Gipc3 mutations and auditory seizures
Audiogenic seizures are a panic episode brought on by sudden high-intensity noise. Auditory seizures in BLSW mice are characterized by uncontrolled running, loss of the righting reflex, and tonic flexion and extension. This seizure susceptibility is developmentally regulated. It peaks at 2–3 weeks of age and almost completely vanishes by adulthood (Misawa et al., 2002). Charizopoulou et al. (2011) found that BLSW mice were most susceptible to seizures at 2–4 weeks of age, and the latency for auditory seizures was significantly shorter than that of C3HeB/FeJ mice in the control group. The seizure latency increased significantly between 3 and 5 weeks of age. However, full tolerance occurred at 6 weeks of age, with no significant difference in latency duration compared to controls. The ABR I-wave amplitude represents the total activity of cochlear nerve fibers projecting from the IHC to the cochlear nucleus. The ABR I-wave amplitude was significantly higher in 2-week-old BLSW mice than in control C3HeB/FEJ mice under 100 dB SPL sound stimulation and that these high amplitudes decreased rapidly at 7 weeks of age. A similar pattern of changes in ABR wave amplitude and seizure latency were also observed. Therefore, it was concluded that the ABR I-wave amplitude decreased after a significant increase, which was associated with epilepsy susceptibility and resistance, respectively. Additionally, the quantitative trait locus (QTL) of jams1 was determined to be located within the critical interval of the QTL of ah15. Furthermore, the Gipc3 transgene could compensate for the hearing defect caused by ah15 in Gipc3343A/A homozygotes and attenuate the susceptibility to auditory convulsions in jams1 by prolonging the latency of auditory seizures. Therefore, there is a direct link between mutations in Gipc3 and the onset and development of auditory seizures in mouse.
There is a lack of relevant reports on the mechanism of acoustic seizure in Gipc3 mutant mice, previous researches hypothesized there could be two causes: 1. cochlear nerve fibers became high spontaneous discharge due to an increase in IHC whole-cell Ca2 + influx (see part 3.3). 2 imbalance of NMDA receptors caused by Gipc3 mutation: In the cochlea, glutamate receptors mediate rapid IHC-related synaptic transmission and glutamate excitotoxicity, which is the key point of nerve damage during auditory trauma (Puel, 1995). Two types of glutamate receptors have been confirmed in the auditory transmission pathway, ionotropic receptors, known as AMPA receptors, and metabotropic receptors, known as NMDA receptors. NMDA receptors are found in the majority of glutamatergic synapses in the mammalian central nervous system and are essential for synaptic transmission, synaptic plasticity, and synaptic development. It has been shown that GIPC is widely expressed in neurons, both pre- and postsynapses (Jeanneteau et al., 2004). GIPC binds to NMDA receptors and modifies the amount of NMDA receptors. According to research by Yi et al. (2007) GIPC also acts as an antagonist, preventing NMDA receptors from interacting with other PDZ proteins. A crucial mechanism for modulating glutamate receptor function is controlling the amount of cell surface receptors. As a member of the GIPC family, Gipc3 mutations may result in an increase in the number of NMDA receptors and disruption of the steady state, both of which lead to auditory seizures in mouse (Figure 1). However, it is unknown why acoustic seizures are only seen in animals with the Gipc3 mutation and why they stop occurring at six weeks after birth.
3.5. Effect of GIPC3 mutations on mitophagy
Mitochondria are the primary site of energy production by aerobic respiration in which, catalyzing the phosphorylation of intracellular ADP to ATP and providing energy. Mitochondria are crucial for maintaining the dynamic equilibrium of cellular redox, signal transduction, and the regulation of apoptosis (Chen and Tang, 2014). Mitophagy is a process that selectively isolates and degrades damaged mitochondria. It is a specific quality control mechanism that plays an important role in maintaining mitochondrial function, integrity, and dynamic homeostasis (Youn et al., 2020). Impaired mitophagy results in the progressive accumulation of defective mitochondria, leading to cell and tissue damage (Dombi et al., 2018). It has been found that the induction of mitophagy attenuates the cytotoxicity of carbonyl cyanide m-chlorophenylhydrazone (CCCP) on auditory cells, thereby protecting the auditory system (Setz et al., 2018). In addition, it has also been established that the upregulation of mitophagy can minimize damage to the inner ear and prevent hearing loss (Ye et al., 2018).
