- 1Centro de Estudios Territoriales Ambientales y Sociales (CETAS-Universidad Nacional de Jujuy), San Salvador de Jujuy, Jujuy, Argentina
- 2Instituto de Ecorregiones Andinas (INECOA), Jujuy, Argentina
- 3Instituto de Ecología Regional (IER), Universidad Nacional de Tucumán (UNT)-Consejo Nacional de Investigaciones Científicas y Técnicas (CONICET), Tucumán, Argentina
- 4Facultad de Ciencias Naturales e Instituto Miguel Lillo, Universidad Nacional de Tucumán (UNT), Tucumán, Argentina
Human population and cities are growing fast, with a concomitant modification of the land surface. Urbanization is driving biodiversity loss and biological homogenization, which impacts human wellbeing. In this study, we evaluated the influence of urbanization on flower visitor assemblage using an interaction network approach. We assessed the effect of different variables at the local and landscape scales on community parameters and network metrics along a gradient of urbanization located in a subtropical montane Yungas forest. We found that local variables affected the richness of flower visitors, which increased with greater flower coverage, high stability of floral resources, and the proportion of exotic plants. Moreover, local variables affected the diversity, nestedness (NODF), and specialization (H2) of the interaction network. Landscape variables, such as altitude and proportion of impervious surface (a proxy of urbanization), affected both the richness of flower visitors and specialization. The effect of urbanization on the richness of flower visitors differed across the altitudinal gradient, with higher impact at higher altitudes. In conclusion, our results indicate that local and landscape variables affect community parameters and the structure of plant-flower visitor networks to different extents and strengths.
Introduction
The field of complex ecological networks has grown in the last two decades [recently reviewed by Guimarães (2020)]. Network theory has become an important tool in community ecology as it provides an efficient representation and characterization of temporal and spatial patterns of community dynamics and the structure of ecological systems (Schwarz et al., 2020; Resasco et al., 2021). Ecological networks help to understand how communities respond to ongoing global environmental change (Schleuning et al., 2016; Tylianakis and Morris, 2017). Studies on network properties at local and landscape scales showed that habitat modification impacts system stability at the network level (Marín et al., 2019) and outweighs the impact on species diversity (Tylianakis et al., 2007; Weiner et al., 2014). For example, habitat modification impacts both species themselves and their interactions, and it has been shown that loss of interactions precedes species loss (Valiente-Banuet et al., 2014). Thus, network analysis often provides a good assessment of the possible effects of changes in habitat on community stability (Kaiser-Bunbury et al., 2010; Sabatino et al., 2010), and the resilience of ecosystems to landscape modifications (Montoya, 2008).
One of the outstanding features of the Anthropocene is that most of the world's population lives in cities. By 2050, current projections indicate that two out of three world inhabitants will live in urban areas and that population growth during this period will happen entirely in cities (United Nations, 2019). Urbanization, a process that manifests through rapidly changing human population and land cover, is generally studied across urban–rural gradients, analyzing changes in plants and animals along a transect from the inner city to surrounding, less-altered ecosystems (McKinney, 2002). The impacts of urbanization on biodiversity occur mainly through the reduction in natural land cover, and also through fragmentation, the introduction of non-native species, and increased temperature (McDonald et al., 2013). Thus, urbanization is considered a major driving force of biodiversity loss and biological homogenization (Savard et al., 2000; Gupta, 2002; McKinney, 2002). In this context, there is recent and increased concern over the conservation of flower visitors in urban environments, considering that pollination is one of the major contributions of nature to human wellbeing (Díaz et al., 2006; Hall et al., 2017; Knight et al., 2018). We studied how the interactions between flower visitors and flowers are structured along an urbanization gradient.
Studies in the past decade revealed that bee community responses to urbanization are often mediated by local and landscape habitat conditions (Quistberg et al., 2016; Theodorou et al., 2020). At the local scale, floral resources are important for flower visitors, as the richness and cover of floral species (Ebeling et al., 2008; Grundel et al., 2010; McCune et al., 2019), constancy of floral resources over time (Stewart and Waitayachart, 2020), and the presence of abundant exotic plants in urban areas (Moroń et al., 2009; Martins et al., 2013; Fenesi et al., 2015) determine the richness of the flower visitor community. At the landscape scale, the most widely studied effects related to urban expansion are those caused by increased impervious surface (e.g., Geslin et al., 2016) and heat (e.g., McCune et al., 2019). In turn, the proportion of impervious surfaces is strongly associated with the composition of bee communities (Burdine and Mccluney, 2019). Urban areas are characterized by being significantly warmer than their surroundings, which can have a strong impact on ectotherms, such as insects (Briere et al., 1999), and thus on their survival rate and fecundity (Sales et al., 2018). Additionally, altitude has a negative effect on the total richness of flower visitors (Bates et al., 2011).
Nevertheless, the effects of urbanization on insects in general, and flower visitors in particular, remain ambiguous and are not completely understood (Threlfall et al., 2015). Existing studies report positive and negative effects, although the latter seems to prevail (McKinney, 2006, 2008). Some studies have found a decrease in the diversity and abundance of pollinating insects from a rural to urban gradient (Ahrne et al., 2009). However, the response to urbanization varies among species (Rodrigues et al., 1993; Osborne et al., 2008), and some bees are equally, or more abundant, in natural vegetation fragments within urban landscapes compared to extensive natural areas (McFrederick and LeBuhn, 2006; Osborne et al., 2008; Wenzel et al., 2020).
Assessing whether the impact of urbanization at the species level scales up to the community level is critical for the conservation of flower visitors and the ecosystem services they provide (Shwartz et al., 2013; Potter and LeBuhn, 2015). One way to assess the community stability in urban areas lies in the use of interaction networks between plants and their flower visitors (Baldock et al., 2015; Kaiser-Bunbury and Blüthgen, 2015), which allow a better understanding of the structure and dynamics of these systems (Vizentin-Bugoni et al., 2014; Knight et al., 2018). To achieve such an assessment, we propose to evaluate changes in the structure of interaction networks at the community level along an urbanization gradient, both at the local and the landscape scales. In particular, we looked at the effect of (1) local urban variables, such as the proportion of exotics plants, flower coverage, and temporal variation of floral coverage, and (2) variation in landscape variables, such as impervious surface (areas covered by buildings), altitude, and land surface temperature, on the structure of plant-flower visitor networks.
Methods
Study area and sites
This study was done in a natural–rural–urban gradient in the Yungas ecoregion of northwestern Argentina, which represents the southernmost extension of the neotropical Andean forest (Oyarzabal et al., 2018; Figure 1). It is one of the most diverse ecoregions in the country and has great importance in terms of the provision of ecosystem services (Malizia et al., 2012). In Argentina, the altitudinal gradient of the Yungas extends between 400 and 2,500 m a.s.l., along which it encompasses three main environmental forest tiers: piedmont (400–900 m a.s.l.), montane (900–1,600 m.a.s.l.), and montane cloud forest (1,600–2,500 m a.s.l.; Brown et al., 1985). Most of the larger urban developments are established in the montane forest valleys. The study area has a subtropical climate, where rainfall occurs mostly during the southern hemisphere summer (ranging between 600 and 2,000 mm annually) with a marked dry season (April–October), and a mean annual temperature that decreases up the altitudinal gradient from 21.5 to 11.5°C.
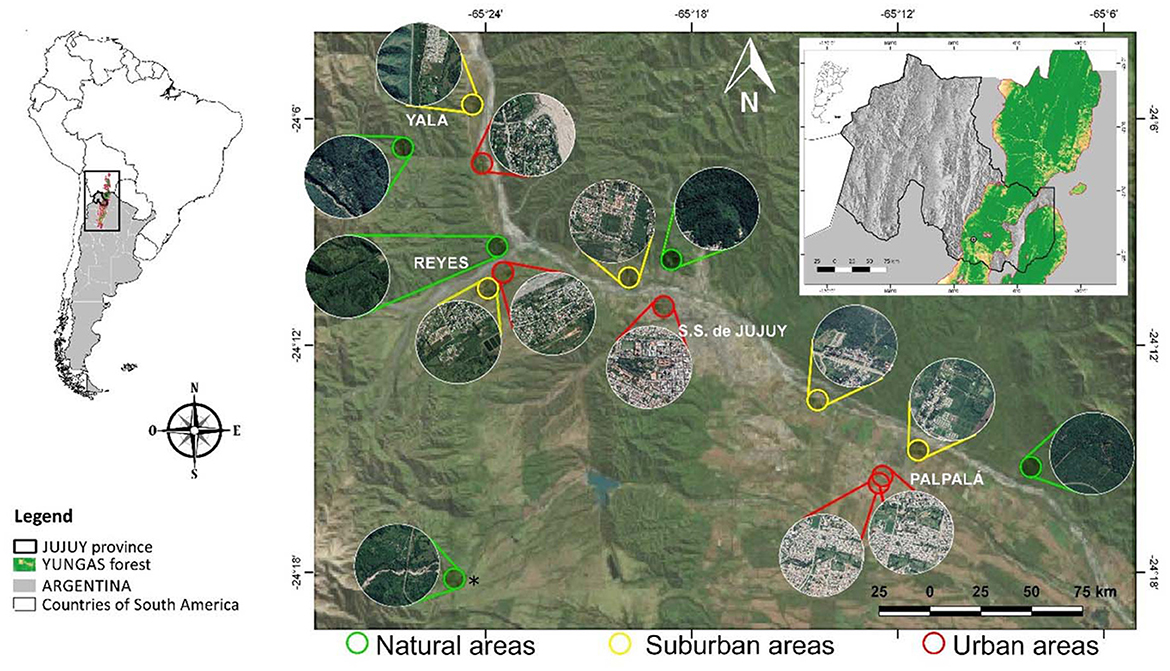
Figure 1. Distribution of the Yungas forest ecoregion in South America (left) and focused on Jujuy province (right). Circles represent 15 sampling sites distributed within the Yungas ecoregion, showing a zoom-in of each. Small circles represent a 500-m ratio from the middle point of each site, where the landscape variables were recorded. Bigger circles represent a zoom-in of each site to visualize the degree of urbanization. Reference: urban sites (red circles); suburban sites (yellow circles), and natural sites nearby (green circles). (*) Natural site located in the Los Paños locality but belonging to one of the Palpalá gradients.
