- 1Department of Environment Studies, Panjab University, Chandigarh, India
- 2Department of Community Medicine and School of Public Health, PGIMER, Chandigarh, India
The present study highlights the dual benefits of landfill gas utilization: mitigating greenhouse gas emissions and generating renewable energy. Municipal waste, containing a large proportion of organic matter, is dumped into Solid Waste Disposal Sites (SWDS), which, after anaerobic decomposition, releases methane (CH4). This study aims to assess the methane emissions from the SWDS of Chandigarh, India, and evaluate the potential for electricity generation from captured landfill gas. Waste samples were obtained using the quartile method and examined for proximate and ultimate analysis. The inventory and estimation of CH4 generation were done using the International Panel on Climate Change (IPCC) methodology, including the potential of electricity generation from the CH4 generated. It has been observed that Chandigarh generates around 350 tons per day (TPD) of waste, with organic material constituting over 50% on average. The estimated amount of municipal waste with observed composition led to the generation of 0.34 Gg/yr of CH4. Depending on the efficiency of the gas energy system, 0.27 MW to 0.77 MW of electric power can be produced in Chandigarh. The study also provides a broader context for solid waste management in India. Analysis of national data revealed that India generates about 160,038.9 TPD of solid waste, with only about half (79,956.3 TPD) being treated. The country has 3,184 existing dumpsites, with significant variations across states. As per the present analysis, when extrapolated to a national scale, India could potentially generate 5,167 MW to 14,355 MW of power from all its SWDS, resulting in annual financial gains of 4.7 to 13 billion USD. These findings underscore the importance of implementing integrated waste management strategies that prioritize waste reduction, efficient treatment, and energy recovery from landfill gas.
Highlights
• 350 TPD of MSW is dumped into Chandigarh landfill, having 44.6% of organic matter.
• Estimation shows that Chandigarh municipal landfill emits 0.34 Gg/yr of methane.
• 0.27–0.77 MW of electric power can be generated from the Chandigarh landfill.
• The city can attain $ 0.15 million of financial gains from the decomposition of waste.
• India could have $ 13 billion of economic gain by utilizing CH4 for energy generation.
1 Introduction
Several anthropogenic activities have contributed to the increasing atmospheric concentration of greenhouse gases (GHGs), exacerbating global warming concerns (Powell et al., 2016). Gases like methane (CH4) and carbon dioxide (CO2), emitted during the anaerobic degradation of Municipal Solid Waste (MSW) in landfills, have also been reported to play a significant contribution to global warming (Ravindra et al., 2015). Nationwide, India's landfill methane emissions are estimated at 1.25–1.68 Tg/year (Mor et al., 2006). Municipal solid waste landfills are a major source of greenhouse gas emissions, particularly methane. Heavy metal concentrations in groundwater near landfills can also pose health risks from long-term exposure (Kaur et al., 2022). A study of the Gazipur landfill in Delhi estimated methane emissions of 15.3 Gg/year, which accounts for about 1%−3% of India's landfill methane emissions (Mor et al., 2006). Various gases released during the decomposition of the municipal waste, which may deteriorate the environmental conditions, are described in Table 1. As shown in Table 1, landfill fuel gas (LFG) comprises mainly CH4 and CO2 followed by N2, O2, sulfides, and -N, in the same order. Furthermore, Leachate from landfills can significantly impact groundwater quality in surrounding areas and the Leachate Pollution Index (LPI) has been used to quantify pollution levels from landfill leachate in India (Mor et al., 2018). A study of three landfill sites in northern India found elevated levels of ammoniacal nitrogen, chemical oxygen demand, and chloride in groundwater samples within 1 km of the landfills (Negi et al., 2020). LFG contains trace amounts of other gases such as hydrogen (H2), carbon monoxide (CO), and disulfides (Mor et al., 2006; Lee et al., 2017). Municipal solid waste landfills harbor distinct microbiomes, which play a critical role in decomposing waste materials (Stamps et al., 2016). The United States Environmental Protection Agency (USEPA, 2001) has reported that methane emissions from Solid Waste Disposal Sites (SWDS), ranging from 19–40 Tg/year, contribute to 3–19% of the net global annual CH4 emissions.
The decomposition of waste in SWDS follows four major phases: initial, transition, acidification, and methanogenesis, as illustrated in Figure 1. As described in the figure, methane is generally produced during the anaerobic decomposition occurring in the last three phases of municipal waste decomposition. LFG emitted from SWDS can be influenced by the duration of the anaerobic transition phase. Moreover, the composition of the municipal waste, i.e., the amount of organic matter, largely influences the quantity of CH4 released from the SWDS. The CH4 emitted from SWDS can be collected and used as fuel. However, in developing nations, it is generally released into the atmosphere and contributes to regional warming. With a global warming potential 21 times higher than carbon dioxide (CO2), Methane gas is capable of increasing the regional atmospheric temperature significantly and hence leading to variations in local climate (IPCC, 2011; Du et al., 2017; Breitenmoser et al., 2019). Activated carbon derived from MSW shows significant potential for CO2 uptake, offering a sustainable solution for waste management and carbon capture (Dissanayake et al., 2020). Effective solid waste management is crucial for maintaining environmental quality and public health in urban areas (Arti et al., 2013; Al-Sabbagh et al., 2023).
