- 1Liverpool Hope Business School, Liverpool Hope University, Liverpool, United Kingdom
- 2Tyndall Centre for Climate Change Research, University of East Anglia, Norwich, United Kingdom
- 3Tyndall Centre for Climate Change Research, School of Engineering, University of Manchester, Manchester, United Kingdom
- 4Hunter Centre for Entrepreneurship, Strathclyde Business School, University of Strathclyde, Glasgow, United Kingdom
- 5Department of Sociology, University of Edinburgh, Edinburgh, United Kingdom
- 6Departamento de Ingeniería Industrial, Universidad de Santiago de Chile, Santiago, Chile
Smart and local energy systems (SLES) offer an alternative to large-scale energy provision and a boost to the transition towards a low-carbon economy. However, the Business Model Canvas (BMC), an increasingly used framework for analyzing SLES, does not adequately describe the multiple value streams that can characterize the business models of numerous SLES projects. In this research, we modify and use the triple-layered BMC to analyze 18 SLES projects selected globally. The decarbonisation, digitalization and democratization associated with SLES, means that the value proposition extends beyond straightforward economic value and towards environmental and social value, and we find a wide reporting gap of environmental and social elements. We argue that the triple-layered BMC, which incorporates the environmental and social layers into its analysis, better reflects real-world business model complexity. We provide a common framework to SLES stakeholders for identifying and adding environmental and social value to their business models.
1. Introduction
Around the world, Smart Local Energy Systems (SLES) are often talked of as a key component of energy systems of the future for satisfying flexible and affordable energy requirements while providing a secure supply through sustainable renewable sources (O'Dwyer et al., 2019; Ceglia et al., 2020). However, there is considerable variety in how the idea of SLES is being put into practice. This variety is not only in terms of scale and components, but also relates to what benefits the SLES is supposed to bring, and to whom; and also, quite fundamentally, how “smart” and “local” are understood. On the one hand, some of the technological elements of these systems are now well established, e.g., renewable electricity generation. In other areas, such as low carbon transport, data systems, heat in buildings, local electricity storage and load flexibility, there is a rapid development of technologies which enable the distributed generation, supply, and storage, to be applied within variable demand settings. However, the business models—that is, the set of practices and relationships through which an organization “creates, delivers and captures value” (Osterwalder and Pigneur, 2010)—through which these technologies will be deployed are still emerging and uncertain. Which business models are eventually adopted and become widespread will be critical to determining what value is created, and for whom, in the transition (Ford et al., 2019).
The Business Model Canvas (BMC) (Osterwalder and Pigneur, 2010) is a well-established tool for analyzing business models, and it is increasingly used to analyze smart or local energy systems (Li and Song, 2019; Braunholtz-Speight et al., 2020; Reis et al., 2021). However, the standard BMC's focus on conventional economic value propositions is limiting, especially for analyzing Smart Local Energy Systems (SLES) as part of the energy transition. Therefore, as the energy transition will depend on integrated economic, environmental and social criteria (Biresselioglu et al., 2020), in this study we utilize the Triple Layered BMC (TL-BMC) to conduct our analysis (Joyce and Paquin, 2016). This approach adds environmental and social layers to the standard economic analysis of the “single-layer” economic BMC.
While decarbonization is a significant driver of the energy transition, different renewable and smart technologies can have very different carbon footprints (Jones et al., 2020); and some SLES, such as the Sino-Singapore Tianjin Eco-city project, may make limited use of renewable energy generation (Hu et al., 2015; Xu et al., 2015). Energy systems also have environmental impacts in other ways, e.g., water and air pollution (Tsoutsos et al., 2005; Alvarez-Herranz et al., 2017), soil degradation (Sayed et al., 2021), land contamination (Feron, 2016), and reduced biodiversity (Dhar et al., 2020). The environmental layer of the TL-BMC points directly to such issues from the business model conception stage.
Regarding social aspects, energy systems are socio-technical systems (Geels and Raven, 2007); they are created by and for people. Questions such as who controls them (Szulecki, 2018), who benefits from them (Hiteva and Sovacool, 2017; Powells and Fell, 2019), or how their operation interacts with other social processes (Pohlmann, 2019; Judson et al., 2020) such as social justice (Jenkins et al., 2016), increased standards in health (Akella et al., 2009) and welfare (Sovacool and Dworkin, 2015), are therefore core to understanding energy systems and the energy transition (Sovacool, 2014).
The social and the environmental layers are intertwined, as changes in behavior, e.g., around travel and consumption patterns, are likely to be critical for successful decarbonization (McLoughlin et al., 2019). How SLES business models interact with these patterns is therefore essential to understand. In sum, an analytical approach that can incorporate all three elements of the “triple bottom line”—of financial, environmental and social value and impacts—is needed.
This paper aims to analyze economic, environmental and social elements of selected benchmark SLES found globally, following the application of the Triple Layer Business Model Canvas (TL-BMC) framework as the analysis tool. Through the TL-BMC analysis for SLES projects, we highlight areas where their business models, or reporting thereof, need to improve their environmental, social and economic impacts. While the economic layer provided by this research does not deviate extensively from other single-layer BMC energy-themed analyses (Braunholtz-Speight et al., 2020; Wu et al., 2020; Hamwi et al., 2021), triple-layered analyses remain either extremely limited (e.g., Lin et al., 2018; focusing on the EV battery business), or unexplored in the case of SLES. We adapt and extend the TL-BMC to SLES by introducing new elements to correspond to the operations of energy projects as distinct from other business sectors, and adding analysis of “smart” and “local” aspects, linked to the TL-BMC social layer.
We analyze 18 SLES projects around the world. Given the emerging state of the sector, we examine a range of different systems, including pilots and demonstration projects and established commercial operations in our selection of cases. Specifically, we ask: what are the most common patterns of economic, environmental and social elements in existing SLES projects? What kind of value do these projects create, for whom? How far does the existing information on these projects capture the potential of SLES, as revealed in a triple-layered approach to understanding business models?
We argue that social and environmental elements remain largely under-reported for most of the selected projects. Therefore, we suggest that a TL-BMC approach could be used to improve reporting and knowledge of the impacts and benefits of SLES, potentially strengthening arguments for their further deployment.
The rest of the paper is structured as follows. Firstly, in Section 2 we outline the TL-BMC, set out our approach to SLES case study selection, and present the empirical examples which we analyze in the paper. In Section 3, we report the results of our analysis of case study projects, covering the economic, environmental and social elements of business models in turn, and use of ‘smart’ and ‘local’. Our discussion, in Section 4, highlights both particular patterns in the results and the general under-reporting of key elements in the environmental and social layers of the TLBMC. Finally in Section 5, we conclude with appropriate suggestions for future SLES projects and their utilization of the TL-BMC for future sector business model development.
2. Materials and methods
A set requirement for a suitable analytical framework which describes sustainable business models for SLES is its ability to contain the aspects of smartness, localness, two-way customer involvement, and energy security of supply in its elements. These aspects are either systemic parts of SLES, or pursued output fundamental to the business model and ensuring SLES seamless operation.
The environmental and social layers of the TL-BMC expand the analysis, taking the ‘single layer’ BMC framework, as conceptualized by Osterwalder and Pigneur (2010), beyond the economic value proposition. While the BMC is a framework that has been used to analyze SLES before (Matusiak, 2016; Dobrowolski and Sułkowski, 2021), the TL-BMC aims to find social and environmental value, critical themes for SLES. Therefore, the latter is an analytical framework that we consider capable of capturing complete SLES business models, by introducing layers that can be overlooked when solely focusing on monetary value.
The social layer of the TL-BMC framework includes energy system aspects such as local communities, employees, end-users, societal culture, scale of outreach, and governance which can act as direct and/or indirect descriptors of localness. The elements of the customer relationship, use phase, and channels act as descriptors of customer involvement, either one-directional from the system services provided to the end-user, or bi-directional: the end-user being involved in making decisions about system operation. Smartness, as technology or customer-centric decision-making, can be described in the TL-BMC elements of channels, activities, resources, and distribution found across the layers. At the same time, the security of energy supply is an integrated part of supplies and outsourcing, production, materials, distribution, and resources of the economic and environmental layers.
The TL-BMC framework demands greater availability of information, adding to the complexity of analysis. Contrasting the increased reporting requirements that better inform the framework, the present research chooses to use and appropriately adjust the TL-BMC by Joyce and Paquin (2016) as the analytical framework. Given the amount of data required to complete a TL-BMC analysis, the framework choice necessitates a shift from the exclusive utilization of academic literature to gray literature as an additional resource, to produce a systematic review using standardized TL-BMC elements and options. We consider the TL-BMC to produce a complete view of SLES features and value to stakeholders and participants.
2.1. Identifying and shortlisting SLES projects
The schematic process for determining the selection of SLES projects to be analyzed is presented in Figure 1. This conceptual framework is followed by detailed description of the methodological steps.
A pilot search was conducted in Scopus, using the terms “Smart OR Local Energy Systems” to acquire a sample of SLES related papers for import into the litsearchr package in R (Grames et al., 2019). The package allowed the production of keywords for a Scopus search following the parameters in Table 1. The bibliographical list was exported from the search engines in an RIS format and imported into Mendeley. Duplicates (11 studies) were removed. The terms; “Case Study; Local Energy,” “Business; Local Energy,” “Community; Local Energy,” “Smart Local Energy Systems” were then used for further filtering the list, removing 17 studies in areas not relevant to this study. The themes removed were focused on healthcare, entrepreneurship, theoretical mathematics, oil and energy. Three of those removed were working papers that had not been excluded by the search string used in Table 2. The resulting list limited the number of studies in the sample to a total of 268.
