- 1Department of Landscape Architecture, Planning and Management, Swedish University of Agricultural Sciences, Alnarp, Sweden
- 2Gothenburg Botanical Garden, Gothenburg, Sweden
- 3Royal Botanic Garden, Kew, Richmond, United Kingdom
- 4Ethiopian Institute of Architecture, Building Construction and City Development, Addis Ababa University, Addis Ababa, Ethiopia
- 5Department of Research, and Collection, Gulele Botanical Garden (GBG), Addis Ababa, Ethiopia
Urbanization in Ethiopia is rapidly reducing green space and biodiversity, and placing ecological stress on trees. Urban street trees (UST) can provide multiple ecosystem services, but need to cope with challenging street ecology and future climate risks. However, UST selection in Ethiopia is poor, and conventional trials and modern breeding are costly and time consuming to apply in Ethiopia. The main objective of the study was to identify potential UST species for two Ethiopian cities; Addis Ababa, and Arba Minch, by studying interspecific differences in functional traits. For the purpose, we created a database with list of 120 candidate UST gathered from multi-stakeholder workshop, literature review, and senior experts survey, and their ecological with their phenological and morphological features. Then we filtered 25 potential UST through framework analysis considering eco-morphological features of the trees. For the 25 species, we measured three types of plant functional traits: conservative traits (leaf mass per area and leaf dry matter content), acquisitive traits (leaf area, specific leaf area and leaf water content) and drought tolerance traits (leaf turgor pressure loss point, and leaf succulence index). Our results showed a strong trade-off between conservative and acquisitive traits, particularly between leaf dry matter content (LDMC) and specific leaf area (SLA) (r = −0.71), consistent with plant economic spectrum theory. Using PCA analysis, we identified three groups of potential USTs (Type I, II and III) that exhibit different adaptation strategies, consistent with Grime’s trait-based classification of universal plant adaptation strategies (CSR). Compared to species with high acquisitive traits (Type II and Type III), Type I species have high conservative and drought-tolerant traits shows better adaptation to harsh road environments, whereas Type II and Type III species may be more advantageous for urban parks and other resource-rich components of urban ecology. We also found significant variation in conservative, acquisitive and drought-tolerant traits among different species, indicating their different adaptation strategies. Our research advances the knowledge of plant adaptation in urban environments and provides a useful method for UST selection.
1 Introduction
The issue of increasing urbanization is a global challenge, with African cities being among the fastest growing in the world (OECD/SWAC, 2020). Currently, approximately 60 million people reside in urban areas in East Africa, and this number is projected to increase to around 0.42 billion by 2050 (Statista, 2023). In Ethiopia, the number of people living in cities is currently 28 million, and this is projected to increase to 43 million by 2037 (Worldometer, 2024). Metropolitan cities in Ethiopia, such as Addis Ababa, Kombolcha, Arba Minch, Adama, Bahir Dar, and Gondar, have undergone significant expansion in recent years (Terfa et al., 2019). As a result, there have been concerns about the impact of this expansion on the urban environment, including the limitation of green spaces (Ayele and Tarekegn, 2020), a reduction in biodiversity (Güneralp et al., 2018), and the generation of pollutants, flooding, and other ecological stresses (Kosa et al., 2017; Ochola, 2018). It is important to consider the potential consequences of urban expansion and take measures to mitigate any negative effects on the environment and local communities. In urban infrastructure, it can be beneficial to incorporate tree plantings along streets. Such trees have the potential to help address issues related to temperature, air pollution, and flooding (Salmond et al., 2016), while also providing site-specific and recreational benefits through their unique seasonal qualities (Zhao et al., 2020). However, it is important to note that for trees to provide these ecosystem services, they must be able to withstand the challenging conditions of urban street environments. The selection of the appropriate tree for the location and climate is therefore crucial, as species selection is often linked to the tree’s long-term growth and vitality; properties important for delivery of ability to provide larger and healthier trees that can offer multiple ecosystem services (Pataki et al., 2021). Recent research suggests that environmental stress, particularly heat waves, droughts, and flooding, may intensify and increase in the future in Ethiopian cities, especially Addis Ababa (Alemu and Dioha, 2020). Therefore, it is important that future urban street trees in Ethiopian cities are able to cope with the challenging street ecology and potential climate risks.
Therefore, to comprehend the adaptation strategies of tree species to future climate risks and novel urban stresses are essential. As per Niklas et al. (2023), evolution compels plants to specialize in unique functional traits to thrive within their ecological niches. Consequently, it is observed that species, which experience drought, tend to exhibit certain characteristics, such as smaller and thicker leaves, greater stem density, and elongated taproots. In contrast, those inhabiting resource-rich and low-stress environments typically display different traits (Asefa et al., 2017). This phenomenon creates a two-dimensional spectrum of plant adaptation strategies (Joswig et al., 2022; Yang et al., 2021). Response-effect trait theory, environmental filtering theory, plant economic spectrum theory, and Grimes’ universal plant adaptation (CSR) theory are some of the plant adaptation theories based on functional traits (Pierce et al., 2017). The Grime’s CSR plant adaptation strategy theory has interpreted as C stands for competitor species, S stands for stress tolerances species, and R stands for ruderal plant species (Pierce et al., 2017). Additionally, Miedema Brown and Anand (2022) further classify traits into effect traits and response traits.
Response traits, such as leaf area (LA), specific leaf area (SLA), leaf dry matter content (LDMC), and specific succulence (LSI), have been found to be strongly associated with plant adaptation to specific stresses. Additionally, effect traits, such as carbon sequestration, have been found to be associated with the provision of ecosystem services (Miedema Brown and Anand, 2022). The plant economic-spectrum theory is a two-dimensional plant adaptation theory that classifies plant adaptation strategies as either acquisitive or conservative based on the association of response functional traits to resource availability (Pierce et al., 2013; Wright et al., 2004). Additionally, Grime’s CSR plant adaptation strategy is aligned with this theory, as it uses response leaf morphological traits to predict the CSR percentage (Pierce et al., 2017). Response leaf morphological traits are used to predict the CSR percentage, as demonstrated by Pierce et al. (2017). Goud et al. (2023), suggest that species with acquisitive traits in the plant economic spectrum are more likely to be competitors, while those with conservative traits are more likely to be stress-tolerators in CSR. Ruderal species, on the other hand, serve as intermediaries between acquisition and conservation. The concept of environmental filtering is considered to have superior analytical ability in correlating specific traits, organism abundance/distribution, and environmental variables/stressors (Asefa et al., 2017), distinguishing it from other theories. This theory is used to guide the selection of urban trees by matching environmental stressors, such as climate variables, and/or edaphic properties, to plant species and eco-morphological trait abundances (Sjöman et al., 2012). According to the theory, it is suggested that environmental stressors may influence plant eco-morphological features. This implies that the organism’s existence and traits are subject to environmental variables in a specific ecology or region (Asefa et al., 2017).
The integrated view of CSR-plant adaptation strategy, plant economic spectrum theory, and the effect response theory of plant functional traits provide a complete version of plant-environment interaction (Ruiz-Guerra et al., 2020). These theories are commonly used to predict ecosystem service modeling, explain species distribution models in their natural environments, understand plant adaptation strategies, and species selection for ecosystem restoration (Charles, 2018; Faucon et al., 2017; Pan et al., 2020). It is also increasingly discussed to understand the unique characteristics or traits that different species have developed through evolution in their natural habitats, to increase the accuracy of tree selection for urban environments (Rodríguez-Santamaría et al., 2022; Sjöman et al., 2018; Watkins et al., 2021). Watkins et al. (2021), led the way in applying the above functional trait-based theories of plant adaptation to urban tree selection. Their research showed that the majority of urban trees were arranged along Grime’s stress tolerance axis. They concluded that these trait-based theories could explain the differences in stress adaptation among different urban tree types. They also presented hypothetical trait-based models of urban trees in relation to the ecological stressors they face in urban environments. This approach could facilitate the identification of potential urban street trees (UST).
Urban street tree (UST) selection has not been studied in Ethiopia, despite the potential benefits of advancing plant functional trait-based theories in species selection. Therefore, in this study, we will advance this field by (i) identifying potential native alternative USTs suitable for stressful urban roads using eco-morphological traits, (ii) classifying species into different functional groups using multivariate trait analysis and CSR, the plant adaptation theory, and (iii) conducting interspecific and intraspecific trait analysis in relation to adaptation strategy to urban road stress. Due to the wide variation in climatic conditions in Ethiopia, we chose to work with two different cities, Addis Ababa and Arba Minch.
2 Methodology
2.1 Study area description
This research addresses two Ethiopian cities, Addis Ababa and Arba Minch, which are located in differing natural conditions about climate, vegetation, and topography. Addis Ababa, Ethiopia’s capital city, is located in the central highlands at 8° 57′ 05″ N to 9° 04′ 10″ N and 38° 42′ 12″ E to 38° 47′ 09″. The city’s altitude ranges from 2,100 to 3,100 metres above sea level, with Dry, Evergreen Afromontane Forest (DAF) vegetation (Heslop-Harrison, 2011). Annual temperatures ranges from 9°C to 25°C (Alemu and Dioha, 2020), while the monthly rainfall ranges from 0 to 300 mm (National Metrology Agency, 2023), and the average rainfall is about 1,213 mm between 1984 to 2013, while it was about 1512.9 mm in 2023. Addis Ababa, the capital city of Ethiopia, has a population of 5.3 million people (Population Stat, 2023) and covers an area of 530 km2 (Statista, 2019). The field data collection was conducted at Gullele Botanical Garden, which is situated in North West of Addis Ababa. Arba Minch is situated in the Great Rift Valley in Southern Ethiopia at 6°02′07” North Latitude and 37°33′23″ East Longitude. The dominant vegetation types are Acacia-Comphora woodland and bushland (ACB) (Heslop-Harrison, 2011). The city’s altitude ranges from 1,200 to 1,400 meters above sea level, and has a warm climate with temperatures ranging from 25° to 35° C. The annual rainfall is approximately 932 mm. Arba Minch City has an estimated population of 100,000 people according to the Geography department of the Arba Minch City Administration, which covers an area of approximately 20 km2. The filed data collection in Arba Minch City was conducted in Arba Minch forest surrounding the city.
2.2 Urban street tree selection process
We hosted a multi-stakeholder workshop (MSW) (Nielsen et al., 2017) with participants from six different cities, and six different universities, and research institutes. In the workshop, we formulated two group of participants based on (i) their home city, and the agro-ecology proximity their home city. For all the groups, we asked to suggest 7–10 candidate urban street trees that could deliver important ecosystem services such as cooling services, storm water management, and climate regulation for the two cities separately. We have also asked senior experts to suggest candidate UST using electronic email from different institutions for the two cities. In Addition, we also searched native urban trees grown in the cities and sister cities from the literatures. Then, we have created a dataset containing a list of 120 candidate UST by combining the list of species from all sources in which 80 of the species are for Addis Ababa and 40 of the species are for Arba Minch. The species were characterized based on their ecological and morphological features (eco-morphological features), and to some extent their phenological features. This was done using botanical books (Bekele, 2007; Friis et al., 2010), are published from a well know publishers, and acknowledged globally distinguished research, and development institutions such as ICRAF (World Agroforestry Research Center), and FAO. In Addition, the books are important for their detailed information to get a species level tree variables are most important to filter most important species. In addition, ICRAF tropical species database, World Flora Online, and JUSTRO Global Plant1 for their well-known recognition as species level plant information. Both the books, and the databases were used to extract eco-morphological features used to filtered the potential USTs. We considered morphological features such as maximum height, DBH, and canopy diameter are important factors to evaluate the tree faillurity risk as suggested by Jahani and Saffariha (2022a,b), and ecosystem delivery potential (Hand et al., 2019). We also extract ecological features including growth altitude range; vegetation types them dominantly grown, life form, phenology, and their interaction with other organisms are important for practioners to understand ecological adaptation of the species. All these information were used to filter the potential UST using framework analysis (Goldsmith, 2021). The analysis framework considered the eco-morphological features of trees, as well as prioritized ecosystem services such as cooling; stormwater management, and climate regulation, along with street plantation requirements (see Figure A1 in Appendix A). The morphological features considered were maximum height, maximum diameter at breast height (DBH), maximum crown width, crown shape, tree life form, and spinelessness. These features are valuable for optimizing plantation space, as recommended by several authors (Dowtin et al., 2023). Similarly, ecological features such as natural habitat conditions, growth altitude range, abundance to the local area, vegetation type where they grow naturally, and non-poisonousness were used as screening criteria in the framework analysis. These are good indicators of adaptation and ecosystem functioning (Behrens, 2010; Sjöman et al., 2012). Additionally, we considered evergreen tree species as a potential indicator of cooling services (Sastry and Barua, 2017). We considered tree species that are suitable for street greening components, such as walkways, cycle paths, median, and pocket plantations. This resulted in a list of 25 potential urban street trees (11 for Addis Ababa and 14 for Arba Minch). These trees are evergreen or semi-evergreen, non-poisonous, and non-spiny native alternative street trees. We excluded both dwarf, and giant trees, while medium sized trees were included to optimize the trade-off between the spaces limitation, and the ecosystem delivery potentials. We there after evaluated the species based on their functional traits (see Table 1).
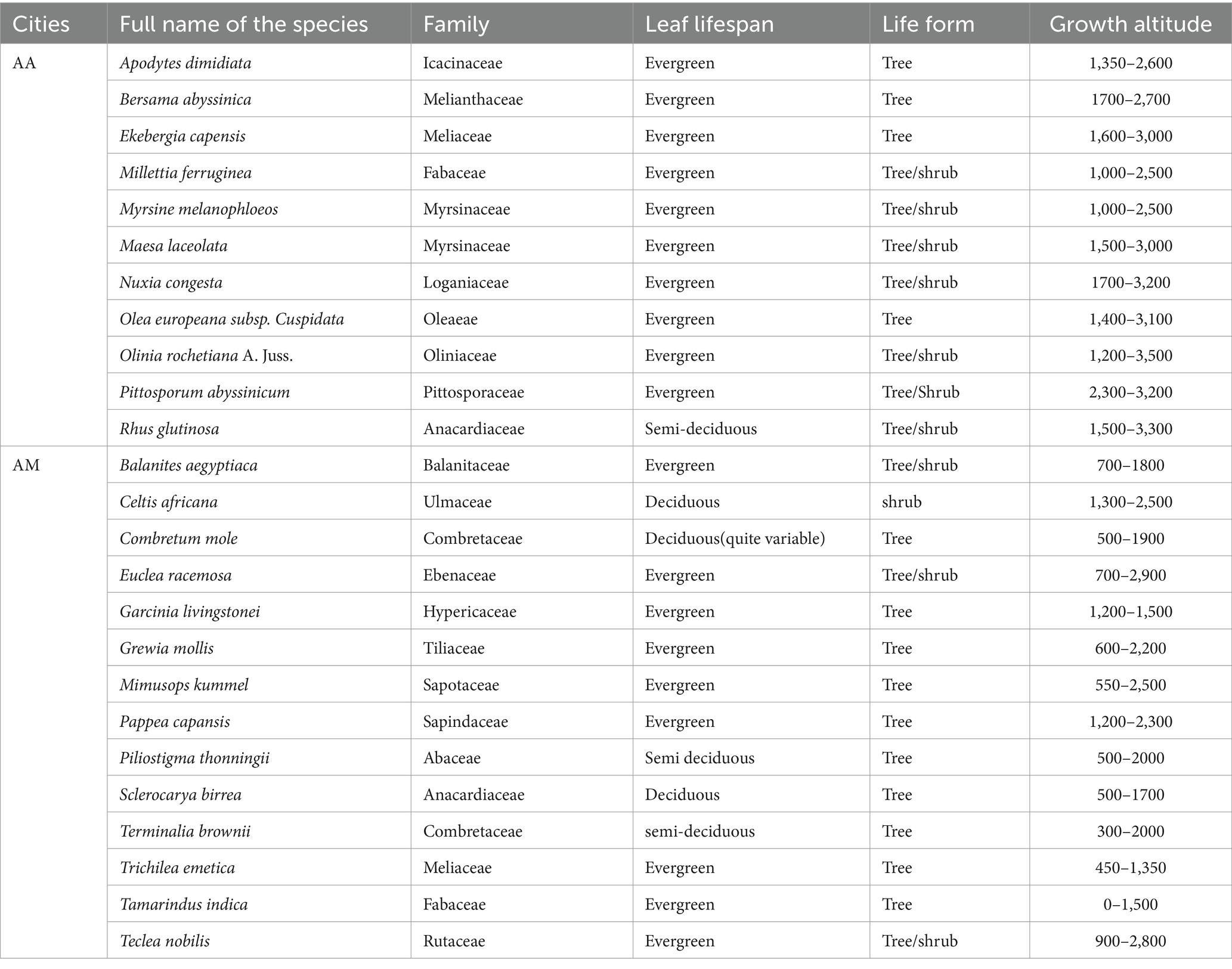
Table 1. Tree species and the characteristics of potential urban street trees we screened for functional trait-based evaluation for Addis Ababa (AA) and Arba Minch (AM).