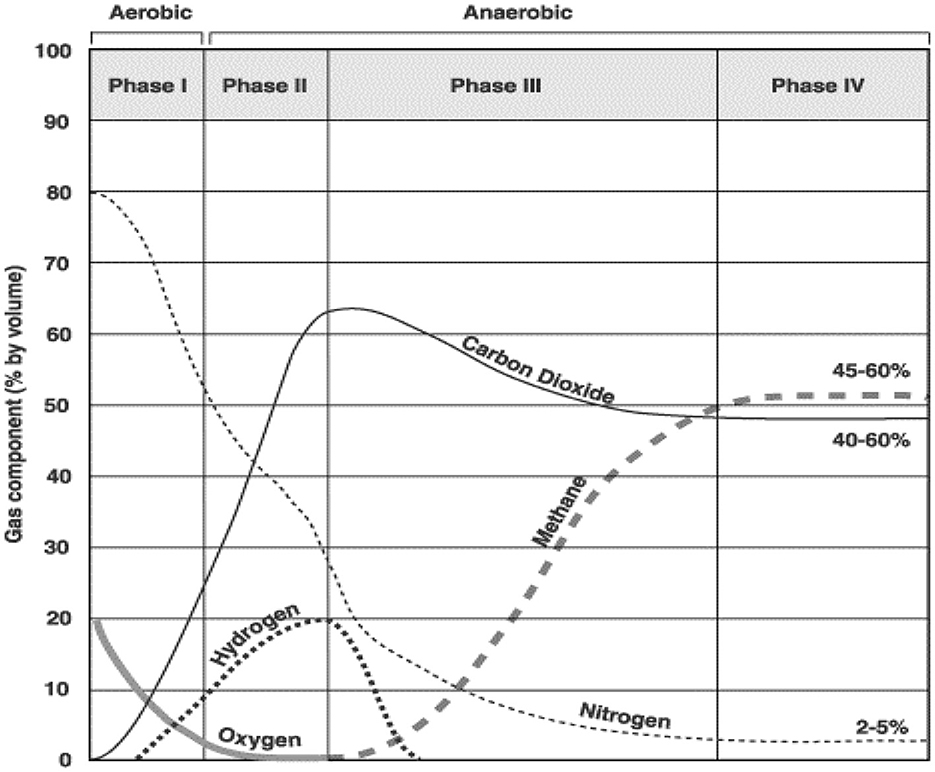
Figure 1. Description of methane generation process in SWDS (Source: Tchobanoglous et al., 1993).
In developing countries, LFG release is a persistent environmental issue (Krause et al., 2016; Powell et al., 2016; Nabavi-Pelesaraei et al., 2017). Limited resources necessitate environmentally sound waste management techniques. Sustainable waste management, incorporating the 3Rs (reduce, recycle, reuse), is crucial for improving urban living standards (Wang and Geng, 2015; Ngwabie et al., 2019). LFG can be utilized for electricity production, mitigating global warming impacts. However, delays in implementing sustainable MSW practices in countries like India result in large-scale waste accumulation and economic losses from electricity imports (Mohan and Bindhu, 2008).
Leachate from landfills can significantly impact groundwater quality in surrounding areas. A study of three landfill sites in northern India found elevated levels of ammoniacal nitrogen (up to 9.8 mg/L), chemical oxygen demand (up to 128 mg/L), and chloride (up to 115 mg/L) in groundwater samples within 1 km of the landfills (Negi et al., 2020). Recently, authorities in India have established stringent policies to implement the waste-to-energy concept. For instance, authorities have increased their investment in energy sectors such as hydro and solar projects to 350 million USD (Ebinger and Potvin, 2016; MOSPI, 2017). Under the new National Energy Policy of 2017, authorities have provided access to free energy to the marginalized community, and for this purpose, authorities are seeking other resources for electricity generation (MoP, 2017). Furthermore, in line with the 2030 Agenda for Sustainable Development (United Nations, 2015), the Indian government has invested in developing alternative energy sources, such as solar, wind, and LFG. These efforts aim to ensure the availability of renewable, sustainable, and environmentally friendly energy for all (MoEFCC, 2015). SWDS, located in India, contributes to 6% of the global methane emissions, which is twice the average contribution from a single country.
Incineration of municipal waste in New Delhi, India, generates approximately 16 MT of CO2 per annum, and it is expected to touch 20 MT per annum by 2020 (Annepu, 2012; Ghosh et al., 2019). Compost derived from municipal solid wastes can be a valuable source of biochar for CO2 capture, contributing to both waste management and climate change mitigation (Basso et al., 2020). However, the lack of data on the generation and collection of MSW in the SWDS of developing countries makes it difficult to ascertain the number of methane emissions (Mor et al., 2006). Therefore, to predict the emissions of GHGs from the SWDS of developing nations, it is crucial to ascertain the characterization and quantity of municipal waste dumped into the SWDS. There are several municipal landfill sites in India, and their composition depends on the consumption behavior of the regional community (Shashidhar and Kumar, 2011; Gollapalli and Kota, 2018).
Several approaches have been developed to address the issue of LFG emissions, such as “LFG capture and utilization,” which involves collecting LFG and using it as a fuel source for electricity generation. The benefits of this method are that it Reduces GHG emissions and generates renewable energy. However, it requires significant infrastructure investment and may not capture all emissions (Themelis and Ulloa, 2007; Rasi et al., 2011). Another method is “Waste reduction and recycling,” minimizing organic waste in landfills through composting and recycling programs (Bogner et al., 2008; Manfredi et al., 2009). It Reduces overall emissions and conserves resources. However, it requires behavioral changes and may be challenging to implement widely. The “Landfill cover systems” methods include implementing engineered covers to oxidize methane (Scheutz et al., 2009; Abichou et al., 2015). “Biofiltration” Using microbial processes to convert methane to CO2 is highly effective. However, it requires careful design and management and may not be suitable for all climates (Gebert and Groengroeft, 2006; Huber-Humer et al., 2008).
While the abive approaches have shown promise, challenges remain in developing countries due to limited resources, lack of data, and implementation difficulties. This study proposes a comprehensive approach that combines waste characterization, methane emission estimation, and potential energy recovery assessment, specifically tailored for developing nations like India. The method proposed in this study differs from previous approaches by focusing on regional waste composition analysis to improve emission estimates, utilizing a first-order decay (FOD) model adapted to local conditions, and assessing the economic potential of methane-based electricity generation in the context of developing nations.
Given the importance of estimating methane generation from national SWDS, evaluating the composition of regional SWDS is crucial. Hence, this study aims to assess the methane (CH4) emissions from the SWDS of Chandigarh, India. To achieve this objective, MSW was characterized at the dumpsite, and a first-order decay model (FOD) was employed to estimate the CH4 production potential of Chandigarh's SWDS. Furthermore, this study will highlight the significance of the waste-to-energy concept in developing nations by illustrating the potential financial gains realized from methane gas-based electricity generation. Ultimately, the findings will contribute to sustainable waste management practices and support India's efforts toward achieving its renewable energy targets. By 2030 and 2050, global GHG emissions from uncontrolled waste disposal systems are predicted to rise to 64% and 76% respectively (Mor and Ravindra, 2023).
