- 1Department of Architecture, Aalto University, Espoo, Finland
- 2Department of Built Environment, Aalto University, Espoo, Finland
- 3Helsinki Institute of Sustainability Science, University of Helsinki, Helsinki, Finland
- 4Department of Energy and Process Engineering, Norwegian University of Science and Technology, Trondheim, Norway
Urban green infrastructure is recognized for its potential to combat biodiversity loss and enhance carbon sequestration in cities. While residential yards constitute a significant part of urban green infrastructure, their role in providing urban ecosystem services remains largely understated. There is a lack of systematic measures for effectively implementing urban vegetation to enhance ecosystem services. The aim of this study is to investigate how different vegetation types typically found in urban residential yards of apartment blocks can enhance carbon sequestration and biodiversity, and how these benefits can be supported through landscape design. The study encompasses an integrative literature review and qualitative analysis. Drawing from a review of previous research, this study identifies the drivers that indicate either carbon sink potential or biodiversity enhancement potential of urban vegetation types. The drivers are then cross-examined to identify the qualities of urban green that potentially strengthen carbon–biodiversity co-benefits. As the key findings we present versatile measures to enhance the potential co-benefits of carbon sinks and biodiversity within urban yards and summarize them in three main categories: plant diversity, provision of good growing conditions and maintenance. The study stresses that the several potential co-benefits of urban green can only be achieved through the selection and prioritization of solutions during the planning and design process. To exemplify this, we demonstrate how the findings from the literature review can be incorporated into the design and management of urban yards. We conclude that the main actions to be addressed in the future planning and design of urban residential yards are (i) establishing diverse planting areas with a mixture of woody and herbaceous plants to encourage species richness and complexity, (ii) optimizing the use of space and growth conditions, and, (iii) implementing maintenance practices that consider both carbon and biodiversity aspects. The study highlights that through enhancing carbon-biodiversity co-benefits urban yards can significantly contribute to major environmental challenges and provide vital ecosystem services within the built urban environments.
1 Introduction
Climate change and biodiversity loss are interlinked, and are major sustainability challenges the world is currently facing (IPBES, 2019; IPCC, 2022). Their causes and consequences manifest especially in urban areas, indicating that solutions to both crises must also occur in cities. Conceptual approaches such as ‘carbon-neutral’ (Laine et al., 2020) or ‘biodiversity-friendly’ cities have accordingly been introduced in response to both global crises (Aronson et al., 2017; Fischer et al., 2020; Kowarik et al., 2020; Rega-Brodsky et al., 2022).
In recent years, ecosystem- and nature-based approaches–including nature-based solutions (NBS) and green–blue infrastructure–have received increasing attention for their potential to address pressing environmental challenges in cities. NBS can be defined as ‘solutions that are inspired and supported by nature, which are cost-effective, simultaneously provide environmental, social and economic benefits and help build resilience’ (European Commission, 2021, p. 17).
NBS are multifunctional, providing multiple ecosystem services (European Commission, 2021). Several previous studies have addressed the synergies and trade-offs of ecosystem services at the landscape scale (Karimi et al., 2021), but few studies have described the indicators of such co-benefits of urban green infrastructure on both climate mitigation and biodiversity (Raymond et al., 2017; Choi et al., 2021; Lampinen et al., 2022; Rega-Brodsky et al., 2022). This is a severe knowledge gap as the urban environment is clearly distinct from its natural counterpart, with different rules of guidance affecting the potential carbon sequestration and storage (CSS) rates, as well as biodiversity. Albeit not strictly accountable as an ecosystem service, biodiversity is closely related to the functionality of an ecosystem (Jax and Heink, 2015). More diverse environments nurture higher ecosystem service level (Le Provost et al., 2023) and thus managing biodiversity can also enhance ecosystem services. The interaction of spatial-, temporal-, and socioeconomic processes associated with urbanization have been suggested to act as a distinct series of hierarchical biogeographic filters, which dictate the organism community composition in urban environments (Aronson et al., 2016).
Residential landscapes are of particular interest as hubs of CSS and biodiversity. Residential land represents 41% of urban areas globally (UN, 2014), and residential yards and domestic gardens constitute a significant part of green infrastructure in cities (Loram et al., 2007; Haase et al., 2019). The private and semi-public yards of urban residentials blocks thus have a significant, though understudied, potential to contribute to urban green infrastructure and tackle climate change and biodiversity loss (Larson et al., 2016; Schmidt and Walz, 2021). Tools such as biotope area factor or green area factor (Juhola, 2018) aim to harness this potential. However, despite such practical implementation, a deeper integrated understanding of how the CSS and biodiversity of residential yards in cities can be preserved and enhanced is still missing.
It is well established that the urban carbon pool is diverse and contributes to terrestrial carbon sinks both locally and globally (Churkina et al., 2010; Hutyra et al., 2011; Nowak et al., 2013). For example, recent research suggests that the urban green can sequester up to 7% of a city’s annual emissions and that almost half of the sinks are based on urban green spaces of the built environment (Havu et al., 2024). The methods for estimating urban carbon pools vary, for example, in terms of resolution and definition of land-use classes (Davies et al., 2013), thus making it difficult to make comparisons. Many assessments only cover above-ground carbon storage, thus resulting in a high emphasis on the role of trees (Ariluoma et al., 2023). While the vegetation coverage in cities is currently shrinking in most urban areas (Richards and Belcher, 2020), especially in residential environments, there is significant potential for CSS increment (Kinnunen et al., 2022). Soil usually forms the most significant carbon storage in residential yards, augmented by choice of vegetation types and maintenance practices (Schmitt-Harsh et al., 2013; Sarzhanov et al., 2017).
The CSS potential of urban residential yards can further be enhanced with both novel solutions–such as biochar or green building surfaces–and by diversifying the current vegetation. A diverse vegetation structure is often the best practice in optimizing above- and below-ground CSS rates, both spatially and temporally (Edmondson et al., 2014c; Gu et al., 2015; Horn et al., 2015), because CSS in yards varies not only between vegetation classes but also with time (Pataki, 2011; Huyler et al., 2014; Havu et al., 2022).
As the residential green can contribute significantly to the total green infrastructure of a city, improving the CSS potential of these green spaces can have a substantial impact on the related carbon sink capacity (Tahvonen and Airaksinen, 2018; Kinnunen et al., 2022). Although carbon stocks in cities are much smaller than emissions, the residential landscape covers large areas, so its impact and potential as a whole is large for both carbon sinks and other ecosystem services (Ariluoma et al., 2021; Schmidt and Walz, 2021). Further, preserving existing carbon sinks and stores is important to prevent associated carbon losses from residential landscapes (Davies et al., 2011; Bae and Ryu, 2015; Trammell et al., 2017).
Residential yards and gardens have also been shown to support considerable biodiversity (Goddard et al., 2010). Besides vascular plants (Thompson et al., 2004), evidence from beetles (Barratt et al., 2015), bumblebees (Gunnarsson and Federsel, 2014), birds (Paker et al., 2014), spiders (Otoshi et al., 2015), and mammals (Van Helden et al., 2020; Kristancic et al., 2022) point to the potential of urban yards and gardens as habitats for diverse species, with specific garden features such as vegetation characteristics (Paker et al., 2014; Van Helden et al., 2020; Kristancic et al., 2022), and management methods (Otoshi et al., 2015; García-Antúnez et al., 2023) shaping the patterns of diversity in these groups.
It is also increasingly recognized that proper restoration techniques, improved management practices, and nature-based solutions are able to improve the fine-scale habitat characteristics in urban environment for many species, thus further supporting biodiversity (Goddard et al., 2010; Aronson et al., 2017). Urban parks can be hotspots for biodiversity (Nielsen et al., 2014), but green roofs, urban brownfields, power line clearings, and stormwater catchments have also been proposed as solutions to enhance urban biodiversity (Lampinen et al., 2015; Kyrö et al., 2020).
The relationships between biodiversity and carbon storage have been extensively studied in different geographic and spatial contexts (Di Marco et al., 2018), but how these relationships play out in residential yards, a key component of green infrastructure in cities, has so far remained understudied. As cities become denser and the amount and size of green areas diminishes (Hautamäki, 2019), the role of residential yards in safeguarding biodiversity and contributing to CSS becomes increasingly important. Only a few previous studies have studied the ecosystem services co-benefits of residential yards (Schmidt and Walz, 2021), and even fewer have presented application-oriented indicators and methods to simultaneously recognize and enhance co-benefits of CSS and biodiversity in yards.
To address this knowledge gap, our study conducts a review that examines the carbon and biodiversity benefits of urban vegetation, consolidating findings from various research domains within the context of urban residential yards of apartment blocks. Within this study, we identify and assess the most effective strategies for promoting CSS and enhancing biodiversity within residential yards situated in densely populated urban areas. Our primary goal is to explore how different types of vegetation, typical of residential yards, contribute to the enrichment of urban carbon sinks and biodiversity. Moreover, we aim to elaborate on how these advantages can be further reinforced through fine-scale landscape design and management solutions.
2 Methodology
The study consists of a four-step integrative literature review (Torraco, 2005; Whittemore and Knafl, 2005; Snyder, 2019), which, according to Torraco, is suitable for assessing and synthesizing the literature on a research topic in a way that enables new theoretical frameworks and perspectives to emerge. First, we composed research questions and defined the scope to guide the review; second, we searched and screened the literature; third, we conducted a thematic data analysis and fourth, as the synthesis of our review, we assessed the co-benefits of CSS and biodiversity (Figure 1).
The review was informed by a two-fold question addressing the key drivers for CSS and biodiversity in vegetation types in urban residential courtyards. The scope has been defined as follows. In this study, a “driver” refers to a characteristic within urban green spaces that enhance CSS and biodiversity. The focus of the review is on studies that introduce specific drivers for CSS and biodiversity, not exact indicators or quantities.
The context of the study is urban residential yards situated within densely developed urban environments, referring to urban blocks and courtyards of multi-story apartment buildings (hereafter called as ‘urban yards’). Urban yards can vary in type and size, but typically, these yards consist of relatively small size vegetated areas and multiple functional requirements such as playgrounds, parking areas and paths, that limit the area available for vegetation (see for example Ariluoma et al., 2021). Moreover, urban residential courtyards can be quite demanding growing environments, for instance, due to shading by the buildings and, quite often, due to underground parking facilities. As to ownership, the models vary from country to country. The most common form is the dualistic system, in which each apartment unit within a building is owned separately and individually, and the owners of the apartments own collectively the common areas, for example yard (Easthope, 2019, p. 4).
Despite the chosen context, the review has not been limited to urban residential yards alone, as there are limited studies on this topic. The review included research literature related to the vegetation types commonly found in densely developed urban areas, such as yards, gardens, allotment gardens or other small-scale urban green spaces, which correspond to the vegetation types and conditions of urban residential courtyards.
As to vegetation types, we applied a typology of carbon-oriented urban vegetation (Ariluoma et al., 2023) which categorizes the vegetation into woody vegetation, herbaceous vegetation, and mixed-types. A more detailed categorization is based on the Green Factor method used in the planning and design of urban yards (Juhola, 2018), consisting of trees, shrubs and climbers, perennials, urban meadows, lawns, green roofs and urban farming plots.
The study focuses on site-scale qualities, excluding large urban green space types, such as urban forests, and landscape-scale characteristics, such as habitat connectivity. The study also excludes green walls, which are not yet sufficiently applied in a Nordic climate region, even though they may have relevant biodiversity potential. Moreover, we excluded small-scale interventions such as the provision of bird feeders, nest boxes, bat boxes and compost heaps, as these measures are not directly dependent on vegetation.
After defining the scope and review questions, searching and screening of literature was conducted by using Scopus. We focused on journal articles published from 2000 until the beginning of 2023 and written in English. The search was limited within titles, abstracts and keywords, and was completed separately for the two research themes, biodiversity and carbon, using search strings such as ‘theme’ AND ´vegetation type’ AND ‘residential’ OR ‘garden’ OR ‘courtyard’ OR ‘yard’ AND ‘urban.’ Vegetation types searched were as follows: tree, shrub, hedge, climber, lawn, grass, perennial, meadow, green roof, vegetated roof, garden plot and urban farming.
The search originally yielded 329 results for CSS and 722 for biodiversity. The results were screened according to following steps:
1. Articles must be written in English, published after 2000, and come from the fields of environmental, biological, or earth sciences.
2. Articles should primarily focus on biodiversity and/or carbon sequestration. Papers solely addressing other ecosystem services were excluded based on titles and abstracts.
