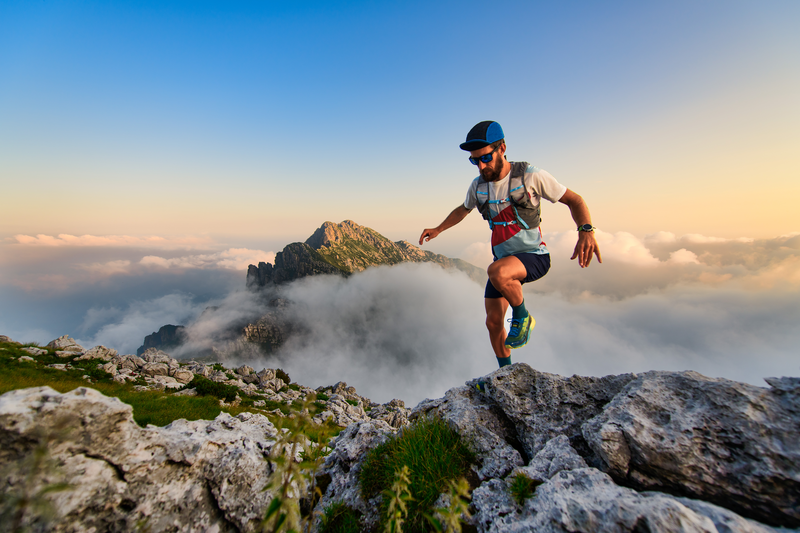
94% of researchers rate our articles as excellent or good
Learn more about the work of our research integrity team to safeguard the quality of each article we publish.
Find out more
ORIGINAL RESEARCH article
Front. Sustain. Cities , 03 May 2024
Sec. Sustainable Infrastructure
Volume 6 - 2024 | https://doi.org/10.3389/frsc.2024.1379554
Introduction: Thermal grids are key to decarbonizing heating and cooling. However, their development is a complex socio-technical process. This study aims to (1) understand the thermal grid ecosystem’s development under changing political, economic and cultural frame conditions, (2) elicit actors’ perception of options for future development, and (3) identify concrete problem situations for the future development of thermal grids and describe how to address them.
Methods: We draw on 18 semi-structured interviews with decision-makers in utilities, municipal administrations, planning and engineering companies and intermediary organizations.
Results: With increased strategic importance of thermal grids, the ecosystem has been in a phase of rapid growth and professionalization in the last 10–15 years. This is reflected in a rapid increase in the number of grids, but also in capability and capacity building and greater quality standards. This transformation has been supported by a resource pool (e.g., knowledge base, capacities and capabilities, networks or decision-support tools), built partly through the value network’s activities and partly through purposeful orchestration by associations, authorities, utilities and other actors. While policy developments, energy price fluctuations and changes in customer perceptions spurred demand, thermal grid development is limited by supply-side constraints, especially a skill shortage. Four interrelated problem situations were identified: (1) Municipalities lack the resources to manage the implementation of their energy strategies. (2) The modernization of thermal grids and integration of low-carbon heat sources entails high transaction costs. (3) The implementation of thermal grids is subject to delays and quality risks, with consequences for the timely achievement of decarbonization goals. (4) Customers face uncertainty on available options, as well as on the systemic impacts of their decisions.
Discussion: Based on current practices, suggestions by the interviewees, as well as a comparison with the international literature, we identify four development areas to address these problem situations: (1) Empower and support municipalities. (2) Manage the scarcity of qualified workforce. (3) Leverage digital technology for the planning, realization and operation of thermal grids. (4) Pilot technological and organizational innovations together. This complements the set of previously suggested measures to support the development of thermal grids, which focused strongly on technical and regulatory aspects.
Thermal grids are key to decarbonize heating, particularly in urban environments where they are often the only practical option for low-carbon heating (Connolly et al., 2014). Thermal grids are strongly localized, leading to diversity in the configuration of local socio-technical systems (Bolton and Hannon, 2016; Paardekooper et al., 2022). This complicates the task of formulating generic strategies and measures (Paardekooper et al., 2022). Indeed, the direction of the transition varies with local context: indicated measures range from optimization and incremental growth (Leoni et al., 2020) to whole-system reconfiguration (Reda et al., 2021). Several distinct socio-technical challenges have been described, such as planning and developing new grids (Busch et al., 2017); (inter-)organizational transformation (Sandoff and Williamsson, 2016; Lygnerud, 2018), changes in technology (Lygnerud, 2019) or digitalization (Peltokorpi et al., 2019); or the embedding in municipal energy strategies (Gabillet, 2015; Späth and Rohracher, 2015).
While most of the literature on thermal grids focuses on technical aspects, various theoretical perspectives have been applied to address these socio-technical challenges: business models (Bolton and Hannon, 2016; Lygnerud, 2019), sustainability transition frameworks (Gabillet, 2015; Bolton and Hannon, 2016; Reda et al., 2021) or legal governance (Heldeweg et al., 2017). Each of these perspectives yielded unique insights: business model studies highlighted the necessary organizational changes within utilities to cope with evolving technological frame conditions and customer requirements (Lygnerud, 2018) and the location-specific prospects of different models (Bolton and Hannon, 2016). Furthermore, this perspective helped suggest concrete organizational measures to address specific problems, such as substation performance (Leoni et al., 2020). Sustainability transition frameworks place thermal grids and their organization in the broader perspective of evolving societal priorities, mapping conflicts and synergies with political and institutional developments. Concretely, studies described the fit (or lack thereof) of thermal grid development with urban planning (Gabillet, 2015) and current routines, incentives and mental models (Bolton and Hannon, 2016; Reda et al., 2021). Studies on legal governance have focused on the regulatory options at different governance levels to orchestrate the multilateral sets of organizations involved in the planning and operation of thermal grids (Heldeweg et al., 2017).
Nevertheless, various gaps were identified in research, practice and at their interface. In business model studies, authors noted the need to look beyond individual organizations. Indeed, the suggested business model changes imply the establishment of new partnerships, requiring a proactive strategy from utilities (Leoni et al., 2020; Lygnerud et al., 2023). Also, the success of local solutions is closely coupled to their context, so that replicating them requires an understanding of the interdependencies between business, infrastructural and institutional aspects, as well as strategic action to achieve alignment between them (Bolton and Hannon, 2016; Reda et al., 2021). From a public governance perspective, authors have noted the need to be aware of frictions and differing viewpoints between actors and to address them in energy policy (Gabillet, 2015; Späth and Rohracher, 2015).
In this paper, we propose that a business ecosystem perspective helps address the challenges of inter-organizational coordination typical of thermal grids. The business ecosystem concept was proposed by Moore (1993) to address the interdependencies between firms organized around a common value proposition. Using the ecosystem as the unit of analysis has given insights on strategic action to orchestrate the interaction of a multilateral set of partners (Adner, 2017; Lingens et al., 2022). The ecosystem perspective enables a joint analysis of value creation for customers, businesses and society (Leviäkangas and Öörni, 2020; Kanda et al., 2021). Regarding thermal grids, Peltokorpi et al. (2019) used an ecosystem perspective to design the inter-organizational arrangement around a digital innovation. Following a case study design (Flyvbjerg, 2006), we investigate the thermal grid ecosystem in Switzerland. Based on 18 semi-structured interviews with industry and public-sector actors, we aim to (1) understand the industry’s development under changing political, economic and cultural frame conditions, (2) elicit actors’ perception of future development options, and (3) identify concrete inter-organizational problem situations and describe how to address them. We focus not only on infrastructure development but consider the diverse resources that enable the industry’s development. For this reason, we place a special focus on the role of digital planning tools—a potentially enabling resource for low-carbon transitions (Bush and Bale, 2019)—as perceived by industry actors. We adopt a normative approach insofar as we see decarbonization as desirable, and we acknowledge that thermal grids have an important part in the process. However, we make no claims on which technology is preferrable in any given situation.
Following an overview of the situation in Switzerland, we describe our analytical framework, data acquisition and analysis (Section 2), present the results of the interviews (Section 3), discuss their implications in the light of international literature (Section 4) and present the conclusions for research and practice (Section 5).
Buildings account for 24% of Switzerland’s GHG emissions (Swiss Federal Office of the Environment, 2020), mostly through fossil-fueled heating. About 70% of final energy demand for space heating is still covered by oil and natural gas (Figure 1, left). Since 2013, Switzerland aimed at a 40% reduction of its 1990-level GHG emissions from the building sector by 2020. However, by 2018, a reduction by only 34% was reached (Swiss Federal Office of the Environment, 2020). This reduction reflects several parallel developments (Bhadbhade et al., 2020): it occurred despite a 30% increase in population and a 50% increase in energy reference area, so that the specific emissions per unit energy reference area decreased by 56% (Swiss Federal Office of the Environment, 2021). This is explained by several developments. First, new and retrofitted buildings have a much lower specific energy demand (Streicher et al., 2018). Second, despite the prevalence of fossil fuels, the share of renewable sources (mostly ambient heat) is increasing. Additionally, some oil-based systems were replaced with natural gas (Swiss Federal Office of Energy, 2020). Finally, increasing winter temperatures reduced demand (Berger and Worlitschek, 2019; Swiss Federal Office of Energy, 2020).
Figure 1. Historical development of final energy use for space heating in Switzerland, by energy carrier (Swiss Federal Office of Energy, 2020) (left). EPCH 2050+ scenarios for space heating under a net-zero energy system (Prognos/Infras/TEP Energy/Ecoplan, 2021) (right).