Jujuy province is the northernmost province of Argentina; it has a low degree of urbanization in general, but it has experienced a 30% population increase from 2001 to 2022 (from 611.888 to 797.955 inhabitants). The most populated area is the capital city, San Salvador de Jujuy (The National Institute of Statistics Censuses, 2022), which is located at 1,200 m a.s.l. The natural environment is represented by a montane forest. However, urban developments have extended to the montane cloud forest in recent years. Other main cities of Jujuy province grew spatially and demographically, from a population of 82,000 inhabitants in the 1970's (National Population, Families and Housing Census, 1970)1 to a current population of 335,406 inhabitants (Permanent Household Survey, fourth quarter of 2014). The main cause of this development lies in a double migratory process in search of work, both from rural areas of the province and from neighboring countries, mainly Bolivia (Boldrini and Malizia, 2017).
We selected five different urbanization gradients in the province of Jujuy, each consisting of three urbanization categories (hereafter called sites), classified according to the percentage of impervious surface in a 500-m radius from the center of the site with a GIS analysis technique used by Owen et al. (2006). Categories of sites were as follows: (1) Urban: 58.6% of the area, on average, occupied by buildings and houses (ranging from 33.1 to 86%), sampling was done in parks; (2) Suburban: areas nearby cities and residential sites, where buildings occupied 30.2% of the area, on average (ranging from 22 to 36.3%), most of the buildings are houses with backyards and sampling was done in vacant lots; (3) Natural: areas with Yungas forest, with < 1.1% covered by buildings (ranging from 0 to 4.4%), and these sites were mostly natural reserves often used for tourism. The sites within each urbanization gradient were separated by at least 1.2 km from each other (Figure 1, Supplementary Table 1). Urbanization gradients were located in different cities, which was the “region” category, and were added as a random effect in the models.
Data collection
At each site, we delimited a one-hectare plot, where we observed plants to record flower visitors and measured local plant variables (see Local and landscape variables). Sampling plant-flower visitor interactions consisted of observation of a focal plant for 5 min and recording all plant visitors that made direct contact with the flower's reproductive parts. We aimed at completing three observation periods for each plant species, but in some cases, we did not find enough individuals. Data were collected between 9 am and 12:30 pm and between 1:30 pm and 5 pm by the same observer throughout the study. At each site, we recorded interactions with all flowering plants present in the plot, including herbaceous plants, shrubs, or trees. For the latter, we only included branches up to 1.80 m high.
When possible, we captured all insect flower visitors before they flew away, using entomological aspirators. Afterward, we labeled all captured individuals and took them to the laboratory for further identification by experts. Apoidea specimens were identified to the lowest taxonomic category possible with the help of experts, while most specimens from Coleoptera, Diptera, Vespidae, and Formicidae were identified as morphospecies. We also identified plants with the help of experts when needed (see Acknowledgments section). To ensure that our sampling included a representative percentage of the flower visitor community, we calculated the sampling completeness of the different levels of urbanization with the Chao1 estimator (Chao, 1987), following Chacoff et al. (2012). We estimated that we recorded 66% of flower visitors in natural areas, 75% in suburban areas, and 68% in urban areas, which mean that our sampling is quite representative of the total flower visitor community in those sites (Amado De Santis and Chacoff, 2020; Supplementary Table 3).
Fieldwork was done during the peak flowering time for three consecutive seasons (2015–2018), between September and February (spring and summer) over five urbanization gradients, and each site was visited between four and six times (Supplementary material). Two of the gradients were sampled during two seasons, and three were sampled in only one season (Supplementary Table 2). This sampling totaled 21 networks.
Community and network metrics
We built a quantitative plant-flower visitor network for each site. For those sites that were assessed during two flowering seasons, we built a separate network for each year (we evaluated the influence of the different seasons and we did not find any effect). For each network, we extracted community and network metrics to analyze. The community metrics include the following: (1) richness (r) is the total number of flower visitor species; (2) Shannon diversity index (d) accounts for both abundance and evenness of the species present; network metrics include the following: (3) specialization index (H2) measures the level of specialization in the entire network, which ranges from zero (extreme generalization) to one (extreme specialization), and is derived from the Shannon index. H2 is not dependent on sample size, and therefore it is robust to changes in sampling intensity and to the number of interacting species in the network (Blüthgen et al., 2006); (4) connectance (c) describes the ratio between the total number of realized (observed) links in a network and the theoretical maximum number of possible links if all visitors and plants were connected (Jordano, 1987); finally, (5) nestedness (NODF) is a network metric that indicates how low-degree species (also called specialists) interact with the subsets of the species with which high-degree species (generalists) interact. Values range from 0 (not nested at all) to 100 (perfect nesting; Almeida-Neto et al., 2008).
Local and landscape variables
We measured environmental variables at the local and landscape scales, which were calculated for each site. Local variables include the following: (1) Flower cover: for each day of sampling, we calculated the percentage of flower cover in 10 random plots of 4 × 4 m, distributed within a 1-hectare plot, each time we visited the site (ranging from 4 to 6 times, Supplementary Table 2) and then we averaged those values to get one value per site. (2) Temporal variation of flower cover: we determined the temporal coefficient of variation (CV) of flower cover at each site throughout the season with the following formula: CVfc= (σ/χ)*100 (χ: absolute mean value of flower cover throughout the flowering season and σ: standard deviation of flower cover), as a measure of the temporal stability of the flower resources offered at each site. (3) Proportion of exotic plant species was calculated per site in relation to the total number of native and exotic plant species.
Landscape variables
These variables were measured using a GIS analysis. (1) Percentage of impervious surface (area with buildings, paved roads, sidewalks, etc.) and natural vegetation cover: it is calculated for each site in a 500-m radius by considering four categories, namely dense and shrub vegetations, natural vegetation (with trees and shrubs), and impervious surface (area with buildings). (2) Ground surface temperature: the maximum ground surface temperature was extracted from MODIS images (Wan et al., 2015), from a series of values obtained from 2015 to 2018. Then, because the available data are reported on a per-year basis, and each season encompasses 2 years (September–March), we averaged the two values to obtain one mean value per season per site. (3) Distance to a natural area: natural vegetation could provide extra resources to flower visitors and thus influence their abundance and richness in urban areas. This variable was calculated as the distance in meters from the middle point of each site to the center of the nearest sector with at least 70% of forest cover in a 500-m radius. (4) Distance to a water body is calculated as the distance from the center of each site to the edge of a lotic water body (e.g., a river), and (5) Altitude is obtained with a GPS.
To avoid multiple tests, before the GLMM, we correlated the local and landscape variables by using Pearson's correlation test (Quinn and Keough, 2002). Variables that were highly correlated (r > 0.70) were discarded from subsequent analysis. At the local scale, we kept the three original variables because their coefficient of correlation was < 0.70. At the landscape scale, we discarded distance to vegetation and a water body as they were highly positively correlated with urbanization. Therefore, we included only the percentage of impervious surface (urbanization), altitude, and surface temperature. We also discarded natural vegetation cover because it was highly negatively correlated with urbanization (Supplementary Table 8).
Statistical analyses
To test the effect of local and landscape-scale variables over the response variables—community and network metrics listed above—we standardized each explanatory variable by subtracting the mean and dividing by 2*SD (Gelman and Hill, 2007). We report the range and mean of the original values in Supplementary Table 4. We used generalized linear mixed models (GLMMs; Crawley, 2007; Logan, 2009; Zuur et al., 2009) to test local and landscape effects separately including three-way interactions. One of the models included community and network metrics as response variables and local-scale variables as explanatory variables. The other model included the same response variables and the landscape-scale variables as explanatory variables (Supplementary Table 5). For frequency data (richness), we used a negative binomial distribution to model the stochastic part of the model, because the data showed over-dispersion. For continuous response variables (Shannon diversity index, specialization index, connectance, and NODF), we used a Gaussian distribution.