By combining site-specific waste characterization, methane emission estimation using the IPCC first-order decay model, and assessment of electricity generation potential, this study provides a holistic framework that is both scientifically robust and practically applicable. The importance of this design lies in its ability to; (a) Accurately estimate methane emissions based on local waste composition and conditions, improving upon generic models. (b) Quantify the environmental impact of SWDS in terms of greenhouse gas emissions. (c) Evaluate the economic potential of landfill gas utilization for electricity generation. (d) Provide actionable insights for policymakers and waste management practitioners to implement sustainable waste management practices and mitigate climate change impacts. This procedure will not only mitigate the global warming impacts of the methane gas released from landfills but also provide an opportunity for implying the waste-to-energy concept.
Therefore, in this study, efforts were also made to determine the amount of electric energy that can be produced by combusting the LFG in different types of energy systems available. The findings will contribute to sustainable waste management practices and support India's efforts toward achieving its renewable energy targets, aligning with the 2030 Agenda for Sustainable Development, India's National Energy Policy of 2017 (MoP, 2017). This approach bridges the gap between environmental assessment and economic feasibility, offering a valuable tool for decision-making in waste management and renewable energy planning, particularly in the context of developing nations like India.
2 Materials and methods
2.1 City profile
Chandigarh, a union territory and planned city in northern India, encompasses an area of 114 km2. According to the 2011 census, the city's population stood at 1.05 million, exhibiting a 17.10% growth over the preceding decade. The solid waste disposal site (SWDS) serving Chandigarh is situated in Dadumajra, a locality on the city's periphery. This facility, which has been operational for 29 years, is now considered outdated and inadequate for the city's current needs.
A critical deficiency of the Dadumajra SWDS is its lack of landfill gas (LFG) collection infrastructure. Consequently, methane, a potent greenhouse gas with a global warming potential 28–36 times that of carbon dioxide over a 100-year period, is continuously emitted into the atmosphere. This not only contributes to climate change but also poses significant environmental and health risks to the surrounding areas.
The site's suboptimal management has led to frequent fire incidents, likely due to the buildup of methane and other combustible gases within the waste mass. These fires exacerbate air pollution and pose potential health hazards to nearby communities. Furthermore, the pervasive malodorous emissions from the landfill have severely compromised the quality of life for residents in the vicinity, raising concerns about long-term health implications and environmental justice issues.
2.2 Characterization of municipal solid waste
MSW samples were collected from Dadumajra's, SWDS, Chandigarh. The sampling was done systematically using the quartile method to ensure representativeness. This method involved dividing the collected waste into parts and selecting samples from each part. After collection, the samples were segregated to determine their physical composition. The collected samples were transferred to the laboratory for preparation and subsequent analysis; the preparation involved drying the samples at 75°C to remove moisture content. Once dried, the samples were cut into smaller pieces and sieved through a 2 mm sieve to ensure uniform particle size for chemical analysis. Proximate analysis was performed to determine the volatile matter and ash content using furnace ignition, adhering to ASTM 02947 standards. Ultimate analysis, which included determining the elemental composition such as Carbon (C), Hydrogen (H), and Nitrogen (N), was conducted using an Organic Elemental Analyzer Flash 2000 following ASTM methods. The data obtained from proximate and ultimate analysis were further statistically analyzed using the Statistical Package for Social Sciences (SPSS, IBM, version 20). The analysis facilitated the understanding of the waste composition and its potential for methane generation, allowing for a comprehensive assessment of the environmental impact of the MSW.
2.3 Methodology for inventory estimation
Inventory assessment of the MSW samples collected from SWDS of Chandigarh was done using the revised IPCC (International Panel on Climate Change) guidelines for national GHG inventories. The IPCC provides two primary methods for estimating methane emissions from MSW: the First Order Decay (FOD) method and the Default (Tier 1) method. The FOD method estimates methane emissions based on the concept that organic waste decays over time in a first-order decay process. This method accounts for the varying decay rates of different waste components and the long-term generation of methane. This method was chosen due to its accuracy in accounting for the varying decay rates of different waste fractions over time, providing a more detailed estimation of methane emissions. The key parameters for this assessment included the waste composition, the rate of decay of different waste fractions, and the methane generation potential. The procedure followed in the FOD method-based estimation of methane emissions from SWDS of Chandigarh is described in Equation 1
Where MSWT depicts the total MSW generated (Gg/yr); MSWF describes the fraction of MSW disposed of at the disposal sites; MCF stands for methane correction factor (fraction); DOC is degradable organic carbon (fraction); DOCF- defines the fraction of dissimilated DOC; F stands for amount of methane in the LFG generated, and R is the methane gas recovered from the SWDS. DOC is equal to the sum of 0:4A, 0:17B, 0:15C, and 0:3D. Here, A, B, C, and D present the amount of different types of waste in the MSW samples.
2.4 Electricity generation
The amount of electricity generated by combusting the LFG in different types of energy systems depends on the efficiency and load of operation. Bove and Lunghi (2006) mentioned five types of energy systems with different electrical efficiency that can be used to recover energy from municipal landfill gas. These gas energy systems, along with their efficiency (η), are enlisted in Table 2. The methane gas produced from landfills can be collected and pumped into the above-mentioned gas energy systems that can further be used to produce electricity as per Equation 2.
Where E stands for the amount of electricity generated (GWh y−1), LHV depicts the lower heating value of methane gas (kWh m−3), which was derived using a model described in https://www.unitrove.com/engineering/tools/gas/natural-gas-calorific-value. The value of LHV for methane was observed to be 9.9 kWh m−3; GCH4 depicts the quantity of methane gas generated in the SWDS (M m3 y−1). This calculation will further be used to calculate the potential of electricity generation in India based on 780 Gg y−1 CH4 emissions from SWDS of India (Singh et al., 2018).
The flow chart shown in Figure 2 outlines the process for analyzing MSW samples from a facility in Chandigarh, India. It describes steps for sample preparation, physical and chemical analysis, and assessment of methane emissions and electricity generation potential. The process involves various laboratory techniques and analytical methods to characterize the waste composition and evaluate its energy recovery possibilities.
