- Departamento de Farmacobiología, Centro de Investigación y de Estudios Avanzados (Cinvestav-Sede Sur), Ciudad de México, Mexico
Endocannabinoids modulate mesolimbic (MSL) dopamine (DA) neurons firing at the ventral tegmental area (VTA). These neurons are activated by copulation, increasing DA release in nucleus accumbens (NAcc). Copulation to satiety in male rats implies repeated ejaculation within a short period (around 2.5 h), during which NAcc dopamine concentrations remain elevated, suggesting continuous neuronal activation. During the 72 h that follow copulation to satiety, males exhibit long-lasting changes suggestive of brain plasticity processes. Enhanced DA neuron activity triggers the synthesis and release of endocannabinoids (eCBs) in the VTA, which participate in several long-term synaptic plasticity processes. Blockade of cannabinoid type 1 receptors (CB1Rs) during copulation to satiety interferes with the appearance of the plastic changes. Glutamatergic inputs to the VTA express CB1Rs and contribute to DA neuron burst firing and synaptic plasticity. We hypothesized that eCBs, released during copulation to satiety, would activate VTA CB1Rs and modulate synaptic plasticity processes involving glutamatergic transmission. To test this hypothesis, we determined changes in VTA CB1R density, phosphorylation, and internalization in rats that copulated to satiety 24 h earlier as compared both to animals that ejaculated only once and to sexually experienced unmated males. Changes in glutamate AMPAR and NMDAR densities and subunit composition and in ERK1/2 activation were determined in the VTA of males that copulated to satiety in the presence or absence of AM251, a CB1R antagonist. The CB1R density decreased and the proportion of phosphorylated CB1Rs increased in the animals that copulated compared to control rats. The CB1R internalization was detected only in sexually satiated males. A decrease in α-amino-3-hydroxy-5-methylisoxazole-4-propionate receptor (AMPAR) density, blocked by AM251 pretreatment, and an increase in the proportion of GluA2-AMPARs occurred in sexually satiated rats. GluN2A- N-methyl-D-aspartate receptor (NMDAR) expression decreased, and GluN2B-NMDARs increased in these animals, both of which were prevented by AM251 pre-treatment. An increase in phosphorylated ERK1/2 emerged in males copulating to satiety in the presence of AM251. Results demonstrate that during copulation to satiety, eCBs activate CB1Rs in the VTA, producing changes in glutamate receptors compatible with a reduced neuronal activation. These changes could play a role in the induction of the long-lasting physiological changes that characterize sexually satiated rats.
Introduction
The mesolimbic (MSL) dopaminergic system is constituted by the dopaminergic neurons of the ventral tegmental area (VTA) that mainly project to the nucleus accumbens (NAcc) (Ikemoto, 2007). This circuit is activated by natural rewarding behaviors, such as sexual activity (Kelley and Berridge, 2002), increasing dopamine (DA) levels in the NAcc. Such DA increases occur after the exposure of male rats to estrous odors, to an inaccessible sexually receptive female, and in response to mating itself (Pfaus et al., 1990; Damsma et al., 1992; Mitchell and Gratton, 1994; Robinson et al., 2001), and are the result of the activation of VTA neurons. This has been demonstrated in studies showing that sex-related cues and copulation increase the expression of c-Fos, a marker of neuronal activity, in the DA neurons of the VTA (Balfour et al., 2006) and that the sexual behavior performance increases VTA neuronal firing (Hernandez-Gonzalez et al., 1997) and activates NAcc medium spiny neurons (MSNs) (Matsumoto et al., 2012). Conversely, the MSL system has been shown to participate in the regulation of sexual motivation and the reinforcing properties of copulatory behavior (Everitt, 1990; Melis and Argiolas, 1995; Pfaus, 2009).
Sexually experienced male rats that are allowed to copulate without restriction with a sexually receptive female will repeatedly ejaculate until becoming sexually satiated. Intense copulation is displayed along 2.5 h, time during which male rats are capable of executing 7 successive ejaculations, on an average, before becoming sexually inhibited. Interestingly, this sexual inhibition lasts 72 h, but the sexually satiated males require 15 days of sexual inactivity to fully recover their initial ejaculatory capacity (Rodríguez-Manzo and Fernández-Guasti, 1994; Rodríguez-Manzo et al., 2011). The duration of this sexual inhibitory state suggests the occurrence of neuroadaptations resulting from intense copulation within a short period. In support of this idea, the sexually satiated rats exhibit other physiological changes in addition to the sexual behavior inhibition, the most conspicuous of which is a generalized sensitization to drug actions, a phenomenon which also lasts 72 h (Rodríguez-Manzo et al., 2011).
It has been demonstrated that during the copulation to exhaustion process, the NAcc DA levels are continuously elevated (Fiorino et al., 1997), indicating the constant activation of MSL DA neurons. Enhanced midbrain DA neuron activity triggers the synthesis and release of endocannabinoids (eCBs) from their cell bodies in the VTA (Lupica and Riegel, 2005). These eCBs act retrogradely, activating presynaptic cannabinoid type 1 receptors (CB1Rs) located on GABAergic (Szabo et al., 2002) and glutamatergic axon terminals (Melis, 2004), where they regulate neurotransmitter release, thereby modulating the firing activity of midbrain DA neurons (Riegel and Lupica, 2004). This eCB-mediated regulation is exerted through the transient inhibition of neurotransmitter release by the following two short-term plasticity phenomena: depolarization-induced suppression of inhibition (DSI), when inhibiting GABA release (Ohno-Shosaku et al., 2001; Wilson and Nicoll, 2001), and depolarization-induced suppression of excitation (DSE), when inhibiting glutamate release (Kreitzer and Regehr, 2001; Melis, 2004). The eCBs are also involved in the induction of long-term forms of synaptic plasticity, namely long-term depression (eCB-LTD) at both, glutamatergic (Gerdeman et al., 2002) and GABAergic synapses (Chevaleyre and Castillo, 2003). Besides, glutamatergic transmission at the VTA has been implicated in the induction of DA neuron burst firing, responsible for massive synaptic DA release in the NAcc (Floresco et al., 2003), as well as in long-term synaptic plasticity phenomena at the VTA (Xin et al., 2016). VTA neurons that are activated by copulation receive glutamatergic inputs (Balfour et al., 2006). Plasticity phenomena of excitatory synaptic transmission involve changes in α-amino-3-hydroxy-5-methylisoxazole-4-propionate (AMPA) and N-methyl-D-aspartate (NMDA) receptor densities and subunit composition (Song and Huganir, 2002; Paoletti et al., 2013).
Previous work from our laboratory showed that the blockade of CB1Rs during copulation to satiety, interferes with the appearance of the long-lasting sexual behavior inhibition and drug hypersensitivity in the sexually satiated rats (Canseco-Alba and Rodríguez-Manzo, 2014; González-Morales and Rodríguez-Manzo, 2020), evidencing the involvement of eCBs in the induction of these phenomena. These effects were replicated by the specific blockade of CB1Rs at the VTA (Canseco-Alba and Rodríguez-Manzo, 2016). On these bases, we hypothesized that eCBs released at the VTA during copulation to satiety could induce synaptic plasticity processes through the activation of CB1Rs that might involve glutamatergic transmission. To test this hypothesis, in the present work, we first determined if there were changes in VTA CB1R density, phosphorylation, and internalization—all events indicative of CB1R activation—in sexually experienced unmated control males and in rats that ejaculated only once or copulated to satiety 24 h earlier. In addition, we established the occurrence of changes in glutamate AMPA receptor (AMPAR) and NMDA receptor (NMDAR) densities and subunit composition in the VTA of male rats that copulated to satiety in the absence or presence of the CB1R antagonist, AM251. Finally, we explored if there were changes in the phosphorylation of the extracellular signal-regulated kinase (pERK 1/2), which has been associated with CB1R activation and to long-term synaptic plasticity processes.
Materials and Methods
Animals
Sexually experienced, adult male (250–300 g b. wt.) and sexually receptive female (200 g b.wt.) Wistar rats from our own vivarium were used in this study. Animals were housed, eight per cage, under inverted light/dark cycle conditions (12 h light: 12 h dark, lights on at 22:00 h), at 22°C, and with free access to food and water. Males were rendered sexually experienced by subjecting them to five independent sexual behavior tests and those animals achieving ejaculation in <15 min, in at least three of these tests, were classified as sexually experienced and selected for the study. Female rats served as sexual stimuli; sexual receptivity was induced by the sequential s.c. injection of estradiol benzoate (13 μg/rat) followed 24 h later by progesterone (7 mg/rat). All experimental procedures (Protocol 0230-16) were approved by our Institutional Internal Committee for the Care and Use of Laboratory Animals (Comité Institucional para el Cuidado y Uso de Animales de Laboratorio, CICUAL), which follows the regulations established in the Mexican Official Norm for the use and care of laboratory animals NOM-062-ZOO-1999.
Sexual Behavior and Sexual Satiety Tests
Sexual behavior tests were conducted 2 h after the beginning of the dark phase of the cycle, in a room under dim red light. Males were individually introduced into polycarbonate cylindrical arenas covered with fine sawdust. A 5-min adaptation period was permitted to the males before introducing a sexually receptive female. Sexual activity was allowed until the achievement of one ejaculation or the attainment of sexual satiety. In this last case, males had ad libitum copulation with a single sexually receptive female until a 90-min period elapsed since the last ejaculation, without accomplishment of another ejaculation, which is the criterion used to consider a male sexually satiated (Rodríguez-Manzo et al., 2011). After reaching the corresponding criteria, animals were returned to their home cages.
Drugs
All drugs were purchased from Sigma-Aldrich (St. Louis, MO, USA). AM251 was dissolved in a mixture of saline solution (98%), Tween80 (1%), and dimethyl sulfoxide (DMSO, 1%) and was i.p. injected. Estradiol benzoate and progesterone were dissolved in sesame oil and s.c. injected.
Antibodies
The following primary antibodies were used: rabbit anti-CB1R, mouse anti-β-actin, goat anti-β-Arrestin2 (β-A2), mouse anti-GluN2B and mouse anti-GLUA2 (Santa Cruz Biotechnology, Dallas, TX, USA); rabbit anti-GluN2A, rabbit anti-GluN1and rabbit anti-GluA1 (Alomone Labs, Jerusalem, Israel); rabbit anti-CB1R phospho S316 (pCB1R) (Abcam, Cambridge, UK), rabbit anti-ERK 1/2 phospho Thr202/Tyr204 (pERK 1/2) (Cell Signaling Technology, Danvers, MA, USA) and mouse anti-VGlut2 (Merck-Millipore, Burlington, MA, USA). Secondary Abs for immunoblotting include HRP-donkey anti-rabbit and HRP-goat anti-mouse (Jackson ImmunoResearch, West Grove, PA, USA). For immunofluorescence, the secondary Abs used were donkey anti-goat Alexa Fluor 488, donkey anti-mouse Alexa Fluor 555, and donkey anti-rabbit Alexa Fluor 647, all from Invitrogen (Thermo Fisher Scientific, San Diego, CA, USA).