2.3 Measurement of leaf and wood functional traits
The street soil compaction, intensification of temperature and water scarcity are the major challenges to grow trees in both Addis Ababa, and Arba Minch. The average soil bulk density in Addis Ababa and Arba Minch is 1.76 g/cm3 (Andualem, 2018) and 1.78 g/cm3 (Toyebo, 2020) respectively, which indicates stronger soil compaction for plant growth (1.10 to 1.60). In addition, concrete, and cobblestone pavements are also major challenges in the street condition. However there is limited documented evidences, plastic, and other chemical soil pollution is moderately increasing in the Addis Ababa (Aschale et al., 2017), and Arba Minch city (Guadie et al., 2021). These all together stressed the plant growth in the street, which urges the need of the stress tolerator species for such stressed environment (Czaja et al., 2020). The atmospheric concertation particulate matter and other pollutant gases are increasing in both Addis Ababa (Kumie et al., 2021; Tefera et al., 2021), and Arba Minch (Dingemanse et al., 2022; Dingemanse and Tademe, 2023) that impose stress on the human beings, and plants. Thus, the use of the proposed functional traits particularly leaf morphological traits enable us a better understanding of the plant adaptation strategies (Zhu et al., 2021), and ecosystem services related to hazard reduction in the urban environment (Gastauer et al., 2020). It is recommended to use multi-trait evaluation rather than relying on single functional traits in plant adaptation research (Wang et al., 2023). Thus, we measured multiple conservative and acquisitive functional traits, which are valuable for understanding adaptation strategies in different tree species. This is because urban environmental stress is complex and the relationship between environment and traits is multi-layered (Maynard et al., 2022; Lefcheck et al., 2015). Based on this recommendation, we measured leaf functional traits and specific stem density (SSD). These traits do not require sophisticated laboratory infrastructure, which makes the traits suitable to use in countries such as Ethiopia, and have shown consistency in plant functional ecology analysis at a global scale with ample scientific evidence available to apply them (Maynard et al., 2022). The measured traits are widely used in plant adaptation theory construction, such as Grimes CSR-theory, fast-slow plant adaptation theories, and LHS theory (Pierce et al., 2017; Reich, 2014).
We collected leaf samples from Gullele Botanical Garden and Arba Minch natural forest using simple random sampling for trees with greater than 5 cm DBH, and five replications (individual trees) for each species between June 19/2023 and July 07/2023 (Ethiopian rainy season). It was difficult to find all the species in one site to establish homogenous temporary sampling plots. Thus, we walk in the total sampling area to find the target plant species. First, we collected five twigs from each individual tree that were healthy and fully exposed to sunlight. We then placed them in a polythene plastic bag, and put them under darker and cool place to reduce water loss until taking to the laboratory within a maximum of 2 h (Pérez-Harguindeguy et al., 2013). In the laboratory, we sampled five healthy, fresh, and fully expanded leaves from different twigs for each species.
Each leaf was scanned using a Canon Scanner to determine the leaf area (LA). The fresh mass was weighed immediately after scanning using an analytical balance with 0.001 g accuracy. The leaves were then dried for 72 h at 70 ̊C using a drying oven, and the dry mass was weighed with the same analytical balance. Wood core samples were taken from each tree to determine stem specific density (SSD) using an increment borer with a diameter of 5.38 mm at a height between 1 and 1.5 metres from the ground. The fresh length of the core samples (in cm) was measured using a ruler and they were then placed in a labeled plastic straw, with both sides plastered. The core samples were placed in a drying oven at 70°C for 72 h, and the dry mass of each sample was measured by removing the straws and using an analytical balance with 0.001 g accuracy. The traits’ significance and calculation are summarized as follows:
• LA (leaf area) represents the total area of one side of the leaf and is a significant functional trait associated with photosynthesis, evapotranspiration, radiation interception, and competition ability of the species (Westoby, 1998). It is also essential for calculating other leaf traits such as SLA, LMA, LSI, and LT. The LA was calculated from the scanned image of the fresh leaf using ImageJ software (Martin et al., 2020).
• LDMC (leaf dry matter content) is defined as the ratio of dry mass to fresh mass, in contrast to LWC, and was determined using partial hydration method (Vaieretti et al., 2007; Vendramini et al., 2002). It is associated with plant net primary production (Smart et al., 2017), plant adaptation strategies (Polley et al., 2022), and leaf chemical composition (Wang et al., 2022). Ecologically, species with higher LDMC tend to have longer leaf lifespan and greater stress tolerance capacity (Maynard et al., 2022).
• LWC (leaf water content); the current LWC was calculated by dividing leaf water mass to leaf fresh mass. The leaf water mass is the differences between leaf fresh mass, and leaf dry mass of a fully expanded, and exposed healthy leaf (Wang et al., 2022). The leaf fresh mass was within a maximum of 2 h to minimize the errors due to water loss instead of rehydration (Pérez-Harguindeguy et al., 2013). LWC influences several other functional traits, including leaf photosynthesis and area, which are critical for plant growth rate, resource use efficiency, leaf gas exchange, and thermal regulation (Wang et al., 2022).
• SLA (specific leaf area); defined as the leaf area per unit of dry mass and is associated with higher relative growth, resource acquisition, and covariance for drought response of plant species (Dwyer et al., 2014). It is one of the most important/commonly used leaf functional traits associated with light irradiance interception and photosynthetic efficiency in a unit of mass assimilated (Meziane and Shipley, 2001). Plants with a higher SLA are characterized by a high relative growth rate and a strong capacity to compete for soil and light resources. They also exhibit higher evapotranspiration activity but are less resistant to biotic and abiotic stresses compared to plants with a lower SLA and higher LMA (Dwyer et al., 2014).
• LMA (leaf area per mass); is mathematically and functionally reversed to SLA, that is, the dry mass of the leaf per fresh leaf area (Pérez-Harguindeguy et al., 2013). LMA is a good indicator of leaf longevity, leaf thickness, and density leaves, which are associated with resistance to damage and stresses (De La Riva et al., 2016). Thus, the combined use of SLA and LMA will allow us to show the trade-off in resource allocation for growth and safety
• SSD (Specific stem density) is calculated as the dry mass of the wood core sample per fresh volume of the wood core sample (Pérez-Harguindeguy et al., 2013). SSD is a conservative trait, indicating that species with higher SSD have greater stress tolerance for both abiotic and biotic stresses, including physical damage and pests (King et al., 2005; Pavanetto et al., 2024). The formula used to determine SSD is SSD=WDM/V (Maynard et al., 2022). WDM represents the dry weight of the wood core sample, while V represents its fresh volume (cm3). The fresh volume can be calculated using the formula V = π*r2*l, where r is the radius of the core sampler (2.15 mm) and l is the length of the fresh wood core sample in mm. Therefore, V in mm3 is equal to 14.52 (mm2)*l. This can be further simplified as SSD=WDM/ (14.52*l), where SSD is in mg mm−3. SSD and LDMC are parallel traits that provide insight into carbon allocation partition between leaf and woody components of trees (Cope et al., 2021).
• LSI (The leaf succulence index) was calculated as the leaf water mass per leaf area (Mantovani, 1998). LSI provides insights into plant adaptation, physiology, and ecology by measuring the water storage capacity of plant leaves. Plants with higher LSI have a greater potential for drought resistance by storing physiologically usable water in their leaves. Such plants are well known for their ability to adapt to semi-arid environments (Guo et al., 2023). Plants with a higher leaf succulence index may be better adapted to urban street ecology due to the prevalence of drought, which is a major challenge in these areas, as well as the potential effects of future climate change (Guo et al., 2023).
• LTPLP (leaf turgor pressure loss point) refers to a plant’s ability to maintain cell turgor pressure under drought stress (Maréchaux et al., 2015; Zhu et al., 2018). Several field and experimental studies have used LTPLP as a strong indicator of drought resistance. These studies define species with a more negative value of LTPLP as more drought-resistant (Maréchaux et al., 2015; Zhu et al., 2018). In our study, we sampled healthy, fully expanded, and exposed leaves from 5 individuals using a similar design to the above traits. From each leaf we took a leaf core sample from the middle of the leaf using the 5 mm diameter leaf disc sampler. We promptly covered the leaf cores with aluminium foils, labelled them, and submerged them in liquid nitrogen for 5 to 10 min. We used the Wescor EliTech Vapro 5,600 Vapor Pressure Osmometer by calibrating according to standard procedure. Subsequently, we removed the samples from the liquid nitrogen and the aluminium foil, and punched the leaf core using a puncher 5 to 10 times and put the sample into the osmometer to read the osmotic potential of the leaf within 10 to 15 min. Calculate the LTLP using the following formula: LTPLP = −0.2554 + 1:1243*Ψp100, where LTPLP and Ψ100 are the turgor pressure loss point and leaf water potential at full turgor pressure, respectively (Zhu et al., 2018) (see Table 2).
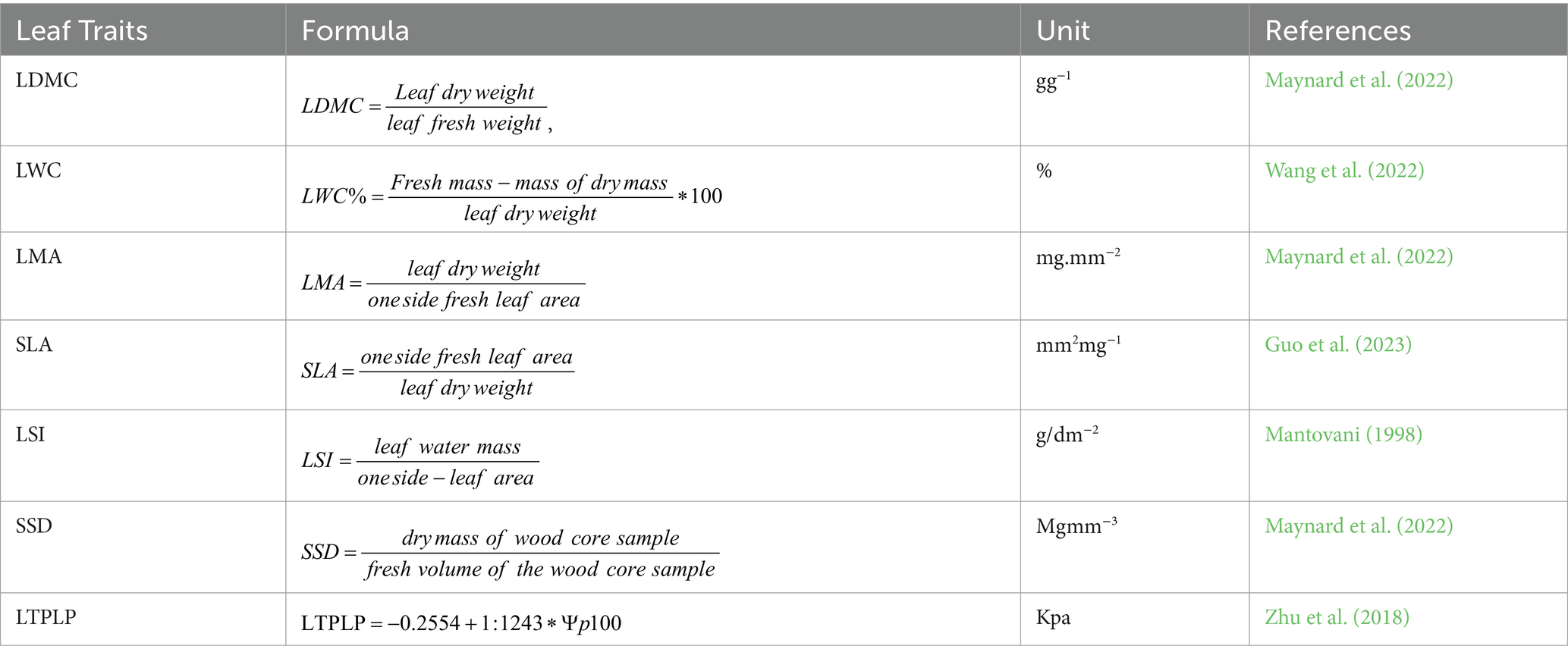
Table 2. Summarizes the functional trait measures for the potential urban trees proposed by the framework analysis above.
2.4 Data analysis
The traits were classified into three categories: acquisitive traits (LA, SLA, and LWC), conservative traits (LMA, LDMC, and SSD), and drought resistance traits (LSI, LT and LTPLP) (Gorné et al., 2022; Maracahipes et al., 2018). Acquisitive traits are positively correlated with resource acquisition, growth rate, and photosynthesis rate, while conservative traits are associated with species stress tolerance and Damage resistance (Maracahipes et al., 2018). Drought tolerance traits are traits specifically associated with water use efficiency and drought resistance (Obispo, 2019). To ensure consistency, we transformed the data into natural logarithm for all functional traits except SSD and LTPLP, as the variation between traits value and scale was not considerable (Lee, 2020). We used Spearman correlation to investigate the non-parametric correlation between the functional traits. Principal component analysis (PCA) was used to investigate trait-to-species ordination, as has been done in several local and global scale trait-based studies (Farrell et al., 2017; Tameirão et al., 2021). Grimes’ trait CSR plant adaptation theory was applied to classify species into competitors, stress-tolerant, and ruderal groups, which is also widely used in plant adaptation research, including urban forestry (Pierce et al., 2017). The percentage of CSR thresholds was calculated and species were assigned as CSR and sub-class of adaptation strategies using an Excel spreadsheet developed by using the LA, SLA, and LDMC based on Pierce et al. (2017). The species ordination along CSR plant adaptation strategies was visualized using the triangular scatter plotting technique. We used one-way ANOVA, and Tukey mean separation technique to investigate inter-species trait variation. A bar chart was also used to display inter, and intra-specific trait variation within species. All inferential statistics were performed using Origin software.2 Since each city has a unique climate and ecology, it is strategically sensible to analysis data for each city separately. This approach ensures that interventions are appropriate for the particular circumstances of each city by providing a locally adapted UST selection that is necessary for efficient urban planning and policy-making.
3 Results
3.1 Correlation between functional traits
Similarly, in Addis Ababa, there was a strong positive correlation coefficient of 0.67 between LDMC and LMA, but this correlation was insignificant in Arba Minch (Table 3). For both cities, LWC was strongly and positively correlated with LA, LSI, and SLA (Table 3), which implies the synergy of acquisitive traits in resource investment for growth and reproduction. Specifically, LTPLP exhibits a negative correlation with SSD, LDMC, and LMA, whereas it shows a positive correlation with LSI, LWC, LA, and SLA (Table 3). It is important to understand that positive correlations should be interpreted inversely, indicating that LTPLP is an indicator trait for drought tolerance. The correlation between LTPLP and conservative and acquisitive traits aligns with plant economics theory, which helps in selecting tree species suitable for urban environments, particularly drought-tolerant ones.