Thermal grids currently cover a small percentage of demand, with heat delivery estimated at 8′500 GWh/a (Hangartner and Hurni, 2022). The sector is diverse: while over 60% of grids are fueled by wood chips, these account only for 30% of energy. The scale of those grids ranges from blocks (<0.1 MW) to small cities (~20 MW). By contrast, few large grids (up to several hundred MW) using heat from waste-to-energy CHP plants account for 45% of heat delivery, primarily in cities. Recent years have seen an increase in grids using renewable energy sources, i.e., biomass, but also ambient heat from rivers, lakes, groundwater or the ground, as well as waste heat from industry, sewage treatment plants or tunnels. While fossil fuels are used in few grids as the main energy carrier, their main role is as a backup or to cover peak loads. Also, while some grids integrate solar thermal or excess heat from buildings, these are rarely the main energy source (Hangartner and Hurni, 2022). Whereas forecasts see a long-term potential about twice or three times as large as the current heat delivery in thermal grids (see below), experts note that the most easily accessible and cost-effective energy sources have already been tapped, so that realizing this potential will be challenging (Hangartner and Hurni, 2022). Particularly promising energy sources are ambient heat, due to the large economic potential (Hangartner and Hurni, 2022) and industrial waste heat, due to potentially lower costs (Zuberi et al., 2021), as well as geothermal energy in the longer term (Swiss Federal Office of Energy, 2021).
Switzerland has committed to achieving net-zero greenhouse gas (GHG) emissions by 2050. The federal climate strategy (Federal Council, 2021) foresees a complete avoidance of GHG emissions from buildings, so that 900′000 residential buildings require a substitution of fossil fuels, in addition to fossil-fueled boilers in commerce and industry.
The national energy perspectives, hereafter “EPCH 2050+” (Prognos/Infras/TEP Energy/Ecoplan, 2021), present a set of model-based scenarios reflecting a net-zero compatible development of the Swiss energy system. For heating, these scenarios foresee a reduction of energy demand by 25% and a quasi-total substitution of fossil fuels (Figure 1, right). Heat delivery through thermal grids is set to double or triple under these scenarios, although researchers have noted that the EPCH2050+ underestimate the potential of renewables-based grids (Suisse, 2021). Thermal grids are particularly important in urban areas with high heat demand density and various constraints (e.g., space, esthetics, noise, difficulty to retrofit buildings) that make other low-carbon systems unviable (Zuberi et al., 2021). In summary, despite some progress, accelerating decarbonization is necessary to reach the net-zero goals by 2050. This is illustrated by the business-as-usual scenario of the EPCH2050+ (“BAU” on Figure 1), where a continuation of current policies and market conditions lead to continued prevalence of fossil fuels and no growth for thermal grids.
Timing is critical (TEP Energy and ECOPLAN, 2020; Zuberi et al., 2021): If new grids are not realized in time, building owners may opt for a decentral solution, decreasing the viability of planned grids (TEP Energy and ECOPLAN, 2020). To realize the potential of low-carbon thermal grids, several recent studies have formulated recommendations to policymakers, authorities, utilities and other actors (Table 1).
As noted in the Introduction, a key challenge to the development of thermal grids is to achieve alignment between a multilateral set of organizations, both in the public and private sector. This study therefore uses the co-evolutionary business ecosystem perspective (CEBEP; Speich and Ulli-Beer, 2023) to investigate the Swiss thermal grid ecosystem. This framework (Figure 2) builds upon a dynamic view of ecosystems (Ma et al., 2018; Shi et al., 2022) and conceptualizes an ecosystem as consisting of a value network (the involved organizations and the relationships between them) and a resource pool enabling the ecosystem’s functioning and further development. The definition of resource is deliberately broad and includes material, financial, human, cognitive, institutional or intangible resources (Ma et al., 2018). The CEBEP assumes a dynamic interaction: the resource pool is enriched through the value network’s activities (e.g., through learning effects), and enables a further development of the value network (e.g., business model innovation). These interactions are referred to as “industrial feedback” and “value network transformation,” respectively. However, the value network and resource pool can also change due to purposeful, strategic actions by members or non-members, i.e., orchestration (Visnjic et al., 2016; Autio, 2021).
Figure 2. Structure of the co-evolutionary business ecosystem perspective (Speich and Ulli-Beer, 2023).
The CEBEP distinguishes between the ecosystem and its environment. The boundary is set so that the value network includes all actors that must interact so that a value proposition can be realized (Adner, 2017). In this case, the value proposition consists of developing thermal grids in accordance with public policy goals. Further, the CEBEP assumes that ecosystems are nested at various level: for example, the ecosystem around thermal grids in a municipality is nested within a thermal grid ecosystem at national level. The environment includes the dimensions that affect the ecosystem, based on the components of a socio-technical regime (Geels, 2004).
The CEBEP studies alignment between value creation and capture for customers, businesses and the public in low-carbon transitions. This framework builds upon a hierarchical conceptualization of value (Leviäkangas and Öörni, 2020): the realization of public policy goals, such as GHG emission reduction pathways, depends on the ability of businesses to generate profits from the deployment of clean technologies. Profitability, in turn, requires an attractive value proposition that creates value for the customer. Between the public and business levels lies another level of value, that of the business ecosystem. Value is created at this level by facilitating value creation and capture and creation for the value network’s members through mediation, complementary resources, knowledge management, etc.
The primary data consists of 18 semi-structured interviews with actors from the thermal grid ecosystem in Switzerland, carried out between September 2022 and January 2023. Most respondents have a leading function (e.g., CEO, business unit leader, municipal energy responsible). The interviews were carried out in-person or online and lasted between 60 and 90 min. An interview protocol was established based on known issues of thermal grid development (Table 1) and recommendations by experts on technical aspects of thermal grids and energy systems.
Organizations were chosen to reflect the heterogeneity of thermal grids in Switzerland regarding degree of urbanization, available energy potentials, current state of development, ownership structures and innovativeness (Table 2). The seniority of most participants further increases the diversity of perspectives (several respondents drew upon their experience from past positions) and ensures that long-term dynamics are accounted for. Following the theoretical sampling approach (Glaser and Strauss, 2017), the sample was not fully defined a priori. Rather, interviews were iteratively conducted, transcribed and analyzed, and new participants were contacted based on further information needs. Some participants were suggested by the respondents themselves. This process was repeated until additional interviews seemed not to yield any new information. To ensure an open conversation, respondents were promised anonymity.
In addition to the ecosystem overview, this study places a specific focus on the role of digital planning tools. For this reason, 16 interviews featured a demonstration of a newly-developed interactive planning tool (Chambers et al., 2023). TESSA (Thermal Energy System Simulation Assistant) is an online software tool for district thermal energy planning with a focus on district heating systems, that is developed as a technology transfer project to bring previous research on building and city simulation and district heating to practitioners. TESSA integrates building energy data (Chambers et al., 2021) and district heat network layout algorithms (Chambers, 2020) into a tool that allows fast design and evaluation of district heat network projects. After this demonstration, respondents were asked about their perception of the potential of such a tool to facilitate the development of thermal grids.
We applied a structured content analysis (Mayring, 2019). The coding approach is primarily deductive and theory-led, as the categories correspond to the CEBEP’s elements (see above). However, codes were inductively refined to differentiate the actor and resource types. Responses to the planning tool demonstration were coded with a separate scheme based on the hierarchy of values underlying the CEBEP (see above): statements on the tool’s potential impact were assigned to one of the four value dimensions (customer, business, ecosystem or public). A second coding dimension, describing the tool’s specific features, was developed inductively.
This analysis is complemented with a second interpretation of the data, where content analysis results are recombined into distinct problem situations. The idea is to decompose the “messy problem” of thermal grid development into smaller, potentially more manageable problem situations (Metlay and Sarewitz, 2012). While the two interpretations are presented sequentially, they were established simultaneously: the identification and description of the problem situations was done iteratively during the coding process, through memo-writing, discussions within the author team and comparison with secondary sources.
In addition, the following data sources were used:
• Unstructured interviews with employees of a city utility, city administration and two planning and engineering firms, during which notes were taken.
• Two workshops with employees of a city utility and city administration, as well as researchers on technical, socio-economic and legal aspects of thermal grids, during which notes were taken.
• Participation in several events (conferences, symposia, networking meetings, etc.) attended by industry members and researchers, between April 2021 and March 2023.
• Regular exchange with researchers specialized on technical and legal aspects of thermal grids within the interdisciplinary research project SWEET-DeCarbCH.
• Policy documents, media reports, communication materials, etc. on the participating organizations were studied before and after the interviews to obtain relevant context information.
Various developments (e.g., the vote on the federal energy strategy in 2017 and the government’s net-zero commitment in 2019) are seen as a major accelerating factor, strengthening the perception of thermal grid development as a necessity. At cantonal level, several cantons have banned new fossil-fueled boilers. These new laws were characterized as a major driver for thermal grid development, whereas the older version was seen as a hindrance. At municipal level, referenda on thermal grid development (e.g., on public credits) were well-supported, indicating high socio-political acceptance. Respondents in cantons and cities with such recent votes were generally optimistic about the policy frame conditions for ambitious decarbonization timelines, despite political opposition to various energy-related law proposals (e.g., CO2 Act). The simple fact that energy is a frequent discussion topic was seen as positive, increasing public awareness of the energy transition.
Energy prices are a dominant theme, particularly in the months prior to the interview campaign. High fossil fuel prices were said to impact customers’ choice, particularly industrial and commercial energy users. In addition, the geopolitical situation led to changing perceptions of price volatility and energy independency, so that thermal grids, perceived as stable and locally embedded, are currently in high demand. According to respondents, this is in line with a general trend regarding renewable and energy-efficient solutions, such as photovoltaics, individual heat pumps and electric vehicles. On the other side, various utilities noted supply-chain issues delaying the delivery of components. This was expected to be a temporary issue, ascribed to global supply chain delays and to the rapid growth of thermal grids.
Historically, the Swiss district heating industry was fragmented, with local developments driven primarily by opportunity (cheap and easily deployable energy source, such as high-temperature excess heat or residual biomass from forestry and wood processing). In the 1980s, grids with fossil-fueled CHPs were realized punctually, which then represented an energy-efficient innovation. Today, three main types of actors can be distinguished: municipal utilities, energy service companies (ESCOs), as well as companies operating grids as a side-business (e.g., farms, sawmills, industrial companies). Some organizations do not fall into a single category, e.g., utilities operating both in their home municipality and elsewhere. Respondents do not expect many new entrants, as they see two high entry barriers: the specific know-how needed to develop and operate thermal grids, and the high degree of trust associated with incumbents, who are mostly locally or nationally anchored organizations with a long history.