We considered each network as an independent observation and thus had 21 networks for the analysis. To ensure that the two gradients (six sites) that were measured during two flowering seasons (Supplementary Table 2) could be considered independent samples, we set season as a random nested factor (1|Region/Season). Because the overall results were not affected by this, we considered that it was justified to analyze all networks as independent observations. This avoided an unbalanced design and the need for more complex models. Only the region was kept as a random effect due to the structure of the sampling design.
For local and landscape effects, we selected the best model (those models that better adjust to our data) by using information-theoretic procedures (Burnham and Anderson, 2004). We considered models with all possible combinations of predictor variables. We calculated Akaike's information criterion corrected for a small sample size (AICc) for each model (Burnham and Anderson, 2004). We compared models based on ΔAICc, which is the difference between the lowest AICc value (e.g., best of suitable models), and AICc for all other models; when the difference between the best models was >2, we selected the model with the lowest AICc, but when the difference was < 2, we used a weighted model selection criterion. The criterion to select the best or the weighted models was based on the AICc weight of a model (wi), which represents the relative likelihood that the specific model is better than all the other models (Burnham and Anderson, 2004). We performed a multiple model selection for each model (Supplementary Tables 6, 7) and, if the model had wi > 0.70, it was considered the best model. If no model reached that value, we calculated the 95% confidence interval limits (CL) for parameter estimates. The CL of the variables that excluded zero was considered significant. Because models of richness at the local and landscape scales exhibited overdispersion (c ˆ = 1.38; c ˆ = 2.03, respectively), we adjusted standard errors and used QAICc for model selection (Burnham and Anderson, 2004). Statistical analyses were done using lme4 (Bates et al., 2015) and MuMIn packages (Barton, 2019) for R software, version 3.5.3 (R Core Team, 2019).
Results
Overall characteristics of pollination networks
We recorded a total of 197 morphospecies of flower-visiting insects, of which 37 were identified at the species level and the rest at the genus, family, and superfamily levels. Hereafter, for the sake of simplification, we will talk about species for both species and morphospecies. We also recorded 121 species of plants; thus, the total number of species in the network was 318 (Supplementary Tables 9, 10). We documented a total of 3,310 visits; of which, 971 visits were recorded in natural areas, 1,120 in suburban areas, and 1,219 in urban areas (Figure 2). We found a total of 107 species of flower visitors in natural and suburban areas and 96 in urban areas. We captured 75 bee species (38% of the total recorded), 64 beetle species (32% of the total recorded), 31 fly species (15% of the total recorded), 14 wasp species (7% of the total recorded), and 12 ants species (6% of the total recorded). Within the bee group, 31 species belonged to Apidae, 19 to Megachilidae, 16 to Halictidae, 3 to Colletidae, and 3 to Andrenidae.
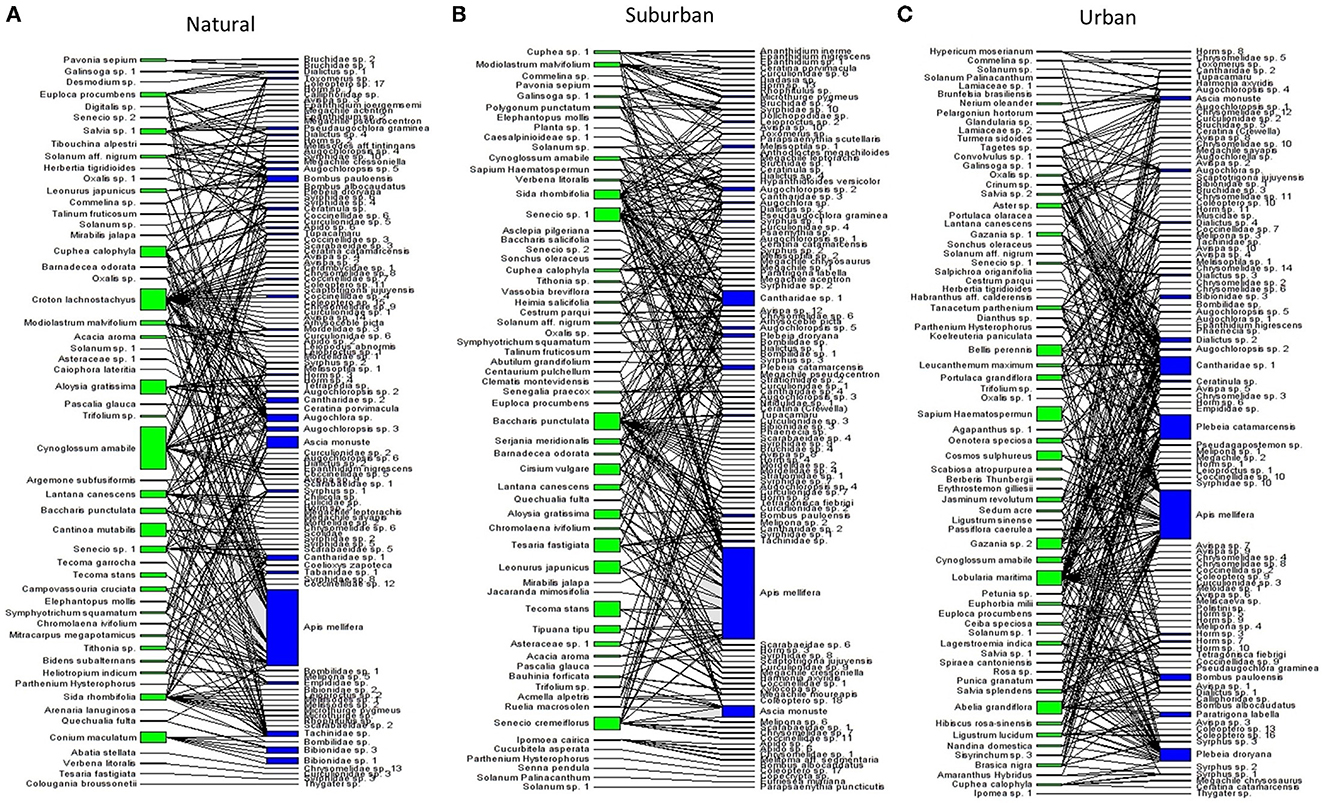
Figure 2. Total interactions of the sites in each urban category. References: (A) natural sites, (B) suburban sites, and (C) urban sites. Green boxes represent plant species/morphospecies and blue boxes represent flower visitor species/morphospecies, named right next to each box. Lines represent interactions and box width is proportional to the number of times that each species was recorded interacting.
Local-scale effects over community and network metrics
The richness of flower visitors was explained by the relation among the proportion of exotic plants, flower coverage, and temporal variation of flower coverage (Table 1). The richness of flower visitors decreased with increasing variability in flower coverage. However, when the variability in flower coverage was low, the richness of flower visitors increased with increasing flower cover and proportion of exotics (Figure 3A). Moreover, high variability in floral resources negatively affected the diversity of interactions (Figure 3B, Table 2).
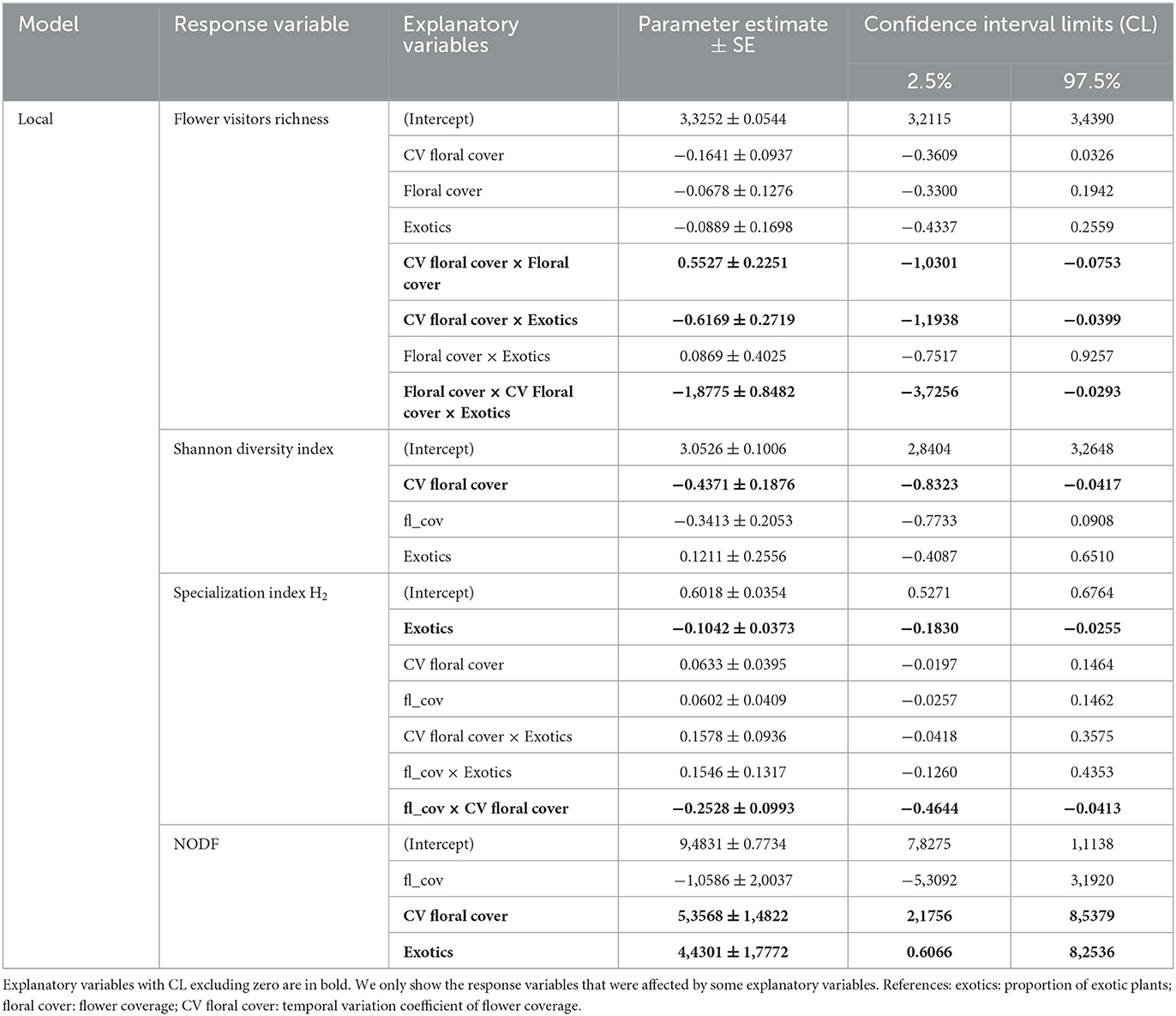
Table 1. Estimates of community and network parameters, SE, and 95% confidence interval limits (CL) for explanatory variables at the local scale.