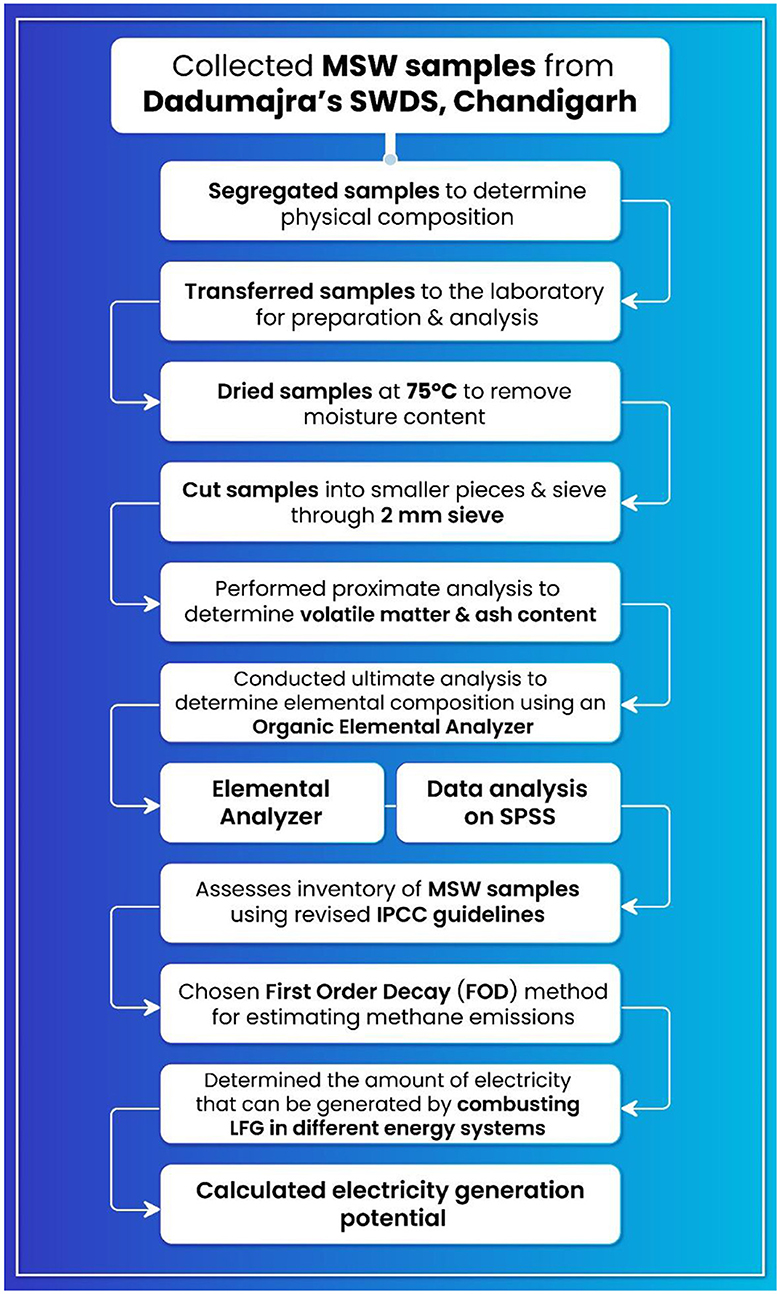
Figure 2. The flowchart outlines a process and methodology for analyzing MSW samples from a facility in Chandigarh, India.
3 Results and discussion
3.1 The physical composition of MSW
It has been observed that the total waste generated in Chandigarh is 380 TPD, and approximately 350 TPD waste reaches the dump site. The observations are derived from comprehensive physical and elemental analysis of the MSW samples, as shown in Tables 3, 4. The waste management situation presents a significant challenge, with detailed observations revealing crucial insights into the composition and characteristics of MSW. Table 3 reveals that organic material constitutes a significant fraction of the MSW, accounting for over 50% on average. Following organic waste, inerts make up approximately 21% of the waste composition. Organic fraction includes all those materials that are putrescible and, hence, aid more generation of GHGs during decomposition. Table 4 provides further insights, indicating that the MSW samples analyzed exhibit a high moisture content, averaging around 50%. This high moisture content primarily originates from the disposal of organic waste, such as vegetables, in the SWDS of Chandigarh. High moisture levels can accelerate the decomposition process in landfills, potentially leading to increased methane generation rates. This observation highlights the importance of considering local waste characteristics, including moisture content, when estimating and modeling landfill gas emissions. These results from Tables 3, 4 serve as initial data for estimating various input parameters crucial for waste management strategies. Observations made from Tables 3, 4 were further used to estimate the values of the various input parameters, as described in Equation 1. The detailed analysis of MSW composition and moisture content provided valuable data for estimating the various input parameters required in the IPCC methodology used for methane emission calculations. The high organic fraction and moisture content observed in Chandigarh's MSW highlight the need for improved waste segregation practices at households and commercial establishments, as well as efficient collection and transportation systems. Separating organic waste from other types of waste allows for specialized treatments like composting or anaerobic digestion, which helps minimize methane emissions from landfills.
3.2 Methane emission estimation
Equation 1 integrates the key parameters such as organic waste content, moisture levels, and temperature variations observed in waste samples (shown in Tables 3, 4). These factors are essential in predicting methane generation rates. The final calculations for Equation 1 are described as follows:
Methane emission (GCH4) (Gg/yr) = (0.38 × 0.35) × 0.4 × 12.83 × 0.77 × 0.5 × (16/12 – 0) × (1 – 0); 0.262,1.3
where 1 Gg/yr = 1,000 tons/yr
MSWT = 0.38 Gg/yr
MSWF= 0.35 Gg/yr
MCF = The IPCC document indicated the value of 0.4 for open dumps <5 m depth.
DOC = ±12.83
DOCF depicts the proportion of DOC that is converted to LFG.
As LFG is produced mainly during the anaerobic phase of methanogenesis, the value of DOCF was estimated using a theoretical model, which is 0.014T+0.28, where T = temperature in °C. Hence, the model is dependent on the temperature of the anaerobic zone (Tabasaran, IPCC document 1996), which was assumed to be 35°C. In this way, the value of DOCF was derived as 0.77.
F is the amount of methane in LFG, and it is equal to 0.5 by default.
R is the amount of methane gas recovered from the SWDS (in Gg/yr). Since landfill gas has not been recovered at the municipal landfill site of Chandigarh, the value of R was considered nil.