3. Articles should specifically address small-scale urban green spaces and/or relevant vegetation types. Studies focusing on large green spaces like urban forests or agricultural lands were excluded. This screening was based on an initial review of the content of the papers.
4. Articles selected for in-depth review must present drivers aimed at enhancing biodiversity or carbon sequestration potential.
When the search method did not produce relevant results for a specific vegetation type, the search was continued by using a ‘snow-balling’ method. Following this method, finally 32 articles related to CSS and 34 to biodiversity were selected for thematic analysis. In the thematic analysis we identified the key factors for enhancing CSS and biodiversity related to various vegetation types typical for urban residential courtyards and summarized the results in two tables. The relevance of the drivers was assessed on the basis of research literature and most of the selected drivers received at least two mentions in literature.
In the last phase, we cross-examined the results in order to find the most potential drivers for co-benefits. This was completed by comparing the list of drivers for CSS and biodiversity according to each vegetation type and by further analyzing the drivers for potential win-win effect. Finally, we synthesized our findings and implications on how the co-benefits can be further enhanced in urban and landscape design and management of urban residential courtyards.
3 Results
3.1 Drivers of CSS potential of urban vegetation
3.1.1 Woody vegetation
Nearly all of the urban aboveground carbon sequestered in vegetation is stored in trees (Davies et al., 2011). Thus, it might be concluded that a simple way to increase carbon stocks in urban yards would be to maximize the number of trees and their biomass (Golubiewski, 2006; Ariluoma et al., 2021). However, in the first years of their life span, urban trees may cause emissions through their growth media that exceed the amount of carbon sequestrated (Riikonen et al., 2017; Havu et al., 2022). Havu et al. (2022) found that for street trees, the compensation point at which trees turn from annual sources to sinks was 14 years after planting. In urban yards, this compensation point may be achieved sooner due to more favorable growing conditions compared to street trees and a possibility for plant litter to accumulate in soil. However, if similar growing media and soil respiration rates apply, trees will likely remain sources of CO2 for several years after planting in garden and park-like conditions too, mainly due to high soil respiration rates (Riikonen et al., 2017). Thus, the lifetime of an urban tree becomes an important factor as only a mature tree is a carbon sink (Nowak et al., 2013). There are large variations in the lifespan and size of mature trees among species. Both native and non-native cultivated species are common (Gaertner et al., 2017). It is also important to ensure the presence of trees of different ages, so younger trees can replace old decaying ones (Lindén et al., 2020). Additionally, as tree roots typically reach deeper soil layers, their presence usually increases the total CSS potential of more mature yards (Golubiewski, 2006; Davies et al., 2011). As such, a diverse vegetation structure is often the best practice in optimizing above- and below-ground CSS rates both spatially and temporally (Edmondson et al., 2014c; Gu et al., 2015; Horn et al., 2015) (see Tables 1, 2).
Tree species appear to have a major impact on potential carbon sinks. Furthermore, species diversity should be taken into account, particularly to enhance resilience to changing climate and other potential threats, such as pests or diseases (Roloff et al., 2009). Some non-native species thrive better in urban environments than native species as they are better adapted to modified and extreme growing conditions (Sjöman et al., 2016; Gillner et al., 2017; Schlaepfer et al., 2020). However, Belaire et al. (2022) found that sites with greater richness in tree species and a greater percentage of native trees had higher carbon sequestration values. Similar results from previous studies in forests indicate that increased tree species richness is associated with higher carbon stocks, carbon fluxes, and total carbon storage (Lecina-Diaz et al., 2018; Belaire et al., 2022). Moreover, evergreen trees and shrubs have been found to enhance the carbon accumulation in soil (Setälä et al., 2016; Lu et al., 2021).
In addition to species characteristics, the life span of an urban tree is affected by several factors. The average life span of trees growing in urban conditions is usually shorter than those growing in natural conditions (Sæbø et al., 2003). This is due to various stress factors, such as the availability of water, heat, pollution, physical damage and soil compaction even during dry periods (Hilbert et al., 2019; Lüttge and Buckeridge, 2020). The mortality of urban trees is at highest during the first years after establishment, but can be minimized through proper maintenance, including watering and protection from damage and vandalism (Sæbø et al., 2003; Hilbert et al., 2019). Young trees require irrigation during the first years after planting in almost all conditions, but especially during dry periods. If the sapling dies, the soil respiration continues, but the compensation point moves even further away, even if the tree is replanted (Havu et al., 2022). Mature trees do not typically require irrigation if they have the possibility to develop a strong root system and access moisture in the deeper layers of soil. This is often limited in urban areas by constructed and compacted soil layers (Sæbø et al., 2003). Trees also have varied strategies to cope with drought, thus further highlighting the role of species selection (Gillner et al., 2017).
For trees, sufficient growth space is one of the major limiting factors for CSS capacity, especially in densely built urban areas. As a result of limited space, as well as other horticultural reasons, trees are often pruned and cut, which diminishes their carbon storage potential (Nowak et al., 2002). However, cutting and pruning woody plants can also lead to more dense and increased growth, and thus to increased carbon sequestration rates, as found in hedgerow systems (Sæbø et al., 2003). The resulting carbon impact depends on how the biomass is processed. Another factor that limits the space suitable for trees in urban yards is shading by buildings. Although some tree species can survive in shade, an exteremely low light intensity is a stress factor for trees and limits photosynthesis, thus decreasing carbon sequestration (Sæbø et al., 2003; Guo et al., 2020).
The typical maintenance of shrubs in yards differs from that of trees. Typical activities include cutting and mulching. Although mulching may benefit growth, excess mulching increases soil respiration and is not necessarily considered an effective way to optimize CSS (Edmondson et al., 2012, 2014c; Lindén et al., 2020). However, plant litter input is critical for the accumulation of organic matter in the soil, and this process can be supported by maintenance, for example, by leaving leaf litter on the ground (Bardgett and Wardle, 2010).
3.1.2 Herbaceous vegetation
The CSS capacity of herbaceous vegetation types is defined by the species composition, growing conditions, and maintenance practices (Ariluoma et al., 2023). Different species are adapted to different growing conditions, but availability of moisture and nutrient richness define plant productivity, which is commonly controlled by standardized growth media products applied in built areas (Kuittinen et al., 2021). Many herbaceous plants are also adapted to wet habitats. Wet conditions seem to increase the organic carbon values in urban soils (Dorendorf et al., 2015; Kavehei et al., 2021), so rain gardens and similar areas that are designed for rainwater management may also enhance soil carbon storage.
The ability of herbaceous plants to act as carbon sinks relies on the interaction between soil and vegetation, specifically the accumulation of carbon within the soil. The process of underground plant production is significantly important because it has been shown to represent a major part of the total biomass production (Steinaker and Wilson, 2008). Plant species diversity is found to be linked to increased soil carbon storage both in urban and non-urban settings (Lange et al., 2015; Schittko et al., 2022), and the results apply to both native and non-native plants (Schittko et al., 2022). Mixing plant species can enhance effective root production on different soil layers. High soil carbon levels are also linked to increased soil microbiota and earthworm diversity (Schittko et al., 2022).
Urban lawns seem to be relatively efficient in transferring carbon in soil due to high productivity enhanced by maintenance (Poeplau et al., 2016). However, the overall relevance of urban grass lawns as a carbon sink is controversial (Lindén et al., 2020). First, at the early stages of development, lawns are a source of carbon, due to grass soil constructions (Shchepeleva et al., 2017). Early-stage carbon loss can be minimized by a soil material with low organic matter content (Shchepeleva et al., 2017), which may lower productivity in the beginning. Second, lawn maintenance is an important source of CO2 emissions. Emissions can be reduced by using low-carbon maintenance with electric/solar-powered machines (Thompson and Kao-Kniffin, 2019). Optimal maintenance frequency (mowing) is important but difficult to define as soil carbon response to maintenance is complicated (Trammell et al., 2017; Thompson and Kao-Kniffin, 2019; Lindén et al., 2020). However, leaving grass litter/clippings on the ground can diminish the need for additional nutrient inputs and raise soil carbon storage (Gu et al., 2015; Lorenz and Lal, 2015; Poeplau et al., 2016). Further, lawns are prone to drought and compaction, both of which can limit the carbon sequestration capacity in residential yards (Francini et al., 2021).
Green roofs have been broadly investigated and several studies show that green roofs sequester carbon on a yearly basis. However, most studies are based on observations during a short interval of time (Shafique et al., 2020). As pointed out by Kinnunen et al. (2022), green roofs lack storage capacity, or at least the capacity is much more limited than for ground-based plantings. Thus, the right choice of materials and plant selection are important, as well to store the carbon after the average life span of a green roof (between 40 and 50 years) (Shafique et al., 2020).
Furthermore, green roof construction has environmental impacts via the material production (Scolaro and Ghisi, 2022), which can be partly compensated by the energy savings and carbon sequestration. Using simple, lightweight green roof designs to avoid material use is recommended to minimize the environmental impacts (Bozorg Chenani et al., 2015; Scolaro and Ghisi, 2022). The weight of soil on green roofs is the main source of environmental impact. However, most life cycle assessment (LCA) studies show that conventional roofs have a higher environmental impact even than green roofs (Scolaro and Ghisi, 2022).
In urban yards, annual plants mostly occur in annual flower beds, meadows in the first years, and gardening patches. A key difference with other vegetation types is that the soil is usually bare outside the growing season and is often disturbed by cultivation, which enhances carbon release (Bae and Ryu, 2015). After the growing season or harvest, the vegetation dies and starts to decompose. Carbon accumulation in soil depends on how the decomposing plant material and soil are treated. High soil carbon levels can be maintained by the additional carbon inputs (manure and compost) (Edmondson et al., 2014a). Edmondson et al. (2014a) suggest that for urban allotment cultivation to be more sustainable, efforts should be made to improve the nutrient and organic matter cycle within cities.
3.1.3 Soil carbon storage
While vegetation plays a crucial role in converting atmospheric carbon into soil organic carbon, the primary climate benefit lies in long-term soil carbon storage (Ariluoma et al., 2021). Any soil disturbance should be avoided and a long life span of all green areas should be guaranteed (Bae and Ryu, 2015; Lindén et al., 2020). The soil microbiome has been found to affect the carbon mineralization, so the development of management practices oriented to promote microbial activity is recommended (Delgado-Baquerizo et al., 2023).
Typically, soil respiration levels are high right after the establishment and in the first years, soil carbon increases slowly (Huyler et al., 2014; Livesley et al., 2016). Results show that the time during which the carbon level stabilizes in urban green areas vary roughly from 30 to 50 years (Pouyat et al., 2009; Lindén et al., 2020; Sapkota et al., 2020). However, older parks and residential sites may contain even higher soil carbon levels and carbon seems to accumulate in deeper soil layers over time (Vasenev et al., 2013; Setälä et al., 2016; Sarzhanov et al., 2017). A woody vegetation layer has been found to increase the soil carbon content in parks, gardens, and allotments (Edmondson et al., 2014a; Setälä et al., 2016).
3.2 Drivers of biodiversity potential of urban vegetation
Plants contribute to different dimensions of biodiversity in urban yards through the taxonomic diversity of plants, by providing habitats for other species, and by facilitating species interactions and ecological functions (Kowarik, 2011; Schlaepfer et al., 2020). Nielsen et al. (2014) found that plant species richness tends to correlate with the number of animal species in urban parks, which is also a common correlation in natural habitats.
Plant taxonomic diversity in cities is often rich but a large part of this is based on non-native and horticultural species. Whether non-native species contribute to urban biodiversity positively or negatively is controversial (Nielsen et al., 2014; Sjöman et al., 2016; Berthon et al., 2021), except for the negative contribution of invasive non-native species that can harmfully spread and invade natural habitats (Gaertner et al., 2017). Some urban insect populations rely on locally adapted non-native species as their food sources (Adams et al., 2020), while herbivorous insects often require a specific native host plant (Burghardt et al., 2009; Narango et al., 2017). Thus, native plants have an impact on the whole food chain and can be considered more valuable for biodiversity on different trophic levels (Narango et al., 2017). Increasing native plants in urban yards has also been found to result in greater bird diversity (Burghardt et al., 2009; Lerman and Warren, 2011). Moreover, planting climate-appropriate or native flora and maintaining landscapes that more closely match conditions in nearby natural areas can increase biodiversity (Adams et al., 2020).
