- 1Regional Centre for Energy and Environmental Sustainability (RCEES), Department of Energy and Petroleum Engineering, School of Engineering, University of Energy and Natural Resources, Sunyani, Ghana
- 2Faculty of Engineering and Technology (FET), University of Buea, Buea, Cameroon
The Intergovernmental Panel on Climate Change (IPCC) 2021 report has noted the perceived rise in severe weather phenomena such as heat radiations, hurricanes, flooding, and droughts and the rising scientific evidence attributing these events to anthropogenic sources of climate change. Cameroon as a nation is equally exposed to these climate vulnerabilities, and contributing to global climate efforts is imperative. She has earmarked the integration of 25% renewables in its electricity production mix and a 32% emission reduction, all as part of her commitment to global climate action. The fresh commitments coupled with a rapidly growing power demand have paved the way for a revolutionized approach to electricity generation in Cameroon. However, the imminent changes, as well as their implications, remain uncertain. This study explores how these emission reduction targets can be achieved through the adoption of a more sustainable power transition, which provides realistic solutions for emission reduction, escaping high carbon pathways. The assessment of the level at which long-term electricity generation scenarios in Cameroon could be renewable energy intensive was done using the Low Emissions Analysis Platform (LEAP) tool following a backcasting approach. The study noted that there is an implementation gap between earmarked policy ambitions and existing measures. The study recommended several opportunities in aspects, such as suitable share of technologies, administrative reforms, and required adjustments within the Nationally Determined Contributions (NDCs), which the government could exploit in the electricity sector to sail across the challenging trade-offs needed to become a sustainable economy in a carbon-constrained world. It equally examines actions that could help close the gap between earmarked policy ambitions and existing pathways and proposes cost-effective methods that were identified as priorities.
1. Introduction
Global action toward increasing clean energy technologies is growing due to the role they play in energy security and climate change mitigation, as well as their perception as the most economically viable option (Iweh et al., 2021). As the perception is rising in the African continent, there are potentials to leapfrog the conventional centralized power grid model of energy supply to a more sustainable grid powered by renewables (Iweh et al., 2022a) since the uptake of clean energy technologies comes with extra benefits for economic productivity and socioeconomic development (Sen and Ganguly, 2017; Singh and Nyuur, 2019). Renewable energy makes up a small share of overall global primary energy consumption, contributing ~12% in 2020 (IRENA, 2021). The share of RE in the global electricity generation mix has experienced a surge in recent years, evidenced by a 16% growth from 2009 to 2019 (IRENA, 2020a). With over 271 MW of RE technologies added in 2020, global RE capacity for electricity generation reached 2,799 GW, contributing over 29% in 2020, according to the International Energy Agency (IEA). Driven by a drastic reduction in capital costs, the added capacity mainly constitutes solar photovoltaic (PV), wind energy, and hydropower (IRENA, 2020a). The installed capacity of renewables is projected to exceed 4,800 GW by 2026 (IRENA, 2021). However, this projected increase is mainly expected in the global north, with Europe, the United States of America, China, and India accounting for over 80% of this capacity. Despite the acute need for electricity in Africa, the continent contributes only 3% of the world's electricity generated from renewables even though there exist abundant RE potentials (IRENA, 2021; IRENA AfDB, 2022).
Cameroon, like most African nations, has contributed insignificantly to global greenhouse gas (GHG) emissions, with ~0.03% (6.5 MTCO2) only in 2019 (IEA, 2020a), and she has, however, set targets to further reduce her emissions. Among other international conventions, she has most recently ratified the Paris Climate Agreement, where various countries have set targets to transition to greener and environmentally friendly energy generation (UNFCCC, 2016a). The government has adopted the National Development Strategy for the period of 2021–2030 (MINEPAT, 2020; Bodjongo et al., 2021) with the main objectives of improving economic growth, accumulating national wealth, and advancing the necessary structural reforms that will facilitate the country's industrialization. To meet this goal, Cameroon intends to increase the installed power capacity to 5,000 megawatts (MW) by 2030 through diversified generation comprising hydroelectricity, solar, thermal (using natural gas as fuel), and biomass-powered power plants (Power Africa, 2019). The installed capacity during the first phase of “Vision 2035” (2010–2020) improved from 933 to 1,650 MW with a shortage of 1,350 MW when compared to the envisaged target of 3,000 MW in 2020 (MINEPAT, 2020). The national electricity access rate in Cameroon is 70% (IEA, 2020b) and has grown considerably compared to the previous years due to a series of projects such as the newly constructed Memve'ele hydroelectric station, the recuperation of the Limbe power plant, and the implementation of some solar photovoltaic (PV) projects. The urban electricity access rate is 98 and 32% for rural areas (IEA, 2020b), implying that an estimated 68% of rural communities are without electricity access in Cameroon. These statistics, which are a reflection of what is obtainable in other countries in sub-Sahara Africa (SSA), convey a narrow part of the reality as it conceals the repeated power outages as a result of the epileptic, outdated, and extremely unstable power grids in this region (Cole et al., 2018). This stresses the crucial necessity for more reliable and efficient unconventional energy systems. This shortfall in power production retards the expansion of economic activities and private investment.
Cameroon, in her NDCs, has earmarked a 25% RE share (excluding hydropower capacity exceeding 5 MW) in the power generation mix and a 32% reduction of GHG emissions (Cameroon Ministry of External Relations, 2015). This measure is quite an urgent step taken by Cameroon, especially as several countries are setting fresh long-term net zero targets or updated Nationally Determined Contributions (NDCs) (UNFCCC, 2016b) in the wake of the 26th Conference of Parties (COP26) held in November 2021. Advancing the renewable energy transition in Cameroon will simultaneously reduce emissions and narrow the irreconcilable difference between rural–urban electrification rates in the country. The dynamics of power demand and supply in Cameroon are pitiably irreconcilable, and the sector presents huge prospects for meeting the Paris Climate Accord through widespread renewable energy deployment. The question of what extent the various RE sources (hydro, wind, biomass, and solar) could contribute to Cameroon's electricity generation mix has not been adequately studied by scholars. While the earmarked 25% of renewable power injection has a huge potential to revolutionize Cameroon's power generation mix, government's current energy plans have not adequately captured the extent to which this can be achievable since the imminent changes, as well as their implications, remain uncertain. Therefore, the study aims to fill the literature gap in the Cameroon power sector by answering three fundamental questions facing the electricity sector:
• Do the current electricity generation expansion pathway and the RE deployment trend meet the 25% RE target in the generation mix by 2035?
• Is the current electricity generation expansion pathway the most cost-effective pathway or technology mix for attaining a 25% RE target?
• What alternative generation expansion pathway has the ability to meet the 25% stated RE target and on recent RE deployment trends?
Consequently, this study explores how the planned 25% renewable injection in the Cameroon NDCs could be achieved through the development of a backcasting energy model using the LEAP tool. This model, to the best of our knowledge, is the first integrated energy model for Cameroon available in the energy studies literature. This approach is pertinent for Cameroon as most of the available literature (Bautista, 2012; Lkhagva, 2014; McPherson and Karney, 2014; Senshaw, 2014) has used LEAP models in different countries. This study adds to the literature on LEAP-based country-level studies on power sector planning scenarios in Ghana (Awopone et al., 2017a), Ethiopia (Senshaw, 2014), Greece (Roinioti et al., 2012), and Taiwan (Huang et al., 2011). The study conducted RE allocation-based analysis in the power network and evaluated their potential economic and environmental impacts on Cameroon's power sector. The model used local energy statistics and data on the recent and future plans regarding energy production and transformation in Cameroon. These data were supported by economic and demographic information as well as the GDP. The study finally suggests the need to review and update the existing RE policies and master plans as well as the current NDC document with readjustment in the renewable quotas and timelines allocated to the various technologies as outlined in the modified scenario. The article highlights the RE deployment trends in the country to trigger policy discussions among stakeholders.
This study starts with a presentation of the Cameroon country profile with an overview of the existing situation in the Cameroonian energy sector. The article further reviews the state of energy supply and consumption in Cameroon. Part of the study involved a quantitative description of the climate change vulnerability in Cameroon and a synopsis of initiatives and policy decisions undertaken in Cameroon. Moreover, the study describes the LEAP tool adopted in the study where aspects like scenario setting, future electricity demand and supply, GHG emissions assessment, cost and benefit study, and cost-optimized power generation. In addition, the study discusses the results obtained from the various scenarios with emphasis on sustainable policy impact in the country. The study concludes with some recommendations, citing some available options that the government could use to advance clean energy in the country.
2. Methods, materials, and the rationale
This study is an analytical and informed evaluation of the state of the electricity sector in Cameroon and some proposed reforms to drive the renewable energy (RE) transition in the power sector. The LEAP tool was used in modeling and simulating the scenarios following an energy backcasting approach. The backcasting energy modeling approach explores past events based on the already known information, i.e., stating a desired future and subsequently working backward to determine strategies and policies that are going to link the future to the existing situation (Brandes and Brooks, 2005). The forecasting approach explores future scenarios with no prior knowledge using the assessment of existing trends (Holmberg and Robèrt, 2000), and this is seemingly the most commonly used modeling method. LEAP is an acronym for Low Emissions Analysis Platform, formerly called Long-range Energy Alternatives Planning, a property of the US-based Stockholm Environment Institute (2021) used in energy policy and climate change evaluation. This tool has seen applications in energy consumption monitoring, sectorial energy resource management, and energy-based greenhouse emission accountability (Lee et al., 2008; Huang et al., 2011; Taoa et al., 2011; Bautista, 2012; Lkhagva, 2014; McPherson and Karney, 2014; Senshaw, 2014; Kemausuor et al., 2015). The current study is on a developing country like Cameroon, with the most often scanty data to build a comprehensive energy model. Hence the LEAP tool is appropriate for the research because it is effective in monitoring energy consumption and conversion in developing countries; it has the capability of setting up energy integration scenarios with a rich environmental assessment database; it comprises several energy technologies with both clean power technologies for industrialized nations and conventional systems (common in emerging nations), and above all, the tool requires relatively less initial data with the possibility of improving the model when complete data for the research area becomes available. The power transition model takes into consideration all the existing and future power plants from the reference year of 2015 to the target year of 2035. Three scenarios are developed, and the allocation of power generators to meet the electricity demand at a particular time is based on the 25% renewable energy target in the Cameroon NDCs by 2035, the GDP, the load duration curve, and the mean plant capacity factor which are entirely stated exogenously. The cost of power generation from specific technologies over time, the carbon tax, and power losses from transmission and distribution (T&D) networks are also considered. The key outputs obtained from the scheme is the growth of electricity generation through various technologies, comprehensive economic inferences of the modeled scenarios, and the trend of emissions from the base year (2015) through 2035.
The Cameroonian LEAP model offers a backcasting energy approach to Cameroon's energy sector, and it is, so far, the first attempt in the Cameroonian context. The three unique scenarios explore the probability of delving into the huge prospects in Cameroon's energy future. With many sources of uncertainty in the future, using several modifications in the scenarios increase the opportunity of identifying a potential future energy pathway for achieving the Cameroon NDC target. This research contributes to augment the policy literature on the available options for improving the deployment of renewable energy, specifically by analyzing the energy supply/consumption of Cameroon, climate change vulnerability, the state of the power sector, and proposals to mitigate these challenges. This is done through the evaluation of the impact of policy (NDCs assessment), cost–benefit study, and cost-optimized power generation.
Data for this study were obtained from the Cameroon Ministry of Economy, Planning and Regional Development (MINEPAT, 2009a), the Cameroon Ministry of Energy and Water Resources (MINEE, 2015), and Cameroon's National Institute of Statistics (Institut National de la Statistique, 2016), annual reports of the country's energy utility (ENEO, 2019), and the Electricity Sector Regulatory Agency (2015), country reports from international institutions such as the International Renewable Energy Agency IRENA (2020b), World Bank (2021), and IEA (2021). Supplementary sources of information were obtained from energy policy-related official documents, desk studies, and online literature. A clear methodology to develop these pathways would assist in informing policy, evaluating required modifications to the current trajectory, enhancing comprehension and acceptance of the proposed ways of meeting the NDC renewable energy targets, and assuring investors that renewable energy transition can be achieved in Cameroon. Figure 1 shows the methodology for the analysis.