The process of mitophagy requires the synergy of multiple factors. Lin et al. (2019) demonstrated that Dynamin-related Protein 1 (Drp1) plays a central role in mitophagy by regulating mitochondrial fission. Inhibition of Drp1 promotes cochlear hair cell senescence and exacerbates age-related hearing loss. Ramonett et al. (2022) demonstrated the interaction between GIPC and Drp1 by immunoprecipitation, among other methods, and found that Drp1 interacts with GIPC through its atypical C-terminal PDZ-binding motif. GIPC directs the aggregation of Drp1 in perinuclear mitochondria, which in turn promotes mitochondrial fission; thus, GIPC-mediated Drp1 retrograde plays a key role in mitochondrial fission. Previous research has demonstrated that the interaction between GIPC and Drp1 promotes mitochondrial fission and thus affects the mitophagy process (Figure 1). Therefore, when GIPC3 is mutated, the interaction between the two may be impacted. This allows for the inhibition of Drp1 aggregation in perinuclear mitochondria and reduced mitophagy, contributing to NSHL.
4. Conclusion and outlook
GIPC3, as a member of the GIPC family, has important implications in physiological and pathological processes, such as signal transduction, substance transport, cell migration, and neurotransmitter release. GIPC3 has been found to be most closely associated with hereditary deafness, and GIPC3 mutations are the foundation of DFNB15, DFNB72, and DFNB95-associated NSHL.
Several mechanisms by which GIPC3 mutations may cause NSHL and acoustic seizures have been suggested. First, Gipc3 is required for postnatal stereocilia maturation and the long-term survival of hair cells and SGNs. When Gipc3 is mutated, the reduced Gipc3 protein expression compromises the inner ear structures, which are used for mechanotransduction and signal amplification. Second, the Gipc3 mutations shift the activation voltage of Ca2+ influx to a more hyperpolarized potential and reverse the modiolar-pillar gradient of maximal Ca2+ influx while increasing the proportion of SGNs with high spontaneous discharge rates. Third, GIPC can adjust the number of NMDA receptors by binding to NMDA receptors, and GIPC can prevent NMDA receptors from interacting with other PDZ proteins, acting as an antagonist. GIPC3 mutations may increase the number of NMDA receptors and disrupt homeostasis, which can result in auditory seizures. Fourth, Drp1 interacts with GIPC through a C-terminal PDZ-binding motif. GIPC directs the aggregation of Drp1 in perinuclear mitochondria, promoting mitophagy. When GIPC3 is mutated, the interaction between the two may be inhibited, which in turn leads to NSHL.
It is unclear how GIPC3 mutations cause sensorineural deafness and acoustic seizures. For example, it is unclear how GIPC3 mutations trigger impaired stereocilia maturation, whether GIPC3 mutations inhibit Drp1-mediated mitophagy, and whether it triggers cochlear autoimmune reactions. To better understand the molecular biological mechanisms underlying genetic deafness due to GIPC3 mutations and to provide interventions, further experimental validation is needed. In conclusion, GIPC3 is a scientifically valuable focus in the study of the developmental mechanisms of hereditary deafness.
Author contributions
XL consulted the literatures and wrote the manuscript. LS and LW participated in drafting of the manuscript and provided oversight for the project. LS was responsible for conducting manuscript evaluation. All authors read and approved the final manuscript.
Funding
This work was supported by the National Natural Science Foundation of China (Grant Nos. NSFC81503372 and 82072953), the Liaoning Provincial Department of Education Project (Grant No. LZ2020033), the Top Young Talent of Liaoning Provincial Government (Grant No. XLYC1907009), and the Dalian Outstanding Young Scientific and Technological Talents (Grant No. 2021RJ12).