Western Blot
Twenty-four hours after the sexual behavior tests, the animals were anesthetized with sodium pentobarbital and thereafter sacrificed by decapitation, their brains extracted in <5 min and cooled in dry ice for 15 s. Using stereotaxic coordinates from a rat brain atlas (Paxinos and Watson, 2009) as a reference, 2 mm thick coronal slices from the region containing the VTA were obtained. Bilateral punches of the VTA (1 mm diameter) from each rat were obtained with the aid of sample punches (Fine Science Tools Inc., CA, USA). Samples of both hemispheres of each rat were pooled, put in a lysis buffer [Tris HCL (50 mM), NaCl (150 mM), Igepal (1%), Triton X-100 (1%)] containing protein phosphatase (Thermo Fisher, catalog # 78428, 100X) and protease (Thermo Fisher, catalog # 78430,100X) inhibitor cocktails, pH = 7.4 and stored at −80°C until further processing. Tissue samples were thawed, homogenized by sonication, and centrifuged at 14,000 rpm, for 30 min, at 4°C; the resulting pellet was removed and protein concentrations were quantified in the supernatant using the modified Lowry method (DC Protein assay instruction manual, BIORAD (Lowry et al., 1951; Peterson, 1979). Bovine serum albumin (BSA, Sigma catalog # 05470) was used to build protein reference standard curves.
From each tissue sample, 25 μg protein, diluted 1:1 in 2X loading buffer (Sigma, catalog # S3401) were boiled to 95°C for 5 min, loaded onto 8% SDS-polyacrylamide gels and separated by electrophoresis for 2 h, at 100 V. A protein molecular weight (MW) standard (Page ruler plus pre-stained protein ladder, Thermo Fisher, catalog # 26620) was added to each gel. Proteins were electrophoretically transferred onto a polyvinylidene fluoride (PVDF) membrane for 1 h, at 100 V, 350 mA. PVDF membranes were blocked for 2.5 h, at room temperature, with 4% milk (Biorad, catalog # 1706404) in Tris-Buffered Saline (TBS) with 1% Tween20 (Sigma, catalog # P9416) (TBS-T) for CB1R and pERK 1/2 Abs, and with 2% milk for the rest of Abs used (GluN1, GluN2A, GluN2B, GluA1, GluA2, and pCB1R). Thereafter, the membranes were incubated with the primary antibody overnight, at 4°C. Membranes were then washed three times with TBS-T for 5–10 min, under agitation, and were incubated with the corresponding secondary antibody for 1.5 h at room temperature. After this, they were washed three times with TBS-T and once with TBS. A chemiluminescence kit (Millipore, catalog #WBKLS0500) was used to reveal the membranes using the BioradChemidoc MP Imaging system (Hercules, CA, USA). Optical density (OD) of the resulting bands was determined with the aid of the ImageJ program (NIH) and the values were normalized with those of β-actin, which served as a charge control. Details on the Abs used for Western blotting are summarized in Table 1.
Immunofluorescence for CB1R and β-A2
Double immunofluorescence and confocal microscopy were used to analyze CB1R co-localization with β-arrestin2 (βA2), as a tool to determine CB1 cannabinoid receptor internalization in the VTA of male rats that ejaculated once or copulated to satiety 24 h earlier.
Tissue Preparation
Twenty-four hours after the sexual behavior tests, animals were deeply anesthetized with sodium pentobarbital (200 mg/kg, i.p.) and intracardially perfused with 250 ml 0.9% sodium chloride, followed by 400 ml paraformaldehyde (4%) in sodium phosphate solution (PBS) buffer 0.1 M supplemented with 0.9% sodium chloride, pH 7.3. At the end of this procedure, brains were extracted from the skull and immediately placed in a cryoprotectant solution (sucrose at 30% in PBS) and stored at 4°C until further use.
Sixty coronal sections (40 μm) of the brain region containing the VTA [coordinates from −5.2 to −6.3 mm anterior to bregma (Paxinos and Watson, 2009)] were obtained using a cryostat (Leica CM1100, Nussloch, Germany); 10 brain sections from each animal, representative of the whole VTA, were used for the immunofluorescence experiments.
Triple Immunofluorescence for CB1R, β-A2, and VGlut2
Tissue sections were washed with PBS, four times, for 10 min and incubated during 2.5 h with a blocking solution [2.0% BSA, (Sigma, catalog # 05470), 6% donkey serum (Sigma, catalog # D9663), 2% gelatin (Sigma, catalog # G7765), and 0.3% Triton X-100 (Sigma, catalog # T9284), all contained in PBS]. All incubations and washes were made under constant stirring. Thereafter, sections were incubated with the CB1R, β-A2, and VGlut2 primary antibodies, diluted in the blocking solution, first for 2 h at room temperature, then for 19 h at 4°C and finally, 2 h at room temperature. After incubations with the primary antibodies, the sections were washed four times, for 10 min each, with a solution containing 0.3% Triton X-100 and 2.0% gelatin mixed in PBS. Subsequently, they were incubated with the secondary antibodies and 1 μl DAPI (Sigma catalog # D8417), diluted in the blocking solution, for 2 h at room temperature. For immunohistochemical controls, the first antibodies were omitted, which resulted in the absence of immunoreactivity. After incubation, the slices were washed four times with 0.1% Triton X-100 in PBS, for 10 min each. Thereafter, the sections were mounted on gelatinized slides using antifade mounting medium (Prolong Diamond antifade kit, Molecular Probes catalog # P36962), and analyzed by confocal microscopy. Details on the Abs used for immunohistochemistry procedures are summarized in Table 2.
Confocal Analysis
Ten coronal brain sections, representative of the whole VTA, of three different rats from each group were used for CB1R and β-A2 immunodetection. The VTA region in these sections was localized with the 10X objective of a confocal microscope (Carl Zeiss, LSM 800 Airyscan). Once identified, the objective was changed to a 40x oil objective for image acquisition. The ZEN v10.0 black software was used for image acquisition and the ZEN 2.3 blue edition software for image processing (Carl Zeiss, Oberkochen, Germany). Images were acquired in the best signal mode with a 1 airy unit (AU) pinhole and the total optical area for the 40x objective was 24,336 μm2. The maximum emission spectrum of each fluorophore was delimited with barrier filters to avoid crosstalk between spectral emission curves. The 450 nm laser was used to detect DAPI, the 488 nm laser to detect Alexa 488, the 555 nm laser to detect Alexa 555, and the 647 nm laser to detect Alexa 647. The final color of each fluorophore (secondary antibodies) in the images was selected from the image software: magenta for Alexa 647 (CB1R), red for Alexa 555 (VGlut2), and green for Alexa 488 (β-A2). The immunoreactivities (IRs) for CB1R and β-A2 and their co-localization were obtained from three different channels (channel 1: Alexa 488 for β-A2, channel 2: Alexa 647 for CB1R, and channel 3: CB1R-IR/β-A2-IR co-localization). The IR values were obtained in μm2, which were provided by the ZEN blue software.
Quantitative Analysis
For the quantification of the CB1R and β-A2 densities, data were expressed as the ratio of each IR protein area/total analyzed area (24,336 μm2), using the following formulae: CB1R-IR = [channel 1 + channel 3 (μm2)]/24,336 μm2; β-A2-IR = [channel 2 + channel 3 (μm2)]/24,336 μm2. CB1R-IR/β-A2-IR co-localization was considered indicative of receptor internalization (Garduño-Gutiérrez et al., 2013). The degree of CB1R internalization was determined dividing IR co-localization values by the total CB1R-IR using the following formula: [channel 3 (co-localization of CB1R-IR and β-A2-IR) (μm2)]/[channel 1 (CB1R-IR area) + channel 3 (co-localization of CB1R-IR and β-A2-IR) (μm2)]. Data of a total of 30 brain sections per group were used for statistical analysis.
Statistical Analyses
Data are expressed as mean ± standard error of the mean (SEM). Changes in protein expression (CB1R, pCB1R, GluA1, GluA2, GluN1, GluN2A, GluN2B, and pERK1/2) were conducted by means of a one-way ANOVA followed by Tukey's test. Paired comparisons were conducted with the unpaired t-test; when normality was not met, the Mann–Whitney U test was used. Immunohistochemistry data were not normally distributed and were therefore analyzed by means of the Kruskal–Wallis ANOVA followed by Dunn's test. The Sigma Plot program, version 12.0 was used for all statistical analyses. In all cases, differences with a P ≤ 0.05 were considered significant.
Experimental Design
Three different groups of males were used to establish possible changes in CB1R and pCB1R densities (n = 5 each) as well as in CB1R internalization (n = 3): (1) sexually experienced males that had no sexual activity 1 week prior to sacrifice, which served as a control group; (2) sexually experienced males that ejaculated once and were sacrificed 24 h later, which were used as a reference for the effects of recent sexual activity; and (3) sexually experienced males that copulated to satiety and were sacrificed 24 h later, which constituted the group of interest.
For the determination of changes in the densities of AMPARs (GluA1 and GLUA2 subunits), NMDARs (GluN1, GluN2A, and GluN2B subunits) and the phosphorylated MAPK ERK1/2 (pERK1/2), a fourth group was included (n = 5). This group consisted of sexually experienced males that copulated to satiety in the presence of the CB1R antagonist, AM251 (0.3 mg/kg, i.p.), administered immediately before the copulation to satiety session, at a dose previously found to interfere with the appearance, 24 h later, of the long-lasting sexual behavior inhibition and drug hypersensitivity that characterize sexually satiated male rats (González-Morales and Rodríguez-Manzo, 2020). These animals were sacrificed 24 h later to find out if eCBs played a role in the putative changes in these proteins in the sexually satiated males. For the WB experiments, animals were anesthetized with sodium pentobarbital and sacrificed by decapitation to obtain the brain samples. For the immunohistochemical experiments, the animals were deeply anesthetized with sodium pentobarbital (200 mg/kg, i.p.), and intracardially perfused.
Experiment 1. Evidence for CB1R Activation in the VTA by Copulation to Satiety
In each of the three groups of males, the density of CB1Rs, as well as changes in the density of phosphorylated CB1Rs, were determined by Western blot. In addition, immunohistochemistry and confocal microscopy were used to establish CB1R co-localization with β-A2, as an indicator of CB1R internalization (Garduño-Gutiérrez et al., 2013).
Experiment 2. Determination of Changes in AMPARs of the VTA Induced by Copulation to Satiety and the Possible Involvement of eCBs in Their Induction
The density of VTA AMPARs was established in each of the four groups of males, by determining the expression of the constitutive GluA1 subunit of these receptors.
The expression of AMPARs containing the GluA2 subunit was also established in each of these groups, establishing the density of the GluA2 subunit. A Western blot procedure was used for all determinations.