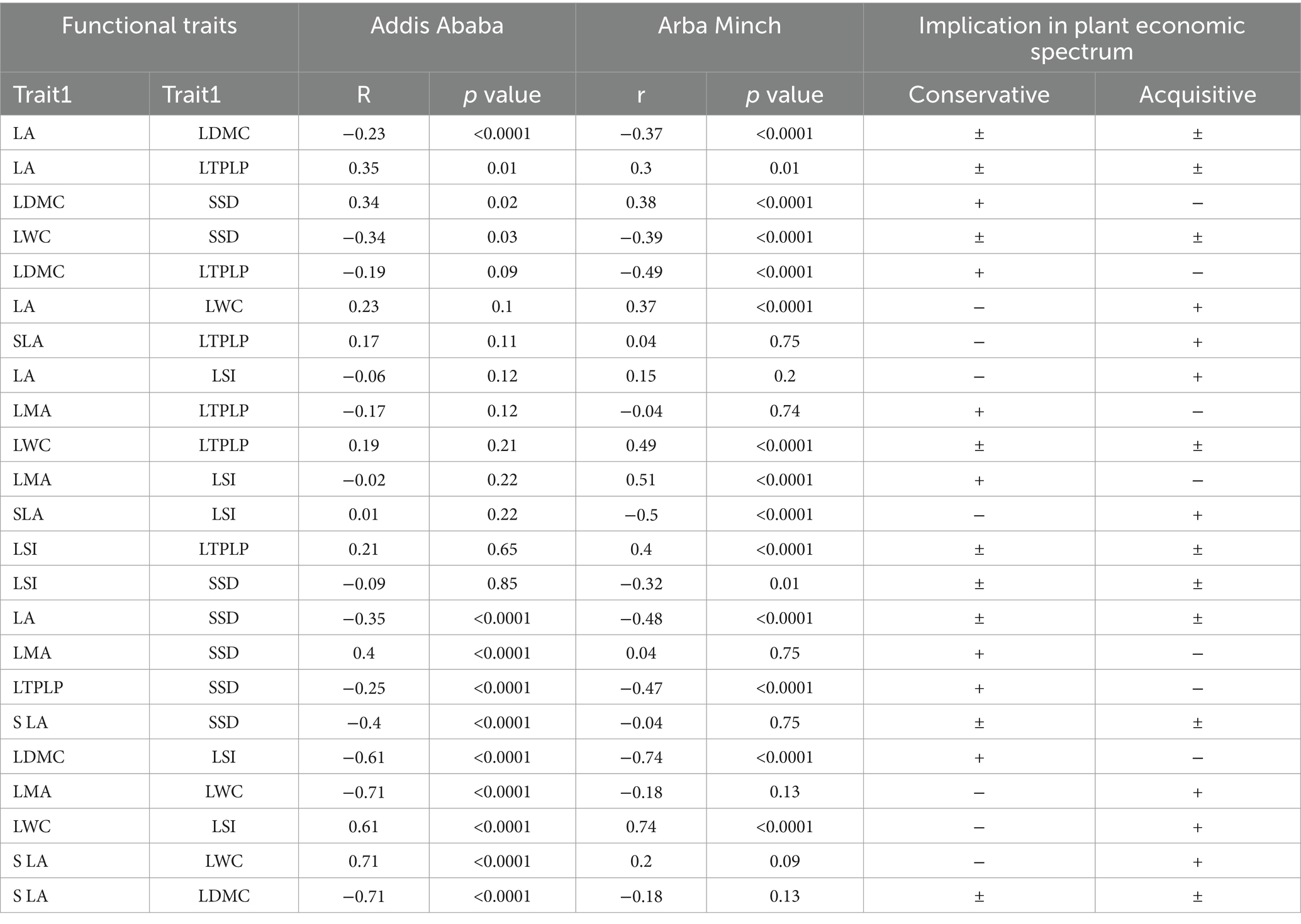
Table 3. Shows the Spearman correlation between the functional traits of species proposed for both cities and the implication of the correlation in two-dimensional plant adaptation strategy (plant economic spectrum).
A strong negative correlation was observed between SLA and LDMC for all species in Addis Ababa (r = −0.71), confirming that the plant economic spectrum theory was applicable to the included species. This theory suggests a trade-off between resource investment for growth and leaf structure construction. Additionally, the strong negative correlation between LDMC and LSI (r = −0.61, and r = −0.74, Addis Ababa, and Arba Minch) is consistent with this theory, as it shows a trade-off between leaf resource investments in structural and leaf water storage cells. Additionally LDMC was positively correlated with specific stem density (SSD) (r = 0.34, and 0.38 in Addis Ababa, and Arba Minch respectively).
3.2 PCA, and grimes CSR plant adaptation theory based classification
The result showed that species in both cities were ordinated with two principal component dimensions (PC1 and PC2) based on PCA and Grimes CSR Plant Adaptation Theory. PC1 was explained by the combinations of acquisitive traits, accounting for 43.24% in Arba Minch and 48.28% in Addis Ababa, while PC2 was more explained by conservative traits, accounting for 30.20% in Arba Minch and 22.7% in Addis Ababa (see Figures 1, 2). This resulted in the identification of three groups of species in both Arba Minch and Addis Ababa, which we will refer to as Type I, Type II, and Type III species (see Figures 1, 2). Type I species are characterized by higher conservative traits, lower acquisitive traits, and a greater tendency towards drought tolerance, as indicated by their ordination with PC2. Type I species for Addis Ababa include Olea europeana subs. Cuspidata, Olinea rochetiana, Apoddytus dimiadata, Nuxia congesta, Pittosporum abyssinica, and Mersine melanopoleous. For Arba Minch, Type I species are Combiritum molle, Euclea racemosa, Grewia mollis, Terminalia brownii, Memosopis kummel, Celtis africana, and Papaea capaensis. Both Type II and Type III species in both cities show higher acquisitive traits and lower conservative traits, as indicated by PC1. However, they differ in their degree of association with the PC1 axis. In Addis Ababa, Ekebergia capensis, Melitia furrugnea, Bersama abyssinica, and Rhus glutinosa are grouped as Type II species, while in Arba Minch, Balanites eagyptiaca, Garcinia livingstonei, Pilostigma thonnningi, Teclea noblis, and Sclerocarya birrea are grouped as Type II species (Figure 1). Only Measa laceolata for Addis Ababa, and Tamarndis indica and Trichilia emetica for Arba Minch are species belonging to the third group (Type III) that show strong ordination with LA, SLA, and LWC (see Figure 2).
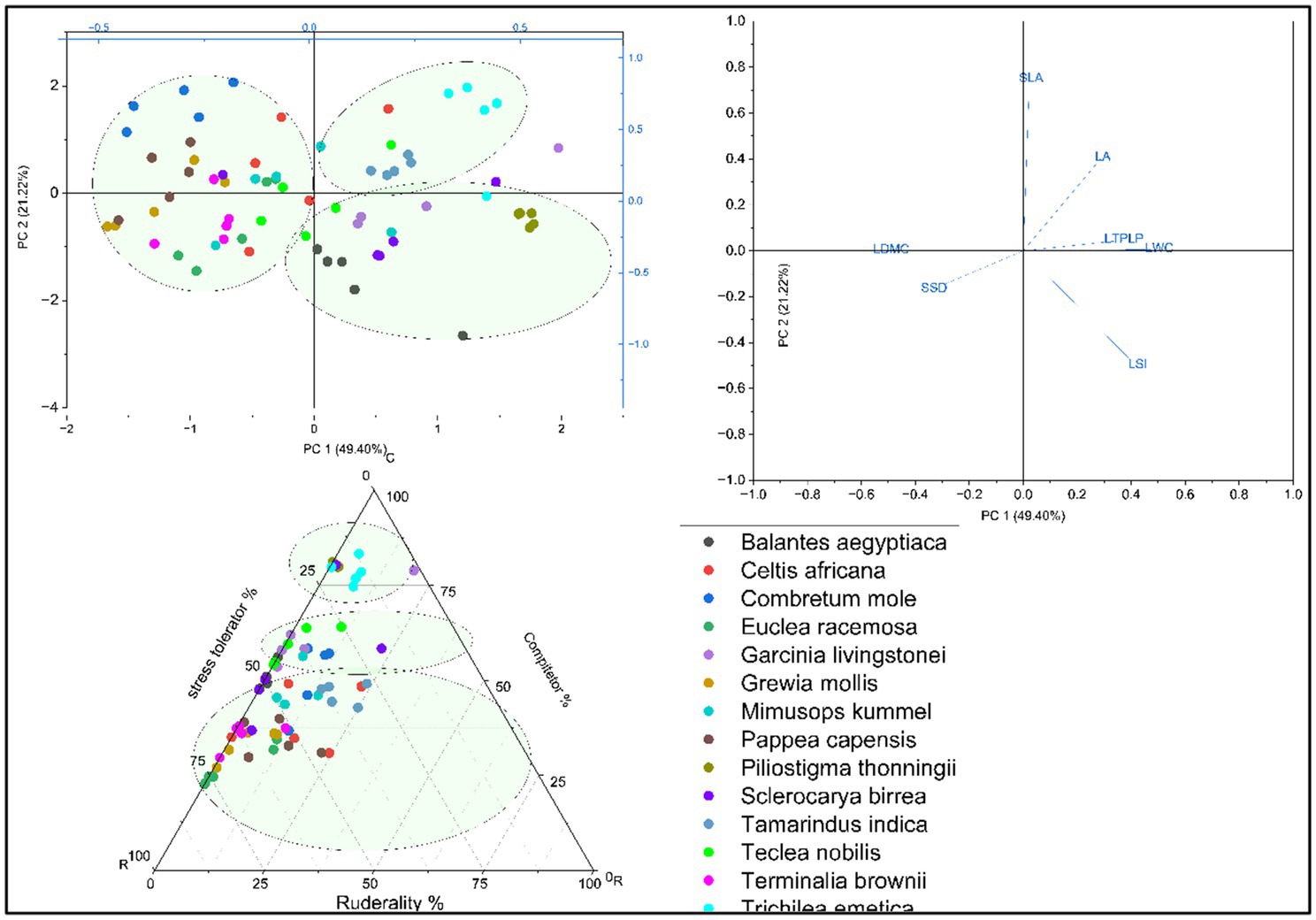
Figure 1. The ordination of species with PCA dimensions, the Grimes CSR plant adaptation strategy axis, and the coordination between the two classifications approaches for Arba Minch species.
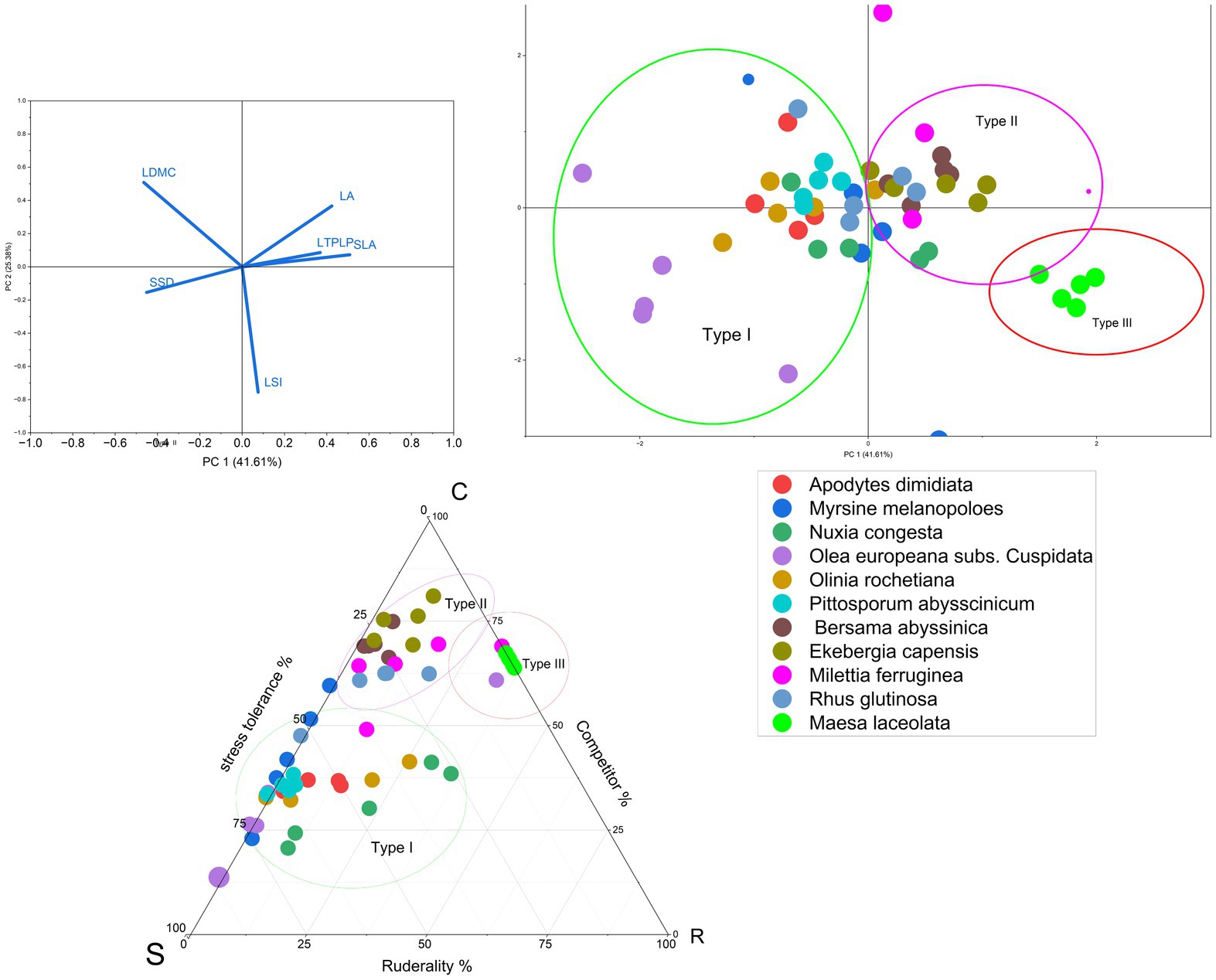
Figure 2. The ordination of species with PCA dimensions, the Grimes CSR plant adaptation strategy axis, and the coordination between the two classifications approaches for Addis Ababa species.
According to Grime’s CSR model Bersama abyssinica, Ekebergia capensis, Milettia ferruginea, Measa lanceolata, and Rhus glutinosa were characterized by S < 50%, R < 50%, and C > 50%. Most of these species were classified as Type II species in the PCA-based classification (Figure 2). Similarly, in the PCA classification, Type I species are characterized as having C < 50%, S > 50%, and R < 50%, which implies that they have a higher potential probability of being drought tolerant. In Arba Minch, Trichilia emetica, Garcinia livingstonei, Combretum mole, and Teclea nobilis are among the species with S < 50%, R < 50%, and C > 50% (Figure 1). However, some species cannot be classified into one of the three groups in both CSR and PCA classification types, as proposed for both cities. Tecla nobilis, Sclerocarya birrea, and Celtis africana from Arba Minch, and mainly Milletia ferruginea and Myrisne melanophloeos from Addis Ababa showed larger intra-specific trait variation, as shown in Figure 2.
3.3 Interspecific functional trait variation among species
The bar chart presented the significant mean variation of all functional traits examined (Figures 3–5) for the species sampled for both Addis Ababa and Arba Minch. In comparison, Bersama abyssinica, Apodytes dimidiata, Myrsine melanophloeos and Pittosporum abysssinicum did not show significant differences in their mean trait values (Figures 3, 4). In Figure 3 Measa lanceolata and Melittia ferruginea show significantly higher SLA than Bersama abyssinica, Apodytes dimidiata, Myrsine melanophloeos, and Olea europeana subsp. Cuspidata for species suggested for Addis Ababa. In Figure 4, Olea europeana subsp. cuspidata, Myrsine melanophloeos, and Pittosporum abysssinicum exhibit higher LMA indicating greater stress tolerance compared to the other species suggested for Addis Ababa. In Figure 4, it can be seen that only Olea europeana subsp. cuspidata had significantly higher SSD, and Measa lanceolata had significantly lower LDMC, and SSD compared to the rest of species suggested for Addis Ababa. Figure 4 also showed that Combretum molle, Euclea racemosa, Grewia mollis, Pappea capensis, and Terminalia brownii have higher LDMC and LMA values compared to Balanites aegyptiaca, Trichlea emetica, Tamarindus indica, Garcinia Livingstonei, and Piliostigma thonningi in Arba Minch. In addition, Pappea capensis and Grewia mollis exhibit higher SSD values than Trichlea emetica, Tamarindus indica, Piliostigma thonningii, Euclea racemosa, and Sclerocarya birrea. SLA, LWC, and LA are leaf morphological traits critical to plant resource acquisition strategies and exhibit similar patterns across species (see Figure 3).