With the inclusion of gray literature, the volume of TL-BMC elements that could be filled out tripled per SLES project. From the literature sample, projects were filtered to satisfy the selection criteria:
• Energy focused.
• Be local (operational coverage).
• Be smart (enhanced input or operation).
• Access to literature.
• Variable geographical location.
Eighteen projects satisfying the criteria were included in the final selection (see Table 3). We chose to exclude projects that exclusively aim to provide heating as a standalone offering, and focus on electricity generation, distribution and consumption modes.
Data extracted from the academic and gray literature included bibliographical information, research questions set by the source, where applicable, the method of approach, and the conclusions and outcomes.
The extraction of triple Business Model Canvas elements follows the work of Lin et al. (2018) analysis of an EV battery industry. At the time of writing, the aforementioned article was the sole resource that had applied the framework to analysis of an energy system. All data included in the spreadsheet are direct quotations from the literature sources used.
The smartness and localness columns in the data spreadsheet explore the elements used in the SLES, following the definitions provided by Ford et al. (2021) and Fuentes González et al. (2021). The elements describing innovation aim to include technologies implemented in the SLES. Innovation can be linked to a technology, e.g., blockchain, or to a technical capability of the system, e.g., islanded mode per grid requirements.
2.2. Adapting the TL-BMC to analyze SLES projects
The framework adaptation used for analysis of SLES is presented in Figure 2 with altered elements described in Table 4. Each TL-BMC layer indicates horizontal coherence, or an integrated approach to investigating an organization's economic, environmental, or social effect. In addition, the three layers are combined to generate vertical coherence by linking the components of each layer to their analogs in the other layers, explaining essential actions and relationships and their repercussions across layers.
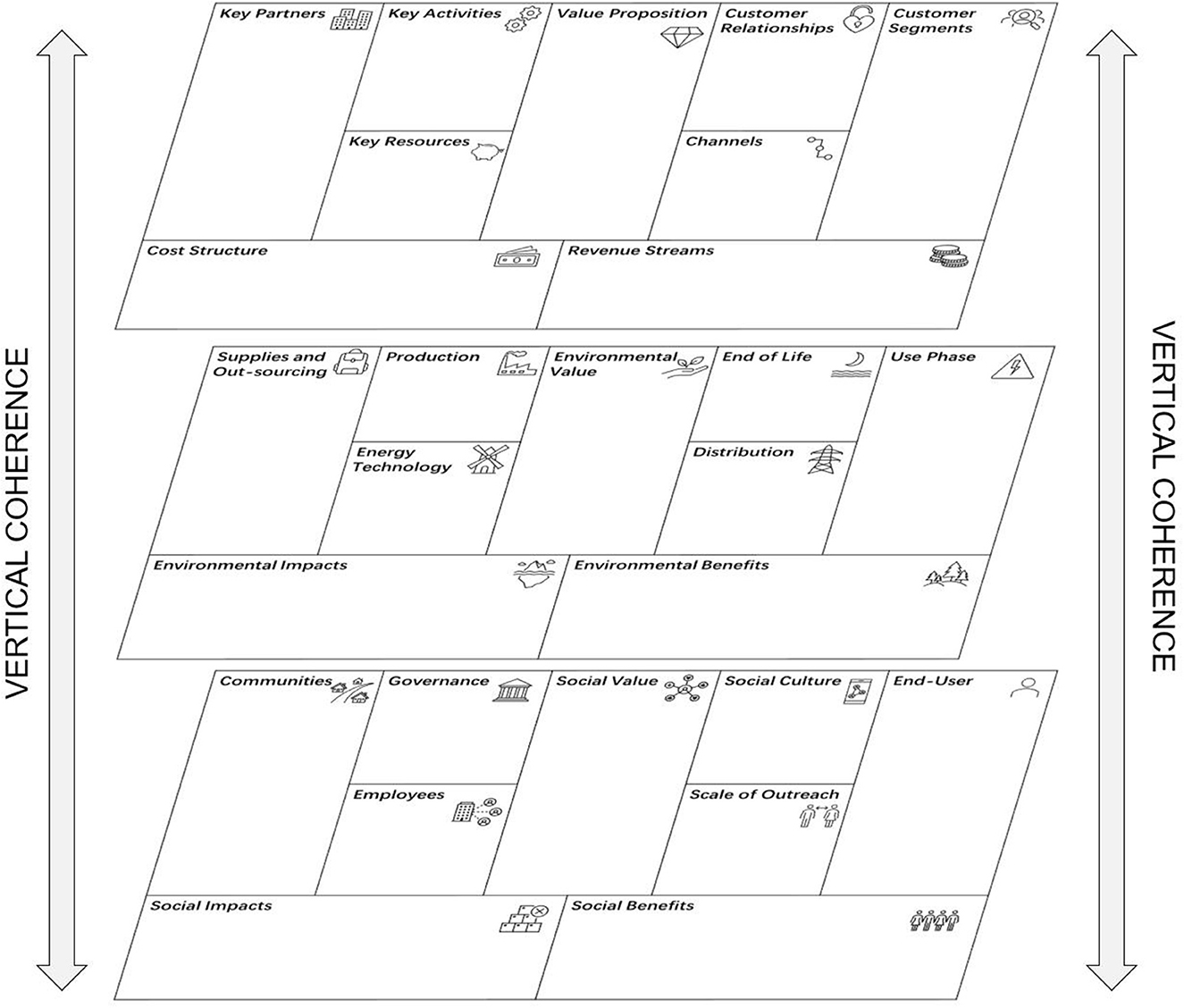
Figure 2. The TL-BMC with left and right arrows signifying vertical coherence across building blocks.
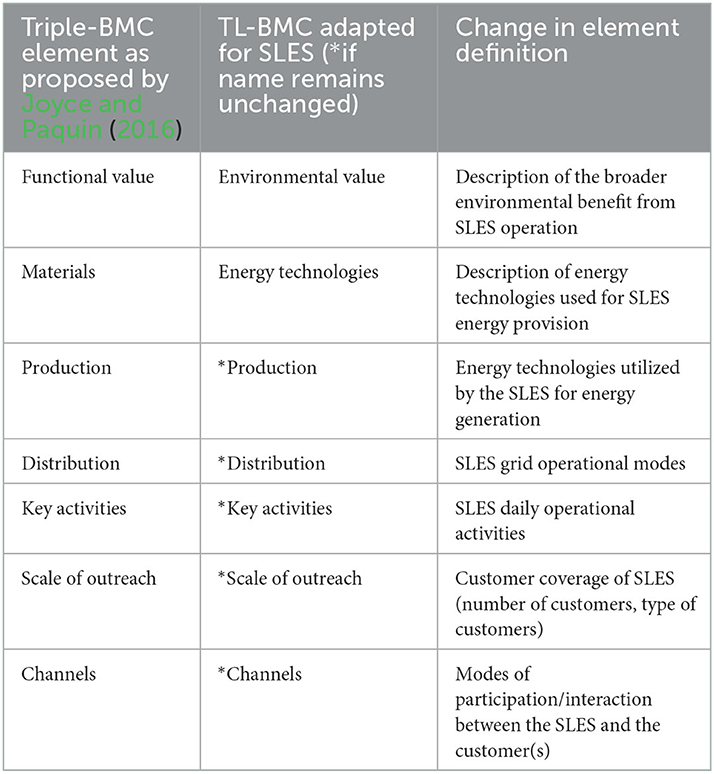
Table 4. Comparison of the TL-BMC as proposed by Joyce and Paquin (2016), and the TL-BMC adapted for SLES.
The main adjustment of the TL-BMC for the energy sector concerns the vertical coherence of blocks placed in matched positions across the economic, environmental, and social layers. The vertical coherence illustrates the adaption of the TL-BMC to cover modes of SLES project operation and participation. The coherence can be demonstrated as an example by looking at the consumer, found in the economic layer, as involved in the environmental layer use phase as part of the SLES operation, and is subsequently described as the end-user of the social layer. Further clarifying this adaptation to the TL-BMC, electricity replaces physical goods as the product, and through the electricity grid, alters the physical distribution element for transport, to include the end-customer. Table 4 provides the changes in the TL-BMC elements and descriptors, which we consider as a requirement for SLES adaptation.
3. Results
The results illustrate the application of the adapted TL-BMC analysis to the 18 SLES projects. A detailed example of the analysis is provided for the Kitakyushu project in Japan; this is an innovative project with sufficient information to complete the majority of TL-BMC elements. A schematic representation of economic, environmental and social layers of the TL-BMC is given, before discussing localness and smartness.
The TL-BMC adaptation ultimately aims at integrating the three layers for the selected SLES projects. The analysis presents the findings and discusses the reported information and trends found across the projects. The example project tests the fit of TL-BMC to SLES and, through adaptation where appropriate, provides the benchmark for functional, sustainable business models for SLES, characterized by simplicity in its communication among experts and non-experts. The approach of fitting an example project to the TL-BMC allows us to present the economic, environmental, and social value, and compare it to other projects. The three-layer value extends further than the economic benefits and highlights any weak elements within the three layers. The analysis aims to underline the urgency for improving reporting, unlocking the unrealized potential for SLES across the social and environmental space. As SLES have not been explored previously through the chosen prism, there are no prior studies to compare our analysis with.
3.1. Economic layer
Analysis of the economic layer of the Kityakushu business model is shown in Figure 3.
