- 1Chair for Strategic Landscape Planning and Management, School of Life Science, Technical University of Munich, Munich, Germany
- 2Center for Catastrophic Risk Management, Social Science Matrix, University of California, Berkeley, Berkeley, CA, United States
- 3Riverlab, Landscape Architecture and Environmental Planning, College of Environmental Design, University of California, Berkeley, Berkeley, CA, United States
Urban rivers deliver a broad range of contributions of nature to human development. The exploitation of the riverine ecosystem services evolves with uncertainty. In addition to the current decreasing of ecosystem health and changing climate, the impact of societal development on the river system directly reduces the potential delivery of future ecosystem services. What are the drivers of the design of urban river parks and what upcoming challenges will need to be addressed? This paper presents the results of an ex-post-analysis and comparative analysis of planning orientations of two urban river parks, the Isar River in Munich (Germany) and the American River in Sacramento (U.S.). The contribution describes the evolution of the urban rivers based on planning strategy, identifies drivers of changes and discusses the upcoming challenges for urban river parks that need to be addressed in the short and long term. The contribution shows that urban river park designs occur in a larger context of human demographic, technological, and economic changes. The contribution does not intend to solve the very complex and urgent challenges the case study sites face but rather to highlight research needs to support adaptation strategies.
Introduction
Due to the presence and dynamics of freshwater, surface water bodies provide a multitude of contributions of nature to human development, which are also known as ecosystem services. Riverine areas are consequently hotspots for economic and cultural development (Wantzen et al., 2016). Because of the close relationship between the eco-physical-chemical system and human societies, surface hydro-systems have been defined as socio-ecological systems (Brierley, 2020), and especially urban rivers (Zingraff-Hamed et al., 2020). The notion of socio-ecological systems was defined by Ostrom (2009) as a system composed of core elements interlinked and in constant interaction (Figure 1). Consequently, every single change within the system potentially positively or negatively affects other components of the system as well as their related functions. In response to the changes in one part of the system, other parts of the system adapt accordingly. Recent research on the application of the socio-ecological concept to river system showed
a close relationship and adaptation capacities between the core elements of the socio-ecological systems: governance, users, biodiversity, and global ecosystem processes (Zingraff-Hamed et al., 2019). Consequently, the riverine socio-ecological system is in constant adaptation and the multiple scales of change of system has challenged planners for more than a few decades. Two main theories emerged. While some authors as Ostrom (2009) argued that the stakeholders will self-organize and adapt their management strategy to reach a long-term resilience, other authors assume that guidance is necessary to accelerate social adaptation processes (Lupp et al., 2021b). Existing guidance includes: (1) maintain diversity and redundancy, (2) manage connectivity, (3) manage slow variables and feedbacks, (4) foster complex adaptive systems thinking, (5) encourage learning, (6) broaden participation, and (7) promote polycentric governance systems (Biggs et al., 2015).
Over-exploitation of the ecosystem services provided by rivers resulted in ecological impacts collectively referred to as the urban stream syndrome (Walsh et al., 2005) in the global North, a concept recently applied to the global South as well (Wantzen et al., 2019). Besides the decrease of ecosystem health, development of society on river systems directly impacts the potential delivery of future ecosystem services (Wantzen et al., 2016). While ecological response to hydro-morphological changes is well-documented in the literature (e.g., Belletti et al., 2020), limited knowledge about the mechanisms at the governance level exist (e.g., Carvalho et al., 2019). These also led to crucial changes within the socio-ecological system. In this paper, we understand river governance as the formal and informal exercises of authority or power from the social system (including the civil society) in order to manage river system, which is according to the definition provided by the UNDP, the World Bank, and the OECD Development Assistance Committee. For sustainability, the understanding of the processes that lead to improvements in or deterioration of hydro-systems is crucial. How did strategic planning and design adapt to changing societies and ecosystems? And how will we adapt to an increasingly changing system? In order to identify key factors of changes in the planning strategy and key challenges that need to be addressed, specifically in the case of urban river parks and waterfronts worldwide, we examine these issues in the context of two simplified examples: the (lower) American River, California, USA and the (upper) Isar River, Bavaria, Germany. The objective of this contribution is not only to revisit past events to help decision makers and practitioners reflect on the past but also to look at the future of our urban rivers. The analysis and results offer new insights and an improved understanding of the problem in adaptation faced by riverine areas in an urban context. The contribution does not intend to solve the very complex and real challenges faced by the case study sites but rather to bring to light research needs to support adaptation strategies.
Methods
Case Study Sites
The case study analysis is based on two gravel-bed rivers (Figure 2) with similar scenic features and hydrological regimes. Both have clear waters, large gravel bars, and quick and intense floods driven by both snowmelts and rainstorms. The American River has a lower average flow but much higher flood peaks (Table 1). Both rivers cross rapidly-growing major cities. All these aspects make our case study site highly comparable and representative of other urban mountainous or nival rivers as well as of some Mediterranean rivers.