A changing perception of thermal grids by customers was noted: Due to current geopolitical developments, regional and national energy independency are valued much higher. As a result, the perception of long-term contracts as too constraining –commonly cited as a high barrier to customer acceptance-decreased. Respondents expect this shift to be more than temporary, but stress the importance of exploiting the current momentum. The interviews also gave indications of the mindsets, perceptions and logics of industry members, through the choice of topics by interviewees, expressed values and opinions, stated causal relationships and choice of vocabulary. Broadly, the three logics identified by Wesseling et al. (2022) are all represented in the industry: engineering, sustainability and market logics.1 The engineering logic, which values technical efficiency and ingenuity, is strongly represented: for instance, respondents expressed aversion toward systems judged inefficient (“we are currently retrofitting (our old grids), they were all built in a way they should not have been built”) or unsound (“these interrelations are not taken seriously enough in my opinion. There are completely arbitrary things …, very large grids where I cannot understand why it was done like this. Technically, this is totally wrong.”). The sustainability logic manifests itself in the often-expressed opinion that decarbonization is necessary, and in actions that cannot be explained by direct pressures or incentives (e.g., decarbonization of an ESCO’s portfolio of grids although this is not a priority of customers; construction and operation of a grid on a non-profit basis and at own risk by an industrial company). Elements of a market logic were expressed by a belief in the benefits of competition for a sound development of organizations (“I do not want (an obligation to connect), we must be competitive. And we are managing that.”). Overall, the engineering and sustainability logics seem more important than the market logic. This is in line with the economics of thermal grids (payback times of several decades, slim profits), and with the background of respondents: many decision-makers have a technical education, either as a vocational or as an engineering degree.
This section characterizes the main actor groups, with the utility as the focal entity. Some generic observations can be made: first, there is a high level of agreement on the necessity to decarbonize. While disagreements exist (e.g., between municipalities and utilities), these concern the choice of grid type and energy carrier or the timing of implementation, but a fundamental opposition to decarbonization was not observed. Second, in the last decade, the industry became markedly more professional: capability and capacity building ensure that new developments are of a higher quality, and older infrastructure is being modernized. Some respondents also indicate a greater status of the industry within the energy sector. Third, there is a skills shortage throughout the entire value network: utilities, engineering and planning offices as well as installation and construction companies struggle to build the necessary capacities and capabilities to satisfy the rapidly increasing customer demand on time. This, combined with supply chain delays, was found to delay new developments and to negatively impact their quality. To summarize, development of thermal grids can be characterized as supply-side limited, with fast-growing customer demand that often cannot be met. This situation is exacerbated by the lack of qualified workforce and material supply chain delays.
Grid operators in Switzerland are heterogeneous regarding organizational size; size, type and number of grids; organizational form and ownership status. A distinction in the scope of action was made between utilities integrated into the municipal administration and those that are legally independent (although these were often under public ownership). In the former case, respondents noted the advantage of high trust, which facilitates credit procurement at favorable conditions. Moreover, they pointed out that public status increased social acceptance: due to political control over investment decisions and tariffs, such utilities are perceived as acting in the public interest. By contrast, respondents from legally independent utilities pointed out a greater freedom in decision-making (the converse was pointed out as a disadvantage by legally dependent utilities) and the ability to start new projects quickly. In such organizations, managers answer to panels composed mostly of experts, so that decision-making is based on economic and technical, rather than political considerations. Another relevant organizational factor is the multi-utility form: interviewees in such utilities pointed out a great potential for synergies, making it easier to offer alternative products to customers who do not wish to connect.
Several respondents have pinpointed to around 2010 the start of a rapid growth phase, leading to important organizational changes, such as the development of dedicated business units for heat in utilities. However, interviewees from utilities described their organization as being capacity-limited for the implementation of new projects. As a result, there is very little competition: ESCOs do not see a priority in acquiring new contracts, and various municipal utilities welcome the presence of external energy providers on their territories to some degree (coordination is sometimes necessary, although this was reported to be a minor issue). Utilities rather prioritize densification and modernization of their existing grids. An exception are utilities whose current revenues depend on natural gas sales: some of these companies are reportedly much more active in the acquisition of new contracts, or actively seek cooperations.
Municipalities assume various roles: strategic and operational planning and coordination, principal for the realization of thermal grids, heat customer, and regulatory authority.2 These interdependent roles are often carried out by different units. Municipalities may have an energy office, sometimes in combination with other topics (e.g., environment, mobility). If the municipality has politically defined energy or emission reduction targets, this office is responsible for elaborating concrete strategies—i.e. for crafting a public value proposition (Moore, 1995). This may include a generic strategy on infrastructural and environmental topics, and/or specific strategies for the heating sector. Another frequent measure is spatial energy planning. Cities with greater infrastructural complexity also formulate target infrastructure plans, where thermal grid development is coordinated with, e.g., natural gas and electricity grids. Ideally, these instruments have a strong coordinating function for utilities, authorities, policymakers and customers. Success factors are a strong involvement of at least the energy office and utilities, but also the civil engineering office and local businesses. As formulated by a utility manager: “Now we have the goal of being CO2-neutral by 2035. (The municipal energy office) formulates scenarios how this could become a reality. Our task is then not to stall, but to bring in our reality so that the scenario can become a plan.” However, this coordinating function is not always fulfilled: municipal plans and strategies are sometimes disregarded. This has been ascribed to quality issues, which weaken acceptance of the plan. Coordination also takes place less formally, e.g., through round tables or regular meetings where misunderstandings and differences in opinion can be clarified. At the operational level, coordination is necessary to align the timelines of infrastructure construction and decarbonization pathways.
Municipalities can act as customers by mandating a utility to build a grid, and by using energy in public buildings. The former type of relationship is often arranged through a concession contract, although some contracts were based on private law [for a detailed characterization of the legal framework around thermal grids (see Abegg and Musliu, 2022)]. Respondents encountered different arrangements, with some municipalities charging a concession fee while others did not. Depending on local arrangements, the requested fee may become prohibitively high. The relationship between utilities and municipalities can be initiated through a call for tender, or through direct contact (actors from both groups have been reported to take the initiative). Since there is little competition between utilities, some ESCOs indicated little participation in calls for tender: “at the moment, with the energy strategy and certainly with the high fossil fuel prices, we and all our colleagues (i.e., other ESCOs) are fully absorbed with our existing projects. If someone issues a call for tender now, they must probably make a great effort to get even one offer.” One consultant also questioned the tendering instrument: since thermal grids are not highly profitable and often supported through public funds, it was deemed questionable to lower costs through tendering. More informally, active support of the municipality was described as an important success factor for developments, in the form of continuous information on planned area developments or zoning changes. A particular situation arises when a municipality owns the infrastructure on its territory. One consultant questioned whether local authorities were the right structures to operate thermal grids, citing slow decision-making and lacking capacities. However, another consultant saw a great potential for capability development, e.g., in municipalities with their own natural gas supplier. In any case, municipalities seem increasingly interested in such options. Conversely, municipalities may choose to sell their assets. This decision process involves the decisions whether to sell, to whom and under which conditions. The latter point entails not only the selling price, but also additional services provided by the new owner or specific clauses, e.g., a binding percentage of renewable energy or compensatory measures in case of project failure. According to one respondent from a local authority, decision-makers in municipalities often do not have the knowledge to consider the full range of options and to appreciate their consequences.
Finally, municipalities also act as regulatory authorities, administering the permitting process (see Abegg and Musliu, 2022). Permitting was described as challenging, delaying or hindering new developments. According to one respondent, this is especially the case when a utility develops thermal grids on a municipality’s territory for the first time. Much effort may be necessary to build trust and establish a partnership—a process that may take several years (cf. Section 3.2, P1).
A third important group is composed of consulting, engineering and planning companies. Their services range from strategic planning and feasibility studies to the detailed planning of grid construction (e.g., pipe layout, hydraulics, detailed construction planning, monitoring in the first operation years)—some of these services are often offered by the same company, while others simultaneously offer construction services. In addition, consultants offer complementary services such as quality management. Also, non-technical services such as product development, project development, consortium building, business planning, marketing and consulting on organizational development are increasingly provided. These activities are sometimes carried out by conventional engineering firms. According to one respondent, this is unfortunate: since this does not correspond to the firm’s core competencies, they were concerned that communication and coordination were not done professionally enough, and that these activities bind the already scarce resources for technical planning. However, other firms have built business units for such activities, and new firms with such a focus have been founded.
These companies also suffer from the generalized workforce shortage in the industry. This manifests itself in different ways: first, there are only few firms with knowledge on thermal grids, and specific skills (e.g., large-scale heating plants, low-temperature grids) are rare. While some firms have long-standing experience, many have only recently started to build competencies. Second, firms are often capacity-limited, so that utilities compete for hiring good planning and engineering companies. Public utilities may be disadvantaged, as public procurement keeps them from building long-standing cooperations. However, respondents pointed out that such long-standing cooperations were neither in the interest of engineering firms (who wish to remain flexible) nor of utilities (who need distinct skills for each project). Therefore, collaborations between utilities and engineering firms usually take place on a project-by-project basis. Third, firms struggle to hire qualified workers. While this shortage concerns all activities, it was described as particularly acute in operative technical planning: “for decarbonization, you need planners who understand what it is about. For example, you must understand hydraulic engineering within the configuration of energy systems so that you can make an implementation plan and monitor construction. Not enough (training) is done at this level currently. You can do a lot on a high conceptual level, but at some point, you need to bring it to the ground, and people are missing there.” While respondents welcome new educational resources (see below), this is seen as an important bottleneck also in the future. Finally, the skills shortage also leads to quality issues. For strategic planning, this is due to the diversity of energy potentials, so that accurately estimating and localizing their size can be challenging. Also, stakeholder is a complex task, and the budget for energy planning may not allow a thorough coordination. For operative planning, the lack of experience in engineering firms is seen as a risk by utilities.