Experiment 3. Establishment of Changes in NMDARs of the VTA Induced by Copulation to Satiety and the Possible Involvement of eCBs in Their Induction
The density of VTA NMDARs was established in the same four groups of animals, by determining the expression of the obligatory, channel-forming GluN1 subunit of these receptors. In addition, the densities of NMDARs containing the GluN2A subunit and of those containing the GluN2B subunit in all the four groups were established by determining the expression of the GluN2A or the GluN2B subunit. All determinations were carried on by Western blot.
Experiment 4. Establishment of Changes in VTA pERK1/2 Densities Induced by Copulation to Satiety and the Possible Involvement of eCBs in Their Induction
The expression of the phosphorylated form of the MAPK ERK1/2 (pERK1/2) was determined by Western blot in each of the four different groups of animals.
Results
Evidence for CB1R Activation in the VTA by Copulation to Satiety
Changes in VTA CB1R density produced by sexual activity are shown in Figure 1. There was a significant effect of mating on CB1R expression [one-way ANOVA, F(2, 12) = 25.20, P < 0.001]. Post-hoc analysis showed a significant decrease in the density of CB1Rs (Figure 1A) in both, the animals that executed one ejaculatory series (1 Ejac) and those that copulated to satiety (sexually satiated) the day before sacrifice, as compared to unmated sexually experienced control males (Tukey's test, P < 0.001 each). By contrast, as shown in Figure 1B, no significant differences in the expression of phosphorylated CB1Rs (pCB1R) were found among groups [one-way ANOVA, F(2, 12) = 2.33, P = 0.14].
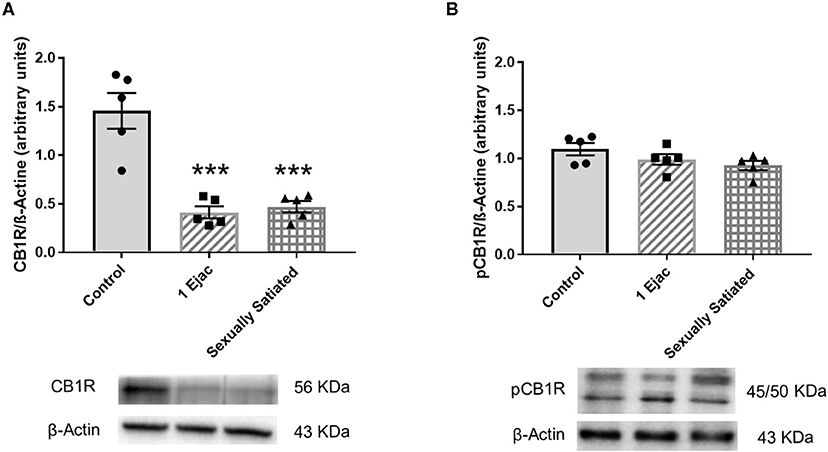
Figure 1. Changes in the densities of CB1R and pCB1R in the VTA of male rats in response to copulation. Western blot analysis showing changes in CB1R (A) and phosphorylated CB1R (pCB1R) (B) densities in the VTA of males that ejaculated once (1 Ejac) and males that copulated to satiety (Sexually satiated) 24 h earlier when compared to control sexually experienced unmated rats (Control). Values are mean ± S.E.M of the protein/β-Actin optical density (O.D.) ratios of 5 rats per group. One-way ANOVA followed by Tukey test, ***P < 0.001 vs. control.
Immunohistochemical and confocal microscopy analyses of mating-induced CB1R internalization is shown in Figure 2. Sample photomicrographs of the VTA, taken at the level of −6.0 mm from bregma (Figure 2A), were included in Figure 2. The upper row of photomicrographs corresponds to control, sexually experienced unmated rats (Figure 2B), the middle row refers to males that ejaculated once (Figure 2C), and the lower row to sexually satiated animals (Figure 2D). Similar patterns of CB1R immunoreactivity (CB1R-IR, magenta) distribution can be observed in all groups, i.e., a substantial amount of CB1R-IR could be detected, which was mainly located intracellularly, uniformly distributed in the cytoplasm of cell bodies with a defined nucleus (DAPI, blue). In addition, CB1R-IR appeared as punctuate marks, outside these rounded structures, that could indicate their presence in fibers. Quantification of CB1R-IR (Figure 2E) did not show statistically significant differences among groups [Kruskal–Wallis ANOVA, H(2, 30) = 3.76, P = 0.15].
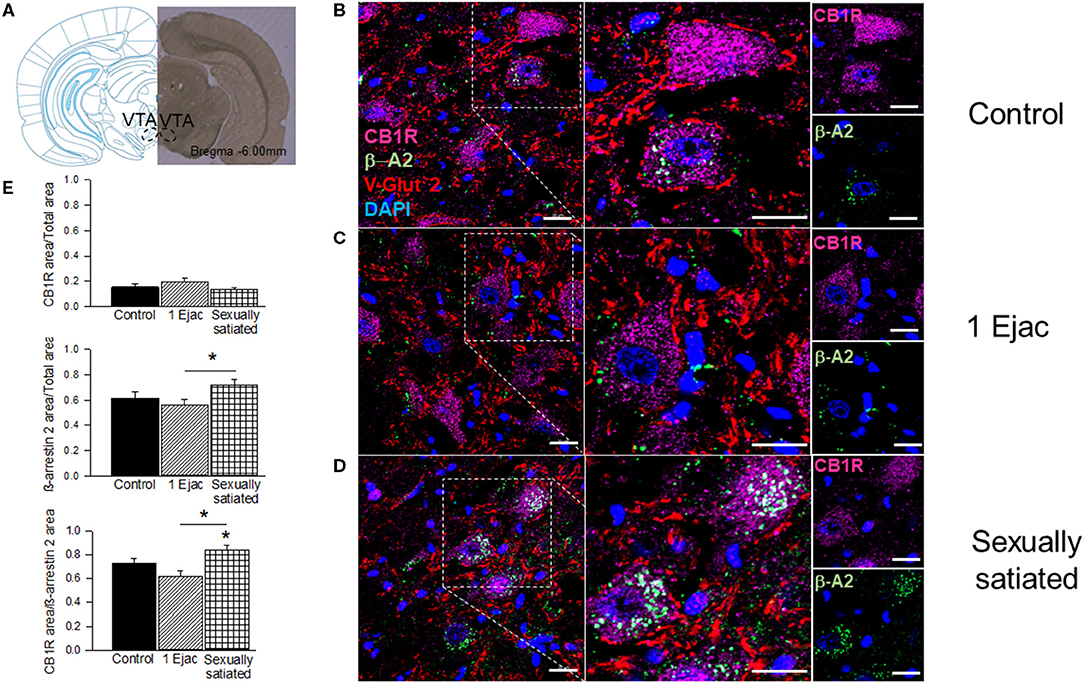
Figure 2. CB1R internalization in the VTA of male rats that copulated to satiety. (A) Shows a coronal brain section, taken at −6.00 mm anterior to bregma, showing the VTA region from where the sample photomicrographs were obtained. (B–D) Show representative confocal photomicrographs from the VTA of unmated sexually experienced male rats (Control), males that ejaculated once (1 Ejac) and males that copulated to satiety (Sexually satiated) 24 h earlier, respectively. The first column shows 40X magnification images of CB1R (Alexa 647, magenta), β-A2 (Alexa 488, green) and VGlut2 (Alexa 555, red) immunoreactivity (IR); cell nuclei appear in blue (DAPI). The second column depicts a 2x enlargement of the selected region with the same IR marks. The third column shows individual CB1R and β-A2 IR-marks in the selected region. Co-localization of CB1R with β-A2- appears in white color. Scale bar 20 μm. (E) Shows the quantification of CB1R-IR, β-A2-IR as well as that of their co-localization. Kruskal-Wallis followed by Dunn's test, *P < 0.05.
Ventral tegmental area β-A2 immunofluorescence (β-A2-IR, green) can be detected in all groups. In the control group (Figure 2B), β-A2-IR appears mainly as punctuating marks, sometimes forming empty circles, and occasionally distributed in the cytoplasm of cell bodies with a defined nucleus (DAPI, blue color). In the samples of males that ejaculated once (Figure 2C), β-A2-IR appears sometimes forming empty circles, which might correspond to cell body membranes. Finally, in the sexually satiated males (Figure 2D), an increase in β-A2-IR, together with a clear redistribution of the immunoreactive mark can be appreciated. In this case, clusters of β-A2-IR can be observed predominantly in the cytoplasm of cell bodies with a defined nucleus. However, punctuate marks can also be seen independently of these cytoplasmic clusters. Quantification of β-A2-IR evidenced statistically significant differences in its expression among groups [Kruskal–Wallis ANOVA, H(2, 30) = 6.89, P = 0.03]. Post-hoc analysis indicated a statistically significant increase in β-A2-IR in the VTA of the sexually satiated males when compared to animals that ejaculated only once (Dunn's test P < 0.05). The first two columns of the sample photomicrographs show the co-localization of CB1Rs with the β-A2 protein, which combined immunofluorescences produced a white color. Higher magnification images (2x) are included in the second column. Colocalization sample photomicrographs contain an additional immunoreactive mark for the glutamate vesicular transporter 2 (VGlut2-IR, red color), which presumably labels glutamatergic inputs to the VTA of subcortical origin, which are the most abundant (Omelchenko and Sesack, 2007). In the photomicrographs of control males, CB1R-IR/β-A2-IR colocalization is almost absent. In this sample image, the coincidence of both immunoreactive marks is only observed in the cytoplasm of one cell. VGlut2-IR is abundant and located mainly outside cell bodies; sometimes this mark coincides with the cell membrane, but the majority of VGlut2-IR can be seen in what appears to represent fibers. The sample photomicrograph of males that ejaculated once lacks CB1R-IR/β-A2-IR colocalization and the majority of VGlut2-IR is observed again in fibers. Finally, in the sample of the sexually satiated males CB1R-IR/β-A2-IR co-localization is evident in the cytoplasm of cell bodies with a defined nucleus, suggesting CB1R internalization. VGlut2-IR appears often in the vicinity of the cells showing CB1R-IR/β-A2-IR colocalization. Quantification of CB1R/β-A2 colocalization showed differences among groups [Kruskal–Wallis ANOVA, H(2, 30) = 15.10, P < 0.001]. A statistically significant increase in the colocalization of both marks was obtained in the sexually satiated males as compared to control unmated males and to animals ejaculating once (Dunn's test, P < 0.05 each).