One widely studied aspect of urban biodiversity is wild bee communities. Bees rely on floral resources, and diverse urban plant communities can provide floral resources throughout the seasons. Increasing floral resources has been suggested as an efficient means for urban bee conservation (Plascencia and Philpott, 2017; Egerer et al., 2020; Gerner and Sargent, 2022).
3.2.1 Woody vegetation
Despite the fact that tree species diversity is often large in cities, typically, only a few species comprise the majority of trees (Sæbø et al., 2003; City of Tampere, 2022), and these are often non-native species (Nielsen et al., 2014; Schlaepfer et al., 2020). Some researchers suggest that planting native trees is a high-impact strategy for optimizing urban biodiversity (Belaire et al., 2022). Native tree species may provide greater foraging and habitat resources for insects, birds, and epiphytic plants (Narango et al., 2017).
In addition to taxonomic diversity of tree species, genetic diversity should be considered when choosing plant material, as this supports biodiversity and the health of urban trees (Sæbø et al., 2003). In an optimal situation, plant material could be selected according to the ecotype of the species. However, currently this information is often not available from nurseries (Sjöman and Watkins, 2020).
Increased tree canopy cover and age diversity are positively associated with urban biodiversity, for example, the richness of bird species in residential yards (Lerman et al., 2021). Trees provide an important habitat for a diverse set of organisms, such as insects, lichens, and fungus (Lundquist et al., 2022). Large old trees are a biodiversity-rich habitat type that is declining globally (Lindenmayer et al., 2012). Veteran trees with hollows and decaying wood material in particular are hotspots for biodiversity (Nielsen et al., 2014; Wetherbee et al., 2021).
Although little research has focused specifically on urban shrubs, many studies emphasize the importance of the diversity and complexity of vegetation communities (e.g., Goddard et al., 2010), in which shrubs play an important role. Shrubs have many of the same qualities as trees, such as providing floral resources for bees throughout the seasons (Gerner and Sargent, 2022). The life span of shrubs is usually shorter than that of trees, so they cannot provide similar habitats for lichens and fungus, for instance. Shrub plantings, as well as climbers, provide shelter, food resources, and nesting places for birds and wildlife (Paker et al., 2014; Rousseau et al., 2015), and many urban wildlife gardening guides mention the importance of a shrub layer for urban wildlife (e.g., RSPB, 2023). Decomposing plant litter often found under shrubs is important for insects and small organisms and can promote soil fauna diversity (Tresch et al., 2019b).
3.2.2 Herbaceous vegetation
Herbaceous perennial plantings and urban grasslands occur in various forms in urban yards and gardens, ranging from ornamentals to semi-natural meadow-type vegetation. Similar to shrubs, the potential species richness of perennial plants is high, so they can provide floral resources throughout the seasons. Additionally, the diversity of grassland species significantly influences soil microbial communities. However, studies indicate a contrasting effect of non-native plant species on soil microarthropod diversity, with an increase in their presence correlating with a decline in microarthropod populations (Schittko et al., 2022).
Herbaceous plants include many endangered cultural species that are typical for traditional agriculture-based grassland environments, but that have lost their habitats as a result of the decline of these landscapes. Urban yards, as well as other built landscapes, can potentially provide alternative habitats for native and endangered species of traditional cultural landscapes (Lampinen, 2020). For example, green roofs can provide an escape for many species familiar to traditional biotopes. In general, studies on green roofs show that they more often support generalists than specialist species, although contrasting observations have been made as well (Kyrö et al., 2020; Kotze et al., 2021). Kyrö et al. (2020) propose that green roofs should be designed so that they mimic local open habitats, for example, by utilizing local seed banks (Nieminen, 2021). Brachet et al. (2019) used the LCA methodology to assess the biodiversity impacts of different green roof types as a whole. They found that extensive green roofs (a substrate layer from 4 to 12 cm) have smaller negative impacts for biodiversity outside the site, as they require fewer material resources than intensive green roof types (a substrate layer exceeding 13 cm).
Urban grasslands, especially intensively managed grass lawns, have also been broadly studied from the perspective of biodiversity. Typically, lawns offer very little opportunity for biodiversity to thrive (Francoeur et al., 2021), but biodiversity measures improve through the use of native plants (Hostetler and Main, 2010). Ignatieva et al. (2020) propose a paradigm shift with urban lawns, based on species-rich native plant communities. Alternative lawn types have been developed and applied, both with spontaneous and carefully designed vegetation. For example, low-growing grass-free vegetation mats have been tested as a biodiverse low-maintenance option for grass lawns (Ignatieva and Hedblom, 2018).
Regarding more traditional lawns, lengthening the mowing interval and timing the mowing to allow for the flowering of herbaceous species can enhance the biodiversity of lawns (Ignatieva et al., 2020). Lawn mowing frequency affects wild bee abundance and insect diversity in yards (Lerman et al., 2018; Francoeur et al., 2021; Proske et al., 2022) and the diversity of plants in the lawn (Watson et al., 2020). However, trimmed grassland can create better grazing opportunities for birds (Pithon et al., 2021). The impacts of typical lawn maintenance practices–such as the management of grass clippings, irrigation, and fertilization–on soil microbial communities and plant species diversity are variable and depend on the specific combination of different practices (Thompson and Kao-Kniffin, 2019), thus making it difficult to interpret the direct and indirect impacts on biodiversity. For example, Crawley et al. (2005) found that turfgrass species richness and microbial diversity declined with soil acidification, caused by the use of a certain combination of fertilizers over a long time. While return of grass clippings has been found to reduce need for fertilizing, it can thus also have an indirect impact on biodiversity (Thompson and Kao-Kniffin, 2019).
3.2.3 Mixed-vegetation communities
Multi-layer complex vegetation that mimics natural ecosystems has often been mentioned as an effective way to create biodiversity in gardens and urban areas, and it is included in the guidelines provided by many wildlife gardening programs (Widows and Drake, 2014; Larson et al., 2022). Complex habitats created by mixing different plant types provide habitats for rich fauna (Goddard et al., 2010; Francoeur et al., 2021). Diverse plant communities have also been found to enhance soil fauna diversity (Tresch et al., 2019a).
Urban garden patches and farming plots are two types of species-rich cultivated habitats. These areas, often managed by residents, are used for growing a variety of crops, including annual plants such as vegetables and flowers. Urban farming plots can vary in size, from small raised beds to larger patches of land. As well as annual plants, these areas can also harbor a high number of wild plants (Seitz et al., 2022). Management of urban gardening plots impacts the vegetation structure and soil quality of these sites, and thus the biodiversity value (Edmondson et al., 2014a; Tresch et al., 2019a,b), to a great extent. For example, the application of compost, often related to urban gardening activities, has been found to positively affect soil fauna communities (Tresch et al., 2019a). In urban gardens, the richness of flowering perennials and the abundant flower resources of annuals promote richness in pollinator species (Egerer et al., 2020).
Areas reserved for nature-based stormwater retention and water quality enhancement in courtyards can consist of various vegetation types. Depending on the type of structure and climate conditions, there is high variation in the level of moisture and occurrence of visible water in these types of habitats. Rain gardens represent a typical rainwater management solution in urban yards and we assume their biodiversity value can be compared to other mixed-type vegetation communities (Osheen Singh, 2019). In general, nature-based stormwater infrastructure has been presented to enhance urban biodiversity, but there is a need for more studies to understand the specific qualities of these solutions, and how they impact on biodiversity (Wang et al., 2023). Water elements with a permanent water surface, such as ponds, provide valuable habitats for invertebrate communities (Hassall and Anderson, 2015), but establishing ponds in urban courtyards is not very common.
3.3 Identifying drivers to enhance co-benefits
The literature review identified key drivers for supporting carbon sinks (Table 1) and biodiversity potential (Table 2) in urban yards across different vegetation types. As a synthesis of the reviews, the drivers that potentially impact positively both carbon sinks and biodiversity, were identified by cross-examining the results of the two thematic reviews. The study reveals there are various ways to simultaneously boost carbon sinks and biodiversity by the planning and management decisions of urban vegetation. The results can be classified under three main topics, encompassing factors related to (i) species diversity, (ii) growing conditions and (iii) maintenance. The drivers, related vegetation types and the potential co-benefits have been further analyzed and presented in Table 3.
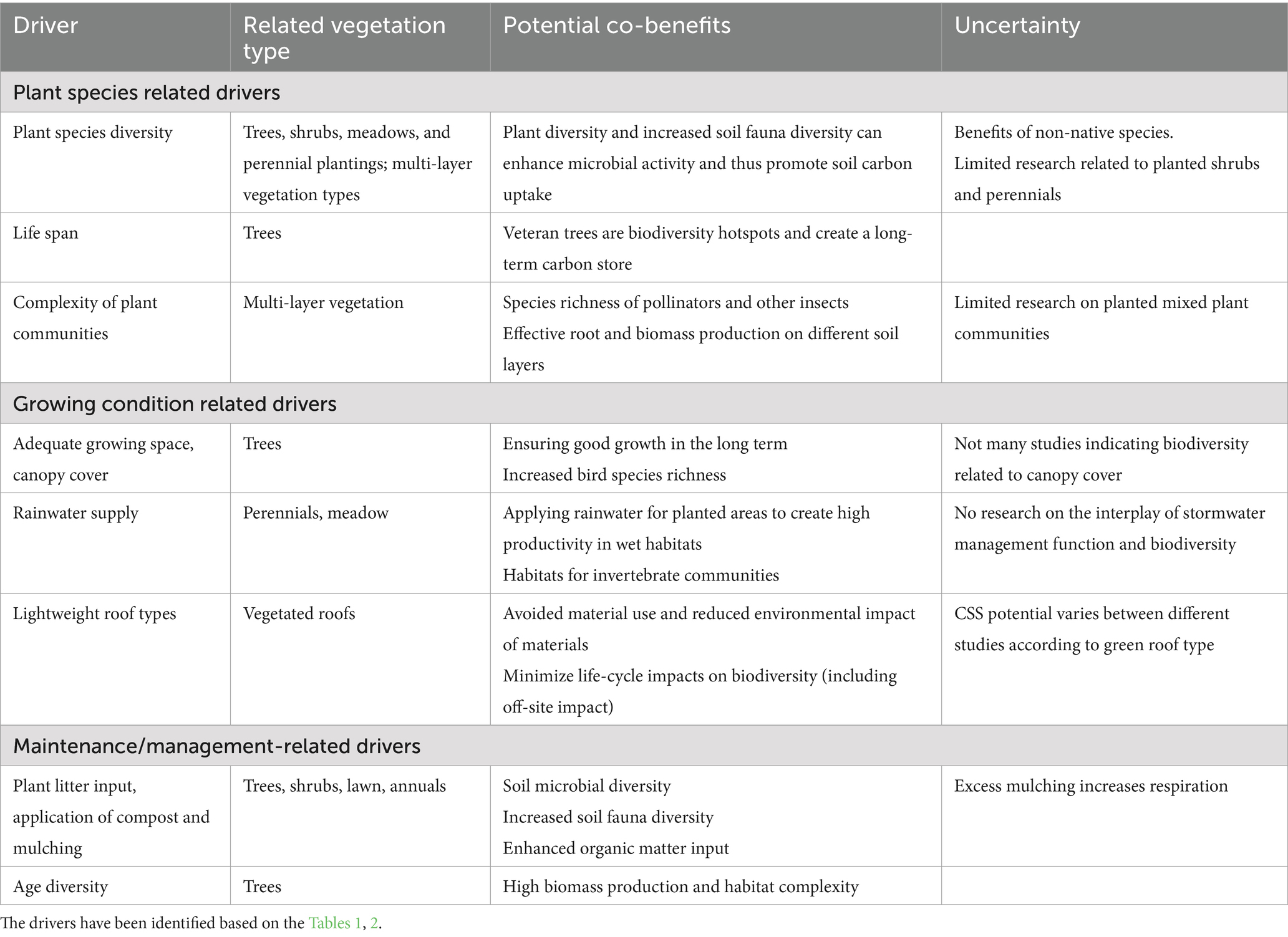
Table 3. Drivers with potential to enhance the co-benefits of biodiversity and CSS, and related vegetation types.
Plant species related factors to enhance co-benefits include increasing plant species diversity and complexity of plant communities and promoting the life span of urban trees. Diversity of plant species has many positive effects on the carbon–biodiversity nexus, but also many uncertainties, especially related to non-native species (e.g., Berthon et al., 2021). Diversity of plant species can have both direct and indirect impacts and concerns all vegetation types. The most obvious interlink highlighted in the literature was found via soil fauna diversity, which is found to increase along with plant species diversity and has a positive impact on soil carbon accumulation (Schittko et al., 2022). Moreover, while plant, fauna, and microbial diversity create complex interaction connections, it is likely that soil microbial activity also plays a role in this context.