The value network contains other important members, whose role is briefly outlined here. Actors upstream of the energy supply chain (e.g., providers of excess heat, biomass, natural gas, oil, etc.) were often in a purely contractual relationship with utilities. For excess heat, various arrangements were described regarding the distribution of activities, responsibilities and risks as well as value capture mechanisms. Potential excess heat providers often face high uncertainty regarding technical or organizational aspects. Also, the willingness to provide excess heat varies greatly between organizations (for example, data center operators have much shorter planning horizons, giving them little incentive to engage in long-term contracts), but also within organizations. Actors of the material supply chain (technology providers, construction companies) are also impacted by workforce shortages and supply-chain delays, further intensifying this problem. Direct contact with investors was limited in most cases, although one respondent cited personal networks as a success factor. In general, it was reported that financing became somewhat easier over the years due to decreasing specific project costs. Gas utilities were said to have limited direct interactions with thermal grid operators. Due, e.g., to energy planning, direct competition in the same area is rare. Finally, landowners and neighbors were mentioned as relevant actors due to the possibility to use legal means to hinder projects.
As described above, the ecosystem is developing quickly. As a result, the value network is changing, interacting dynamically with the resource pool (Figure 3). An often-mentioned theme is greater professionalism, reflected, e.g., in the capability and capacity building of utilities and engineering firms. This manifests itself not only in quantitative growth, but also in a quality increase: new infrastructure is more efficient, and old infrastructure is currently being modernized. This development is supported through fostering shared resources. For example, while a major bottleneck (see Section 3.1.2), the workforce has been developed both through orchestration (targeted continuing education offers by educational institutions and private companies, with coordination by industry associations; see also Table 3), but also as a result of the value network’s activity, since workers acquire experience on the job and are sometimes trained in-house. Another vital resource is a knowledge base, consisting of standards and norms, but also including guidelines such as a planning handbook (Nussbaumer et al., 2018) and long-term perspectives (Sres and Nussbaumer, 2014). Also here, these resources were often developed purposefully, e.g., by industry associations and mandated firms, but knowledge is also shared less formally: some respondents had received requests from other utilities for help on topics for which they had developed specific expertise. Another side of the value network transformation are new offerings, e.g., low-temperature grids. Also here, the skills required must be developed. The diffusion of such offerings benefits from legitimacy, an intangible resource built through successful applications. A third aspect of transformation is excess heat integration. Due to the heterogeneity of potential excess heat providers, the process of identifying them and negotiating their involvement is often situation specific. There may different perceptions of exploitable potentials within a single company (and different prioritizations of energy-related issues vs. the company’s other activities), so that the success of such an initiative depends upon which employees are involved. Excess heat recuperation in thermal grids can be costly or risky for the heat provider, so that personal conviction was described as a necessary condition. One industrial excess heat provider argued out of a sustainability logic (see Section 3.1.1), citing the will of the company to contribute to decarbonization. For the orchestrator, such an initiative can also be costly and challenging, and requires skills to “sense and seize” such opportunities. A barrier has been spotted in this respect: whereas orchestrating such initiatives requires in-depth technical knowledge– usually possessed by utilities and engineering firms –organizations with such capabilities are capacity-limited and may not feel competent to carry out coordination tasks.
Figure 3. Elements of the national resource pool and ecosystem dynamics. For items marked with an orange circle, orchestration is/was necessary (see Table 3).
Respondents were asked about their perception of technological options for the improvement and development of thermal grids. Some of these options are already in use, so that responses include both prospective statements and experience sharing. While the initial interview protocol contained a list of technological options, further options were spontaneously cited. This section presents the opinions collected during the interviews (we stress that this does not constitute an assessment of technological suitability by us).
Integrating renewable energy sources and excess heat is an important potential benefit of thermal grids. However, respondents highlighted the challenges of integrating energy sources other than biomass, high-grade excess heat or deep geothermal energy (the latter being seen as a long-term option) into high-temperature grids due to the temperature difference (grid topology was also mentioned as a challenging topic). Reducing supply and/or return temperature is therefore a prerequisite, but is itself challenging. In various grids, supply temperature is dynamic, following seasonal or diurnal schedules. While positive experiences were made with seasonal schedules (i.e., lower temperature in summer than in winter), the benefits of night-time temperature reduction were questioned due to compensation effects in the morning. For permanent temperature reduction, several challenges were mentioned: First, there is a trade-off between temperature reduction and transport capacity, which constrains grid expansion if the supply temperature is being reduced. Therefore, prioritization is necessary. Second, due to the demand-side dependency, it can take decades before the temperature can be reduced. As an alternative, decentral solutions for users with high-temperature needs (e.g., for processes) were suggested. Also, planned building retrofits are a window of opportunity, if the utility is aware of them. Third, the feasibility and effectiveness of temperature reduction measures depends on building retrofit status and technical configuration of the grid (e.g., pressure, pipe statics, substations, etc.). Costs and benefits are therefore situation-specific and must be estimated through a detailed techno-economic analysis. A relevant engineering challenge is to devise intelligent, context-sensitive measures to maximize the utilization of production and transport capacity (e.g., integration of users with a low-temperature demand profile into the return flow). Respondents described the problem as socio-technical, rather than purely technological: First, the distribution of costs and responsibilities between utilities, building owners and HVAC companies is an open question. Limited impact on energy demand is expected (especially in retrofitted buildings). Therefore, building owners have little incentive to implement temperature-reduction measures. Utilities should seek win-win solutions to incentivize change or increase acceptance of such measures. Second, efficient energy use is not always monitored or enforced by the utility. Indeed, given human resource constraints, there is not enough pressure to act if the heat generation or transport capacity is not at its limit. A final challenge arises from liability issues if the substation is owned by the customer.
Sectoral strategic documents see a large potential to integrate ambient or low-grade excess heat (Sres and Nussbaumer, 2014), although development has been slow (Hangartner and Hurni, 2022). These energy sources are suitable for low-temperature grids, where the fluid is transported at near-ambient temperature to neighborhood- or building-level heat pumps. Several interviewees with different roles had direct experience with low-temperature grids, providing a use-informed perspective.
The decentral heat pumps may be owned by (and fall under the responsibility of) either the utility or the customer. In the former case, cost-efficient and reliable operation requires specific skills and/or advanced automation solutions. Therefore, the construction of such grids entails technical risks if the utility does not possess prior experience. The latter case is potentially less interesting economically, since it consists only of primary energy delivery (of little economic value) rather than a product. For utilities, this has been described as “better than nothing” if no other option is feasible. On the demand side, respondents reported a lack of understanding of technical aspects by builders or building owners, which may lead to a low acceptance of specific energy efficiency measures (e.g., temperature-dependent tariffs).
A further advantage of low-temperature grids are synergies between heating and cooling. However, since the future cooling demand is uncertain, the risks of developing district cooling infrastructure were pointed out. On the demand-side, a growing interest for solutions combining heating and cooling (especially free-cooling) for building complexes was noted. However, the technical potential is often overestimated by potential customers.
Swiss utilities are required by law to implement bidirectional communication-enabled meters (“smart meters”) to monitor electricity use. Although no such requirement exists for thermal grids, the use of meters enabling data transmission is seen as standard in new grids. In existing grids, however, this is still rare. Nevertheless, various potential benefits were highlighted: First, there is a high (and considerably low-cost) potential for smart metering to locate inefficient substations, allowing utilities to determine the most impactful interventions for temperature reduction in the short-term. Second, high-resolution measurements enable optimization on the supply and demand sides, through energy saving, better portfolio management (i.e., temporal prioritization of different heat sources) and grid decongestion. Based on experiences in building energy optimization, an energy saving potential of 10–15% was cited. The optimization potential is particularly high in grids that are not yet operating at their full capacity (i.e., connections of users are ongoing, typically in the first 15 years), as most hydraulic problems occur in this period. Third, energy use data can be used to create additional value for end users while promoting energy efficiency, e.g., through apps enabling monitoring. However, various barriers to the diffusion of smart meters were cited: For utilities, there is a potential trade-off, as optimization decreases energy sales (c.p.). On the customer side, a perceived loss of control may be rejected (in the respondents’ experience, Swiss customers highly value autonomy). Also, the value of smart metering may not be fully realized due to privacy constraints (e.g., if data must be stored in pseudo-anonymized form, as is the case in the water sector, targeted interventions in case of malfunction or inefficiency are not possible). Finally, high transaction costs are expected in existing grids (e.g., renegotiation of contracts, redefinition of technical connection conditions). Due to high costs (including transaction costs), uncertain benefits and little pressure to act (current heat generation and transport capacities are still sufficient), some (especially smaller) utilities are reluctant to invest in this area.
A central challenge of the Swiss energy system is a potential energy shortage in the winter half-year. For this reason, seasonal thermal energy storage (TES) is a salient topic (Duran Adroher et al., 2022). However, utilities and municipalities expressed caution regarding the integration of long-term storage into existing, high-temperature grids, citing high investment costs and a lack of space. Another barrier was the coordination between heat generation and distribution in cases where these are operated by different organizations. Some of the participating organizations operated long-term TES at low-temperatures (20°C–30°C) in boreholes or aquifers. This was described as a promising option for further development, while noting that applications are often limited to grids serving energy-efficient buildings. As an alternative to TES, some respondents expect synthetic gases to play an important role in the future, although opinions diverged on the capacity to produce synthetic gases in Switzerland.
As thermal grids are being rolled-out, some respondents expect a coupling of grids into larger networks, as seen, e.g., in Denmark. Some developments in this direction are at an advanced conceptual stage, including the integration of various heat sources. This also includes the coupling of currently independent, neighborhood-scale grids with larger networks, which presents challenges (regarding the compatibility of technical installations), but also opportunities (energy-efficient neighborhood grids as potential consumers fed by the return flow of high-temperature grids). In one case, a planned supra-municipal grid was canceled due to resistance in one municipality. This suggests greater risks of project failure and/or coordination costs for more complex grids.