Focusing on the electricity sector makes an interesting case since research on industry and country level in Africa shows huge consequences as a result of interrupted electricity network: As the occurrence of power outages increases by 1%, the output of a firm is estimated to reduce by 3.3% in a short run, and the gross domestic product (GDP) per capita reduces by 2.9% in the long run (Andersen and Dalgaard, 2013; Mensah, 2018). The disruption of services, such as electricity, and water, heavily impacts small firms with a low ability to cope, and this limits entrepreneurship and competition (Alby et al., 2013; Poczter, 2017). Moreover, defective utility infrastructure reduces the number of industries hosted by a country and hence weakens its attractiveness to international investors (World Bank, 2019). These dynamics usually fall back on the citizens through reduced employment and increased costs for consumers. The Cameroon Ministry of Economy, Planning and Regional Development reports losses of 5% of annual GDP as a result of an insufficient and unreliable supply of power. Hence, there is an urgent need for reliable and sufficient power supply because the distressing rationing system, the reduced industrial activity, the loss of jobs, and disturbances in public life are revelations of what presently seems to be a recurrent hindrance to Cameroon's development program.
2.1. Conception of scenarios
The study built scenarios from a common reference scenario, out of which three additional scenarios (alternative) were generated to achieve a RE-intensive and low-carbon power system in Cameroon. The three other scenarios developed are the stated scenario, the modified scenario, and the optimized scenario. The reference scenario was conceived from the Cameroon government's energy policy ambitions in the Electricity Sector Development Plan, the RE Master Plan, and the Rural Electrification Master Plan (REMP) (MINEE, 2006; AER, 2017; Korea Energy Economics Institute, 2017), which runs from 2015 to 2035. These three other scenarios (alternative) assess the possibilities and costs of meeting Cameroon's NDC targets by 2035. Based on the methodology for scenario planning originally presented by Schwartz (1991), a five-step scenario development approach was adopted. These steps consist of (i) identification of the focal issue to be examined; (ii) determination of key factors influencing the focal issue; (iii) evaluation of key factors to get the critical factors; (iv) selection of scenario logic based on the identified critical factors; and (v) analysis of resulting scenarios. This adapted methodology was used to develop the business-as-usual scenario and the three alternative scenarios. The three scenarios express various pathways to achieving the 25% renewable energy target earmarked in the Cameroonian NDCs. These scenarios are characterized mainly by progressively more aggressive allocation of the RE technologies into the power generation mix.
2.1.1. The business-as-usual (BAU) scenario
The BAU scenario is based on the nation's current electricity generation trajectory and planned power system measures as outlined in the policy document on the Energy Sector Development Plan (PDSEN) (MINEE, 2006; Korea Energy Economics Institute, 2017) with objectives to exploit Cameroon's abundant hydropower potentials, conversion of older oil-powered plants (Limbe, Ahala, Logbaba, Dibamba, Bamenda, Oyomabang, Mbalmayo, and Ebolowa thermal plants) to natural gas plants while increasing capacities of existing natural gas plants (Kribi gas plant). Development of renewable energy technology sees a slower growth rate compared to adoption rates in the RE Master Plan and Rural Electrification Master Plan (REMP) (AER, 2017; Korea Energy Economics Institute, 2017), the NDCs (Cameroon Ministry of External Relations, 2015), and the “Vision 2035” (MINEPAT, 2009b). This policy document shows the country's 20-year power generation plan, where investments in power generation will be guided by the pillars in the report. The BAU scenario presents the evolution of Cameroon's power generation from 2015 (base year) up to the year 2035 with no substantial new power sector policies except the pre-existing ones considered in the scenario. New capacity under this scenario and alternative scenarios is assumed to be added in 2022. Electricity demand projections are mostly influenced by projections in GDP and increase in population. In the base year (2015), power generation in Cameroon was dominated by hydropower (55%), followed by fossil fuel (44%) and, finally, renewables (1%). The total installed capacity used in the base year was 1,361.50 MW, and the various capacities are shown in Table 1. These data were obtained from the Cameroon Ministry of Energy and Water Resources.
2.1.2. The stated scenario
Cameroon NDC targets aim to achieve a 25% share (5,953.8 GWh out of the 23,815.2 GWh) of RE in the generation mix by 2035, as stated in the NDCs (Cameroon Ministry of External Relations, 2015). Unlike the BAU scenario, the stated scenario assumes the renewable energy target is met in the initially specified generation ratio on the policy document submitted by the government to the UNFCCC's secretariat. This scenario is the basis for the backcasting approach used in this study. The renewable energy ambitions within the Cameroon NDCs anticipate power generation by 2035 from non-renewable large hydro (15,607 GWh), small hydro (2,579 GWh), wind energy (464 GWh), solar PV (1,345 GWh), biomass (1,611 GWh), and natural gas (1,882 GWh). In the same scenario, there are plans to generate electricity from petroleum products for up to 928 GWh with no intentions to use coal-fired power plants. Unlike the BAU scenario, the stated scenario assumes the renewable energy targets are met in the officially specified energy generation ratio. Figure 2 shows the percentage of the various sources of power used in this scenario.
2.1.3. The modified scenario
The modified scenario was conceived on the basis that the renewable energy mix in the BAU and stated scenarios (official NDC targets) do not represent the ideal renewable energy mix in Cameroon. For example, an 11% target of small hydro (Figure 2) in the total generation mix by 2035 amid abundant solar resources raises concerns about the applicability and sustainability of small hydro installations in Cameroon. A 7% biomass share (Figure 2) also raises economic concerns with regard to the food–energy nexus in addition to the unpopular nature of processes involved in biomass-powered plants (such as pyrolysis, gasification, fermentation, and combustion), especially in developing countries like Cameroon. Depending on feedstock properties, power generation from biomass also raises environmental issues, among others, like terrestrial acidification and particulate matter formation (Paletto et al., 2019). There were no considerations in the NDCs to identify generation technologies that have the potential to generate the least cost and more adaptable power in the Cameroonian context. This aspect is essential because it guides decisions in determining the most economical generation expansion plan since investment in power technologies mostly depends on cost and emission capacity. Thus, the various technology targets in the NDCs do not express the least-cost pathway for renewable energy development in Cameroon, and there is, therefore, a need to redevelop the Cameroon NDC scenario that considers actual realities like resource availability, ease of use of technology, access to system components and economic viability of the technology. The modified scenario is different from the stated scenario in that the various renewable energy technology share targets were developed based on the researchers' knowledge of Cameroon's power system structure, levelized costs of energy (LCOE), and applicability of each technology, and the country's rural electrification targets.
2.1.4. The optimized scenario
The optimized scenario typically seeks the least-cost pathway to achieving the 25% renewable energy target by 2035. The main difference between the optimized and the other scenarios is that the LEAP and the OSeMOSYS optimization model were enabled to endogenously add the required capacity necessary to meet demand and the 25% renewable energy target. The optimized scenario aimed at identifying the generation expansion pathway that adequately meets electricity demand at the lowest discounted net present value (NPV) costs over the entire study period. The cost minimization objective in OSeMOSYS was subject to constraints of capital costs, fixed and variable operation and maintenance costs, and externality costs. The dispatch and capacity addition were also determined by the LEAP/OSeMOSYS framework (Awopone et al., 2017a; Stockholm Environment Institute, 2022). OSeMOSYS is an independent optimization tool that has been integrated into the LEAP environment and used for long-term energy planning. It is modular and capable of building sophisticated energy models with relatively small data requirements. This scenario operates on the assumption of a more aggressive policy instrument with high goals of radically increasing economic growth and the country's commitment to cheap, environmentally friendly energy technologies than the modified scenario. This scenario also assesses the total cost of achieving the 25% renewable energy target from 2015 to 2035, unlike the modified scenarios, which start the assessment in 2022.
2.1.5. Main assumptions in the model
The base year of 2015 and a bottom-up scenario modeling methodology were utilized for this study. The year 2015 was chosen due to data availability and its overlapping nature on most future energy expansion documents in the country. This also provided an opportunity to validate the model results against subsequent past years. Hydropower installations of ≤ 5 MW in Cameroon are classified as RE systems. The RE sources considered in this study are small hydro, solar PV, wind, and biomass. A fixed discount rate of 10% was considered for the duration of the study.
Cameroon's GDP under all scenarios was assumed to grow from USD 32.21 billion in 2015 to USD 82.06 billion, an average growth rate of 5% annually (World Bank, 2021). The population was also assumed to grow at 2.5% annually, i.e., from 23.3 million in 2015 to 38.18 million by 2035 (World Bank, 2021). A household size of 5 was used for this study (United Nations, 2014; World Bank, 2021). However, total national electricity needs were projected to rise at an annual average of 6.7% from 2015 to 2035, according to official projections from the RE master plan and the PDSEN (MINEE, 2006; Korea Energy Economics Institute, 2017), and this value was used in the model. Household electricity access rates were also assumed to grow according to national projections. In addition, T&D losses increased from 36.27 in 2015 to 38.93% in 2019 and were then assumed to reduce to 25% by 2035 (ENEO, 2017, 2019). This assumed power loss reduction is based on the utility company's earmarked measures, such as the deployment of prepaid meters and the Supervisory Control and Data Acquisition (SCADA) systems intended to reduce system losses. The reserve margin was also assumed to grow from 5% in 2015 to 10% by 2035. The total electricity demand in Cameroon was 5.41 TWh in 2015 (ENEO, 2017), with an overall installed power capacity of 1,315.79 MW (MINEE, 2015) consisting mainly of large hydro (55%) fossil fuel (44%) and renewables (1%). An hourly temporal resolution was considered to account for the intermittency of variable RE sources. Thus, a yearly shape consisting of the total electricity demand of Cameroon for each hour of the year (a total of 8,760 h) was used. Figure 3 shows the annual shape of electricity demand used in the study.
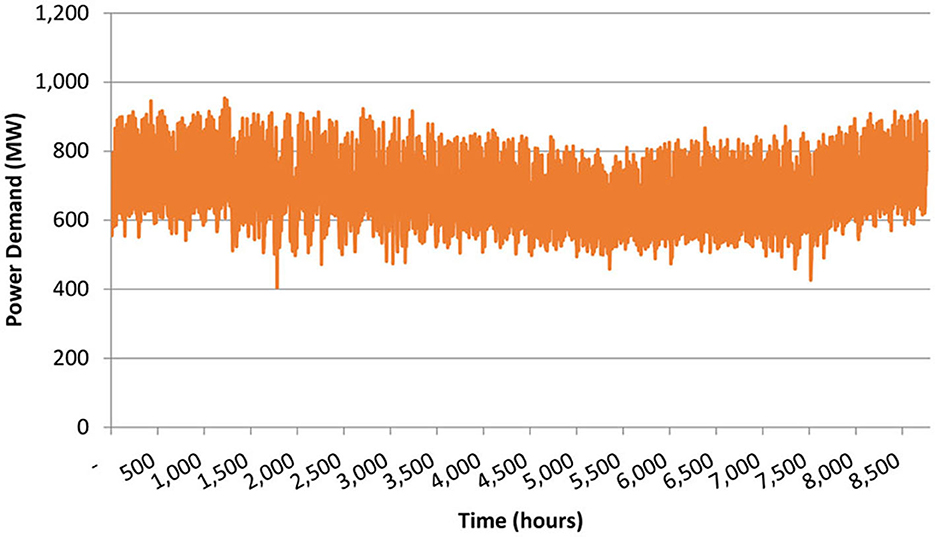
Figure 3. Annual shape of electricity demand in Cameroon in 2019 (source: authors' construct from data provided by ENEO).
The costs of electricity generation by technology considered in this study are the capital costs and operation and maintenance (O&M) costs. These costs (Table 2) were obtained from IRENA Renewable Energy Costs 2019 (IRENA, 2020b) and the NREL Annual Technology Baseline (National Renewable Energy Laboratory (NREL), 2020). Fossil-fuel price projections over the study period were obtained from the World Energy Model 2020 and the Annual Energy Outlook 2021 (Energy Information Administration (EIA), 2021; IEA, 2021). Natural gas prices were projected to increase from 3.2 $/mBtu in 2015 to 3.8 $/mBtu in 2035, while crude oil prices increased from 40.76 $/barrel in 2015 to 110.4 %/barrel in 2035. Table 2 shows the cost of generation technology, while Table 3 shows the fuel cost projections used in this study.
For the characteristics of the generation technology used in the model, Table 4 summarizes the capacity factors and the power factors of the plants. The study assumes that there are sufficient transmission and distribution capacity and grid improvements over the course of the generation expansion period.