Figure 3 shows that the Kitakyushu project offers multiple value propositions compared to the typical customer focus of the BMC. Value streams for SLES can incorporate “socialized” value, as found in many SLES projects, and shown in Figure 4.
Looking at the projects from a monetary perspective, including Kitakyushu (Figure 3), it can be argued that short-term financial gains, “cost-saving,” compared to a longer term, broader perspective on economic value, would factor in the value of energy security and greater integration of renewables. Value proposition in SLES therefore does include a broader term generated values. As such, the common cost saving option can be the driving force for renewable integration and security of supply (Zahedi, 2011), but “cost-saving” was not the exclusive value proposition of the projects analyzed. Instead, value propositions relied on multiple sources of value, enhancing the projects' long-term economic resilience (Timeus et al., 2020). Figure 4 demonstrates the multiple value propositions pursued by the individual projects. A common proposed combination of value streams concerns integration of renewable energy sources (RES) as a means to enhance security of supply (Chalvatzis and Ioannidis, 2017; Piacentino et al., 2019). Our research however finds that the academic and gray literature does not explicitly distinguish this combination as a value proposition. This lack of explicit and detailed statements of value propositions is typical in many elements across TL-BMC layers.
For the six projects basing their value proposition on promoting SLES (Supplementary Figure 1), we find that their purpose is to act as a demonstrator for future projects, with the Sino-Singapore Tianjin Eco-city project as a prime example in terms of scale and extent. Pre-commercial systems can create complexities when considering value streams, as they aim to test new system viability to advance knowledge. These projects do not necessarily focus on provision of a customer value proposition; however, we include these in analysis because other value propositions would not necessarily be excluded at commercialization stage. The enhancement of human capital, in terms of expertise and new partnerships, are opportunities realized through deployment of SLES. The Brooklyn Microgrid for example bases its value proposition on creation of Peer-to-Peer (P2P) exchange of electricity between customers. To further disaggregate the economic layer value streams, the isolated value proposition across projects, treated as a sum deriving from those combined offers, is presented in Figure 4. This shows that the most common SLES value stream is renewable integration, present in 10 projects, followed by energy security which is found in nine projects.
Confirming the findings of Fuentes González et al. (2021), SLES projects have diverse partnerships. Joint efforts between government organizations, private initiatives, and research/academic institutions are predominant, highlighting the complexity of projects, which require multiple fields of expertise and/or additional finance to enable deployment. The activities require either physical or remote attendance for managing project operations, as a hint to automation that points toward the utilization of smart solutions within projects' offerings. Project resources include renewable generation, and operational hardware, e.g., equipment and controllers. Human resources, labeled in this analysis as “People” (Supplementary Figure 2C), are necessary for deployment and operation.
The majority of the projects concern both consumers and prosumers, while half also include industrial customers (Supplementary Figure 3A). The inclusion of all economic sectors is explicit only in the Kitakyushu project, where all customers within location boundaries are eligible to participate. While domestic sector customers are the focus of the majority of projects, exclusive focus on industry as the customer base is found in only one project (LEMENE, Finland). The commercial sector is not exclusively targeted by any of the projects. While most project strategies put customers at the center (Supplementary Figure 3B), as expected in commercial standalone applications (Battula et al., 2020), we highlight that, in the case of Kitakyushu, partnerships and developers also seek direct collaboration with the customer base to enable decision making and to optimize future steps. Collaboration is directly linked with the community as the main channel between customers and project; this is also applicable to the Samsø project through local ownership of project elements. Focusing further on project examples, in the Samsø SLES case, engagement with the local community is the channel between project and customer, which builds tolerance and support for project participants. The Kitakyushu SLES follows an alternate approach, with future pathways for development and operation based on real-time information and automation bi-directionally between project and customer.
A multitude of sources generate revenue streams, as Supplementary Figure 4A highlights. The majority of projects base their revenue stream on sales of electricity. However, exact financial returns are not publicly available in all cases, as four projects do not report relevant data. Apart from electricity sales, the monetization of services such as flexibility, the receipt of subsidies, or re-investment of profits toward project development, our findings show that costs are backed by governments grants (Kitakyushu), and communities through ownership of wind turbines and associated subsidies. In addition to explicit cost descriptions, we consider maintenance and developer costs incurred in the SLES projects. Our findings, presented in Supplementary Figure 4B, show that electricity costs and development costs backed by communities are present in four projects. In three projects the costs are met by collaborative effort between government and private sector or, in two projects, exclusively by government. Using the maintenance and developer costs explicitly described in one project, we infer that such costs are incurred in all SLES projects. Two of the projects (Berchidda, Green Campus Smart Grid) do not report the cost structure.
3.2. Environmental layer
The environmental TL-BMC layer includes all development, operation and decommissioning, effectively presenting the energy project life cycle. Despite the selected projects representing the most significant available literature to conduct business model analysis, information is not accessible for all elements of the environmental layer. In focus are the end-of-life and environmental impacts elements. End-of-life processes are not described for 17 out of the 18 projects included in Table 3, and are only explicitly described in the case of Samsø. Five projects provide information on the expected environmental impacts, but, with the exception of Samsø, 4 of those projects do not meet all the selection criteria set. We discuss this broader issue of environmental reporting in Section 4.
We replaced ‘functional value’, in the original TL-BMC framework, with environmental value of a SLES project. This indicates the key achievements or targets for a SLES project. Options in the element include an increase in renewables capacity and subsequent reduction of carbon emissions; energy access and increased efficiency: all strong arguments for developing SLES. The initial iteration of the triple-BMC (Joyce and Paquin, 2016) enables us to define the life cycle assessment functional units (e.g., reduction of 1 ton greenhouse gas emissions), then provide the environmental value through fulfilled or desired output targets of the SLES operation within a given time-frame (e.g., reduction of 100 tons of emissions in a year).
Multiple value propositions are present in the environmental layer of the Kitakyushu SLES in Figure 5, while Figure 6 sums the standalone findings from all 18 projects.
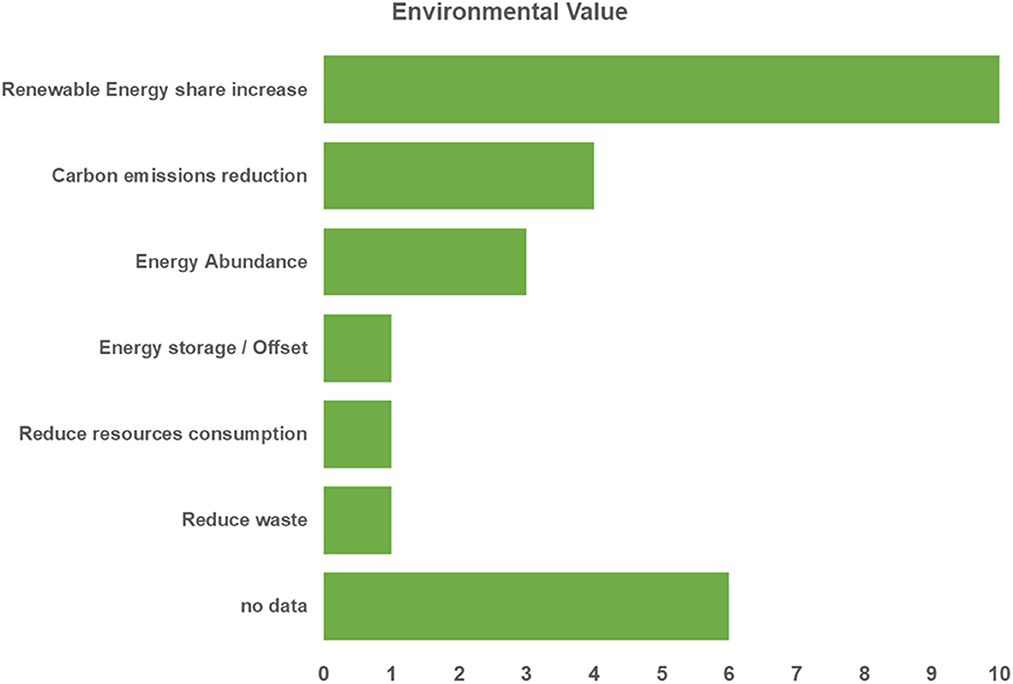
Figure 6. Number of SLES projects reporting on environmental value of the environmental layer of the TL-BMC.
As an example, the environmental layer of a standalone project (Kitakyushu) is shown in Figure 5, while the complete reporting and classification of environmental value in SLES from the review sample is shown in Figure 6. While the Kitakyushu SLES project provides a detailed characterization of the elements in environmental and social layers, in most cases environmental value is not explicitly described. As such, our predominant finding is “no data”.
Where data is available, the environmental value of SLES projects mainly concerns the increase in renewable energy generation and reduced carbon emissions. Non-combined environmental value propositions exist in more than one project when this concerns the share of increased renewable energy in the mix. The Sino-Singapore project demonstrates broad and ambitious targets extending further than RES integration, by aiming to reduce waste production and resource consumption (The World Bank, 2009; Zhan and de Jong, 2017). The majority of the projects utilize further integration of RES in the electricity mix, with the desired outcome of either reducing carbon emissions (Samsø, Isles of Scilly, Kitakyushu, Ramea), or enhancing security of supply (Orkney, Simris, Rotuma). While the derived benefits of RES penetration in an energy system are expected, the Brooklyn Microgrid (Mengelkamp et al., 2018), Utsira (Nakken et al., 2006), and LEMENE (Energiayhteisö, 2018), report on environmental value more broadly by focusing on RES integration.