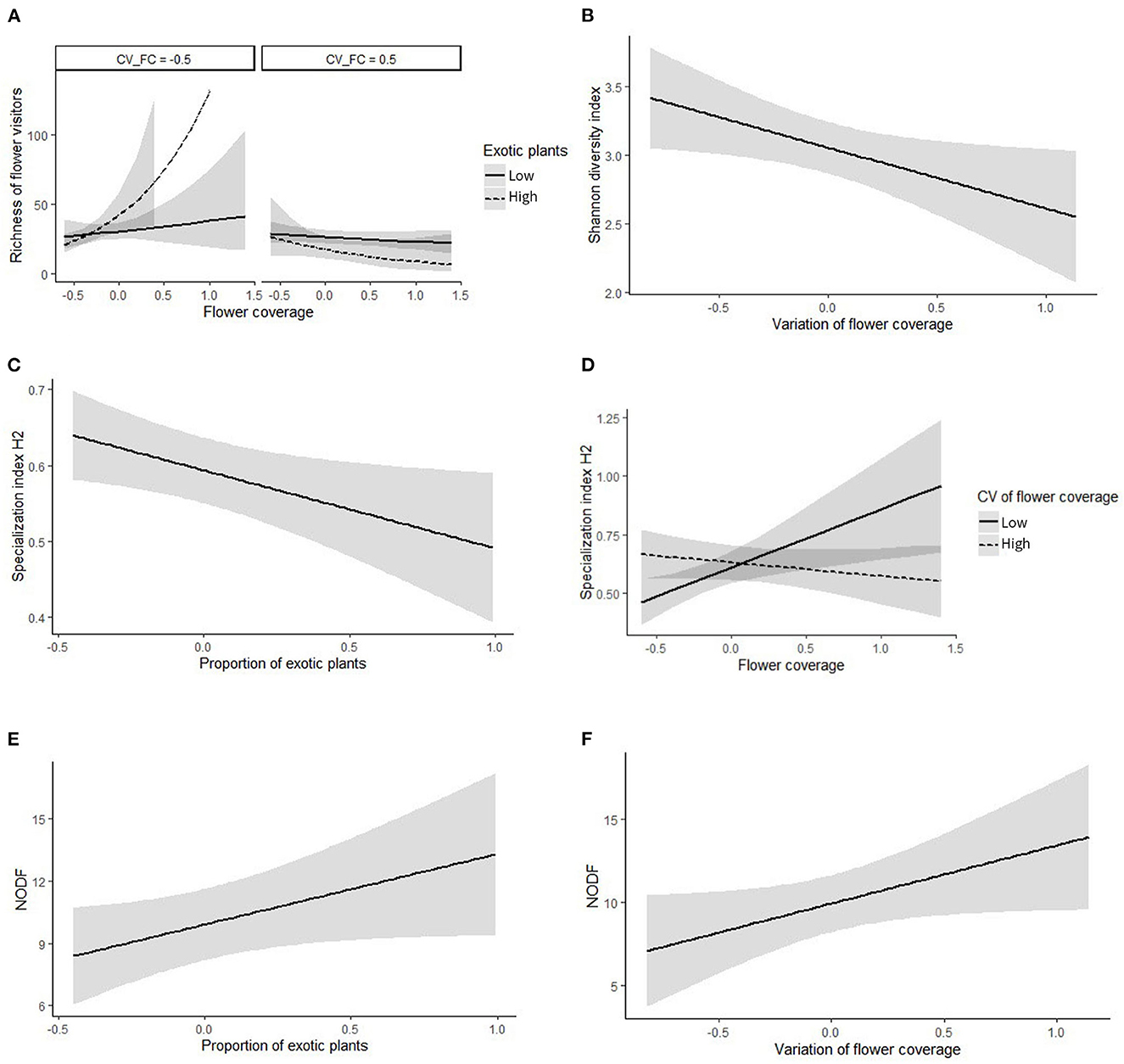
Figure 3. Response of community parameters and network metrics to different local scale variables. (A) Response of richness of flower visitors to flower coverage, for different proportions of exotic plants at two different values of the coefficient of temporal variation of flower coverage (CV_FC). The values of the explanatory variables are standardized, their equivalence is as follows: flower coverage −0.5 = 3%, 0.5 = 18%, 1 = 27%; coefficient of variation of flower coverage, CV_FC: −0.5 = 23% (low variation in floral resources), CV_FC: 0.5 = 75% (high variation in floral resources); the proportion of exotics plants: low = 4%; high = 46%. (B) Response of the Shannon diversity index to temporal variation of flower coverage; (C) response of the specialization index to the proportion of exotic plants; and the responses of NODF to (D) proportion of exotic plant species, and (E) temporal variation of flower coverage. References: the values of the explanatory variables are standardized. The equivalence of each value is as follows: proportion of exotic plants: −0.4 = 3%; 0 = 20%; 0.8 = 58%; temporal variation of flower coverage: CV_FC −0.5 = 23% (low resources variation), 0 = 50% (medium resources variation), CV_FC 0.5 = 75% (high resources variation). (F) Response of the specialization index H2 to flower coverage, at two different levels and the coefficient of temporal variation of flower coverage. The value of the explanatory variables are standardized, we show the equivalence of each value: flower coverage −0.5 = 3%, 0.5 = 18%, 1 = 27%; temporal variation of flower coverage: low = 23% (low variation in floral resources), high = 75% (high variation in floral resources).
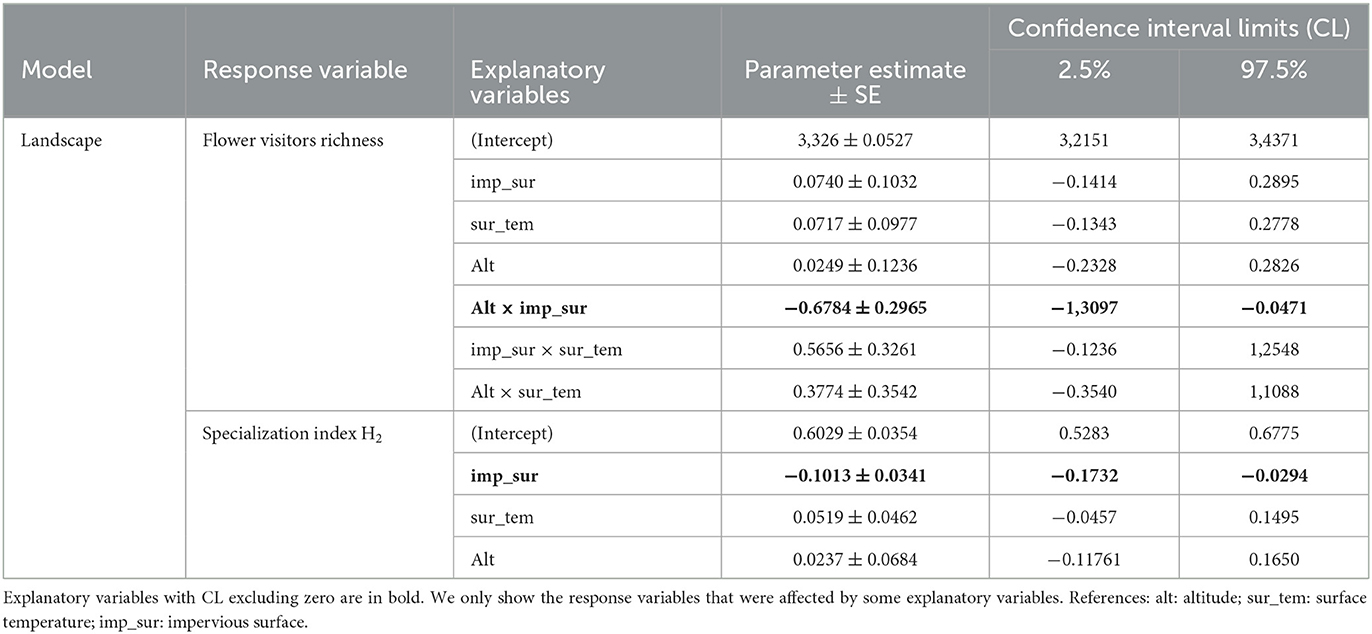
Table 2. Estimates of community and network parameters, SE, and 95% confidence interval limits (CL) for explanatory variables at the landscape scale.