The calculation results are 0.262 Gg/yr and 1.3 Gg/yr, respectively, indicating the range of potential methane emissions from the site. These emissions are significant as methane is a potent greenhouse gas, and understanding these emissions helps in planning mitigation strategies for climate change impacts.
OX in Equation 1 stands for oxidation factor, and it defines the amount of methane gas that comes in contact with atmospheric oxygen to oxidize itself. In the present study, OX was assumed to be zero. From these calculations, the value for CH4 emissions from Chandigarh's landfill site comes out to be 0.34 Gg/yr, indicating the regional and overall impacts of the massive methane generation into the atmosphere. Similar observations were reported in landfill sites in other parts of India. Mor et al. (2006) reported that 15.3 Gg/yr of methane is emitted from the Gazipur landfill in Delhi, India. Solid waste disposal sites, which are the main spot for waste collection in Delhi, India, contribute to about 80% of the GHG emissions in the region. Moreover, the composition of the waste dumped in the landfills depends on the local culture and habits and regional diversity in the population. Similarly, in India, the composition of MSW is significantly distinct from that of other developed and developing countries. MSW of developing countries such as Europe and the United States of America (USA), a more significant proportion of slowly degradable waste, was reported. On the other hand, in India, the concentration of wet waste is comparatively higher; hence, landfill gases are produced at the earliest stage.
Therefore, to estimate the contribution of SWDS in the emission of methane gas into the atmosphere, it is crucial to have an extensive understanding of the detailed emission factors system. A clear picture of regional and global impacts of methane emissions from SWDS may prioritize it as a hot issue among the authoritarians and direct them to initiate necessary controlling measures. LFG released from SWDS can be decreased by enhancing the composting of MSW, capturing the LFG, and further utilizing it as fuel. The latter step will not only provide environmental benefits but also assist India in earning carbon credits. The efficient incorporation of such techniques is only possible if its establishment is based on regional needs and demands. Therefore, in this study, the recovery of methane gas from landfills and further utilization in electricity generation has also been shown as an opportunity to mitigate greenhouse impacts and electricity production.
3.3 Potential of electricity generation from landfill-generated methane gas
The decomposition of municipal waste dumped into landfill sites continuously produces methane gas that can cause global warming if not stored and used for electricity generation. However, this methane can be captured and used for electricity generation, turning a potential environmental hazard into a valuable energy resource. This section presents the potential of electricity generation from methane gas produced under Chandigarh's SWDS. The possibility of generating electricity by utilizing different types of gas energy systems is described in Table 5.
As depicted in Table 5, the amount of power produced by Chandigarh's SWDS ranges from 0.27 MW to 0.77 MW, depending on the type of gas energy system used. The current electricity price in India (65 rupees per watt) translates to potential annual financial gains of 0.23 to 0.68 million USD for Chandigarh. The implications are even more significant when scaled up to a national level. India could potentially generate 5,167 MW to 14,355 MW of power from all its SWDS, resulting in annual financial gains of 4.7 to 13 billion USD.
This analysis underscores the dual benefits of landfill gas utilization: mitigating greenhouse gas emissions and generating renewable energy. It also highlights the economic incentives for implementing such systems. The wide range in potential power generation and financial gains reflects the variability in energy conversion efficiencies of different gas energy systems and the scale of implementation. Moreover, harnessing landfill gas for energy production aligns with sustainable waste management practices and circular economy principles. It not only addresses the environmental concerns associated with methane emissions but also provides a local source of renewable energy, potentially reducing reliance on fossil fuels.
This study's approach, utilizing waste characterization and the IPCC first-order decay model for methane emission estimation, offers a balance between accuracy and practicality. Compared to other methods, such as the US EPA's LandGEM, our approach emphasizes site-specific data, potentially improving estimation accuracy. While direct measurement techniques like a closed chamber or atmospheric tracer methods provide actual emission data, they are more resource-intensive and limited in spatial or temporal coverage. Our method allows for long-term projections and is less resource-intensive, though it may not capture short-term fluctuations as accurately. Unlike mass balance approaches, which offer comprehensive carbon flow analysis, our study focuses specifically on methane emissions and energy recovery potential, providing targeted insights for waste management practitioners. Table 6 summarizes these comparisons.
3.4 Overview of total solid waste generation in India
Table 7 highlights the amount of solid waste generation, treatment, and landfilling across different states and union territories in India for the year 2020–21 (CPCB, 2021). The total solid waste generation of 160,038.9 tons per day (TPD) is a substantial figure, and it is essential to manage this waste effectively to mitigate its adverse impacts. From Table 7, it has been noticed that the top five states generating the highest amounts of solid waste are Maharashtra (22,632.71 TPD), Tamil Nadu (13,422 TPD), Gujarat (10,373.79 TPD), Uttar Pradesh (14,710 TPD), and Karnataka (11,085 TPD). The union territories of Delhi (10,990 TPD) and Chandigarh (513 TPD) also generate significant amounts of solid waste.
While the data from Table 7 indicates that 79,956.3 TPD of waste is treated. The states with the highest reported quantities of treated waste are Maharashtra (15,056.1 TPD), Gujarat (6,946 TPD), Karnataka (6,817 TPD), and Madhya Pradesh (6,472 TPD). For some states, such as Arunachal Pradesh and Bihar, data on the quantity of treated waste is not available. Proper treatment methods, such as composting, recycling, and waste-to-energy processes, can significantly reduce the amount of waste destined for landfills and mitigate greenhouse gas emissions (Guerrero et al., 2013). Landfilling remains a prevalent practice in many states, with Delhi, Rajasthan, Punjab, Haryana, and Tamil Nadu reporting significant quantities of landfilled waste. The total amount of solid waste landfilled in India, as per Table 7, is 29,427.2 TPD. The states and union territories with the highest reported quantities of landfilled waste are Delhi (5,533 TPD), Rajasthan (5,082.16 TPD), Punjab (2,384.82 TPD), Haryana (2,167.51 TPD), and Tamil Nadu (2,301.04 TPD). Some of the states, such as Chhattisgarh, Assam, Mizoram, and Uttar Pradesh, have reported minimal landfilling of solid waste. Landfills are known to be major sources of methane emissions, which contribute to global warming (Themelis and Ulloa, 2007; Bogner et al., 2008).