The complexity of plant communities is closely connected to species diversity, but refers especially to structural complexity, meaning that woody and herbaceous plants of different sizes exist in the same habitat. Complex multi-layer vegetation types in urban areas are not very well studied, and only a few references were found addressing these types of plant communities. The clearest evidence of biodiversity value was found from research on urban bee communities benefiting complex vegetation communities that provide resources for various pollinator species throughout the seasons (e.g., Ayers and Rehan, 2021). Multi-layer vegetation types can also utilize space efficiently, both above and below ground, which promotes effective root and biomass production and thus can enhance carbon sinks (Steinaker and Wilson, 2008).
Based on our review, large old trees emerge as one of the most prominent indicators of carbon–biodiversity co-benefits. Although the lifespan of urban trees can be influenced by various factors, their species determine their ability to thrive in an urban environment. Veteran trees, in particular, serve as biodiversity hotspots and offer valuable long-term carbon storage both above and below ground (e.g., Nowak et al., 2013; Nielsen et al., 2014). Enhancing the co-benefits of carbon storage and biodiversity can be achieved by providing optimal growth conditions for urban trees, including adequate growth space and access to water. Furthermore, the age diversity of trees, achievable through long-term management practices, serves as an indicator of both high habitat value and evidence of a long-term carbon sink.
In addition to functioning as a stormwater management system, individual studies address the benefits of nature-based stormwater solutions as urban habitats (Hassall and Anderson, 2015) as well as for the carbon and nitrogen cycle (e.g., Kavehei et al., 2021). It is evident that applying rainwater to plants can promote ecosystem productivity and has potential positive effects on invertebrate communities. The review indicates that wet habitats with meadow and perennial vegetation are particularly effective in providing co-benefits.
Based on our analysis, one strategy to promote carbon–biodiversity synergies, especially on urban blocks, is the application of lightweight green roof systems. The life cycle impacts of green roof systems have been well studied and several papers indicate the overall positive impacts of green roofs. Their biodiversity benefits are well justified (e.g., Kyrö et al., 2020). If the whole life cycle of a green roof system is considered, uncertainties remain related to the end-of-life stage of the green roof as well as to the construction emissions.
A few drivers to clearly enhance CSS-biodiversity co-benefits address the maintenance of urban vegetation. Applications of decomposing plant litter, compost or mulching all have similar impacts on CSS and biodiversity. These drivers were found to support carbon accumulation and rich soil fauna across all vegetation types, provided they are not used excessively (e.g., Lindén et al., 2020). Decomposing organic matter can also have several other beneficial impacts on the structure and quality of the soil by, for instance, releasing nutrients for the plants, which may improve plant growth and thus enhance carbon sequestration (e.g., Poeplau et al., 2016).
4 Discussion and practical implications
4.1 Assessing the co-benefits potential in urban residential landscapes
Through the review and cross-examination of the drivers supporting CSS and biodiversity potential of urban vegetation types, we identified several measures to enhance the carbon-biodiversity nexus in urban courtyards. In the following chapters we discuss how these synergies can be integrated in the planning, design and management of urban residential yards. Further, several drivers presented in Tables 1, 2 can enhance either biodiversity or carbon sinks independently, without compromising the other.
4.1.1 Creating species-rich complex planting areas with both woody and herbaceous species
A characteristic of urban yards is limited space, which affects the amount of vegetated area and especially the number of big trees. However, a common feature is the versatile use of vegetation types, such as shrubs, perennials, lawn, and vegetated roofs that can be designed and managed to provide various ecosystem services (Ariluoma et al., 2021; Schmidt and Walz, 2021). Dense multi-layered planting areas can be established in even small yards and can provide more benefits per unit than simple-structure vegetation (Edmondson et al., 2014a; Gu et al., 2015; Horn et al., 2015).
In urban areas, tree species selection is heavily influenced by human preference, often leading to a significant proportion of exotic species. The study highlights the importance of considering tree species selection both from an ecological and a functional point of view. Species selection should be based on species diversity and lifespan, and large tree species should be favored over small ones when possible. In cold climates, evergreen tree species can enhance soil carbon accumulation through high root production, low decomposability of needle litter and low soil respiration (Lu et al., 2021). However, the acidification of soil caused by the accumulation of needle litter may restrict the growth of other plant species, which needs to be considered in planting design.
Shrubs are a commonly found vegetation type in urban courtyards due to their easy maintenance and establishment. Instead of mass-planting areas containing a few species, courtyards allow for the creation of diverse shrub-planting areas with high species diversity. Shrub areas can also be mixed with other vegetation types to create multi-layered planting areas, offering even more foraging resources and more effective use of root space (Steinaker and Wilson, 2008; Goddard et al., 2010).
Perennial and meadow vegetation have good potential to support native species, even endangered ones, and provide resources for pollinators (Ignatieva et al., 2020; Lampinen, 2020). In urban yards, species-rich semi-natural perennial planting designs should be preferred. Considering the relatively small size and shady growing conditions for meadow patches, their diversity potential may be low in urban courtyards. Thus, semi-natural planting areas with plants carefully selected for the site and maintained to support the richness of species may be more valuable than a typical natural meadow.
4.1.2 Providing optimal growth conditions and optimizing the use of space
The spatial arrangement of vegetation is typically affected by the arrangement of yard functions (areas for play, lounging, and waste management etc.), and limited by the space reservations for parking, emergency routes and the pedestrian path system of the yards. Since the benefits of green are at least partly related to the surface area, extending the overall area covered with vegetation as much as possible within the plots is a central approach to enhance climate and biodiversity benefits. Rather than organizing vegetation patches in small areas, one obvious way to enhance the carbon–biodiversity nexus is to combine the areas into larger patches of multi-species layered vegetation communities (Goddard et al., 2010; Francoeur et al., 2021). This requires a careful design and prioritization of the functions already in the early phases of planning. For instance, car parking located outside the plots in centralized facilities enables car-free courtyards and, thus, more space for vegetation.
Due to limited space enclosed by buildings, the possibilities for planting large trees and creating high tree canopy cover in urban courtyards can be rather limited. However, maximizing the number of trees and ensuring adequate growing space both above and below ground are some of the most obvious solutions to promoting ecosystem services in urban yards. By organizing the yard functions, utilizing the space under the trees and possibly by sharing some functions with adjacent plots, yard space can be optimized for trees. Also creating larger blocks with a shared yard space could provide possibilities for extended tree cover or larger trees. To optimize the canopy cover in the long term (Lindén et al., 2020; Lerman et al., 2021), we recommend a phasing plan for tree planting to be prepared. Also paying attention to the early maintenance of trees will create a good start for carbon–biodiversity co-benefits to develop in the yards (Sæbø et al., 2003).
Impermeable surfaces, used for various functions, obviously limit the vegetated areas in courtyards. However, rainwater run-off from these areas can be managed to establish rain gardens and ponds, which can create rich habitats and enhance carbon sinks if provided with a suitable plant selection and permanent moisture (Dorendorf et al., 2015; Kavehei et al., 2021; Pille and Säumel, 2021). Also, rainwater from the roofs can be applied to create small ponds with permanent water (Hassall and Anderson, 2015). Availability of water through nature-based solutions in the yards can also support the growth and health of trees and other plants, thus simultaneously enhancing several ecosystem services (Meineke and Frank, 2018). However, water management requires careful planning, as both excess drainage of urban areas and accumulation of water may be harmful for trees. In Nordic coastal regions, for instance, a surplus of precipitation, combined with poor drainage, can cause death to urban trees by leading to oxygen deficiency (Sæbø et al., 2003).
Although the application of vegetated roofs has already become more common in new urban developments, they still cover only a small percentage of the total roof area in cities. Due to the large amount of potential surface area, green roofs–especially lightweight green roof systems with native local species–have great potential to enhance carbon-smart biodiversity in urban courtyards (Brachet et al., 2019; Shafique et al., 2020). However, the long-term accumulation of carbon on green roof systems requires more attention in research. Moreover, green walls and greenery on facades, such as those created by climbing plants, have also been proposed as solutions to increase urban green in places where the space is limited, but both practical applications and research on the benefits is still scarce, especially in the Nordic climate (Manso et al., 2021).
4.1.3 Applying maintenance practices that enhance carbon-biodiversity synergies
The presence of decomposing organic matter plays a key role in several ecological functions. To enhance soil fauna diversity and carbon accumulation, leaves and other plant litter can be left in the planting areas to decompose (Schittko et al., 2022). Recycling plant litter on site can also help avoid the need for excess mulching, which is a common maintenance activity. While mulching has been found to increase carbon content in the topsoil under certain conditions, it also increases soil respiration, thereby releasing CO2 back into the atmosphere (Tresch et al., 2019a; Lindén et al., 2020).
Lawns are often a dominant vegetation type in urban yards. Lawn can sequester carbon surprisingly well, but construction and maintenance emissions can outweigh these benefits (Poeplau et al., 2016). Lawn maintenance impacts both the soil carbon cycle and species composition. Due to the wide variability in practices and climatic influences, pinpointing the effects of specific management inputs is challenging. Additionally, the great variability in plant species composition of urban grasslands, which influences the maintenance requirements to sustain specific lawn quality, complicates the definition of universal maintenance recommendations (Trammell et al., 2017). This underscores the importance of locally adapted best-practice guidelines. Establishing lawns using diverse native grass species, while also optimizing carbon flux by leaving grass clippings and by employing smart need-based maintenance with low carbon electric machinery, can mitigate the negative effects of lawn management (Thompson and Kao-Kniffin, 2019). However, while lawns in general have a very low biodiversity value, the most effective action may involve replacing lawns with other vegetation types (Ignatieva et al., 2020).
4.2 Implications for the planning of urban green infrastructure
Our study focuses on fine-scale improvements of urban vegetation in urban yards, which create a small-scale but widespread pattern of urban habitats (Loram et al., 2007). Recently, the complementary use of private and public green space by wildlife has garnered more attention (Mimet et al., 2020), and private gardens have been suggested to play a key part in ecological land-use complementation if located close to city parks (e.g., Colding, 2007). We suggest that urban residential yards should be considered part of the strategically developed green infrastructure network of cities. For example, Nielsen et al. (2014) found that increases in the size of the available habitat increases species diversity in urban parks. The ability of relatively small urban habitats to create larger patch sizes and to decrease isolation effects (Nielsen et al., 2014) should be addressed more in urban planning and design, and the targets should be defined in the early phase of the planning process. However, small and fragmented habitats also found in urban environments can augment biodiversity (Soanes et al., 2019) and provide other ecosystem services, and should be considered as part of the green infrastructure.
Carbon sequestration and storage potential does not directly benefit from ecological networks and habitat connectivity, but they certainly benefit from actions taken to preserve existing vegetation and soil with as little disturbance as possible (e.g., Bae and Ryu, 2015). Preserving existing natural habitats is important for biodiversity, but brownfield sites have also been found to harbor biodiversity values that should be preserved as part of the development (Angold et al., 2006). Consequently, preserving existing nature and soil both in and outside the urban development may be the most effective way to enhance the co-benefits of biodiversity conservation and carbon sinks. To achieve maximum CSS impact, Kinnunen et al. (2022) suggest that urban planning should focus on diversifying the vegetation typologies of residential yards as well as integrating novel technologies, such as biochar utilization, to purposefully increase the CSS potential of the residential environment. Further, as urban environments are characterized by relatively intensive disturbances to vegetation and soil compared to their natural counterparts due to construction cycles, preserving existing carbon sinks and storages is important to prevent associated carbon losses from residential landscapes (Bae and Ryu, 2015; Trammell et al., 2017).
In densely built areas, where space for vegetation is limited, solutions are needed to improve the ecological quality of the environment and to provide important ecosystem services, such as heat-island mitigation, health benefits, and stormwater management. Based on our review, many fine-scale improvements to enhance the carbon–biodiversity link can be reached with relatively small efforts in even small green spaces. The same solutions are also likely to enhance other urban ecosystem services (e.g., Larson et al., 2016; Choi et al., 2021). Overall, we suggest that the planning and management of urban vegetation should be optimized to enhance the ecosystem services provision over the whole life cycle of urban green. For example, in newly built areas, a fast-growing pioneer vegetation layer is initially needed to compensate for the respiration and emissions caused by construction works. In the long term, the role of large trees is important. Maintenance has an important role and guidelines should be developed to better address the multiple benefits of urban green. The trade-offs should also be understood, but according to our analyses, trade-offs between biodiversity and carbon benefits are much less likely than co-benefits. The most obvious possible trade-offs are related to the use of non-native plants, which requires more careful consideration and guidelines.