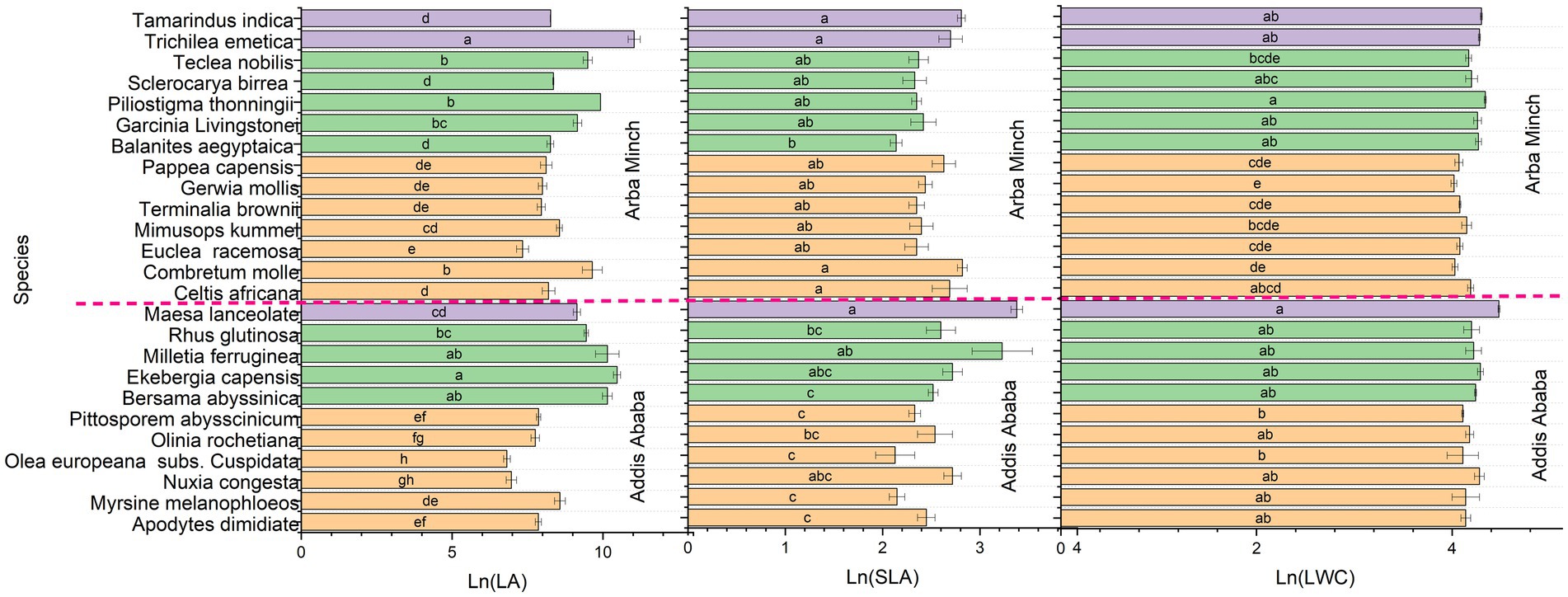
Figure 3. The significant variation of plant functional traits among species based on the natural logarithm value of traits and where LA is the leaf area, LWC is the leaf water content; SLA is the specific leaf area. *Species sharing the same letter are not significant within each city.
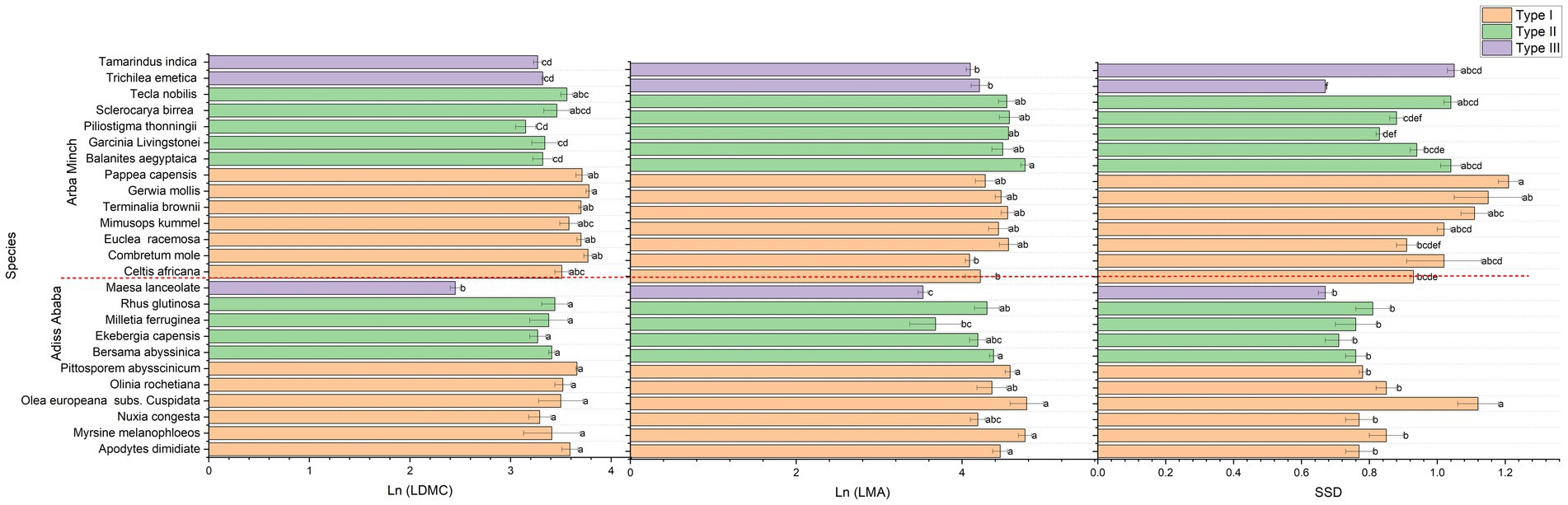
Figure 4. the significant variation of plant functional traits among species based on the natural logarithm value of traits except for SSD and LTPLP, and where LDMC is the leaf dry matter contents, LMA is the leaf mass per area, SSD is the specific stem density, and for Addis Ababa, and Arba Minch. *Species sharing the same letter are not significant within each city.
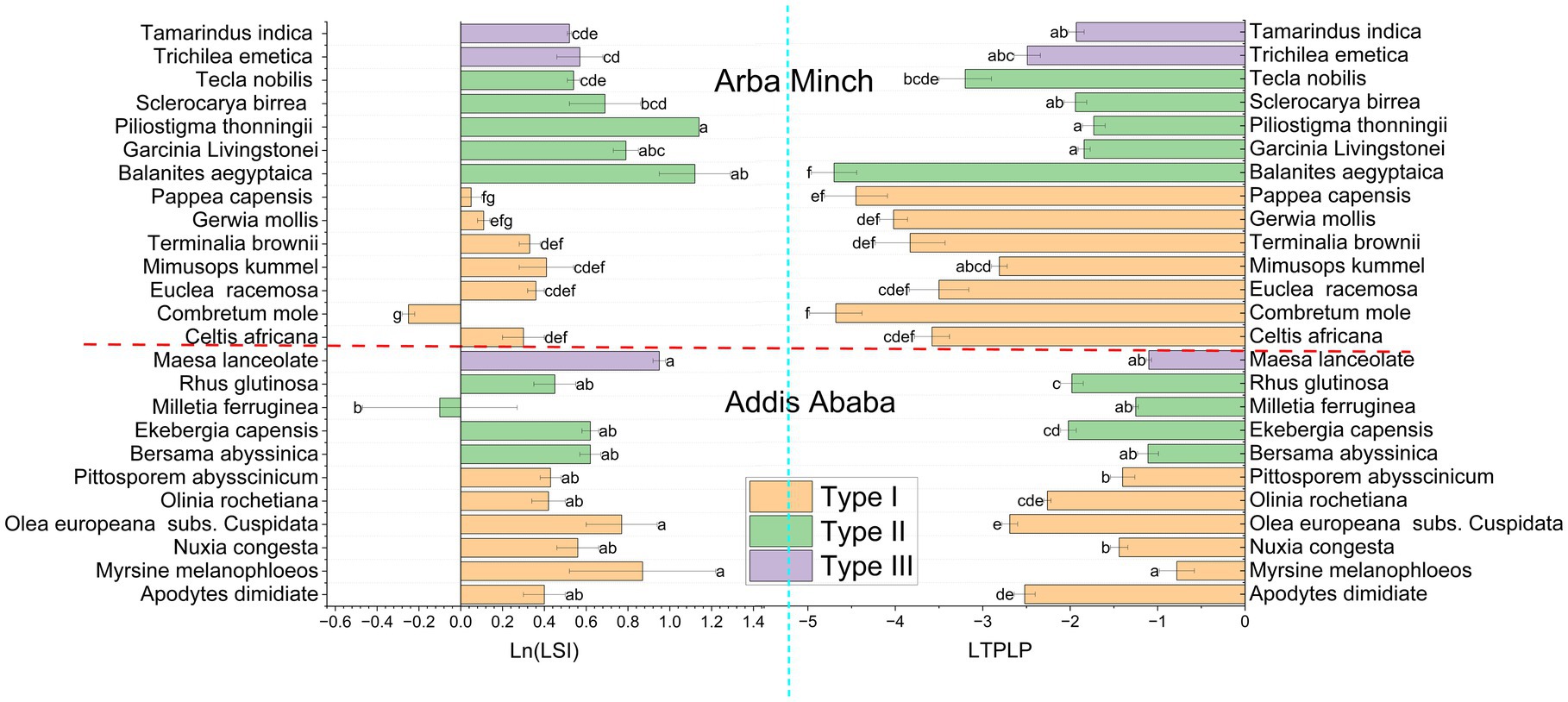
Figure 5. The significant variation of LSI and LTPLP for both Addis Ababa, and Arba Minch. Where LSI is the leaf succulence index, and LTPLP is the leaf turgor pressure loss point. *Species shared the same letters within each city are not significant differences for each traits.
In Figure 5, Olea europeana subsp. cuspidata, Apodytes dimidiata, Olinia rochetiana, Ekebergia capensis, and Rhus glutinosa showed significantly more negative values of LTPLP compared to Measa lanceolata, Melittia ferruginea, Nuxia congesta, and Bersama abyssinica for species suggested for Addis Ababa. Figure 5 also Balanites aegyptiaca, Combretum mole, Celtis africana, Euclea racemose, Grewia mollis, Pappea capensis, and Terminalia brownii had more negative LTPLP compared to Garcinia Livingstonei, Piliostigma thonningii, Sclerocarya birrea, Trichilea emetica, and Tamarindus indica in species suggested for Arba Minch. In terms of plant adaptation strategy, the inter-specific trait variance was generally consistent and aligned with the acquisitive, conservative, and drought tolerance traits for most of the species (see Figures 3–5). For example, Olea europeana subsp. cuspidata, Grewia mollis, and Pappea capansis exhibited higher LDMC and SSD, lower values of LA, SLA, and LWC, and a more negative LTPLP, demonstrating the stress-tolerance of these species. Species with Measa laceolata and Trichilea emetica exhibited a reverse adaptation strategy, which is a more competitive adaptation strategy, and have compound and broad leaves for both. In contrast, the more conservative species in this study have simple and short leaves. This is also true phonologically. The species in this study that are more conservative traits have simple and small leaves, while species with high acquisitive trait values have compound and broad leaves. This trend is consistent for both cities (see Table 2).
4 Discussion
4.1 Linking plant functional adaptation to urban street ecosystem
Elevations of heatwaves, drought, pollution, and flooding are significant stresses on urban trees, reducing tree survival, growth potential, and ecosystem service provision (Aalipour and Nikbakht, 2021; Liu et al., 2023). Furthermore, urban streets present a multitude of ecological stressors for trees, including a lack of soil volume, paved area, drought, heat, waterlogging, and pollution. These stressors are even more pronounced than those observed in other urban plantation sites (Czaja et al., 2020; Beecham and Lucke, 2015). Consequently, matching the plant adaptation traits (Maynard et al., 2022) with significant urban stresses for trees in the urban street ecology improves the urban tree selection (Levinsson et al., 2024; Sjöman, 2015; Sjöman et al., 2012). In line with this research, efforts are being made to develop functional trait-based urban tree selection frameworks. Grilo et al. (2022) developed a trait-based framework to address the interaction of anthropogenic effects on the phenotypic expression of traits, ecosystem service delivery, and ecosystem service-based urban planning. Andersson et al. (2021) have developed a framework to link effect and response traits, and the concept of filter traits concerning sustainable urban planning. Farrell et al. (2022) also introduced a trait-based framework for the selection of appropriate trees for Australian cities in the context of climate change adaptation. All the aforementioned frameworks concur that stress-tolerant plants are more adaptable to the stress-laden, ever changing, and confined urban plantation ecology.
We found that Watkins et al. (2021) trait based framework for urban forest species selection is more relevant to apply for this study. This is because of Watkins et al. (2021) developed a simplified urban tree selection process by matching Grime’s CSR triangular plant adaptation strategy and the two-dimensional plant economic spectrum theories in relation to hypothetical urban plantation sites. We have illustrated this framework to match our tested species by plotting species along the S and C Grimes axes (see Figures 6, 7). The plot is corroborated by correlation analysis, multi-trait variation, and intra-specific trait variation analysis, which imply that the species mainly represented are Olea europeana subsp. cuspidata, Apodytes dimidiata, Rhus glutinosa, Ekebergia capensis, and Olinia rochetiana adapting the urban ecology of Addis Ababa (Figure 6). It is also likely that the species Terminalia brownii, Pappea capensis, Grewia mollis, and Euclea racemosa will adapt to the urban street ecology in Arba Minch city (Figure 7) given their higher values of LDMC, SSD, and LMA compared to other species. A negative LTPLp value indicates a strong drought tolerance indicator trait, which is consistent with the drought tolerance potential of these species. This justifies our recommendations.
