- 1Department of Engineering, University of Naples “Parthenope”, Naples, Italy
- 2International PhD Programme, Department of Science and Technology, UNESCO Chair Environment, Resources and Sustainable Development, Parthenope University of Naples, Naples, Italy
- 3Department of Medicine, Surgery and Dentistry, Scuola Medica Salernitana, University of Salerno, Baronissi, Italy
This study evaluates the perspectives of urban mining in the framework of the circular economy (CE) and starts with a brief analysis of the size of global and urban metabolism and the role that plays materials and waste streams such as construction and demolition waste (C&DW) and waste from electronic and electrical equipment (WEEE). These can be considered as temporary stocks or deposits to be mined in the future, thus shedding light on the concept of recycling potential, end-of-life functional recycling, and material concentration. The recycling potential could be very variable as in the case of metals. The average concentration of some metals (e.g., gold) in WEEE shows that it is higher per ton of electronic product compared to the amount in mining ores. This explains the importance of the concept of urban mining in the circular economy (CE) transition, given that the CE concept was born to address the challenges of high resources consumption rates and worsening environmental problems. The urban mining phenomenon becomes timely and extremely important for cities as they are relevant hubs of materials and energy consumption and source of environmental and social impacts in external areas due to mining and extraction activities. This study points to the need for creating and establishing strong synergies between the concept of CE and urban mining and the role of cities as innovators in finding circular solutions by incorporating more socially just urban mining activities to improve urban resource management, land use, and local and global wellbeing.
Introduction
Construction and demolition waste (C&DW) and waste from electrical and electronic equipment (WEEE) are the two most relevant waste streams available in large and increasing quantities in our societies and cities at the global level (Krausmann et al., 2018; Shittu et al., 2021; Wiedenhofer et al., 2021). The European Union identified C&DW and WEEE within the priority product value chains in the Circular Economy Action Plan. The latter pointed out the challenges coming from key value chains, evidencing the need for “urgent, comprehensive, and coordinated actions” capable of addressing their proper management (European Commission, 2020). This shows that there is the political awareness pertaining to the negative environmental and social impacts associated with these waste streams in their life cycle, and, on the other hand, on their potential environmental, energetic and socioeconomic value (Borthakur and Govind, 2017; Shittu et al., 2021).
However, such potential value is still not fully perceived by the stakeholders in the construction industry (Silva et al., 2017; Ghisellini et al., 2021a), resulting in dumping, landfilling or illegal abandonment of these potentially reusable/recyclable materials, which could be an alternative to their natural substitutes (Silva et al., 2017). The recycling rates are still low, as less than one third of C&DW is recovered at the global level (World Economic Forum, 2021). Similarly, about 20% of global Electronic and Electrical Equipment (EEE) is properly managed according to sound environmental criteria (Forti et al., 2020). In 2019, in the European Union (EU), where the WEEE regulation is recognized among the best in the world, about 50% of the electronic waste generated remains uncollected (EUROSTAT, 2022). Moreover, high social justice concerns arise from unprocessed WEEE that often ends up in landfills and dumpsites in developing countries, causing serious harm to the surrounding eco-systems and human populations, with bad and child labor conditions and many toxins entering the local groundwater system (Awasthi and Li, 2017).
C&DW and WEEE streams could be among the main contributors to the urban mining phenomenon (Koutamanis et al., 2018; Gidarakos and Akcil, 2020; Ottoni et al., 2020). In fact, they are stocked in large quantities in our societies and cities and, still, lack adequate and effective planning for their recovery (Di Giacomo, 2021) thus preventing the exploitation of their ores locally e.g., at the city level as alternative ones to those extracted in natural mining (Graedel, 2011).
Against this background, this study focuses on the concept of “urban mining” as an important circular economy strategy and a process (encompassing the operations of collection, separation, sorting, and processing) Graedel (2011) at city level for waste streams, e.g., C&DW, WEEE, and related materials, products, components for their return into a new production cycle (Ottoni et al., 2020). In the circular economy (CE) model, the exploration of the concept of “urban mining” has a different perspective compared to the case of the recycling economy (Van Buren et al., 2016). Therefore, a central message of this study is that the CE framework is important for optimizing the urban mining process (Borghi et al., 2018) and for widening the attention to the other CE practices for the recovery of products or waste beyond recycling, such as the reuse, repair or refurbishment (Arora et al., 2017; Ghisellini et al., 2019; Ottoni et al., 2020). As evidenced by Stahel (1981), the “reuse” and “recycling” have different effects on the flows of materials and goods of an economy. Reuse leads to a slowdown of the flow of materials from production to recycling. The recycling closes the loops between post-use waste and production and does not affect the speed of the “flows” of materials and good.
It is expected that CE and urban mining as a strategy would be beneficial in reducing the demand of raw materials upstream from cities (Ji et al., 2020) and the associated environmental (Ulgiati et al., 2010) and social impacts (UNEP, 2011; Mancini and Sala, 2018), increasing the awareness of planning for their more long-term sustainability (Ingwersen, 2011). The CE research is currently devoting much more attention to the social and justice aspects of CE practices, and this involves taking into account such aspects in the urban mining concept being part of the CE practical framework (Ottoni et al., 2020).
Understanding global and urban metabolism of C&DW and WEEE in cities
The amount of mined and accumulated materials (defined as anthropogenic resources) from the Industrial Revolution onward is more than 80% of the world natural resources. Aldebei and Dombi (2021) highlight that a large share of the accumulated materials is mainly waste now. It is estimated that 35% of the in-use materials in 2010 will be disposed of as waste by 2030, which is about the same amount of waste generated in the last 110 years (Krausmann et al., 2018).
The anthropogenic stock of gold, silver, lead, and zinc is higher than the known natural deposits, while the anthropogenic stock of copper and iron is equal to known natural deposits Johansson et al., 2013; Krook and Baas, 2013; Cossu and Williams, 2015; Nakamura and Halada, 2015; Zhang et al., 2017).
In this perspective, it is no surprise that the most recent studies evaluating the economy-wide dynamics of materials stocks and flows at the global level (for 14 materials in nine world regions), from 1900 to 2035, have shown that there are no clear signs of material stock saturation and a stabilization of resource material flows, except for the Industrial Old World, Middle East, Northern Africa, Latin America, and Caribbean where the stock per capita levels could approach a saturation after 2035 (Wiedenhofer et al., 2021). Figure 1 shows the trends from 1900 to 2015 about material stock per capita. This further confirms the previous analysis by Krausmann et al. (2018) and Haas et al. (2015).
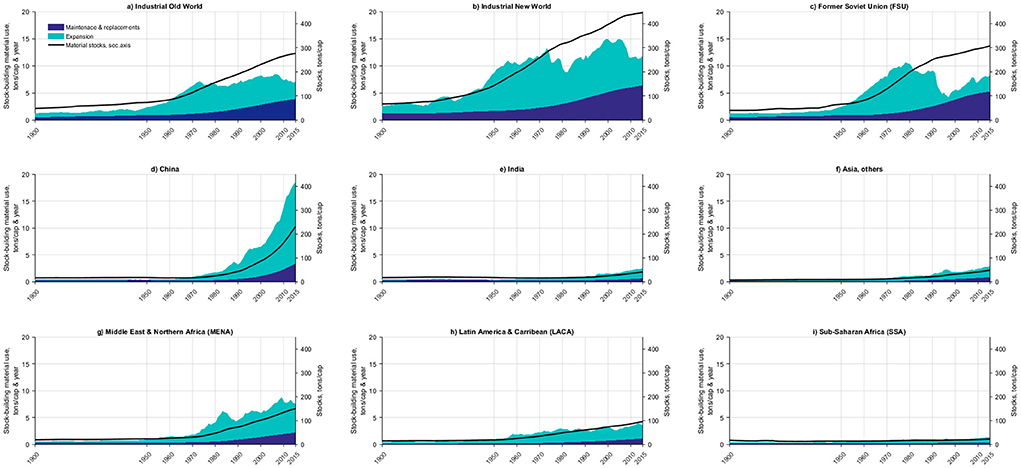
Figure 1. Material stocks in nine world regions and their expansion versus maintenance and replacement flows in the period 1900–2015. Source: (Wiedenhofer et al., 2021). Courtesy of Dr. Dominik Wiedenhofer, Institute of Social Ecology Vienna (SEC), Department of Economics and Social Sciences (WiSo), University of Natural Resources and Life Sciences, Vienna (BOKU).
Industrialized regions have a high material stock per capita and a low growth rate of the material stocks, while, in emerging regions of Asia, the material stock per capita is lower, while the growth rate of materials stock is higher. China's material stocks are growing at 7.6% per year (Wiedenhofer et al., 2021). At the urban level, the material flow analysis of mega cities such as Guangzhou shows high material consumption triggered by urban construction activities that represent half of the consumption of non-metallic materials (Cui et al., 2019).
Cities concentrate in their area, the large part of the country's population and economic activities. For this reason, they are characterized by high consumption flows of materials and energy (Graedel, 1999), which are the source of environmental impacts in terms of consumption of natural resources, emissions, and waste at the local and global scales (Niza et al., 2009). Cities can be considered as organisms, and the study of their metabolism represents a good knowledge base in a policy perspective for monitoring its evolution over time and evaluating the effects of changes aimed at promoting a more sustainable management of the material and energy flows (Graedel, 1999).