Enhancing the carbon–biodiversity nexus also requires public acceptance (Lampinen et al., 2023). Previous studies have demonstrated that ‘wild’ vegetation in urban areas may elicit positive, but also negative associations, such as ‘unkempt,’ ‘disorganized,’ or ‘messy’ (Gobster and Hull, 2000; Williams and Cary, 2002; Lampinen et al., 2021). Similarly, carbon and biodiversity-wise maintenance practices and leaving plant litter on the ground, for example, may conflict with perceptions of well-maintained urban green spaces (Lampinen et al., 2023). The social acceptability of nature-based solutions can be supported by promoting awareness, effective communication, and resident engagement, such as by providing opportunities to participate in design and management (Lampinen et al., 2022). Residents are not only users of their green spaces but also decision-makers of their yards (García-Antúnez et al., 2023). Previous research has shown that community-led ecological participation, such as community gardening, can increase biodiversity and wellbeing (Dennis and James, 2016).
Improving the carbon–biodiversity nexus requires measures at all planning levels and scales, from city-scale urban planning to neighborhood-level urban design and site-scale landscape design and management. Different planning levels require research-based and applicable knowledge on biodiversity and the carbon outcomes of urban green infrastructure. Although numerous sustainability tools address biodiversity, assessment methods differ widely (Salati et al., 2022). Similarly, carbon calculations and life cycle assessment for urban environments focus on the building sector rather than urban green infrastructure (Kuittinen et al., 2021). Improving biodiversity and the carbon nexus of urban environments requires a more holistic evaluation of the environmental impacts of the construction sector, focusing on the overall quality and synergies of different actions. Implementing biodiversity and carbon smartness necessitates multi-stakeholder engagement of municipalities, businesses, and residents.
Moreover, long-term impacts should be monitored as both carbon and biodiversity benefits require a long-term commitment from the different stakeholders.
For example, auditing and coaching programs of private urban green spaces, with specific criteria, could be launched to support sustainable yard management. Rather than focusing on exact species surveys and carbon measurements, monitoring can focus on the identified drivers and best-management practices that can support biodiversity and carbon sinks in the long term. In the European Union’s Nature Restoration Law proposal (European Parliament, 2024) GIS-based monitoring on canopy and green space cover in urban ecosystems is proposed as means to ensure biodiversity and provision of ecosystem services. In addition, monitoring methods that build upon GIS-approaches such as the 3–30-300 principle (Konijnendijk, 2023) can be utilized to supplement these regional level assessments at the local level.
4.3 Implications for further research
Although existing research has identified the climate and biodiversity contribution of urban vegetation, the synergies between these aspects require further attention. Even though a vast body of literature acknowledges the co-benefits of green infrastructure, studies often remain at a rather theoretical level and lack profound empirical evidence (e.g., Elmqvist et al., 2013; Schwarz et al., 2017; Belaire et al., 2022; Schittko et al., 2022). Moreover, the link between carbon sinks and biodiversity benefits is not often addressed, although the two represent the major environmental challenges of the time. While the biodiversity values of urban gardens are well studied, urban residential yards of apartment buildings have often been overlooked in urban carbon sink and ecological network studies (Davies et al., 2013; Edmondson et al., 2014b). Further practice-oriented research is needed to inform planning and design, and to add knowledge on how to address these challenges and bridge the gap between research and practice. Moreover, monitoring is needed to understand how maintenance practices should be oriented to support the link between carbon sequestration and biodiversity. For better identifying the positive drivers and impacts, efficient techniques for measuring and assessing the benefits of vegetation in urban context should be developed.
In urban areas, the composition of plant species often differs markedly from the natural environment, which may create new kind of biodiversity, sometimes called a novel habitat (e.g., Hobbs et al., 2009); eventually, this may be considered the new normal (Gaertner et al., 2017). Urban areas can provide important habitats for various species, (e.g., Matteson and Langellotto, 2011; Santos et al., 2022), but further understanding is needed regarding how dense residential land use and contemporary yard management affect species interactions and ecological functions. For instance, the European hedgehog (Erinaceus europaeus), once common in urban gardens, has dramatically declined, partly due to changes in gardening practices, although the exact reasons remain unclear (Taucher et al., 2020). Our study has highlighted the potential of nature-based stormwater management solutions, which is clearly a multifunctional solution. Yet the actual application of these solutions in urban areas has large variation, making it difficult to draw clear conclusions on the overall impacts of rainwater management systems in urban yards. Furthermore, a more systematic integration of native vegetation in urban landscape can provide benefits, that still require more studies especially in the face of changing climatic conditions.
Urban high-density residential yards are a typology that has not yet been broadly studied. Typologies can also vary significantly across countries. Most of the literature on yards focuses on low-density residential gardens, but often without specifying the context or the type of garden or vegetation (e.g., Loram et al., 2007; Larson et al., 2016; Tahvonen and Airaksinen, 2018). However, different urban typologies have differences in their potential to enhance both carbon sinks and biodiversity. For example, podium yards built on top of parking facilities are commonly used in urban areas, but their life cycle impacts are not well known. Overall, to develop ecologically sustainable dense residential landscapes, and to be able to compare different approaches, we need a more holistic understanding of carbon flows and biodiversity impacts of various typologies of urban development, over the whole life cycle (Nielsen et al., 2014; Kuittinen et al., 2021).
5 Conclusion
We conclude that despite the limited size and challenging growing conditions, the possibilities to enhance the potential co-benefits of carbon sinks and biodiversity within urban residential yards are versatile. Based on our study, the solutions with the most potential are (i) establishing diverse planting areas with a mixture of woody and herbaceous plants to encourage species richness and complexity, (ii) optimizing the use of space and growth conditions, and, (iii) implementing maintenance practices that consider both carbon and biodiversity aspects. Built ecosystems within urban residential yards can be species-rich and provide habitats as valuable as natural environments if they are designed and managed to support these qualities. Simultaneously, many species-rich vegetation communities can function as effective carbon sinks. Multi-layered vegetation types should be applied in densely built areas, where space is limited. Trees are an important vegetation type that gain more value as they mature, making it essential to invest in species selection and growth condition. Increasing the age diversity of trees can sustain canopy cover and carbon sinks in the long term. Urban grasslands also play a crucial role in both biodiversity and carbon sequestration, especially when managed to support species diversity and soil carbon accumulation, particularly in wet habitats. Furthermore, in densely built urban areas, utilization of rooftops and lightweight vegetated roofs resembling dry meadows can provide a low-carbon solution, offering habitats for species that would otherwise not find conditions to survive. The requirements of urban vegetation, both in terms of space and growing conditions, should be considered in the early phases of planning. Moreover, it is crucial to develop maintenance strategies adapted to various climate conditions that enhance carbon-biodiversity synergies. As cities become denser, the role of residential green grows, and its multifunctional benefits gain importance–for climate, biodiversity, and human well-being alike.
Author contributions
MA: Writing – original draft, Methodology, Formal analysis, Conceptualization, Writing - review and editing. AK: Writing – original draft, Validation, Conceptualization. JL: Writing – original draft, Validation, Conceptualization. RH: Writing – review & editing, Validation, Supervision, Methodology, Funding acquisition. JO: Writing – review & editing, Validation, Supervision.
Funding
The author(s) declare that financial support was received for the research, authorship, and/or publication of this article. This study has been supported by CO-CARBON project, funded by the Strategic Research Council (SRC) established within the Academy of Finland (grant nos. 335202, 336238, 335203).
Conflict of interest
The authors declare that the research was conducted in the absence of any commercial or financial relationships that could be construed as a potential conflict of interest.
Publisher’s note
All claims expressed in this article are solely those of the authors and do not necessarily represent those of their affiliated organizations, or those of the publisher, the editors and the reviewers. Any product that may be evaluated in this article, or claim that may be made by its manufacturer, is not guaranteed or endorsed by the publisher.
References
Adams, B. J., Li, E., Bahlai, C. A., Meineke, E. K., McGlynn, T. P., and Brown, B. V. (2020). Local- and landscape-scale variables shape insect diversity in an urban biodiversity hot spot. Ecol. Appl. 30:e02089. doi: 10.1002/eap.2089
Angold, P. G., Sadler, J. P., Hill, M. O., Pullin, A., Rushton, S., Austin, K., et al. (2006). Biodiversity in urban habitat patches. Sci. Total environ., urban environmental research in the UK: the urban regeneration and the environment (NERC URGENT) Programme and associated studies, No. 360, pp. 196–204.
Ariluoma, M., Leppänen, P.-K., Tahvonen, O., Hautamäki, R., and Ryymin, A. (2023). A framework for a carbon-based urban vegetation typology - a thematic review. Environ. Dev. 47:100899. doi: 10.1016/j.envdev.2023.100899
Ariluoma, M., Ottelin, J., Hautamäki, R., Tuhkanen, E.-M., and Mänttäri, M. (2021). Carbon sequestration and storage potential of urban green in residential yards: a case study from Helsinki. Urban For. Urban Green. 57:126939. doi: 10.1016/j.ufug.2020.126939
Aronson, M. F., Lepczyk, C. A., Evans, K. L., Goddard, M. A., Lerman, S. B., MacIvor, J. S., et al. (2017). Biodiversity in the city: key challenges for urban green space management. Front. Ecol. Environ. 15, 189–196. doi: 10.1002/fee.1480
Aronson, M. F. J., Nilon, C. H., Lepczyk, C. A., Parker, T. S., Warren, P. S., Cilliers, S. S., et al. (2016). Hierarchical filters determine community assembly of urban species pools. Ecology 97, 2952–2963. doi: 10.1002/ecy.1535
Ayers, A. C., and Rehan, S. M. (2021). Supporting bees in cities: how bees are influenced by local and landscape features. Insects 12:128. doi: 10.3390/insects12020128
Bae, J., and Ryu, Y. (2015). Land use and land cover changes explain spatial and temporal variations of the soil organic carbon stocks in a constructed urban park. Landsc. Urban Plan. 136, 57–67. doi: 10.1016/j.landurbplan.2014.11.015
Bardgett, R. D., and Wardle, D. A. (2010). Aboveground-belowground linkages: Biotic interactions, ecosystem processes, and global change, Oxford series in ecology and evolution. Oxford, NY: Oxford University Press.
Barratt, B. I. P., Dickinson, K. J. M., Freeman, C., Porter, S., Johnstone, P. D., Wing, J., et al. (2015). Biodiversity of Coleoptera and other invertebrates in urban gardens: a case study in a New Zealand city. Insect. Conserv. Divers. 8, 428–437. doi: 10.1111/icad.12120
Belaire, J. A., Higgins, C., Zoll, D., Lieberknecht, K., Bixler, R. P., Neff, J. L., et al. (2022). Fine-scale monitoring and mapping of biodiversity and ecosystem services reveals multiple synergies and few tradeoffs in urban green space management. Sci. Total Environ. 849:157801. doi: 10.1016/j.scitotenv.2022.157801
Berthon, K., Thomas, F., and Bekessy, S. (2021). The role of ‘nativeness’ in urban greening to support animal biodiversity. Landsc. Urban Plan. 205:103959. doi: 10.1016/j.landurbplan.2020.103959
Bozorg Chenani, S., Lehvävirta, S., and Häkkinen, T. (2015). Life cycle assessment of layers of green roofs. J. Clean. Prod. 90, 153–162. doi: 10.1016/j.jclepro.2014.11.070
Brachet, A., Schiopu, N., and Clergeau, P. (2019). Biodiversity impact assessment of building’s roofs based on life cycle assessment methods. Build. Environ. 158, 133–144. doi: 10.1016/j.buildenv.2019.04.014
Burghardt, K. T., Tallamy, D. W., and Gregory Shriver, W. (2009). Impact of native plants on bird and butterfly biodiversity in suburban landscapes. Conserv. Biol. 23, 219–224. doi: 10.1111/j.1523-1739.2008.01076.x
Choi, C., Berry, P., and Smith, A. (2021). The climate benefits, co-benefits, and trade-offs of green infrastructure: a systematic literature review. J. Environ. Manag. 291:112583. doi: 10.1016/j.jenvman.2021.112583
Churkina, G., Brown, D. G., and Keoleian, G. (2010). Carbon stored in human settlements: the conterminous United States. Glob. Change Biol. 16, 135–143. doi: 10.1111/j.1365-2486.2009.02002.x
City of Tampere. (2022). Tampereen kaupunkipuulinjaus. Available at: https://www.tampere.fi/luonto-ja-ymparisto/puistot/kaupunkipuut#kaupunkipuulinjaus.