Specialization of the network (H2) decreased when the proportion of exotics increased (Figure 3C). Furthermore, this index was also influenced by the interaction between flower coverage and its variability. When the variability in flower cover was low, the specialization index increased with increasing flower cover (thus high stability promoted specialization in the community), but when variability was high, the specialization index did not change with flower cover (Figure 3D). While the connectance of the network was not affected by any local-scale variable (Supplementary Table 6), nestedness (NODF) increased with an increasing proportion of exotic plants and variability in flower cover (Figures 3E, F, respectively).
Landscape effect over community parameters and network metrics
We found that the effect of the percentage of impervious surface on flower visitor richness differs with altitude. At low altitudes, the richness of flower visitors increased when the proportion of impervious surfaces increased. But at high altitudes, richness decreases with increasing impervious surface (Figure 4A, Table 2).
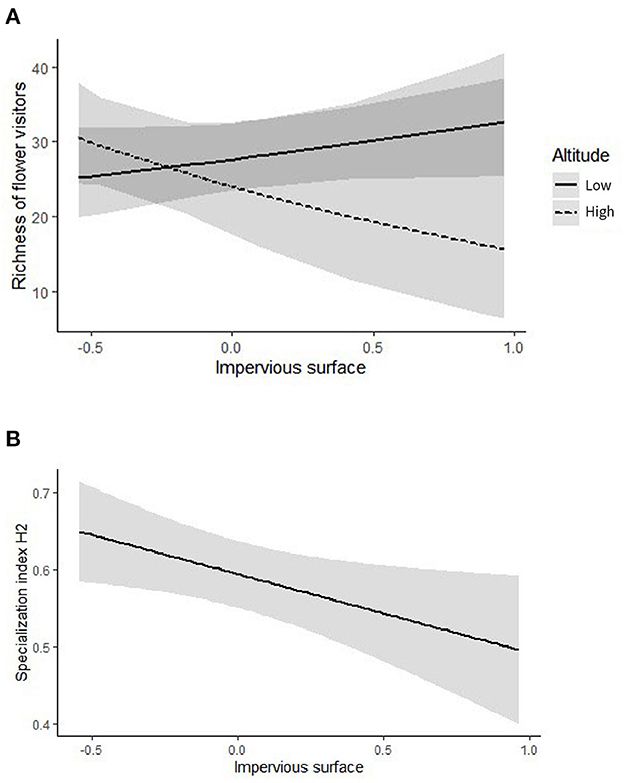
Figure 4. Response of community parameters and network metrics to different landscape scale variables. (A) Response of the richness of flower visitors to the interaction between the percentage of impervious surface and altitude. The value of the explanatory variables are standardized, we show the equivalence of each value: impervious surface −0.4 = 4%, 0.1 = 35%, 0.6 = 65%; low altitude = 1,120 m, high altitude = 1,550 m. (B) Response of the specialization index H2 to the urbanization gradient. Each triangle represents a value of the specialization index H2 for each of the 21 communities analyzed. The value of the explanatory variables are standardized, we show the equivalence of each value: impervious surface −0.4 = 4%, 0.1 = 35%, 0.6 = 65%.
The specialization of the network (H2) was negatively affected by impervious surface, suggesting that urbanization induces a more generalized assemblage (Figure 4B, Table 2). The Shannon diversity index was not affected by any landscape explanatory variable.
Discussion
In this study, we evaluated the influence of urbanization over the assemblage of flower visitors using an interaction network approach, in an area of Argentinian Yungas. We measured different variables at local and landscape scales and evaluated the effect of those variables over community and network metrics. We found that both local and landscape-scale variables differently affected community parameters and network metrics. At the local scale, specifically, temporal variability of resources had complex effects over most of the parameters evaluated, while landscape-scale variables affected both network specialization and flower visitor richness.
At the local scale, we found that the stability of flower resources affected the richness of flower visitors and the level of generalization of the network. Increasing the stability of floral resources increased the richness of flower visitors and diversity of interactions and decreased the level of nestedness. It has been shown that the constancy of floral resources over time can lead to a high richness of flower visitors (Stewart and Waitayachart, 2020). Conversely, the diversity of flower visitors was negatively affected by decreasing stability of floral resources as was documented previously by Winfree et al. (2011) who found that flower visitors respond to changes in floral resources. As was observed in other studies, the availability of floral resources can mitigate the effect of urbanization on flower visitors (Burdine and Mccluney, 2019; Baldock, 2020; Wenzel et al., 2020). Moreover, urban sites could be a better place than suburban and rural areas by offering large amounts of floral resources (Lynch et al., 2021) and nesting sites (Frankie et al., 2005; Matteson et al., 2008; Hülsmann et al., 2015), as well as a more stable availability of those resources over time, as plants are replaced periodically in parks within urban areas. Our findings highlight the importance of constant and reliable availability of floral resources within urban areas to maintain a high richness and diversity of flower visitors.
Increasing the proportion of exotic flowers within the community, usually used as ornamental in urban areas (Smith et al., 2005), also affected flower visitors and their interactions. Many studies report a negative effect of exotic plants over flower visitors (Moroń et al., 2009; Martins et al., 2013; Fenesi et al., 2015), while others show no effect of plant status (native or exotic) on network structure (Vilà et al., 2009). We found that exotic flowers, when constant, increased flower visitor richness, probably because they provide important resources. Additionally, we found that an increasing proportion of exotic plants leads to a more generalist and nested network. The increase in generalist interactions can result from having more generalist flower visitor species or generalist plants (exotic plants may have floral morphologies that are more accessible to any flower visitor). Yet in our sites, both generalist and specialist flower visitors (specifically bees) are found equally distributed in the gradient studied (Amado De Santis and Chacoff, 2020). Alternatively, increased generalization may be related to a reduction in the number of native plants, a common trend found in urbanization gradients (Bertin, 2002; Chocholoušková and Pyšek, 2003; Tait et al., 2005). As a result, specialized interactions between native plants and flower visitors might have been lost (Traveset and Richardson, 2006; Aizen et al., 2008). Moreover, exotic plants contribute to an increase in flower visitor niche overlap (Marrero et al., 2017), thus, an increasing number of exotic plants may be playing a key role in interspecific competition among flower visitors, which could lead to a more generalist behavior by flower visitors.
Nestedness, the most common structure of mutualistic networks (Bascompte et al., 2003), has been linked to resilience, in theoretical models, as increasing nestedness increased resilience (Thébault and Fontaine, 2010). We noticed that the prevalence of exotic plant species along with low floral resource stability throughout the flowering season increased the level of nestedness of the plant-flower visitor networks. This agrees with previous studies where they show a positive relationship between nestedness and the presence of exotics (Stouffer et al., 2014). Exotic plants are generally involved in generalist interactions, and it has been recently found that they can even contribute more than natives to nestedness in networks of urban areas (Zaninotto et al., 2023). Exotic plants can play an important role in increasing the level of nestedness, thus probably increasing the resilience of these communities in urban areas, as they provide resources in moments when native flowers are not flowering. In this case, the nested pattern may be related to the tendency of having bigger networks toward urban areas (Bascompte et al., 2003; Vázquez and Aizen, 2004) and an increased generalism in the interactions. This interpretation agrees with Pigozzo and Viana (2010), who suggested that nestedness could be related to the ability of different species to exploit the different range of resources (generalist vs. specialist). To our knowledge, low stability in floral resources followed by an increase in nestedness in the community is a new and interesting result, probably related to the different timing of flowering of native vs. exotics or to the increased phenological period of flowering. Further studies could confirm its generality and contribute to understanding the mechanisms behind it.
At the landscape scale, the percentage of impervious surface affected network structure, while temperature and distance to bigger natural areas had no detectable effects. We found that the effect of the percentage of impervious surface on flower visitor richness differs with altitude. At the lowest sites, the richness of flower visitors increased with an increasing proportion of impervious surfaces, but the effect was the opposite at high altitudes. Species richness of flower visitors has been shown to decrease with altitude (Arroyo et al., 1985; Hodkinson, 2005; Ramos-Jiliberto et al., 2010), as in our study, despite the narrow altitude range of our sites (1,031–1,681 m.a.s.l.). The differential effect of urbanization on the richness of flower visitors depending on altitude might be the result of a more specialized, restricted, or limited set of species toward higher altitudes, but further study to understand the mechanism behind this pattern would be needed.