United Nations Sustainable Development Goals and within such a framework, the Goal 11 (Sustainable cities and communities) (Serranti, 2021), and sub-goals 11.6 draw the attention to the goals of reducing by the year 2030 the environmental impacts of cities, improving air quality and municipal waste management practices. Furthermore, are suggested the strengthening of national and regional development planning to promote positive economic, social and environmental relationships between urban, per-urban and rural areas (11a) and the adoption and implementation of policies and plans “toward inclusion, resource efficiency, mitigation and adaptation to climate change, resilience to disasters….” (11b) (United Nations, 2022).
There are many studies in the international literature about the urban metabolism of cities or metropolitan areas (Barles, 2009; Niza et al., 2009; Rosado et al., 2014; Hoekman and Von Blottnitz, 2016; Cui et al., 2019) showing the input and output (products, co-products, and emissions) to and from the investigated urban system. Studies do not always provide the total amount of materials used (Domestic Material Consumption, DMC) by a city or metropolitan area in a disaggregated manner in terms of material categories. However, Rosado et al. (2014) analyze the DMC by material categories and subcategories from the year 2003 to the year 2009 for the Metropolitan area of Lisbon. Their data show that, in the year 2009, the total DMC was 21,455,650 tons. The high share in total DMC was that of nonmetallic minerals (45%), followed by biomass (25%), fossil fuels (18%), metallic minerals (6%).
Stone, cement, and sand are the most significant materials consumed in the non-metallic minerals category, while iron, steel-alloying metals, and ferrous metals have a share by 80% in totally consumed metallic minerals. The same order of relevance can be found also for other indicators of material flow analysis (provided in the study only for the year 2005) such as DMI (domestic extraction of materials plus imports of materials), while, in net addition to stocks, the nonmetallic minerals category is the most relevant (82%), followed by metallic minerals, biomass, fossil fuels, chemical, and others. Finally, in domestic material output, emissions to air have a share by 53% in total Domestic Processed Output (DPO), followed by exports (28%), MSW (9%), C&DW (6%), wastewater (3%) (Rosado et al., 2014).
Infrastructures, buildings, machineries, and vehicles can be considered as temporary stocks of resource materials or deposits that are accumulated in cities and, in the future, could be mined once they have achieved their service life. In differentiated urban solid waste, C&DW, WEEE, and end-of-life vehicles (Modoi and Mihai, 2022) are further resources available in relevant quantities in cities with a good recycling potential (Heckens, 2021). The recycling potential is an indicator of the potential environmental and energy benefits obtainable from a recycled material (Thormark, 2006). By recycling, e.g., some building materials, such as steel and aggregates, it is possible to recover about 29% of the embodied energy used for the manufacturing and transport of such building materials (Thormark, 2006; Blengini, 2009).
The recycling potential depends on different factors, such as the material concentration and composition, product composition, dispersed use, and product contamination (Heckens, 2021), and is strictly related to the functional end-of-life recycling (UNEP, 2011). The latter measures the efficiency in recovering a material in its whole life cycle and, e.g., for some metals (available in WEEE or C&DW streams), can be higher than 50% of the whole value or much lower (UNEP, 2011). Half of the value is, in fact, lost during collection, transport, separation, sorting, and recycling of the metal. In this regard, Graedel (2011) and colleagues (UNEP, 2011) studied the end-of-life functional recycling for sixty metals by constructing the “periodic table of recyclability” (Figure 2). From their evaluation emerges that only some metals used in pure forms (copper, lead, gold, silver, platinum, palladium and rhodium) or in alloys (e.g. aluminum, titanium, chromium, manganese, iron, cobalt) are easy to recycle and the functional end-of-life recycling is higher than 50%. Beyond these metals the end-of-life functional recycling is lower as can be seen from Figure 2. The challenge is then to match in product design the features of high performances of materials as well as the need for keeping high their recycling potential (Graedel, 2011).
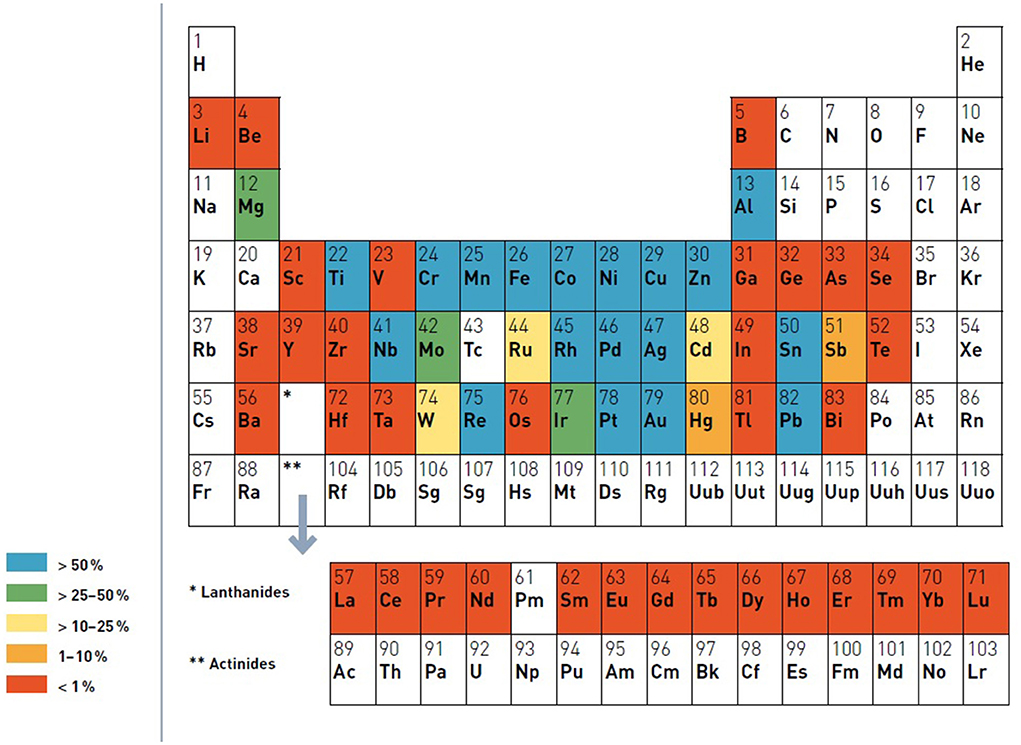
Figure 2. The periodic table of global average end-of-life functional recycling for sixty metals. Source: UNEP (2011).
In the CE model, the Ellen Mac Arthur Foundation since in its early reports suggested the importance of focusing on pure materials1, with the purpose of taking the full advantages of closing the loops. However, this involves the reorganization and optimization of global production system and the participation in the involved changes by all the stakeholders and industries in the supply chains (World Resource Forum, 2022).
After this, it is also interesting to shed light on the average concentration of precious metals in WEEE reported in Table 1 as it is higher in wastestreams compared to the amount in mining ores. For example, in Ontario mines, gold grades of 18/20 grams gold/ton are extracted. About 30 grams/ton is considered of high grade. However, for underground mining, several ounces of gold per ton are considered to be of high-grade, while 5 grams gold/ton is usually economically viable 2.
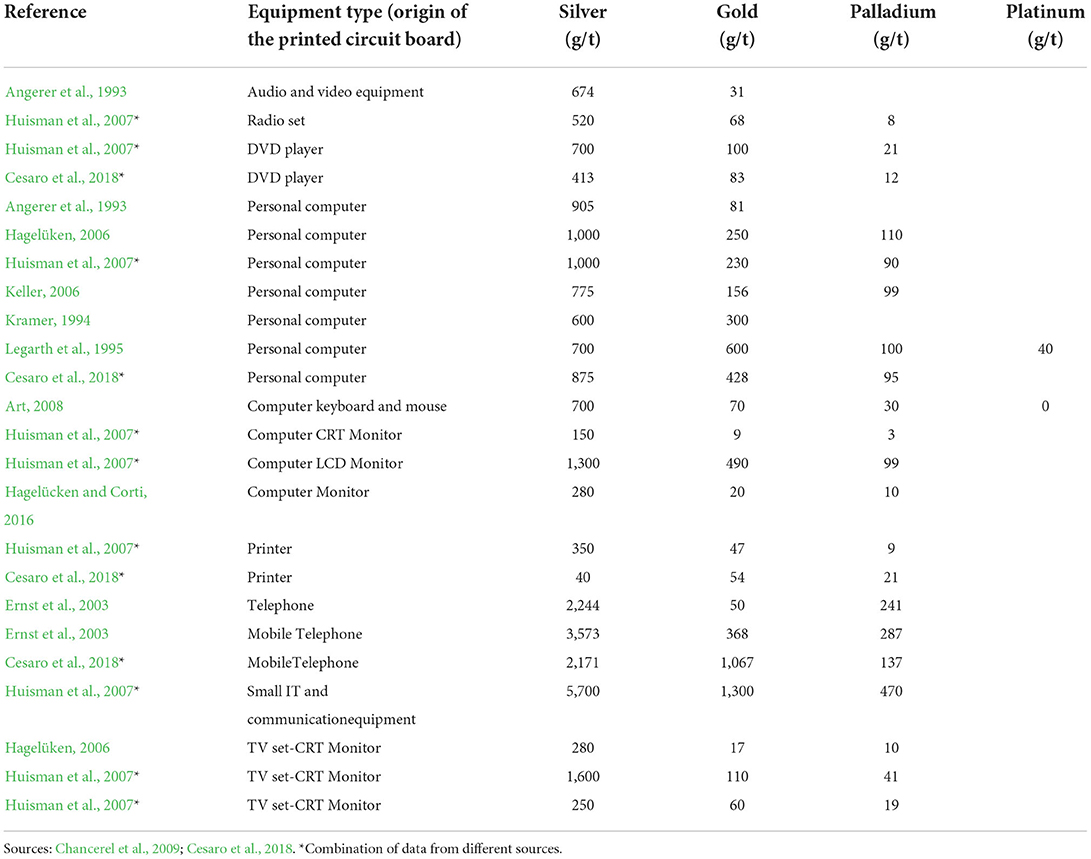
Table 1. Average concentration of precious metals in printed circuit boards from different equipment types.