Changes in AMPARs of the VTA Induced by Copulation to Satiety and Involvement of eCBs in Their Induction
The density of VTA AMPARs, determined by the expression of their constitutive GluA1 subunit, showed significant differences among groups [one-way ANOVA, F(2, 12) = 5.33, P = 0.02] (Figure 3A). Post-hoc analysis showed that the density of the GluA1 subunit decreased significantly in the sexually satiated rats. This decrease was statistically significant as compared to males ejaculating once (P < 0.05), and very close to significance when compared to the control group (P = 0.054). Interestingly, in the animals that copulated to satiety in the presence of the CB1R antagonist AM251 (Figure 3A), a significant increase in the expression of the GluA1 subunit was found as compared to the sexually satiated rats without treatment (t-test, P < 0.002), suggesting that the eCBs released during the sexual satiety development contributed to the decrease in AMPAR density.
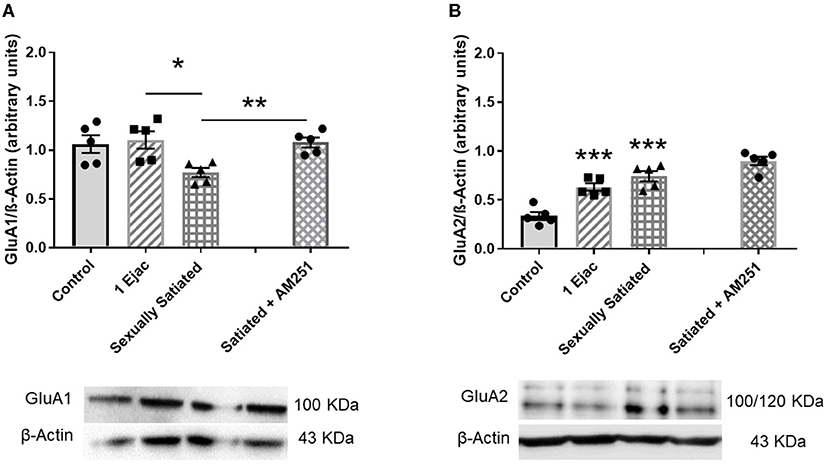
Figure 3. Changes in AMPAR density and subunit composition in the VTA of sexually satiated rats and involvement of eCBs in their induction. Western blot analysis showing changes in GluA1 (A) and GluA2 AMPAR subunit (B) densities in the VTA of control sexually experienced unmated rats (Control), males that ejaculated once (1 Ejac) or copulated to satiety (Sexually satiated) 24 h earlier, and of males that copulated to satiety in the presence of the CB1R antagonist AM251 (Satiated + AM251). Differences among untreated groups with different sexual conditions were determined by means of a one-way ANOVA followed by Tukey test, ***P < 0.001; *P < 0.05. A comparison between the rats that copulated to satiety in the absence or presence of AM251 was conducted with the unpaired t-test **P < 0.01. Values are mean ± S.E.M of the protein/β-Actin optical density (O.D.) ratios of 5 rats per group.
There were changes also in GluA2 subunit expression among groups [one-way ANOVA, F(2, 12) = 22.71, P < 0.001]. In this case, a significant increase in the expression of this subunit was found both in the animals ejaculating once (P = 0.001) and in the sexually satiated males (P < 0.001), when compared with control rats (Figure 3B). This increase was not modified in the males that copulated to satiety in the presence of the CB1R antagonist (Figure 3B), indicating that eCBs were not involved in this change.
Changes in NMDARs of the VTA Induced by Copulation to Satiety and Involvement of eCBs in Their Induction
Ventral tegmental area NMDAR density, determined through the measurement of its constitutive subunit GluN1, in the animals with different sexual activity showed differences among groups [one-way ANOVA, F(2, 12) = 6.05, P = 0.015]. Post-hoc analysis indicated a statistically significant increase in GluN1 expression in the sexually satiated males only when compared to males ejaculating once (P = 0.012), but not in comparison with the control group (Figure 4). In the animals in which CB1Rs were blocked with AM251 during sexual satiety development, NMDAR expression was not significantly modified when compared to males copulating to satiety in the absence of treatment (Figure 4).
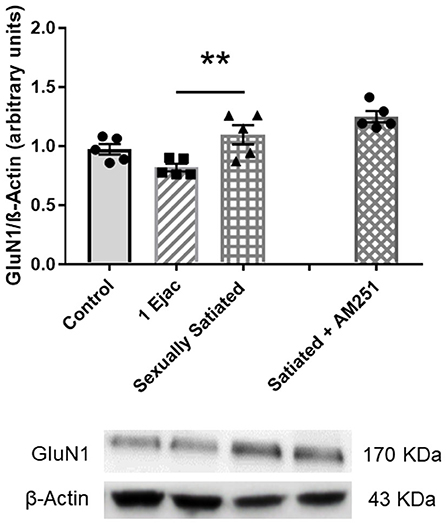
Figure 4. VTA NMDAR density does not change in response to sexual activity in male rats. Western blot analysis showing changes in the expression of the NMDAR constitutive GluN1 subunit in the VTA of control sexually experienced unmated rats (Control), males that ejaculated once (1 Ejac) or copulated to satiety (Sexually satiated) 24 h earlier, and of males that copulated to satiety in the presence of the CB1R antagonist AM251 (Satiated + AM251). Differences among untreated groups with different sexual conditions were determined by means of a one-way ANOVA followed by Tukey test, **P < 0.01. A comparison between the rats that copulated to satiety in the absence or presence of AM251 (Satiated + AM251) was conducted with the unpaired t-test, n.s. Values are mean ± S.E.M of the protein/β-Actin optical density (O.D.) ratios of 5 rats per group.
Establishment of changes in the expression of the GluN2A and the GluN2B NMDA subunits in the distinct groups is shown in Figure 5. As it can be seen, significant changes in GluN2A subunit expression were found among groups [one-way ANOVA, F(2, 12) = 14.3, P < 0.001], which was statistically significantly reduced in the sexually satiated males as compared to both control rats (P < 0.001) and animals ejaculating once (P = 0.01) (Figure 5A). Measurement of the GluN2B subunit also showed a significant effect of mating on its expression [one-way ANOVA, F(2, 12) = 14.5, P < 0.001], increasing its density in both, the sexually satiated males (P = 0.004) and those ejaculating once (P < 0.001) as compared to control animals (Figure 5B). Pre-treatment with the CB1R antagonist in the animals copulating to satiety prevented the GluN2A subunit decrease (Mann–Whitney U test, P = 0.03) and the GluN2B subunit increase (t-test, P = 0.005) in the sexually satiated males (Figures 5A,B, respectively).
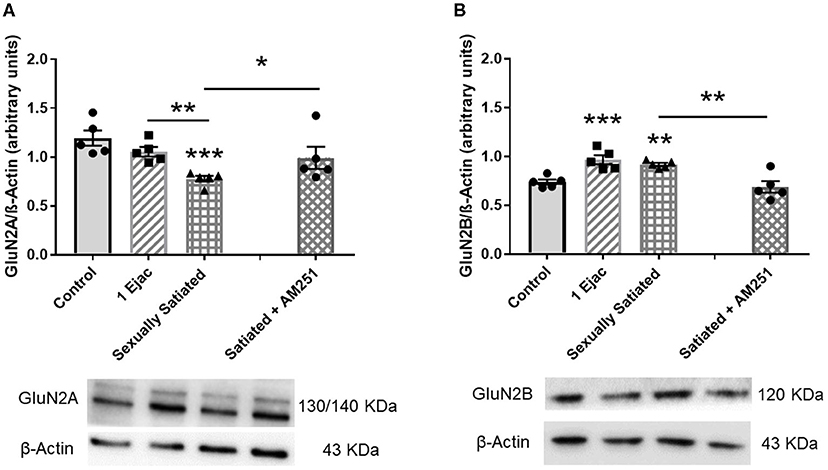
Figure 5. Changes in VTA NMDAR subunit composition produced by copulation to satiety in male rats and involvement of eCBs in their induction. Western blot analysis showing changes in GluN2A (A) and GluN2B (B) NMDAR subunit expression in the VTA of control sexually experienced unmated rats (Control), males that ejaculated once (1 Ejac) or copulated to satiety (Sexually satiated) 24 h earlier, and of rats that copulated to satiety in the presence of the CB1R antagonist AM251 (Satiated + AM251). Differences among untreated groups with different sexual conditions were determined by means of a one-way ANOVA followed by Tukey test, ***P < 0.001, **P < 0.01. A comparison between the rats that copulated to satiety in the absence or presence of AM251 (Satiated + AM251) was conducted with the Mann-Whitney U-test, *P < 0.05 or the unpaired t-test **P < 0.01. Values are mean ± S.E.M of the protein/β-Actin optical density (O.D.) ratios of 5 rats per group.
Changes in pERK1/2 in the VTA Induced by Copulation to Satiety and Involvement of eCBs in Their Induction
The expression of pERK1/2 was not modified by sexual activity (Figure 6) [one-way ANOVA, F(2, 12) = 3.12, P = 0.08]. However, in the males that copulated to satiety in the presence of AM251, a statistically significant increase in pERK1/2 expression was found when compared to untreated sexually satiated animals (t-test, P = 0.04), suggesting that eCBs hinder the phosphorylation of this MAPK (Figure 6).
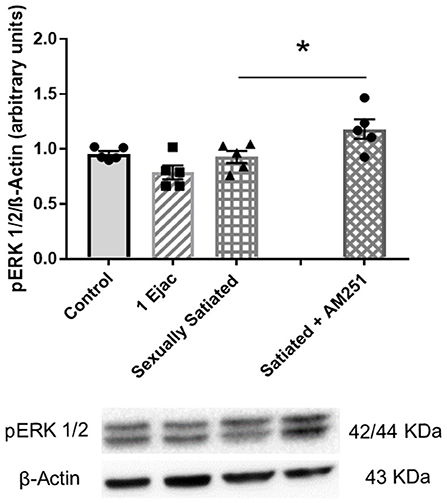
Figure 6. Involvement of eCBs in the changes in pERK 1/2 expression in the VTA of sexually satiated rats. Western blot analysis showing changes in pERK1/2 expression in the VTA of control sexually experienced unmated rats (Control), males that ejaculated once (1 Ejac) or copulated to satiety (Sexually satiated) 24 h earlier, and of rats that copulated to satiety in the presence of the CB1R antagonist AM251 (Satiated + AM251). Differences among untreated groups with different sexual conditions were determined by means of a one-way ANOVA, n.s. A comparison between the rats that copulated to satiety in the absence or presence of AM251 (Satiated + AM251) was conducted with the unpaired t-test, *P < 0.05. Values are mean ± S.E.M of the protein/β-Actin optical density (O.D.) ratios of 5 rats per group.
Discussion
Main findings of the present series of experiments are as follows: (1) copulation to satiety promotes the release of eCBs in the VTA, which activate CB1Rs and produce their internalization and downregulation; (2) 24 h after the intense sexual activity that takes place during sexual satiety development, changes in VTA glutamate AMPAR density and subunit composition and in NMDAR subunit composition are detected, coinciding with the period of sexual inhibition that characterizes sexually exhausted male rats; (3) the eCBs released during sexual activity are involved in the induction of changes in AMPAR and NMDAR, through the activation of CB1Rs, and prevent ERK1/2 phosphorylation in the sexually satiated rats.