The proportion of impervious surfaces negatively affected the specialization of the network. This result agrees with Aizen et al. (2012), who concluded that specialized plant-flower visitor interactions are particularly prone to be lost with increasing habitat fragmentation and other anthropogenic disturbances. Moreover, this could be related to a greater percentage of exotic plants in urban areas, which contribute to an increase in niche overlap among flower visitors, as exotic species often have less restricted morphologies, and therefore tend to be more generalistic, on average, than native species (Marrero et al., 2017). This scenario might favor mostly generalist flower visitors and could affect the reproduction of native plants present in these sites (Totland et al., 2006). In this sense, many species of flower visitors interact with generalist exotic plants in highly invaded networks, and there is a trend of increased dependency with time on those exotic species (Aizen et al., 2008). This situation could lead to a more generalist flower visitor assemblage.
Overall, our results suggest that, at the community level, flower visitors are affected by urbanization, although this is dependent on the spatial scale. Local-scale factors have strong effects on the parameters measured, and local and landscape effects both affect the richness and the specialization levels of the network in different ways. Therefore, our findings highlight the importance of factors at the local scale—i.e., the stability of floral resources in their effect on community and network metrics. Our results suggest that urban residents along with garden managers can promote the presence of pollinators by managing floral resources, specifically increasing their stability through the spring and summer. Our results are encouraging because local conditions can be relatively easily managed, while landscape or context variables, such as habitat cover, are more complex and economically costly to handle. With the information offered here, we encourage decision-makers to be very cautious in the implementation of development plans in urban environments located in forests at high altitudes. In conclusion, the network approach provides valuable information to achieve more sustainable development of urban expansion with respect to the flower visitor assemblages when managing territorial development plans and maintenance of public green spaces such as urban squares and parks.
Data availability statement
The original contributions presented in the study are included in the article/Supplementary material, further inquiries can be directed to the corresponding author.
Author contributions
Data collection and analysis were performed by AA and NC. The first draft of the manuscript was written by AA. NC and SL commented on previous versions of the manuscript and read, discussed, and approved the final manuscript. All authors contributed to the study's conception and design. All authors contributed to the article and approved the submitted version.
Funding
This project was partly supported by the National Research Council of Argentina (CONICET) by mean of a Doctoral fellowship, the SIGMA Xi Foundation, a PIUNT Project from the National University of Tucumán, Argentina (UNT), and CONICET Project (PUE N 023 GASPARRI).
Acknowledgments
We would like to thank Mariano Lucia and Leopoldo Alvarez who helped with Flower visitors identification, Alberto Slanis for plants identification, Carlos Cabrera for GIS analysis, offering site classification and maps, and Agostina Ferro for calculations of land surface temperature.
Conflict of interest
The authors declare that the research was conducted in the absence of any commercial or financial relationships that could be construed as a potential conflict of interest.
Publisher's note
All claims expressed in this article are solely those of the authors and do not necessarily represent those of their affiliated organizations, or those of the publisher, the editors and the reviewers. Any product that may be evaluated in this article, or claim that may be made by its manufacturer, is not guaranteed or endorsed by the publisher.
Supplementary material
The Supplementary Material for this article can be found online at: https://www.frontiersin.org/articles/10.3389/frsc.2023.1086076/full#supplementary-material
Footnotes
1. ^Available online at: https://www.censo.gob.ar/.
References
Ahrne, K., Bengtsson, J., and Elmqvist, T. (2009). Bumble bees (Bombus spp) along a gradient of increasing urbanization. J. Pak. Med. Assoc. 67, 1664–1669. doi: 10.1371/journal.pone.0005574
Aizen, M. A., Morales, C. L., and Morales, J. M. (2008). Invasive mutualists erode native pollination webs. PLoS Biol. 6, 0396–0403. doi: 10.1371/journal.pbio.0060031
Aizen, M. A., Sabatino, M., and Tylianakis, J. M. (2012). Specialization and rarity predict nonrandom loss of interactions from mutualist networks. Science 335, 1486–1489. doi: 10.1126/science.1215320
Almeida-Neto, M., Guimarães, P. R. J., Loyota, R. D., and Ulrich, W. (2008). A consistent metric for nestedness analysis in ecological systems: Reconciling concept and measurement. Oikos 13, 16644. doi: 10.1111/j.0030-1299.2008.16644.x
Amado De Santis, A. A., and Chacoff, N. P. (2020). Urbanization affects composition but not richness of flower visitors in the yungas of Argentina. Neotrop. Entomol. 20, 772. doi: 10.1007/s13744-020-00772-z
Arroyo, M. T. K., Armesto, J. J., and Primack, R. B. (1985). Community studies in pollination ecology in the high temperate Andes of central Chile II. Effect of temperature on visitation rates and pollination possibilities. Plant Systemat. Evol. 149, 187–203. doi: 10.1007/BF00983305
Baldock, K. C. (2020). Opportunities and threats for pollinator conservation in global towns and cities. Curr. Opin. Insect Sci. 38, 63–71. doi: 10.1016/j.cois.2020.01.006
Baldock, K. C. R., Goddard, M. A., Kunin, W. E., Potts, S. G., Stone, G. N., and Memmott, J. (2015). Managing Urban Areas for Insect Pollinators: As Town and Cities Continue to Grow How Can Land Managers Help Insect Pollinators in Urban Areas? Living With Environmental Change Policy and Practice Notes. Note No.20 - Insect Pollinators Initiative. December, ed A. Liddon, Wiltshire: Newcastle University.
Bascompte, J., Jordano, P., Melián, C. J., and Olesen, J. M. (2003). The nested assembly of plant-animal mutualistic networks. Proc. Natl. Acad. Sci. U. S. A. 100, 9383–9387. doi: 10.1073/pnas.1633576100
Bates, A. J., Sadler, J. P., Fairbrass, A. J., Falk, S. J., Hale, J. D., and Matthews, T. J. (2011). Changing bee and hoverfly pollinator assemblages along an urban-rural gradient. PLoS ONE 6, 23459. doi: 10.1371/journal.pone.0023459
Bates, D., Mächler, M., Bolker, B. M., and Walker, S. C. (2015). Fitting linear mixed-effects models using lme4. J. Stat. Softw. 67, i01. doi: 10.18637/jss.v067.i01
Bertin, R. I. (2002). Losses of native plant species from Worcester, Massachusetts. Rhodora. 104, 325–349. Available online at: http://www.jstor.org/stable/23313508
Blüthgen, N., Menzel, F., and Blüthgen, N. (2006). Measuring specialization in species interaction networks. BMC Ecol. 6, 9. doi: 10.1186/1472-6785-6-9
Boldrini, P., and Malizia, M. (2017). Urbanizaciones informales en ciudades inter- medias. Los casos del Gran San Salvador de Jujuy y Gran San Miguel de Tucumán (Noroeste argentino). Cuaderno Urbano. Espacio Cultura Sociedad 23, 85–106. doi: 10.30972/crn.23233571
Briere, J. F., Pracros, P., Le Roux, A. Y., and Pierre, J. S. (1999). A novel rate model of temperature-dependent development for arthropods. Environ. Entomol. 28, 22–29. doi: 10.1093/ee/28.1.22
Brown, A., Chalukian, S., and Malmierca, L. (1985). Estudio florístico-estructural de un sector de selva semidecidua del noroeste argentino. I: Composición florística, densidad y diversidad. Darwiniana 26, 27–41.