The concept of urban mining in the CE model
The concept of “urban mining” is the key in the CE model and the parallelism with the traditional mining activities help in understanding why it is so important. Let us remember that the concept of CE emerged in response to the need for reducing the demand of finite natural resources and better use them in the whole life cycle of products, components, and materials (Ghisellini et al., 2016; Geissdoerfer et al., 2017; Kirchherr et al., 2017; Panchal et al., 2021; Oluleye et al., 2022). It is suggested that, under a CE, the global society would live on Earth within the means of its energy and material resources (Deutz, 2020) being conceived as a closed system (Boulding, 1966).
Mining activities are sources of global and local environmental (Nuss and Eckelman, 2014; Fu et al., 2021) and social impacts (Xavier et al., 2021), including primary forest loss (Butsic et al., 2015), conflicts events, and fighting activities to maintain the control of a mining area (Berman et al., 2017), not to mention they contribute to the worsening of climate change (UNEP, 2011; Liu et al., 2020, 2021; Fu et al., 2021). Mancini and Sala (2018) evidence further social impacts such as land use competition of mining activities with those of the local population that encounter land expropriation, displacement, and resettlement, becoming a cause of food insecurity. Environmental and human health negative impacts also arise due to the use of explosives and other chemical substances such as cyanide, which cause toxic and carcinogenic effects. Furthermore, water use competition and contamination can negatively impact local communities and their economic activities, preventing fishery activities that support their livelihoods (Mancini and Sala, 2018).
In the CE perspective, the suggested approach is necessarily “cradle to cradle” in analogy with the natural systems where the concept of waste does not exist as “nothing that contains available energy or useful material is lost” (Frosch, 1992). The CE maximizes and prolongs the value of natural and man-made resources over time (Ellen MacArthur Foundation, 2012), preserving their integrity by the most appropriate product design strategies (Stahel, 1981; Bocken et al., 2016). This stresses the importance of the adoption of the concept of “urban mining” and integrates the latter within the practical framework of the CE since the first stages of the life cycle of a product (Serranti, 2021). The recovery of materials is the main driver of the concept of “urban mining” (Chen and Shi, 2020; Serranti, 2021; Xavier et al., 2021), distinguishing it from “landfill mining” where the goal is not the recovery of materials but the solution of the problem at the disposal level “end of pipe treatment” (Aldebei and Dombi, 2021). “Urban mining” is also linked to the economic feasibility of the whole process as in the case of traditional mining (Aldebei and Dombi, 2021). The recovery of C&DW materials such as, in particular, steel is profitable. The same is for e-waste materials even if they depend on market prices (Favot and Massarutto, 2019). Zheng et al. (2021) have calculated that the mean urban mining costs of copper are about 3,000 US$ per ton lower than the virgin mining costs (5,500 US$) and price (7,500 US$). The mean urban mining cost for aluminum is about 1,660 US$, while the virgin mining cost is 2,500 US$, and the price is 2,200 US$.
The implementation of urban mining as a strategy in CE transition should be a goal shared by all the stakeholders, provided the existence of environmental and socioeconomic benefits of urban mining, and these are measured in a scientific manner such as by means of MFA and LCA (as we will show in the next Section Environmental and social benefits of urban mining). In this view, increasing the awareness of all the involved stakeholders that “urban mining is about sustaining the resources on which all of technology depends, whether or not it is economical at the present time. Metals are gifts from the stars that were generated over billions of years; we should treat them with awe and respect they deserve and devise ways to recycle them over and over. Only then will sustain ability become a reality” (Graedel, 2011).
Environmental and social benefits of urban mining
The evaluation of the environmental benefits of urban mining for WEEE is rather complex due to the existence of a wide range of products, components, and materials (Biganzoli et al., 2015). A few studies have focused on a macro scale (e.g., nation, region, province scales) perspective (Withanage and Habib, 2021). Biganzoli et al., 2015 assessed the whole life cycle of WEEE from the collection in the Lombardy region (Northern Italy) until their final treatment in secondary plants and consequent material and energy recovery of the treated WEEE. The authors combined the Material Flow Accounting method with the Life Cycle Assessment. In the latter, they also included the accounting of the avoided materials and energy benefits coming from the treatment of 1 ton of collected WEEE. Their results show that the amount of material savings for steel and commercial glass has been the highest compared to the other materials that can be recovered over the five categories of WEEE. In Italy, the latter are R1 (Heaters and Refrigerators), R2 (Large Household Appliances), R3 (TV and monitors), R4 (Small Household Appliances), R5 (Lighting Equipment). The saved amounts for steel resulted in: 471 kg/ton (R1 category), 665 kg/ton (R2 category), 384 kg/ton (R3 category, FPDs), 490 kg/tons (R4 category). Material savings for commercial glass amounting to 800 kg/ton was mainly recovered in the R5 category. Precious materials savings mainly resulted in R3 and R4 categories and, in lower percentages, in R2 categories. R1 and R5 categories do not contain precious materials.
The results for the R4 category (where are included, e.g., mobile phones) show the following precious material savings: gold (5 g/ton), palladium (10 g/ton), silver (180 g/ton). In the year 2011, 9,849 tons of R4 have been collected in the Lombardy region. As a result, the potential savings of avoided primary precious materials was in the R4 category: 49.24 kg of gold, 98.49 kg of palladium, 1,772.82 kg of silver. The authors concluded that the environmental benefits of the recovery of the whole WEEE collected in the regional system have been higher than the overall impacts due to the recovery activities for most of the environmental categories (except human toxicity-cancer effects and freshwater ecotoxicity).
The recovery of C&DW is also beneficial for the environment. The available literature evaluated the environmental and socioeconomic benefits and costs of the C&DW management systems at the national scale in Finland (Dahlbo et al., 2015) and in some Italian regions: Lombardy in Northern Italy (Borghi et al., 2018) and Iodice et al. (2021) in Campania Region (Sourthern Italy). Moreover, Blengini and Garbarino (2010) considered the provincial scale (Torino in Northern Italy) as well as Ghisellini et al. (2021a) investigated the C&DW management system of the Metropolitan City of Naples. These two provinces shared similar C&DW composition compared to the Lombardy region where a high fraction (80%) of the total amount of C&DW generated consisted of mixed C&DW3. Blengini and Garbarino (2010) showed that avoided landfilling and avoided Natural Aggregates (NA) transportation are two relevant factors in determining the environmental sustainability of Recycle Aggregates from C&DW compared to NA. Ghisellini et al. (2021a) also show that the avoidance of landfilling and the avoided production of primary materials (steel, concrete, gravel, aluminum, other virgin materials) due to the recycling of 1 ton of C&DW have the potential of generating energetic and environmental benefits while reducing the dependence on fossil energy and the contribution to all the considered environmental impact categories (e.g., Global Warming, Ozone formation, Terrestrial Acidification, Land use, etcetera). The potential environmental benefits resulted higher than the environmental impacts of the recycling of C&DW and the highest contribution to the environmentally avoided characterized impacts resulted from steel recycling as the latter avoids the production of primary steel and the associated release of GHG emissions (145.29 kg CO2 equiv./ton of C&DW). Finally, the results by Borghi et al. (2018) evidence that the environmental benefits due to the recycling of the C&DW are higher than the environmental costs, only in a best-case scenario compared to the current scenario of the investigated year 2014. In the best-case scenario, they assumed the recycling of all the C&DW and no landfilling, the use of electricity in the recycling plants, and reduced transport distances. They concluded by providing some suggestions for improving the C&DW management system and its benefits. In fact, they highlighted the need for improving the quality of RA by means of the promotion of selective demolition on-site, with the goal of obtaining purer C&DW materials entering in the recycling plants. As such, the adoption of the concepts of design for reuse/recycling or design for disassembly is considered important in enhancing the circularity rate of a construction product (such as, e.g., buildings), the reuse of C&D products and components in new products or the recycling of C&D materials into recycled aggregates of higher quality (Zhang et al., 2012; Duan et al., 2015; Ghisellini et al., 2018).