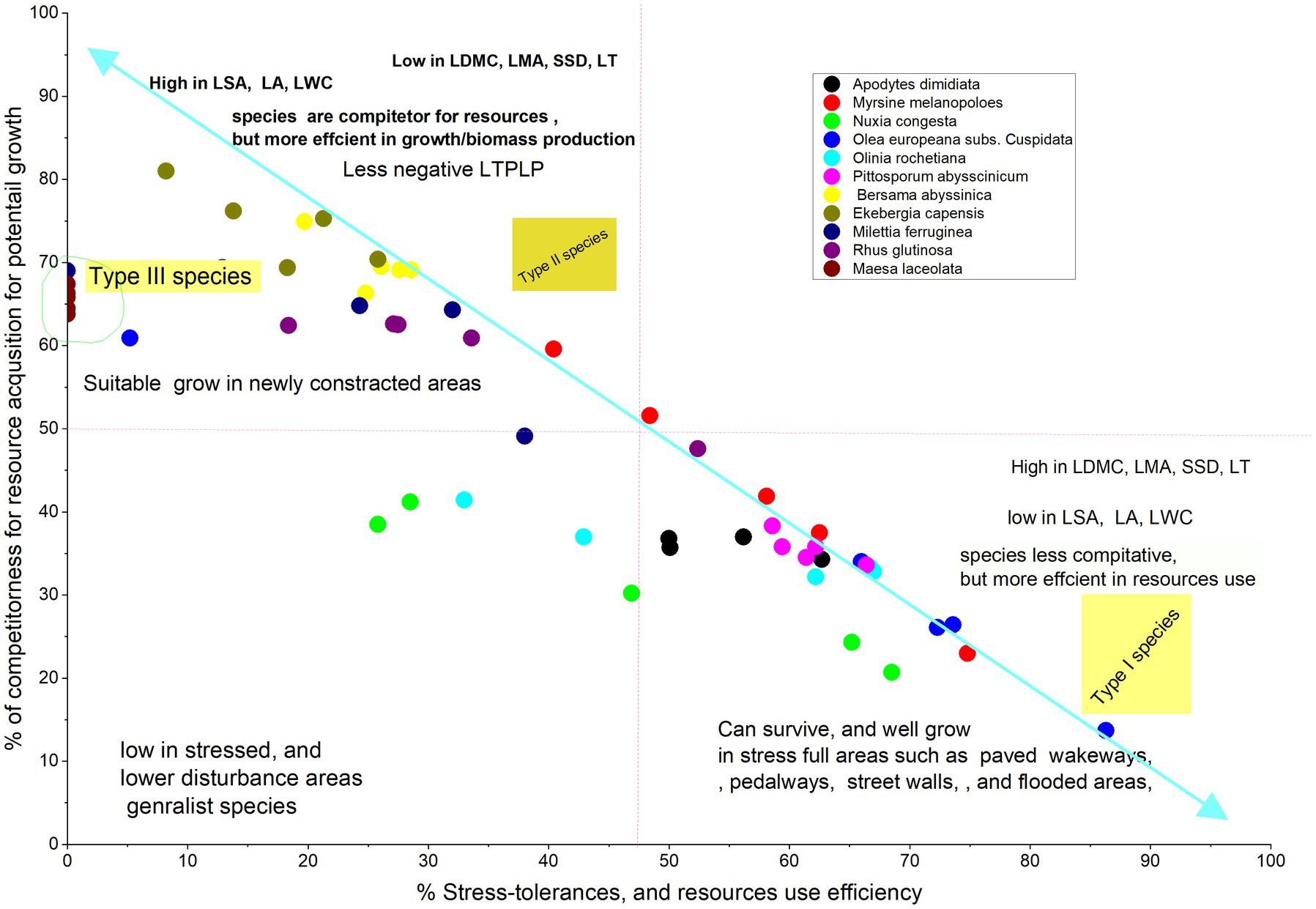
Figure 6. Shows the ordination between the PCA based functional groups of species with the CSR percentages according to Grimes theory, which confirms the consistency of the classification, where LTPLP is the leaf turgor pressure loss point, C is the competitor, and S is the stress tolerance: species proposed to Addis Ababa.
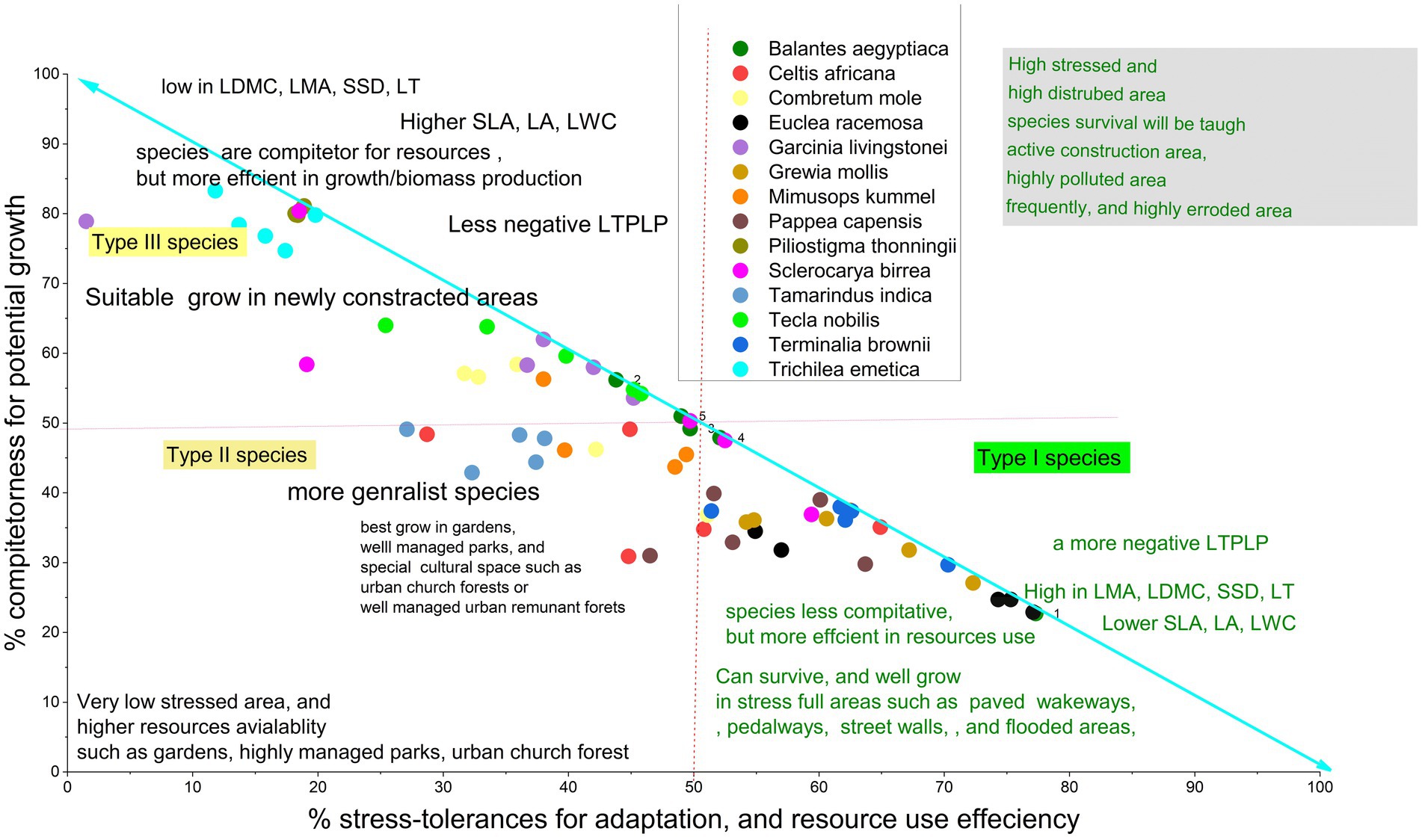
Figure 7. Shows the ordination between the PCA based functional groups of species with the CSR percentages according to Grimes theory, which confirms the consistency of the classification, where LTPLP is the leaf turgor pressure loss point, C is the competitor, and S is the stress tolerance for species proposed to Arba Minch.
4.2 The eco-morphology feature for potential USTs selection
The study highlights adaptation strategies of potential USTs using functional traits in response to various abiotic stresses. Using eco-morphology features of trees, we identified USTs that could be suitable for streets in each of these two distinct cities. It is our contention that the use of eco-morphological features in UST selection has the potential to optimize the potential of trees to fit the street ecology in size, ecosystem services provision and stress adaptation (Edward and Sadowski, 2007). Tree morphological features we have considered include maximum height, maximum DBH, maximum crown width, and the leaf lifespan those together imply the ecosystem service potential of UST including cooling services, stormwater management, and carbon sequestration (Simovic et al., 2024). According to Jahani and Saffariha (2022a,b) some of these morphological features has been used to predict the urban tree faillurity risk together with other soil, and climatic variables. However predicting tree faillurity risk is a crucial aspect for urban street tree management, we used these variables from the perspective of ecological adaptation.
It is thought that urban streets are best suited to medium-sized trees, both in terms of their height and width (Coleman et al., 2022). This is because they are more suitable to the street structure where too large trees increase conflicts with buildings and decrease accessibility (Zairuddin et al., 2020). In an Ethiopian setting, it is also important to consider the aboveground power lines, to avoid repeated and unwanted pruning. Considering ecological features such as the range of growth altitude, types of vegetation in which the species naturally grows, and local abundance of a species could be useful markers of a species’ potential to adapt to urban conditions with similar ecological growing conditions (Sjöman et al., 2012). The use of these indicators in urban tree selection is consistent with the environmental filtering theory (Asefa et al., 2017).
4.3 Trait to-trait correlation of the potential USTs
The negative correlation between conservative and acquisitive traits in this study lends support to this two-dimensional spectrum of plant adaptation (Joswig et al., 2022) and the trade-offs in resource mobilisation for safety, as well as growth and reproduction at a community level (Maynard et al., 2022).
The negative correlation between conservative and acquisitive traits suggests a trade-off in resource allocation between safety investment (survival, tolerances) and investment for growth processes, according to the fast-slow growth perspective of the plant economic spectrum theory. One of the main negative correlations we found did between LA and SSD is consistent with research findings reported by Baraloto et al. (2010) (r = −0.339) from research on the species they called Neotropical species of the South American rainforest and Watkins et al. (2021) (r = −0.46) in urban tree species in Northern Europe. We also found a strong negative correlation between LDMC and SLA (r = −0.71). This is consistent with studies on SLA and LDMC reported from China (Jiang et al., 2021) and South America (Gorné et al., 2022). LTPLP is also negatively correlated with conservative traits and positively correlated with acquisitive traits (Table 3), which is in line with the study conducted on a global scale reported by Zhu et al. (2018). The consistency of the trait correlation aligns with the plant economic spectrum, and with the theory of CSR, which highlights the importance of functional traits in understanding species adaptation to urban and non-urban areas, and in analyzing resource investment trade-offs for safety and growth (Maynard et al., 2022). It would be an oversimplification to suggest that the correlation of traits at the plant community level reveals species’ conservatism or acquisitiveness at the species level. However, it does provide valuable insight for further analysis.
4.4 Functional groups of the species and adaptation to urban environment
The PCA analysis showed three species groups (Type I, Type II, and Type III, for each city) resulted from the ordination of tree species with functional traits differently along the two principal components (Figures 1, 2). The PCA result appeared to align with the theory of the plant economic spectrum, and Grime’s universal plant adaptation (CSR) theory (Grime, 19,779). These findings were then summarized as follows: It is our understanding that Apodytes dimidiata, Myrsine melanophloeos, Nuxia congesta, Olea europeana subsp. cuspidata, Olinia rochetiana, and Pitotospurum abysssinicum were classified as Type I for Addis Ababa city (Figure 2). It is notable that significantly, smaller LA, and SLA distinguishes these species, higher LMA, SSD, and a more negative LTPLP than Type II and Type III (see Figures 3–5). In addition, Bersama abyssinica, Ekebergia capensis, Rhus glutinosa, and Melittia ferruginea were included as Type II species (Figure 2). It could be said that these species are characterized by a compound leaf type with a larger LA than Type I and Type III species (Measa laceolata) (Figure 2). It would appear that Type I species are generally more conservative than Type II and Type III species, implying they might have higher probability to adapt the urban street ecology. On Arba Minch, we observed that Type I species include Combretum molle, Celtis africana, Euclea racemosa, Grewia mollis, Terminalia brownii, Pappea capensis, and Mimusops kummel. It is our understanding that, Balanites aegyptiaca, Sclerocarya birrea, Garcinia livingstonei, Teclea nobilis and Pilostigma thonningi were classified as type II species (Figure 1). It is worth noting that only Trichilea emetica and Tamarindus indica were found in the Type III group in Arba Minch. Interestingly, a similar leaf phenology pattern was found between the types I and Type II species in Arba Minch and Addis Ababa. This suggests that Type I species may have simple or small leaf type, high LDMC, and LMA, while Type II and Type III species may have either compound leaves or broad leaf structures, large LA, and SLA in Addis Ababa. In a similar fashion to the species observed in Addis Ababa, Type I species exhibited significantly higher LDMC, SSD, and a more negative LTPLP than both Type II and Type III in Arba Minch. Meanwhile, in Arba Minch, Type II species demonstrated significantly higher LMA than both Type I and Type III, in a manner similar to Type I species (Figure 3).
Previous research has indicated that species with higher conservative traits, such as LDMC, LMA, and SSD, may be more stress-tolerant than species with higher acquisitive traits. Huang et al. (2020) have suggested a potential positive association between LMA and water use efficiency (r2 = 0.22) in California, USA. Horike et al. (2023) also indicated a potential positive association between LMA and drought recovery of urban shrubs in Kyoto City, Japan. Esperon-Rodriguez et al. (2020) demonstrated a notable association between LTPLP and annual precipitation and temperature gradients, with the study conducted in a city with a different location from the inner city to the outer of Sydney, Australia. The correlation of PC1 and PC2 with LTPLP (drought tolerances indicator trait) and with the S, and C percentages of the Grimes adaptation strategy suggests that the PCA-based classification and CSR classification of the current research may be aligned for both cities (Figure 8). This agreement provides further confidence in the species classification and matches adaptation behavior with the novel urban plantation ecologies such as streets, parks, and gardens (Levinsson et al., 2024; Schütt et al., 2022).
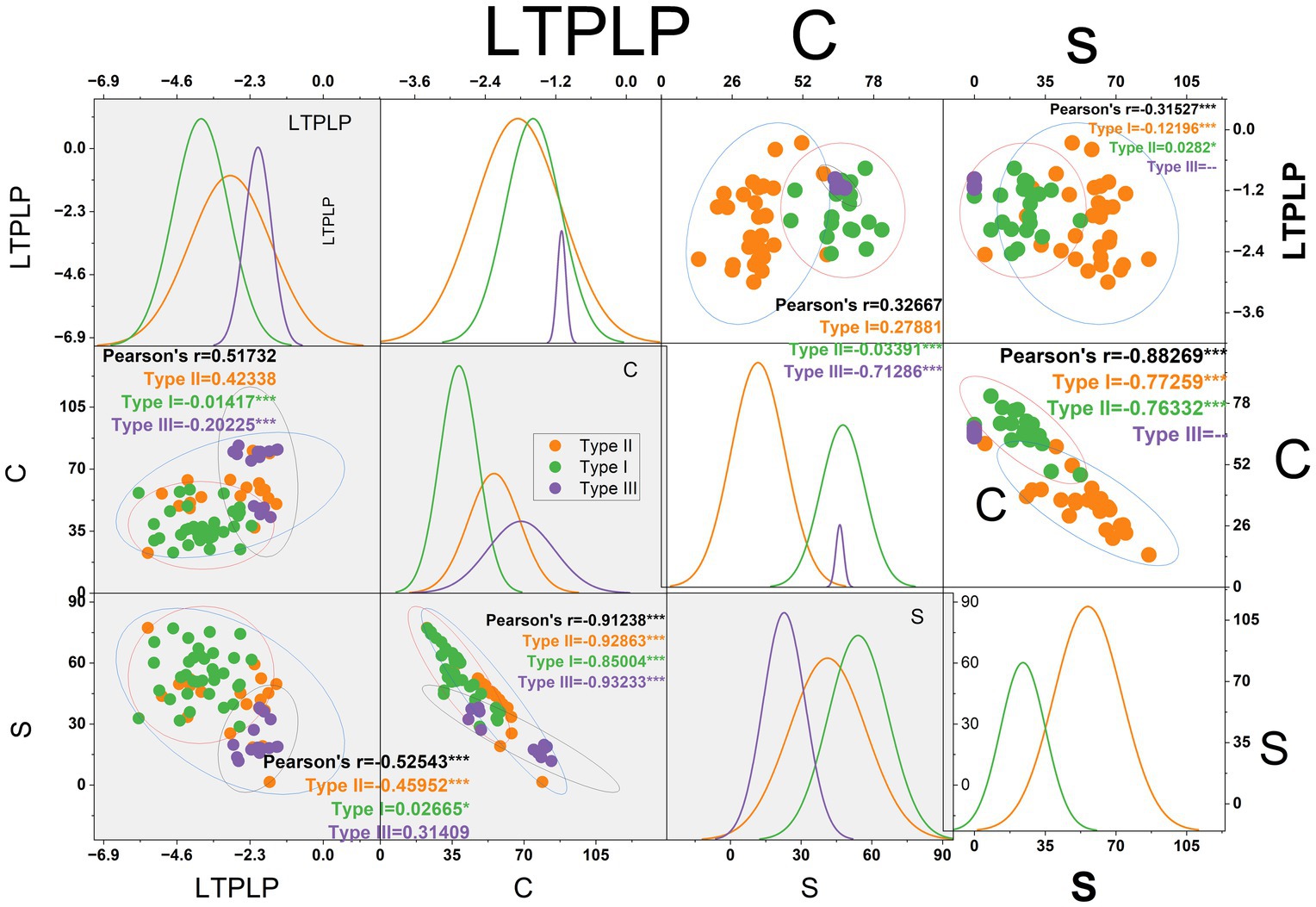
Figure 8. Shows the correlation between the PC1, PC2, LTPLP, and S, C axes of CSR percentages according to Grimes theory, which confirms the consistency of the classification, where LTPLP is the leaf turgor pressure loss point, C is the competitor, and S is the stress tolerances expressed in percentage.