Respondents’ feedback to the interactive planning tool TESSA (cf. Section 2.2) was coded with a separate, two-dimensional scheme (Table 4): categories correspond to the tool’s features and the level at which value creation is expected (cf. Section 2.2). Feedback addressed three distinct features: data; modeling and optimization capabilities; and the interactive graphic interface. The underlying data, particularly building-level heat demand estimations, attracted much interest. Indeed, while this information is crucial, existing datasets (such as the federal registry of buildings and dwellings, or publicly available hectare-level energy demand) were described as difficult to obtain, too coarse or not reliable or up-to-date enough. Even for an initial assessment, data must be acquired from municipalities or from the customers themselves, which is time-consuming. TESSA’s data collection therefore enables quick initial assessments, allowing developers to decide whether more extensive analyses are worthwhile. Besides building characteristics, the integration with further datasets, such as localized energy sources, local meteorological time series and technical or techno-economic parameter values, was seen as valuable, as it reduces search costs and the risk of mistakes in data transfer. Some respondents mentioned further datasets that would improve the tool’s value, such as infrastructure geometries or land ownership. It was pointed out that an estimate or model of customers’ probability to connect would enable a more realistic assessment of the grid’s returns over time. Besides development, the tool’s data collection was also seen as valuable for strategic planning by municipal authorities, as development areas with a high GHG emission reduction potential can be prioritized.
Table 4. Value creation potential of the interactive planning tool TESSA as perceived by respondents.
The tool’s simulation and optimization capabilities were described as automating the work of planners, with potential time savings. Since planners deal with diverse issues, many of which are out of the scope of technical models, the tool is seen as an aid rather than a replacement. Different use cases were envisioned: while some respondents only saw applications at the (pre-)feasibility stage, others imagined an iterative process where the tool is used to optimize the grid design based on experiences in the field. For a robust assessment of viability, one respondent suggested a sensitivity analysis feature, where the effect of design parameters and uncertain conditions (e.g., customers’ willingness to connect) can be assessed. It was also noted that applying the model enabled learning about a location’s potential for thermal grids. This contributes to the local knowledge base (cf. Section 3.1.3) and facilitates coordination between diverse actors, such as utilities, engineering firms and various departments of municipal authorities. At the ecosystem level, this was noted to contribute to the professionalization of the industry (cf. Section 3.1.3).
The tool’s potential as a boundary object3 was strengthened by the interactive interface and the model’s execution speed. According to several respondents, this potential extends beyond technical planning and enables communication with further stakeholders. For municipal authorities, communicating the economically viable potential for thermal grids, rather than just technical potentials, makes the idea of thermal grids more tangible to citizens and may foster social acceptance. For utilities, the interface was seen as a means to build trust with investors, executive boards or public decision-making bodies. Finally, it was mentioned that the tool may be valuable to customers: by increasing transparency on a grid’s technical characteristics, cost structure and environmental impact, it could help customers make a more informed choice when deciding whether to connect.
Besides these potentials, respondents have noted potential interfaces with existing tools for technical planning, asset management and customer engagement. The value of a new tool was said to depend greatly on its interoperability with existing solutions: “If you build your tool, I think the connection to other tools, the interfaces would be important. Build open systems, not proprietary systems. I think that’s over. Nowadays the value lies in the data and its interpretation but not in the software itself.” Nevertheless, various interviewees report using proprietary solutions themselves. These observations suggest that, if the trend toward open systems continues, there is further potential for complementary tools along the decision-making process.
We identify various problem situations to decompose the messy problem of thermal grid development into smaller, less complex problems (Metlay and Sarewitz, 2012). Whereas the value network description presents the perspective of various actor groups, this section focuses on the relationships between them. For each problem situation, we summarize solutions that were either suggested by interviewees or proposed previously (Table 1).
P1: Municipalities lack the resources to adequately manage the implementation of their energy and climate strategies.
As described in Section 3.1.2, the relationship between municipalities and utilities is partly a principal-agent situation (Eisenhardt, 1989), where the municipality (principal) tasks the utility (agent) with the development of thermal grids as a means to fulfil public policy goals. Interests and activities are not always fully aligned, e.g., regarding the choice of energy carriers and grid type, feasibility of implementation timelines, the nature of contracts, or the suitability of specific policy proposals. Another principal-agent situation exists between municipalities and energy planners in the domain of strategic energy planning. The workforce shortage leads to quality issues in spatial energy planning, so that important energy potentials are sometimes omitted, potential service areas are defined with little regard for feasibility or cost-efficiency, or proposals advancing particular interests are taken up uncritically.
Municipalities’ difficulties in specifying and enforcing the “contract”4 with utilities and planners can be ascribed to a lack of human resources and specific knowledge (this issue is most prevalent in smaller and mid-sized towns, but some aspects were observed in larger cities). Indeed, municipal employees responsible for energy matters deal with diverse issues (sometimes, their tasks include non-energy related issues), preventing them from gaining specific expertise. The same applies to policymakers, dealing with an even broader range of issues. This also applies to municipal authorities’ regulatory role: The permitting process was cited as a major barrier to the timely development of thermal grids, impacting the timeline or technical configuration of the project. On one hand, some respondents mentioned a defensive approach (due, e.g., to concerns about the administration’s workload during construction) or a general distrust toward using public goods (e.g., energy sources, space) for private activities. On the other hand, the difficulty of the permitting process was ascribed to limited knowledge of the matter by authorities, with limited insight into how such processes are handled elsewhere. Generally, these difficulties will likely increase with the construction of networks spanning the territory of several municipalities, which some respondents expect to take place more frequently.
Various ways to approach this issue were suggested. One respondent stated that cantonal (state) governments, who are by law responsible for reducing GHG emissions from buildings, should be more active. A concrete example would be an independent quality control of municipal energy plans by cantonal authorities. However, one respondent from a municipal authority found the assistance from cantonal authorities particularly helpful. This suggests heterogeneity in the level and quality of assistance by cantonal governments. Another approach is the dissemination of insights from research and practice in a form that is easily accessible to municipal employees with limited time and expertise, e.g., guidelines and checklists. Targeted continued education offers and experience-sharing platforms were also mentioned, although the diversity of tasks and background of municipal employees and policymakers and their limited time represents a challenge.
P2: The modernization of thermal grids and integration of low-carbon heat sources entails high transaction costs.
The need for coordination to develop new thermal grids is a well-known problem, so that various facilitating measures have been suggested (Table 1). This study shows that the integration of low-carbon energy sources, which often presupposes modernization of existing infrastructure, also entails great coordination efforts.
The various, sometimes interdependent solutions to increase energy efficiency, reduce temperatures, enable the integration of renewable heat sources and avoid grid congestion include steps outside the control of utilities and therefore require coordination. In particular, the choice of building owners to conduct energy retrofits impacts the feasibility of temperature reduction measures. Also, especially large-scale consumers may possess flexible loads that could be controlled in a demand-side scheme. Further, building professionals (HVAC installers and planners) should be engaged to implement efficient solutions on the secondary side, including substations. These options entail various transaction costs: the windows of opportunity for modernization measures, arising from building retrofits, are not always known early enough. Also, it is challenging to find schemes that incentivize building owners and building professionals to collaborate. Finally, demand-side interventions often require the renegotiation or reformulation of contracts and technical connection conditions.
Excess heat providers (industrial companies, operators of data centers, power plants, etc.) are another actor category whose integration entails high transaction costs. The distribution of responsibilities and the value capture mechanisms are a matter of negotiation. Various barriers to closer integration have been identified: potential excess heat providers may not be aware of such a possibility; they may face high uncertainty regarding technical, economic or organizational aspects; or they may show little interest in an activity unrelated to their core business. In addition, perception of collaboration options (e.g., expected benefits, perceived risks or general interest in the topic) varies greatly within a single organization, so that success depends on the addressee within an organization.
This situation is problematic: Communication and coordination are often performed by engineering companies or utilities, who do not see this as their core business and would welcome more professional communication and coordination. Also, these tasks bind the already scarce resources of technical experts. Finally, although the value of modernization measures is well-perceived by utilities (see Section 3.1.4), such measures are often not undertaken due to lacking capacities (cf. P3). Nevertheless, these problems seem to be under-addressed in existing applied research (Table 1) and the secondary literature studied (e.g., planning documents).
P3: The implementation of thermal grids is subject to delays and quality risks, threatening the timely achievement of decarbonization goals.
A skill shortage was identified as a major issue by nearly all respondents. In various cities, while respondents were optimistic about policy and economic frame conditions, workforce shortage was a major concern for the timely realization of decarbonization strategies. Construction is already prone to delays (e.g., due to permitting issues, objections) and coordination of the construction schedule with civil engineering is already crucial. Additional delays and uncertainty will therefore compound these challenges. In addition, supply-chain delays also affect project timelines, although these effects were expected to be more transient. The workforce shortage leads not only to delays in project execution, but also to quality issues in strategic and technical planning. This increases transaction costs: contracts (e.g., between planners and utilities) have become more complex, with clauses on delays and quality being added by both sides. Also, the schedule becomes less predictable, which dissuades potential customers (see P4). Finally, windows of opportunity (e.g., the heating system replacement of large buildings) cannot always be seized due to lacking capacities. As a result, reaching public decarbonization goals becomes more challenging.
Continued education programs (Table 1) were assessed positively, although the number of participants was still deemed too low. This issue was reported to be present across the whole energy sector and linked to long-term issues, such as a low attractivity of technical careers or of employment in the municipal administration. Some approaches to manage this scarcity were discussed: capacity building in specialized communication and coordination reduces the workload of technical experts who can then focus on their core competencies. Also, some efficiency potential was ascribed to automation, e.g., in technical planning (cf. Section 3.1.5) or construction. Nevertheless, respondents stressed that these tools would be an aid rather than a replacement of skilled workers. Also, as in P1, practically oriented knowledge transfer tools such as guidelines and checklists were seen as useful to increase efficiency and quality, e.g., when used by workers whose technical background is in a different domain.
P4: Customers face uncertainty on available options, as well as on the systemic impacts of their decisions.
Lock-in effects due to the adoption of individual heating solutions were a concern for many respondents. A major issue is the uncertainty related to the development schedule: where a grid is planned, building owners often do not know when a connection will be possible. If too many building owners choose a decentral solution, the economic viability of a thermal grid becomes uncertain. To address this problem, there are municipal initiatives to publish the planned service areas of thermal grids as geodata, and a project in this direction is ongoing at federal level. However, the actual development timeline is highly uncertain (cf. P3), so that these data are often published without an expected date of completion. The information gap for customers is therefore not fully addressed.