The supplies and outsourcing element (Supplementary Figure 5A) represent the provisions required for realizing environmental value at development stage, but are not considered essential to project operation, except for energy input where conventional energy sources are part of the design. Seven projects do not explicitly report their data. The most significant supplies and outsourcing include equipment, and the input stream of energy. While we consider consultancy and consumables essential for development of a SLES project, their importance is not translated into reporting. The production element (Supplementary Figure 5B) describes the source used by a SLES operator to provide energy to customers, focusing on the origin and not the core technology. Where reported, the combination of renewables with storage solutions or heat are the predominant categories. Conventional energy generation is present, either as the main source of electricity production in the Sino-Singapore project (Hu et al., 2015; Marnay et al., 2016) or transformed to other end-uses such as heat (The World Bank, 2009). We rename the materials element (Supplementary Figure 5C) of the TL-BMC framework as “Energy Technology” to fit SLES and enhance clarity. We define this as the energy generation technologies used, including the equipment used for electricity generation and the equipment for operations or automation. The Sino-Singapore project is found to use gas generators in conjunction with RES and CHP (US Energy Information Administration, 2011; Hu et al., 2015). With that exception, the combination of renewable energy technologies is a dominant category for energy provision within SLES projects, where reported.
The use-phase element includes customers' involvement that contributes to environmental value. Looking into examples, the environmental value is from electricity consumption using RES, resulting in reduced carbon emissions. Under-reporting is evident for this element (Supplementary Figure 6A) in most cases, except the Brooklyn micro-grid project that employs P2P trading and energy consumption during its use phase. The End-of-Life (EoL) element (Supplementary Figure 6B) in SLES describes the decommissioning process. We assume that EoL occurs when the lifespan of SLES materials or equipment is over, i.e., 20–25 years for installed solar panels (Sisodia et al., 2020) or wind turbines (Salameh et al., 2018) or replacing obsolete system management equipment. Similar to the use phase, EoL is under-explored, with only the Samsø project reporting any data. The latter reports using older wind turbine decommissioning certificates as an economic incentive (Sperling, 2017). The distribution element (Supplementary Figure 6C), applied to SLES, refers to energy transfer, as opposed to physical transportation of goods (García-Muiña et al., 2020; Pardalis et al., 2020). While most projects do not report on their distribution element, four projects use an islanded mode, and three others use a microgrid.
The environmental impacts element in the environmental layer of a SLES describes the costs or negative impacts generated from conception and to operation. This element does not include customers' perception, which is part of the social TL-BMC layer. The majority of the projects do not report on that element (Supplementary Figure 7A). Two projects account for carbon emissions in the energy mix due to generation (Samsø, Sino-Singapore), while two report expected transmission losses (Ramea, Utsira) and energy losses due to oversizing (Simris).
Environmental benefits (Supplementary Figure 7B) describe the ecological value created through operation. The limited reporting of environmental benefits is a surprise and a missed opportunity for projects to display their environmental credentials. Eight projects do not report on environmental benefits at all, matching the under-reporting found in the environmental value element. For projects that provide information, reduced carbon emissions are the main environmental benefit option; we also find the integration of renewable energy sources and electric vehicles' promotion as a main benefit (Green Campus Smart Grid in Finland), which also points to carbon emissions reduction.
3.3. Social layer
The social layer of the TL-BMC captures the relationship between the involved communities and each of the projects. It represents how each project ultimately creates social value. To provide a representative example of this TL-BMC layer, Figure 7 presents the analysis of the Kitakyushu project.
A range of value propositions are combined both for the Kitakyushu project (Figure 7) as well as the standalone offerings of the reviewed SLES (Figure 8).
We define the social value element for SLES (Figure 7) as the project's aim for social development. We include both the benefits to SLES users in terms of e.g., better quality of life, improved access to energy, and cultural changes; and the promotion and spread of an “energy conscious” mindset, facilitating transition to a lower-carbon system. This approach can enable energy management cooperation and communication between users to benefit the wider participating community. A combination of options that provide value is dominant in those projects with detailed data. Energy security and access with social development are found in four projects (Orkney, Isles of Scilly, Kitakyushu, Ramea). In contrast, an equal number of projects (Samsø, Simris, Hvaler, Green Campus Smart Grid) aim to create value by demonstrating the benefits of RES and innovation to society. Three projects primarily create social value through social development (Sino-Singapore), energy security and energy access combined (Gotland), or access to energy exclusively (LEMENE). Eight of the projects, when compared to other options, do not provide detailed data on social value, similar to the lack of data throughout the environmental layer.
As SLES operate within a set geographical boundary, the local communities element (Supplementary Figure 8A) aims to capture those party to the project, within that geographical location. The communities can vary from organizational to end-user level, when an individual user has a stake in the project.
The “no data” option is again prevailing. Five projects reporting data define public and private organizations contributing to SLES; residents and local businesses follow with three projects; only one project has private businesses as sole participants. Governance (Supplementary Figure 8B) extends further than the partnerships element in the economic layer of the TL-BMC to represent all decision making bodies. Again, most projects report no data explicitly; of those that do report on governance, the local administration is the dominant decision-maker (four projects). Other options are fragmented, with two projects governed by state legislation and local administration or the TSO/DSO/Supplier. Involvement of academic institutions, local administration and private businesses is reported by one project (Samsø), while another project (the Brooklyn microgrid) explicitly reports that peer-to-peer market access policies are not yet set.
We consider the employees element (Supplementary Figure 8C) as a qualitative variable, rather than simply the number of people on a SLES payroll, and we examine to what extent the workforce is local, non-local, and/or indirectly employed (i.e., by third parties or on a temporary contractor basis). The lack of data reporting on employability is a missed opportunity for the stakeholders to enhance community support and improve location attractiveness (van der Waal, 2020). The Brooklyn Microgrid, Orkney and the Isles of Scilly report on employing a workforce indirectly, either as the necessary M&O personnel required for Brooklyn (Mengelkamp et al., 2018), or management responsibilities and volunteering for Orkney (van der Waal, 2020), or the creation of a not-for-profit community energy company for the Isles of Scilly (Hitachi Europe, 2018). We find a mix of local and indirect workforce in Samsø and Sino-Singapore, and local workforce for Kitakyushu, where 1,300–6,000 local jobs have been realized by the project (Sasakura, 2015; Gao et al., 2016). The “generic workforce” element describes employment only at a particular stage of the project. For example the Ramea project describes involvement of 12 employees erecting a wind turbine (Jones, 2010).
The social culture element (Supplementary Figure 9A) is the local community's attitude toward a SLES and its operation. This social layer element evaluates a successful project deployment, from conception to long-term operation stages. For example, four projects (Brooklyn Microgrid, Orkney, Samsø, Utsira), provide evidence of local community appreciation of the projects' environmental and social responsibility. In addition, the majority of the projects that report on that element seem to seek to establish a cooperative attitude toward SLES development and operation (Kitakyushu, Simris, Ramea, Hvaler, Gotland, Rotuma) but do not define this as ultimately achieving “smartness” status, comparable to the aims of Kitakyushu. Social culture is also characterized by environmental (LEMENE) or social (Sino-Singapore) responsibility. The scale of outreach element (Supplementary Figure 9B) describes the number of participating residential, commercial and industry customers. While no data are reported for seven projects, numbers of customers range from 1–10 (Utsira) to 100–1,000 (four projects) up to over 1,000 customers (6 projects). Only one project (Green Campus Smart Grid, Finland) exclusively serves industrial and commercial customers.
We define the end-user benefit element (Supplementary Figure 9C), as the benefit(s) to energy users, described as “core customers” in the economic layer of the Business Model Canvas. Where data are present, four projects report improvement in both quality of life and the environment, commonly considered interconnected (Keles, 2012); however, an equal number of projects consider only quality of life improvement, including improved voltage regulation, access to telecommunications or promotion of technology, with no reference to the environment. Three projects create new value streams for the customer base, such as opportunity to invest in and own a share of generation technologies (e.g., a wind turbine in Samsø SLES), while two projects improve supply security, effectively eliminating uncertainty, e.g., electrical blackouts (Jakstas, 2020).
The social impacts element (Supplementary Figure 10A) examines social costs, from planning to operational stage. We find that social impacts are predominantly not reported. The relevant literature does not address negative social impacts for 13 of the projects, a significant majority. For projects that directly report social impacts, Samsø and Orkney focus on public acceptance. Kitakyushu and Rotuma find parts of local communities reluctant to adopt a new way of living, while another two are equally divided between poor expected results from operation (Hvaler), or distrust (Simris) toward stakeholders. Social benefits (Supplementary Figure 10B) closely align with social value and are more reported in the literature than negative social impacts. Eight projects have limited detail. Of the remaining 12 projects, five consider their contribution to improving the community's quality of life, while two favor economic or social development. We classify social development as the product of collective thinking about energy efficient usage patterns, overall leading to smarter customers that do not require the use of automation to regulate their consumption, matching the case of Kitakyushu (Figure 7). The three remaining projects aim to benefit the local community through population growth, which is the ultimate goal set by the Samsø as necessary to its community survival (Jantzen et al., 2018), the reduction of fuel poverty, and creation of a closed-loop for profits from the project's operation.
3.4. Smartness and localness
In this final section of the analysis, we go beyond the TL-BMC framework in two ways, considering the ways where the projects under review can be considered “smart” and “local.” These added elements are important to our analysis as they provide an operational project benchmark; set stakeholder/customer or geographical boundaries, and provide technical maturity levels. All the indicators have a horizontal and vertical knock-on effect on the three TL-BMC layers.