Conflict of interest
The authors declare that the research was conducted in the absence of any commercial or financial relationships that could be construed as a potential conflict of interest.
Publisher’s note
All claims expressed in this article are solely those of the authors and do not necessarily represent those of their affiliated organizations, or those of the publisher, the editors and the reviewers. Any product that may be evaluated in this article, or claim that may be made by its manufacturer, is not guaranteed or endorsed by the publisher.
References
Ain, Q., Nazli, S., Riazuddin, S., Jaleel, A. U., Riazuddin, S. A., Zafar, A. U., et al. (2007). The autosomal recessive nonsyndromic deafness locus DFNB72 is located on chromosome 19p13.3. Hum. Genet. 122, 445–450. doi: 10.1007/s00439-007-0418-z
Ammar-Khodja, F., Bonnet, C., Dahmani, M., Ouhab, S., Lefevre, G. M., Ibrahim, H., et al. (2015). Diversity of the causal genes in hearing impaired Algerian individuals identified by whole exome sequencing. Mol. Genet. Genomic Med. 3, 189–196. doi: 10.1002/mgg3.131
Asgharzade, S., Tabatabaiefar, M. A., Mohammadi-Asl, J., and Chaleshtori, M. H. (2018). A novel missense mutation in GIPC3 causes sensorineural hearing loss in an Iranian family revealed by targeted next-generation sequencing. Int. J. Pediatr. Otorhinolaryngol. 108, 8–11. doi: 10.1016/j.ijporl.2018.01.006
Avraham, K. B., Hasson, T., Steel, K. P., Kingsley, D. M., Russell, L. B., Mooseker, M. S., et al. (1995). The mouse Snell’s waltzer deafness gene encodes an unconventional myosin required for structural integrity of inner ear hair cells. Nat. Genet. 11, 369–375. doi: 10.1038/ng1295-369
Azaiez, H., Booth, K. T., Ephraim, S. S., Crone, B., Black-Ziegelbein, E. A., Marini, R. J., et al. (2018). Genomic landscape and mutational signatures of deafness-associated genes. Am. J. Hum. Genet. 103, 484–497. doi: 10.1016/j.ajhg.2018.08.006
Bitarafan, F., Seyedena, S. Y., Mahmoudi, M., and Garshasbi, M. (2020). Identification of novel variants in Iranian consanguineous pedigrees with nonsyndromic hearing loss by next-generation sequencing. J. Clin. Lab. Anal. 34:e23544. doi: 10.1002/jcla.23544
Booth, R. A., Cummings, C., Tiberi, M., and Liu, X. J. (2002). GIPC participates in G protein signaling downstream of insulin-like growth factor 1 receptor. J. Biol. Chem. 277, 6719–6725. doi: 10.1074/jbc.M108033200
Chadha, S., Kamenov, K., and Cieza, A. (2021). The world report on hearing, 2021. Bull. World Health Organ. 99:42. doi: 10.2471/BLT.21.285643
Charizopoulou, N., Lelli, A., Schraders, M., Ray, K., Hildebrand, M. S., Ramesh, A., et al. (2011). Gipc3 mutations associated with audiogenic seizures and sensorineural hearing loss in mouse and human. Nat. Commun. 2:201. doi: 10.1038/ncomms1200
Chen, A., Wayne, S., Bell, A., Ramesh, A., Srisailapathy, C. R., Scott, D. A., et al. (1997). New gene for autosomal recessive non-syndromic hearing loss maps to either chromosome 3q or 19p. Am. J. Med. Genet. 71, 467–471.