Various approaches for customer acquisition under uncertainty have been suggested (Table 1), such as providing adequate temporary solutions for heating replacement, or involving point-of-sale actors (e.g., real estate managers, installers or planners) in marketing. These approaches were implemented in various ways: for example, municipal administrations grant exceptional permits for the temporary installation of fossil-based systems, or utilities take over existing heating systems in an ESCO scheme where grid construction is planned. Building professionals were approached differently in different locations: some utilities and municipalities actively coordinated with them and devised a fair repartition of tasks (cf. Table 3), sometimes due to the action of policymakers concerned with effects on local employment. In other cases, no such relationship was established. One utility reported a conflict of interest with building energy planners (which were interested in planning more complex systems, such as building-level heat pumps), which must be actively managed, e.g., by mandating a second opinion from another firm. These examples show that involving point-of-sale actors for the information of customers involves additional coordination effort (cf. P2).
Perceived environmental friendliness and regional embeddedness are part of the customer value proposition of thermal grids. However, this aspect may not be perceived clearly by building owners. Also, most customers are not able to assess the systemic impacts of their decisions, so that it was suggested that contributing to regional energy efficiency may be another, under-exploited motivation for customers. Respondents suggested that these aspects should be communicated more explicitly, e.g., through independent consulting, possibly on a public mandate. As noted in Section 3.1.5, interactive visualizations were seen as a useful tool to communicate the systemic and environmental benefits of thermal grids.
This section contextualizes the case study and connects the problem situations to approaches presented abroad. As seen in Section 1.1, thermal grids have so far played a marginal role in Switzerland, although they have long been present locally. However, they are now seen as an important instrument of decarbonization—a development that has been accompanied by capacity and capability building, increased professionalism and the development of a common knowledge base. Therefore, Switzerland can be compared in some respects to countries with a long history of district heating, whereas many aspects are more comparable to “learning countries” (Bush et al., 2016). While the current state and necessary transitions varied greatly between countries (Paardekooper et al., 2022), this study shows that heterogeneity must also be considered between municipalities.
As reflected in the focus of applied research (Table 1), the roll-out of thermal grids is an important part of the low-carbon heating transition. Therefore, some of the developments and challenges are seen in other “learning countries.” For example, the important role of municipalities and the capacity limitations they face was also noted in the UK (Bush et al., 2016). Strategic planning as a success factor was likewise a common theme. A contribution of this study is the observation that planning is more successful when embedded in a lasting process, which requires a long-term commitment of the municipality and energy provider, the capacity to build realistic scenarios and adequate geodata on energy demand. By contrast, a “one-off” approach to spatial energy planning, as often practiced, risks a low acceptance and bindingness of the energy plan, in addition to the quality issues raised (cf. Section 3.1.2.3). Relatedly, P1 and P2 point to a lack of what Ayrault (2022) calls “collective expertise,” i.e., the capacity of diverse actors to collectively discuss the value of thermal grids and to design policies and governance arrangements accordingly. Such shortcomings were observed by Ayrault (2022) in France, hindering the sustainable valuation of thermal grids beyond decarbonization. Such observations are partly confirmed by this study, as the public value of thermal grids was usually defined in terms of decarbonization only. However, the customer perspective suggests a more differentiated perception, with valorization of regional resources and energy independency seen as an added value. This contrasts with observations that customers take infrastructure and heat delivery for granted (Paiho and Saastamoinen, 2018)—except in the case of low-temperature grids, where this was seen as an issue (Section 3.1.4.2).
In other respects, the challenges identified here have been encountered in countries where thermal grids are more mature. This concerns developments beyond the roll-out of high-temperature grids, such as the integration of low-temperature energy sources, and modernization of existing infrastructure. As seen in P2, such changes entail high coordination costs, which prohibits utilities from pursuing them. In contrast to P1, this is mostly relevant in cities where thermal grids are already present for a long time. Recent research has investigated technical, but also organizational and customer-centric aspects of such innovations in the framework of pilot projects (Peltokorpi et al., 2019; Ma et al., 2020). Indeed, the challenges of such modernizations are both technical and organizational (Lygnerud, 2018; Paiho and Saastamoinen, 2018; Peltokorpi et al., 2019) and technical and business development should be undertaken together (Lygnerud et al., 2023). This study confirms that transitioning to low-temperature grids is challenging (Lygnerud, 2019), with the build-up of operating skills as a new key resource as a substantial challenge.
This section identifies four development areas to address the problem situations identified above, by combining the solutions suggested above (Section 3.2) with insights from the international literature.
D1: Empower and support municipalities.
As suggested above, municipalities can be supported by authorities at higher levels, which have more resources and expertise. Also, local expertise can be strengthened through adequate instruments (Ayrault, 2022), such as guidelines or checklists, or by advanced education offers for practitioners. Since the challenges of thermal grids are highly situation-specific (Paardekooper et al., 2022; Weisskopf et al., 2022), we suggest that multiple case studies will be more useful for experience sharing and knowledge transfer than attempts to formulate generic policy measures—thus applying the insights of Flyvbjerg (2006) to practice at local level.
D2: Manage the scarcity of qualified workforce.
This study is in line with Briggs et al. (2022), who suggested that skill shortages are under-appreciated in energy research and strategy, although such issues have been reported in various countries and energy subsectors (Vasseur et al., 2013; Lucas et al., 2018). While targeted education offers help alleviate the problem, this skill shortage stems from complex causes for which a quick solution is unlikely. Therefore, we propose that it is essential to find ways to manage this lasting problem. As mentioned under P3, there is scope for non-technical offerings such as project development, coordination and marketing: adequate offerings would relieve technical experts from tasks which are not their core competency. Also, automation of some tasks in planning and construction may increase process efficiency, although it was stressed that such tools are a complement to expert knowledge rather than a replacement. Finally, we recommend that possible workforce-related delays are explicitly addressed in long-term planning by municipalities and utilities. To this end, decision-support tools that make explicit the causal relationships between staffing and performance may be useful (Bianchi and Montemaggiore, 2008).
D3: Leverage digital technology for the planning, realization and operation of thermal grids.
While capacity limitations are hinder improved planning processes, digital tools integrating various datasets and making them easily available facilitate strategic energy planning (cf. Section 3.1.5). Relatedly, interactive planning tools may help build “collective expertise” (see Section 4.1). This is in line with Bertelsen et al. (2021), who identified energy plans as boundary objects that enable collective sensemaking and thus shape infrastructure development decisions. This also means that the value of such tools extends beyond decision support and enable communication with investors, customers, citizens, etc. (cf. Section 3.1.5). While the focus was on technical planning tools, we note successful applications of digital technologies in other steps, such as a multi-sided platform using geodata to connect heterogeneous users and support strategic decisions, or a mobile app enabling users to monitor their heat consumption (see also Volkova et al., 2019). These results point to the potential of digital tools to address systemic challenges such as P3 or P4. To realize this potential, interoperability is a key success factor.
D4: Pilot technical and organizational innovations together.
As seen in P2, grid modernization and involving external heat providers entail high transaction costs. Building upon the observation that technical and organizational development should be coupled (Lygnerud et al., 2023), we suggest that pilot projects cover both technical innovations and the organizational arrangements to operate them. For example, Peltokorpi et al. (2019) applied an action research approach to formulate generic design principles for an economically sustainable operation of demand-side management in thermal grids based on a case study. Such research can provide blueprints for further implementations, thus decreasing search costs. Other organizational innovations may include matchmaking organizations intermediating between grid operators and external heat providers (see Zaoual and Lecocq, 2018 for some examples from industrial ecology) or arrangements between utilities, building professionals and building owners to enable temperature reduction. Innovative collaboration forms between researchers from various disciplines and practitioners, such as living labs (Voytenko et al., 2016), can be valuable for such pilot studies. Indeed, this study was conducted in a project (SWEET-DeCarbCH) that enables such collaboration by connecting technical and non-technical researchers with practitioners from utilities, municipalities and engineering firms.
This study provides a description of the Swiss thermal grid ecosystem, a breakdown of the messy problem of thermal grid development into a structured problem identification, aligned with a portfolio of solutions to address the identified problem situations. The ecosystem has been in a phase of rapid growth and professionalization in the last 10–15 years. While earlier developments were primarily driven by opportunity, thermal grids are now seen as a means to reach public policy goals, leading to policy support and high social acceptance. This development is reflected in a rapid increase in the number of grids, but also in capability and capacity building and greater quality standards. This transformation has been supported by a resource pool (e.g., knowledge base, capacities and capabilities, networks or decision-support tools), built partly through the value network’s activities and partly through purposeful resource orchestration by associations, authorities, utilities and other actors. While policy developments at federal, cantonal and municipal levels, as well as—particularly in the past year—energy price fluctuations and changes in energy consumers’ priorities and perceptions led to rapidly increasing customer demand, the further development of thermal grids is limited by supply-side constraints, especially a skills shortage. Besides the development of new thermal grids, this study also inquired on the perception by ecosystem actors of modernization options, e.g., through supply and/or return temperature reduction, integration of new renewable or excess heat sources, integration of large-scale thermal energy storage, synergies between heating and cooling, the digitalization, as well as motivational tariffs. Despite their high potential, such measures are often not on the immediate agenda. This is due to the complexity of actor coordination, generating high transaction costs. Also, the expected benefits of such measures are currently not seen as worth the risks associated with them—financial, but also in terms of loss of trust and acceptance.
Furthermore, we have identified four problem situations, themselves decomposable into specific misalignments of goals or activities (arising from incomplete and different appreciation of potentials, risks and consequences of measures by different actors; information asymmetry between municipalities, utilities and planning companies on feasible and desirable solutions; lack of awareness on technical and organizational options; different prioritization of issues; differential impacts of workforce shortage on actors’ capabilities) and specific instances of resource inadequacy (such as the lack of common resources, e.g., qualified workforce or underused potentials to leverage of available resources, e.g., planning and operational data).