Extending beyond the TL-BMC framework, localness (Supplementary Figure 11A) characterizes a project in terms of energy generation and consumption within the geographical boundaries of the community that it benefits. Other localness-related options include local management in Urja Upatyaka and Kytakyushu, local ownership for Samsø and Berchidda, establishing a local energy market (Eskdale and Brooklyn Microgrid), or local decision-making (South Tyrol).
Smartness (Supplementary Figure 11B) mainly translates to use of automation equipment in the systems analyzed. Smart energy management is the dominant option for including smartness elements in SLES, with eight projects utilizing relevant solutions, while similar options, based on automation, include smart grid, trading platforms and smart devices for end-users. As an exception, for the Kitakyushu SLES, smartness is perceived as advancing collective thinking, leading to smarter customers and self-management of energy consumption without the need for automation to regulate behavior.
3.5. The lack of data
Our research collects secondary data and matches them to each element of the TL-BMC. However, as discussed regarding the end-of-life element, throughout the data collection and assignment process in each layer, there is a persistent pattern of no-data reporting. While this is typically classified as data noise, it highlights a more significant problem. The underreporting or under-exploration of elements is evident in the environmental and social layers, as shown in Figure 9. The figure shows that the economic layer of the TL-BMC is well covered by academic and gray literature, with data available to fill 92% of the possible economic layer elements. However, the social (53%) and, even more prominently, the environmental layers (46%) see reporting significantly diminished across all elements of each of the layers. In the previous discussion sections, we see that SLES business models can extend further than the economic layer to form new value streams across the environmental and social layers.
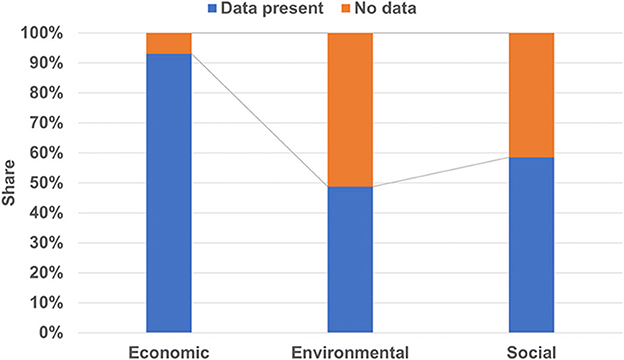
Figure 9. Schematic comparison of “no data” reporting per TL-BMC layer deriving from all reviewed literature sources.
4. Discussion
As an analytical framework, the TL-BMC enables detailed investigation of business models applied in SLES projects. In particular, it provides a tool to identify value beyond the solely economic, encompassing environmental and social layers. Analyses of business model composition using the TL-BMC can hence identify both strong and weak aspects of existing projects, acting as an enabler for successful SLES development and operation by highlighting the full range of value relevant to effectiveness.
However, the framework requires highly detailed information to complete its 27 elements, 9 for each layer. As such, the availability of data from all partners across a consortium is crucial. Complexity is magnified by the requirement to provide distinctive definitions and criteria for all elements. In addition, layers and elements overlap, and require careful consideration for appropriately informing the framework, as TL-BMC horizontal coherence points toward the elements not being either mutually exclusive. While the framework does not demand academic expertise to cover the information per element and per layer, it requires judgement and evaluation to make it horizontally and vertically coherent.
4.1. A critical approach requirement for the TL-BMC framework application in SLES
For example, within the environmental layer, benefit and value overlap in the theme of carbon emissions reduction (Figure 6; Supplementary Figure 7B). While environmental benefit can express the mitigation of quantified carbon output, environmental value translates to a desired and, therefore, pivotal output focusing on the aim of the business model itself: improved environmental quality through emissions savings.
In the social layer, benefits gained by individual end-users of the system are part of overall social value, but the latter also includes wider effects that follow from individual gains. In the case of a SLES, this might look like e.g., individual households saving money on energy bills and therefore being able to spend more on other things, resulting in a boost to the local (or wider) economy. Social value also includes changes captured in the TL-BMC “communities,” “governance” and “workforce” elements. For example, a SLES may lead to the creation of local jobs, or to strengthened local social networks and political participation as a result of the experience of engaging.
The two extra layers of the TL-BMC enable us to highlight integral components of value creation in SLES, for example the utilization of renewable energy instead of fossil fuel sources. Where renewable energy generation is adopted, this is expressed in numerous but distinct elements across the three BMC layers. In the economic layer, RES are captured in the key resources contributing to the value proposition. The contribution of renewable energy is magnified by the environmental layer, where renewable energy becomes part of production, energy technology, environmental value, and environmental benefits. Similarly, in the social layer renewable energy is integral to social value and impacts. All layers include options that derive from the use of renewable energy sources. However, the options for each element, and how the business model is built from exact information, are distinct, highlighting the importance of the triple-layered analysis. Exemplifying this, Kitakyushu looks at the use of RES primarily to achieve a 50% carbon emissions reduction (Gao et al., 2016), while the Brooklyn Microgrid sees the end-users choosing the RES energy mix and trading energy locally as part of the P2P offering (Mengelkamp et al., 2018).
4.2. Informing the TL-BMC
Exploring additional business model elements, the TL-BMC drew attention to end-of-life planning for materials and equipment. We consider this environmental layer element as key to minimizing environmental damage during the decommissioning stage. It has a direct effect on local communities, but also broader implications through waste exports (Invernizzi et al., 2020). Our analysis found that reporting of the end-of-life element of SLES projects was weak across the sample, except for the case of Samsø. While there is extensive research on the decommissioning process of various RES technologies, such as onshore wind and solar (Hall et al., 2020; Vargas and Chesney, 2021), the projects themselves do not report end-of-life processes. Therefore, we characterize these issues not as under-explored, but as under-reported; and suggest that an opportunity exists for the partnerships to provide more clarity over the SLES life cycle in their projects. The inclusion of end-of-life reporting will effectively promote environmental and social layers' inclusion in business models. Improved end-of-life reporting will enhance transparency over the project life-cycle, setting expectations and potentially enabling social and environmental impacts to be mitigated, while hidden costs are identified. End-of-life reporting can therefore provide additional reasons for the creation of TL-BMC business models (Purnell et al., 2018), by adding long-term clarity to stakeholders. Such reporting can also stimulate SLES concepts where renewable energy replaces current generators at designated sites, resulting either in greater capacity and/or more efficiency, resulting in a net gain in power output. Described as “repowering,” this is a reduced risk option for investors (Del Río et al., 2011; Szumilas-Kowalczyk et al., 2020).
The majority of projects that provide reporting (67%) on social benefits offer both economic and social development to the community, assuming that increased quality of life covers both. However, the reinvestment of profit in the community does not seem to translate into an increase in local workforce. The existing literature finds energy-related projects to involve a local workforce to enable seamless operation, advance economic development within the involved geographic region (Luke et al., 2017; Sperling, 2017), either for logistics or as means for compensation (Kerr et al., 2017). However, most of the assessed SLES projects do not match this pattern, pointing at another case of under-reporting.
In a similar context, lack of reporting about negative social or environmental impacts from a SLES is made increasingly problematic, further highlighting weak areas and creating urgency for further reporting. While the lack of data relevant to social or environmental benefits might point to under-exploration, the lack of reporting on negative environmental impacts, as found in e.g., Samsø, can be problematic for development and operation of the SLES. This has broader implications for the trust of participating communities, as negative impacts can result from end-of-life practices.
4.3. Smartness and localness
Looking beyond the TL-BMC elements, the main driver of smartness for smart and local energy systems is perceived as the application of automated processes that minimize operational or end-user input, to maximize revenue, user comfort and energy efficiency. Ofgem defines automation as the enabler of smartness (HM Government and Ofgem, 2017), while autonomous operation or digitization and data collection (Ding et al., 2011) remain key for smartness. In our analysis of SLES projects, however, the TL-BMC enables us to find an alternative definition of smartness. While the majority of projects base their smartness offering on energy management, the Kitakyushu project sees the formation of the energy-conscious end-user as the defining element of smartness. In this, the end-user, part of the social layer, is the core element that characterizes successful application of smartness. This definition goes further than equipment for automation and real-time data collection; it also sees the end-user as gaining ability to account for their energy-related actions and to make conscious decisions about energy consumption; technology and equipment act as the enabler toward that achievement.
In contrast to smartness, the localness element presents a homogenous starting point, defining its boundaries as local renewable generation for feeding the system. A definition based on geographical location is prominent, being used by 11 SLES projects, matching the findings of Ford et al. (2021). Only a minority of projects define localness in terms of community or social aspects, such as local management or local decision-making. Therefore, localness is described within the reviewed projects, as placing generation in geographical proximity to end-users to in order to mitigate energy security or supply intermittency issues, rather than putting the users in development, operation, and/or control of the project. This approach restricts the potential for democratization, a fundamental component brought by SLES systems to communities (Soutar and Mitchell, 2018) as well as the social justice in business development and practices. It highlights the approach of numerous partnerships for prioritizing grid robustness and monetary value, pursued above community involvement and benefit.
The TL-BMC facilitates the realization of additional arguments for development of partnerships for SLES. Benefits for communities, such as grid robustness and monetary gains, primary motivations for joining a SLES described in social value and social benefit, can incentivize communities to host or develop a project within their administrative or geographical boundaries. A catalyst for the partnerships involved is the social culture element. This element can aid the partnerships in forming appropriate strategies and effectively promote SLES to the local community. Looking at the core partnerships element, the application of the chosen analytical framework makes evident that successful SLES require partnerships. We see state authorities, private companies, and research institutes engaged in collaborative efforts that range from planning to the operational stage to establish SLES projects.