Activation of CB1Rs at presynaptic inputs onto VTA DA neurons results from “on demand” postsynaptic eCB release during periods of increased DA neuronal activity (Lupica and Riegel, 2005). These eCBs exert control over MSL DA neuron activity by modulating GABAergic and glutamatergic inputs at the VTA (Riegel and Lupica, 2004). Sexual activity is a stimulus that enhances MSL DA neuron firing and, therefore, could promote eCB mobilization. The present study provides evidence showing that both, moderate (a single ejaculatory series) and intense copulation (until sexual satiety) activate CB1Rs at the VTA and induce their downregulation. Thus, 24 h after sexual activity, we found a significant reduction in VTA CB1R density in both copulating groups. Prolonged exposure to CB1R agonists is a condition found to produce CB1R downregulation, requiring new protein synthesis for receptor recovery (Hsieh et al., 1999). The majority of the available data on CB1R downregulation has been found following chronic tetrahydrocannabinol (THC) or synthetic cannabinoid agonist treatment (Sim-Selley, 2003), while only few studies report changes in the expression of CB1R in the brain induced by endogenous cannabinoids, and these are frequently associated with diseases involving pathological neuronal excitation. For example, a decrease in CB1R density has been documented in epileptic human hippocampal tissue (Ludányi et al., 2008), in rat hippocampus as a result of chronic stress (Hill et al., 2005; Hillard, 2014), chronic oxidative stress (Li et al., 2020), and in the ventral midbrain of patients with Parkinson's disease (Van Laere et al., 2012). The present study gives evidence for a reduction in VTA CB1R density under physiological conditions, produced by sexual activity. Little is known about the mechanisms involved in regulating the CB1R expression; again, these mechanisms have been related to disease states (Miller and Devi, 2011). Since the release of eCBs was produced by a physiological stimulus in our model, the mechanisms involved in CB1R density reduction are probably those acting in the natural receptor cycle, involving CB1R internalization and subsequent receptor degradation (Hsieh et al., 1999). The decrease in CB1R expression here reported suggests that copulation might induce a prolonged increase in eCB levels in the VTA.
No changes in the expression of phosphorylated CB1Rs (pCB1Rs) were found in any of the copulating groups of rats as compared to unmated control males. However, the lack of change in pCB1R expression, combined with the decrease in CB1R density in the VTA of these animals, implies that a larger proportion of the expressed CB1Rs is phosphorylated 24 h after sexual activity. These data strongly suggest that the proportion of pCB1Rs increases in the VTA of male rats as a result of sexual activity, thereby evidencing CB1R activation. The G protein-coupled receptor (GPCR) phosphorylation followed by binding an arrestin is a common pathway for uncoupling GPCRs, like the CB1R, from G protein-dependent signaling (Moore et al., 2007). G protein receptor kinases (GRKs) are recruited to the plasma membrane and participate in phosphorylating the agonist-occupied receptor, increasing the affinity of β-arrestins for the receptor; β-arrestin binding prevents further receptor activation. Thus, ligand-induced CB1R phosphorylation results in receptor desensitization and β-arrestin recruitment (Nogueras-Ortiz and Yudowski, 2016). β-Arrestins also work as a scaffold for the endocytic machinery that internalizes GPCRs. The recruitment of β-arrestin2 (β-A2) has been associated with the internalization process of the CB1R (Gyombolai et al., 2013). In the present study, no difference in the magnitude of VTA pCB1Rs expression was found between animals that executed mild and intense copulation the day before, though an increase in CB1R/β-A2 co-localization, interpreted as indicative of CB1R internalization, was only found in the sexually satiated rats. It has been reported that, in contrast to synthetic cannabinoid agonists, which cause rapid CB1R internalization, an analog of the endogenous cannabinoid anandamide causes internalization only at high concentrations (Hsieh et al., 1999). Thus, it could be thought that after one ejaculation, the eCBs released by DA neurons activated CB1Rs inducing their phosphorylation but did not attain the concentrations needed to induce CB1R internalization, while in the animals that copulated to satiety, DA neurons were activated repeatedly, releasing eCBs for a longer period and reaching the concentrations needed to induce CB1R internalization. Another possible interpretation for this differential result is related to the timing of CB1R internalization, since short-term agonist exposure has been reported to induce rapid CB1R internalization and recycling (Hsieh et al., 1999). This rapid process could have occurred in the animals that experienced one ejaculation and therefore, CB1R/β-A2 colocalization was no longer observed 24 h later. In contrast, the repeated activation of DA neurons during copulation to satiety could have released a larger amount of eCBs over a longer lasting period, inducing several cycles of CB1R internalization, which were still detectable 24 h later. To the best of our knowledge, this is the first time that evidence is provided for in vivo CB1R internalization in VTA neurons, mediated by endogenous cannabinoids that were released by a natural stimulus, under physiological conditions. In this sense, this finding is of particular significance as it provides direct information on the functioning of the endogenous cannabinoid system in the MSL circuit, specifically in the VTA, contributing to our understanding of the role of the endogenous cannabinoid system in the function of the central nervous system.
The VTA neurons that are activated by copulation in male rats, identified by the mating-induced expression of Fos protein, receive glutamatergic afferents from the medial prefrontal cortex (mPFC) (Tzschentke, 2001; Balfour et al., 2006), the pedunculopontine nucleus (PPT), and the laterodorsal tegmental (LDT) area (Clements and Grant, 1990). In addition, local glutamatergic neurons were identified in the VTA (Yamaguchi et al., 2007; Morales and Root, 2014), which form functional synapses onto VTA dopaminergic neurons (Dobi et al., 2010; Wang et al., 2015). The sex-activated VTA neurons contain NMDARs, whose constitutive GluN1 subunit is phosphorylated in response to sexual behavior and sex-associated cues (Balfour et al., 2006). Thus, glutamatergic transmission at the VTA participates in the stimulation of the MSL system that takes place during sexual activity. Besides, it has been shown that the activation of NMDARs in this brain region is necessary to enable DA neurons to switch between tonic and phasic firing patterns (Sombers et al., 2009). Both, sexual cues and mating itself, trigger mesolimbic DA neuron phasic firing, resulting in an increase in DA release at the NAcc (Pfaus et al., 1990; Damsma et al., 1992; Wenkstern et al., 1993; Robinson et al., 2001). Together, these data show that glutamatergic synapses onto DA cell bodies in the VTA play an important role in the response of MSL DA neurons to sexual activity, a natural rewarding stimulus (Floresco et al., 2003).
In the present work, we provide evidence for changes in VTA glutamate receptor density and subunit composition that were detected in the sexually satiated rats 24 h after intense sexual activity. Thus, a decrease in the density of VTA AMPARs, concomitant to a significant rise in the expression of AMPARs containing the GluA2 subunit were found in the sexually satiated rats. Repeated activation of Ca2+- permeable AMPARs has been reported to produce a long-lasting switch in their subunit composition, from GluA2-lacking to GluA2-containing receptors (Liu and Cull-Candy, 2000). Thus, the increased expression of the GluA2 subunit found in the sexually satiated males, suggests that the proportion of GluA2-containing AMPARs was increased as a result of the repeated activation of VTA neurons by glutamate during copulation to satiety. It is well-established that the presence of the GluA2 subunit in AMPARs renders these receptors impermeable to Ca2+ and Zn2+ and slows the channel kinetics (Liu and Zukin, 2007). Therefore, the predominance of GluA2-containing AMPARs results in a reduction of synaptic activity. On the other side, the decrease in the number of AMPAR may result from their removal from the cell surface by internalization and their subsequent lysosomal degradation. This phenomenon is associated with a specific form of long-term plasticity: long-term depression (LTD) (Chater and Goda, 2014). LTD of excitatory synapses can be produced by a switch in the composition of AMPARs from GluA2-lacking to GluA2-containing receptors, which show a lower single channel conductance (Mameli et al., 2007), and requires a loss of AMPARs from the synapse (Chater and Goda, 2014). The decrease in AMPAR expression and the increase in GluA2-containing receptor expression observed in this work in the sexually satiated rats are in line with those producing LTD.
As to the NMDAR, its density in the VTA was increased in the sexually satiated animals as compared to males that ejaculated only once but was not importantly modified with respect to control animals, in contrast to the evident changes found in the expression of NMDAR subunits. In this last case, a significant decrease in the expression of the GluN2A subunit, concomitant to a significant increase in that of the GluN2B subunit were found in the VTA of the sexually exhausted males, suggesting that the subunit composition of NMDARs is changed. Such changes in NMDAR composition would be relevant, as the GluN2A subunit confers these receptors a lower affinity for glutamate, faster kinetics, and greater channel open probability (Lau and Zukin, 2007). Synaptic activity regulates the molecular composition of NMDARs in such a manner that there is a relative abundance of GluN2A containing receptors in active synapses, which shifts to a reduction relative to the abundance of GluN2B containing NMDARs in inactive synapses (Ehlers, 2003). Therefore, a presumed increase in the proportion of GluN2B-NMDARs, with slower kinetics and reduced channel open probability, with regard to GluN2A-NMDARs, characterized by a high channel open probability and rapid deactivation (Paoletti et al., 2013), would result in a reduced activity of the neurons in which these changes occur. It could be speculated that the changes in expression of NMDAR subunits found in the VTA of the sexually satiated rats, might indicate a reduced activity of VTA glutamatergic synapses during the sexual inhibitory state of these animals. An increase in GluN2B subunit expression was also found in the males that ejaculated once, suggesting that glutamatergic stimulation provided by mild sexual activity is sufficient to initiate changes in NMDAR subunit composition.
We found only one study investigating changes in the expression of glutamate ionotropic receptors associated with male sexual activity in the MSL system. In that work, an increase in NMDAR density without changes in the expression of GluA1 and GluA2 AMPAR subunits was found in the NAcc of male rats, 24 h after the last of five consecutive daily mating sessions involving one ejaculation per day (Pitchers et al., 2012). Together with present findings, these data show that a natural rewarding behavior like sexual activity can induce changes in glutamate receptor expression in the MSL system.
Sexually satiated male rats do not respond to the presence of a sexually receptive female 24 h after copulation to satiety. This is an unexpected behavior since, on the one side, these animals are proofed sexually competent rats that rested around 20 h after intense sexual activity when presented to the new sexual partner and, on the other, copulation is an instinctive behavior that should be triggered by an adequate sign stimulus: in this case, the sexually receptive female. Considering that glutamatergic stimulation at the VTA participates in changing the activity pattern of DA neurons toward phasic firing, a phenomenon that occurs in response to rewarding stimuli, changes in the subunit composition of NMDARs, might reduce or cancel the ability of DA neurons to produce this phasic firing, thereby contributing to the lack of response of the sexually satiated rats to the presence of a sexually receptive female. Though the neuronal composition of the VTA is heterogeneous and, in addition to DA neurons (65%), there is an important population of GABA neurons (around 30%) and a small proportion of glutamate neurons (around 5%) (Dobi et al., 2010; Morales and Margolis, 2017). These GABA neurons exhibit NMDAR-mediated plasticity (Nelson et al., 2018) and can therefore be both, targets of glutamate and contribute indirectly to the induction of changes in DA neuron firing activity (Bouarab et al., 2019).