However, sampling of the trees for this study has been conducted at the microhabitat level. Some species showed higher intra-species trait variation, and are not grouped to the above PCA-based and CSR-based classification. It is possible that eco-typic variation (Millien et al., 2006) and phenotypic variation (Behm and Kiers, 2014) are the sources of such large intra-species trait variations. Some species have been observed to exhibit both competitive and stress-tolerance (CS) Grimes adaptation strategies, which appear to be beneficial in areas that are subject to some degree of stress and disturbance, such as parks, pocket plantations, and well-managed median plantation areas.
4.5 Inter-specific trait variation
In addition to community-level analysis, it would be beneficial to gain a deeper understanding of trait variation at the species level. This could help us to better comprehend species-level fitness, reproduction, and future climate adaptation at a local, spatial, and regional scale (Auger and Shipley, 2013; May et al., 2017). However, it is worth noting that there is currently a lack of site-specific and species-specific functional trait and physiology research evidence for Ethiopian tree species. In light of this, our findings are presented in the context of established trait-based plant adaptation theories, with a particular focus on Grimes CSR and plant economics spectrum theory. Our findings suggest that Bersama abyssinica, Apodytes dimidiata, Measa melanophloeos, Olea europeana subsp. cuspidata and Pitotospurum abysssinicum may have higher LMA values than Measa lanceolata, Melittia ferruginea and Ekebergia capansis. It is worth noting that the only significant differences in terms of SSD and LDMC were observed between Olea europeana subsp. cuspidata and Measa lanceolata in Addis Ababa (Figure 4). The SSD implies the mechanical strength, and LDMC implies the leaf stiffness together corroborated to explain stress resistances of the species meaning that Olea europeana subsp. Cuspidata is most likely stress resistances compared to Measa laceolata while these traits are no sufficient to compare the rest of the species and looking other traits might be valuable. In Arba Minch, Balanites aegyptiaca exhibited a higher LMA compared to other species, while the Combretum mole, Euclea racemosa, Petrosporum capense, and Terminalia brownii displayed a higher LDMC (Figure 6). Pappea capensis, Grewia mollis, and Terminalia brownii exhibited significantly higher SSD values. It is thought that plants with high LMA are characterised by a more robust structure, which allows them to resist biotic and abiotic stress while growing slowly, and have longer leaf lifespans and lower leaf nutrient content (De La Riva et al., 2016). On the other hand, it is possible that species such as Olinia rochetiana, O europeana, Grewia mollis, and Terminalia brownii will have higher conservative traits, including LMA, LDMC, and SSD. This could suggest that they may be more tolerant to stresses in urban areas or other environments (May et al., 2017; Pescador et al., 2016).
It would appear that Measa laceolata had significantly higher LWC and SLA than Olea europeana subsp. cuspidata and Pitotospurum abysssinicum. This could be indicative of Measa laceolata being a fast-growing species with a high resource acquisition potential compared to other species. Meanwhile, Melittia ferruginea showed the lowest value of LSI compared to other species proposed for Addis Ababa (Figure 4), and Pappea capensis, Grewia mollis, and Terminalia brownii showed significantly higher SSD values and lowest values of LWC, and LSI in Arba Minch (Figures 3–5). This could be explained by the case that SLA and LWC are associated with resource acquisition plant functions such as photosynthesis, evapotranspiration, and light interception (Firn et al., 2019; Wilson et al., 1999), which are the most important metabolic activities associated with growth rate and reproduction in combination. It may therefore be the case that species such as Measa laceolata and Trichilea emetica, which have very large LA, SLA, and LWC, could be more suitable for production forestry or well-managed urban habitats such as gardens, urban forests, and parks than stressed areas. Bekele (2007, p. 504) has explained that Trichilea emetica is susceptible to pests, which is in line with our findings work of Eggli and Nyffeler (2009) and Lim et al. (2020) highlights the importance of leaf succulence. A study conducted in central southern Ethiopia on the natural forest also showed significant differences in SLA between evergreen tree species (Podocarpus falcatus and Prunus africana) (Seyoum et al., 2012).
Olea europeana subsp. cuspidata > Apodytes dimidiata, > Pittospurum abysscinicum > Ekebergia capensis > Rhus Glutunosa from species suggested for Addis Ababa (Figure 5), and Balanites aegyptiaca, Combretum mole, Tecea nobils, Grewia mollis and Terminalia brownii from species suggested for Arba Minch are the five top species showed more negative LTPLP values (Figure 5). These species have higher probability to adapt the street ecology, which is frequently affected by water deficient, hence LTPLP is thought to be the powerful indicator of drought tolerance as is confirmed by several field based (Sjöman et al., 2018; Zhu et al., 2018), and experimental research findings (Wiström et al., 2023). Brodribb et al. (2003), Santa Rosa National Park, Costa Rica found that LTPLP showed significant intra-specific variation.
4.6 Intra-specific trait variation and eco-typic variation as challenge selection
In addition to inter-specific trait variation, species for both cities showed also intra-specific trait variations where Terminalia brownii from Arba Minch and Milietia ferruginea from Addis Ababa showed the largest intra-specific trait variation. Intra-specific trait variation might be the result from genetic diversity (Kothari et al., 2023), but also can be affected by interaction with neighboring trees, light availability, and soil resources variations at micro-scale level (Auger and Shipley, 2013; Pierick et al., 2021). However, large intra-specific variation concerning drought tolerance, which is shown in the dataset (Figure 9) can indicate an undiscovered potential to obtain specific ecotypes and genotypes with an unusually high tolerance for the species, which can then be selected in order to increase the capacity of tolerance, which should be further explored (Sjöman et al., 2024). The ecotypic variation may impose challenges in species selection, which brought differences in growth performances, stress-tolerances, and adaptation to the specific environmental conditions (Westerband et al., 2021; Zhu and Xu, 2021). To address these challenges, researchers often need to conduct extensive field trials and genetic studies to identify the most suitable ecotypes for specific conditions. This approach helps in selecting plant varieties that are well adapted to the local environment as indicated by Van den Berg et al. (2017). However, it is one of the major challenges yet addressed in this study and needs to be in future research.
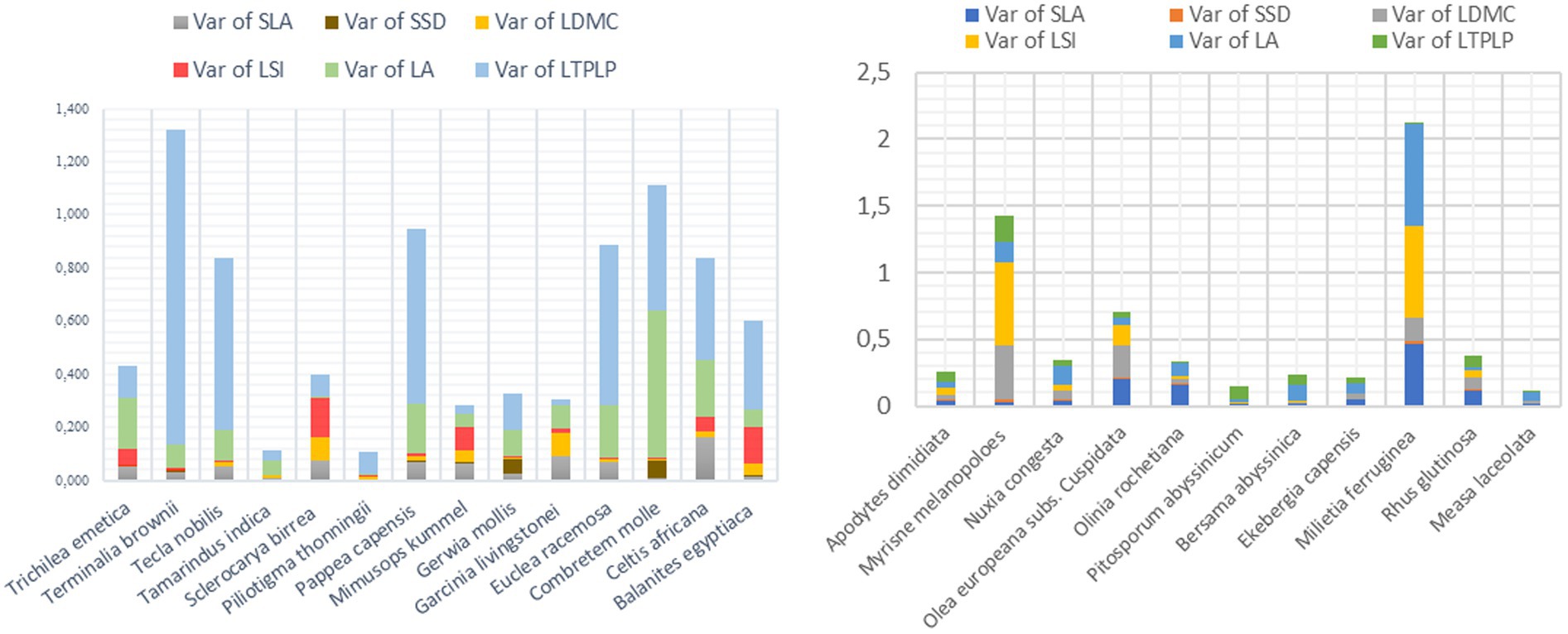
Figure 9. The intra-specific trait-variation within species based on the transformed data into natural logarithmic except SSD, and LTPLP (A) Addis Ababa, and (B) Arba Minch.
5 Conclusion
This study demonstrates the use of both the eco-morphological characteristics of trees and quantitative functional trait analysis for UST selection. A framework analysis was employed to identify 25 native, medium-sized, and non-poisonous tree species (11 for Addis Ababa city and 14 for Arba Minch city) using tree eco-morphological traits as a main screening criterion. In the context of UST tree selection, it is crucial to consider the potential of proposed species to adapt to the challenging street ecology and the impact of future climate risks. The observed trade-off between conservative and acquisitive traits in this study highlights the relevance of functional traits in relation to the plant economic spectrum theory and Grime’s plant adaptation theory. The consistency between the PCA-based and Grime’s CSR classifications demonstrates the specialization of the tree species sampled into different adaptation strategy functional groups, mainly stress-tolerant and competitor groups. Species classified as Type I species according to PCA and stress-tolerant species may have a higher potential to survive the stressful urban street ecologies. Trees in both cities showed significant differences in most of the functional traits, indicating that the sampled species respond differently to the same stresses. Consequently, trees exhibiting higher conservative and drought-tolerant traits, such as higher leaf dry matter content (LDMC), leaf mass per area (LMA), and leaf turgor pressure loss point (LTPLP) are more likely to adapt to the harsh street environment. Conversely, species with higher conservative traits, such as leaf area (LA), specific leaf area (SLA), and leaf water content (LWC), are more likely to provide higher ecosystem services and are suitable in low-stress areas such as urban parks and gardens. The most significant discrepancy to be addressed in future studies is that some species exhibited considerable intraspecific trait variation, which could be attributed to eco-typic or phenotypic variation. This makes it challenging to interpret the results in the context of urban stress adaptation. Furthermore, this research represents the inaugural study on urban street selection and a functional trait-based approach to species selection in the Ethiopian context. It could serve as a valuable reference for future research as well as for the practice. Nevertheless, we are keenly aware of the necessity for further research, encompassing field-level studies and experimental investigations, to substantiate our findings and enhance urban tree resilience. There is also a need to expand the field of study towards other cities with different eco-climatic conditions than in the study areas.
Data availability statement
The raw data supporting the conclusions of this article will be made available by the authors, without undue reservation.
Author contributions
GT: Conceptualization, Data curation, Formal analysis, Methodology, Software, Writing – original draft, Writing – review & editing, Visualization. HS: Conceptualization, Data curation, Investigation, Methodology, Writing – review & editing. ÅS: Conceptualization, Resources, Supervision, Validation, Writing – review & editing, Project administration. KY: Conceptualization, Investigation, Resources, Supervision, Writing – review & editing. BB: Writing – review & editing. ET: Writing – review & editing. AL: Conceptualization, Investigation, Methodology, Resources, Supervision, Validation, Writing – review & editing, Funding acquisition.
Funding
The author(s) declare that financial support was received for the research, authorship, and/or publication of this article. The research was funded by the Swedish Research Council grant no. 2021-05733.
Acknowledgments
We would like to thank all the stakeholders in Ethiopia taking part in the workshops, and field works providing guidance to identify relevant native Ethiopian tree species. I also appreciate the helpful comments and suggestions from the course leaders “How to write and publish.”
Conflict of interest
The authors declare that the research was conducted in the absence of any commercial or financial relationships that could be construed as a potential conflict of interest.
Publisher’s note
All claims expressed in this article are solely those of the authors and do not necessarily represent those of their affiliated organizations, or those of the publisher, the editors and the reviewers. Any product that may be evaluated in this article, or claim that may be made by its manufacturer, is not guaranteed or endorsed by the publisher.
Footnotes
References
Aalipour, H., and Nikbakht, A. (2021). Urban Landscape, Trees Physiological and Environmental Stresses, Challenges and Solutions. August, 36.
Alemu, Z. A., and Dioha, M. O. (2020). Climate change and trend analysis of temperature: the case of Addis Ababa, Ethiopia. Environ. Syst. Res. 9, 1–15. doi: 10.1186/s40068-020-00190-5
Andersson, E., Haase, D., Anderson, P., Cortinovis, C., Goodness, J., Kendal, D., et al. (2021). What are the traits of a social-ecological system: towards a framework in support of urban sustainability. npj Urban Sustain. 1:14. doi: 10.1038/s42949-020-00008-4
Andualem, H. (2018). Investigation into some of the engineering properties of soils found in wereda 10 Of Bole Sub City, Addis Ababa, Ethiopia. MSc Thesis, Addis Ababa, Ethiopia: Addis Ababa Science and Technology University.
Aschale, M., Sileshi, Y., Kelly-Quinn, M., and Hailu, D. (2017). Pollution Assessment of Toxic and Potentially Toxic Elements in Agricultural Soils of the City Addis Ababa, Ethiopia. Bull. Environ. Contam. Toxicol. 98, 234–243. doi: 10.1007/s00128-016-1975-4
Asefa, M., Cao, M., Zhang, G., Ci, X., Li, J., and Yang, J. (2017). Environmental filtering structures tree functional traits combination and lineages across space in tropical tree assemblages. Sci. Rep. 7, 1–11. doi: 10.1038/s41598-017-00166-z
Auger, S., and Shipley, B. (2013). Inter-specific and intra-specific trait variation along short environmental gradients in an old-growth temperate forest. J. Veg. Sci. 24, 419–428. doi: 10.1111/j.1654-1103.2012.01473.x
Ayele, A., and Tarekegn, K. (2020). The impact of urbanization expansion on agricultural land in Ethiopia: A review. Environ. Socio-Econ. Stud. 8, 73–80. doi: 10.2478/environ-2020-0024
Baraloto, C., Paine, C. E. T., Poorter, L., Beauchene, J., Bonal, D., Domenach, A. M., et al. (2010). Decoupled leaf and stem economics in rain forest trees. Ecol. Lett. 13, 1338–1347. doi: 10.1111/j.1461-0248.2010.01517.x
Beecham, S., and Lucke, T. (2015). Street trees in paved urban environments - the benefits and challenges, pp. 24–36.