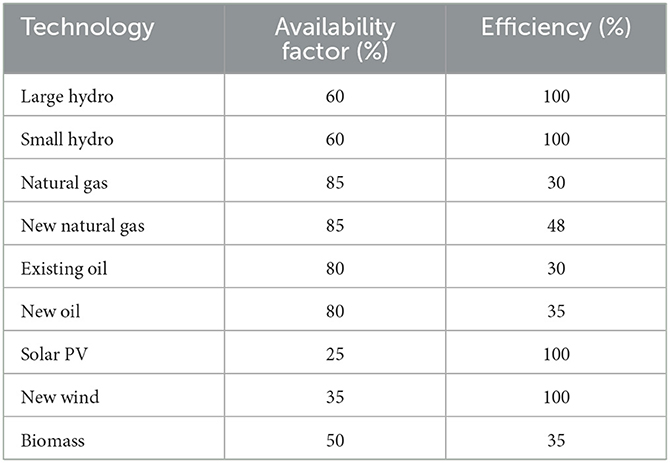
Table 4. Power generation technology characteristics in the study (International Renewable Energy Agency, 2020).
The seasonal profiles for solar PV, wind, and hydropower in the LEAP model were considered as annual average values, and they were modeled in the form of availability. Availability or the availability factor is the percentage of time a power plant can generate electricity in a specified period (8,760 h for this study) (IEA, 2020b; Stockholm Environment Institute, 2021).
2.2. Sensitivity analysis
Although perfect foresight is assumed in this study, electricity generation costs are dependent on several factors, such as discount rates, technology capital costs, O&M costs, and feedstock fuel costs. These factors are largely uncertain and cannot be accurately forecasted. However, feedstock fuel costs are arguably the most volatile, given that they depend largely on external stimuli. Thus, a fuel price sensitivity analysis is conducted to assess the impact of feedstock price changes on the cumulative costs of the scenarios. A fuel price uncertainty of ±50% was adopted in this study. This assumption stems from the high instability of fossil-fuel prices in recent years.
3. Cameroon country profile
Cameroon is within the Central Africa sub-region, and the country is situated at a latitude of 6° North and a longitude of 12° East (Kwaye et al., 2015). The country is exposed to a fairly good solar radiation, with an average value of 4.9 kWh/m2/day (IEEE, 2018). Electricity generated from hydro resources dominates the generation mix, and only <5% of the country's potential is under exploitation (ANDRITZ Hydro, 2019). Despite the enormous RE potentials, Cameroon has no clear RE policy to harness these resources for power generation (Njoh et al., 2019). The country's institutional energy policy structure provides some faint information on government's position toward renewables as a possible source of power generation in Cameroon. Again, this is only limited to some renewables like solar, hydro, and wind with no details on how private stakeholders' interest can be guaranteed if they decide to invest. These modern energy services provide a huge potential for local community empowerment since the resources can be exploited locally and at a small scale, supporting rural development and electrification. However, this requires strong policy objectives, enabling regulations and a balanced institutional setup in addition to viable business models to accelerate renewable energy deployment.
At the national level, according to the Ministry of Finance, the economic growth rate was estimated at 3.9% in 2019, compared to a 4.1% rate recorded in 2018. This drop in economic activity falls within a context characterized by the persistence of the socio-political crisis in the North West/South West Regions, as well as the terrorist threats in the Far-North Region, the fire incident at the National Oil Refinery that occurred in May 2019, and a significant increase in oil and gas production. Concerning inflation, it rose significantly by 2.5% in 2019 compared to 1.1% in 2018 (ENEO, 2019).
3.1. Cameroon energy supply/consumption
The primary supply of energy in Cameroon comes from biofuels and waste (70.58%), followed by crude oil (20.17%), natural gas (5.34%), hydropower (3.90%), and other renewable sources (0.01%) like solar, geothermal, and wind. Historical trends in energy supply showed a steady rise in energy supply from 1990 to 2005, with a recession from 2005 to 2007 before steadily rising right up to 2018 (Figure 6) (IEA, IRENA, UNSD, World Bank, WHO, 2020). The most used forms of energy in the country are biomass (74.22%), petroleum products (18.48%), and electric power (7.30%). The country's overall energy usage in 2018 was estimated at 7.41 Mtoe; made up mostly of traditional biomass.
Overall energy consumption within sectors shows 63.68% for the residential sector, followed by 14.92% for both the public service and commercial sector, the transport sector (13.82%), the industry sector (5.15%), the agriculture/forestry sector (0.07%), non-energy use (0.88%), and other sectors (1.48%), as shown in Figure 4 (IEA, IRENA, UNSD, World Bank, WHO, 2020). Figure 4 shows the percentage of energy consumption by sector, and Figure 5 shows the trend of primary energy usage by source in Cameroon from 1990 to 2019. Figure 6 shows the trend of primary energy supply by source in Cameroon from 1990 to 2019, while Figure 7 showed the percentage of primary energy supply in Cameroon in 2019.
3.2. Climate change vulnerability and carbon dioxide (CO2) emission in Cameroon
The United Nation Development Program's (UNDP's) climate change report on Cameroon (UNDP, 2008) indicates a mean annual temperature rise of 0.7°C between 1960 and 2007, implying an average temperature rise of 0.15°C per decade. The report further showed that the temperature increase in the country was highest (0.19°C per decade) in the months of April and May. Nevertheless, the Northern part of the country had the months of January, September, November, and December with the highest temperature rise (0.2–0.4°C per decade). Temperature forecasts, according to data from the UNDP, predicted an average annual temperature rise of 1.0–2.9°C by 2060 and 1.5–4.7°C by 2090 (Ngnikam and Tolale, 2009). The projected temperature rise was more in the Northern and Eastern parts of Cameroon and lesser in the coastal areas.
The annual rainfall in Cameroon dropped by 2.9 mm each month, implying a 2.2% per decade from 1960. The years 2003 and 2005 have witnessed low rainfalls in the country, and forecasts of average annual rainfall are expected to change from −12 to +20 mm per month (−8 to +17%) in 2090, implying a +1 to +3 mm per month (0–2%) (UNDP, 2008). There are concerns that Cameroon's lowland coastal zones could be susceptible to rising sea levels (Ngnikam and Tolale, 2009). All these projected vulnerabilities justify why the country needs to target sectors such as the power sector with the potentials to mitigate these future problems.
Cameroon has a generally insignificant emission history, with the power sector contributing a small amount (~3.95%) of the total emissions of the country (USAID, 2014). Although the power sector relatively contributes very little to the country's overall emissions (MINEE, 2015), improvements in the power sector will indirectly reduce emissions in other sectors, such as industry, where some of their production operations can be done with the use of clean power. As part of her commitments to the Paris Agreement, Cameroon has set to reduce GHG emissions by 32% by 2035 compared to a reference scenario (Cameroon Ministry of External Relations, 2015). Agriculture represents more than half of the overall emissions in the country. Carbon dioxide (CO2) emissions from energy combustion in the country were stable at ~6 MTCO2e in 2014, after an annual growth rate of 8% per year between 2006 and 2014 (ENERDATA, 2019). The country's per capita emissions are similar to that of most sub-Saharan African countries (excluding South Africa) at a value of 0.2 TCO2/inhabitant in 2019 (ENERDATA, 2019). Figure 8 shows the emission history of Cameroon. Due to the growing electricity demand in Cameroon, a low-carbon power network is crucial to decreasing CO2 emissions from other sectors like industry, transport, and buildings.
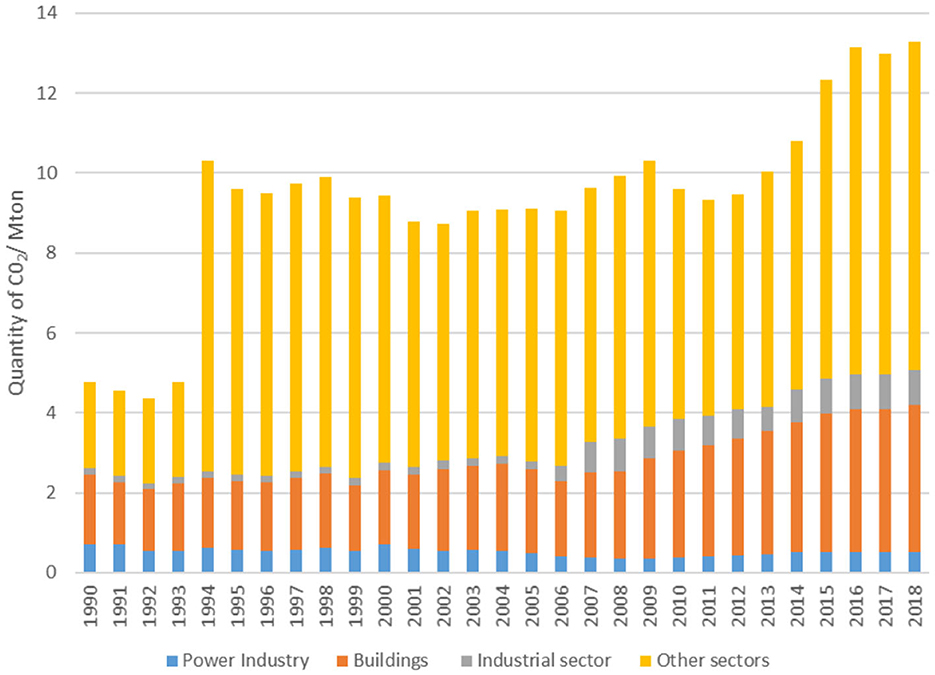
Figure 8. Sectorial trend of CO2 emissions in Cameroon from 1990 to 2018 (Crippa et al., 2019).
3.3. Developments in the power sector in Cameroon
Power generation and distribution in Cameroon are managed by Energy of Cameroon (ENEO). This para-statal company went operational on 12 September 2014 through the purchase of part of the shared power company between the state of Cameroon and an American company, la Société de l′Electricité du Cameroun, branded by its French acronym as AES-SONEL. Before then, a national power company with its French acronym SONEL was created in 1974 through the amalgamation of the Cameroon Power Company (POWERCAM) of the former British Southern Cameroons and the electricity corporation (Electricité du Cameroun) of the former French Cameroun (Njoh, 1992). The deregulation of the power sector led to the privatization of the state-run SONEL (power utility) in 2001. The production and T&D of electricity were divided in 2011 and were all managed by AES-SONEL right up to September 2014 when ENEO took over and continued before the taking over of the transmission section by the state-owned Société Nationale de Transport d'Electricité (SONATREL) in 2018 (Marcos et al., 2017).
According to ENEO (energy utility) 2019 report, 51% of the company's shares are owned by Actis, a venture group in the United Kingdom, whereas 44% of shares are owned by the government of Cameroon and 5% by its employees. The company's generation sources include; 73.30% hydro, 26.66% thermal, and 0.04% solar. As of 2019, ENEO's installed power generation assets were up to 998.939 MW from 37 power plants, divided into 13 grid-connected and 24 off-grid thermal plants. ENEO's contribution to Cameroon's electricity generation amounts to 65.42%, Memve'ele upto 13.82%, Kribi Power Development Company (KPDC) upto 14.15%, Dibamba Power Development Company (DPDC) upto 5.63%, and AGGREKO upto 0.98% (ENEO, 2019). The transmission grid has 24 substations, an overall line length of 1,944.29 km of HV (high voltage), 15,081.48 km of MV (medium voltage), and 15,209.25 km of low voltage (LV). The distribution network measures up to 11,450 km of 5.5–33 kV and 11,158 km of 220–380 kV. As of 31 December 2019, ENEO had over 1,360,027 customers, with over 45% in Douala and Yaounde (ENEO, 2019). Cameroon has 2,327 MW of installed capacity (GET.invest, 2020), dominated by large hydroelectric and thermal plants, and the government has continued to plan toward the rising demand by prioritizing the development of large hydropower and thermal power plants.
Cameroon operates three independent grids, namely: the Southern Interconnected Grid (SIG), the Northern Interconnected Grid (NIG), and the Eastern Isolated Grid (EIG) (ENEO, 2019). Presently, electric power transfer among the three grids does not exist, but there are, however, imminent plans within the timeframe of 2030–2035 to connect the 3 grids to form a common network. At the moment, the various grids have different transmission voltages; 90 and 225 kV for the SIG, 90 and 110 kV for the NIG, and 30 kV for the Eastern network (ENEO, 2017). The grid (T&D) grew from 15 to 30% between the years 2010 and 2014, with the network suffering from under-investment (MINEE, 2015). Power losses are severe in the distribution network (32.03%) and less in the transmission network (6.3%) (ENEO, 2019). The National Electricity Transmission Company, a state-run power transmission enterprise, manages the transmission grid. Regarding future power expansion and interconnection of the 3 grids, transmission voltages of 225 and 400 kV are expected to be developed with the aim of exporting electricity to Nigeria, Chad, Central African Republic, Congo, and neighboring countries.