One of the goals of the present study was to determine the possible participation of the eCBs released during copulation to satiety in the changes in glutamatergic transmission resulting from intense sexual activity. Our results show that the blockade of the actions of eCBs at CB1R during copulation to satiety, by the pretreatment with the CB1R antagonist AM251, interfered with the decrease in AMPAR density and blocked the changes in the expression of NMDAR subunits, but did not modify the changes in the subunit composition of AMPARs. The question emerges as to the mechanisms involved in these eCB-induced changes in glutamatergic receptors.
It has been shown that prolonged DA neuron depolarization within the VTA induces the release of eCBs, which activate CB1Rs at glutamatergic presynaptic terminals suppressing glutamatergic transmission onto DA neurons (Melis, 2004), through the short-term plasticity phenomenon called DSE (Kreitzer and Regehr, 2001). It has been considered that DSE plays a neuroprotective role against glutamate-induced excitotoxicity (Diana and Marty, 2004). The changes in VTA glutamatergic receptors reported here were detected particularly in the sexually satiated rats and are therefore consistent with a longer or repeated glutamatergic receptor stimulation. The eCBs have been found to produce also long-term plasticity phenomena, like long-term depression (eCB-LTD) of glutamatergic transmission (Robbe et al., 2002), requiring either long or repeated CB1R activation to be induced (Diana and Marty, 2004). This eCB-LTD has been observed in VTA DA neurons and is produced by a decrease in glutamate release, presumably exerted by 2-AG through the activation of CB1Rs (Haj-Dahmane and Shen, 2010). This form of plasticity is mechanistically different from the eCB-mediated mGluR-LTD, also described in the VTA (Bellone and Lüscher, 2005), in which the postsynaptic group I mGluR activation, triggers the synthesis and release of 2-AG, presumed to mediate a switch in AMPAR subunit composition to induce LTD. The excitatory synapses onto DA neurons of the VTA contain GluA2-lacking AMPARs and mGluR-LTD induces an increase in the intracellular calcium of DA neurons (Morikawa et al., 2003) leading to eCB release (Wilson and Nicoll, 2002) that depend on the switch from GluA2-lacking to GluA2-containing AMPARs. These two forms of LTD of glutamatergic synapses on DA neurons of the VTA coexist and might be part of the mechanisms contributing to the induction of the long-lasting physiological changes observed in sexually satiated rats. We have demonstrated that interfering with CB1R activation during sexual satiety development hinders the appearance of the long-lasting sexual behavior inhibition and drug hypersensitivity in rats that copulate to satiety (González-Morales and Rodríguez-Manzo, 2020). Blockade of CB1Rs during intense copulation could have interfered with eCB-LTD but not with mGluR-LTD, since the released glutamate would have activated mGluRs. Present data showing that CB1R blockade during copulation to satiety did not cancel the increase in GluA2-containing AMPARs support this notion.
Although eCB-mediated LTD plasticity phenomena may play a role in the induction of the long-lasting plastic changes that characterize sexually exhausted rats, their dependence on CB1R activation makes eCB-LTD the most likely candidate. Electrophysiological studies should be conducted to evaluate this possibility.
Extracellular signal-regulated receptor kinase (ERK) participates in the induction of some forms of LTD (Gallagher et al., 2004; Grueter et al., 2006; Kellogg et al., 2009) and cannabinoid receptors are known to activate ERK (Derkinderen et al., 2003); therefore, we decided to look for changes in ERK phosphorylation in the VTA of sexually satiated rats that might be related to CB1R activation. Our data failed to detect changes in pERK1/2 expression in the VTA of the copulating males; however, blockade of eCB actions during intense copulation resulted in an increase in VTA pERK1/2 expression in the sexually satiated males, suggesting that the released eCBs hinder the increase in the phosphorylation of this MAP kinase. Contrary to present results, CB1R-mediated ERK1/2 phosphorylation has been shown to occur and to participate in eCB-dependent LTD at inhibitory synapses (I-LTD) onto VTA DA neurons, as a result of repeated cocaine exposure (Pan et al., 2011). We could not find any data on CB1R-mediated ERK1/2 phosphorylation at excitatory synapses; however, it has been reported that sustained CB1R stimulation activates ERK1/2 only transiently and that the duration of ERK1/2 activation is regulated by CB1R desensitization (Daigle et al., 2008). However, in our study the observed increase in pERK1/2 expression in the AM251 pre-treated males did not involve CB1R activation but must have been the result of stimulating other type of receptors, for instance, NMDA glutamate receptors (Krapivinsky et al., 2003).
In sum, the results of the present work show that eCBs are released in the VTA in response to both mild and intense sexual activity; however, only in the rats that copulated to satiety did eCBs induce excitatory synaptic plasticity. These data suggest that the magnitude of eCB release was different in these two groups. Copulation to satiety appears to have released a larger amount of eCBs in the VTA, which impacted glutamatergic transmission presumably onto DA neurons, although VTA GABA neurons expressing glutamate receptors could also be a target. The nature of the glutamate receptor changes promoted by eCBs is consistent with a reduction in the excitatory effects of glutamatergic input, which might result in a reduced VTA DA and maybe also GABA neuron activity. The present work provides evidence for a physiological role of eCB release from VTA DA neurons in vivo, that might represent a protective mechanism against excessive activation produced by heightened natural rewarding stimulation.
Conclusion
Evidence implicates eCBs in the induction of the long-lasting physiological changes observed in sexually satiated rats: the sexual inhibition and generalized sensitization to drug actions. The MSL system, specifically the VTA, has been found to play a significant role in the control of these two phenomena (Canseco-Alba and Rodríguez-Manzo, 2014; González-Morales and Rodríguez-Manzo, 2020). CB1Rs on axon terminals synapsing onto DA neurons in this brain region are the target of these eCB-mediated actions and glutamatergic transmission appears to be a principal player. The results of the present series of experiments demonstrate that eCBs are released in the VTA during copulation to satiety and activate CB1Rs producing changes in glutamate receptors that are consistent with a reduction of glutamate excitatory effects onto DA and perhaps GABA neurons.
Data Availability Statement
The raw data supporting the conclusions of this article will be made available by the authors, without undue reservation.
Ethics Statement
The animal study was reviewed and approved by Comité Institucional para el Cuidado y Uso de Animales de Laboratorio (CICUAL) at CINVESTAV.
Author Contributions
GR-M conceived and designed the study and wrote the manuscript. EG-M and RG-G performed the experiments and participated in the drafting of the manuscript. EG-M prepared the figures. All authors analyzed the data, discussed the results, and commented on the manuscript.
Funding
This work was supported by a grant from the Consejo Nacional de Ciencia y Tecnología to GR-M [Grant 220772]. The data presented here were part of the Ph.D. dissertation of EG-M, who received a scholarship from CONACYT (number 339464).
Conflict of Interest
The authors declare that the research was conducted in the absence of any commercial or financial relationships that could be construed as a potential conflict of interest.
Publisher's Note
All claims expressed in this article are solely those of the authors and do not necessarily represent those of their affiliated organizations, or those of the publisher, the editors and the reviewers. Any product that may be evaluated in this article, or claim that may be made by its manufacturer, is not guaranteed or endorsed by the publisher.
Acknowledgments
We acknowledge the technical assistance of María de los Ángeles Ceja and thank her for animal housing and care.