Colding, J. (2007). “Ecological land-use complementation” for building resilience in urban ecosystems. Landsc. Urban Plan. 81, 46–55. doi: 10.1016/j.landurbplan.2006.10.016
Crawley, M. J., Johnston, A. E., Silvertown, J., Dodd, M., De Mazancourt, C., Heard, M. S., et al. (2005). Determinants of species richness in the park grass experiment. Am. Nat. 165, 179–192. doi: 10.1086/427270
Davies, Z. G., Dallimer, M., Edmondson, J. L., Leake, J. R., and Gaston, K. J. (2013). Identifying potential sources of variability between vegetation carbon storage estimates for urban areas. Environ. Pollut. 183, 133–142. doi: 10.1016/j.envpol.2013.06.005
Davies, Z. G., Edmondson, J. L., Heinemeyer, A., Leake, J. R., and Gaston, K. J. (2011). Mapping an urban ecosystem service: quantifying above-ground carbon storage at a city-wide scale. J. Appl. Ecol. 48, 1125–1134. doi: 10.1111/j.1365-2664.2011.02021.x
Delgado-Baquerizo, M., García-Palacios, P., Bradford, M. A., Eldridge, D. J., Berdugo, M., Sáez-Sandino, T., et al. (2023). Biogenic factors explain soil carbon in paired urban and natural ecosystems worldwide. Nat. Clim. Chang. 13, 450–455. doi: 10.1038/s41558-023-01646-z
Dennis, M., and James, P. (2016). User participation in urban green commons: exploring the links between access, voluntarism, biodiversity and well-being. Urban For. Urban Green. 15, 22–31. doi: 10.1016/j.ufug.2015.11.009
Di Marco, M., Watson, J. E. M., Currie, D. J., Possingham, H. P., and Venter, O. (2018). The extent and predictability of the biodiversity–carbon correlation. Ecol. Lett. 21, 365–375. doi: 10.1111/ele.12903
Dorendorf, J., Eschenbach, A., Schmidt, K., and Jensen, K. (2015). Both tree and soil carbon need to be quantified for carbon assessments of cities. Urban For. Urban Green. 14, 447–455. doi: 10.1016/j.ufug.2015.04.005
Easthope, H. (2019). “The politics and practices of apartment living” in The politics and practices of apartment living. ed. H. Easthope (Cheltenham, UK: Edward Elgar Publishing)
Edmondson, J. L., Davies, Z. G., Gaston, K. J., and Leake, J. R. (2014a). Urban cultivation in allotments maintains soil qualities adversely affected by conventional agriculture. J. Appl. Ecol. 51, 880–889. doi: 10.1111/1365-2664.12254
Edmondson, J. L., Davies, Z. G., McCormack, S. A., Gaston, K. J., and Leake, J. R. (2014b). Land-cover effects on soil organic carbon stocks in a European city. Sci. Total Environ. 472, 444–453. doi: 10.1016/j.scitotenv.2013.11.025
Edmondson, J. L., Davies, Z. G., McHugh, N., Gaston, K. J., and Leake, J. R. (2012). Organic carbon hidden in urban ecosystems. Sci. Rep. 2:963. doi: 10.1038/srep00963
Edmondson, J. L., O’Sullivan, O. S., Inger, R., Potter, J., McHugh, N., Gaston, K. J., et al. (2014c). Urban tree effects on soil organic carbon. PLoS One 9:e101872. doi: 10.1371/journal.pone.0101872
Egerer, M., Cecala, J. M., and Cohen, H. (2020). Wild bee conservation within urban gardens and nurseries: effects of local and landscape management. Sustain. For. 12:293. doi: 10.3390/su12010293
Elmqvist, T., Fragkias, M., Goodness, J., Güneralp, B., Marcotullio, P. J., McDonald, R. I., et al. (2013). Urbanization, biodiversity and ecosystem services: Challenges and opportunities. Dordrecht: Springer Netherlands.
Esperon-Rodriguez, M., Ordoñez, C., van Doorn, N. S., Hirons, A., and Messier, C. (2022). Using climate analogues and vulnerability metrics to inform urban tree species selection in a changing climate: The case for Canadian cities. Landscape and Urban Planning 228:104578. doi: 10.1016/j.landurbplan.2022.104578
European Commission. (2021). Communication-from-the-commission-to-the-european-parliament-and-the-council. Brussels: European Commission.
European Parliament. (2024). Nature restoration law proposal. Available at: https://www.europarl.europa.eu/doceo/document/TA-9-2024-0089_EN.pdf.
Fischer, L. K., Neuenkamp, L., Lampinen, J., Tuomi, M., Alday, J. G., Bucharova, A., et al. (2020). Public attitudes toward biodiversity-friendly greenspace management in Europe. Conserv. Lett. 13:e12718. doi: 10.1111/conl.12718
Francini, G., Hui, N., Jumpponen, A., Kotze, D. J., and Setälä, H. (2021). Vegetation type and age matter: how to optimize the provision of ecosystem services in urban parks. Urban For. Urban Green. 66:127392. doi: 10.1016/j.ufug.2021.127392
Francoeur, X. W., Dagenais, D., Paquette, A., Dupras, J., and Messier, C. (2021). Complexifying the urban lawn improves heat mitigation and arthropod biodiversity. Urban For. Urban Green. 60:127007. doi: 10.1016/j.ufug.2021.127007
Gaertner, M., Wilson, J. R. U., Cadotte, M. W., MacIvor, J. S., Zenni, R. D., and Richardson, D. M. (2017). Non-native species in urban environments: patterns, processes, impacts and challenges. Biol. Invasions 19, 3461–3469. doi: 10.1007/s10530-017-1598-7
García-Antúnez, O., Lindgaard, J., Lampinen, J., and Stahl Olafsson, A. (2023). Gardening for wildlife: a mixed-methods exploration of the factors underlying engagement in wildlife-friendly gardening. People Nat. 5, 808–825. doi: 10.1002/pan3.10450
Gerner, E. E., and Sargent, R. D. (2022). Local plant richness predicts bee abundance and diversity in a study of urban residential yards. Basic Appl. Ecol. 58, 64–73. doi: 10.1016/j.baae.2021.11.004
Gillner, S., Korn, S., Hofmann, M., and Roloff, A. (2017). Contrasting strategies for tree species to cope with heat and dry conditions at urban sites. Urban Ecosyst. 20, 853–865. doi: 10.1007/s11252-016-0636-z
Gobster, P. H., and Hull, R. B. (2000). Restoring nature: Perspectives from the social sciences and humanities. Washington, DC: Island Press.
Goddard, M. A., Dougill, A. J., and Benton, T. G. (2010). Scaling up from gardens: biodiversity conservation in urban environments. Trends Ecol. Evol. 25, 90–98. doi: 10.1016/j.tree.2009.07.016
Golubiewski, N. E. (2006). Urbanization increases grassland carbon pools: effects of landscaping in Colorado’s front range. Ecol. Appl. Publ. Ecol. Soc. Am. 16, 555–571. doi: 10.1890/1051-0761(2006)016[0555:uigcpe]2.0.co;2
Gu, C., Crane, J., Hornberger, G., and Carrico, A. (2015). The effects of household management practices on the global warming potential of urban lawns. J. Environ. Manag. 151, 233–242. doi: 10.1016/j.jenvman.2015.01.008
Gunnarsson, B., and Federsel, L. M. (2014). Bumblebees in the city: abundance, species richness and diversity in two urban habitats. J. Insect Conserv. 18, 1185–1191. doi: 10.1007/s10841-014-9729-2
Guo, Z., Zhang, Z., Wu, X., Wang, J., Zhang, P., Ma, D., et al. (2020). Building shading affects the ecosystem service of urban green spaces: carbon capture in street canyons. Ecol. Model. 431:109178. doi: 10.1016/j.ecolmodel.2020.109178
Haase, D., Jänicke, C., and Wellmann, T. (2019). Front and back yard green analysis with subpixel vegetation fractions from earth observation data in a city. Landsc. Urban Plan. 182, 44–54. doi: 10.1016/j.landurbplan.2018.10.010
Hassall, C., and Anderson, S. (2015). Stormwater ponds can contain comparable biodiversity to unmanaged wetlands in urban areas. Hydrobiologia 745, 137–149. doi: 10.1007/s10750-014-2100-5
Hautamäki, R. (2019). Contested and constructed greenery in the compact city: a case study of Helsinki City plan 2016. J. Landsc. Archit. 14, 20–29. doi: 10.1080/18626033.2019.1623543
Havu, M., Kulmala, L., Kolari, P., Vesala, T., Riikonen, A., and Järvi, L. (2022). Carbon sequestration potential of street tree plantings in Helsinki. Biogeosciences 19, 2121–2143. doi: 10.5194/bg-19-2121-2022
Havu, M., Kulmala, L., Lee, H. S., Saranko, O., Soininen, J., Ahongshangbam, J., et al. (2024). CO2 uptake of urban vegetation in a warming Nordic city. Urban For. Urban Green. 94:128261. doi: 10.1016/j.ufug.2024.128261
Hilbert, D. R., Roman, L. A., Koeser, A. K., Vogt, J., and van Doorn, N. S. (2019). Urban tree mortality: a literature review. Arboric. Urban For. 455, 167–200. doi: 10.13140/RG.2.2.25953.15204
Hobbs, R. J., Higgs, E., and Harris, J. A. (2009). Novel ecosystems: implications for conservation and restoration. Trends Ecol. Evol. 24, 599–605. doi: 10.1016/j.tree.2009.05.012
Horn, J., Escobedo, F. J., Hinkle, R., Hostetler, M., and Timilsina, N. (2015). The role of composition, invasives, and maintenance emissions on urban forest carbon stocks. Environ. Manag. 55, 431–442. doi: 10.1007/s00267-014-0400-1
Hostetler, M., and Main, M. (2010). Native landscaping vs. exotic landscaping: what should we recommend? J. Ext. 48:10. doi: 10.34068/joe.48.05.10
Hutyra, L. R., Yoon, B., and Alberti, M. (2011). Terrestrial carbon stocks across a gradient of urbanization: a study of the Seattle, WA region. Glob. Change Biol. 17, 783–797. doi: 10.1111/j.1365-2486.2010.02238.x
Huyler, A., Chappelka, A. H., Prior, S. A., and Somers, G. L. (2014). Influence of aboveground tree biomass, home age, and yard maintenance on soil carbon levels in residential yards. Urban Ecosyst. 17, 787–805. doi: 10.1007/s11252-014-0350-7
Ignatieva, M., Haase, D., Dushkova, D., and Haase, A. (2020). Lawns in cities: from a globalised urban green space phenomenon to sustainable nature-based solutions. Land 9:73. doi: 10.3390/land9030073
Ignatieva, M., and Hedblom, M. (2018). An alternative urban green carpet. Science 362, 148–149. doi: 10.1126/science.aau6974
IPBES (2019). Global assessment report on biodiversity and ecosystem services of the intergovernmental science-policy platform on biodiversity and ecosystem services. Zenodo 2019:7041. doi: 10.5281/zenodo.5657041
IPCC. (2022). Climate change 2022: Impacts, adaptation and vulnerability. Contribution of Working Group II to the Sixth Assessment Report of the Intergovernmental Panel on Climate Change.