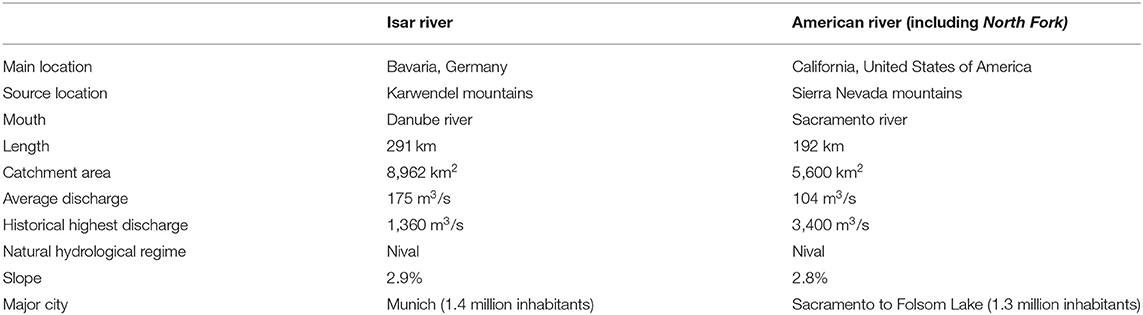
Table 1. River characteristics (data source: www.hnd.bayern.de and https://waterdata.usgs.gov/nwis).
Isar River
The Isar River (Figure 3) is a mountainous river with its headwaters in the Karwendel Mountains in the Alps (elevation 1,600 m) and flowing down to its confluence with the Danube River in a broad glacially-carved valley. The river's nival hydrological regime (Table 1), is characterized by snowmelt floods as well as short and violent rain-driven floods in the summer (Böhm and Wetzel, 2006). The large gravel bars of the Isar are valued for their aesthetic and cultural values (Im, 2011). The largest city crossed by the Isar is Munich, capital of the state of Bavaria, and one of the weathiest (in terms of GRP per capita) in Europe.
American River
The American River (Figure 4) has its headwaters in the high Sierra Nevada mountains (up to elevation 2,415 m) and flows downstream to its confluence with the Sacramento river at the City of Sacramento in the Central Valley of California. Of the three tributary forks that form the mainstem, the NorthMiddle, and South Forks (Table 1). The America River receives heavy recreational use, with rafting and kayaking popular in the upper mountainous reaches, and kayaking and canoeing in the lower reaches. The American River crosses the city of Sacramento, the capital of the U.S. state of California, whose economy would rank 5th in GDP globally if it were an independent country.
Analysis
To identify the drivers and challenges of urban river modification, the research was based principally on case study analysis, which provided a practice-oriented approach and an integrative review of publications in the peer-reviewed literature (as indexed in Scopus), as well as gray literature available online, and documentation of case study sites (including online publications of the public authorities, and blogs of the NGOs). The analysis focused on three main aspects: (1) the history of the hydrological, ecological, social and morphological changes of the river, (2) the driver of changes, and (3) the emerging and future challenges that are identified and that need to be addressed by strategic planning. In order to achieve the objectives of the contribution, a methodological approach was designed based on two core elements: an ex-post analysis of cases studies, and a comparative analysis (Figure 5).
Case Study Analysis
The case study analysis was based on an exhaustive literature review of the publications on the case study sites available online via the database Scopus and a non-exhaustive literature review of the gray literature on the case study sites found in the local library and online. Regarding the American River, searching for “American River” returned 673 documents published between 1857 and 2021. After selecting the appropriate subject areas (i.e., environmental, agricultural and biological, earth and planetary, engineering, and social sciences as well as multidisciplinary study) 540 publications remained. An automatic screening for keywords (N = 299) was followed by an expert-based screening of the titles (N = 104). While all these publications contributed to the case study analysis, to identify remaining and emerging challenges, we focused on publications from the last 10 years (N = 48). Searching for “Isar River” in Scopus returned 51 documents published between 1973 and 2021. After the same selection procedure, 33 publications remained. The relatively low number of peer-reviewed publication is compensated by more gray literature in German (N = 28). A total of 99 publications (48 for the American River and 51 for the Isar River) have been analyzed using the MaxQDA software for a qualitative content analysis.
Comparative Analysis
The comparative analysis focused on the case study sites in order to distinguish similarities and differences. Each case is unique in its setting, history and timeline. However, the comparative analysis identified periods with similar characteristic. It also enabled us to understand the issues and corresponding strategies in a wider context than that of the case study sites.
Results
The comparative analysis of the case study sites was done via a literature view focused on the historical evolution of the two systems. We identified four periods with similar trends, as described in this section.
Period 1: Balancing Risks and Advantages
The first period identified is characterized by both (a) an extensive exploitation of the ecosystem services, mostly water, food and wood supply, e.g., deer and large ungulates such as elk, salmon namely Chinook salmon Oncorhynchus tshawytscha on the American River and Danube salmon Hucho hucho on the Isar River), fruits and vegetables and (b) strategic city planning.