4. Simulation results and discussion
The electricity demand, generation capacity and associated costs, and corresponding environmental emissions are presented in this section. To clearly interpret the results, all the outputs of the various scenarios are discussed on the basis of power demand, installed capacity, the cost–benefit analysis, and environmental assessment. The analysis is based on the power sector with a backcast from the 25% RE target stated in the Cameroon NDCs. The results obtained from various scenarios are presented using charts for simplicity.
4.1. Electricity demand
The Cameroon electricity demand from 2015 to 2035 under all four scenarios is shown in Figure 11. The same demand growth was used for all scenarios, and it increased from 5.41 TWh in 2015 to 19.79 TWh in 2035. The 2035 value of 19.79 TWh was lower compared to official projections of 23.73 TWh, an indication that the set ambitions failed to consider trends in generation expansion. This is attributed to a slower capacity addition than originally planned by the government. This is further supported by a 15% deviation of projected values between 2015 and 2019 in the Energy Sector Development Plan (PDSEN) (MINEE, 2006) compared to actual demand published by the electric utility company ENEO (Korea Energy Economics Institute, 2017; ENEO, 2019). Power demand growth is influenced by the rise in population and urbanization.
Another reason for the high government demand projections is based on the wish expressed in the National Energy Action Plan for Poverty Reduction (MINEE, 2005), the Vision 2035 (MINEPAT, 2009b), and the GESP (MINEPAT, 2009a), where the government plans to achieve universal access by 2035 and the need to minimize unhealthy cooking practices that relies on the intensive use of biomass to be replaced with electricity and liquefied petroleum gas (LPG). This involves the deployment of widespread energy systems, especially in rural areas, to improve the livelihoods of its inhabitants. Figure 9 shows the trends in Cameroon's power demand from 2015 to 2035.
4.2. Installed generation capacities
The demand growth and reserve margin increased from 5 to 10%, while the installed capacity increased from 1.32 GW in 2015 to 4.68 GW by 2035 under the BAU scenario. This scenario has observed the generation capacity that has more than tripled within the 20-year period. The percentage increase corroborated similar studies by Awopone et al. (Awopone et al., 2017b) in Ghana, where installed capacity was predicted to increase by over three times within a similar study period. From the simulation, the installed capacity in 2035 under the stated, modified, and optimized scenarios were, respectively, 4.89, 5.30, and 4.66 GW. Power generation in all scenarios was through large hydro, natural gas, thermal, and renewables consisting of biomass, small hydro (<5 MW), solar, and wind. The fuels presently used in thermal plants are heavy fuel oil (HFO), natural gas, and light fuel oil (LFO). However, power plants using HFO and LFO will be phased out by 2035, with natural gas exclusively used in operating these thermal plants. Power generated from biomass sources includes municipal solid waste and agriculture waste (timber waste, sugar cane waste, waste from oil palms, and cocoa waste) with prospective generation technologies that involve combustion and anaerobic digestion. The generation of power from these RE technologies is advantageous in that power is produced and utilized around load centers (distributed generation) or fed into the power grid. The total installed capacity under the various scenarios is shown in Figure 10.
From Figure 10, additional capacity of 0.21 and 0.62 GW are, respectively, needed in the stated and modified scenarios, while a capacity of 0.21 GW less is needed under the optimized scenario. The modified scenario has the highest installed capacity due to the consideration of more solar PV in achieving the 25% renewable energy mix. Higher total installed capacities in the alternative scenarios compared to the BAU scenario was as a result of the relatively low-capacity credit of renewable energy sources compared to conventional generation. This agrees with findings from studies in Ghana (Awopone et al., 2017a,b) and Panama (McPherson and Karney, 2014).
Large hydropower continues to be the dominant source under all scenarios, with shares of 58.7, 50, 46.1, and 55.4% under the BAU, stated, modified, and optimized scenarios, respectively, in 2035. This is backed by the fact that Cameroon has huge hydropower potentials (23 GW) that needs exploitation, especially as the country intends to export electricity to neighboring Chad (AfDB, 2017), as highlighted in her National Development Strategy (2020–2030). Similarly, renewable energy constitutes 12.9, 27.8, 33.3, and 20.2%, respectively. Energy generated under all scenarios increased from 6.5 TWh in 2015 to 26.38 TWh by 2035. This represents an increase of 5% from 2015 to 2035. The increasing impacts of climate change in sub-Saharan Africa, causing periodic droughts, drop in reservoir water levels, and subsequently, electricity crises, especially in the dry season, have seen a reduced dependence on hydropower in many countries. This is illustrated in generation expansion studies in Nigeria (Aliyu et al., 2013; Emodi et al., 2017), Ghana (Awopone et al., 2017a,b), and Africa (Ouedraogo, 2017), which see a greater increase in shares of natural gas and renewables. Although Cameroon has a huge hydropower potential which is poised to play a key role in the Central African Power Pool (CAPP), the nation ought to reconsider her generation expansion objectives in the context of her energy security (Kenfack et al., 2021). Renewables should be prioritized for development as in the alternative scenarios. The percentage share of various technologies under the different scenarios in 2035 is shown in Figure 11.
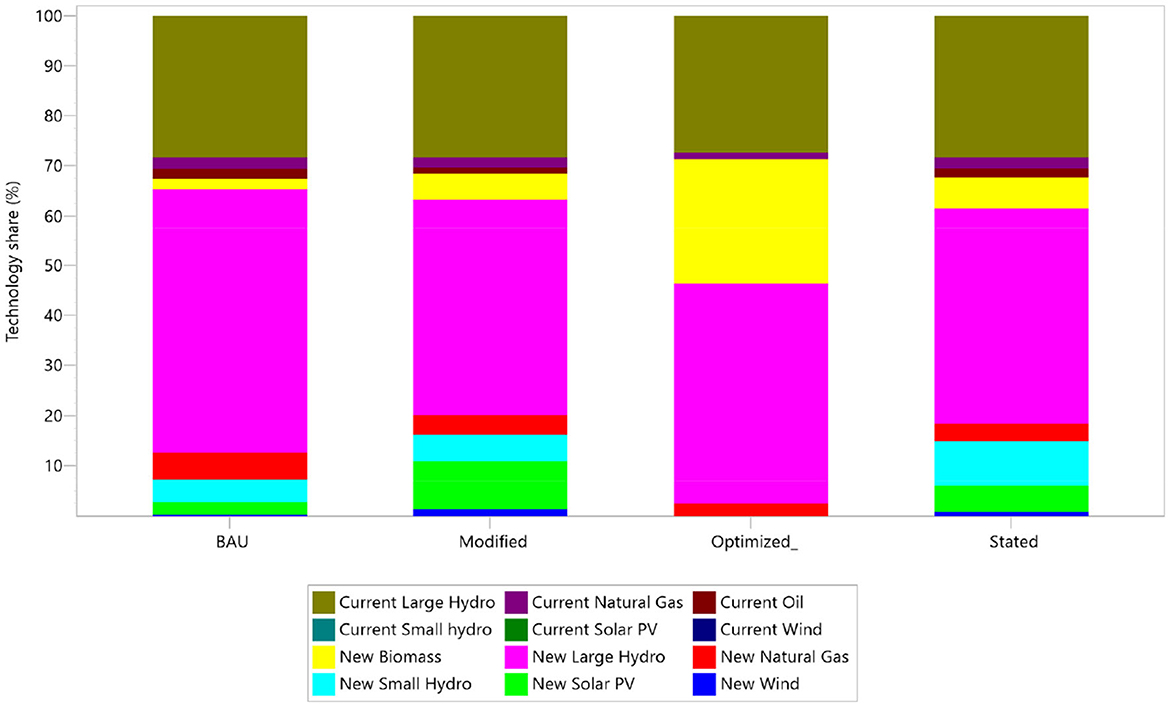
Figure 11. Percentage share of electricity generation by technology in 2035 under various scenarios.
Under the optimized scenario, the main endogenously added renewables are biomass (New Biomass), which occupies the entire 25% share of renewable injection. This is due to the consideration of biomass to be the least-cost renewable energy resource in Cameroon owing to the fact that Cameroon is part of the Congo basin forest with 25,000,000 hectares of forest coverage (three-fourths of her landmass), ranking her the third country in sub-Saharan Africa in terms of biomass potentials. In general, this scenario would have been the best option; however, given that energy projects in developing countries are influenced by government's ambitions, accessibility of technology, and contextual adaptability, this scenario is not suitable for Cameroon.
Cameroon generates billions of tons of waste yearly from agricultural and agro-industrial activities as well as hospitals which are causing huge environmental problems. This waste has no commercial value in Cameroon, and transforming this waste into electricity could potentially solve the environmental pollution issue and the huge power deficit in the country by embarking on biogas production, which could be further used as fuel for electricity generation. This also has the capability to increase employment opportunities and create new revenue streams within the country. It is worth noting that Cameroon is the breadbasket of the CEMAC sub-region with a commendable agricultural prowess, where the farming sector is driving the economy and safeguarding the food security of inhabitants. Major crops produced include maize, cassava, millet, rice, sweet and Irish potatoes, macabo, taro, yam, peanut, sorghum, bean, and soy (Vintila et al., 2019), which are generating huge waste. These wastes could also be transformed into biofuels that can power generation systems. Cameroon is the biggest coffee and cocoa producer in the Central Africa sub-region (Vintila et al., 2019) and also has an annual cattle production of 7 million, 8 million small ruminants, 2 million pigs, and 50 million poultry (Tagne et al., 2021). In addition, Cameroon is one of African's main palm oil producers (Gelder and German, 2011), with a production capacity of ~210,000 tons of palm oil in 2011 (Feintrenie, 2012). All these activities generate a large amount of waste that could be used for power generation without necessarily indulging in indiscriminate forest exploitation. Table 5 shows the annual agricultural biomass residues in Cameroon.
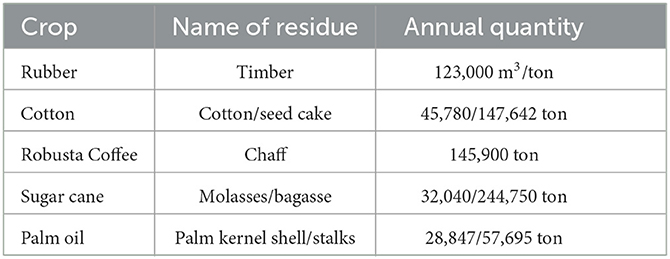
Table 5. Potential of agricultural biomass residues available in Cameroon (Ngnikam et al., 2009).
More attention is given to petroleum and large hydropower, with gross neglect of the huge amount of waste that could be used for power generation. There is no national policy on the exploitation of biofuels into useful electricity. The regulatory framework involving policy formulation to effective implementation does not exist. Given that strategic policy is the driver of positive change, putting in place appropriate policies and regulatory frameworks would assist in upscaling the level of waste to electricity in the country. This is why the optimum scenario, which is dominated by biomass, is illusive for Cameroon at the moment.
4.3. Economic analysis
The cumulative net discounted costs (fuel costs, capital costs, and O&M costs) at a 10% discount rate and 2015 US dollars under all scenarios are shown in Figure 13. Under the BAU scenario, the cumulative discounted costs [net present value (NPV)] reached $1,490.8 million in 2035. The stated and modified scenarios witnessed additional costs of $93.2 and $122.3 million, respectively, required to meet the 25% renewable energy mix in the NDCs. Contrarily, the optimized scenario witnessed a cost reduction of $87.6 million, indicating extra financial investments in the alternative scenarios (bar the optimized scenario) will be needed to enable Cameroon to achieve her 25% renewable energy target. Figure 12 shows the net present value of various energy technologies under all scenarios from 2015 to 2035.
An environmental externality cost of $5/MTCO2e in 2022, which rises to $15/MTCO2e by 2035, was introduced in this study. This aimed to highlight the environmental impacts of attaining the 25% renewable energy targets under different pathways and quantifying the potential revenue if carbon pricing was to be introduced in Cameroon. Under the BAU scenario, a cumulative carbon revenue of up to $27 million is achievable. However, the stated, modified, and optimized scenarios witnessed potential carbon revenues of $7.1, $3.3, and $16.4 million, respectively. The difference in costs of the alternative scenarios though the same 25% renewable energy target is due to the different share in capacity additions of technologies. It was observed from the environmental externality costs analysis that the country has a small potential for carbon taxation. This is partly due to the low contribution of fossil fuels to the generation mix and low electricity demand (generation) in Cameroon. These are some of the reasons why the implementation of carbon taxation in sub-Saharan Africa has stalled. Although studies are ongoing in Senegal and Cote d'Ivoire, only South Africa is presently implementing carbon taxation in Africa (World Bank, 2020). Higher carbon revenue could be gotten with increased carbon taxes, such as the carbon tax of R120/ton on average (~6.6 USD) in South Africa or the $20/ton used as an assumption in a study in Ghana (Awopone et al., 2017b). However, it will increase fuel costs, increase electricity costs, and eventually increase the costs of living in the short term, although it will lead to a faster uptake of renewables in the long term (Konrad-Adenauer-Stiftung, 2020; Organization for Economic, Cooperation, and Development, 2021).