The recycling of materials from waste (including C&DW or E-Waste) creates employment benefits (Gálvez-Martos and Istrate, 2020), both in developed [EPA (Environmental Protection Agency), 2020; Ghisellini and Ulgiati, 2020a; Alsheyab, 2022] and developing countries (Asante et al., 2019; Ezeudu et al., 2022). However, C&DW recycling compared to those of WEEE creates employment opportunities at the local level (Hossain et al., 2018; Iodice et al., 2021), while WEEE recycling could be performed very far from the point of collection and generation (Abalansa et al., 2021). Moreover, in the case of WEEE recycling appropriate technologies, infrastructures, and management plans should be adopted as well as rules and regulations be enforced at the political local level to prevent the potential negative impacts on the health of workers (Umair et al., 2015). Vulnerable local groups such as women and, in particular, children involved in e-waste recycling are exposed to very poor working conditions and are often common, e.g., in Pakistan, despite the country having ratified the ILO conventions C182 and C138 (Umair et al., 2015) that promote the abolition of child labor4. (McMahon et al., 2021). With recurring droughts as a result of climate change in many African countries, food security is threatened, but when local communities are employed by recycling activities, some income can be earned and used to buy food, thus preventing hunger (Okwu et al., 2022). Adequate technological and financial investments from developed countries, including the sharing of knowledge, should be transferred to developing countries along with the exports of WEEE to promote better recycling operations and favor a more just CE (Abalansa et al., 2021).
Solutions for improving urban mining of C&DW and WEEE management systems
Circular economy principles listed in Table 2 include the adoption of product design (such as design for disassembly, repair, reuse, recycling, reversable buildings, design for product life extension) (Charef et al., 2022), the reduction of the amount of C&DW or WEEE, the repair of products (in particular, for WEEE), reuse of products, materials and components as well as the recycling of materials at the end of their life (Bocken et al., 2016). The adoption of all the CE principles/solutions faces many challenges/barriers as their implementation requires changes in all societal systems (political-legislative, economic, cultural, technical-technological) (Davico, 2004; Fusco-Girard, 2016). The adoption of the solutions depends on different factors and, in particular, from favorable systems conditions such as the presence of policies, the vision of policy makers and their commitment toward the achievement of the CE goals, their capacity of involving and communicating with stakeholders, the role of universities, the involvement of non-profit organizations, collaborations along with the entire production chain and among different sectors and so on (Ellen MacArthur Foundation, 2012; Bosone, 2020; Ghisellini and Ulgiati, 2020b). The principles/solutions in Table 2 have been ordered according to the waste hierarchy (Zhang et al., 2022). The order can also be considered an order that ranks the most promising solutions in terms of their probable realization. The first principles, such as design, reduce, and repair, require changes, in particular, in the cultural mindset and the dissemination of new knowledge. This is the case of design for disassembly both in WEEE and C&DW sectors and design for product life extension whose adoption should be promoted at the political level, also by means of economic incentives (Giorgi et al., 2022). Moreover, a cultural change toward a less consumerist behavior should be done for WEEE as it is a strong barrier to the adoption of the reduction principle (Abalansa et al., 2021). However, policies/regulations in the case of C&DW (e.g., in China) are mainly focused on recycling rather than on the reduction of the amount of C&DW (Ghisellini et al., 2018; Huang et al., 2018), thus creating barriers to much virtuous and less costly solutions (in economic and technical terms) over the waste hierarchy (Zhang et al., 2022).
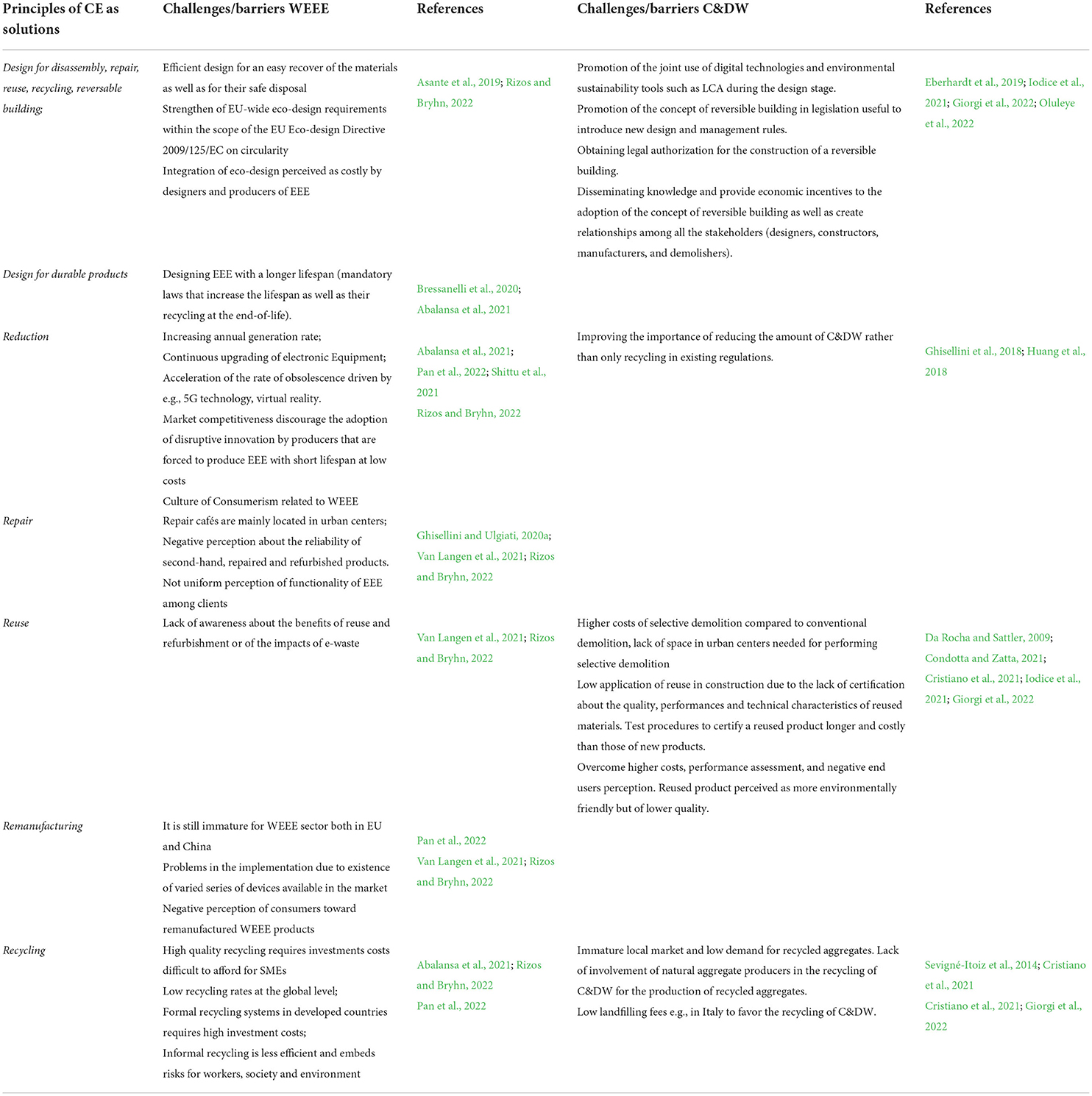
Table 2. A summary of the main CE principles and related challenges/barriers retrieved from the literature about WEEE and C&DW sectors.
The other CE principles/solutions, such as reuse, remanufacturing, and recycling, require technological investments rendering much difficult and costly their realization compared to the first set of principles as evidenced by Rizos and Bryhn (2022). The latter authors pointed out the difficulties of SMEs in embarking on a recycling of higher quality for WEEE. In a similar manner, the adoption of best practices, such as selective demolition that would facilitate C&DW reuse, would generate environmental and social benefits but higher costs requiring political tools for supporting such practice (Iodice et al., 2021).
Discussion and conclusions: Moving toward circular and just urban mining
In this brief study, we have shown some data on the trends of the current global consumption and management of natural resources. The data show that their use is still very inefficient, given the high amount of waste generated disposed of in landfills and not recovered as also in the case of C&DW and WEEE. CE proposes to rethink and reverse the pattern of the linear model of consumption of natural resources by means of different solutions for improving production and consumption efficiency and having as central the concept of waste elimination by design, design for disassembly, repair/reuse/recycling/refurbishment (Oluleye et al., 2022). We have underlined that framing the concept of urban mining in the CE practical model is a central message that this study would like to convey as it is relevant for the optimization of the whole urban mining process and prolong the use of virgin materials for achieving more sustainable patterns in their consumption. We have seen by a brief analysis of the metabolism of the Metropolitan city of Lisbon that, in DMC, a relevant share has the consumption of non-metallic minerals, biomass, fossil fuels, and metallic minerals. Stone, cement, and sand are the most significant materials consumed in the nonmetallic minerals category, while iron, steel-alloying metals, and ferrous metals have a share by 80% in total consumed metallic minerals. These materials are stocked temporarily in cities in the form of buildings, infrastructures, durable goods, and so on. The opportunities for their recycling at the end of life (for metals but also for non-metallic materials) are variable, depending, in particular, by their purity. In this view, a key point is the product design for optimizing their recycling potential. In that, the CE model is conceptually focused on product design contrary to the recycling economy model, and this, in turn, provides advantages for optimizing the urban mining as a process within its framework.