References
Almada, M., Amaral, C., Diniz-Da-Costa, M., Correia-Da-Silva, G., Teixeira, N. A., and Fonseca, B. M. (2016). The endocannabinoid anandamide impairs in vitro decidualization of human cells. Reproduction 152, 351–361. doi: 10.1530/REP-16-0364
Atkin, G., Moore, S., Lu, Y., Nelson, R. F., Tipper, N., Rajpal, G., et al. (2015). Loss of F-box only protein 2 (Fbxo2) disrupts levels and localization of select NMDA receptor subunits, and promotes aberrant synaptic connectivity. J. Neurosc. 35, 6165–6178. doi: 10.1523/JNEUROSCI.3013-14.2015
Balfour, M. E., Brown, J. L., Yu, L., and Coolen, L. M. (2006). Potential contributions of efferents from medial prefrontal cortex to neural activation following sexual behavior in the male rat. Neuroscience 137, 1259–1276. doi: 10.1016/j.neuroscience.2005.11.013
Bellone, C., and Lüscher, C. (2005). mGluRs induce a long-term depression in the ventral tegmental area that involves a switch of the subunit composition of AMPA receptors. Eur. J. Neurosci. 21, 1280–1288. doi: 10.1111/j.1460-9568.2005.03979.x
Bouarab, C., Thompson, B., and Polter, A. M. (2019). VTA GABA neurons at the interface of stress and reward. Front. Neural Circuits 13:78. doi: 10.3389/fncir.2019.00078
Canseco-Alba, A., and Rodríguez-Manzo, G. (2014). Low anandamide doses facilitate male rat sexual behaviour through the activation of CB1 receptors. Psychopharmacology 231, 4071–4080. doi: 10.1007/s00213-014-3547-9
Canseco-Alba, A., and Rodríguez-Manzo, G. (2016). Intra-VTA anandamide infusion produces dose-based biphasic effects on male rat sexual behavior expression. Pharmacol. Biochem. Behav. 150–151, 182–189. doi: 10.1016/j.pbb.2016.11.004
Chater, T. E., and Goda, Y. (2014). The role of AMPA receptors in postsynaptic mechanisms of synaptic plasticity. Front. Cell. Neurosci. 8:401. doi: 10.3389/fncel.2014.00401
Chevaleyre, V., and Castillo, P. E. (2003). Heterosynaptic LTD of hippocampal GABAergic synapses: a novel role of endocannabinoids in regulating excitability. Neuron 38, 461–472. doi: 10.1016/S0896-6273(03)00235-6
Clements, J. R., and Grant, S. (1990). Glutamate-like immunoreactivity in neurons of the laterodorsal tegmental and pedunculopontine nuclei in the rat. Neurosci. Lett. 120, 70–73. doi: 10.1016/0304-3940(90)90170-E
Daigle, T. L., Kearn, C. S., and Mackie, K. (2008). Rapid CB1 cannabinoid receptor desensitization defines the time course of ERK1/2 MAP kinase signaling. Neuropharmacology 54, 36–44. doi: 10.1016/j.neuropharm.2007.06.005
Damsma, G., Pfaus, J., Wenkstern, D., Phillips, A., and Fibiger, H. (1992). Sexual behaviour increases dopamine transmission in the nucleus accumbens and striatum of male rats: comparison with novelty and locomotion. Behav. Neurosci. 106, 181–191. doi: 10.1037/0735-7044.106.1.181
Derkinderen, P., Valjent, E., Toutant, M., Corvol, J. C., Enslen, H., Ledent, C., et al. (2003). Regulation of extracellular signal-regulated kinase by cannabinoids in hippocampus. J. Neurosci. 23, 2371–2382. doi: 10.1523/JNEUROSCI.23-06-02371.2003
Diana, M. A., and Marty, A. (2004). Endocannabinoid-mediated short-term synaptic plasticity: depolarization-induced suppression of inhibition (DSI) and depolarization- induced suppression of excitation (DSE). Br. J. Pharmacol. 142, 9–19. doi: 10.1038/sj.bjp.0705726
Dobi, A., Margolis, E. B., Wang, H. L., Harvey, B. K., and Morales, M. (2010). Glutamatergic and nonglutamatergic neurons of the ventral tegmental area establish local synaptic contacts with dopaminergic and nondopaminergic neurons. J. Neurosci. 30, 218–229. doi: 10.1523/JNEUROSCI.3884-09.2010
Ehlers, M. D. (2003). Activity level controls postsynaptic composition and signaling via the ubiquitin-proteasome system. Nat. Neurosci. 6, 231–242. doi: 10.1038/nn1013
Everitt, B. J. (1990). Sexual motivation: a neural and behavioural analysis of the mechanisms underlying appetitive and copulatory responses of male rats. Neurosci. Biobehav. Rev. 14, 217–232. doi: 10.1016/S0149-7634(05)80222-2
Fiorino, D. F., Coury, A., and Phillips, A. G. (1997). Dynamic changes in nucleus accumbens dopamine efflux during the coolidge effect in male rats. J. Neurosci. 17, 4849–4855. doi: 10.1523/JNEUROSCI.17-12-04849.1997
Floresco, S. B., West, A. R., Ash, B., Moorel, H., and Grace, A. A. (2003). Afferent modulation of dopamine neuron firing differentially regulates tonic and phasic dopamine transmission. Nat. Neurosci. 6, 968–973. doi: 10.1038/nn1103
Gallagher, S. M., Daly, C. A., Bear, M. F., and Huber, K. M. (2004). Extracellular signal-regulated protein kinase activation is required for metabotropic glutamate receptor-dependent long-term depression in hippocampal area CA1. J. Neurosci. 24, 4859–4864. doi: 10.1523/JNEUROSCI.5407-03.2004
Garduño-Gutiérrez, R., León-Olea, M., and Rodríguez-Manzo, G. (2013). Different amounts of ejaculatory activity, a natural rewarding behavior, induce differential mu and delta opioid receptor internalization in the rat's ventral tegmental area. Brain Res. 1541, 22–32. doi: 10.1016/j.brainres.2013.10.015
Gerdeman, G. L., Ronesi, J., and Lovinger, D. M. (2002). Postsynaptic endocannabinoid release is critical to long-term depression in the striatum. Nat. Neurosci. 5, 446–451. doi: 10.1038/nn832
González-Morales, E., and Rodríguez-Manzo, G. (2020). Endocannabinoids mediate long-lasting behavioural and physiological changes in male rats induced by the repeated activation of the mesolimbic system by copulation to satiety. Behav. Brain Res. 383:112510. doi: 10.1016/j.bbr.2020.112572
Grueter, B. A., Gosnell, H. B., Olsen, C. M., Schramm-Sapyta, N. L., Nekrasova, T., Landreth, G. E., et al. (2006). Extracellular-signal regulated kinase 1-dependent metabotropic glutamate receptor 5-induced long-term depression in the bed nucleus of the stria terminalis is disrupted by cocaine administration. J. Neurosci. 26, 3210–3219. doi: 10.1523/JNEUROSCI.0170-06.2006
Gyombolai, P., Boros, E., Hunyady, L., and Turu, G. (2013). Differential β-arrestin2 requirements for constitutive and agonist-induced internalization of the CB1 cannabinoid receptor. Mol. Cell. Endocrinol. 372, 116–127. doi: 10.1016/j.mce.2013.03.013
Haj-Dahmane, S., and Shen, R. Y. (2010). Regulation of plasticity of glutamate synapses by endocannabinoids and the cyclic-AMP/protein kinase A pathway in midbrain dopamine neurons. J. Physiol. 588, 2589–2604. doi: 10.1113/jphysiol.2010.190066
Hayn, M. H., Ballesteros, I., de Miguel, F., Coyle, C. H., Tyagi, S., Yoshimura, N., et al. (2008). Functional and immunohistochemical characterization of CB1 and CB2 receptors in rat bladder. Urology 72, 1174–1178. doi: 10.1016/j.urology.2008.03.044
Hernandez-Gonzalez, M., Guevara, M. A., Morali, G., and Cervantes, M. (1997). Subcortical multiple unit activity changes during rat male sexual behavior. Physiol. Behav. 61, 285–291. doi: 10.1016/S0031-9384(96)00367-8
Hill, M. N., Patel, S., Carrier, E. J., Rademacher, D. J., Ormerod, B. K., Hillard, C. J., et al. (2005). Downregulation of endocannabinoid signaling in the hippocampus following chronic unpredictable stress. Neuropsychopharmacology 30, 508–515. doi: 10.1038/sj.npp.1300601
Hillard, C. J. (2014). Stress regulates endocannabinoid-CB1 receptor signaling. Semin. Immunol. 26, 380–388. doi: 10.1016/j.smim.2014.04.001
Hsieh, C., Brown, S., Derleth, C., and Mackie, K. (1999). Internalization and recycling of the CB1 cannabinoid receptor. J. Neurochem. 73, 493–501. doi: 10.1046/j.1471-4159.1999.0730493.x
Ikemoto, S. (2007). Dopamine reward circuitry: two projection systems from the ventral midbrain to the nucleus accumbens-olfactory tubercle complex. Brain Res. Rev. 56, 27–78. doi: 10.1016/j.brainresrev.2007.05.004
Kelley, A. E., and Berridge, K. C. (2002). The neuroscience of natural rewards: relevance to addictive drugs. J. Neurosci. 22, 3306–3311. doi: 10.1523/JNEUROSCI.22-09-03306.2002
Kellogg, R., Mackie, K., and Straiker, A. (2009). Cannabinoid CB1 receptor-dependent long-term depression in autaptic excitatory neurons. J. Neurophysiol. 102, 1160–1171. doi: 10.1152/jn.00266.2009
Krapivinsky, G., Krapivinsky, L., Manasian, Y., Ivanov, A., Tyzio, R., Pellegrino, C., et al. (2003). The NMDA receptor is coupled to the ERK pathway by a direct interaction between NR2B and RasGRF1. Neuron 40, 775–784. doi: 10.1016/S0896-6273(03)00645-7
Kreitzer, A. C., and Regehr, W. G. (2001). Retrograde inhibition of presynaptic calcium influx by endogenous cannabinoids at excitatory synapses onto Purkinje cells. Neuron 29, 717–727. doi: 10.1016/S0896-6273(01)00246-X
Lai, T. K. Y., Zhai, D., Su, P., Jiang, A., Boychuk, J., and Liu, F. (2019). The receptor-receptor interaction between mGluR1 receptor and NMDA receptor: a potential therapeutic target for protection against ischemic stroke. FASEB J. 33, 14423–14439. doi: 10.1096/fj.201900417R
Lau, C. G., and Zukin, R. S. (2007). NMDA receptor trafficking in synaptic plasticity and neuropsychiatric disorders. Nat. Rev. Neurosci. 8, 413–426. doi: 10.1038/nrn2153
Li, R., Huang, Z., Luo, J., Luo, H., and Wang, W. (2020). Downregulation of the CB1-mediated endocannabinoid signaling underlies D-Galactose-induced memory impairment. Front. Mol. Neurosci. 13:130. doi: 10.3389/fnmol.2020.00130
Lin, H. C., Tseng, Y. C., Mao, S. C., Chen, P. S., and Gean, P. W. (2011). GABAA receptor endocytosis in the basolateral amygdala is critical to the reinstatement of fear memory measured by fear-potentiated startle. J. Neurosci. 31, 8851–8861. doi: 10.1523/JNEUROSCI.0979-11.2011
Liu, S. J., and Zukin, R. S. (2007). Ca2+-permeable AMPA receptors in synaptic plasticity and neuronal death. Trends Neurosci. 30, 126–134. doi: 10.1016/j.tins.2007.01.006
Liu, S. Q. J., and Cull-Candy, S. G. (2000). Synaptic activity at calcium-permeable AMPA receptors induces a switch in receptor subtype. Nature 405, 454–458. doi: 10.1038/35013064
Lowry, O. H., Rosebrough, N. J., Farr, A. L., and Randall, R. J. (1951). Protein measurement with the Folin phenol reagent. J. Biol. Chem. 193, 265–275. doi: 10.1016/S0021-9258(19)52451-6
Ludányi, A., Eross, L., Czirják, S., Vajda, J., Halász, P., Watanabe, M., et al. (2008). Downregulation of the CB1 cannabinoid receptor and related molecular elements of the endocannabinoid system in epileptic human hippocampus. J. Neurosci. 28, 2976–2990. doi: 10.1523/JNEUROSCI.4465-07.2008
Lupica, C. R., and Riegel, A. C. (2005). Endocannabinoid release from midbrain dopamine neurons: a potential substrate for cannabinoid receptor antagonist treatment of addiction. Neuropharmacology, 48, 1105–1116. doi: 10.1016/j.neuropharm.2005.03.016
Mameli, M., Balland, B., Luján, R., and Lüscher, C. (2007). Rapid synthesis and synaptic insertion of GluR2 for mGluR-LTD in the ventral tegmental area. Science 317, 530–533. doi: 10.1126/science.1142365
Matsumoto, J., Urakawa, S., Hori, E., de Araujo, M. F. P., Sakuma, Y., Ono, T., et al. (2012). Neuronal responses in the nucleus accumbens shell during sexual behavior in male rats. J. Neurosci. 32, 1672–1686. doi: 10.1523/JNEUROSCI.5140-11.2012
Melis, M. (2004). Endocannabinoids mediate presynaptic inhibition of glutamatergic transmission in rat ventral tegmental area dopamine neurons through activation of CB1 receptors. J. Neurosci. 24, 53–62. doi: 10.1523/JNEUROSCI.4503-03.2004
Melis, M. R., and Argiolas, A. (1995). Dopamine and sexual behavior. Neurosci. Biobehav. Rev. 19, 19–38. doi: 10.1016/0149-7634(94)00020-2
Miller, L. K., and Devi, L. A. (2011). The highs and lows of cannabinoid receptor expression in disease: mechanisms and their therapeutic implications. Pharmacol. Rev. 63, 461–470. doi: 10.1124/pr.110.003491
Mitchell, J. B., and Gratton, A. (1994). Involvement of mesolimbic dopamine neurons in sexual behaviors: implications for the neurobiology of motivation. Rev. Neurosci. 5, 317–330. doi: 10.1515/REVNEURO.1994.5.4.317
Moore, C. A. C., Milano, S. K., and Benovic, J. L. (2007). Regulation of receptor trafficking by GRKs and arrestins. Annu. Rev. Physiol. 69, 451–482. doi: 10.1146/annurev.physiol.69.022405.154712
Morales, M., and Margolis, E. B. (2017). Ventral tegmental area: cellular heterogeneity, connectivity and behaviour. Nat. Rev. Neurosci. 18, 73–85. doi: 10.1038/nrn.2016.165
Morales, M., and Root, D. H. (2014). Glutamate neurons within the midbrain dopamine regions. Neuroscience 282, 60–68. doi: 10.1016/j.neuroscience.2014.05.032
Morikawa, H., Khodakhah, K., and Williams, J. T. (2003). Two intracellular pathways mediate metabotropic glutamate receptor-induced Ca2+ mobilization in dopamine neurons. J. Neurosci. 23, 149–157. doi: 10.1523/JNEUROSCI.23-01-00149.2003
Nelson, A. C., Williams, S. B., Pistorius, S. S., Park, H. J., Woodward, T. J., Payne, A. J., et al. (2018). Ventral tegmental area GABA neurons are resistant to GABA(A) receptor-mediated inhibition during ethanol withdrawal. Front. Neurosci. 12:131. doi: 10.3389/fnins.2018.00131
Nogueras-Ortiz, C., and Yudowski, G. A. (2016). The multiple waves of cannabinoid 1 receptor signaling. Mol. Pharmacol. 90, 620–626. doi: 10.1124/mol.116.104539
Ohno-Shosaku, T., Maejima, T., and Kano, M. (2001). Endogenous cannabinoids mediate retrograde signals from depolarized postsynaptic neurons to presynaptic terminals. Neuron 29, 729–738. doi: 10.1016/S0896-6273(01)00247-1
Omelchenko, N., and Sesack, S. R. (2007). Glutamate synaptic inputs to ventral tegmental area neurons in the rat derive primarily from subcortical sources. Neuroscience 146, 1259–1274. doi: 10.1016/j.neuroscience.2007.02.016
Pan, B., Zhong, P., Sun, D., and Liu, Q.-S. (2011). Extracellular signal-regulated kinase signaling in the ventral tegmental area mediates cocaine-induced synaptic plasticity and rewarding effects. J. Neurosci. 31, 11244–11255. doi: 10.1523/JNEUROSCI.1040-11.2011
Paoletti, P., Bellone, C., and Zhou, Q. (2013). NMDA receptor subunit diversity: impact on receptor properties, synaptic plasticity and disease. Nat. Rev. Neurosci. 14, 383–400. doi: 10.1038/nrn3504
Paxinos, G., and Watson, C. (2009). The Rat Brain in Stereotaxic Coordinates, 6th Edn. Cambridge, MA: Academic Press.