Jax, K., and Heink, U. (2015). Searching for the place of biodiversity in the ecosystem services discourse. Biol. Conserv. 191, 198–205. doi: 10.1016/j.biocon.2015.06.032
Juhola, S. (2018). Planning for a green city: the green factor tool. Urban For. Urban Green. 34, 254–258. doi: 10.1016/j.ufug.2018.07.019
Karimi, J. D., Corstanje, R., and Harris, J. A. (2021). Bundling ecosystem services at a high resolution in the UK: trade-offs and synergies in urban landscapes. Landsc. Ecol. 36, 1817–1835. doi: 10.1007/s10980-021-01252-4
Kavehei, E., Iram, N., Rezaei Rashti, M., Jenkins, G. A., Lemckert, C., and Adame, M. F. (2021). Greenhouse gas emissions from stormwater bioretention basins. Ecol. Eng. 159:106120. doi: 10.1016/j.ecoleng.2020.106120
Kinnunen, A., Talvitie, I., Ottelin, J., Heinonen, J., and Junnila, S. (2022). Carbon sequestration and storage potential of urban residential environment – a review. Sustain. Cities Soc. 84:104027. doi: 10.1016/j.scs.2022.104027
Konijnendijk, C. C. (2023). Evidence-based guidelines for greener, healthier, more resilient neighbourhoods: introducing the 3–30–300 rule. J. For. Res. 34, 821–830. doi: 10.1007/s11676-022-01523-z
Kotze, D. J., Ghosh, S., Hui, N., Jumpponen, A., Lee, B. P. Y.-H., Lu, C., et al. (2021). Urbanization minimizes the effects of plant traits on soil provisioned ecosystem services across climatic regions. Glob. Change Biol. 27, 4139–4153. doi: 10.1111/gcb.15717
Kowarik, I. (2011). Novel urban ecosystems, biodiversity, and conservation. Environ. Pollut. 159, 1974–1983. doi: 10.1016/j.envpol.2011.02.022
Kowarik, I., Fischer, L. K., and Kendal, D. (2020). Biodiversity conservation and sustainable urban development. Sustain. For. 12, 1–8. doi: 10.3390/su12124964
Kristancic, A. R., Kuehs, J., Beal Richardson, B., Baudains, C., St Hardy, G. E. J., and Fleming, P. A. (2022). Biodiversity conservation in urban gardens – pets and garden design influence activity of a vulnerable digging mammal. Landsc. Urban Plan. 225:104464. doi: 10.1016/j.landurbplan.2022.104464
Kuittinen, M., Zernicke, C., Slabik, S., and Hafner, A. (2021). How can carbon be stored in the built environment? A review of potential options. Archit. Sci. Rev. 66, 91–107. doi: 10.1080/00038628.2021.1896471
Kyrö, K., Kotze, D. J., Müllner, M. A., Hakala, S., Kondorosy, E., Pajunen, T., et al. (2020). Vegetated roofs in boreal climate support mobile open habitat arthropods, with differentiation between meadow and succulent roofs. Urban Ecosyst. 23, 1239–1252. doi: 10.1007/s11252-020-00978-4
Laine, J., Heinonen, J., and Junnila, S. (2020). Pathways to carbon-neutral cities Prior to a National Policy. Sustain. For. 12:2445. doi: 10.3390/su12062445
Lampinen, J., García-Antúnez, O., Lechner, A. M., Stahl Olafsson, A., Gulsrud, N. M., and Raymond, C. M. (2023). Mapping public support for urban green infrastructure policies across the biodiversity-climate-society -nexus. Landsc. Urban Plan. 239:104856. doi: 10.1016/j.landurbplan.2023.104856
Lampinen, J., García-Antúnez, O., Olafsson, A. S., Kavanagh, K. C., Gulsrud, N. M., and Raymond, C. M. (2022). Envisioning carbon-smart and just urban green infrastructure. Urban For. Urban Green. 75:127682. doi: 10.1016/j.ufug.2022.127682
Lampinen, J., Ruokolainen, K., and Huhta, A.-P. (2015). Urban power line corridors as novel habitats for grassland and alien plant species in South-Western Finland. PLoS One 10:e0142236. doi: 10.1371/journal.pone.0142236
Lampinen, J., Tuomi, M., Fischer, L. K., Neuenkamp, L., Alday, J. G., Bucharova, A., et al. (2021). Acceptance of near-natural greenspace management relates to ecological and socio-cultural assigned values among European urbanites. Basic Appl. Ecol. 50, 119–131. doi: 10.1016/j.baae.2020.10.006
Lange, M., Eisenhauer, N., Sierra, C. A., Bessler, H., Engels, C., Griffiths, R. I., et al. (2015). Plant diversity increases soil microbial activity and soil carbon storage. Nat. Commun. 6:6707. doi: 10.1038/ncomms7707
Larson, K. L., Lerman, S. B., Nelson, K. C., Narango, D. L., Wheeler, M. M., Groffman, P. M., et al. (2022). Examining the potential to expand wildlife-supporting residential yards and gardens. Landsc. Urban Plan. 222:104396. doi: 10.1016/j.landurbplan.2022.104396
Larson, K. L., Nelson, K. C., Samples, S. R., Hall, S. J., Bettez, N., Cavender-Bares, J., et al. (2016). Ecosystem services in managing residential landscapes: priorities, value dimensions, and cross-regional patterns. Urban Ecosyst. 19, 95–113. doi: 10.1007/s11252-015-0477-1
Le Provost, G., Schenk, N. V., Penone, C., Thiele, J., Westphal, C., Allan, E., et al. (2023). The supply of multiple ecosystem services requires biodiversity across spatial scales. Nat. Ecol. Evol. 7, 236–249. doi: 10.1038/s41559-022-01918-5
Lecina-Diaz, J., Alvarez, A., Regos, A., Drapeau, P., Paquette, A., Messier, C., et al. (2018). The positive carbon stocks-biodiversity relationship in forests: co-occurrence and drivers across five subclimates. Ecol. Appl. Publ. Ecol. Soc. Am. 28, 1481–1493. doi: 10.1002/eap.1749
Lerman, S. B., Contosta, A. R., Milam, J., and Bang, C. (2018). To mow or to mow less: Lawn mowing frequency affects bee abundance and diversity in suburban yards. Biol. Conserv. 221, 160–174. doi: 10.1016/j.biocon.2018.01.025
Lerman, S. B., Narango, D. L., Avolio, M. L., Bratt, A. R., Engebretson, J. M., Groffman, P. M., et al. (2021). Residential yard management and landscape cover affect urban bird community diversity across the continental USA. Ecol. Appl. 31:e02455. doi: 10.1002/eap.2455
Lerman, S. B., and Warren, P. S. (2011). The conservation value of residential yards: linking birds and people. Ecol. Appl. Publ. Ecol. Soc. Am. 21, 1327–1339. doi: 10.1890/10-0423.1
Lindén, L., Riikonen, A., Setälä, H., and Yli-Pelkonen, V. (2020). Quantifying carbon stocks in urban parks under cold climate conditions. Urban For. Urban Green. 49:126633. doi: 10.1016/j.ufug.2020.126633
Lindenmayer, D. B., Laurance, W. F., and Franklin, J. F. (2012). Global decline in large old trees. Science 338, 1305–1306. doi: 10.1126/science.1231070
Livesley, S. J., McPherson, E. G., and Calfapietra, C. (2016). The urban Forest and ecosystem services: impacts on urban water, heat, and pollution cycles at the tree, street, and City scale. J. Environ. Qual. 45, 119–124. doi: 10.2134/jeq2015.11.0567
Loram, A., Tratalos, J., Warren, P. H., and Gaston, K. J. (2007). Urban domestic gardens (X): the extent & structure of the resource in five major cities. Landsc. Ecol. 22, 601–615. doi: 10.1007/s10980-006-9051-9
Lorenz, K., and Lal, R. (2015). Managing soil carbon stocks to enhance the resilience of urban ecosystems. Carbon Manag. 6, 35–50. doi: 10.1080/17583004.2015.1071182
Lu, C., Kotze, D. J., and Setälä, H. M. (2021). Evergreen trees stimulate carbon accumulation in urban soils via high root production and slow litter decomposition. Sci. Total Environ. 774:145129. doi: 10.1016/j.scitotenv.2021.145129
Lundquist, M. J., Weisend, M. R., and Kenmore, H. H. (2022). Insect biodiversity in urban tree pit habitats. Urban For. Urban Green. 78:127788. doi: 10.1016/j.ufug.2022.127788
Lüttge, U., and Buckeridge, M. (2020). Trees: structure and function and the challenges of urbanization. Trees 37, 9–16. doi: 10.1007/s00468-020-01964-1
Manso, M., Teotónio, I., Silva, C. M., and Cruz, C. O. (2021). Green roof and green wall benefits and costs: a review of the quantitative evidence. Renew. Sust. Energ. Rev. 135:110111. doi: 10.1016/j.rser.2020.110111
Matteson, K. C., and Langellotto, G. A. (2011). Small scale additions of native plants fail to increase beneficial insect richness in urban gardens. Insect Conserv. Divers. 4, 89–98. doi: 10.1111/j.1752-4598.2010.00103.x
Meineke, E. K., and Frank, S. D. (2018). Water availability drives urban tree growth responses to herbivory and warming. J. Appl. Ecol. 55, 1701–1713. doi: 10.1111/1365-2664.13130
Mimet, A., Kerbiriou, C., Simon, L., Julien, J.-F., and Raymond, R. (2020). Contribution of private gardens to habitat availability, connectivity and conservation of the common pipistrelle in Paris. Landsc. Urban Plan. 193:103671. doi: 10.1016/j.landurbplan.2019.103671
Narango, D. L., Tallamy, D. W., and Marra, P. P. (2017). Native plants improve breeding and foraging habitat for an insectivorous bird. Biol. Conserv. 213, 42–50. doi: 10.1016/j.biocon.2017.06.029
Nielsen, A. B., van den Bosch, M., Maruthaveeran, S., and van den Bosch, C. K. (2014). Species richness in urban parks and its drivers: a review of empirical evidence. Urban Ecosyst. 17, 305–327. doi: 10.1007/s11252-013-0316-1
Nieminen, J. (2021). Luonnonkasvillisuuden jatkokäytön mahdollisuudet. Available at: https://www.tampere.fi/sites/default/files/2022-09/hiedanrannan_luonnonkasvillisuuden_jatkokaytto.pdf.
Nowak, D. J., Greenfield, E. J., Hoehn, R. E., and Lapoint, E. (2013). Carbon storage and sequestration by trees in urban and community areas of the United States. Environ. Pollut. 178, 229–236. doi: 10.1016/j.envpol.2013.03.019
Nowak, D., Stevens, J. C., Sisinni, S. M., and Luley, C. J. (2002). Effects of urban tree management and species selection on atmospheric carbon dioxide. J. Arboric. 28, 113–122. doi: 10.48044/jauf.2002.017
Osheen Singh, K. K. (2019). “Rain garden–a solution to urban flooding: a review” in Sustainable engineering, lecture notes in civil engineering. eds. A. K. Agnihotri, K. Reddy, and A. Bansal (Singapore: Springer), 27–35.
Otoshi, M. D., Bichier, P., and Philpott, S. M. (2015). Local and landscape correlates of spider activity density and species richness in urban gardens. Environ. Entomol. 44, 1043–1051. doi: 10.1093/ee/nvv098
Paker, Y., Yom-Tov, Y., Alon-Mozes, T., and Barnea, A. (2014). The effect of plant richness and urban garden structure on bird species richness, diversity and community structure. Landsc. Urban Plan. 122, 186–195. doi: 10.1016/j.landurbplan.2013.10.005
Partridge, D. R., and Clark, J. A. (2018). Urban green roofs provide habitat for migrating and breeding birds and their arthropod prey. PLOS ONE 13:e0202298. doi: 10.1371/journal.pone.0202298
Pataki, D. E. (2011). Coupling biogeochemical cycles in urban environments: ecosystem services, green solutions, and misconceptions. Front. Ecol. Environ. 9, 27–36. doi: 10.1890/090220
Pille, L., and Säumel, I. (2021). The water-sensitive city meets biodiversity: habitat services of rain water management measures in highly urbanized landscapes. Ecol. Soc. 26:art23. doi: 10.5751/ES-12386-260223
Pithon, J. A., Duflot, R., Beaujouan, V., Jagaille, M., Pain, G., and Daniel, H. (2021). Grasslands provide diverse opportunities for bird species along an urban-rural gradient. Urban Ecosyst. 24, 1281–1294. doi: 10.1007/s11252-021-01114-6
Plascencia, M., and Philpott, S. M. (2017). Floral abundance, richness, and spatial distribution drive urban garden bee communities. Bull. Entomol. Res. 107, 658–667. doi: 10.1017/S0007485317000153
Poeplau, C., Marstorp, H., Thored, K., and Kätterer, T. (2016). Effect of grassland cutting frequency on soil carbon storage – a case study on public lawns in three Swedish cities. Soil 2, 175–184. doi: 10.5194/soil-2-175-2016
Pouyat, R. V., Yesilonis, I. D., and Golubiewski, N. E. (2009). A comparison of soil organic carbon stocks between residential turf grass and native soil. Urban Ecosyst. 12, 45–62. doi: 10.1007/s11252-008-0059-6
Proske, A., Lokatis, S., and Rolff, J. (2022). Impact of mowing frequency on arthropod abundance and diversity in urban habitats: a meta-analysis. Urban For. Urban Green. 76:127714. doi: 10.1016/j.ufug.2022.127714
Raymond, C. M., Frantzeskaki, N., Kabisch, N., Berry, P., Breil, M., Nita, M. R., et al. (2017). A framework for assessing and implementing the co-benefits of nature-based solutions in urban areas. Environ. Sci. Pol. 77, 15–24. doi: 10.1016/j.envsci.2017.07.008
Rega-Brodsky, C. C., Aronson, M. F. J., Piana, M. R., Carpenter, E. S., Hahs, A. K., Herrera-Montes, A., et al. (2022). Urban biodiversity: state of the science and future directions. Urban Ecosyst. 25, 1083–1096. doi: 10.1007/s11252-022-01207-w
Richards, D. R., and Belcher, R. N. (2020). Global changes in urban vegetation cover. Remote Sens. 12:23. doi: 10.3390/rs12010023
Riikonen, A., Pumpanen, J., Mäki, M., and Nikinmaa, E. (2017). High carbon losses from established growing sites delay the carbon sequestration benefits of street tree plantings – a case study in Helsinki, Finland. Urban For. Urban Green. 26, 85–94. doi: 10.1016/j.ufug.2017.04.004
Roloff, A., Korn, S., and Gillner, S. (2009). The climate-species-matrix to select tree species for urban habitats considering climate change. Urban For. Urban Green. 8, 295–308. doi: 10.1016/j.ufug.2009.08.002
Rousseau, J. S., Savard, J.-P. L., and Titman, R. (2015). Shrub-nesting birds in urban habitats: their abundance and association with vegetation. Urban Ecosyst. 18, 871–884. doi: 10.1007/s11252-014-0434-4
RSPB. (2023). The RSPB wildlife charity: Nature reserves & wildlife conservation [WWW document]. The RSPB. Available at: https://www.rspb.org.uk/ (Accessed May 1, 2023).