We have shown that the recycling of C&DW and WEEE can provide environmental and social benefits, but these processes should be governed to prevent undesirable effects such as in the case of WEEE where, currently, their export for recycling toward developing countries is a matter of high concern, in particular for vulnerable people. Indeed, recycling is only one of the options for strengthening urban mining in the CE framework as other solutions (shown in Section Solutions for improving urban mining of C&DW and WEEE management systems) are available. In this regard, some experiences about cities show how they are catalysts of innovation and extraordinary laboratories for experimenting and testing eco-innovative solutions (Prendeville et al., 2018; Repair, 2018), (Mazzarella and Amenta, 2022), incorporating more circular and socially just urban mining activities (Ghisellini and Ulgiati, 2020a) also useful for improving urban resource management and urban land use (Ulgiati and Zucaro, 2019). Repair cafès and companies specialized in the reuse/remanufacturing of e-waste in big Italian cities (e.g., Turin and Rome) are applying the principles of the CE in a way that is beneficial for the economy, the environment, and people. The repair, reuse, or remanufacturing of an EEE can save up to 80% of the materials needed to produce a new product. Such activities can also be sources of new job opportunities for young people or immigrants (Atlanteeconomiacircolare, electronics 2022)5.
Good practices beyond recycling can also be found for C&DW across Europe and at the global level (Legambiente, 2016, 2017; Ramakrishna et al., 2021; Oluleye et al., 2022). Several building projects show that, by means of the creation of a synergy between circular design and production, it is possible to design buildings that are easily disassembled at the end of life, and their C&D materials can be reused again for new buildings6. Furthermore, it is also possible to construct buildings made of a high share of recovered materials available, e.g., in a short distance of about 15 km from the construction site (Legambiente, 2016, 2017).
Finally, the monitoring and collection of data of urban mining and the CE implementation by means of different tools (including material flow accounting, life cycle assessment, social life cycle assessment) and related indicators are essential in order to improve the traceability of products at the end of their life and evaluate the distribution of the costs, benefits, and opportunities for the society and environment (Graedel, 2019; McLaren et al., 2020; Schroder, 2020; Niskanen et al., 2021). The research project, such as the just transition to circular economy that is funding this research, is the key to creating the needed knowledge base to understand the distribution of the benefits across all the involved stakeholders. Economic activities, due to the presence of externalities, do not always develop in a way that is socially sound, requiring the adoption of policies that correct such market failures and better align CE implementation with the three pillars of sustainable development (Ghisellini et al., 2021b). As just above mentioned, the case of WEEE recycling is exemplary as the European Model of WEEE management is currently mainly driven by the principle of economic efficiency, disregarding those of the environmental and social justice. Another limitation of the model is that it is unable to treat all the WEEE collected in the EU countries, and most of them are exported toward developing countries. In this way, the CE model perpetuates the same logic and values of the linear and recycling economy (Mah, 2021) and does not promote environmental and social innovation and environmental and social justice. We conclude claiming that the opportunities for urban mining in the CE framework are still rather unexplored in terms of resource valorization [agreeing with Zucaro et al. (2022)] and potential social benefits justifying the urgency of improving their awareness in all the stakeholders.
Data availability statement
The original contributions presented in the study are included in the article/supplementary material, further inquiries can be directed to the corresponding author.
Author contributions
PG contributed to the conception and design of the study and wrote the first and further revised drafts of the study. All authors contributed to the manuscript revision, read, and approved the submitted version.
Funding
This research has been funded by the European Commission's research program Horizon 2020-SC5-2020-2 scheme, Grant Agreement 101003491 (JUST Transition to the Circular Economy project) and the project Realizing the Transition toward the Circular Economy: Models, Methods, and Applications (ReTraCE), funded by the H2020-MSCA ITN-2018 program (Grant No. 814247).
Conflict of interest
The authors declare that the research was conducted in the absence of any commercial or financial relationships that could be construed as a potential conflict of interest.
Publisher's note
All claims expressed in this article are solely those of the authors and do not necessarily represent those of their affiliated organizations, or those of the publisher, the editors and the reviewers. Any product that may be evaluated in this article, or claim that may be made by its manufacturer, is not guaranteed or endorsed by the publisher.
Abbreviations
C&DW, Construction and demolition waste; CE, Circular Economy; EU, European Union; REE, Rare Earth Elements; WEEE, Waste from Electrical and Electronic Equipment.
Footnotes
1. ^https://reports.weforum.org/toward-the-circular-economy-accelerating-the-scale-up-across-global-supply-chains/reorganize-and-streamline-pure-materials-flows/
2. ^https://www.mining.com/web/making-the-grade-understanding-exploration-results/
3. ^The average composition in the study area (Province of Torino) by Blengini and Garbarino was: 47.3% mixed C&DW 170,904:28.6%; soil and stones, 170,504:15.7%; bitouminous mixtures, 170,302:15.7%; concrete, bricks, and tiles, 170,107:5.1%; concrete, 170,101:2.3%; other fractions are below 1%. The average composition of C&DW in the Metropolitan City of Naples (Ghisellini et al., 2021a) is the following: Mixed C&DW, 170,904, 47.37%; soil and stones, 170,504, 24.81%; iron and steel, 170,405: 7.03%; bitouminous mixtures, 170,302, 5.25%; concrete, 170,101: 6.69%; dredging spoils, 170,506: 4.5%; track ballast, 170,508: 1.6%; other fractions are below 1%.
4. ^ILO Conventions on child labor. Available online at: https://www.ilo.org/ipec/facts/ILOconventionsonchildlabour/lang–en/index.htm (accessed July 4, 2022).
5. ^See, e.g. Reware: https://economiacircolare.com/atlante/reware/#field-group-tab-2 and Astelav: https://economiacircolare.com/atlante/astelav/#images (accessed April 27, 2022).
6. ^Circular Pavillion in Amsterdam. Available online at: https://circl.nl/themakingof/en/ (accessed March 27, 2022).
References
Abalansa, S., El Mahrad, B., Icely, J., and Newton, A. (2021). Electronic waste, an environmental problem exporteddeveloping countries: the GOOD, the BAD and the UGLY. Sustainability 13, 5302, doi: 10.3390/su13095302
Aldebei, F., and Dombi, M. (2021). Mining the built environment: telling the story of urban mining. Buildings 11, 388. doi: 10.3390/buildings11090388
Alsheyab, M. A. T. (2022). Recycling of construction and demolitionwaste and its impacton climatechange and sustainabledevelopment. Int. J. Environ. Sci. Technol. 19, 2129–2138. doi: 10.1007/s13762-021-03217-1
Angerer, G., B?tcher, K., and Bars, P. (1993). Verwertung von Elektronikschrott - Stand der Technik.
Arora, R., Paterok, K., BanerJee, A., and Saluja, M. S. (2017). Potential and relevance of urban mining in the context of sustainable cities. IIMB Manag. Rev. 29, 210–224. doi: 10.1016/j.iimb.2017.06.001
Art, S. (2008). Personal communication with S. Art, Sales and Customer Service. Hoboken, NJ; Belgium: Umicore Precious Metals Refining.
Asante, K. A., Amoyaw-Osei, Y., and Agusa, T. (2019). E-wasterecycling in Africa: risks and opportunities. Curr. Opin. Gree Sustain. Chem. 18, 109–117. doi: 10.1016/j.cogsc.2019.04.001
Awasthi, A. K., and Li, J. (2017). Management of electrical and electronic waste: a comparative evaluation of China and India. Renew. Sust. Energ. Rev. 76, 434–447. doi: 10.1016/j.rser.2017.02.067
Barles, S. (2009). Urban metabolism of Paris and itsregion. J. Ind. Ecol. 13, 898–913. doi: 10.1111/j.1530-9290.2009.00169.x
Berman, N., Couttiner, M., Rohner, D., and Thoenig, M. (2017). This mine is mine!how minerals fuel conflicts in Africa. Am. Econ. Rev. 107, 1564–1610. doi: 10.1257/aer.20150774
Biganzoli, L., Falbo, A., Forte, F., Grosso, M., and Rigamonti, L. (2015). Mass balance and life cycle assessment of the waste electrical and electronic equipment management system implemented in Lombardia Region (Italy). Sci. Total Environ. 524–w525, 361–375. doi: 10.1016/j.scitotenv.2015.04.041
Blengini, G. A. (2009). Life cycle of buildings, demolition and recycling potential: a case study in Turin, Italy. Build. Environ. 44, 319–330. doi: 10.1016/j.buildenv.2008.03.007
Blengini, G. A., and Garbarino, E. (2010). Resources and waste management in Turin (Italy): the role of recycledaggregatesin the sustainable supply mix. J. Clean. Prod. 18, 1021–1030. doi: 10.1016/j.jclepro.2010.01.027
Bocken, N. M. P., de Pauw, I., Bakker, C., and van der Grinten, B. (2016). Product design and business model strategies for a circular economy. J. Indu. Prod. Eng. 33, 308–320. doi: 10.1080/21681015.2016.1172124
Borghi, G., Pantini, S., and Rigamonti, L. (2018). Life cycle assessment of non-hazardous construction and demolition waste (CDW) management in lombardy region (Italy). J. Clean. Prod. 184, 815–825. doi: 10.1016/j.jclepro.2018.02.287
Borthakur, A., and Govind, M. (2017). Emerging trends in consumers' E-waste disposal behaviour and awareness: a worldwide overview with special focus on India. Resour. Conserv. Recycl. 117, 102–113. doi: 10.1016/j.resconrec.2016.11.011
Bosone, M. (2020). “Culture as a driver of circular urban regeneration,” in Technological Innovation for the Built Environment, eds S. Viola, Z. Ul Abedin. Cutlural and Creative Industries. (La Scuola di PitagoraEditrice: Napoli).
Boulding, K. (1966). “The economics of the coming spaceship earth,” in Environmental Quality in a Growing Economy, eds Jarret (Baltimore, MD: Johns Hopkins University Press), 3–14.