Another policy consideration in Cameroon that could encourage the deployment of renewables is the green credit policy, a financial instrument where banks are instructed to grant loans only to companies with strict environmental compliance. However, it is reported by a study in China that this scheme had negative consequences on the ability of companies to innovate (Zhang et al., 2022). Three concerns were raised by the study, namely: (i) It was challenging for highly polluting companies under this policy to acquire more credit and favorable loan interest rates within a short term, resulting in insufficient funds to research on innovative environmentally friendly products, (ii) Implementing this policy will cause restrictions of highly polluting companies to get green credits from commercial banks, which will push companies to increase commercial credits instead of debt financing, and (iii) The fact that green projects usually have high risk, long cycles, and huge assessment cost is a barrier.
4.4. Environmental analysis
The LEAP tool categorizes energy emissions into two constituents; emissions from the demand side, which are emissions coming from the point of energy usage such as refrigerators, waste disposal, land use, and cars, and these type of emissions were ignored in this study. The second category is those from the point of energy transformation, such as power generation, and this category is the lone type considered in the study.
Greenhouse gas emissions under the BAU, stated, modified, and optimized scenarios increased from 0.82 MTCO2e in 2015 to 1.2, 0.9, 0.8, and 0.4 MTCO2e, respectively, in 2035. Over the 20-year study period, cumulative emissions of 28.1 MTCO2e were observed. The emissions from various scenarios were persistently rising due to an expected increase in power generation capacity. However, cumulative emission savings of 4.2, 1.7, and 19.2 MTCO2e compared to the BAU scenario were observed under the stated, modified, and optimized scenarios, respectively. The cumulative GHG emissions of alternative scenarios in Cameroon compared to the BAU scenario are shown in Figure 13. According to her NDCs, Cameroon is committed to reducing GHG emissions by 32 MTCO2e between 2010 and 2035 (Cameroon Ministry of External Relations, 2015). This reduction is supposed to come from all sectors, including agriculture, buildings, and transport sectors. For the electricity generation sector, it mandated GHG emission increase by only ~84% in the Mitigation scenario, compared to over 175% in the Reference scenario, when both are compared to 2010 values. It is observed from the study that emissions compared to base-year values increased by 146% instead of decreasing. This indicates Cameroon's current generation expansion trajectory digressed from that intended, so meeting her NDC commitments is not possible. However, the expected emission reductions are experienced in the stated, modified, and optimized scenarios where emissions are reduced by 110, 98, and 49%, respectively, compared to 2015 values. Thus, it is important that the country increase its uptake of renewables if it intends to effectively decarbonize the generation section and meet its net zero-carbon commitments. Figure 13 shows the cumulative GHG emissions of the different scenarios with respect to the BAU scenario.
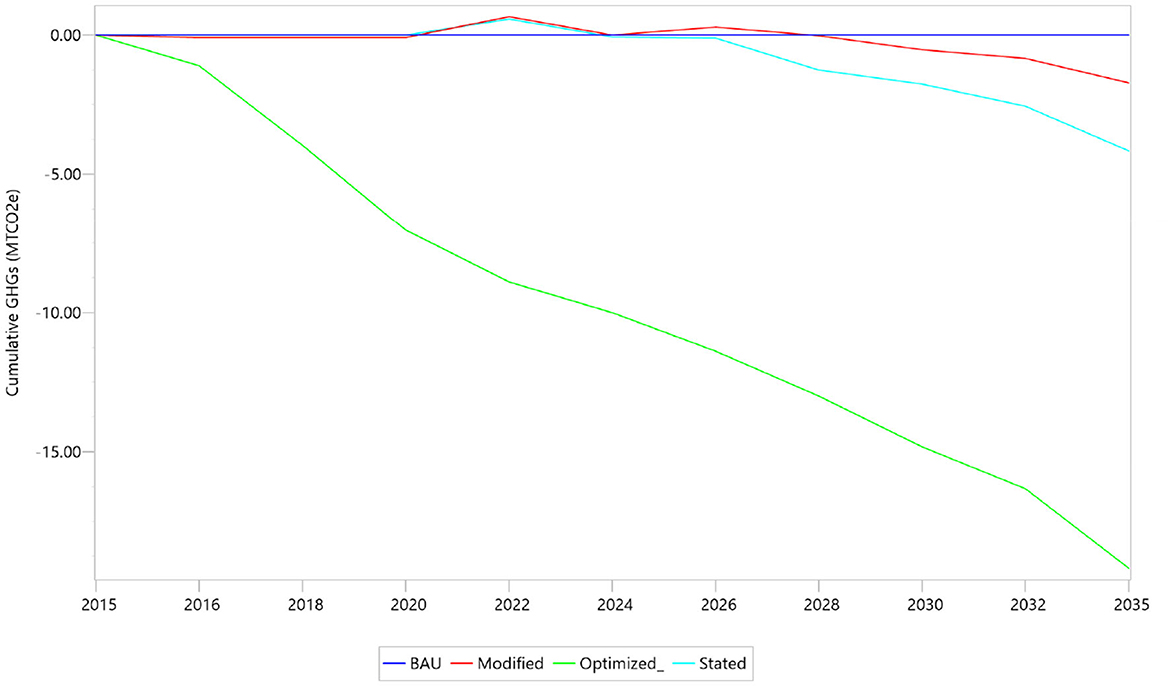
Figure 13. Cumulative GHG emissions savings of the different scenarios with respect to the BAU scenario.
4.5. Sensitivity results
The results from the feedstock fuel sensitivity analysis (±50% variation) are presented in Table 6.
As expected in Table 6, only the feedstock fuel cost is affected. Furthermore, it is observed that changes in fuel cost have a significant impact on the optimized scenario (up to 80%), contrary to other scenarios. However, lower fuel costs lead to a reduction in capital costs and O&M costs while resulting in additional externality and fuel costs. This is due to the LEAP/OSeMOSYS least-costs framework favoring the addition of fossil-fuel generation plants over renewables. Higher fossil-fuel power plant development results in higher emissions and, consequently, high externality costs. Under higher fuel costs, this trend is reversed due to the high costs of owning and operating fossil-fuel power plants. This emphasizes the impact of fossil-fuel prices and carbon taxes on the uptake of renewable energy technologies.
The sensitivity of the optimized scenario to changes in fuel costs, contrary to the other scenarios, also highlights the unsuitability of cost optimization models. These models, like the LEAP/OSeMOSYS framework, rely on a series of parametric and structural assumptions (such as fuel costs and discount rates) in assessing scenarios. However, these assumptions are often narrow and subject to uncertainty, which is not accounted for in the scenario development process (DeCarolis et al., 2012; Poncelet et al., 2016). Thus, cost optimization models are not recommended for assessing the clean energy transition, given that they fail to appropriately capture the systematic changes in real-world transitions (Trutnevyte, 2016).
4.6. Summary of results and perspectives
From the study, Cameroon's current generation trajectory represented by the BAU scenario is incapable of meeting her NDC objectives. However, meeting the objective per the stated scenario, though with the least costs, does not result in the highest emission savings or sustainable development. The modified scenario, though with the highest costs, represents an ideal 25% renewable energy mix for Cameroon. In terms of capacity, cost, and emission reduction, the scenarios ranked in descending order are the optimized scenario, the stated scenario, and the modified scenario. The optimized scenario considers only biomass to fulfill the 25% renewable target of the NDCs and ignores other sources like solar, hydro, and wind. The energy potentials in Cameroon are such that biomass resources are not evenly distributed across the country (huge biomass and hydro resources are concentrated in the southern part, while high wind and solar resources are in the Northern part); hence, there is a need for diversity in energy supply. Biomass technology for power generation is still very unpopular in Cameroon, with only a few pilot projects from agro-industrial companies [such as SOSUCAM, SODECOTON, SOCAPALM, MAISCAM, and the Cameroon Development Cooperation (CDC)] and allocating a huge percentage biomass penetration amid other competitive and more accessible technologies is irrational. In addition, there are no government policies that intensively consider the development of biomass power generation in Cameroon, which is the reason why this sector has been inactive since the country's independence. This makes the optimum scenario and stated scenario unsuitable for Cameroon. Therefore, the scenario that is suitable for Cameroon is the modified scenario since it rationally allocates the various RE technologies based on the accessibility of technology, availability of RE resources, and the country's economic readiness. In Cameroon, policy support for solar PV is increasing with a wave of economic incentives such as a tax break of 10 years for solar PV developers (Ngalame, 2022) and the exemption from value-added tax (VAT) on imported solar accessories (Cameroon National Assembly, 2011; Electricity Sector Regulatory Agency, 2015). In addition, solar PV is able to compete equally with off-grid systems having battery storage to substitute diesel generators used in some communities or offer backup electricity supply in cases of an unreliable grid.
Due to the high cost of grid extension to some remote areas, the government, in the rural electrification master plan (AER, 2017), plans to develop local mini-grids using RE technologies. Since this initiative started, the government has continued to electrify off-grid communities with solar PV. No off-grid community in Cameroon is powered by a biofuel-based electricity system, and there are no signals that this is happening in the short term as solar and hydro are currently given more priority. This shows the government's reluctance to the advancement of biomass-based generation despite the enormous resource potentials.
As shown in Table 2, solar PV has a lower capital cost (2,052 $/kW in 2015) than biomass generation (3,000 $/kW in 2015). However, biomass has a higher capacity factor (60%) than solar PV (25%), as shown in Table 4. However, the O&M costs of solar PV technology are lower than that of biomass generation. If the system LCOE is considered the basis for assessment, biomass with a lower LCOE will be the selected option because (i) the higher capacity factor of 60% compared to 25% for solar PV and (ii) the low costs of biomass feedstock in Cameroon which is almost free. It is worth noting that biomass feedstock is not free in developed countries. Nonetheless, the proposed “modified scenario” considered more solar PV than biomass. This is due to the maturity of the technology and the public perception in Cameroon. This is evidenced by the uptake of 14.19 MW solar PV, 0.3 MW small hydropower, and 0 MW biomass since 2015, despite a higher target in the other scenarios. This study equally supports the call for Cameroon to update her NDCs, like other countries have done during the COP26 in Glasgow, with more quantifiable statistics on the requirements for achieving this noble government ambition on combating climate change. Thus, the three fundamental questions facing the RE and the electricity sector of Cameroon, as stated in the introduction, have been examined by this study, and the insights are summarized as follows:
• The installed capacity by 2035 reaches 4.89 GW under the BAU scenario and only attains a 12% RE target. This implies the current generation expansion trajectory of Cameroon is inadequate to meet her 25% planned RE target. Moreover, the current deployment trend is unlikely to achieve the planned RE technology share. For example, the current trend is seeing a greater focus on solar PV than on biomass and small hydropower stated in the NDCs.
• Apart from the optimized scenario, the current generation expansion pathway (BAU scenario) is the most cost-efficient, given that an additional cost of at least $94 million is required in the alternative scenarios. However, optimal pathways are usually not ideal for decision-making (Trutnevyte, 2016).
• The modified scenario, which accounts for recent RE development trends (i.e., more solar PV and wind energy), attains the 25% RE target by 2035, at only $122.3 million more in additional costs and at 6% more in emission savings compared to the BAU scenario.
From the findings, the current trajectory (BAU scenario) would be appropriate if decision-making was based exclusively on economic factors. However, economics was neither the basis for the Paris Climate Agreement nor the basis for the country's ratification of the Paris Accord and the development of her NDCs. Thus, the current policy framework for RE development is inadequate. An appropriate policy review while considering the recommendations in the subsequent section is thus necessary to redirect the nation's development trajectory.
However, the study is subject to some limitations. Cameroon is characterized by increasing and frequent power outages due, in part, to the dilapidated nature of the power grid (ENEO, 2019). Like most countries in SSA, the country has resorted to backup generators (gensets) as a stopgap to deal with grid deficiencies. The total installed capacity of existing gensets in Cameroon is estimated at ~100 MW (IFC, 2019). Due to a number of factors, such as non-inclusion in official policy documents, suppressed demand, and paucity of data on the energy generation profiles caused by their spontaneous operation, the study does not integrate the energy generated from these gensets. Thus, the study results may not accurately reflect the nation's real energy generation, total emissions, or future generation expansion trajectory.