Burdine, J. D., and Mccluney, K. E. (2019). Interactive effects of urbanization and local habitat characteristics influence bee communities and flower visitation rates. Oecologia 190, 715–723. doi: 10.1007/s00442-019-04416-x
Burnham, K. P., and Anderson, D. R. (2004). Multimodel Inference: Understanding AIC and BIC in Model Selection. Sociol. Method. Res. 33, 261–304. doi: 10.1177/0049124104268644
Chacoff, N. P., Vázquez, D. P., Lomáscolo, S. B., Stevani, E. L., Dorado, J., and Padrón, B. (2012). Evaluating sampling completeness in a desert plant-pollinator network. J. Anim. Ecol. 81, 190–200. doi: 10.1111/j.1365-2656.2011.01883.x
Chao, A. (1987). Estimating the population size for capture-recapture data with unequal catchability. J. Anim. Ecol. 43, 783–791. doi: 10.2307/2531532
Chocholoušková, Z., and Pyšek, P. (2003). Changes in composition and structure of urban flora over 120 years: A case study of the city of Plzen. Flora 198, 366–376. doi: 10.1078/0367-2530-00109
Díaz, S., Fargione, J., Chapin, F. S., and Tilman, D. (2006). Biodiversity loss threatens human well-being. PLoS Biol. 4, 1300–1305. doi: 10.1371/journal.pbio.0040277
Ebeling, A., Klein, A. M., Schumacher, J., Weisser, W. W., and Tscharntke, T. (2008). How does plant richness affect pollinator richness and temporal stability of flower visits? Oikos 117, 1808–1815. doi: 10.1111/j.1600-0706.2008.16819.x
Fenesi, A., Vágási, C. I., Beldean, M., Földesi, R., Kolcsár, L. P., Shapiro, J. T., et al. (2015). Solidago canadensis impacts on native plant and pollinator communities in different-aged old fields. Basic Appl. Ecol. 16, 335–346. doi: 10.1016/j.baae.2015.03.003
Frankie, G. W., Thorp, R. W., Schindler, M., Hernandez, J., Ertter, B., and Rizzardi, M. (2005). Ecological patterns of bees and their host ornamental flowers in two Northern California cities. J. Kansas Entomol. Soc. 78, 227–246. doi: 10.2317/0407.08.1
Gelman, A., and Hill, J. (2007). Data Analysis Using Regression and Multilevel/Hierarchical Models Data. Cambridge University Press, 55. doi: 10.1017/CBO9780511790942
Geslin, B., Le Féon, V., Folschweiller, M., Flacher, F., Carmignac, D., Motard, E., et al. (2016). The proportion of impervious surfaces at the landscape scale structures wild bee assemblages in a densely populated region. Ecol. Evol. 6, 6599–6615. doi: 10.1002/ece3.2374
Grundel, R., Jean, R. P., Frohnapple, K. J., Glowacki, G. A., Scott, P. E., and Pavlovic, N. B. (2010). Floral and nesting resources, habitat structure, and fire influence bee distribution across an open-forest gradient. Ecol. Appl. 20, 1678–1692. doi: 10.1890/08-1792.1
Guimarães, Jr. P. R. (2020). “The structure of ecological networks across levels of organization. Ann. Rev. Ecol. Evol. Systemat. 51, 433–460. doi: 10.1146/annurev-ecolsys-012220-120819
Gupta, A. (2002). Geoindicators for tropical urbanization. Environ. Geol. 42, 736–742. doi: 10.1007/s00254-002-0551-x
Hall, D. M., Camilo, G. R., Tonietto, R. K., Ollerton, J., Ahrné, K., Arduser, M., et al. (2017). The city as a refuge for insect pollinators. Conserv. Biol. 31, 24–29. doi: 10.1111/cobi.12840
Hodkinson, I. D. (2005). Terrestrial insects along elevation gradients: Species and community responses to altitude. Biol. Rev. Cambridge Philos. Soc. 80, 489–513. doi: 10.1017/S1464793105006767
Hülsmann, M., von Wehrden, H., Klein, A. M., and Leonhardt, S. D. (2015). Plant diversity and composition compensate for negative effects of urbanization on foraging bumble bees. Apidologie 46, 760–770. doi: 10.1007/s13592-015-0366-x
Jordano, P. (1987). Patterns of mutualistic interactions in pollination and seed dispersal: Connectance, dependence asymmetries, and coevolution. Am. Naturalist 129, 657–677. doi: 10.1086/284665
Kaiser-Bunbury, C. N., and Blüthgen, N. (2015). Integrating network ecology with applied conservation: A synthesis and guide to implementation. AoB Plants 7, plv076. doi: 10.1093/aobpla/plv076
Kaiser-Bunbury, C. N., Muff, S., Memmott, J., Müller, C. B., and Caflisch, A. (2010). The robustness of pollination networks to the loss of species and interactions: A quantitative approach incorporating pollinator behaviour. Ecol. Lett. 13, 442–452. doi: 10.1111/j.1461-0248.2009.01437.x
Knight, T. M., Ashman, T. L., Bennett, J. M., Burns, J. H., Passonneau, S., and Steets, J. A. (2018). Reflections on, and visions for, the changing field of pollination ecology. Ecol. Lett. 21, 1282–1295. doi: 10.1111/ele.13094
Logan, M. (2009). Biostatistical Design and Analysis Using R: A Practical Guide. John Wiley & Sons Ltd. doi: 10.1002/9781444319620
Lynch, L., Kangas, M., Ballut, N., Doucet, A., Schoenecker, K., Johnson, P., et al. (2021). Changes in land use and land cover along an urban-rural gradient influence floral resource availability. Curr. Landsc. Ecol. Rep. 64, 1. doi: 10.1007/s40823-021-00064-1
Malizia, L., Pacheco, S., Blundo, C., and Brown, A. D. (2012). Caracterización altitudinal, uso y conservación de las Yungas subtropicales de Argentina. Ecosistemas 21, 53–73. Available online at: https://www.revistaecosistemas.net/index.php/ecosistemas/article/view/27
Marín, L., Martínez-Sánchez, M. E., Sagot, P., Navarrete, D., and Morales, H. (2019). Floral visitors in urban gardens and natural areas: Diversity and interaction networks in a neotropical urban landscape. Basic Appl. Ecol. 43, 3–15. doi: 10.1016/j.baae.2019.10.003
Marrero, H. J., Torretta, J. P., Vázquez, D. P., Hodara, K., and Medan, D. (2017). Exotic plants promote pollination niche overlap in an agroecosystem. Agri. Ecosyst. Environ. 239, 304–309. doi: 10.1016/j.agee.2017.01.027
Martins, A. C., Goncalves, R. B., and Melo, G. A R. (2013). Changes in wild bee fauna of a grassland in Brazil reveal negative effects associated with growing urbanization during the last 40 years. Zoologia 30, 157–176. doi: 10.1590/S1984-46702013000200006
Matteson, K. C., Ascher, J. S., Langellotto, G. A., Matteson, K. C., and Ascher, J. S. (2008). Bee richness and abundance in New York city urban gardens bee richness and abundance in New York city urban gardens. Ann. Entomol. Soc. Am. 101, 140–150. doi: 10.1603/0013-8746(2008)101[140:BRAAIN]2.0.CO;2
McCune, F., Normandin, É., Mazerolle, M. J., and Fournier, V. (2019). Response of wild bee communities to beekeeping, urbanization, and flower availability. Urban Ecosyst. 19, 909. doi: 10.1007/s11252-019-00909-y
McDonald, R. I., Marcotullio, P. J., and Güneralp, B. (2013). Urbanization and global trends in biodiversity and ecosystem services. Urban. Biodiv. Ecosyst. Serv. 3, 31–52. doi: 10.1007/978-94-007-7088-1_3
McFrederick, Q. S., and LeBuhn, G. (2006). Are urban parks refuges for bumble bees Bombus spp. (Hymenoptera: Apidae)? Biol. Conserv. 129, 372–382. doi: 10.1016/j.biocon.2005.11.004
McKinney, M. L. (2002). Urbanization, Biodiversity, and Conservation: The impacts of urbanization on native species are poorly studied, but educating a highly urbanized human population about these impacts can greatly improve species conservation in all ecosystems. Bioscience. 52, 883–890. doi: 10.1641/0006-3568(2002)052[0883:UBAC]2.0.CO;2
McKinney, M. L. (2006). Urbanization as a major cause of biotic homogenization. Biol. Conserv. 127, 247–260. doi: 10.1016/j.biocon.2005.09.005
McKinney, M. L. (2008). Effects of urbanization on species richness: A review of plants and animals. Urban Ecosyst. 11, 161–176. doi: 10.1007/s11252-007-0045-4
Montoya, D. (2008). Habitat loss, dispersal, and the probability of extinction of tree species. Commun. Integr. Biol. 1, 146–147. doi: 10.4161/cib.1.2.6998
Moroń, D., Lenda, M., Skórka, P., Szentgyörgyi, H., Settele, J., and Woyciechowski, M. (2009). Wild pollinator communities are negatively affected by invasion of alien goldenrods in grassland landscapes. Biol. Conserv. 142, 1322–1332. doi: 10.1016/j.biocon.2008.12.036
Osborne, J. L., Martin, A. P., Shortall, C. R., Todd, A. D., Goulson, D., Knight, M. E., et al. (2008). Quantifying and comparing bumblebee nest densities in gardens and countryside habitats. J. Appl. Ecol. 45, 784–792. doi: 10.1111/j.1365-2664.2007.01359.x
Owen, S. M., MacKenzie, A. R., Bunce, R. G. H., Stewart, H. E., Donovan, R. G., Stark, G., et al. (2006). Urban land classification and its uncertainties using principal component and cluster analyses: A case study for the UK West Midlands. Lands. Urb. Plan. 78, 311–321. doi: 10.1016/j.landurbplan.2005.11.002
Oyarzabal, M., Clavijo, J., Oakley, L., Biganzoli, F., Tognetti, P., Barberis, I., et al. (2018). Unidades de vegetación de la Argentina. Ecología Austral 28, 040–063. doi: 10.25260/EA.18.28.1.0.399
Pigozzo, C. M., and Viana, B. F. (2010). Estrutura da rede de interações entre flores e abelhas em ambiente de caatinga. Oecologia Australis 14, 100–114. doi: 10.4257/oeco.2010.1401.04
Potter, A., and LeBuhn, G. (2015). Pollination service to urban agriculture in San Francisco, CA. Urb. Ecosyst. 18, 885–893. doi: 10.1007/s11252-015-0435-y
Quinn, G. P., and Keough, M. J. (2002). Experimental Design and Data Analysis for Biologists. Cambridge: Cambridge University Press. doi: 10.1017/CBO9780511806384
Quistberg, R. D., Bichier, P., and Philpott, S. M. (2016). Landscape and local correlates of bee abundance and species richness in urban gardens. Environ. Entomol. 45, 592–601. doi: 10.1093/ee/nvw025
R Core Team (2019). A Language and Environment for Statistical Computing. Vienna: R Foundation for Statistical Computing. Available online at: http://www.rproject.org/index.html (accessed March 2022).