Behm, J. E., and Kiers, E. T. (2014). A phenotypic plasticity framework for assessing intraspecific variation in arbuscular mycorrhizal fungal traits. J. Ecol. 102, 315–327. doi: 10.1111/1365-2745.12194
Behrens, F. M.-L. (2010). Selecting public street and park trees for urban environments: the role of ecological and biogeographical criteria. Research archive. Lincoln.Ac.Nz 163.
Bekele, A. (2007). Useful trees and shrubs of Ethiopia: identification, propagation and management for 17 agroclimatic zones. Relma 559.
Brodribb, T. J., Holbrook, N. M., Edwards, E. J., and Gutiérrez, M. V. (2003). Relations between stomatal closure, leaf turgor and xylem vulnerability in eight tropical dry forest trees. Plant Cell Environ. 26, 443–450. doi: 10.1046/j.1365-3040.2003.00975.x
Charles, L. S. (2018). Plant functional traits and species selection in tropical forest restoration. Trop. Conserv. Sci. 11, 1–4. doi: 10.1177/1940082918784157
Coleman, A. F., Harper, R. W., Eisenman, T. S., Warner, S. H., and Wilkinson, M. A. (2022). Street tree structure, function, and value: a review of scholarly research (1997–2020). Forests 13:1779. doi: 10.3390/f13111779
Cope, O. L., Lindroth, R. L., Helm, A., Keefover-Ring, K., and Kruger, E. L. (2021). Trait plasticity and trade-offs shape intra-specific variation in competitive response in a foundation tree species. New Phytol. 230, 710–719. doi: 10.1111/nph.17166
Czaja, M., Kołton, A., and Muras, P. (2020). The complex issue of urban trees-stress factor accumulation and ecological service possibilities. Forests 11:932. doi: 10.3390/F11090932
De La Riva, E. G., Olmo, M., Poorter, H., Ubera, J. L., and Villar, R. (2016). Leaf mass per area (LMA) and its relationship with leaf structure and anatomy in 34 Mediterranean woody species along a water availability gradient. PLoS One 11, 1–18. doi: 10.1371/journal.pone.0148788
Dingemanse, J. D., Abiyu, M. A., Tesfaye, K. G., and Roro, F. F. (2022). Using student science to identify research priority areas for air pollution in a university environment: an Ethiopian case study. Clean Air J. 32:2. doi: 10.17159/caj/2022/32/2.13470
Dingemanse, J. D., and Tademe, A. (2023). Technical article developing and testing a PM2 5 low-cost sensor in Ethiopia under ambient and indoor air pollution conditions. Clean Air J. 33, 1–15. doi: 10.17159/caj/2023/33/2.16488
Dowtin, A. L., Cregg, B. C., Nowak, D. J., and Levia, D. F. (2023). Towards optimized runoff reduction by urban tree cover: A review of key physical tree traits, site conditions, and management strategies. Landsc. Urban Plan. 239:104849. doi: 10.1016/j.landurbplan.2023.104849
Dwyer, J. M., Hobbs, R. J., and Mayfield, M. M. (2014). Specific leaf area responses to environmental gradients through space and time. Ecology 95, 399–410. doi: 10.1890/13-0412.1
Edward, F. G., and Sadowski, L. (2007). Choosing Suitable Trees for Urban and Suburban Sites: Site Evaluation and Species Selection Site Evaluation (No. ENH 1057), Chapter 7, The urban forest Hurricane Recovery Program, Florida.
Eggli, U., and Nyffeler, R. (2009). Living under temporarily arid conditions - succulence as an adaptive strategy. Bradleya 27, 13–36. doi: 10.25223/brad.n27.2009.a10
Esperon-Rodriguez, M., Rymer, P. D., Power, S. A., Challis, A., Marchin, R. M., and Tjoelker, M. G. (2020). Functional adaptations and trait plasticity of urban trees along a climatic gradient. Urban For. Urban Green. 54:126771. doi: 10.1016/j.ufug.2020.126771
Farrell, C., Livesley, S. J., Arndt, S. K., Beaumont, L., Burley, H., Ellsworth, D., et al. (2022). Can we integrate ecological approaches to improve plant selection for green infrastructure? Urban For. Urban Green. 76:127732. doi: 10.1016/j.ufug.2022.127732
Farrell, C., Szota, C., and Arndt, S. K. (2017). Does the turgor loss point characterize drought response in dryland plants? Plant Cell Environ. 40, 1500–1511. doi: 10.1111/pce.12948
Faucon, M.-P., Houben, D., and Lambers, H. (2017). Plant Functional Traits: Soil and Ecosystem Services. Trends Plant Sci. 22, 385–394. doi: 10.1016/j.tplants.2017.01.005
Firn, J., McGree, J. M., Harvey, E., Flores-Moreno, H., Schütz, M., Buckley, Y. M., et al. (2019). Leaf nutrients, not specific leaf area, are consistent indicators of elevated nutrient inputs. Nat. Ecol. Evol. 3, 400–406. doi: 10.1038/s41559-018-0790-1
Friis, I., Demissew, S., and van Breugel, P.van, (2010). Atlas of the potential vegetation of Ethiopia. Denmark: The Royal Danish Academy of Sciences and Letters
Gastauer, M., Sarmento, P. S. D. M., Santos, V. C. A., Caldeira, C. F., Ramos, S. J., Teodoro, G. S., et al. (2020). Vegetative functional traits guide plant species selection for initial mineland rehabilitation. Ecol. Eng. 148:105763. doi: 10.1016/j.ecoleng.2020.105763
Goldsmith, L. J. (2021). Using framework analysis in applied qualitative research. Qual. Rep. 26, 2061–2076. doi: 10.46743/2160-3715/2021.5011
Gorné, L. D., Díaz, S., Minden, V., Onoda, Y., Kramer, K., Muir, C., et al. (2022). The acquisitive–conservative axis of leaf trait variation emerges even in homogeneous environments. Ann. Bot. 129, 709–722. doi: 10.1093/aob/mcaa198
Goud, E. M., Agrawal, A. A., and Sparks, J. P. (2023). A direct comparison of ecological theories for predicting the relationship between plant traits and growth. Ecology 104. doi: 10.1002/ecy.3986
Grilo, F., McPhearson, T., Santos-Reis, M., and Branquinho, C. (2022). A trait-based conceptual framework to examine urban biodiversity, socio-ecological filters, and ecosystem services linkages. npj Urban Sustain. 2, 1–8. doi: 10.1038/s42949-022-00077-7
Guadie, A., Yesigat, A., Gatew, S., Worku, A., Liu, W., Ajibade, F. O., et al. (2021). Evaluating the health risks of heavy metals from vegetables grown on soil irrigated with untreated and treated wastewater in Arba Minch, Ethiopia. Sci. Total Environ. 761: 143302. doi: 10.1016/J.Scitotenv.2020.143302
Güneralp, B., Lwasa, S., Masundire, H., Parnell, S., and Seto, K. C. (2018). Urbanization in Africa: Challenges and opportunities for conservation. Environ. Res. Lett. 13:015002. doi: 10.1088/1748-9326/aa94fe
Guo, B., Arndt, S. K., Miller, R. E., Szota, C., and Farrell, C. (2023). How does leaf succulence relate to plant drought resistance in woody shrubs? Tree Physiol. 43, 1501–1513. doi: 10.1093/treephys/tpad066
Hand, K., Doick, K. J., and Moss, J. L. (2019). Ecosystem services delivery by large stature urban trees. Forest Research; Research Report, i–iv, 1–24. Available at: https://www.forestresearch.gov.uk/research/ecosystem-services-delivery-large-stature-urban-trees/ (Accessed December 10, 2023).
Heslop-Harrison, J. S. (2011). Atlas of the potential vegetation of Ethiopia. Ann. Bot. 107, vi–vii. doi: 10.1093/aob/mcq242
Horike, H., Kinoshita, T., Kume, A., and Hanba, Y. T. (2023). Responses of leaf photosynthetic traits, water use efficiency, and water relations in five urban shrub tree species under drought stress and recovery. Trees-Struct. Funct. 37, 53–67. doi: 10.1007/s00468-021-02083-1
Huang, S., Knight, C. A., Hoover, B. K., and Ritter, M. (2020). Leaf functional traits as predictors of drought tolerance in urban trees. Urban For. Urban Green. 48:126577. doi: 10.1016/j.ufug.2019.126577
Jahani, A., and Saffariha, M. (2022a). Environmental decision support system for Plane trees failure prediction: A comparison of multi-layer perceptron and random forest modeling approaches. Agrosyst. Geosci. Environ. 5. doi: 10.1002/agg2.20316
Jahani, A., and Saffariha, M. (2022b). Tree failure prediction model (TFPM): machine learning techniques comparison in failure hazard assessment of Platanus orientalis in urban forestry. Nat. Hazards 110, 881–898. doi: 10.1007/s11069-021-04972-7
Jiang, F., Cadotte, M. W., and Jin, G. (2021). Individual-level leaf trait variation and correlation across biological and spatial scales. Ecol. Evol. 11, 5344–5354. doi: 10.1002/ece3.7425
Joswig, J. S., Wirth, C., Schuman, M. C., Kattge, J., Reu, B., Wright, I. J., et al. (2022). Climatic and soil factors explain the two-dimensional spectrum of global plant trait variation. Nat. Ecol. Evol. 6, 36–50. doi: 10.1038/s41559-021-01616-8
King, D. A., Davies, S. J., Nur Supardi, M. N., and Tan, S. (2005). Tree growth is related to light interception and wood density in two mixed dipterocarp forests of Malaysia. Funct. Ecol. 19, 445–453. doi: 10.1111/j.1365-2435.2005.00982.x
Kosa, A., Juhar, N., and Mohammed, I. (2017). Urbanization in Ethiopia: expropriation process and rehabilitation mechanism of evicted peri-urban farmers (policies and practices). Int. J. Econ. Manage. Sci. 6:451. doi: 10.4172/2162-6359.1000451
Kothari, D., Pargaien, N., Tewari, L. M., Dikshit, H. K., Mishra, G. P., Aski, M. S., et al. (2023). Genetic variation for traits related to phosphorus use efficiency in vigna species. Agronomy 13:305. doi: 10.3390/agronomy13020305
Kumie, A., Worku, A., Tazu, Z., Tefera, W., Asfaw, A., Boja, G., et al. (2021). Fine particulate pollution concentration in Addis Ababa exceeds the WHO guideline value: Results of 3 years of continuous monitoring and health impact assessment. Environ. Epidemiol. 5:p e155. doi: 10.1097/EE9.0000000000000155
Lee, D. K. (2020). Data transformation: a focus on the interpretation. Korean J. Anesthesiol. 73, 503–508. doi: 10.4097/kja.20137
Lefcheck, J. S., Bastazini, V. A. G., and Griffin, J. N. (2015). Choosing and using multiple traits in functional diversity research. Environ. Conserv. 42, 104–107. doi: 10.1017/S0376892914000307
Levinsson, A., Emilsson, T., Sjöman, H., and Wiström, B. (2024). Using stomatal conductance capacity during water stress as a tool for tree species selection for urban stormwater control systems. Urban For. Urban Green. 91:128164. doi: 10.1016/j.ufug.2023.128164
Lim, S. D., Mayer, J. A., Yim, W. C., and Cushman, J. C. (2020). Plant tissue succulence engineering improves water-use efficiency, water-deficit stress attenuation and salinity tolerance in Arabidopsis. Plant J. 103, 1049–1072. doi: 10.1111/tpj.14783
Liu, Y., Jin, S., and Li, G. (2023). Editorial: Physiological ecology of trees under environmental stresses. Front. Plant Sci. 14, 1–3. doi: 10.3389/fpls.2023.1158821
Mantovani, A. (1998). A method to improve leaf succulence quantification. Braz. Arch. Biol. Technol. 42. doi: 10.1590/S1516-89131999000100002
Maracahipes, L., Carlucci, M. B., Lenza, E., Marimon, B. S., Marimon, B. H., Guimarães, F. A. G., et al. (2018). How to live in contrasting habitats? Acquisitive and conservative strategies emerge at inter-and intraspecific levels in savanna and forest woody plants. Perspect. Plant. Ecol. Evol. Syst. 34, 17–25. doi: 10.1016/j.ppees.2018.07.006
Maréchaux, I., Bartlett, M. K., Sack, L., Baraloto, C., Engel, J., Joetzjer, E., et al. (2015). Drought tolerance as predicted by leaf water potential at turgor loss point varies strongly across species within an Amazonian forest. Funct. Ecol. 29, 1268–1277. doi: 10.1111/1365-2435.12452
Martin, T. N., Fipke, G. M., Winck, J. E. M., and Marchese, J. A. (2020). Image J software as an alternative method for estimating leaf area in oats. Acta Agron. 69, 162–169. doi: 10.15446/acag.v69n3.69401
May, R. L., Warner, S., and Wingler, A. (2017). Classification of intra-specific variation in plant functional strategies reveals adaptation to climate. Ann. Bot. 119, 1343–1352. doi: 10.1093/aob/mcx031
Maynard, D. S., Bialic-Murphy, L., Zohner, C. M., Averill, C., van den Hoogen, J., Ma, H., et al. (2022). Global relationships in tree functional traits. Nat. Commun. 13, 1–12. doi: 10.1038/s41467-022-30888-2
Meziane, D., and Shipley, B. (2001). Direct and indirect relationships between specific leaf area, leaf nitrogen and leaf gas exchange. Effects of irradiance and nutrient supply. Ann. Bot. 88, 915–927. doi: 10.1006/anbo.2001.1536
Miedema Brown, L., and Anand, M. (2022). Plant functional traits as measures of ecosystem service provision. Ecosphere 13, 1–13. doi: 10.1002/ecs2.3930
Millien, V., Kathleen Lyons, S., Olson, L., Smith, F. A., Wilson, A. B., and Yom-Tov, Y. (2006). Ecotypic variation in the context of global climate change: Revisiting the rules. Ecol. Lett. 9, 853–869. doi: 10.1111/j.1461-0248.2006.00928.x
National Metrology Agency . (2023). Climate of City: Addis Ababa. Available at: http://ethiomet.gov.et/climates/climate_of_city/3352/Addis%20Ababa (Accessed September 12, 2023).