Chen, H., and Tang, J. (2014). The role of mitochondria in age-related hearing loss. Biogerontology 15, 13–19. doi: 10.1007/s10522-013-9475-y
de Jonge, J. J., Batters, C., O’Loughlin, T., Arden, S. D., and Buss, F. (2019). The MYO6 interactome: Selective motor-cargo complexes for diverse cellular processes. FEBS Lett. 593, 1494–1507. doi: 10.1002/1873-3468.13486
De Vries, L., Lou, X., Zhao, G., Zheng, B., and Farquhar, M. G. (1998). GIPC, a PDZ domain containing protein, interacts specifically with the C terminus of RGS-GAIP. Proc. Natl. Acad. Sci. U.S.A. 95, 12340–12345. doi: 10.1073/pnas.95.21.12340
Diaz-Horta, O., Duman, D., Foster, J. II, Sirmaci, A., Gonzalez, M., Mahdieh, N., et al. (2012). Whole-exome sequencing efficiently detects rare mutations in autosomal recessive nonsyndromic hearing loss. PLoS One 7:e50628. doi: 10.1371/journal.pone.0050628
Ding, X., Philip, S., Martin, B. K., Pang, Y., Burkett, S., Swing, D. A., et al. (2017). Survival of BRCA2-Deficient Cells Is Promoted by GIPC3, a Novel Genetic Interactor of BRCA2. Genetics 207, 1335–1345. doi: 10.1534/genetics.117.300357
Dombi, E., Mortiboys, H., and Poulton, J. (2018). Modulating mitophagy in mitochondrial disease. Curr. Med. Chem. 25, 5597–5612. doi: 10.2174/0929867324666170616101741
Frank, T., Khimich, D., Neef, A., and Moser, T. (2009). Mechanisms contributing to synaptic Ca2+ signals and their heterogeneity in hair cells. Proc. Natl. Acad. Sci. U.S.A. 106, 4483–4488. doi: 10.1073/pnas.0813213106
Fuchs, P. A., Glowatzki, E., and Moser, T. (2003). The afferent synapse of cochlear hair cells. Curr. Opin. Neurobiol. 13, 452–458. doi: 10.1016/s0959-4388(03)00098-9
Gao, X., Huang, S. S., Yuan, Y. Y., Xu, J. C., Gu, P., Bai, D., et al. (2017). Identification of TMPRSS3 as a significant contributor to autosomal recessive hearing loss in the Chinese population. Neural Plast. 2017:3192090. doi: 10.1155/2017/3192090
Jeanneteau, F., Diaz, J., Sokoloff, P., and Griffon, N. (2004). Interactions of GIPC with dopamine D2, D3 but not D4 receptors define a novel mode of regulation of G protein-coupled receptors. Mol. Biol. Cell 15, 696–705. doi: 10.1091/mbc.e03-05-0293
Kannan-Sundhari, A., Yan, D., Saeidi, K., Sahebalzamani, A., Blanton, S. H., and Liu, X. Z. (2020). Screening consanguineous families for hearing loss using the miamiotogenes panel. Genet. Test Mol. Biomarkers 24, 674–680. doi: 10.1089/gtmb.2020.0153
Katoh, M. (2013). Functional proteomics, human genetics and cancer biology of GIPC family members. Exp. Mol. Med. 45:e26. doi: 10.1038/emm.2013.49
Khan, A., Han, S., Wang, R., Ansar, M., Ahmad, W., and Zhang, X. (2019). Sequence variants in genes causing nonsyndromic hearing loss in a Pakistani cohort. Mol. Genet. Genomic Med. 7:e917. doi: 10.1002/mgg3.917