Bressanelli, G. M., Saccani, N., Pigosso, D. C. A., and Perona, M. (2020). Circular Economy in the WEEE industry: a systematic literature review and a research agenda. Sustainable Production and Consumption 23, 174–188. doi: 10.1016/j.spc.2020.05.007
Butsic, V., Baumann, M., Shortland, A., Waler, S., and Kuemmerle, T. (2015). Conservation and conflict in the Democratic Republic of Congo: the impacts of warfare, mining, and protected areas on deforestation. Biol. Conserv. 191, 266–273. doi: 10.1016/j.biocon.2015.06.037
Cesaro, A., Belgiorno, V., Vaccari, M., Jandric, A., Chung, T. D., Dias, M. I., et al. (2018). A device-specificprioritization strategy based on the potentialfor harm to human health in informal WEEE recycling. Environ. Sci. Pollut. Res. 25, 683–692, doi: 10.1007/s11356-017-0390-7
Chancerel, P., Meskers, C. E. M., Hagelukan, C., and Rotter, V. S. (2009). Assessment of precious metal flows during preprocessing of waste electrical and electronic equipment. J. Indust. Ecol. 13, 791–810. doi: 10.1111/j.1530-9290.2009.00171.x
Charef, R., Lu, W., and Hall, D. (2022). The transition to the circular economy of the constructionindustry: Insights intosustainableapproaches to improve the understanding. J. Clean. Prod. 364, 132421. doi: 10.1016/j.jclepro.2022.132421
Chen, P., and Shi, X. Q. (2020). [Predicting the recycling potential and evaluating the environmental benefits of waste electrical and electronic equipment in Beijing-Tianjin-Hebei]. Huan Jing Ke Xue. 41, 1976–1986. doi: 10.13227/j.hjkx.201909249
Condotta, M., and Zatta, E. (2021). Reuse of building elements in the architectural practice and the Europeanregulatorycontext: inconsistencies and possibleimprovements. J. Clean. Prod. 318, 128413. doi: 10.1016/j.jclepro.2021.128413
Cossu, R., and Williams, I. D. (2015). Urban mining: concepts, terminology, challenges. Waste Manag. 45, 1–3. doi: 10.1016/j.wasman.2015.09.040
Cristiano, S., Ghisellini, P., D'Ambrosio, G., Xue, J., Nesticò, A., Gonella, F., et al. (2021). Construction and demolitionwaste in the Metropolitan City of Naples,Italy: state of the art, circular design, and sustainable planning opportunities. J. Clean. Prod. 293, 125856. doi: 10.1016/j.jclepro.2021.125856
Cui, X., Wang, X., and Feng, Y. (2019). Examiningurbanmetabolism: a material flow perspective on citiesand theirsustainability. J. Clean. Prod. 214, 767–781. doi: 10.1016/j.jclepro.2019.01.021
Da Rocha, C. G., and Sattler, M. A. (2009). A discussion on the reuse of building componentsin Brazil: an analysis of major social, economical and legalfactors. Resour. Conserv. Recycl. 54, 104–112. doi: 10.1016/j.resconrec.2009.07.004
Dahlbo, H., Bachér, J., Lähtinen, K., Jouttijärvi, T., Suoheimo, P., Mattila, T., et al. (2015). Construction and demolitionwaste management e a holisticevaluation of environmental performance. J. Clean. Prod. 107, 333–341. doi: 10.1016/j.jclepro.2015.02.073
Deutz, P. (2020). “Circular economy,” in International Encyclopedia of Human Geography 2nd Edn,193–201. Available online at: https://www.sciencedirect.com/referencework/9780081022962/international-encyclopedia-of-human-geography
Duan, H., Wang, J., and Huang, Q. (2015). Encouraging the environmentally sound management of C&D waste in China: an integrative review and research agenda. Renew. Sustain. Energy Rev. 43, 611–620. doi: 10.1016/j.rser.2014.11.069
Eberhardt, L. C. M., Birgisdottir, H., and Birkved, M. (2019). Potential of circular economy in sustainable buildings. IOP Conf. Ser. Mater. Sci. Eng. 471, 092051. doi: 10.1088/1757-899X/471/9/092051
Ellen MacArthur Foundation (2012). Towards the Circular Economy. Available online at: https://www.aquafil.com/assets/uploads/ellen-macarthur-foundation.pdf (accessed July 8, 2022).
EPA (Environmental Protection Agency) (2020). Recycling Economic Information Report 2020. Available online at: https://www.epa.gov/sites/default/files/2020-11/documents/rei_report_508_compliant.pdf (accessed April 26, 2022).
Ernst, T., Popp, R., Wolf, M., and van Eldik, R. (2003). Analysis of eco-relevant elements and noble metals in printed wiring boards using AAS, ICP-AES and EDXRF. Anal. Bioanal. Chem. 375, 805–814. doi: 10.1007/s00216-003-1802-8
European Commission (2020). Communication From the Commission to the European Parliament, The Council, The European Economic and Social Committee and The Committee of The Regions a New Circular Economy Action Plan for a Cleaner and More Competitive Europe Com/2020/98 Final Available online at: https://eur-lex.europa.eu/legal-content/EN/TXT/?qid=1583933814386&uri=COM:2020:98:FIN (accessed January 05, 2022).
EUROSTAT (2022). Available online at: https://ec.europa.eu/eurostat/statistics-explained/index.php?title=Waste_statistics_-_electrical_and_electronic_equipment&oldid=552555 (accessed March 02, 2022).
Ezeudu, O., Agunwamba, J. C., Ugochukwu, U., and Oraelosi, T. (2022). Circular economy and frugal innovation: a conceptual nexus. Environ. Sci. Pollut. Res. 29, 29719–29734. doi: 10.1007/s11356-022-18522-6
Favot, M., and Massarutto, A. (2019). Rare-earth elements in the circular economy: The case of yttrium. J. Environ. Manage. 240, 504–510. doi: 10.1016/j.jenvman.2019.04.002
Forti, V., Balde, C. P., Kuehr, R., and Bel, G. (2020). The Global E-waste Monitor 2020: Quantities, flows and the circular economy potential. United Nations University (UNU)/United Nations Institute for Training and Research (UNITAR) - co-hosted SCYCLE Programme, International Telecommunication Union (ITU) and International Solid Waste Association (ISWA), Bonn/Geneva/Rotterdam. Available online at: https://www.itu.int/en/ITU-D/Environment/Documents/Toolbox/GEM_2020_def.pdf (accessed August 28, 2022).
Frosch, R. A. (1992). Industrial ecology: A philosophical introduction. Proc. Natl. Acad. Sci. U. S. A. 89, 800–803. doi: 10.1073/pnas.89.3.800
Fu, C., Zhang, Y., Deng, T., and Daigo, I. (2021). The evolution of material stock research, From exploring to rising to hot studies. J. Ind. Ecol. 26, 462–476. doi: 10.1111/jiec.13195
Fusco-Girard, L. (2016). The city and the territory system: towards the “new humanism” paradigm. Agric. Sci. Procedia 8, 542–551. doi: 10.1016/j.aaspro.2016.02.070
Gálvez-Martos, J.-L., and Istrate, I.-R. (2020). “Construction and demolitionwaste management,” in Advances in Construction and Demolition Waste Recycling: Management, Processing and Environmental Assessment, eds F. Pacheco-Torgal, Y. Ding, F Colangelo, R. Tuladhar, A. Koutamanis, UK: Woodhead Publishing.
Geissdoerfer, M., Savaget, P., Bocken, N. M. P., and Hultink, E. J. (2017). The circular economy e a new sustainabilityparadigm? J. Clean. Prod. 143, 757–768. doi: 10.1016/j.jclepro.2016.12.048
Ghisellini, P., Cialani, C., and Ulgiati, S. (2016). A review on circular economy: the expected transition to a balanced interplay of environmental and economic systems. J. Clean. Prod. 114, 11–32. doi: 10.1016/j.jclepro.2015.09.007
Ghisellini, P., Ncube, A., D'Ambrosio, G., Passaro, R., and Ulgiati, S. (2021a). Potential energy savings from circular economy scenarios based on construction and agri-food waste in Italy. Energies 14, 8561. doi: 10.3390/en14248561
Ghisellini, P., Passaro, R., and Ulgiati, S. (2021b). Revisiting keynes in the light of the transition to circular economy. Cir. Econ. Sustain. 1, 143–171. doi: 10.1007/s43615-021-00016-1
Ghisellini, P., Ripa, M., and Ulgiati, S. (2018). Exploringenvironmental and economic costsand benefits of a circular economy approach to the construction and demolitionsector. A literature review. J. Clean. Prod. 178, 618–643. doi: 10.1016/j.jclepro.2017.11.207
Ghisellini, P., Santagata, R., Zucaro, A., and Ulgiati, S. (2019). Circular patterns of wasteprevention and recovery. E3S Web Conf. 119, 00003. doi: 10.1051/e3sconf/201911900003
Ghisellini, P., and Ulgiati, S. (2020a). Circular economy transition in Italy. Achievements, perspectives and constraints. J. Clean. Prod. 243, 118360. doi: 10.1016/j.jclepro.2019.118360
Ghisellini, P., and Ulgiati, S. (2020b). “Managing the transition to the circular economy,” in Handbook of the Circular Economy, eds M. Brandão, D. Lazarevic, G. Finnvenden (Cheltenham; Northampton, MA: Edward Elgar Publishing).