Furthermore, perfect foresight was assumed in developing the scenarios in this study. However, the parametric and structural assumptions used in the scenarios, such as technology characteristics and fuel costs, are uncertain and subject to variability in time. Energy models, especially the cost-optimization models (optimized scenario), have been proven to have systematic biases, do not account for uncertainty, and consider narrow assumptions as presented in DeCarolis et al. (2012) and Trutnevyte (2016). Thus, the exact figures of this study ought to be interpreted with caution. Moreover, the findings from this study are meant to trigger stakeholder discussions and redirect policy in Cameroon.
5. Recommendation for sustainable power sector expansion in Cameroon
Prices of solar PV, wind energy systems, and battery storage systems continue to decrease rapidly. Data from IRENA indicate a drastic drop in the weighted average levelized cost of power of utility-scale globally on solar PV, onshore wind, and battery storage by 77, 35, and 85%, respectively, between 2010 and 2018 (IRENA, 2018; Bloomberg, 2019). These trends in cost bring fresh potentials for the extensive deployment of renewables and an all-inclusive power sector decarbonization agenda that were not anticipated in previous policy formulation. Cameroon, therefore, needs to take steps through partnering with countries with a reputable history of manufacturing renewable energy components, sign win–win agreements on technology transfer, and have these equipment delivered at a further low cost. This will drastically reduce the cost of acquisition of these equipment by the utility company and private individuals, hence accelerating their deployment in the country. Below are some policy proposals for a swift transition to renewables in Cameroon.
5.1. The need for an all-inclusive sector planning
An appraisal of the institutional setting of the Cameroonian electricity sector in the domain of both policy and operations shows several gaps and possible misalignment between authority and accountability. This is more evident in the area of planning power demand, PPA negotiations, management of rural electrification, and tariff setting (Electricity Sector Regulatory Agency, 2015). In advanced power grids, utility operators usually have the duty of demand forecasting and the development of medium-term supply strategies in collaboration with the state regulator and other stakeholders. In Cameroon, the government, through policy, has imposed tough supply and access targets to the power utility (ENEO) (Castalia Advisory Group, 2015) while ironically reducing the scope of the flexibility of tariff regulation by utilities. This exposes the utility to a situation of unmet demand, leading to the risk of disrupting the utility's future expansion plans.
We recommend that government should protect ENEO from any risk that forestalls the company's future expansion plans by offering financial reparation guarantees or granting flexibility premiums sufficient enough to cover the impact of probable over-commitment on the electricity supply. The utility should, from time to time, conduct medium-term (5–10 years) demand forecasting studies as part of its duty for integrated RE system planning. Moreover, the overlapping authority among different ministries related to the power sector (Electricity Sector Regulatory Agency (ARSEL), 2020) has always been a source of potential conflict which has hampered, in one way or the other, the utility's ability to fully engage in both on-grid and off-grid electricity access development programs. The government should make steps toward establishing an autonomous Rural Electrification Agency or similar structure that would manage the numerous initiatives geared toward expanding power supply and particularly optimizing the allocation of funding. Simultaneously, the government should establish an organized approach toward the development of industrial zones in the country with the intention of matching industrial development with electricity requirements, laying emphasis on the impact on power demand. This approach could include some extra support from an Industrial Zone Electricity Agency with incentives for demand-side management from ENEO, the county's power utility. The value proposition embodied in robust, rational, and resilient plans for clean electricity generation must be widely advertised to potential international and national investors. This would further push the argument that the electricity supply eases the creation of industries, hence economic growth.
5.2. Decentralizing the energy governance structure
The power sector in Cameroon operates a highly centralized governance structure, at the top of which is the Ministry of Energy (Njoh et al., 2019), led by a minister. Even though the ministry has regional and divisional offices all over the country, all major decisions on the power sector are taken in Yaounde, the country's capital. Only the most ordinary decisions are made at the regional and district levels, ignoring the contribution of the local population to meeting their energy needs (Iweh et al., 2022b). The government should create technical working groups at regional and district levels on climate change and RE through the development of a nationwide action plan that clearly identifies the duty of stakeholders. The group will support and build synergy among inter-sectorial technical commissions, and the regional secretariat will have an entity authorized to coordinate energy initiatives. This will enhance collaboration between the district and the central government's energy centers. At divisional levels, technical committees on energy initiatives could be established for inter-sectorial coordination, and divisional administrators should apply strategies that encourage community input. The communication channels and support systems of women and youth groups should be empowered so that problems that need high-level intervention can be resolved and the progress of the program can be evaluated.
5.3. Adopt a continuous policy review and revision process
Cameroon has outlined a number of ambitions (Vision 2035) (MINEPAT, 2009b) that seek to improve economic growth, accelerate wealth creation, and improve the living standards of its citizens. Since economic development is associated with an increase in electricity supply, the obvious tendency is equally pursuing ambitious plans in the power sector. Nonetheless, there are concerns about how soon Cameroon's vision for 2035 can be realized (Ekeke and Nfornah, 2016), especially when one considers some volatile and uncertain external factors. Therefore, cautious planning in the country's energy policy that strongly supports renewable energy development through robust plans matched by measurable outcomes (reliable supply, affordable power, and improved electricity access) should be implemented. This reduces the risk of unjustifiable energy expenses that do not reflect actual outcomes.
Cameroon urgently needs to prioritize the development of programs that continuously monitor and adjust the timing of long-term expansion projects to avoid a mismatch between power production capacity and grid assets on the one hand and the actual evolving demand and load profile on the other hand. The weakness in Cameroon is that long-term projects rarely have a monitoring mechanism that evaluates progress with the intention of managing risk and delivering in a timely manner. To escape this conundrum, Cameroon should actively develop flexible and responsive power supply plans that establish demand-driven actions for capacity building with dedicated lead times of 1–3 years, with a procedure ready to monitor key indicators so as to determine which indicator needs more attention. This strategy will require the utility (ENEO) and government to liaise with energy developers to build win–win commercial models having Power Purchase Agreements (PPAs) with enhanced flexibility and clear implementation processes. These models should be realizable both in terms of timing and estimated budget. The already set out renewable energy goals, if properly monitored, will upscale the deployment of RE in the power generation mix.
5.4. The establishment of Feed-in-Tariffs (FiTs)
Countries with a successful track record of RE development have adapted various economic instruments to enhance growth. Cameroon has limited incentives geared toward improving the RE penetration in the country (Njoh et al., 2019). The Cameroon electricity market requires a FiTs scheme which will help attract private sector involvement in the sector. The FiTs scheme makes RE projects economically feasible through the compensation of differences in the cost of energy between conventional and RE systems. This is either done through government subsidies or tax levies on consumers, and the government later shifts the financial burden directly or indirectly to users or the public. Notwithstanding the financial implication on either government or consumers, RE development would improve social welfare and lessen environmental threats to citizens.
The initial phase could mean the government soliciting funds through the global energy transfer Feed-in-Tariffs (GET-FiTs) program, a policy scheme where developed nations fund RE projects in developing countries for the application of FiTs. The government could request funding from international institutions or foreign countries (Germany, Japan, Belgium, Canada, China, etc.) that are actively doing business in Cameroon. This will help Cameroon to secure a FiTs premium with a reduction in the burden, and independent power producers (IPPs) with RE generators will sell electricity at a cost pre-contracted with the utility company. Those who use electricity from renewable sources are compensated with premiums from government and foreign funders.
5.5. Local content development for renewable power generation equipment
The cost of RE deployment has further added in developing countries like Cameroon due to the need to import generation equipment, which acts as a barrier to the rapid growth of renewable energy projects. However, there are already some community micro power projects in Cameroon, such as that of Baleng (Mungwe et al., 2016), where most of the power equipment (hydro turbine, penstock, etc.) were locally manufactured by the villagers. There are potentials for developing local manufacturing competencies in the country, and scaling up the percentage of local content for renewables can assist in reducing costs and contributing to its rapid deployment. It is anticipated that the existing local content in the electricity value chain will grow as knowledge and expertise improve, but this, however, greatly depends on government's commitment to supporting this initiative. Some prospects for local content development exist in inverters and components like LED lamps, as a few universities within the country are already making prototypes that could be scaled. In addition, there exist substantial options for more Cameroonian content in operations and maintenance, installation, and related services. These aspects are crucial to the speedy development of RE in Cameroon.
Renewable energies are also a factor of social transformation in training and employment while granting a certain economic autonomy and energy security to local communities. Better supervision of this sector will make a substantial contribution over the next few years to the creation of a new economic model by allowing the development of scientific, technological, and industrial know-how, the impact of which will already be perceptible in the medium and long term in Cameroon. The government should focus on technology transfer, creating the best conditions for long-term uptake of the new technologies by tailoring them to local needs and engaging local actors and business partners to construct technical and market development capacity.
5.6. Emphasis on the development of off-grid systems
Cameroon aims to rapidly expand access to electricity through the deployment of renewables, 25% of renewables by 2035 compared to the current <1% (MINEE, 2015) RE penetration. The challenge of realizing these targets is daunting without suitable measures. Any genuine method that seeks to meet this access target will need to emphasize off-grid electrification solutions: Certainly, they require an intensive mobilization for off-grid power supply. The present off-grid development plans need further clarification in terms of choice of technology, funding models, tariff system, and relationship management among private developers. However, an increase in off-grid expansion poses some challenges with regard to off-grid generation and pursuing simultaneous plans for grid expansion. One pertinent issue is the possible future of off-grid users when the main grid arrives, especially when off-grid assets have not been completely paid back at the time of arrival of the main grid. The difficulty at this point is to select suitable compensation mechanisms that help repay the assets.
In addition, considerations for grid power supply will vary significantly across the country since the various regions of Cameroon have dissimilar resource potentials, different load profiles, and variable needs for grid improvement and backup electricity supply with different mixes of on-grid and off-grid electricity access. The relationship between grid developers and off-grid developers will require careful management both at regional and local levels. A common national strategy toward investing in the sector, requesting a connection, managing loads, and tariff collection is perhaps not feasible. This poses a potential challenge to the idea of applying a universal electricity tariff and combined subsidies and financial models. Other issues include power losses mostly in the regional and local distribution network, and the method of tariff collection in villages which will require a robust local management scheme.
5.7. Access to carbon credits and mobilization of funds
The Clean Development Mechanism (CDM) is a funding source for Cameroon. It was set up by United Nations through the Kyoto Protocol to finance environmentally friendly projects. Certainly, Cameroon can take advantage of the CDM in financing renewable energy projects. This economic instrument permits developed countries to meet up with part of their emission reduction commitments by financing projects in developing countries that reduce GHG emissions since this is believed to be less expensive in developing countries. The fundamental requirement of the CDM is that the invested projects should be in line with the host country's development priorities. The CDM has a wide range of technologies it considers, among which is renewable energy technologies. Cameroon ratified the UNFCCC in October 1994, Kyoto Protocol in July 2002, and most recently the Paris Climate Accord in 2015. In 2006, Cameroon established a national commission for the CDM, while the Ministry of Environment and Nature Protection instituted a national agency to execute CDM projects (Ngnikam, 2009). To date, only the Hygiene and Sanitation Company of Cameroon (HYSACAM), which is in charge of the collection and treatment of municipal solid waste, has benefited from this funding source. Another related funding source Cameroon can benefit from is the Carbon Finance for Sustainable Energy in Africa (CF-SEA) which is funded by a joint World Bank—United Nations support and supervised by the United Nations Environment Program (UNEP). The CF-SEA program has supported several CDM clean energy projects in Cameroon. Cameroon has benefited from ~60 MW of CDM projects with an emission reduction potential of ~2 million tons of CO2 (REEGLE, 2015). Another potential source of funding for Cameroon is the loss and damage fund adopted during COP27, where developing nations with high vulnerability to the climate crisis will be supported (United Nations Environment Programme, 2022). These funds can be used in the deployment of clean energy technologies for power generation.
6. Conclusion
Apart from reducing emissions, renewable energy equally has potentially positive impacts on sustainable development, and it is an argument widely supported in the economic literature. If the rural–urban dichotomy in electricity access rate in Cameroon and other countries in SSA could be reduced, especially through RE technologies, it would go a long way to improve rural productivity, alleviate poverty, reduce unemployment, and rural–urban migration.