Lin, H., Xiong, H., Su, Z., Pang, J., Lai, L., Zhang, H., et al. (2019). Inhibition of DRP-1-dependent mitophagy promotes cochlea hair cell senescence and exacerbates age-related hearing loss. Front. Cell Neurosci. 13:550. doi: 10.3389/fncel.2019.00550
Misawa, H., Sherr, E. H., Lee, D. J., Chetkovich, D. M., Tan, A., Schreiner, C. E., et al. (2002). Identification of a monogenic locus (jams1) causing juvenile audiogenic seizures in mice. J. Neurosci. 22, 10088–10093. doi: 10.1523/JNEUROSCI.22-23-10088.2002
Ohn, T. L., Rutherford, M. A., Jing, Z., Jung, S., Duque-Afonso, C. J., Hoch, G., et al. (2016). Hair cells use active zones with different voltage dependence of Ca2+ influx to decompose sounds into complementary neural codes. Proc. Natl. Acad. Sci. U.S.A. 113, E4716–E4725. doi: 10.1073/pnas.1605737113
Petrova, N. V., Marakhonov, A. V., Balinova, N. V., Abrukova, A. V., Konovalov, F. A., Kutsev, S. I., et al. (2021). Genetic variant c.245A>G (p.Asn82Ser) in GIPC3 gene is a frequent cause of hereditary nonsyndromic sensorineural hearing loss in chuvash population. Genes (Basel) 12:820. doi: 10.3390/genes12060820
Prahst, C., Heroult, M., Lanahan, A. A., Uziel, N., Kessler, O., Shraga-Heled, N., et al. (2008). Neuropilin-1-VEGFR-2 complexing requires the PDZ-binding domain of neuropilin-1. J. Biol. Chem. 283, 25110–25114. doi: 10.1074/jbc.C800137200
Puel, J. L. (1995). Chemical synaptic transmission in the cochlea. Prog. Neurobiol. 47, 449–476. doi: 10.1016/0301-0082(95)00028-3
Ramonett, A., Kwak, E. A., Ahmed, T., Flores, P. C., Ortiz, H. R., Lee, Y. S., et al. (2022). Regulation of mitochondrial fission by GIPC-mediated Drp1 retrograde transport. Mol. Biol. Cell 33:ar4. doi: 10.1091/mbc.E21-06-0286
Ramzan, K., Al-Owain, M., Allam, R., Berhan, A., Abuharb, G., Taibah, K., et al. (2013). Homozygosity mapping identifies a novel GIPC3 mutation causing congenital nonsyndromic hearing loss in a Saudi family. Gene 521, 195–199. doi: 10.1016/j.gene.2013.03.042
Rehman, A. U., Gul, K., Morell, R. J., Lee, K., Ahmed, Z. M., Riazuddin, S., et al. (2011). Mutations of GIPC3 cause nonsyndromic hearing loss DFNB72 but not DFNB81 that also maps to chromosome 19p. Hum. Genet. 130, 759–765. doi: 10.1007/s00439-011-1018-5
Ren, T. (2005). The cochlear amplifier and Ca2+ current-driven active stereocilia motion. Nat. Neurosci. 8, 132–134. doi: 10.1038/nn0205-132
Roux, I., Hosie, S., Johnson, S. L., Bahloul, A., Cayet, N., Nouaille, S., et al. (2009). Myosin VI is required for the proper maturation and function of inner hair cell ribbon synapses. Hum. Mol. Genet. 18, 4615–4628. doi: 10.1093/hmg/ddp429
Saitoh, T., Mine, T., and Katoh, M. (2002). Molecular cloning and characterization of human GIPC3, a novel gene homologous to human GIPC1 and GIPC2. Int. J. Oncol. 20, 577–582.