4.4. Policy implications
The results suggest important implications for policy-makers. The UK energy field is dominated by large-scale actors and commercial finance (Hall et al., 2016). Reliance of the system on natural gas for electricity generation has led to high wholesale energy prices, causing an increasing number of UK energy suppliers to be sold to dominant actors, or enter administration in 2022 (Ofgem, 2022), effectively highlighting the need for administrative and regulation reform of the UK energy market (The Guardian, 2022).
This economic uncertainty can have several effects. On the one hand, it may lead national governments to mitigate perceived risks by locking into existing power generation and proven distribution technologies at the expense of a decentralized system. Such centralization would significantly affect the creation of new sustainable business models since business model innovation is greatly influenced by the current regulatory environment. There is a risk that policymakers could lose sight of the environmental layer of energy business models in their pursuit of short-term energy security (Climate Action Tracker, 2022).
On the other hand, the crisis, with its impacts on fuel poverty (University of York, 2022) could highlight how the reliance on natural gas is unsustainable, not just in environmental terms, but also economically and socially (IEA, 2022). The adapted TL-BMC framework we develop and present in this paper can help support this more positive outcome for sustainability. It can be used to make the case for a more innovative and decentralized approach to energy systems by showing how they can create multiple layers of value, and identifying synergies between the layers (e.g., improving energy efficiency would address both the decarbonization goal and the energy security goal, as would developing UK-based renewable energy sources).
5. Conclusions
The paper systematically reviewed worldwide SLES project reporting, from demonstrators to operational projects. Eighteen projects were selected for analysis using the TL-BMC. The assessment has helped to clarify new value streams that extend further than the economic value proposition. We conclude however that social and environmental elements, or even the entire layers, remain under-reported.
The TL-BMC framework could nevertheless be used in future as a method for assessing the building blocks and value streams of new SLES business models. It is a useful analytical tool to improve reporting and knowledge of the impacts and benefits, potentially strengthening arguments for further deployment of SLES. Creating novel business models which generate environmental and social value equal to financial value can strengthen the argument for SLES promoting environmental benefits and social development. Those benefits include carbon emissions reduction (Gupta and Zahiri, 2020), collective decision-making (Chatfield and Reddick, 2016), energy security and access (Dincer and Acar, 2017; Bačeković and Østergaard, 2018) and RES share increase. Therefore, the adoption of the TL-BMC highlights the benefits of SLES and demonstrates how they might contribute to tackling climate change by reducing greenhouse gas emissions and social inequality through improved access to energy. We consider the utilization of TL-BMC to be an enabler of a novel rationale across SLES projects that can unlock a modular development approach.
The value and novelty of SLES alter how benefits are conceived, depending significantly on the projects' geographical location. Local energy systems do not only affect local communities and end-customers but, in addition, are themselves affected by aspects of the local context, e.g., culture, geography, regional policy. Different aspects of SLES value across the three layers may be emphasized in different localities, and smartness and localness may be perceived differently. For example, smartness may be defined in terms of the understanding and behavior of end-users, and not by the processes for securing smartness, such as automation. The available literature defines the goal of SLES as community creation of a sustainable and fair environmental and energy use pattern, using automation as the means to achieve environmental and social goals.
Sustainable business models, facilitated by frameworks such as the TL-BMC, enable the realization of existing and future environmental and social value. Policy-makers should focus on prioritizing comprehensive reporting procedures for operators and developers, to fully unlock sustainable business model value creation, through well-informed elements currently overlooked or under-reported.
We believe that our adapted TL-BMC framework will continue to be useful to inform the design and monitoring of future SLES business models. We have made sure to validate the framework by testing it on a range of SLES projects internationally. This has allowed us to critique the framework and adjust it to the SLES sector, to ensure its validity and consistency across the projects it is applied to. In terms of critique, we find the framework to have significant requirements in data reporting, with the three layers adding to complexity, but its ability to better describe all elements comprising an SLES, make it an optimal choice for future-oriented policy-making and development.
Our policy recommendations for future and existing projects, where applicable, further focus on pathways derived from limitations identified in the analysis. End-of-life planning and related processes should be included in the conception of any SLES project. This will set the bar for the environmental value offered, and give further detail on decommissioning costs, which would otherwise be hidden during operation, as well as guiding vertical coherence in a TL-BMC setting enhancing the efficiency of adopted environmental action in both the economic and social layers. Our analysis explicitly presents the value existing in SLES, in a multitude of elements across economic, environmental and social layers. We propose that the TL-BMC can be a best-fit tool of choice, to conduct a comprehensive, objective and complete analysis of new value streams from SLES projects.
Future research is needed to examine under-explored and under-reported elements of value to enable the realization of the TL-BMC, across the “energy ecosystem” sectors, as a useful guide for the full-cycle identification and scope of value for SLES. As such, future research can spark the involvement of a wide variety of social and environmental disciplines in SLES projects, producing environmental and social value by past SLES experience, previously unrealized, and commissioned by academia and/or industrial partners.
Integrating the environmental and social layers, and their promotion as integral structural parts of the business model, from concept to decommissioning, will increase project value, community involvement, and SLES acceptance. Stakeholder benefits increase in line with locating and exploiting underlying value streams, attracting new investment funds and increasing clarity across the project lifecycle. The utilization, and/or better reporting, of SLES features in the novel TL-BMC framework can further improve their attractiveness as an integral part of the future of energy generation, distribution, and use.
In summary, the application of the TL-BMC analysis to SLES showcases untapped elements of business models that can utilize alternate value factors by different stakeholders. The multi-layered SLES project value is not exclusively captured by financial metrics. Progress on environmental targets and social gains for communities, leading to the creation of a mutually beneficial relationship between developers-operators and users-communities remains urgent.
Data availability statement
The original contributions presented in the study are included in the article/Supplementary material, further inquiries can be directed to the corresponding author.
Author contributions
DP wrote the paper, contributed to data collection, and performed the analyses. All authors contributed to the article and approved the submitted version.
Funding
This research was supported by the Engineering and Physical Sciences Research Council (EPSRC) as part of the EnergyREV Consortium (grant number EP/S031863/1). EnergyREV was established in December 2018 under the UK's Industrial Strategy Challenge Fund Prospering from the Energy Revolution programme. It brings together a team of over 60 researchers across 22 UK universities to help drive forward research and innovation in Smart Local Energy Systems.
Acknowledgments
The authors thank the EnergyREV Consortium and Professor Jo Patterson of Cardiff University for her support during the data collection, writing, and submission process.
Conflict of interest
The authors declare that the research was conducted in the absence of any commercial or financial relationships that could be construed as a potential conflict of interest.
Publisher's note
All claims expressed in this article are solely those of the authors and do not necessarily represent those of their affiliated organizations, or those of the publisher, the editors and the reviewers. Any product that may be evaluated in this article, or claim that may be made by its manufacturer, is not guaranteed or endorsed by the publisher.
Supplementary material
The Supplementary Material for this article can be found online at: https://www.frontiersin.org/articles/10.3389/fsuep.2022.1058534/full#supplementary-material
References
Akella, A. K., Saini, R. P., Sharma, M. P. (2009). Social, economical and environmental impacts of renewable energy systems. Renew. Energy 34, 390–396. doi: 10.1016/j.renene.2008.05.002
Alvarez-Herranz, A., Balsalobre-Lorente, D., Shahbaz, M., Cantos, J. M. (2017). Energy innovation and renewable energy consumption in the correction of air pollution levels. Energy Policy 105, 386–397. doi: 10.1016/j.enpol.2017.03.009
Bačeković, I., Østergaard, P. A. (2018). Local smart energy systems and cross-system integration. Energy 151, 812–825. doi: 10.1016/j.energy.2018.03.098
Battula, S., Tesfatsion, L., Wang, Z. (2020). A customer-centric approach to bid-based transactive energy system design. IEEE Trans. Smart Grid 11, 4996–5008. doi: 10.1109/TSG.2020.3008611
Biresselioglu, M. E., Demir, M. H., Demirbag Kaplan, M., Solak, B. (2020). Individuals, collectives, and energy transition: analysing the motivators and barriers of European decarbonisation. Energy Res. Soc. Sci. 66, 101493. doi: 10.1016/j.erss.2020.101493
Braunholtz-Speight, T., Sharmina, M., Manderson, E., McLachlan, C., Hannon, M., Hardy, J., et al. (2020). Business models and financial characteristics of community energy in the UK. Nat. Energy 5, 169–177. doi: 10.1038/s41560-019-0546-4
Ceglia, F., Esposito, P., Marrasso, E., Sasso, M. (2020). From smart energy community to smart energy municipalities: literature review, agendas and pathways. J. Clean. Prod. 254, 120118. doi: 10.1016/j.jclepro.2020.120118
Chalvatzis, K. J., Ioannidis, A. (2017). Energy supply security in the EU: benchmarking diversity and dependence of primary energy. Appl. Energy. 207, 465–476. doi: 10.1016/j.apenergy.2017.07.010
Chatfield, A. T., Reddick, C. G. (2016). Smart city implementation through shared vision of social innovation for environmental sustainability: a case study of Kitakyushu, Japan. Soc. Sci. Comput. Rev. 34, 757–773. doi: 10.1177/0894439315611085
Climate Action Tracker (2022). Global Reaction to Energy Crisis Risks Zero Carbon Transition. Available online at: https://climateactiontracker.org/publications/global-reaction-to-energy-crisis-risks-zero-carbon-transition/ (accessed December 4, 2022).