Solutions to address these problem situations are diverse (actor and resource orchestration, business model innovation). Thermal grids are often only moderately attractive economically, with long payback times and slim profits. Furthermore, there is a limited number of organizations able to build and operate thermal grids, with little competition. Therefore, market mechanisms are limited to yield solutions for the problem situations described here. On the other hand, thermal grids generate public value, as they align with public decarbonization goals. Therefore, we see an active role of public authorities at federal, cantonal and municipal levels in initiating such measures, in accordance with the distribution of competences, but also of capabilities and capacities. While applied research on thermal grids in Switzerland has so far focused on public policy and regulation (see Table 1), we complement this research by adopting an (inter-)organizational perspective. While municipal authorities may initiate local measures such as living labs, they may benefit from additional resources (e.g., guidelines for the practical implementation of energy strategies or an efficient permitting process) to be developed nationally, and/or from operational support from higher-level authorities with larger capacities (e.g., for the quality control of spatial energy plans). There is, however, scope for active orchestration by actors other than authorities. For example, devising appropriate value capture arrangements to align the incentives of utilities, HVAC installers and building owners may be a task for utilities, if they possess the necessary capabilities. Also, software solutions such as decision-support or advanced project management tools may be offered by private developers. Furthermore, some solutions involve the creation and operation of (strategic) platforms. These platforms may take different formats (e.g., digital or organizational) and different business models (e.g., for-profit or community-based). Determining the appropriate model is left as an open question, as these are likely situation-specific.
The datasets presented in this article are not readily available because participants were promised anonymity and confidentiality for the interviews. Requests to access the datasets should be directed to bWF0dGhpYXMuc3BlaWNoQHpoYXcuY2g=.
Ethical review and approval was not required for the study on human participants in accordance with the local legislation and institutional requirements. Written informed consent from the (patients/participants OR patients/participants legal guardian/next of kin) was not required to participate in this study in accordance with the national legislation and the institutional requirements.
MS: Conceptualization, Data curation, Formal analysis, Funding acquisition, Investigation, Methodology, Visualization, Writing – original draft, Writing – review & editing. JC: Funding acquisition, Investigation, Methodology, Writing – review & editing. SU-B: Conceptualization, Funding acquisition, Methodology, Project administration, Validation, Writing – review & editing.
The author(s) declare financial support was received for the research, authorship, and/or publication of this article. The research published in this report was carried out with the support of the Swiss Federal Offce of Energy SFOE as part of the SWiss Energy research for the Energy Transition (SWEET) project DeCarbCH. The authors would like to thank SFOE for its financial support. The contents and conclusions of this report are the sole responsibility of the authors.
The authors declare that the research was conducted in the absence of any commercial or financial relationships that could be construed as a potential conflict of interest.
All claims expressed in this article are solely those of the authors and do not necessarily represent those of their affiliated organizations, or those of the publisher, the editors and the reviewers. Any product that may be evaluated in this article, or claim that may be made by its manufacturer, is not guaranteed or endorsed by the publisher.
1. ^These logics represent so-called ideal types and do not describe the thinking of individual people or organizations. Rather, actors’ mindset and behavior are conceptualized as being influenced, to a various degree, by several logics.
2. ^In a federal state such as Switzerland, the concrete organizational structure of municipal administrations is highly heterogeneous. A detailed characterization of this diversity would be beyond the scope of this work. For this reason, the description in this paragraph is somewhat generalized and simplified.
3. ^Following Taylor et al. (2014), we define a boundary object as an object that facilitates communication and decision-making across actors of diverse social and professional backgrounds.
4. ^In the principal-agent framework, the contract is a metaphor for the principal’s expectations and does not necessarily refer to a formal contract. Indeed, some situations were encountered where the municipality entered contractual agreements that were not in line with its stated energy policy—i.e. it was not able to enforce its metaphorical “contract.”
Abegg, A., and Musliu, N. (2022). Die Fernwärmeversorgung—eine rechtliche Einordnung. Sui Generis 43:203. doi: 10.21257/sg.203
Autio, E. (2021). Orchestrating ecosystems: a multi-layered framework. Innovations 24, 96–109. doi: 10.1080/14479338.2021.1919120
Ayrault, J. (2022). Co-creating valuations and valuating co-creation in sustainable public infrastructures: the case of district heating (Université Paris Sciences et Lettres). Available at: https://pastel.archives-ouvertes.fr/tel-04049991
Berger, M., and Worlitschek, J. (2019). The link between climate and thermal energy demand on national level: a case study on Switzerland. Energ. Buildings 202:109372. doi: 10.1016/j.enbuild.2019.109372
Bertelsen, N., Caussarieu, M., Petersen, U. R., and Karnøe, P. (2021). Energy plans in practice: the making of thermal energy storage in urban Denmark. Energy Res. Soc. Sci. 79:102178. doi: 10.1016/j.erss.2021.102178
Bhadbhade, N., Yilmaz, S., Zuberi, J. S., Eichhammer, W., and Patel, M. K. (2020). The evolution of energy efficiency in Switzerland in the period 2000–2016. Energy 191:116526. doi: 10.1016/j.energy.2019.116526
Bianchi, C., and Montemaggiore, G. B. (2008). Enhancing strategy design and planning in public utilities through “dynamic” balanced scorecards: insights from a project in a city water company. Syst. Dyn. Rev. 24, 175–213. doi: 10.1002/sdr.395
Bolton, R., and Hannon, M. (2016). Governing sustainability transitions through business model innovation: towards a systems understanding. Res. Policy 45, 1731–1742. doi: 10.1016/j.respol.2016.05.003
Briggs, C., Atherton, A., Gill, J., Langdon, R., Rutovitz, J., and Nagrath, K. (2022). Building a ‘fair and fast’ energy transition? Renewable energy employment, skill shortages and social licence in regional areas. Renew Sustain Energy Transit 2:100039. doi: 10.1016/j.rset.2022.100039
Busch, J., Roelich, K., Bale, C. S. E., and Knoeri, C. (2017). Scaling up local energy infrastructure; an agent-based model of the emergence of district heating networks. Energy Policy 100, 170–180. doi: 10.1016/j.enpol.2016.10.011
Bush, R. E., and Bale, C. S. E. (2019). Energy planning tools for low carbon transitions: an example of a multicriteria spatial planning tool for district heating. J. Environ. Plan. Manag. 62, 2186–2209. doi: 10.1080/09640568.2018.1536605
Bush, R. E., Bale, C. S. E., and Taylor, P. G. (2016). Realising local government visions for developing district heating: experiences from a learning country. Energy Policy 98, 84–96. doi: 10.1016/j.enpol.2016.08.013
Chambers, J. (2020). Computationally scalable geospatial network and routing analysis through multi-level spatial clustering. MethodsX 7:101072. doi: 10.1016/j.mex.2020.101072
Chambers, J., Cozza, S., and Patel, M. (2023). The GENEAP project: digitalising and automating planning of district heating and cooling. J. Phys. Conf. Ser. 2600:082003. doi: 10.1088/1742-6596/2600/8/082003
Chambers, J., Rittman-Frank, M., and Patel, M. (2021). Presentation of new geospatial datasets for renewable thermal energy systems modelling in Switzerland. J. Phys. Conf. Ser. 2042:012003. doi: 10.1088/1742-6596/2042/1/012003
Connolly, D., Lund, H., Mathiesen, B. V., Werner, S., Möller, B., Persson, U., et al. (2014). Heat roadmap Europe: combining district heating with heat savings to decarbonise the EU energy system. Energy Policy 65, 475–489. doi: 10.1016/j.enpol.2013.10.035
Duran Adroher, N., Lüchinger, R., Villasmil, W., and Worlitschek, J. (2022). Saisonale Wärmespeicher. Aqua & Gas. Available at: https://www.aquaetgas.ch/energie/fernwärme/20221026_ag11_saisonale-wärmespeicher/
Ecoplan. (2021). Ergebnisse einer Umfrage zur heutigen und künftigen Finanzierung des Fernwärmeausbaus. Available at: https://www.fernwaerme-schweiz.ch/fernwaerme-deutsch/allgemeine-Fragen/FWUF_sb_2021_07_05d.pdf
Eisenhardt, K. M. (1989). Agency theory: an assessment and review. Acad. Manage. Rev. 14:57. doi: 10.2307/258191
Federal Council. (2021). Switzerland’s Long-Term Climate Strategy. Available at: https://www.newsd.admin.ch/newsd/message/attachments/65879.pdf
Flyvbjerg, B. (2006). Five misunderstandings about case-study research. Qual. Inq. 12, 219–245. doi: 10.1177/1077800405284363
Gabillet, P. (2015). Energy supply and urban planning projects: Analysing tensions around district heating provision in a French eco-district. Energy Policy 78, 189–197. doi: 10.1016/j.enpol.2014.11.006
Geels, F. W. (2004). From sectoral systems of innovation to socio-technical systems. Res. Policy 33, 897–920. doi: 10.1016/j.respol.2004.01.015
Hangartner, D., and Hurni, A. (2022). Liste “Thermische Netze”—Auswertungsbericht 2021. Available at: https://pubdb.bfe.admin.ch/de/publication/download/10878
Heldeweg, M. A., Sanders, M. P. T., and Brunnekreef, A. V. (2017). Legal governance of smart heat infrastructure development under modes of liberalization; how to analyze and overcome deadlocks in heat projects. Energy Sustain. Soc. 7:24. doi: 10.1186/s13705-017-0127-y
Kanda, W., Geissdoerfer, M., and Hjelm, O. (2021). From circular business models to circular business ecosystems. Bus. Strateg. Environ. 30, 2814–2829. doi: 10.1002/bse.2895
Leoni, P., Geyer, R., and Schmidt, R.-R. (2020). Developing innovative business models for reducing return temperatures in district heating systems: approach and first results. Energy 195:116963. doi: 10.1016/j.energy.2020.116963
Leviäkangas, P., and Öörni, R. (2020). From business models to value networks and business ecosystems—what does it mean for the economics and governance of the transport system? Util. Policy 64:101046. doi: 10.1016/j.jup.2020.101046
Lingens, B., Huber, F., and Gassmann, O. (2022). Loner or team player: how firms allocate orchestrator tasks amongst ecosystem actors. Eur. Manag. J. 40, 559–571. doi: 10.1016/j.emj.2021.09.001
Lucas, H., Pinnington, S., and Cabeza, L. F. (2018). Education and training gaps in the renewable energy sector. Sol. Energy 173, 449–455. doi: 10.1016/j.solener.2018.07.061
Lygnerud, K. (2018). Challenges for business change in district heating. Energy Sustain. Soc. 8:20. doi: 10.1186/s13705-018-0161-4
Lygnerud, K. (2019). Business model changes in district heating: the impact of the technology shift from the third to the fourth generation. Energies 12:1778. doi: 10.3390/en12091778
Lygnerud, K., Popovic, T., Schultze, S., and Støchkel, H. K. (2023). District heating in the future—thoughts on the business model. Energy 278:127714. doi: 10.1016/j.energy.2023.127714
Ma, Z., Knotzer, A., Billanes, J. D., and Jørgensen, B. N. (2020). A literature review of energy flexibility in district heating with a survey of the stakeholders’ participation. Renew. Sustain. Energy Rev. 123:109750. doi: 10.1016/j.rser.2020.109750
Ma, Y., Rong, K., Mangalagiu, D., Thornton, T. F., and Zhu, D. (2018). Co-evolution between urban sustainability and business ecosystem innovation: evidence from the sharing mobility sector in Shanghai. J. Clean. Prod. 188, 942–953. doi: 10.1016/j.jclepro.2018.03.323
Mayring, P. (2019). Qualitative content analysis: demarcation, varieties, developments. Forum Qual Sozialforschung Forum Qual Soc Res 20:3343. doi: 10.17169/fqs-20.3.3343
Metlay, D., and Sarewitz, D. (2012). Decision strategies for addressing complex, “messy” problems. The Bridge, 42. Available at: https://www.nae.edu/62558/Decision-Strategies-for-Addressing-Complex-Messy-Problems
Moore, M. H. (1995). Creating public value. Strategic Management in Government. Cambridge & London: Harvard University Press.