Ramos-Jiliberto, R., Domínguez, D., Espinoza, C., López, G., Valdovinos, F. S., Bustamante, R. O., et al. (2010). Topological change of Andean plant–pollinator networks along an altitudinal gradient. Ecol. Complex. 7, 86–90. doi: 10.1016/j.ecocom.2009.06.001
Resasco, J., Chacoff, N. P., and Vázquez, D. P. (2021). Plant–pollinator interactions between generalists persist over time and space. Ecology 102, 1–7. doi: 10.1002/ecy.3359
Rodrigues, J. J. S., Brown, K. S., and Ruszczyk, A. (1993). Resources and conservation of neotropical butterflies in urban forest fragments. Biol. Conserv. 64, 3–9. doi: 10.1016/0006-3207(93)90377-D
Sabatino, M., Maceira, N., and Aizen, M. (2010). Direct effects of habitat area on interaction diversity in pollination webs. Ecol. Appl. 23, 515–522. doi: 10.1890/09-1626.1
Sales, K., Vasudeva, R., Dickinson, M. E., Godwin, J. L., Lumley, A. J., Michalczyk, Ł., et al. (2018). Experimental heatwaves compromise sperm function and cause transgenerational damage in a model insect. Nat. Commun. 9, 1–11. doi: 10.1038/s41467-018-07273-z
Savard, J. P. L., Clergeau, P., and Mennechez, G. (2000). Biodiversity concepts and urban ecosystems. Lands. Urb. Plan. 48, 131–142. doi: 10.1016/S0169-2046(00)00037-2
Schleuning, M., Fründ, J., Schweiger, O., Welk, E., Albrecht, J., Albrecht, M., et al. (2016). Ecological networks are more sensitive to plant than to animal extinction under climate change. Nat. Commun. 7, 1–9. doi: 10.1038/ncomms13965
Schwarz, B., Vázquez, D. P., CaraDonna, P. J., Knight, T. M., Benadi, G., Dormann, C. F., et al. (2020). Temporal scale-dependence of plant–pollinator networks. Oikos 2020, 1–14. doi: 10.1111/oik.07303
Shwartz, A., Cheval, H., Simon, L., and Julliard, R. (2013). Virtual garden computer program for use in exploring the elements of biodiversity people want in cities. Conserv. Biol. 27, 876–886. doi: 10.1111/cobi.12057
Smith, R. M., Thompson, K., Hodgson, J. G., Warren, P. H., and Gaston, K. J. (2005). Urban domestic gardens (IX): Composition and richness of the vascular plant flora, and implications for native biodiversity. Biol. Conserv. 129, 312–322. doi: 10.1016/j.biocon.2005.10.045
Stewart, A. B., and Waitayachart, P. (2020). Year-round temporal stability of a tropical, urban plant-pollinator network. PLoS ONE 15, 1–12. doi: 10.1371/journal.pone.0230490
Stouffer, D. B., Cirtwill, A. R., and Bascompte, J. (2014). How exotic plants integrate into pollination networks. J. Ecol. 102, 1442–1450. doi: 10.1111/1365-2745.12310
Tait, C. J., Daniels, C. B., and Hill, R. S. (2005). Changes in species assemblages within the Adelaide metropolitan area, Australia, 1836-2002. Ecol. Appl. 15, 346–359. doi: 10.1890/04-0920
The National Institute of Statistics Censuses (2022). INDEC. Available online at: https://www.indec.gob.ar/indec/web/Nivel3-Tema-2-41 (accessed July 2022).
Thébault, E., and Fontaine, C. (2010). Stability of ecological communities and the architecture of mutualistic and trophic networks. Science 329, 853–856. doi: 10.1126/science.1188321
Theodorou, P., Radzevičiute, R., Lentendu, G., Kahnt, B., Husemann, M., Bleidorn, C., et al. (2020). Urban areas as hotspots for bees and pollination but not a panacea for all insects. Nat. Commun. 11, 1–13. doi: 10.1038/s41467-020-14496-6
Threlfall, C. G., Walker, K., Williams, N. S. G., Hahs, A. K., Mata, L., Stork, N., et al. (2015). The conservation value of urban green space habitats for Australian native bee communities. Biol. Conserv. 187, 240–248. doi: 10.1016/j.biocon.2015.05.003
Totland, Ø., Nielsen, A., Bjerknes, A. L., and Ohlson, M. (2006). Effects of an exotic plant and habitat disturbance on pollinator visitation and reproduction in a boreal forest herb. Am. J. Bot. 93, 868–873. doi: 10.3732/ajb.93.6.868
Traveset, A., and Richardson, D. M. (2006). Biological invasions as disruptors of plant reproductive mutualisms. Trends Ecol. Evol. 21, 208–216. doi: 10.1016/j.tree.2006.01.006
Tylianakis, J. M., and Morris, R. J. (2017). Ecological networks across environmental gradients. Ann. Rev. Ecol. Evol. Systemat. 48, 25–48. doi: 10.1146/annurev-ecolsys-110316-022821
Tylianakis, J. M., Tscharntke, T., and Lewis, O. T. (2007). Habitat modification alters the structure of tropical host-parasitoid food webs. Nature 445, 202–205. doi: 10.1038/nature05429
United Nations (2019). World Population Prospects (Issue 141). Avaialble online at: http://www.ncbi.nlm.nih.gov/pubmed/12283219 (accessed September 2022).
United Nations (2019). World Population Prospects 2019: Highlights (ST/ESA/SER.A/423). New York, NY: Department of Economic and Social Affairs Population Division.
Valiente-Banuet, A., Aizen, M. A., Alcántara, J. M., Arroyo, J., Cocucci, A., Galetti, M., et al. (2014). Beyond species loss: the extinction of ecological interactions in a changing world. Funct. Ecol. 29, 299–307. doi: 10.1111/1365-2435.12356
Vázquez, D. P., and Aizen, M. A. (2004). Asymmetric specialization: A pervasive feature of plant-pollinator interactions. Ecology 85, 1251–1257. doi: 10.1890/03-3112
Vilà, M., Bartomeus, I., Dietzsch, A. C., Petanidou, T., Steffan-Dewenter, I., Stout, J. C., et al. (2009). Invasive plant integration into native plant-pollinator networks across Europe. Proc. Royal Soc. B 276, 3887–3893. doi: 10.1098/rspb.2009.1076
Vizentin-Bugoni, J., Maruyama, P. K., and Sazima, M. (2014). Processes entangling interactions in communities: Forbidden links are more important than abundance in a hummingbird-plant network. Proc. Royal Soc. B 281, 2397. doi: 10.1098/rspb.2013.2397
Wan, B., Guo, Q., Fang, F., Su, Y., and Wang, R. (2015). Mapping US urban extents from MODIS data using one-class classification method. Remot. Sens. 7, 10143–10163. doi: 10.3390/rs70810143
Weiner, C. N., Werner, M., Linsenmair, K. E., and Blüthgen, N. (2014). Land-use impacts on plant-pollinator networks: Interaction strength and specialization predict pollinator declines. Ecology 95, 466–474. doi: 10.1890/13-0436.1
Wenzel, A., Grass, I., Belavadi, V. V., and Tscharntke, T. (2020). How urbanization is driving pollinator diversity and pollination – A systematic review. Biol. Conserv. 241, 108321. doi: 10.1016/j.biocon.2019.108321
Winfree, R., Bartomeus, I., and Cariveau, D. P. (2011). Native pollinators in anthropogenic habitats. Ann. Rev. Ecol. Evol. Systemat. 42, 1–22. doi: 10.1146/annurev-ecolsys-102710-145042
Zaninotto, V., Thebault, E., and Dajoz, I. (2023). Native and exotic plants play different roles in urban pollination networks across seasons. Oecologia 201, 525–536. doi: 10.1007/s00442-023-05324-x
Keywords: urbanization, flower visitor, assemblage, interaction network, network structure, Jujuy, local and landscape scale
Citation: Amado De Santis AA, Lomáscolo SB and Chacoff NP (2023) Effects of urbanization on the structure of plant-flower visitor network at the local and landscape levels in the northern Argentinian Yungas forest. Front. Sustain. Cities 5:1086076. doi: 10.3389/frsc.2023.1086076
Received: 01 November 2022; Accepted: 28 March 2023;
Published: 12 May 2023.
Edited by:
Gabriella Maselli, University of Salerno, ItalyReviewed by:
Pablo Torres-Lima, Metropolitan Autonomous University, MexicoJana RuŽičková, ELKH-ELTE-MTM Integrative Ecology Research Group, Hungary
Copyright © 2023 Amado De Santis, Lomáscolo and Chacoff. This is an open-access article distributed under the terms of the Creative Commons Attribution License (CC BY). The use, distribution or reproduction in other forums is permitted, provided the original author(s) and the copyright owner(s) are credited and that the original publication in this journal is cited, in accordance with accepted academic practice. No use, distribution or reproduction is permitted which does not comply with these terms.
*Correspondence: Alejandro A. Amado De Santis, aleamado_2@hotmail.com