Del Río, P., Calvo Silvosa, A., Iglesias Gómez, G. (2011). Policies and design elements for the repowering of wind farms: a qualitative analysis of different options. Energy Policy 39, 1897–1908. doi: 10.1016/j.enpol.2010.12.035
Dhar, A., Naeth, M. A., Jennings, P. D., El-Din, M. G. (2020). Perspectives on environmental impacts and a land reclamation strategy for solar and wind energy systems. Sci. Total Environ. 718, 134602. doi: 10.1016/j.scitotenv.2019.134602
Dincer, I., Acar, C. (2017). Smart energy systems for a sustainable future. Appl. Energy 194, 225–235. doi: 10.1016/j.apenergy.2016.12.058
Ding, Y., Decker, C., Vassileva, I., Wallin, F., Beigl, M. (2011). “A smart energy system: distributed resource management, control and optimization,” in 2011 2nd IEEE PES International Conference and Exhibition on Innovative Smart Grid Technologies, 1–8. doi: 10.1109/ISGTEurope.2011.6162720
Dobrowolski, Z., Sułkowski, Ł. (2021). Business model canvas and energy enterprises. Energies 14, 7198. doi: 10.3390/en14217198
Energiayhteisö, L. L. (2018). A Self-Sufficient and Intelligent Energy System. Lempaalan Energia. Available online at: http://www.lempaalanenergia.fi/files/upload_pdf/21540/LEMENE%20esite.pdf
Feron, S. (2016). Sustainability of off-grid photovoltaic systems for rural electrification in developing countries: a review. Sustainability. 8, 1326. doi: 10.3390/su8121326
Ford, R., Maidment, C., Fell, M., Vigurs, C., Morris, M. (2019). A Framework for Understanding and Conceptualising Smart Local Energy Systems. Strathclyde: EnergyREV, University of Strathclyde Publishing, 1–18.
Ford, R., Maidment, C., Vigurs, C., Fell, M. J., Morris, M. (2021). Smart local energy systems (SLES): a framework for exploring transition, context, and impacts. Technol. Forecast. Social Change 166, 120612. doi: 10.1016/j.techfore.2021.120612
Fuentes González, F., Webb, J., Sharmina, M., Hannon, M., Pappas, D., Tingey, M. (2021). Characterising a local energy business sector in the United Kingdom: participants, revenue sources, and estimates of localism and smartness. Energy 223, 120045. doi: 10.1016/j.energy.2021.120045
Gao, W., Fan, L., Ushifusa, Y., Gu, Q., Ren, J. (2016). Possibility and challenge of smart community in Japan. Proc. Soc. Behav. Sci. 216, 109–118. doi: 10.1016/j.sbspro.2015.12.015
García-Muiña, F. E., Medina-Salgado, M. S., Ferrari, A. M., Cucchi, M. (2020). Sustainability transition in industry 4.0 and smart manufacturing with the triple-layered business model canvas. Sustainability 12, e2364. doi: 10.3390/su12062364
Geels, F. W., Raven, R. P. J. M. (2007). Socio-cognitive evolution and co-evolution in competing technical trajectories: biogas development in denmark (1970–2002). Int. J. Sustain. Dev. World Ecol. 14, 63–77. doi: 10.1080/13504500709469708
Grames, E. M., Stillman, A. N., Tingley, M. W., Elphick, C. S. (2019). An automated approach to identifying search terms for systematic reviews using keyword co-occurrence networks. Methods Ecol. Evol. 10, 1645–1654. doi: 10.1111/2041-210X.13268
Gupta, R., Zahiri, S. (2020). “Meta-study of smart and local energy system demonstrators in the UK: Technologies, leadership and user engagement,” in IOP Conference Series: Earth and Environmental Science (IOP Publishing), p. 022049
Hall, R., João, E., Knapp, C. W. (2020). Environmental impacts of decommissioning: onshore versus offshore wind farms. Environ. Impact Assess. Rev. 83, 106404. doi: 10.1016/j.eiar.2020.106404
Hall, S., Foxon, T. J., Bolton, R. (2016). Financing the civic energy sector: how financial institutions affect ownership models in Germany and the United Kingdom. Energy Res. Soc. Sci. 12, 5–15. doi: 10.1016/j.erss.2015.11.004
Hamwi, M., Lizarralde, I., Legardeur, J. (2021). Demand response business model canvas: a tool for flexibility creation in the electricity markets. J. Cleaner Prod. 282, 124539. doi: 10.1016/j.jclepro.2020.124539
Hitachi Europe (2018). Isles of Scilly and Smart Energy Islands Project Update: July 2018—Addressing Energy's Changing Landscape. Available online at: https://www.hitachi.eu/sites/default/files/fields/document/press-release/2018-07-23_isles_of_scilly_and_smart_energy_islands_final_0.pdf (accessed March 20, 2022).
Hiteva, R., Sovacool, B. (2017). Harnessing social innovation for energy justice: a business model perspective. Energy Policy. 107, 631–9. doi: 10.1016/j.enpol.2017.03.056
HM Government and Ofgem (2017). Upgrading Our Energy System. London: HM Government and Ofgem, 1–126.
Hu, M. C., Wu, C. Y., Shih, T. (2015). Creating a new socio-technical regime in China: evidence from the Sino-Singapore Tianjin Eco-City. Futures 70, 1–12. doi: 10.1016/j.futures.2015.04.001
IEA (2022). World Energy Outlook 2022 Shows the Global Energy Crisis Can be a Historic Turning Point Towards a Cleaner and More Secure Future. Available online at: https://www.iea.org/news/world-energy-outlook-2022-shows-the-global-energy-crisis-can-be-a-historic-turning-point-towards-a-cleaner-and-more-secure-future (accessed December 4, 2022).
Invernizzi, D. C., Locatelli, G., Velenturf, A., Love, P. E. D., Purnell, P., Brookes, N. J. (2020). Developing policies for the end-of-life of energy infrastructure: coming to terms with the challenges of decommissioning. Energy Policy 144, 111677. doi: 10.1016/j.enpol.2020.111677
Jakstas, T. (2020). “Chapter 5—What does energy security mean?” in Energy Transformation towards Sustainability, eds M. Tvaronavičiene and B. Slusarczyk, eds (Amsterdam: Elsevier), 99–112. doi: 10.1016/B978-0-12-817688-7.00005-7
Jantzen, J., Kristensen, M., Christensen, T. H. (2018). Sociotechnical transition to smart energy: the case of Samso 1997–2030. Energy 162, 20–34. doi: 10.1016/j.energy.2018.07.174
Jenkins, K., McCauley, D., Heffron, R., Stephan, H., Rehner, R. (2016). Energy justice: a conceptual review. Energy Res. Soc. Sci. 11, 174–182. doi: 10.1016/j.erss.2015.10.004
Jones, C., Gilbert, P., Stamford, L. (2020). Assessing the climate change mitigation potential of stationary energy storage for electricity grid services. Environ. Sci. Technol. 54, 67–75. doi: 10.1021/acs.est.9b06231
Jones, G. (2010). Ramea Report. Nalcor Energy. Available online at: https://nalcorenergy.com/wp-content/uploads/2016/12/nalcorenergyrameareport_january2010.pdf
Joyce, A., Paquin, R. L. (2016). The triple layered business model canvas: a tool to design more sustainable business models. J. Clean. Prod. 135, 1474–1486. doi: 10.1016/j.jclepro.2016.06.067
Judson, E., Fitch-Roy, O., Pownall, T., Bray, R., Poulter, H., Soutar, I., et al. (2020). The centre cannot (always) hold: Examining pathways towards energy system de-centralisation. Renew. Sustain. Energ. Rev. 118, 109499. doi: 10.1016/j.rser.2019.109499
Keles, R. (2012). The quality of life and the environment. Proc. Soc. Behav. Sci. 35, 23–32. doi: 10.1016/j.sbspro.2012.02.059
Kerr, S., Johnson, K., Weir, S. (2017). Understanding community benefit payments from renewable energy development. Energy Policy. 105, 202–211. doi: 10.1016/j.enpol.2017.02.034
Li, R., Song, H. (2019). “Study on business model of virtual power plant based on Osterwalder Business Model Canvas,” in 2019 IEEE 3rd International Electrical and Energy Conference (CIEEC), 1842–1846. doi: 10.1109/CIEEC47146.2019.CIEEC-2019632
Lin, Y., Li, J., Huang, X., Guo, Z., Sun, Y., Zhang, R. (2018). “Exploring a sustainable business routing for China's new energy vehicles: BYD as an example BT,” in Advances in Green Energy Systems and Smart Grid, eds K. Li, J. Zhang, M. Chen, Z. Yang, and Q. Niu (Singapore: Springer Singapore), 70–80. doi: 10.1007/978-981-13-2381-2_7
Luke, N., Zabin, C., Velasco, D., Collier, R. (2017). Diversity in California's Clean Energy Workforce: Access to Jobs for Disadvantaged Workers in Renewable Energy Construction. University of California. 1–19. Available online at: https://laborcenter.berkeley.edu/pdf/2017/Diversity-in-Californias-Clean-Energy-Workforce.pdf
Marnay, C., Mauzy, J., Shaffer, B., Agate, W., Vitiello, S., Karali, N. (2016). White Paper on Benefit Analysis of Smart Grid Projects.