Nielsen, M. V., Bryndum, N., and Bedsted, B. (2017). Organising stakeholder workshops in research and innovation - Between theory and practice. J. Public Deliberation 13, 1–24. doi: 10.16997/jdd.285
Niklas, K. J., Peijian, S., Johan, G., Julian, S., and Ülo, N. (2023). Leaf functional traits: ecological and evolutionary implications. Front. Plant Sci. 14:1169558. doi: 10.3389/978-2-83252-086-4
Ochola, G. O. (2018). Urbanization and Environmental Stress: A Review of Impacts of Urban Development on the Environment in Kenya. Int. J. Environ. Sci. Nat. Res. 14, 68–72. doi: 10.19080/ijesnr.2018.14.555889
Pan, Y., Cieraad, E., Armstrong, J., Armstrong, W., Clarkson, B. R., Colmer, T. D., et al. (2020). Global patterns of the leaf economics spectrum in wetlands. Nat. Commun. 11, 1–9. doi: 10.1038/s41467-020-18354-3
Pataki, D. E., Alberti, M., Cadenasso, M. L., Felson, A. J., McDonnell, M. J., Pincetl, S., et al. (2021). The Benefits and Limits of Urban Tree Planting for Environmental and Human Health. Front. Ecol. Evol. 9, 1–9. doi: 10.3389/fevo.2021.603757
Pavanetto, N., Carmona, C. P., Laanisto, L., Niinemets, Ü., and Puglielli, G. (2024). Trait dimensions of abiotic stress tolerance in woody plants of the Northern Hemisphere. Glob. Ecol. Biogeogr. 33, 272–285. doi: 10.1111/geb.13788
Pérez-Harguindeguy, N., Díaz, S., Garnier, E., Lavorel, S., Poorter, H., Jaureguiberry, P., et al. (2013). New handbook for standardised measurement of plant functional traits worldwide. Aust. J. Bot. 61, 167–234. doi: 10.1071/BT12225
Pescador, D. S., Sierra-Almeida, Á., Torres, P. J., and Escudero, A. (2016). Summer freezing resistance: A critical filter for plant community assemblies in Mediterranean high mountains. Front. Plant Sci. 7, 1–9. doi: 10.3389/fpls.2016.00194
Pierce, S., Brusa, G., Vagge, I., and Cerabolini, B. E. L. (2013). Allocating CSR plant functional types: The use of leaf economics and size traits to classify woody and herbaceous vascular plants. Funct. Ecol. 27, 1002–1010. doi: 10.1111/1365-2435.12095
Pierce, S., Negreiros, D., Cerabolini, B. E. L., Kattge, J., Díaz, S., Kleyer, M., et al. (2017). A global method for calculating plant CSR ecological strategies applied across biomes world-wide. Funct. Ecol. 31, 444–457. doi: 10.1111/1365-2435.12722
Pierick, K., Leuschner, C., and Homeier, J. (2021). Topography as a factor driving small-scale variation in tree fine root traits and root functional diversity in a species-rich tropical montane forest. New Phytol. 230, 129–138. doi: 10.1111/nph.17136
Polley, H. W., Collins, H. P., and Fay, P. A. (2022). Community leaf dry matter content predicts plant production in simple and diverse grassland. Ecosphere 13, 1–10. doi: 10.1002/ecs2.4076
Population Stat . (2023). Addis Ababa, Ethiopia Population. Available at: https://populationstat.com/ethiopia/addis-ababa (Accessed April 24, 2024)
Reich, P. B. (2014). The world-wide "fast-slow" plant economics spectrum: A traits manifesto. J. Ecol. 102, 275–301. doi: 10.1111/1365-2745.12211
Rodríguez-Santamaría, K., Zafra-Mejía, C. A., and Rondón-Quintana, H. A. (2022). Macro-Morphological Traits of Leaves for Urban Tree Selection for Air Pollution Biomonitoring: A Review. Biosensors (Basel). 12:812. doi: 10.3390/bios12100812
Ruiz-Guerra, B., Velázquez-Rosas, N., Díaz-Castelazo, C., and Guevara, R. (2020). “Functional plant traits and plant-herbivore interactions” in Evolutionary ecology of plant-herbivore interaction. eds. J. Núñez-Farfán and P. Valverde (Cham: Springer). 10, 191–207. doi: 10.1007/978-3-030-46012-9_10
Salmond, J. A., Tadaki, M., Vardoulakis, S., Arbuthnott, K., Coutts, A., Demuzere, M., et al. (2016). “Health and climate related ecosystem services provided by street trees in the urban environment” in Environmental health: a global access science source (vol. 15) (London, United Kingdom: Bio Med Central Ltd.).
Sastry, A., and Barua, D. (2017). Leaf thermotolerance in tropical trees from a seasonally dry climate varies along the slow-fast resource acquisition spectrum. Sci. Rep. 7, 1–11. doi: 10.1038/s41598-017-11343-5
Schütt, A., Becker, J. N., Gröngröft, A., Schaaf-Titel, S., and Eschenbach, A. (2022). Soil water stress at young urban street-tree sites in response to meteorology and site parameters. Urban For. Urban Green. 75. doi: 10.1016/j.ufug.2022.127692
Seyoum, Y., Fetene, M., Strobl, S., and Beck, E. (2012). Foliage dynamics, leaf traits, and growth of coexisting evergreen and deciduous trees in a tropical montane forest in Ethiopia. Trees 26, 1495–1512. doi: 10.1007/s00468-012-0723-6
Simovic, M., Mueller, K. E., Mcmahon, S. M., and Medeiros, J. S. (2024). Functional traits and size interact to influence growth and carbon sequestration among trees in urban greenspaces. Funct. Ecol. 38, 967–983. doi: 10.1111/1365-2435.14505
Sjöman, H. (2015). Doctoral Thesis-Trees for tough urban sites - Learning from nature Trees for Tough Urban Sites Learning from Nature Henrik Sjöman Faculty of Landscape Planning. Alnarp, Sweden: Horticulture and Agricultural Science Swedish University of Agricultural Sciences.
Sjöman, H., Gunnarsson, A., Pauleit, S., and Bothmer, R. (2012). Selection approach of Urban Trees for inner-City environments: Learning from nature. Arboric Urban For 38, 194–204. doi: 10.48044/jauf.2012.028
Sjöman, H., Hirons, A. D., and Bassuk, N. L. (2018). Improving confidence in tree species selection for challenging urban sites: a role for leaf turgor loss. Urban Ecosyst. 21, 1171–1188. doi: 10.1007/s11252-018-0791-5
Sjöman, H., Watkins, H., Kelly, L. J., Hirons, A., Kainulainen, K., Martin, K. W. E., et al. (2024). Resilient trees for urban environments: The importance of intraspecific variation. Plants People Planet. 1–10. doi: 10.1002/ppp3.10518
Smart, S. M., Glanville, H. C., del Blanes, M. C., Mercado, L. M., Emmett, B. A., Jones, D. L., et al. (2017). Leaf dry matter content is better at predicting above-ground net primary production than specific leaf area. Funct. Ecol. 31, 1336–1344. doi: 10.1111/1365-2435.12832
Statista . (2019). Annual Growth-Rate-of-Urban-Population-in-East-Africa-from 2000 to 2018 by country. Available at: https://www.statista.com/statistics/1171587/growth-rate-of-urban-population-in-east-africa-by-country/ (Accessed September, 2023).
Statista (2023). Forecast of the total population of Africa from 2020 to 2050 [WWW Document]. Available at: https://www.statista.com/statistics/1224205/forecast-of-the-total-population-of-africa/ (Accessed September 20, 2023)
Tameirão, L. B. S., Caminha-Paiva, D., Negreiros, D., Veloso, M. D. D. M., Berbara, R. L. L., Dias, L. E., et al. (2021). Role of environmental filtering and functional traits for species coexistence in a harsh tropical montane ecosystem. Biol. J. Linn. Soc. 133, 546–560. doi: 10.1093/biolinnean/blaa181¨
Tefera, W., Kumie, A., Berhane, K., Gilliland, F., Lai, A., Sricharoenvech, P., et al. (2021). Source apportionment of fine organic particulate matter (PM2.5) in central Addis Ababa, Ethiopia. Int. J. Environ. Res. Public Health 18, 1–13. doi: 10.3390/ijerph182111608
Terfa, B. K., Chen, N., Liu, D., Zhang, X., and Niyogi, D. (2019). Urban expansion in Ethiopia from 1987 to 2017: Characteristics, spatial patterns, and driving forces. Sustainability (Switzerland) 11, 1–21. doi: 10.3390/su11102973
Toyebo, S. (2020). Impact factor: 4.295 Investigation of engineering properties of soils found in Arba Minch Zuria (Limat to Shara), Toyebo Saol. Int. J. Adv. Res. 6.
Vaieretti, M. V., Díaz, S., Vile, D., and Garnier, E. (2007). Two measurement methods of leaf dry matter content produce similar results in a broad range of species. Ann. Bot. 99, 955–958. doi: 10.1093/aob/mcm022
Van den Berg, G. J., Verryn, S. D., Chirwa, P. W., and van Deventer, F. (2017). Genetic parameters and genotype by environment interaction of Eucalyptus grandis populations used in intraspecific hybrid production in South Africa. South For 79, 287–295. doi: 10.2989/20702620.2016.1254900
Vendramini, F., Díaz, S., Gurvich, D. E., Wilson, P. J., Thompson, K., and Hodgson, J. G. (2002). Leaf traits as indicators of resource-use strategy in floras with succulent species. New Phytol. 154, 147–157. doi: 10.1046/j.1469-8137.2002.00357.x
Wang, H., Colin Prentice, I., Wright, I. J., Warton, D. I., Qiao, S., Xu, X., et al. (2023). Leaf economics fundamentals explained by optimality principles. Sci. Adv. 9, 1–11. doi: 10.1126/sciadv.add5667
Wang, Z., Huang, H., Wang, H., Peñuelas, J., Sardans, J., Niinemets, Ü., et al. (2022). Leaf water content contributes to global leaf trait relationships. Nat. Commun. 13, 1–9. doi: 10.1038/s41467-022-32784-1
Watkins, H., Hirons, A., Sjöman, H., Cameron, R., and Hitchmough, J. D. (2021). Can Trait-Based Schemes Be Used to Select Species in Urban Forestry? Front. Sustain. Cities 3. doi: 10.3389/frsc.2021.654618
Westerband, A. C., Funk, J. L., and Barton, K. E. (2021). Intraspecific trait variation in plants: A renewed focus on its role in ecological processes. Ann. Bot. 127, 397–410. doi: 10.1093/aob/mcab011
Westoby, M. (1998). A leaf-height-seed (LHS) plant ecology strategy scheme. Plant Soil 199, 213–227. doi: 10.1023/A:1004327224729
Wilson, P. J., Thompson, K., and Hodgson, J. G. (1999). Specific leaf area and leaf dry matter content as alternative predictors of plant strategies. New Phytol. 143, 155–162. doi: 10.1046/j.1469-8137.1999.00427.x
Wiström, B., Emilsson, T., Sjöman, H., and Levinsson, A. (2023). Experimental evaluation of waterlogging and drought tolerance of essential Prunus species in central Europe. For. Ecol. Manag. 537:120904. doi: 10.1016/j.foreco.2023.120904
Worldometer (2024). Worldometer; Population of Eastern Africa (2023 and historical) [WWW Document]. Available at: https://www.worldometers.info/world-population/eastern-africa-population/ (Accessed March 8, 2024).
Wright, I. J., Westoby, M., Baruch, Z., Bongers, F., Cavender-Bares, J., Chapin, T., et al. (2004). The worldwide leaf economics spectrum. Nature 428, 821–827. doi: 10.1038/nature02403
Yang, Y., Gou, R., Li, W., Kassout, J., Wu, J., Wang, L., et al. (2021). Leaf trait covariation and its controls: a quantitative data analysis along a subtropical elevation gradient. J. Geophys. Res. Biogeosci. 126, 1–13. doi: 10.1029/2021JG006378
Zairuddin, N. S., Othman, N., and Abdul Malek, N. (2020). A Review on Tree Sensitive Urban Design (TSUD) approach as potentials streetscape design guidelines. Environ. Behav. Proc. J. 5, 215–221. doi: 10.21834/e-bpj.v5i13.2086
Zhao, L., Qin, A., Zhao, C., Duan, K., Wang, J., and Wen, J. (2020). Responses of composition and functional traits of plant functional groups to different habitats in Jiayuguan Caohu Wetland. Shengtai Xuebao 40, 822–833. doi: 10.5846/stxb201811052396
Zhu, J., Cao, Y., He, W., Xu, Q., Xu, C., and Zhang, X. (2021). Leaf functional traits differentiation in relation to covering materials of urban tree pits. BMC Plant Biol. 21, 1–11. doi: 10.1186/s12870-021-03316-8
Zhu, S. D., Chen, Y. J., Ye, Q., He, P. C., Liu, H., Li, R. H., et al. (2018). Leaf turgor loss point is correlated with drought tolerance and leaf carbon economics traits. Tree Physiol. 38, 658–663. doi: 10.1093/treephys/tpy013
Zhu, J., and Xu, C. (2021). Intraspecific differences in plant functional traits are related to urban atmospheric particulate matter. BMC Plant Biol. 21, 1–7. doi: 10.1186/s12870-021-03207-y
Appendix A
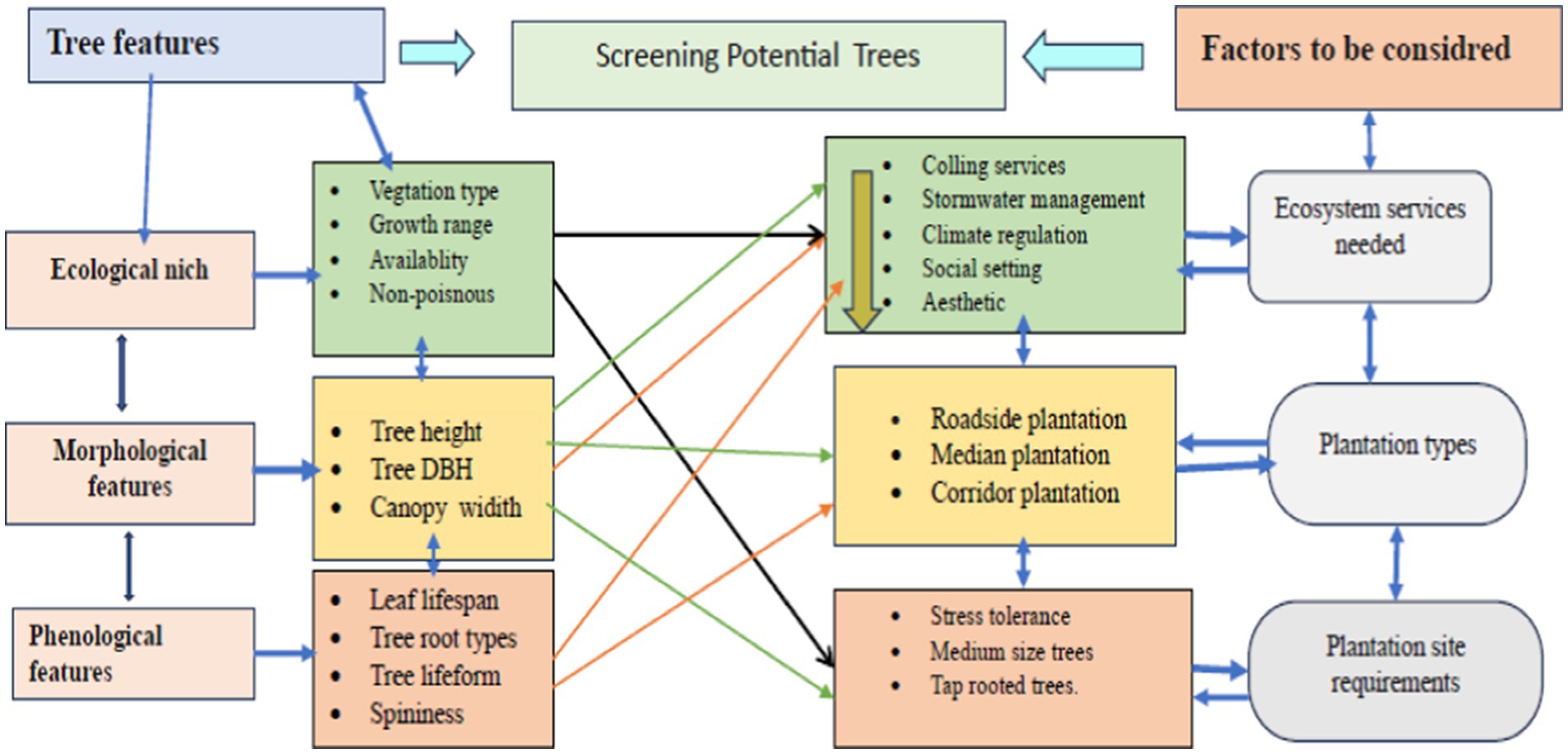
Figure A1. Framework analysis to select the potential urban street tree selection for further functional trait based evaluation.
Keywords: acquisitive traits, conservative traits, plant adaptation strategy, plant functional traits, urban street tree
Citation: Tamene G, Sjöman H, Sang ÅO, Yeshitla K, Belay B, Tesfaye E and Levinsson A (2024) A functional trait-based assessment of urban street tree selection for Ethiopia. Front. Sustain. Cities. 6:1451930. doi: 10.3389/frsc.2024.1451930
Edited by:
Jianquan Cheng, Manchester Metropolitan University, United KingdomReviewed by:
Sakhawat Shah, Huazhong Agricultural University, ChinaAli Jahani, University of Tehran, Iran
Copyright © 2024 Tamene, Sjöman, Sang, Yeshitla, Belay, Tesfaye and Levinsson. This is an open-access article distributed under the terms of the Creative Commons Attribution License (CC BY). The use, distribution or reproduction in other forums is permitted, provided the original author(s) and the copyright owner(s) are credited and that the original publication in this journal is cited, in accordance with accepted academic practice. No use, distribution or reproduction is permitted which does not comply with these terms.
*Correspondence: Gebretsadik Tamene, gebretsadik.melak.tamene@slu.se