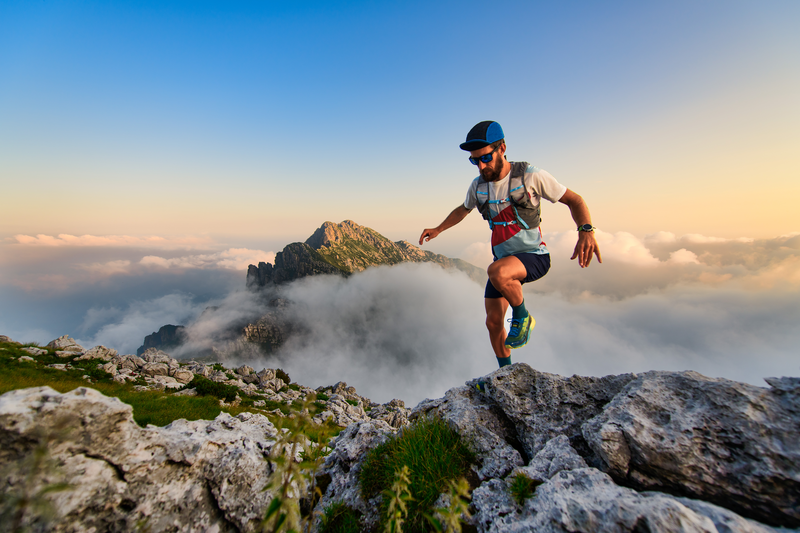
94% of researchers rate our articles as excellent or good
Learn more about the work of our research integrity team to safeguard the quality of each article we publish.
Find out more
ORIGINAL RESEARCH article
Front. Sustain. Cities
Sec. Urban Greening
Volume 7 - 2025 | doi: 10.3389/frsc.2025.1519375
The final, formatted version of the article will be published soon.
You have multiple emails registered with Frontiers:
Please enter your email address:
If you already have an account, please login
You don't have a Frontiers account ? You can register here
Various research has been conducted to enhance thermal comfort as a disturbed indicator in the unbalanced development of built environments. Some researchers have focused on the form and geometry of urban blocks as influential factors. Also, some have studied the effect of greenery in urban walls on improving thermal comfort. By defining 6 categories and 22 subgroups of the geometry of urban blocks in Tehran, this research is focused on identifying the effect of green walls on urban blocks in Tehran and the effect on thermal comfort in summer. Different geometries were changed based on changes in the direction of wind corridors, the height of blocks, and shading. The simulation of scenarios to conduct studies has been done in ENVI-met software. The results showed that changing the building typology (even by applying green walls) was insufficient to provide outdoor thermal comfort in Tehran; however, the separate cubes scenario provided better outdoor thermal conditions . The results of this study highlighted the importance of solar shading for outdoor spaces, as well as considering adaptive opportunities in site design for landscape architects and site designers.
Keywords: Building morphology, Urban canyon, Outdoor thermal comfort, ENVI-met, Urban blocks, Green wall
Received: 06 Nov 2024; Accepted: 10 Feb 2025.
Copyright: © 2025 Heydari, Yeganeh and Pourmahabadian. This is an open-access article distributed under the terms of the Creative Commons Attribution License (CC BY). The use, distribution or reproduction in other forums is permitted, provided the original author(s) or licensor are credited and that the original publication in this journal is cited, in accordance with accepted academic practice. No use, distribution or reproduction is permitted which does not comply with these terms.
* Correspondence:
Mansour Yeganeh, Tarbiat Modares University, Tehran, Iran
Disclaimer: All claims expressed in this article are solely those of the authors and do not necessarily represent those of their affiliated organizations, or those of the publisher, the editors and the reviewers. Any product that may be evaluated in this article or claim that may be made by its manufacturer is not guaranteed or endorsed by the publisher.
Research integrity at Frontiers
Learn more about the work of our research integrity team to safeguard the quality of each article we publish.