Matusiak, B. E. (2016). “Local balancing system from the business model canvas perspective,” in E3S Web of Conferences, eds M. Filipowicz, M. Dudek, T. Olkuski, and K. Styszko (Les Ullies: EDP Sciences), 55. doi: 10.1051/e3sconf/20161000055
McLoughlin, N., Corner, A., Clarke, J., Whitmarsh, L., Capstick, S., Nash, N. (2019). Mainstreaming Low-Carbon Lifestyles. Oxford: Climate Outreach. 1–36. Available online at: https://climateoutreach.org/download/15949/?tmstv=1673024609
Mengelkamp, E., Gärttner, J., Rock, K., Kessler, S., Orsini, L., Weinhardt, C. (2018). Designing microgrid energy markets: a case study: the Brooklyn microgrid. Appl. Energy. 210, 870–880. doi: 10.1016/j.apenergy.2017.06.054
Nakken, T., Strand, L. R., Frantzen, E., Rohden, R., Eide, P. O. (2006). “The Utsira wind-hydrogen system-operational experience,” in European Wind Energy Conference and Exhibition 2006, EWEC 2006, 223–231.
O'Dwyer, E., Pan, I., Acha, S., Shah, N. (2019). Smart energy systems for sustainable smart cities: current developments, trends and future directions. Appl. Energy 237, 581–597. doi: 10.1016/j.apenergy.2019.01.024
Ofgem (2022). Check Who's Taken Over Your Energy Supply. Ofgem. Available online at: https://www.ofgem.gov.uk/publications/check-whos-taken-over-your-energy-supply (accessed December 4, 2022).
Osterwalder, A., Pigneur, Y. (2010). Business Model Generation—A Handbook for Visionaries, Game Changers and Challengers Striving to Defy Outmoded Business Models and Design Tomorrow's Enterprises. The Medieval Ous: Imitation, Rewriting, and Transmission in the French Tradition.
Pardalis, G., Mahapatra, K., Mainali, B. (2020). A triple-layered one-stop-shop business model canvas for sustainable house renovations. IOP Conf. Ser. Earth Environ. Sci. 588, 22060. doi: 10.1088/1755-1315/588/2/022060
Piacentino, A., Duic, N., Markovska, N., Mathiesen, B. V., Guzović, Z., Eveloy, V., et al. (2019). Sustainable and cost-efficient energy supply and utilisation through innovative concepts and technologies at regional, urban and single-user scales. Energy 182, 254–268. doi: 10.1016/j.energy.2019.06.015
Pohlmann, A. (2019). Dismantling the relationship between energy innovations and power. Energy Policy 133, 110904. doi: 10.1016/j.enpol.2019.110904
Powells, G., Fell, M. J. (2019). Flexibility capital and flexibility justice in smart energy systems. Energy Res. Soc. Sci. 54, 56–59. doi: 10.31235/osf.io/3nja6
Purnell, P., Velenturf, A., Jensen, P., Cliffe, N., Jopson, J. (2018). “Developing technology, approaches and business models for decommissioning of low-carbon infrastructure: E 4 LCID,” in Low Carbon Infrastructure Decommissioning Workshop.
Reis, F. G., Gonçalves, I., Lopes, A. R. M, Henggeler Antunes, C. (2021). Business models for energy communities: a review of key issues and trends. Renew. Sustain. Energy Rev. 144, 111013. doi: 10.1016/j.rser.2021.111013
Salameh, J. P., Cauet, S., Etien, E., Sakout, A., Rambault, L. (2018). Gearbox condition monitoring in wind turbines: a review. Mech. Syst. Signal Process. 111, 251–264. doi: 10.1016/j.ymssp.2018.03.052
Sasakura, T. (2015). Result of the Kitakyushu Smart Community Creation. Tokyo: Fuji Electric Co. Ltd.
Sayed, E. T., Wilberforce, T., Elsaid, K., Rabaia, M. K. H., Abdelkareem, M. A., Chae, K.-J., et al. (2021). A critical review on environmental impacts of renewable energy systems and mitigation strategies: wind, hydro, biomass and geothermal. Sci. Total Environ. 766, 144505. doi: 10.1016/j.scitotenv.2020.144505
Sisodia, G. S., Awad, E., Alkhoja, H., Sergi, B. S. (2020). Strategic business risk evaluation for sustainable energy investment and stakeholder engagement: a proposal for energy policy development in the Middle East through Khalifa funding and land subsidies. Bus. Strategy Environ. 29, 2789–2802. doi: 10.1002/bse.2543
Soutar, I., Mitchell, C. (2018). Towards pragmatic narratives of societal engagement in the UK energy system. Energy Res. Soc. Sci. 35, 132–139. doi: 10.1016/j.erss.2017.10.041
Sovacool, B. K. (2014). What are we doing here? Analyzing fifteen years of energy scholarship and proposing a social science research agenda. Energy Res. Soc. Sci. 1, 1–29. doi: 10.1016/j.erss.2014.02.003
Sovacool, B. K., Dworkin, M. H. (2015). Energy justice: conceptual insights and practical applications. Appl. Energy. 142, 435–444. doi: 10.1016/j.apenergy.2015.01.002
Sperling, K. (2017). How does a pioneer community energy project succeed in practice? The case of the Samsø Renewable Energy Island. Renew. Sustain. Energy Rev. 71, 884–897. doi: 10.1016/j.rser.2016.12.116
Szulecki, K. (2018). Conceptualizing energy democracy. Environ. Polit. 27, 21–41. doi: 10.1080/09644016.2017.1387294
Szumilas-Kowalczyk, H., Pevzner, N., Giedych, R. (2020). Long-term visual impacts of aging infrastructure: challenges of decommissioning wind power infrastructure and a survey of alternative strategies. Renew. Energy 150, 550–560. doi: 10.1016/j.renene.2019.12.143
The Guardian (2022). 'Too Slow to Act': Ofgem's 'Failures' Cost Energy Consumers Billions, Say MPs. Available online at: https://www.theguardian.com/business/2022/nov/13/too-slow-to-act-ofgem-failures-cost-energy-consumers-billions-say-mps (accessed December 4 2022).
The World Bank (2009). Sino-Singapore Tianjin Eco-City: A Case Study of an Emerging Eco-City in China. Singapore: World Bank, 168. doi: 10.1596/28143
Timeus, K., Vinaixa, J., Pardo-Bosch, F. (2020). Creating business models for smart cities: a practical framework. Public Manage. Rev. 22, 726–745. doi: 10.1080/14719037.2020.1718187
Tsoutsos, T., Frantzeskaki, N., Gekas, V. (2005). Environmental impacts from the solar energy technologies. Energy Policy 33, 289–296. doi: 10.1016/S0301-4215(03)00241-6
University of York (2022). More than Half of UK Households to be in Fuel Poverty by the New Year, According to New Report—News and Events. University of York. Available online at: https://www.york.ac.uk/news-and-events/news/2022/research/fuel-poverty-uk/ (accessed December, 4, 2022).
US Energy Information Administration (2011). Smart Grids Around the World. Washington, DC: US Energy Information Administration.
van der Waal, E. C. (2020). Local impact of community renewable energy: a case study of an Orcadian community-led wind scheme. Energy Policy 138, 111193. doi: 10.1016/j.enpol.2019.111193
Vargas, C., Chesney, M. (2021). End of life decommissioning and recycling of solar panels in the United States. A real options analysis. J. Sustain. Finance Invest. 11, 82–102. doi: 10.1080/20430795.2019.1700723
Wu, C., Wei, Z., Han, H., Xue, G., Huang, H., Fu, A. (2020). “Research on source-grid-load-energy storage collaborative service business model based on business model canvas approach,” in 2020 12th IEEE PES Asia-Pacific Power and Energy Engineering Conference (APPEEC), 1–5. doi: 10.1109/APPEEC48164.2020.9220601
Xu, Y., Ling, P., Li, X., Li, G., Cui, S. (2015). “Gas prediction of Sino-Singapore Tianjin eco-city based on ARMA model,” in 2015 3rd International Conference on Advances in Energy and Environmental Science (New York, NY: Atlantis Press), 1405–1409. doi: 10.2991/icaees-15.2015.261
Zahedi, A. (2011). A review of drivers, benefits, and challenges in integrating renewable energy sources into electricity grid. Renew. Sustain. Energy Rev. 15, 4775–4779. doi: 10.1016/j.rser.2011.07.074
Keywords: Triple Layer Business Model Canvas, Business Model Canvas, smart energy, local energy, community energy
Citation: Pappas D, Braunholtz-Speight T, Hannon M, Webb J, Fuentes González F and Sharmina M (2023) Business models for smart local energy systems—A triple layered perspective. Front. Sustain. Energy Policy 1:1058534. doi: 10.3389/fsuep.2022.1058534
Received: 30 September 2022; Accepted: 30 December 2022;
Published: 19 January 2023.
Edited by:
Dimitrios Zafirakis, University of West Attica, GreeceReviewed by:
Reza Hafezi, National Research Institute for Science Policy (NRISP), IranEdmilson Moutinho Dos Santos, University of São Paulo, Brazil
Copyright © 2023 Pappas, Braunholtz-Speight, Hannon, Webb, Fuentes González and Sharmina. This is an open-access article distributed under the terms of the Creative Commons Attribution License (CC BY). The use, distribution or reproduction in other forums is permitted, provided the original author(s) and the copyright owner(s) are credited and that the original publication in this journal is cited, in accordance with accepted academic practice. No use, distribution or reproduction is permitted which does not comply with these terms.
*Correspondence: Dimitrios Pappas, dim.pappas@outlook.com