Setz, C., Benischke, A. S., Pinho Ferreira Bento, A. C., Brand, Y., Levano, S., Paech, F., et al. (2018). Induction of mitophagy in the HEI-OC1 auditory cell line and activation of the Atg12/LC3 pathway in the organ of Corti. Hear. Res. 361, 52–65. doi: 10.1016/j.heares.2018.01.003
Shang, G., Brautigam, C. A., Chen, R., Lu, D., Torres-Vazquez, J., and Zhang, X. (2017). Structure analyses reveal a regulated oligomerization mechanism of the PlexinD1/GIPC/myosin VI complex. Elife 6:e27322. doi: 10.7554/eLife.27322
Siddiqi, S., Ismail, M., Oostrik, J., Munawar, S., Mansoor, A., Kremer, H., et al. (2014). A canonical splice site mutation in GIPC3 causes sensorineural hearing loss in a large Pakistani family. J. Hum. Genet. 59, 683–686. doi: 10.1038/jhg.2014.86
Sindura, K. P., and Banerjee, M. (2019). An immunological perspective to non-syndromic sensorineural hearing loss. Front. Immunol. 10:2848. doi: 10.3389/fimmu.2019.02848
Sirmaci, A., Edwards, Y. J., Akay, H., and Tekin, M. (2012). Challenges in whole exome sequencing: An example from hereditary deafness. PLoS One 7:e32000. doi: 10.1371/journal.pone.0032000
Sloan-Heggen, C. M., Bierer, A. O., Shearer, A. E., Kolbe, D. L., Nishimura, C. J., Frees, K. L., et al. (2016). Comprehensive genetic testing in the clinical evaluation of 1119 patients with hearing loss. Hum. Genet. 135, 441–450. doi: 10.1007/s00439-016-1648-8
Spudich, G., Chibalina, M. V., Au, J. S., Arden, S. D., Buss, F., and Kendrick-Jones, J. (2007). Myosin VI targeting to clathrin-coated structures and dimerization is mediated by binding to Disabled-2 and PtdIns(4,5)P2. Nat. Cell Biol. 9, 176–183. doi: 10.1038/ncb1531
van Beeck Calkoen, E. A., Engel, M. S. D., van de Kamp, J. M., Yntema, H. G., Goverts, S. T., Mulder, M. F., et al. (2019). The etiological evaluation of sensorineural hearing loss in children. Eur. J. Pediatr. 178, 1195–1205. doi: 10.1007/s00431-019-03379-8
Varsano, T., Dong, M. Q., Niesman, I., Gacula, H., Lou, X., Ma, T., et al. (2006). GIPC is recruited by APPL to peripheral TrkA endosomes and regulates TrkA trafficking and signaling. Mol. Cell Biol. 26, 8942–8952. doi: 10.1128/MCB.00305-06
Ye, B., Fan, C., Shen, Y., Wang, Q., Hu, H., and Xiang, M. (2018). The antioxidative role of autophagy in hearing loss. Front. Neurosci. 12:1010. doi: 10.3389/fnins.2018.01010
Yi, Z., Petralia, R. S., Fu, Z., Swanwick, C. C., Wang, Y. X., Prybylowski, K., et al. (2007). The role of the PDZ protein GIPC in regulating NMDA receptor trafficking. J. Neurosci. 27, 11663–11675. doi: 10.1523/JNEUROSCI.3252-07.2007
Youn, C. K., Jun, Y., Jo, E. R., and Cho, S. I. (2020). Age-related hearing loss in C57BL/6J mice is associated with mitophagy impairment in the central auditory system. Int. J. Mol. Sci. 21:7202. doi: 10.3390/ijms21197202
Yu, C., Feng, W., Wei, Z., Miyanoiri, Y., Wen, W., Zhao, Y., et al. (2009). Myosin VI undergoes cargo-mediated dimerization. Cell 138, 537–548. doi: 10.1016/j.cell.2009.05.030
Keywords: GIPC3, auditory system, hereditary deafness, organ of Corti, gene mutations
Citation: Li X, Shi L and Wang L (2023) A review of the mechanisms underlying the role of the GIPC3 gene in hereditary deafness. Front. Synaptic Neurosci. 14:1101587. doi: 10.3389/fnsyn.2022.1101587
Received: 18 November 2022; Accepted: 16 December 2022;
Published: 06 January 2023.
Edited by:
Atsuo Fukuda, Hamamatsu University School of Medicine, JapanReviewed by:
Thomas Friedman, National Institute on Deafness and Other Communication Disorders (NIH), United StatesJose Antonio Lopez-Escamez, Universidad de Granada, Spain
Copyright © 2023 Li, Shi and Wang. This is an open-access article distributed under the terms of the Creative Commons Attribution License (CC BY). The use, distribution or reproduction in other forums is permitted, provided the original author(s) and the copyright owner(s) are credited and that the original publication in this journal is cited, in accordance with accepted academic practice. No use, distribution or reproduction is permitted which does not comply with these terms.
*Correspondence: Lin Shi, c2hpbGluNTE2QHNpbmEuY29t; Liang Wang,
d2FuZ2xpYW5nQGRtdS5lZHUuY24=