Gidarakos, E., and Akcil, A. (2020). WEEE under the prism of urban mining. Waste Manag. 102, 950–951. doi: 10.1016/j.wasman.2019.11.039
Giorgi, S., Lavagna, M., Wang, K., Osmani, M., and Liu, G. (2022). Drivers and barrierstowardscircular economy in the building sector: stakeholder interviews and analysis of fiveEuropean countries policies and practices. J. Clean. Prod. 336, 130395. doi: 10.1016/j.jclepro.2022.130395
Graedel, T. E. (2011). The prospect for urban mining. The bridge: linking engineering and society. Urban Sustainability. The Bridge 41, 43–50. Available online at: https://www.nae.edu/47182/The-Prospects-for-Urban-Mining
Graedel, T. E. (2019). Material flow analysis from origin to evolution. Environ. Sci. Technol. 53, 12188–12196. doi: 10.1021/acs.est.9b03413
Haas, W., Krausmann, F., Wiedenhofer, D., and Heinz, M. (2015). How circular is the global economy? An assessment of material flows, waste production, and recycling in the european union and the world in 2005. J. Indust. Ecol. 19, 765–777. doi: 10.1111/jiec.12244
Hagelücken, C., and Corti, C. (2016). Recycling of gold from electronics: costeffective use through “Design for Recycling”. Gold Bull. 43, 209–220. doi: 10.1007/BF03214988
Hagelüken, C. (2006). “Improving metal returns and eco-efficiency in electronics recycling - a holistic approach for interface optimisation between pre-processing and integrated metals smelting and refining, in Proceedings of the IEEE International Symposium on Electronics & the Environment (San Francisco, CA: IEEE), 218–223.
Heckens, T. (2021). Governance of the World's Mineral Resources, Beyond the Foreseeble Future. Netherlands: Elsevier.
Hoekman, P., and Von Blottnitz, H. (2016). Cape town'smetabolism, insights from a material flow analysis. J. Ind. Ecol. 21, 1237–1249. doi: 10.1111/jiec.12508
Hossain, U., Poon, C. S., Dong, Y. H., Lo, I. M. C., and Cheng, J. C. P. (2018). Development of social sustainabilityassessmentmethodand a comparative case study on assessingrecycledconstructionmaterials. Int. J. Life Cycle Assess 23,1654–1674. doi: 10.1007/s11367-017-1373-0
Huang, B., Wang, X., Kua, H., Geng, Y., Bleischwitz, R., and Ren, J. (2018). Construction and demolitionwaste management in China through the 3R principle. Resour. Conserv. Recycl. 129, 36–44. doi: 10.1016/j.resconrec.2017.09.029
Huisman, J., Magalini, F., Kuehr, R., Maurer, C., Ogilvie, S., Poll, J., et al. (2007). 2008 Review of Directive 2002/96 on Waste Electrical and Electronic Equipment. Final Report. Bonn: United Nations University.
Ingwersen, W. W. (2011). Emergy as a life cycle impact assessment indicator, a gold mining case study. J. Indust. Ecol. 15, 550–567. doi: 10.1111/j.1530-9290.2011.00333.x
Iodice, S., Garbarino, E., Cerreta, M., and Tonini, D. (2021). Sustainability assessment of construction and demolition waste management applied to an Italian case. Waste Manag. 128, 83–98. doi: 10.1016/j.wasman.2021.04.031
Ji, X, Han, M., and Ulgiati, S. (2020). Optimal allocation of direct and embodied arable land associated to urban economy: Understanding the options deriving from economic globalization. Land Use Policy 91, 104392. doi: 10.1016/j.landusepol.2019.104392
Johansson, N., Krook, J., Eklund, M., and Berglund, B. (2013). An integrated review of concepts and initiatives for mining the technosphere: towards a new taxonomy. J. Clean. Prod. 55, 35–44. doi: 10.1016/j.jclepro.2012.04.007
Keller, M. (2006). Assessment of Gold Recovery Processes in Banaglore, India and Evaluation of an Alternative Recycling Path for Printed Wiring Boards. Empa: Institute for Spatial and Landscape Planning, Regional Resource Management; Technology and Society Lab.
Kirchherr, J., Reike, D., and Hekkert, M. (2017). Conceptualizing the circular economy: An analysis of 114 definitions. Resour. Conserv. Recycl. 127, 221–232. doi: 10.1016/j.resconrec.2017.09.005
Koutamanis, A., Reijn, B., and van Bueren, E. (2018). Urban mining and buildings: a review of possibilities and limitations. Resour. Conserv. Recycl. 138, 32–39. doi: 10.1016/j.resconrec.2018.06.024
Kramer, T. (1994). “Mechanische Aufbereitung von Leiterplatten [Mechanical processing of printed circuit boards],” in Verwertung von Elektro- und Elektronikger?aten [Recycling of electrical and electronic appliances].
Krausmann, F., Lauk, C., Haas, W., and Wiedenhofer, D. (2018). From resource extraction to outflows of wastes and emissions: the socioeconomic metabolism of the global economy, 1900–2015. Glob. Environ. Change 52 131–140. doi: 10.1016/j.gloenvcha.2018.07.003
Krook, J., and Baas, L. (2013). Getting serious about mining the technosphere: a review of recent landfill mining and urban mining research. J. Clean. Prod. 55, 1–9. doi: 10.1016/j.jclepro.2013.04.043
Legambiente (2016). Secondo Rapportodell'Osservatorio Recycle. Available online at:https://www.legambiente.it/sites/default/files/docs/dossier_recycle_2016.pdf (accessed March 4, 2022).
Legambiente (2017). Rapporto Osservatorio Recycle. Available online at: at:https://www.legambiente.it/sites/default/files/docs/rapporto_recycle_2017.pdf (accessed March 27, 2022).
Legarth, J. B., Altmg, L., Danzer, B., Tartler, D., Brodersen, K., Scheller, H., et al. (1995). New strategy in the recycling of printed circuit. Circuit World. 21, 10–15. doi: 10.1108/eb044031
Liu, Y., Li, H., An, H., Santagata, R., Liu, X., and Ulgiati, S. (2021). Environmental and economicsustainability of key sectors in China'ssteelindustry chain: an application of the emergy accounting approach. Ecol. Indic. 129, 103011. doi: 10.1016/j.ecolind.2021.108011
Liu, Y., Li, H., Huang, S., An, H., Santagata, R., and Ulgiati, S. (2020). Environmental and economic-related impact assessment of iron andsteel production. A call for sharedresponsibility in global trade. J. Clean. Prod. 269, 122239. doi: 10.1016/j.jclepro.2020.122239
Mah, A. (2021). Future-proofing capitalism: the paradox of the circular economy for plastics. Global Environ. Politics 21, 121–142. doi: 10.1162/glep_a_00594
Mancini, L., and Sala, S. (2018). Social impact assessment in the mining sector: review and comparison of indicators frameworks. Res. Policy 57, 98–111. doi: 10.1016/j.resourpol.2018.02.002
Mazzarella, C., and Amenta, L. (2022). “The Circular Metabolic Urban Landscape,” in Regenerative Territories GeoJournal Library, Vol. 128, eds L. Amenta, M. Russo, and A. van Timmeren (Springer, Cham).
McLaren, D., Niskanen, J., and Anshelm, J. (2020). Reconfiguringrepair: contestedpolitics and values of repair challenge instrumental discourses found in circular economies literature. Resour. Conserv. Recycl. 8, 100046, doi: 10.1016/j.rcrx.2020.100046
McMahon, K., Ryan-Fogarty, Y., and Fitzpatrick, C. (2021). Estimating job creation potential of compliant WEEE pre-treatment in Ireland. Resour. Conserv. Recycl. 166, 105230. doi: 10.1016/j.resconrec.2020.105230
Modoi, O.-C., and Mihai, F.-C. (2022). E-waste and end-of-life vehicles management and circular economy initiatives in Romania. Energies 15, 1120. doi: 10.3390/en15031120
Nakamura, T., and Halada, K. (2015). “Potential of urban mine,” in SpringerBriefs in Applied Sciences and Technology. Urban Mining Systems (Springer, Tokyo).