Sæbø, A., Benedikz, T., and Randrup, T. B. (2003). Selection of trees for urban forestry in the Nordic countries. Urban For. Urban Green. 2, 101–114. doi: 10.1078/1618-8667-00027
Salati, M., Bragança, L., and Mateus, R. (2022). Sustainability assessment on an urban scale: context, challenges, and Most relevant indicators. Appl. Syst. Innov. 5:41. doi: 10.3390/asi5020041
Santos, M., Moreira, H., Cabral, J. A., Gabriel, R., Teixeira, A., Bastos, R., et al. (2022). Contribution of home gardens to sustainable development: perspectives from a supported opinion essay. Int. J. Environ. Res. Public Health 19:13715. doi: 10.3390/ijerph192013715
Sapkota, M., Young, J., Coldren, C., Slaughter, L., and Longing, S. (2020). Soil physiochemical properties and carbon sequestration of urban landscapes in Lubbock, TX, USA. Urban For. Urban Green. 56:126847. doi: 10.1016/j.ufug.2020.126847
Sarzhanov, D. A., Vasenev, V. I., Vasenev, I. I., Sotnikova, Y. L., Ryzhkov, O. V., and Morin, T. (2017). Carbon stocks and CO2 emissions of urban and natural soils in central Chernozemic region of Russia. Catena 158, 131–140. doi: 10.1016/j.catena.2017.06.021
Schittko, C., Onandia, G., Bernard-Verdier, M., Heger, T., Jeschke, J. M., Kowarik, I., et al. (2022). Biodiversity maintains soil multifunctionality and soil organic carbon in novel urban ecosystems. J. Ecol. 110, 916–934. doi: 10.1111/1365-2745.13852
Schlaepfer, M. A., Guinaudeau, B. P., Martin, P., and Wyler, N. (2020). Quantifying the contributions of native and non-native trees to a city’s biodiversity and ecosystem services. Urban For. Urban Green. 56:126861. doi: 10.1016/j.ufug.2020.126861
Schmidt, K., and Walz, A. (2021). Ecosystem-based adaptation to climate change through residential urban green structures: co-benefits to thermal comfort, biodiversity, carbon storage and social interaction. One Ecosyst. 6:e65706. doi: 10.3897/oneeco.6.e65706
Schmitt-Harsh, M., Mincey, S. K., Patterson, M., Fischer, B. C., and Evans, T. P. (2013). Private residential urban forest structure and carbon storage in a moderate-sized urban area in the Midwest, United States. Urban For. Urban Green. 12, 454–463. doi: 10.1016/j.ufug.2013.07.007
Schwarz, N., Moretti, M., Bugalho, M. N., Davies, Z. G., Haase, D., Hack, J., et al. (2017). Understanding biodiversity-ecosystem service relationships in urban areas: a comprehensive literature review. Ecosyst. Serv. 27, 161–171. doi: 10.1016/j.ecoser.2017.08.014
Scolaro, T. P., and Ghisi, E. (2022). Life cycle assessment of green roofs: a literature review of layers materials and purposes. Sci. Total Environ. 829:154650. doi: 10.1016/j.scitotenv.2022.154650
Seitz, B., Buchholz, S., Kowarik, I., Herrmann, J., Neuerburg, L., Wendler, J., et al. (2022). Land sharing between cultivated and wild plants: urban gardens as hotspots for plant diversity in cities. Urban Ecosyst. 25, 927–939. doi: 10.1007/s11252-021-01198-0
Setälä, H. M., Francini, G., Allen, J. A., Hui, N., Jumpponen, A., and Kotze, D. J. (2016). Vegetation type and age drive changes in soil properties, nitrogen, and carbon sequestration in urban parks under cold climate. Front. Ecol. Evol. 4:93. doi: 10.3389/fevo.2016.00093
Shafique, M., Xue, X., and Luo, X. (2020). An overview of carbon sequestration of green roofs in urban areas. Urban For. Urban Green. 47:126515. doi: 10.1016/j.ufug.2019.126515
Shchepeleva, A. S., Vasenev, V. I., Mazirov, I. M., Vasenev, I. I., Prokhorov, I. S., and Gosse, D. D. (2017). Changes of soil organic carbon stocks and CO2 emissions at the early stages of urban turf grasses’ development. Urban Ecosyst. 20, 309–321. doi: 10.1007/s11252-016-0594-5
Sjöman, H., Morgenroth, J., Sjöman, J. D., Sæbø, A., and Kowarik, I. (2016). Diversification of the urban forest—can we afford to exclude exotic tree species? Urban For. Urban Green. 18, 237–241. doi: 10.1016/j.ufug.2016.06.011
Sjöman, H., and Watkins, J. H. R. (2020). What do we know about the origin of our urban trees? – a north European perspective. Urban For. Urban Green. 56:126879. doi: 10.1016/j.ufug.2020.126879
Snyder, H. (2019). Literature review as a research methodology: an overview and guidelines. J. Bus. Res. 104, 333–339. doi: 10.1016/j.jbusres.2019.07.039
Soanes, K., Sievers, M., Chee, Y. E., Williams, N. S. G., Bhardwaj, M., Marshall, A. J., et al. (2019). Correcting common misconceptions to inspire conservation action in urban environments. Conserv. Biol. 33, 300–306. doi: 10.1111/cobi.13193
Steinaker, D. F., and Wilson, S. D. (2008). Phenology of fine roots and leaves in forest and grassland. J. Ecol. 96, 1222–1229. doi: 10.1111/j.1365-2745.2008.01439.x
Tahvonen, O., and Airaksinen, M. (2018). Low-density housing in sustainable urban planning – scaling down to private gardens by using the green infrastructure concept. Land Use Policy 75, 478–485. doi: 10.1016/j.landusepol.2018.04.017
Taucher, A. L., Gloor, S., Dietrich, A., Geiger, M., Hegglin, D., and Bontadina, F. (2020). Decline in distribution and abundance: urban hedgehogs under pressure. Anim. Open Access J. MDPI 10:1606. doi: 10.3390/ani10091606
Thompson, K., Hodgson, J. G., Smith, R. M., Warren, P. H., and Gaston, K. J. (2004). Urban domestic gardens (III): composition and diversity of lawn floras. J. Veg. Sci. 15, 373–378. doi: 10.1111/j.1654-1103.2004.tb02274.x
Thompson, G. L., and Kao-Kniffin, J. (2019). Urban grassland management implications for soil C and N dynamics: a microbial perspective. Front. Ecol. Evol. 7:7. doi: 10.3389/fevo.2019.00315
Torraco, R. J. (2005). Writing integrative literature reviews: guidelines and examples. Hum. Resour. Dev. Rev. 4, 356–367. doi: 10.1177/1534484305278283
Trammell, T. L. E., Pouyat, R. V., Carreiro, M. M., and Yesilonis, I. (2017). Drivers of soil and tree carbon dynamics in urban residential lawns: a modeling approach. Ecol. Appl. 27, 991–1000. doi: 10.1002/eap.1502
Tresch, S., Frey, D., Bayon, R.-C. L., Mäder, P., Stehle, B., Fliessbach, A., et al. (2019a). Direct and indirect effects of urban gardening on aboveground and belowground diversity influencing soil multifunctionality. Sci. Rep. 9:9769. doi: 10.1038/s41598-019-46024-y
Tresch, S., Frey, D., Le Bayon, R.-C., Zanetta, A., Rasche, F., Fliessbach, A., et al. (2019b). Litter decomposition driven by soil fauna, plant diversity and soil management in urban gardens. Sci. Total Environ. 658, 1614–1629. doi: 10.1016/j.scitotenv.2018.12.235
Van Den Berge, S., Vangansbeke, P., Baeten, L., Vanhellemont, M., Vanneste, T., De Mil, T., et al. (2021). Biomass increment and carbon sequestration in hedgerow-grown trees. Dendrochronologia. 70, 125894. doi: 10.1016/j.dendro.2021.125894
Van Helden, B. E., Close, P. G., and Steven, R. (2020). Mammal conservation in a changing world: can urban gardens play a role? Urban Ecosyst. 23, 555–567. doi: 10.1007/s11252-020-00935-1
Vasenev, V. I., Stoorvogel, J. J., and Vasenev, I. I. (2013). Urban soil organic carbon and its spatial heterogeneity in comparison with natural and agricultural areas in the Moscow region. Catena 107, 96–102. doi: 10.1016/j.catena.2013.02.009
Wang, M., Sun, C., and Zhang, D. (2023). Opportunities and challenges in green stormwater infrastructure (GSI): a comprehensive and bibliometric review of ecosystem services from 2000 to 2021. Environ. Res. 236:116701. doi: 10.1016/j.envres.2023.116701
Watson, C. J., Carignan-Guillemette, L., Turcotte, C., Maire, V., and Proulx, R. (2020). Ecological and economic benefits of low-intensity urban lawn management. J. Appl. Ecol. 57, 436–446. doi: 10.1111/1365-2664.13542
Wetherbee, R., Birkemoe, T., Burner, R. C., and Sverdrup-Thygeson, A. (2021). Veteran trees have divergent effects on beetle diversity and wood decomposition. PLoS One 16:e0248756. doi: 10.1371/journal.pone.0248756
Whittemore, R., and Knafl, K. (2005). The integrative review: updated methodology. J. Adv. Nurs. 52, 546–553. doi: 10.1111/j.1365-2648.2005.03621.x
Widows, S. A., and Drake, D. (2014). Evaluating the National Wildlife Federation’s certified wildlife habitat™ program. Landsc. Urban Plan. 129, 32–43. doi: 10.1016/j.landurbplan.2014.05.005
Keywords: carbon sinks, biodiversity enhancement, urban residential yards, landscape design, urban green infrastructure, urban vegetation
Citation: Ariluoma M, Kinnunen A, Lampinen J, Hautamäki R and Ottelin J (2024) Optimizing the co-benefits of biodiversity and carbon sinks in urban residential yards. Front. Sustain. Cities. 6:1327614. doi: 10.3389/frsc.2024.1327614
Edited by:
Rieke Hansen, Hochschule Geisenheim University, GermanyReviewed by:
Ieva Misiune, Vilnius University, LithuaniaAnne-Kathrin Schneider, Johann Heinrich von Thünen Institute, Germany
Copyright © 2024 Ariluoma, Kinnunen, Lampinen, Hautamäki and Ottelin. This is an open-access article distributed under the terms of the Creative Commons Attribution License (CC BY). The use, distribution or reproduction in other forums is permitted, provided the original author(s) and the copyright owner(s) are credited and that the original publication in this journal is cited, in accordance with accepted academic practice. No use, distribution or reproduction is permitted which does not comply with these terms.
*Correspondence: Mari Ariluoma, mari.ariluoma@aalto.fi