The study is a synthesis of quantitative analysis that explores the pathway to renewable energy transition in Cameroon's power sector through a policy perspective. The appraisal of power sector initiatives shows several conflicting energy generation ambitions by different agencies in the country, and this is a clear indication that there is sadly limited collaboration among related ministries that can cooperatively tackle the energy transition. The study posits that government policies should endeavor to reduce operational barriers to renewable energy deployment, particularly by empowering local skill acquisition through learning-by-doing, as this will increase adoption rates. Policy mechanisms that enhance the implementation of FiT, the unbundling of the over-centralized energy governance structure, and stakeholder cooperation are a favorable environment for renewables. The Cameroon government has recognized that regional cooperation is crucial in galvanizing efficiencies and economies of scale that result in the deployment of renewables in a coordinated way and is currently working on the Chad-Cameroon power project. This approach is effective due to Cameroon's huge renewable energy potential and her willingness to be involved in the large-scale deployment of shared renewable resources for electricity generation. However, a lot still needs to be done in the area of embracing an integrated method to transboundary issues, such as trade, regional setup, regulatory frameworks, and policies. The establishment of structures which clarifies these cross-border issues would allow the countries within the sub-region to benefit from coordinated access to regional renewable resources at a reasonable cost. Projections from IRENA (2015) have indicated that RE project deals in Africa will provide power at some of the cheapest costs globally. Therefore, the abundance of solar, biomass, and hydropower in Cameroon renders these technologies economically competitive and promising for meeting emission reduction commitments in the electricity sector, especially with the rapidly decreasing costs of renewable technologies. The institutional setup in the sector in Cameroon needs to improve its ability to plan projects properly so that future investments are executed in a harmonized and economically viable manner. The reviewed power ambitions in some policy document like the REMP, the energy sector development plan, and the NDCs shows ambitiously contradictory targets within overlapping time periods. The government needs to harmonize the project coordination unit that will work on developing an updated and Harmonized Energy Master Plan (HEMP) since the existing ones are obsolete and no longer representative. The HEMP should conduct an assessment of the planned generation expansion projects with an emphasis on those that were unsuccessful within set time scales and provide solutions to fast-track their implementation. Future research direction should consider energy models that account, to the greatest extent, for uncertainty parameters. Uncertainties in fuel costs, energy demand, fuel availability, and so on. The future study could also consider grid integration studies and power system analysis to ensure the safe and reliable integration of renewables in the grid.
Data availability statement
The original contributions presented in the study are included in the article/supplementary material, further inquiries can be directed to the corresponding author.
Author contributions
The energy backcasting model which was the basis of the analysis was executed by CI and YA. It was later refined by YA, SG, ET, EE-D, and FD before the application of the LEAP tool. Reviewing literature, data gathering, analysis, and compiling the draft manuscript were piloted by CI and YA under the supervision of SG, ET, EE-D, and FD. All authors contributed to the article and approved the submitted version.
Acknowledgments
The authors acknowledge the support from the World Bank through the African Centers of Excellence (ACE)—Impact Project with the Regional Center for Energy and Environmental Sustainability (RCEES)—Ghana, the West African Research Association (WARA), and other contributors for their input.
Conflict of interest
The authors declare that the research was conducted in the absence of any commercial or financial relationships that could be construed as a potential conflict of interest.
Publisher's note
All claims expressed in this article are solely those of the authors and do not necessarily represent those of their affiliated organizations, or those of the publisher, the editors and the reviewers. Any product that may be evaluated in this article, or claim that may be made by its manufacturer, is not guaranteed or endorsed by the publisher.
Abbreviations
BAU, business as usual; CDM, clean development mechanism; CF-SEA, carbon finance for sustainable energy in Africa; COP, conference of parties; ENEO, energy of Cameroon; ESDP, energy sector development plan; FiTs, Fit-in-Tariffs; GDP, gross domestic product; GESP, growth and employment strategy paper; GET-FiTs, global energy transfer feed-in tariffs; GHG, greenhouse gas; GW, gigawatt; GWh, gigawatt hour; HEMP, harmonized energy master plan; HFO, heavy fuel oil; IEA, international energy agency; IPCC, intergovernmental panel on climate change; IPPs, independent power producers; IRENA, international renewable energy agency; LCOE, levelized costs of energy; LEAP, low emissions analysis platform; LFO, light fuel oil; LPG, liquefied petroleum gas; MTCO2e, metric tons of carbon dioxide equivalence; MW, megawatts; NDCs, nationally determined contributions; NREL, national renewable energy laboratory; O&M, operation and maintenance; PPA, power purchase agreements; PV, photovoltaic; RE, renewable energy; REMP, rural electrification master plan; SSA, Sub-Sahara Africa; T&D, transmission and distribution; UNDP, United Nations Development Program; UNEP, United Nations Environment Program; UNFCCC, United Nations Framework Convention on Climate Change; US, United States; XAF, Central African Franc.
References
AER (2017). Plan Directeur d'Electrification Rurale du Cameroun (Rural Electrification Master Plan), Yaounde: Rural Electrification Agency (AER).
AfDB (2017). Cameroon and Chad Power Interconnection Project. Abidjan: African Development Bank Group (AfDB).
Alby, P., Dethier, J. J., and Straub, S. (2013). Firms operating under electricity constraints in developing countries. World Bank Econ. Rev. 27, 109–132. doi: 10.1093/wber/lhs018
Aliyu, A. S., Ramli, A. T., and Saleh, M. A. (2013). Nigeria electricity crisis: power generation capacity expansion and environmental ramifications. Energy 61, 354–367. doi: 10.1016/j.energy.2013.09.011
Andersen, T. B., and Dalgaard, C. J. (2013). Power outages and economic growth in Africa. Energy 38, 19–23. doi: 10.1016/j.eneco.2013.02.016
ANDRITZ Hydro (2019). Hydronews Hydropower-Africa-Cameroon. Stattegger Strasse. Available online at: https://www.andritz.com/hydro-en/hydronews/hydropower-africa/cameroon (accessed January 07, 2022).
Awopone, A. K., Zobaa, A. F., and Banuenumah, W. (2017a). Techno-economic and environmental analysis of power generation expansion plan of Ghana. Energy Policy 104, 13–22. doi: 10.1016/j.enpol.2017.01.034
Awopone, A. K., Zobaa, A. F., and Banuenumah, W. (2017b). Assessment of optimal pathways for power generation system in Ghana. Civil Environ. Eng. 4, 1–13. doi: 10.1080/23311916.2017.1314065
Bautista, S. (2012). A sustainable scenario for Venezuelan power generation sector in 2050 and its costs. Energy Policy 44, 331–340. doi: 10.1016/j.enpol.2012.01.060
Bodjongo, M. J. M., Ekome, G. C. E., and Essomme, F. K. O. (2021). Analysis of the gap in enterprise access to renewable energy between rural and urban areas in Cameroon. Environ. Econ. 12, 39–52. doi: 10.21511/ee.12(1).2021.04
Brandes, O. M., and Brooks, D. B. (2005). The Soft Path for Water in a Nutshell. Canada: Friends of the Earth.
Cameroon Ministry of External Relations (2015). Intended Nationally Determined Contributions (INDC). Yaounde: MINREX.
Cameroon National Assembly (2011). Law No. 2011/022 of 14 December 2011 Governing the Electricity Sector in Cameroon. Yaounde: Cameroon National Assembly.
Castalia Advisory Group (2015). Evaluation of Rural Electrification Concessions in sub-Saharan Africa Detailed Case Study: Cameroon. Washington, DC: World Bank.
Cole, M. A., Elliott, R. J. R., Occhiali, G., and Strobl, E. (2018). Power outages and firm performance in Sub-Saharan Africa Saharan Africa. J. Dev. Econ. 134, 150–159. doi: 10.1016/j.jdeveco.2018.05.003
Crippa, M., Oreggioni, G., Guizzardi, D., Muntean, M., Schaaf, E., Lo Vullo, E., et al. (2019). Fossil CO2 and GHG Emissions of All World Countries−2019 Report. Luxembourg: Publications Office of the European Union.
DeCarolis, J., Hunter, K., and Sreepathi, S. (2012). The case for repeatable analysis with energy economy optimization models. Energy Econ. 34, 1845–1853. doi: 10.1016/j.eneco.2012.07.004
Ekeke, E., and Nfornah, S. C. (2016). Policy Brief: The Importantce of a Flexible Energy Policy in Cameroon for a Conducive Business Environment. Available online at: https://choforche.wordpress.com/2016/09/14/policy-brief-the-importance-of-aflexible-energy-policy-in-cameroon-for-a-conducive-business-environment-14-september-2016-by-eposi-ethel-ekeke-and-sirri-caroline-nfornah/ (accessed July 29, 2021).
Electricity Sector Regulatory Agency (2015). Renewable Energy and Rural Electrification in Cameroon: Guides of Promotion of Investments. Yaounde: ARSEL.
Electricity Sector Regulatory Agency (ARSEL) (2020). Electricity Sector, Legal and Institutional Framework. Yaounde: Electricity Sector Regulatory Agency (ARSEL). Available online at: http://arsel-cm.org/electricity-sector/legal-and-institutional-framework/market-structure/?lang=en (accessed February, 2020).
Emodi, N. V., Emodi, C. C., Murthy, G. P., and Emodi, A. S. A. (2017). Energy policy for low carbon development in Nigeria: a LEAP model application. Renew. Sustain. Energy Rev. 68, 247–261. doi: 10.1016/j.rser.2016.09.118
ENEO (2019). 2019 Annual Report: ENEO is Transforming the Electricity Service. Douala: Energy of Cameroon (ENEO).
ENERDATA (2019). Energy Market—Cameroon. Douala: ENERDATA. Available online at: https://www.enerdata.net/estore/energy-market/cameroon/ (accessed August 02, 2021).
Energy Information Administration (EIA) (2021). Annual Energy Outlook 2021. Washington, DC: U.S. Energy Information Administration.
Feintrenie, L. (2012). Transfer of the Asian Model of Palm Development from Indonesia to Cameroon. Washington, DC: World Bank conference on land and poverty, April 23-25.
Gelder, W., and German, L. (2011). Biofuel Finance: Global Trends in Biofuel Finance in Forest-Rich Countries of Asia, Africa and Latin America and Implications for Governance. CIFOR Infobriefs No. 36. Bogor: CIFOR infobriefs.
GET.invest (2020). Country Briefs: Insights and Data to Help You Make Informed Decisions. Bonn. Available online at: https://www.get-invest.eu/market-information/country-briefs/ (accessed October 08, 2021).
Holmberg, J., and Robèrt, K. H. (2000). Backcasting: a framework for strategic planning. Int. J. Sustain. Dev. World Ecol. 7, 291–308. doi: 10.1080/13504500009470049
Huang, Y., Bor, Y. J., and Peng, C. Y. (2011). The long-term forecast of Taiwan's energy supply and demand: LEAP model application. Energy Policy 39, 6790–6803. doi: 10.1016/j.enpol.2010.10.023
IEA (2020a). Data and Statistics. Paris: IEA. Available online at: https://www.iea.org/data-and-statistics (accessed June 8, 2022).
IEA (2020b). Electricity Access Database: World Energy Outlook-2020, Washington, DC: International Energy Agency (IEA).
IEA IRENA, UNSD, World Bank, WHO. (2020). Tracking SDG 7: The Energy Progress Report. Washington DC: International Energy Agency, International Renewable Energy Agency, United Nations Statistics, World Bank, World Health Organization.
IEEE (2018). Assessment of Renewable Energy Resources in Cameroon and Special Regards on Energy Supply. New York, NY: IEEE. Available online at: http://ieeexplore.ieee.org/document/7180807/?Reload=true (accessed July 28, 2021).
IFC (2019). The Dirty Footprint of the Broken Grid: The Impacts of Fossil Fuel Back-Up Generators in Developing Countries. Washington, DC: International Finance Corporation.
Institut National de la Statistique (2016). Note de synthèse de l'Evolution de l'inflation au cours des neufs premiers mois de l'année. Yaounde. Available online at: www.statistics-cameroon.org (accessed September 03, 2022).
International Renewable Energy Agency (2020). Renewable Power Generation Gas 2019. Abu Dhabi: IRENA.
IRENA (2015). Africa 2030: Roadmap for a Renewable Energy Future. Abu Dhabi: International Renewable Energy Agency (IRENA).
IRENA (2018). Power Generation Costs in 2018. Abu Dhabi: International Renewable Energy Agency (IRENA).
IRENA (2020a). IRENA Renewable Energy Costs 2019. Abu Dhabi: International Renewable Energy Agency (IRENA).
IRENA (2020b). Renewable Power Generation Costs 2019. Abu Dhabi: International Renewable Energy Agency.