In the context of dumping solid waste in India, Figure 3 illustrates the state-wise distribution of existing dumpsites across India. A total of 3,184 dumpsites were identified across different states and union territories. The number of dumpsites varied significantly among states, ranging from Nil to 609. Uttar Pradesh reported the highest number of dumpsites (609), followed by Madhya Pradesh (326), Maharashtra (237), Tamil Nadu (210), and Rajasthan (197). Conversely, Lakshadweep reported no dumpsites, while Andaman and Nicobar Islands, Chandigarh, and Sikkim reported only 1, 1, and 2 dumpsites, respectively.
The state-wise distribution of dumpsites in India reveals significant disparities in solid waste management infrastructure across the country. The high number of dumpsites, totaling 3,184, suggests a widespread reliance on this waste disposal method, which may have serious environmental and public health implications (Kumar et al., 2004). The high waste generation rates in different states and substantial variation in dumpsite numbers among states likely reflect differences in factors such as population size, urbanization rates, industrial activity, and local waste management policies (Sharholy et al., 2008; Srivastava et al., 2020). States with larger populations and higher levels of urbanization, such as Uttar Pradesh and Maharashtra, unsurprisingly report higher numbers of dumpsites. This correlation aligns with previous studies that have linked rapid urbanization and population growth to increased solid waste generation and management challenges in developing countries (Kaza et al., 2018).
The absence of dumpsites in Lakshadweep and the low numbers in some union territories and smaller states could indicate more efficient waste management practices, limited waste generation due to smaller populations, or potentially, a lack of formal waste disposal infrastructure. Figure 4 presents the year-wise trend of solid waste generation in India from 2010 to 2023. The graph shows a clear and consistent upward trend in waste generation over this 14-year period. In 2010, the solid waste generated was approximately 39 million metric tons. By 2023, this figure had nearly doubled to about 78 million metric tons. The consistent upward trend in solid waste generation is a cause for significant concern. The increasing trend can be attributed to several factors, such as population growth, rapid urbanization, economic growth, and changing consumption patterns.
4 Conclusions
This comprehensive study highlights the complex landscape of India's solid waste management sector, focusing on methane emissions from municipal solid waste disposal sites (SWDS) and the potential for energy recovery. The concept of this study offers a comprehensive and practical approach to address this critical issue in developing countries. By analyzing Chandigarh's waste generation, composition, and methane emissions, the research reveals significant environmental impacts and opportunities for improvement. The study reveals that Chandigarh generates approximately 350 tons per day (TPD) of municipal solid waste, with over 50% being organic material. Using IPCC-based methodology, the study estimates methane emissions of 0.34 Gg/year from Chandigarh's landfill alone, highlighting the substantial environmental and health risks posed by SWDS. The high organic content of the waste, determined through physical and elemental analysis, indicates rapid landfill gas (LFG) generation potential. Importantly, the study identifies a promising avenue for mitigation through landfill gas utilization, with Chandigarh's SWDS capable of generating 0.27–0.77 MW of electricity. When extrapolated nationally, India's SWDS could potentially produce 5,167–14,355 MW of power, offering a dual opportunity to reduce greenhouse gas emissions and generate renewable energy. These findings underscore the importance of implementing integrated waste management strategies that prioritize waste reduction, efficient treatment, and energy recovery from landfill gas. The research not only contributes to the scientific understanding of landfill gas dynamics but also provides practical insights for policymakers and waste management professionals. By quantifying the methane emission scenario and energy recovery potential, the study presents a compelling case for adopting sustainable waste management practices and landfill gas utilization technologies.
Data availability statement
The raw data supporting the conclusions of this article will be made available by the authors, without undue reservation.
Author contributions
SM: Data curation, Formal analysis, Supervision, Writing – original draft, Writing – review & editing. KK: Data curation, Formal analysis, Writing – review & editing. KR: Writing – original draft, Writing – review & editing, Formal analysis.
Funding
The author(s) declare that no financial support was received for the research, authorship, and/or publication of this article.
Acknowledgments
We acknowledge the Panjab University, Chandigarh.
Conflict of interest
The authors declare that the research was conducted in the absence of any commercial or financial relationships that could be construed as a potential conflict of interest.
Publisher's note
All claims expressed in this article are solely those of the authors and do not necessarily represent those of their affiliated organizations, or those of the publisher, the editors and the reviewers. Any product that may be evaluated in this article, or claim that may be made by its manufacturer, is not guaranteed or endorsed by the publisher.
References
Abichou, T., Kormi, T., Yuan, L., Johnson, T., and Francisco, E. (2015). Modeling the effects of vegetation on methane oxidation and emissions through soil landfill final covers across different climates. Waste Manag. 36, 230–240. doi: 10.1016/j.wasman.2014.11.002
Al-Sabbagh, M. K., Alkhayat, A., Alshbib, H. O., Alshammari, M., AlAli, A., Alawadhi, H., et al. (2023). An overview of solid waste management and privatization in Kingdom of Bahrain. Front. Environ. Sci. 11:1266710. doi: 10.3389/fenvs.2023.1302711
Annepu, R. K. (2012). Sustainable solid waste management in India. [master's thesis]. [New York (NY)]: Columbia University.
Arti, S., Ayushman, M., and Bhawana, M. (2013). Urban sprawl development and need assessment of landfills for waste disposal: a case study of Bilaspur Municipal Corporation of Chhattisgarh, India. J. Environ. Res. Dev. 7, 1718–1725.