Niskanen, J., McLaren, D., and Anshelm, J. (2021). Repair for a broken economy: lessons for circular economy from an international interview study of repairers. Sustainability 13, 2316, doi: 10.3390/su13042316
Niza, S., Rosado, L., and Ferrão, P. (2009). Urban metabolismmethodological advances in urbanmaterial flow accounting based on thelisbon case study. J. Ind. Ecol. 13, 384–405. doi: 10.1111/j.1530-9290.2009.00130.x
Nuss, P., and Eckelman, M. J. (2014). Life cycle assessment of metals: a scientific synthesis. PLoS ONE 9, e101298. doi: 10.1371/journal.pone.0101298
Okwu, O., Hursthouse, A., Viza, E., and Idoko, L. (2022). New models to reduce the health risks of informal WEEE recyclers in MTN phone village, rumukurushi, port harcourt, Nigeria. Toxics 10, 84. doi: 10.3390/toxics10020084
Oluleye, B. I., Chan, D. W. M., Saka, A. B., and Olawumi, T. O. (2022). Circular economy research on building construction and demolition waste: a global review of current trends and future research directions. J. Clean. Prod. 357, 131927. doi: 10.1016/j.jclepro.2022.131927
Ottoni, M., Dias, P., and Xavier, L. E. (2020). A circular approach to the e-waste valorization through urban mining in Rio de Janeiro, Brazil. J. Clean. Prod. 261, 120990. doi: 10.1016/j.jclepro.2020.120990
Pan, X., Wong, C. W. Y., and Li, C. (2022). Circular economy practices in the waste electrical and electronicequipment (WEEE) industry: A systematic review and future research agendas. J. Clean. Prod. 365, 132671. doi: 10.1016/j.jclepro.2022.132671
Panchal, R., Singh, A., and Diwan, H. (2021). Does circular economy performance lead to sustainable development? – A systematic literature review. J. Environ. Manage 293, 112811. doi: 10.1016/j.jenvman.2021.112811
Prendeville, S., Cherim, E., and Bocken, E. (2018). Circular cities: mapping six cities in transition. Environ. Innov. Soc. Transit. 26, 171–194. doi: 10.1016/j.eist.2017.03.002
Ramakrishna, S., Pervaiz, M., Tjong, J., Ghisellini, P., and Sain, M. M. (2021). Low-Carbon Materials: Genesis, Thoughts, Case Study, and Perspectives. Circular Economy and Sustainability. doi: 10.1007/s43615-021-00135-9
Repair. (2018). D5.3 Eco-Innovative Solutions Naples. Available online at: http://h2020repair.eu/wp-content/uploads/2019/10/Deliverable-5.3-Eco-Innovative-Solutions-Naples.pdf (accessed August 28, 2022).
Rizos, V., and Bryhn, J. (2022). Implementation of circular economy approaches in the electrical and electronicequipment (EEE) sector: barriers, enablers and policy insights. J. Clean. Prod. 338, 130617. doi: 10.1016/j.jclepro.2022.130617
Rosado, L., Niza, S., and Ferrão, P. (2014). A material flow accountingcase study of the lisbon metropolitan area using the urbanmetabolism analyst model. J. Indust. Ecol. 18, 84–101. doi: 10.1111/jiec.12083
Schroder, P. (2020). Promoting a Just Transition to a An Inclusive Circular Economy. Chatham House. Available online at: https://www.chathamhouse.org/2020/04/promoting-just-transition-inclusive-circular-economy (accessed March 4, 2022).
Serranti, S. (2021). Urban Mining: l'alternativasostenibileallosfruttamentodellerisorse non rinnovabili (In Italian). Available online at: https://educazionecivica.zanichelli.it/urban-mining-l-alternativa-sostenibile-allo-sfruttamento-delle-risorse-non-rinnovabili (accessed March 4, 2022).
Sevigné-Itoiz, E., Gasol, C. M., Rieradevall, J., and Gabarell, X. (2014). Environmental consequences of recycling aluminum old scrap in a global market. Resour. Conserv. Recycl. 89, 94–103. doi: 10.1016/j.resconrec.2014.05.002
Shittu, O. S., Williams, I. D., and Shaw, P. J. (2021). Global E-waste management: can WEEE make a difference? A review of e-waste trends, legislation, contemporary issues and future challenges. Waste Manag. 120, 549–563. doi: 10.1016/j.wasman.2020.10.016
Silva, R. V., De Brito, J., and Dhir, R. K. (2017). Availability and processing of recycled aggregates within the construction and demolition supply chain: a review. J. Clean. Prod. 143, 598–614. doi: 10.1016/j.jclepro.2016.12.070
Stahel, W. (1981). “The product-life factor,” in An Inquiry into the Nature of Sustainable Societies: the Role of the Private Sector, eds S. Grinton (Houston, TX: HARC), 72–96.
Thormark, C. (2006). The effect of material choice on the total energy need and recycling potential of a building. Build. nviron. 41, 1019–1026. doi: 10.1016/j.buildenv.2005.04.026
Ulgiati, S., Ascione, M., Bargigli, S., Cherubini, F., Federici, M., Franzese, P. P., et al. (2010). “Multi-method and multi-scale analysis of energy and resource conversion and use,” in Energy Options Impact on Regional Security. NATO Science for Peace and Security Series C: Environmental Security, eds F. Barbir and S. Ulgiati (Dordrecht: Springer).
Ulgiati, S., and Zucaro, A. (2019). Challenges in urban metabolism: sustainability and well-being in cities. Front. Sustain. Cities 1, 1. doi: 10.3389/frsc.2019.00001
Umair, S., Björklund, A., and Petersen, E. E. (2015). Social impact assessment of informal recycling of electronic ICT waste in Pakistan using UNEPz SETAC guidelines. Resour. Conserv. Recycl. 95, 46–57. doi: 10.1016/j.resconrec.2014.11.008
UNEP. (2011). Recycling Rates of Metals - A Status Report: A Report of the Working Group on the Global Metal Flows to the International Resource Panel, eds T. E. Graedel, J. Allwood, J. -P. Birat, B. K. Reck, S. F. Sibley, G. Sonnemann, M. Buchert, and C. Hagelüken. Available online at: https://www.resourcepanel.org/reports/recycling-rates-metals (accessed August 28, 2022).
United Nations (2022). 11 Sustainable Cities and Communities. Available online at: https://www.globalgoals.org/goals/11-sustainable-cities-and-communities/ (accessed September 12, 2022).
Van Buren, N., Demmers, M., Van derHeijden, R., and Witlox, F. (2016). Towards a circulareconomy: the role of Dutch logisticsindustries and governments. Sustainability8, 647. doi: 10.3390/su8070647
Van Langen, S. K., Vassillo, C., Ghisellini, P., Restaino, D., Passaro, R., and Ulgiati, S. (2021). Promotingcircular economy transition: a study aboutperceptions and awareness by different stakeholders groups. J. Clean. Prod. 316, 128166. doi: 10.1016/j.jclepro.2021.128166
Wiedenhofer, D., Fishman, T., Plank, B., Miatto, A., Lauk, C, Haas, W., et al. (2021). Prospects for a saturation of humanity's resource use? An analysis of material stocks and flows in nine world regions from 1900 to 2035. Glob. Environ. Change 71, 102410. doi: 10.1016/j.gloenvcha.2021.102410
Withanage, S. V., and Habib, K. (2021). Life cycleassessment and material flow analysis: two under-utilized tools for informing E-waste management. Sustainability 13, 7939. doi: 10.3390/su13147939
World Economic Forum (2021). How Construction Innovations are Enabling the Transition to a Circular Economy. Available online at: https://www.weforum.org/agenda/2021/11/how-construction-innovations-enabling-circular-economy/ (accessed January 5, 2022).
World Economic Forum (2022). Focus on Pure Materials Stock Management at the Outset. Available online at: https://reports.weforum.org/toward-the-circular-economy-accelerating-the-scale-up-across-globalsupply-chains/focus-on-pure-materials-stock-management-at-the-outset/ (accessed August 28, 2022).
Xavier, L. H., Giese, E. C., Ribeiro-Duthie, A. C., and Lins, F. A. F. (2021). Sustainability and the circular economy: a theoretical approach focused on e-waste urban mining. Res. Policy 74, 101467. doi: 10.1016/j.resourpol.2019.101467
Zhang, C., Hu, M., Di Maio, F., Sprecher, B., Yang, X., and Tukker, A. (2022). An overview of the wastehierarchy framework for analyzing the circularity in construction and demolitionwaste management in Europe. Sci. Total Environ. 803, 149892. doi: 10.1016/j.scitotenv.2021.149892
Zhang, S., Ding, Y., Liu, B., and Chang, C.-C. (2017). Supply and demand of some critical metals and present status of their recycling in WEEE. Waste Manag. 65, 113–127. doi: 10.1016/j.wasman.2017.04.003
Zhang, X., Wu, Y., and Shen, L. (2012). Application of low waste technologies for design and construction: a case study in Hong Kong. Renew. Sustain. Energy Rev. 16, 2973–2979. doi: 10.1016/j.rser.2012.02.020
Zheng, X., Xiao, T., Xu, G., Albalghiti, E., Shan, G., and Li, J. (2021). Comparing the costs and benefits of virgin and urban mining. J. Manag. Sci. Eng. 7, 98–106. doi: 10.1016/j.jmse.2021.05.002
Keywords: circular economy, construction and demolition waste, waste electrical and electronic equipment, urban mining, circular urban mining, just urban mining
Citation: Ghisellini P, Ncube A, Casazza M and Passaro R (2022) Toward circular and socially just urban mining in global societies and cities: Present state and future perspectives. Front. Sustain. Cities 4:930061. doi: 10.3389/frsc.2022.930061
Received: 27 April 2022; Accepted: 15 August 2022;
Published: 30 September 2022.
Edited by:
Amalia Zucaro, Italian National Agency for New Technologies, Energy and Sustainable Economic Development (ENEA), ItalyReviewed by:
Graziano Salvalai, Politecnico di Milano, ItalyAndrew Hursthouse, University of the West of Scotland, United Kingdom
Copyright © 2022 Ghisellini, Ncube, Casazza and Passaro. This is an open-access article distributed under the terms of the Creative Commons Attribution License (CC BY). The use, distribution or reproduction in other forums is permitted, provided the original author(s) and the copyright owner(s) are credited and that the original publication in this journal is cited, in accordance with accepted academic practice. No use, distribution or reproduction is permitted which does not comply with these terms.
*Correspondence: Patrizia Ghisellini, patrizia.ghisellini@uniparthenope.it