IRENA and AfDB (2022). Renewable Energy Market Analysis: Africa and Its Regions—A Summary for Policy Makers. Abu Dhabi and Abidjan: International Renewable Energy Agency and African Development Bank.
Iweh, C. D., Gyamfi, S., Tanyi, E., and Effah-Donyina, E. (2021). Distributed generation and renewable energy integration into the grid: prerequisites, push factors, practical options, issues and merits. Energies 14, 1–34. doi: 10.3390/en14175375
Iweh, C. D., Semassou, G. C., and Ahouansou, H. R. (2022a). The optimization of hybrid renewables for rural electrification: techniques and the design problem. Int. J. Eng. Trends Technol. 70, 222–239. doi: 10.14445/22315381/IJETT-V70I9P223
Iweh, C. D., Semassou, G. C., Ahouansou, H. R., and Nsanyuy, W. B. (2022b). The sustainability of renewable energy-based electrification projects in Cameroon: technical, institutional and policy perspectives. Netw. Ind. Quart. 24, 8–17.
Kemausuor, F., Nygaard, I., and Mackenzie, G. A. (2015). Prospects for bioenergy use in Ghana using long range energy alternative planning model energy alternatives planning model. Energy 93, 672–682. doi: 10.1016/j.energy.2015.08.104
Kenfack, J., Nzotcha, U., Voufo, J., Ngohe-Ekam, P. S., Nsangou, J. C., and Bignom, B. (2021). Cameroon's hydropower potential and development under the vision of Central Africa power pool (CAPP): a review. Jean Calvin Nsangou Blaise Bignom 151, 1–16. doi: 10.1016/j.rser.2021.111596
Konrad-Adenauer-Stiftung (2020). Carbon Pricing in Sub-Saharan Africa. Yaounde: Presses universitaires d'Afrique (P.U.A).
Korea Energy Economics Institute (2017). A Study for Establishment of the Master Plan of Renewable Energy in Cameroon: Annex I of the final report Supporting Analysis Report. Ulsan: Korea Energy Economics Institute (KEEI).
Kwaye, M. P., Benfield, J., and Anglani, N. (2015). Assessment of Renewable Energy Resources in Cameroon and Special Regards on Energy Supply. In: 2015 5th International Youth Conference on Energy (IYCE). Pisa: IYCE. doi: 10.1109/IYCE.2015.7180807
Lee, S., Park, J. W., Song, H. J., Maken, S., and Filburn, T. (2008). Implication of CO2 capture technologies options in electricity generation in Korea. Energy Policy 36, 326–334. doi: 10.1016/j.enpol.2007.09.018
Lkhagva, J. (2014). The Long-Term Forecasting of the Mongolian Energy Demand and Supply Using the Long-Range Energy Alternatives Planning System (LEAP). Seoul: Seoul National University.
Marcos, S., Henley, G., Meng, J., and Ochieng, E. (2017). Mini Grid Market Opportunity Assessment: Cameroon Carbon Trust. New York, NY: United Nations Environment Programme (UNEP) and ECOWAS Centre for Renewable Energy and Energy Efficiency.
McPherson, M., and Karney, B. (2014). Long-term scenario alternatives and their implications: LEAP model application of Panama's electricity sector. Energy Policy 68, 146–157. doi: 10.1016/j.enpol.2014.01.028
Mensah, J. T. (2018). Jobs! Electricity Shortages and Unemployment in Africa. Washington, DC: Policy Research Working Paper Series 8415, The World Bank. doi: 10.1596/1813-9450-8415
MINEE (2005). Plan d'Action National Energie pour la Réduction de la Pauvreté. Yaounde: Ministry of Energy and Water Resources (MINEE).
MINEE (2006). Assistance au Ministère de l'Energie et de l'Eau dans l'élaboration du Plan de Développement à long terme du Secteur de l'Électricité Horizon 2030. Yaounde: Ministry of Energy and Water Resources (MINEE).
MINEE (2015). Situation Énergétiques du Cameroun. Yaounde: Ministry of Energy and Water Resources (MINEE).
MINEPAT (2009a). Cameroon Vision 2035. Yaounde: Ministry of Economy, Planning and Regional Development (MINEPAT).
MINEPAT (2009b). Growth and Employment Strategy Paper. Yaounde: Ministry of Economy, Planning and Regional Development (MINEPAT).
MINEPAT (2020). Stratégie Nationale de Développement 2020–2030 (SND30): Pour la Transformation Structurelle et le Développement Inclusif . Yaounde. Available online at: http://cdnss.minsante.cm/sites/default/files/Strat%C3%A9gie%20Nationale%20de%20D%C3%A9veloppement%20SND30_Fench.pdf (accessed July 31, 2021).
Mungwe, J., Mandelli, S., and Colombo, E. (2016). Community pico and micro hydropower for rural electrification: experiences from the mountain regions of Cameroon. AIMS Energy 4, 190–205. doi: 10.3934/energy.2016.1.190
National Renewable Energy Laboratory (NREL) (2020). 2020 Annual Technology Baseline. Golden, CO: NREL.
Ngalame, N. E. (2022). Cameroon Slashes Taxes to Tap its Solar Power Potential. London, UK: Thompson Reuters Foundation. Available online at: https://news.trust.org/item/20220527120734-m3eph/ (accessed July 4, 2022).
Ngnikam, E. (2009). Renewable Energies in West Africa: Cameroon Country Chapter. Munich: GTZ Regional Report.
Ngnikam, E., Hofer, A., and Kraft, D. (2009). Renewable Energy in West Africa: Country Chapter. Bonn: Federal Ministry for Economic Cooperation and Development.
Ngnikam, E., and Tolale, E. (2009). Energy Systems: Vulnerability—Adaptation—Resilience. Regional Focus: sub-Saharan Africa—Cameroon. Paris: HELIO International Cameroon.
Njoh, A. J. (1992). The institutional framework for housing policy administration in Cameroon. Habitat Int. 16, 43–57.
Njoh, A. J., Etta, S., Essia, U., Ngyah-Etchutambe, I., Enomah, L. E., Tabrey, H. T., et al. (2019). Implications of institutional frameworks for renewable energy policy administration: case study of the Esaghem, Cameroon community PV solar electrification project. Energy Policy 128, 17–24. doi: 10.1016/j.enpol.2018.12.042
Organization for Economic Cooperation, and Development. (2021). Carbon Pricing in Times of COVID-19: Carbon Pricing in South Africa. Paris: Organization for Economic Cooperation and Development.
Ouedraogo, N. S. (2017). Modeling sustainable long-term electricity supply-demand in Africa. Appl. Energy 190, 1047–1067. doi: 10.1016/j.apenergy.2016.12.162
Paletto, A., Bernardi, S., Pieratti, E., Teston, F., and Romagnoli, M. (2019). Assessment of environmental impact of biomass power plants to increase the social acceptance of renewable energy technologie. Heliyon 5, e02070. doi: 10.1016/j.heliyon.2019.e02070
Poczter, S. (2017). You can't count on me: the impact of electricity unreliability on productivity. Agric. Resour. Econ. Rev. 46, 579–602. doi: 10.1017/age.2016.38
Poncelet, K., Kaminski, S., and Delarue, E. (2016). “Ability and limitations of optimization models for computing the equilibrium in competitive energy markets,” in TME Working Paper Energy and Environment, Leuven: KU Leuven Energy Institute 1–31.
REEGLE (2015). Cameroon Energy Profile. Vienna. Available online at: http://www.reegle.info/countries/cameroon-energy-profile/cm (accessed October 13, 2021).
Roinioti, A., Koroneos, C., and Wangensteen, I. (2012). Modeling the Greek energy system: scenarios of clean energy use and their implication. Energ Policy 50, 711–722. doi: 10.1016/j.enpol.2012.08.017
Sen, S., and Ganguly, S. (2017). Opportunities, barriers and issues with renewable energy development: a discussion. Renew. Sustain. Energy Rev. 69, 1170–1181. doi: 10.1016/j.rser.2016.09.137
Senshaw, D. A. (2014). Modeling and Analysis of Long-Term Energy Scenarios for Sustainable Strategies of Ethiopia. Flensburg: Zentrale Hochschulbibliothek Flensburg.
Singh, N., and Nyuur, R. R. B. (2019). Renewable energy development as a driver of economic growth: evidence from multivariate panel data analysis. Sustainability 11, 1–18. doi: 10.3390/su11082418
Stockholm Environment Institute (2021). Low Emissions Analysis Platform. Stockholm. Available online at: https://leap.sei.org/help/leap.htm#t=Concepts%2FIntroduction.htm (accessed May 3, 2021).
Stockholm Environment Institute (2022). Setting Up Optimization. Stockholm. Available online at: https://leap.sei.org/help/leap.htm#t=Optimization%2FSetting_Up_Optimization_in_LEAP.htmandrhsearch=optimizationandrhhlterm=optimizationandrhsyns=%20 (accessed June 26, 2022).
Tagne, R. F. T., Dong, X. A. S. G., Kaiser, S., and Ulgiati, S. (2021). Technologies, challenges and perspectives of biogas production within an agricultural context. The case of China and Africa. Environ. Dev. Sustain. 23:14799–14826. doi: 10.1007/s10668-021-01272-9
Taoa, Z., Liu, Z., and Zhao, C. Z. (2011). Research on the prospects of low-carbon economic development in China based on LEAP model. Energy Proced. 5, 695–699. doi: 10.1016/j.egypro.2011.03.123
Trutnevyte, E. (2016). Does cost optimization approximate the real-world energy transition? Energy 106, 182–193. doi: 10.1016/j.energy.2016.03.038
UNDP (2008). UNDP Climate Change Country Profile. New York, NY: United Nation Development Program (UNDP).
UNFCCC (2016a). Cameroon Deposits Instrument of Ratification of Paris Agreement. Bonn: UNFCCC. Available online at: https://newsroom.unfccc.int/news/cameroon-deposits-instrument-of-ratification-of-paris-agreement (accessed October 26, 2022).
UNFCCC (2016b). Nationally Determined Contributions (NDCs). Bonn: UNFCCC. Available online at: https://unfccc.int/ndc-information/nationally-determined-contributions-ndcs (accessed October 26, 2022).
United Nations (2014). World Urbanization Prospects: The 2014 Revision, Highlights. New York, NY: United Nations Department of Economic and Social Affairs, Population Division.
United Nations Environment Programme (2022). COP27 Ends with Announcement of Historic Loss and Damage Fund. Nairobi: UNEP. Available online at: https://www.unep.org/news-and-stories/story/cop27-ends-announcement-historic-loss-and-damage-fund (accessed December 28, 2022).
USAID (2014). Greenhouse Gas Emissions in the West Africa Region. New York, NY: United States Agency for International Development (USAID).
Vintila, T., Ionel, I., Tiegam, R. F. T., Wächter, A. R., Julean, C., and Gabche, A. S. (2019). Residual biomass from food processing industry in cameroon as feedstock for second-generation biofuels. BioResources 14, 3731–3745. doi: 10.15376/biores.14.2.3731-3745
World Bank (2019). Electricity Uptake for Economic Transformation in Sub-Saharan Africa. Washington DC: World Bank.
World Bank (2021). World Bank Data Bank. Washington, DC: World Bank. Available online at: https://databank.worldbank.org/home.aspx (accessed May 03, 2021).
Keywords: climate action, climate change, backcasting, carbon pathways, emission reduction, LEAP tool, long-term scenarios
Citation: Iweh CD, Ayuketah YJA, Gyamfi S, Tanyi E, Effah-Donyina E and Diawuo FA (2023) Driving the clean energy transition in Cameroon: A sustainable pathway to meet the Paris climate accord and the power supply/demand gap. Front. Sustain. Cities 5:1062482. doi: 10.3389/frsc.2023.1062482
Received: 06 October 2022; Accepted: 23 January 2023;
Published: 17 February 2023.
Edited by:
Prashant Kumar, University of Surrey, United KingdomReviewed by:
Nyong Princely Awazi, The University of Bamenda, CameroonFranco Ruzzenenti, University of Groningen, Netherlands
Copyright © 2023 Iweh, Ayuketah, Gyamfi, Tanyi, Effah-Donyina and Diawuo. This is an open-access article distributed under the terms of the Creative Commons Attribution License (CC BY). The use, distribution or reproduction in other forums is permitted, provided the original author(s) and the copyright owner(s) are credited and that the original publication in this journal is cited, in accordance with accepted academic practice. No use, distribution or reproduction is permitted which does not comply with these terms.
*Correspondence: Chu Donatus Iweh, aXdlaGRvbmFAZ21haWwuY29t