Basso, D., Patuzzi, F., Weiss-Hortala, E., Castaldi, M. J., and Baratieri, M. (2020). Compost from municipal solid wastes as a source of biochar for CO2 capture. Chem. Eng. Technol. 43, 1336–1349. doi: 10.1002/ceat.201900108
Bogner, J., Pipatti, R., Hashimoto, S., Diaz, C., Mareckova, K., Diaz, L., et al. (2008). Mitigation of global greenhouse gas emissions from waste: conclusions and strategies from the Intergovernmental Panel on Climate Change (1996) Fourth Assessment Report. Working Group III (Mitigation). Waste Manag. Res. 26, 11–32. doi: 10.1177/0734242X07088433
Bogner, J. E., Spokas, K. A., and Chanton, J. P. (2011). Seasonal greenhouse gas emissions (methane, carbon dioxide, nitrous oxide) from engineered landfills: Daily, intermediate, and final California cover soils. J. Environ. Qual. 40, 1010–1020. doi: 10.2134/jeq2010.0407
Bove, R., and Lunghi, P. (2006). Electric power generation from landfill gas using traditional and innovative technologies. Energy Convers. Manag. 47, 1391–1401. doi: 10.1016/j.enconman.2005.08.017
Breitenmoser, L., Gross, T., Huesch, R., Rau, J., Dhar, H., Kumar, S., et al. (2019). Anaerobic digestion of biowastes in India: opportunities, challenges and research needs. J. Environ. Manage. 236, 396–412. doi: 10.1016/j.jenvman.2018.12.014
CPCB (2021). Annual Report 2020-21 on Implementation of Solid Waste Management Rules, 2016. Central Pollution Control Board, Government of India. Available at: https://cpcb.nic.in/uploads/MSW/MSW_AnnualReport_2020-21.pdf (accessed July 15, 2024).
Dissanayake, P. D., You, S., Igalavithana, A. D., Xia, Y., Bhatnagar, A., Gupta, S., et al. (2020). Novel insights into activated carbon derived from municipal solid waste for CO2 uptake: synthesis, adsorption isotherms and scale-up. J. Environ. Chem. Eng. 8:104069. doi: 10.1016/j.jece.2020.104069
Du, M., Peng, C., Wang, X., Chen, H., Wang, M., and Zhu, Q. (2017). Quantification of methane emissions from municipal solid waste landfills in China during the past decade. Renew. Sust. Energ. Rev. 78, 272–279. doi: 10.1016/j.rser.2017.04.082
Ebinger, C. K., and Potvin, J. (2016). India's Energy and Climate Policy. Brookings Institution. Available at: https://www.brookings.edu/wp-content/uploads/2016/07/india_energy_climate_policy_ebinger.pdf (accessed July 15, 2024).
Gebert, J., and Groengroeft, A. (2006). Passive landfill gas emission - Influence of atmospheric pressure and implications for the operation of methane-oxidising biofilters. Waste Manag. 26, 245–251. doi: 10.1016/j.wasman.2005.01.022
Ghosh, P., Shah, G., Chandra, R., Sahota, S., Kumar, H., Vijay, V. K., et al. (2019). Assessment of methane emissions and energy recovery potential from the municipal solid waste landfills of Delhi, India. Bioresour. Technol. 272, 611–615. doi: 10.1016/j.biortech.2018.10.069
Gollapalli, M., and Kota, S. H. (2018). Methane emissions from a landfill in north-east India: performance of various landfill gas emission models. Environ. Pollut. 234, 174–180. doi: 10.1016/j.envpol.2017.11.064
Guerrero, L. A., Maas, G., and Hogland, W. (2013) Solid waste management challenges for cities in developing countries. Waste Manag. 33, 220–232. doi: 10.1016/j.wasman.2012.09.008
Huber-Humer, M., Gebert, J., and Hilger, H. (2008). Biotic systems to mitigate landfill methane emissions. Waste Manag. Res. 26, 33–46. doi: 10.1177/0734242X07087977
IPCC (2011). “Technical summary,” in Climate Change: The Scientific Basis (Cambridge: Cambridge University Press).
Kaur, K., Mor, S., Vig, N., and Ravindra, K. (2022). Assessing the risk of landfill leachate on the groundwater quality of Chandigarh. J. Environ. Biol. 43, 593–603. doi: 10.22438/jeb/43/4/MRN-2097
Kaza, S., Yao, L., Bhada-Tata, P., and Van Woerden, F. (2018). What a Waste 2.0: A Global Snapshot of Solid Waste Management to 2050. World Bank Publications. doi: 10.1596/978-1-4648-1329-0
Krause, M. J., Chickering, G. W., Townsend, T. G., and Reinhart, D. R. (2016). Critical review of the methane generation potential of municipal solid waste. Crit. Rev. Environ. Sci. Technol. 46, 1117–1182. doi: 10.1080/10643389.2016.1204812
Kumar, S., Gaikwad, S. A., Shekdar, A. V., Kshirsagar, P. S., and Singh, R. N. (2004). Estimation method for national methane emission from solid waste landfills. Atmos. Environ. 38, 3481–3487. doi: 10.1016/j.atmosenv.2004.02.057
Lee, U., Han, J., and Wang, M. (2017). Evaluation of landfill gas emissions from municipal solid waste landfills for the life-cycle analysis of waste-to-energy pathways. J. Clean. Prod. 166, 335–342. doi: 10.1016/j.jclepro.2017.08.016
Manfredi, S., Tonini, D., and Christensen, T. H. (2009). Landfilling of waste: accounting of greenhouse gases and global warming contributions. Waste Manag. Res. 27, 825–836. doi: 10.1177/0734242X09348529
MoEFCC (2015). India's Intended Nationally Determined Contributions - Towards Climate Justice. Ministry of Environment Forest and Climate Change, Government of India. Available at: https://unfccc.int/sites/default/files/NDC/2022-06/INDIA%20INDC%20TO%20UNFCCC.pdf (accessed July 15, 2024).
Mohan, S., and Bindhu, B. K. (2008). Effect of phase separation on anaerobic digestion of kitchen waste. J. Environ. Eng. Sci. 7, 91–103. doi: 10.1139/S07-039
MoP (2017). DRAFT National Energy Policy NITI Aayog. Ministry of Power, Government of India. Available at: https://www.niti.gov.in/sites/default/files/2022-12/NEP-ID_27.06.2017.pdf.pdf (accessed July 15, 2024).
Mor, S., De Visscher, A., Ravindra, K., Dahiya, R. P., Chandra, A., and Van Cleemput, O. (2006). Induction of enhanced methane oxidation in compost: temperature and moisture response. Waste Manag. 26, 381–388. doi: 10.1016/j.wasman.2005.11.005
Mor, S., Negi, P., and Ravindra, K. (2018). Assessment of groundwater pollution by landfills in India using leachate pollution index and estimation of error. Environ. Nanotechnol. Monitor. Manag. 10, 467–476. doi: 10.1016/j.enmm.2018.09.002
Mor, S., and Ravindra, K. (2023). Municipal solid waste landfills in lower- and middle-income countries: environmental impacts, challenges and sustainable management practices. Proc. Safety Environ. Protect. 174, 510–530. doi: 10.1016/j.psep.2023.04.014
MOSPI (2017). Energy Statistics, 2017. Ministry of Statistics and Programme Implementation, Government of India. Available at: https://mospi.gov.in/sites/default/files/publication_reports/Energy_Statistics_2017r.pdf.pdf (accessed July 15, 2024).