Peterson, G. L. (1979). Review of the folin phenol protein quantitation method of Lowry, Rosebrough, Farr, and Randall. Anal. Biochem. 100, 201–220. doi: 10.1016/0003-2697(79)90222-7
Pfaus, J. G. (2009). Pathways of sexual desire. J. Sex. Med. 6, 1506–1533. doi: 10.1111/j.1743-6109.2009.01309.x
Pfaus, J. G., Damsma, G., Nomikos, G. G., Wenkstern, D. G., Blaha, C. D., Phillips, A. G., et al. (1990). Sexual behavior enhances central dopamine transmission in the male rat. Brain Res. 530, 345–348. doi: 10.1016/0006-8993(90)91309-5
Pitchers, K. K., Schmid, S., Di Sebastiano, A. R., Wang, X., Laviolette, S. R., Lehman, M. N., et al. (2012). Natural reward experience alters AMPA and NMDA receptor distribution and function in the nucleus accumbens. PLoS ONE 7:e34700. doi: 10.1371/journal.pone.0034700
Riegel, A. C., and Lupica, C. R. (2004). Independent presynaptic and postsynaptic mechanisms regulate endocannabinoid signaling at multiple synapses in the ventral tegmental area. J. Neurosci. 24, 11070–11078. doi: 10.1523/JNEUROSCI.3695-04.2004
Robbe, D., Kopf, M., Remaury, A., Bockaert, J., and Manzoni, O. J. (2002). Endogenous cannabinoids mediate long-term synaptic depression in the nucleus accumbens. Proc. Nat. Acad. Sci. U.S.A. 99, 8384–8388. doi: 10.1073/pnas.122149199
Robinson, D. L., Phillips, P. E. M., Budygin, E. A., Trafton, B. J., Garris, P. A., and Wightman, R. M. (2001). Sub-second changes in accumbal dopamine during sexual behavior in male rats. Neuroreport 12, 2549–2552. doi: 10.1097/00001756-200108080-00051
Rodríguez-Manzo, G., and Fernández-Guasti, A. (1994). Reversal of sexual exhaustion by serotonergic and noradrenergic agents. Behav. Brain Res. 62, 127–134. doi: 10.1016/0166-4328(94)90019-1
Rodríguez-Manzo, G., Guadarrama-Bazante, I. L., and Morales-Calderón, A. (2011). Recovery from sexual exhaustion-induced copulatory inhibition and drug hypersensitivity follow a same time course: two expressions of a same process? Behav. Brain Res. 217, 253–260. doi: 10.1016/j.bbr.2010.09.014
Scherma, M., Qvist, J. S., Asok, A., Huang, S. S. C., Masia, P., Deidda, M., et al. (2020). Cannabinoid exposure in rat adolescence reprograms the initial behavioral, molecular, and epigenetic response to cocaine. Proc. Natl. Acad. Sci. U.S.A. 117, 9991–10002. doi: 10.1073/pnas.1920866117
Sim-Selley, L. J. (2003). Regulation of cannabinoid CB1 receptors in the central nervous system by chronic cannabinoids. Crit. Rev. Neurobiol. 15, 91–119. doi: 10.1615/CritRevNeurobiol.v15.i2.10
Sombers, L. A., Beyene, M., Carelli, R. M., and Wightman, R. M. (2009). Synaptic overflow of dopamine in the nucleus accumbens arises from neuronal activity in the ventral tegmental area. J. Neurosci. 29, 1735–1742. doi: 10.1523/JNEUROSCI.5562-08.2009
Song, I., and Huganir, R. L. (2002). Regulation of AMPA receptors during synaptic plasticity. Trends Neurosci. 25, 578–588. doi: 10.1016/S0166-2236(02)02270-1
Szabo, B., Siemes, S., and Wallmichrath, I. (2002). Inhibition of GABAergic neurotransmission in the ventral tegmental area by cannabinoids. Eur. J. Neurosci. 15, 2057–2061. doi: 10.1046/j.1460-9568.2002.02041.x
Travaglia, A., Bisaz, R., Cruz, E., and Alberini, C. M. (2016). Developmental changes in plasticity, synaptic, glia and connectivity protein levels in rat dorsal hippocampus. Neurobiol. Learn. Mem. 135, 125–138. doi: 10.1016/j.nlm.2016.08.005
Tzschentke, T. M. (2001). Pharmacology and behavioral pharmacology of the mesocortical dopamine system. Progr. Neurobiol. 63, 241–320. doi: 10.1016/S0301-0082(00)00033-2
Van Laere, K., Casteels, C., Lunskens, S., Goffin, K., Grachev, I. D., Bormans, G., et al. (2012). Regional changes in type 1 cannabinoid receptor availability in Parkinson's disease in vivo. Neurobiol. Aging 33, 620.e1–620.e8. doi: 10.1016/j.neurobiolaging.2011.02.009
Wang, H., Treadway, T., Covey, D. P., Cheer, J. F., and Lupica, C. R. (2015). Cocaine-induced endocannabinoid mobilization in the ventral tegmental area. Cell Rep. 12, 1997–2008. doi: 10.1016/j.celrep.2015.08.041
Wenkstern, D., Pfaus, J. G., and Fibiger, H. C. (1993). Dopamine transmission increases in the nucleus accumbens of male rats during their first exposure to sexually receptive female rats. Brain Res. 618, 41–46. doi: 10.1016/0006-8993(93)90426-N
Wilson, R. I., and Nicoll, R. A. (2001). Endogenous cannabinoids mediate retrograde signalling at hippocampal synapses. Nature 410, 588–592. doi: 10.1038/35069076
Wilson, R. I., and Nicoll, R. A. (2002). Endocannabinoid signaling in the brain. Science 296, 678–682. doi: 10.1126/science.1063545
Xin, W., Edwards, N., and Bonci, A. (2016). VTA dopamine neuron plasticity—the unusual suspects. Eur. J. Neurosci. 44, 2975–2983. doi: 10.1111/ejn.13425
Xu, A. L., Zheng, G. Y., Ye, H. Y., Chen, X. D., and Jiang, Q. (2020). Characterization of astrocytes and microglial cells in the hippocampal CA1 region after transient focal cerebral ischemia in rats treated with Ilexonin A. Neural Regen. Res. 15, 78–85. doi: 10.4103/1673-5374.264465
Yamaguchi, T., Sheen, W., and Morales, M. (2007). Glutamatergic neurons are present in the rat ventral tegmental area. Eur. J. Neurosci. 25, 106–118. doi: 10.1111/j.1460-9568.2006.05263.x
Keywords: endocannabinoids, CB1 receptors, AMPA and NMDA glutamate receptors, mesolimbic circuit, ventral tegmental area, synaptic plasticity, sexual satiety, natural reward
Citation: Rodríguez-Manzo G, González-Morales E and Garduño-Gutiérrez R (2021) Endocannabinoids Released in the Ventral Tegmental Area During Copulation to Satiety Modulate Changes in Glutamate Receptors Associated With Synaptic Plasticity Processes. Front. Synaptic Neurosci. 13:701290. doi: 10.3389/fnsyn.2021.701290
Received: 27 April 2021; Accepted: 08 July 2021;
Published: 18 August 2021.
Edited by:
Jeffrey G. Edwards, Brigham Young University, United StatesReviewed by:
Ryan David Shepard, National Institute of Neurological Disorders and Stroke (NINDS), United StatesHumberto Salgado, Universidad Autónoma de Yucatán, Mexico
Copyright © 2021 Rodríguez-Manzo, González-Morales and Garduño-Gutiérrez. This is an open-access article distributed under the terms of the Creative Commons Attribution License (CC BY). The use, distribution or reproduction in other forums is permitted, provided the original author(s) and the copyright owner(s) are credited and that the original publication in this journal is cited, in accordance with accepted academic practice. No use, distribution or reproduction is permitted which does not comply with these terms.
*Correspondence: Gabriela Rodríguez-Manzo, grodrigu@cinvestav.mx