Nussbaumer, T., Thalmann, S., Jenni, A., and Ködel, J. (2018). Planungshandbuch Fernwärme (1.2). Available at: https://www.verenum.ch/Dokumente/PLH-FW_V1.2.pdf
Paardekooper, S., Lund, H., Thellufsen, J. Z., Bertelsen, N., and Mathiesen, B. V. (2022). Heat roadmap Europe: strategic heating transition typology as a basis for policy recommendations. Energ. Effic. 15:32. doi: 10.1007/s12053-022-10030-3
Paiho, S., and Saastamoinen, H. (2018). How to develop district heating in Finland? Energy Policy 122, 668–676. doi: 10.1016/j.enpol.2018.08.025
Peltokorpi, A., Talmar, M., Castrén, K., and Holmström, J. (2019). Designing an organizational system for economically sustainable demand-side management in district heating and cooling. J. Clean. Prod. 219, 433–442. doi: 10.1016/j.jclepro.2019.02.106
Prognos/Infras/TEP Energy/Ecoplan. (2021). Energieperspektiven 2050+—Kurzbericht. Available at: https://pubdb.bfe.admin.ch/de/publication/download/10323
Reda, F., Ruggiero, S., Auvinen, K., and Temmes, A. (2021). Towards low-carbon district heating: investigating the socio-technical challenges of the urban energy transition. Smart Energy 4:100054. doi: 10.1016/j.segy.2021.100054
Sandoff, A., and Williamsson, J. (2016). “Business models for district heating” in Advanced District heating and cooling (DHC) systems, Ed. R. Wiltshire (Sawston, Cambridge, UK: Woodhead Publishing) 293–317.
Shi, X., Luo, Y., Hou, H., Rong, K., and Shi, Y. (2022). Exploring the process of business ecosystem emergence from value chains: insights from the Chinese Mobile phone industry. Manag. Organ. Rev. 18, 4–42. doi: 10.1017/mor.2021.39
Späth, P., and Rohracher, H. (2015). Conflicting strategies towards sustainable heating at an urban junction of heat infrastructure and building standards. Energy Policy 78, 273–280. doi: 10.1016/j.enpol.2014.12.019
Speich, M., and Ulli-Beer, S. (2023). Applying an ecosystem lens to low-carbon energy transitions: a conceptual framework. J. Clean. Prod. 398:136429. doi: 10.1016/j.jclepro.2023.136429
Sres, A., and Nussbaumer, B. (2014). Weissbuch Fernwärme Schweiz—VFS Strategie. Langfristperspektiven für erneuerbare und energieeffiziente Nah-und Fernwärme in der Schweiz. Schlussbericht Phase 2: GIS-Analyse und Potentialstudie. Available at: https://www.fernwaerme-schweiz.ch/fernwaerme-deutsch-wAssets/docs/Dienstleistungen/Weissbuch/Fernwaerme_Weissbuch-deutsch.pdf
Sres, A., and Wirz, A. (2021). Risiko-Absicherung thermische Netze. Available at: https://pubdb.bfe.admin.ch/de/publication/download/10761
Streicher, K. N., Padey, P., Parra, D., Bürer, M. C., and Patel, M. K. (2018). Assessment of the current thermal performance level of the Swiss residential building stock: statistical analysis of energy performance certificates. Energ. Buildings 178, 360–378. doi: 10.1016/j.enbuild.2018.08.032
Suisse, A. E. E. (2021). Wissenschaftlicher Beirat der AEE SUISSE begrüsst Energieperspektiven 2050, weist aber auf kritische Punkte und sofortigen politischen Handlungsbedarf hin. Available at: https://aeesuisse.ch/wp-content/uploads/2021/08/AEE-SUISSE-WiBi-Energieperspektiven-2050.pdf
Swiss Federal Office of Energy. (2020). Analyse des schweizerischen Energieverbrauchs 2000–2019 nach Verwendungszwecken. Available at: https://pubdb.bfe.admin.ch/de/publication/download/10260
Swiss Federal Office of Energy. (2021). Potenzial von Fernwärme-und Fernkälteanlagen. Available at: https://www.newsd.admin.ch/newsd/message/attachments/69676.pdf
Swiss Federal Office of the Environment. (2020). Switzerland’s Greenhouse Gas Inventory 1990–2018. Available at: https://www.bafu.admin.ch/bafu/en/home/topics/climate/state/data/climate-reporting/previous-ghg-inventories.html
Swiss Federal Office of the Environment. (2021). Kenngrössen zur Entwicklung der Treibhausgasemissionen in der Schweiz 1990–2019. Available at: https://www.bafu.admin.ch/dam/bafu/de/dokumente/klima/fachinfo-daten/kenngroessen_thg_emissionen_schweiz.pdf.download.pdf/Kenngrössen_2021_D.pdf
Taylor, P. G., Upham, P., McDowall, W., and Christopherson, D. (2014). Energy model, boundary object and societal lens: 35 years of the MARKAL model in the UK. Energy Res. Soc. Sci. 4, 32–41. doi: 10.1016/j.erss.2014.08.007
TEP Energy and ECOPLAN. (2020). Erneuerbare-und CO2-freie Wärmeversorgung Schweiz. Available at: https://waermeinitiative.ch/de/fakten
Vasseur, V., Kamp, L. M., and Negro, S. O. (2013). A comparative analysis of photovoltaic technological innovation systems including international dimensions: the cases of Japan and the Netherlands. J. Clean. Prod. 48, 200–210. doi: 10.1016/j.jclepro.2013.01.017
Visnjic, I., Neely, A., Cennamo, C., and Visnjic, N. (2016). Governing the City. Calif. Manage. Rev. 59, 109–140. doi: 10.1177/0008125616683955
Volkova, A., Latõšov, E., Mašatin, V., and Siirde, A. (2019). Development of a user-friendly mobile app for the national level promotion of the 4th generation district heating. Int J Sustain Energy Plan Manage 20, 21–36. doi: 10.5278/ijsepm.2019.20.3
Voytenko, Y., McCormick, K., Evans, J., and Schliwa, G. (2016). Urban living labs for sustainability and low carbon cities in Europe: towards a research agenda. J. Clean. Prod. 123, 45–54. doi: 10.1016/j.jclepro.2015.08.053
Weisskopf, T., Streit, D., Deschaintre, L., and Robineau, J.-L. (2022). Beschleunigung des Ausbaus Thermischer Netze. Projektbericht. Available at: https://pubdb.bfe.admin.ch/de/publication/download/11019
Wesseling, J., Kieft, A., Fuenfschilling, L., and Hekkert, M. (2022). How socio-technical regimes affect low-carbon innovation: global pressures inhibiting industrial heat pumps in the Netherlands. Energy Res. Soc. Sci. 89:102674. doi: 10.1016/j.erss.2022.102674
Zaoual, A.-R., and Lecocq, X. (2018). Orchestrating circularity within industrial ecosystems: lessons from iconic cases in three different countries. Calif. Manage. Rev. 60, 133–156. doi: 10.1177/0008125617752693
Keywords: thermal grid, district heating and cooling, business ecosystem, low-carbon transition, Switzerland
Citation: Speich M, Chambers J and Ulli-Beer S (2024) Current and future development of thermal grids in Switzerland: an organizational perspective. Front. Sustain. Cities. 6:1379554. doi: 10.3389/frsc.2024.1379554
Received: 31 January 2024; Accepted: 11 April 2024;
Published: 03 May 2024.
Edited by:
Faisal Ahammed, University of South Australia, AustraliaReviewed by:
Harald Rohracher, Linköping University, SwedenCopyright © 2024 Speich, Chambers and Ulli-Beer. This is an open-access article distributed under the terms of the Creative Commons Attribution License (CC BY). The use, distribution or reproduction in other forums is permitted, provided the original author(s) and the copyright owner(s) are credited and that the original publication in this journal is cited, in accordance with accepted academic practice. No use, distribution or reproduction is permitted which does not comply with these terms.
*Correspondence: Matthias Speich, bWF0dGhpYXMuc3BlaWNoQHpoYXcuY2g=
Disclaimer: All claims expressed in this article are solely those of the authors and do not necessarily represent those of their affiliated organizations, or those of the publisher, the editors and the reviewers. Any product that may be evaluated in this article or claim that may be made by its manufacturer is not guaranteed or endorsed by the publisher.
Research integrity at Frontiers
Learn more about the work of our research integrity team to safeguard the quality of each article we publish.