Nabavi-Pelesaraei, A., Bayat, R., Hosseinzadeh-Bandbafha, H., Afrasyabi, H., and Chau, K. W. (2017). Modeling of energy consumption and environmental life cycle assessment for incineration and landfill systems of municipal solid waste management–A case study in Tehran Metropolis of Iran. J. Clean. Prod. 148, 427–440. doi: 10.1016/j.jclepro.2017.01.172
Negi, P., Mor, S., and Ravindra, K. (2020). Impact of landfill leachate on the groundwater quality in three cities of North India and health risk assessment. Environ. Dev. Sustain. 22, 1455–1474. doi: 10.1007/s10668-018-0257-1
Ngwabie, N. M., Wirlen, Y. L., Yinda, G. S., and VanderZaag, A. C. (2019). Quantifying greenhouse gas emissions from municipal solid waste dumpsites in Cameroon. Waste Manag. 87, 947–953. doi: 10.1016/j.wasman.2018.02.048
Powell, J. T., Townsend, T. G., and Zimmerman, J. B. (2016). Estimates of solid waste disposal rates and reduction targets for landfill gas emissions. Nat. Clim. Change 6, 162–165. doi: 10.1038/nclimate2804
Rasi, S., Läntelä, J., and Rintala, J. (2011). Trace compounds affecting biogas energy utilisation – a review. Energy Convers. Manag. 52, 3369–3375. doi: 10.1016/j.enconman.2011.07.005
Ravindra, K., Kaur, K., and Mor, S. (2015). System analysis of municipal solid waste management in Chandigarh and minimization practices for cleaner emissions. J. Clean. Prod. 89, 251–256. doi: 10.1016/j.jclepro.2014.10.036
Scheutz, C., Kjeldsen, P., Bogner, J. E., De Visscher, A., Gebert, J., Hilger, H. A., et al. (2009). Microbial methane oxidation processes and technologies for mitigation of landfill gas emissions. Waste Manag. Res. 27, 409–455. doi: 10.1177/0734242X09339325
Scheutz, C., Samuelsson, J., Fredenslund, A. M., and Kjeldsen, P. (2011). Quantification of methane emissions from 15 Danish landfills using the mobile tracer dispersion method. Waste Manag. 31, 1009–1018. doi: 10.1016/j.wasman.2011.01.015
Sharholy, M., Ahmad, K., Mahmood, G., and Trivedi, R. C. (2008). Municipal solid waste management in Indian cities – a review. Waste Manag. 28, 459–467. doi: 10.1016/j.wasman.2007.02.008
Shashidhar and Kumar, O. M.. (2011). Municipal solid waste management of Warangal city, India. J. Environ. Res. Dev. 6, 111–121.
Singh, C. K., Kumar, A., and Roy, S. S. (2018). Quantitative analysis of the methane gas emissions from municipal solid waste in India. Sci. Rep. 8:2913. doi: 10.1038/s41598-018-21326-9
Spokas, K., Bogner, J., Chanton, J. P., Morcet, M., Aran, C., Graff, C., et al. (2003). Methane mass balance at three landfill sites: What is the efficiency of capture by gas collection systems? Waste Manag. 23, 419–429. doi: 10.1016/j.wasman.2005.07.021
Srivastava, R. K., Shetti, N. P., Reddy, K. R., and Aminabhavi, T. M. (2020). Sustainable energy from waste organic matters via efficient microbial processes. Sci. Tot. Environ. 722:137927. doi: 10.1016/j.scitotenv.2020.137927
Stamps, B. W., Lyles, C. N., Suflita, J. M., Masoner, J. R., Cozzarelli, I. M., Kolpin, D. W., et al. (2016). Municipal solid waste landfills harbor distinct microbiomes. Front. Microbiol. 7:534. doi: 10.3389/fmicb.2016.00534
Tchobanoglous, G., Theisen, H., and Vigil, S. (1993). Integrated Solid Waste Management: Engineering Principles and Management Issues. New York: McGraw-Hill.
Themelis, N. J., and Ulloa, P. A. (2007). Methane generation in landfills. Renew. Energy 32, 1243–1257. doi: 10.1016/j.renene.2006.04.020
United Nations (2015). Transforming our world: the 2030 agenda for sustainable development. A/RES/70/1. Available at: https://documents.un.org/doc/undoc/gen/n15/291/89/pdf/n1529189.pdf?token=jdlFch1VPLQ6Xi4qpY&fe=true (accessed July 15, 2024).
USEPA (2001). Total, fixed, and volatile solids in water, solids and biosolids, EPA-821- R-01-015. Washington, DC: USEPA.
USEPA (2005). Landfill Gas Emissions Model (LandGEM) Version 3.02 User's Guide. EPA-600/R-05/047. Washington, DC: USEPA.
Keywords: methane emissions, municipal solid waste, electricity generation, first-order decay, waste-to-energy
Citation: Mor S, Kaur K and Ravindra K (2024) Methane emissions from municipal landfills: a case study of Chandigarh and economic evaluation for waste-to-energy generation in India. Front. Sustain. Cities 6:1432995. doi: 10.3389/frsc.2024.1432995
Received: 15 May 2024; Accepted: 25 July 2024;
Published: 23 August 2024.
Edited by:
Amalia Zucaro, Italian National Agency for New Technologies, Energy and Sustainable Economic Development (ENEA), ItalyReviewed by:
I. Wayan Koko Suryawan, Pertamina University, IndonesiaMohsen Karimi, University of Porto, Portugal
Copyright © 2024 Mor, Kaur and Ravindra. This is an open-access article distributed under the terms of the Creative Commons Attribution License (CC BY). The use, distribution or reproduction in other forums is permitted, provided the original author(s) and the copyright owner(s) are credited and that the original publication in this journal is cited, in accordance with accepted academic practice. No use, distribution or reproduction is permitted which does not comply with these terms.
*Correspondence: Khaiwal Ravindra, khaiwal@yahoo.com