At the American River, the earlier settlers of the central valley, the Nisenan, established settlements of up to 500 inhabitants close enough to the river to benefit from water supply but high enough to avoid seasonal floods. In the wet winters they moved to nearby high ground to escape the annual floods (Hayes, 2005). The American River was “discovered” in 1808 by Spanish explorers, and farmers soon settled along the Sacramento rivers and its tributaries. They benefited from rich soil fertilized by floods for agriculture and an abundant supply of clean water. The first settlements, Sutter Fort (1839) at the mouth of the American River and “El Rancho del Paso” (1844), were built on slightly higher ground (Figure 6) as the builders were well aware of the possible damages caused by floods (Hayes, 2005).
The record of historic economic activities at the Isar is well-documented. As a natural route between Italy, the Alps and the Danube, the Isar River was used since prehistoric times as a trade route and the Isar hosted the largest known raft ports in Europe during the Bronze Age (Rädlinger et al., 2012). The main form of exploitation of ecosystem services was the production and downstream transport of wood timbers tied as rafts. Records of the municipal archive indicate that at the beginning of the nineteenth century more than 8,000 rafts landed in Munich each year. The flat timber rafts were designed to fit the hydro-morphology of the Isar, and allowed navigation with even a few centimeters of water with minimal river modification required to aid their navigation, e.g., navigating cascades (Neumann et al., 2011). At the Isar, urban development gave rise to the need for flood protection. However, inhabitants of the Isar valley mostly kept a safe distance from the river, being already familiar with the intensity of the floods (Schellman, 1990; Mallach, 1997; Scheuermann, 1998; Küster et al., 2011; Rädlinger et al., 2012). While most of the city stayed out above the level of frequent floods until the fourteenth century, development subsequently began outside of eastern city walls of Munich within the floodplain. A few flood protection structures were also built, especially at port cities such as Munich. However, the Isar flood plain was used for large recreational areas rather than dense urbanization (Döring and Binder, 2010). In 1789, the English Garden was designed. Stretching from the city center to the northeastern city limits, the English Garden is one of the world's largest urban public parks. In 1812, only one bridge crossed the Isar in Munich and the other cities remained on one side of the river (mostly east) as the water flooded westward.
Period 2: Overexploitations, Natural Hazards and Conflicts
The second period in both cases lasted around 30–50 years. It started with (a) a sudden increase of economical exploitation of a few ecosystem services, namely gold (in the Lower American River) and energy provision (in the Isar River) and (b) an increased effort to fight against the natural processes of the river, such as floods. With economic targets supported by technological advancements, both case study sites entered in a new phase characterized by massive hydro-morphological changes. This period ended with the first regulations to keep the conflict between exploiting riverine ecosystem services and other uses under control.
At the American River, the discovery of gold (1849) at the American River caused a radical turn in its history. Immigrants came from all parts of the world (Swindle, 2000) and worked in the mines, e.g., Mississippi bar (Figure 6) or Farmer's digging. Mining techniques caused massive hydrological modifications. Gold mines required large quantities of water to wash out the sediment and extract gold. In 1851, diversion of water dried out a section of the American River for dry digging on the one hand, and on the other hand, the Natoma Water Company built canals that brought water to the mines for the sluices (Morris and von Sayler, 2019). With the development of new techniques such as hydraulicking, the use of high pressured water jets to wash parts of mountains into sluices, the water consumption greatly increased. In 1871, 34,000,000 gallons of water per day were used to produce $32,000 worth of gold with a profit of around $12,000 over 100 days (Hayes, 2005). The canal system capacity increased rapidly to reach 4,420,000,000 gallons a day. Mining was the cause of emerging conflicts between the farmers cultivating the floodplain and the miners producing massive quantities of debris that caused a significant rise of the riverbed and threatened agricultural lands. Farmers sued, and in a landmark legal decision (the ‘Sawyer Decision’), hydraulic mining was limited and largely (though not entirely) stopped in 1884 (Kelley, 1959) (Figure 7).
At the Isar River, water power existed since medieval times in the form of small mills on secondary arms of the river (Rädlinger et al., 2012). In the late nineteenth century, Germany wanted to be energy independent and Alpine rivers were heavily developed for hydropower production (Bäumler, 1988). Between 1896 and 1930, 13 hydro-powerplants were built on the Isar River or on side canals requiring significant modification of the hydro-morphology (Figure 6; Neumann et al., 2011). In 1924, a massive water diversion canal was built in Krün to supply the Walchensee-Kochelsee hydropower plant, the largest high-pressure storage power plant in Germany and one of the world's biggest, with an annual energy production capacity of 124 Megawatt hours. For three decades, these water diversions caused the riverbed to be dry for most of the year (Karl et al., 1998; Döring and Binder, 2010; Küster et al., 2011; Zingraff-Hamed et al., 2021). In response to increasing concerns from conservationists and recreational users about the lack of water in the riverbed, in 1946, Article 141 was added to the Bavarian Constitution (Freistaat Bayern, 1946), outlining the obligation to protect the social function of nature, rivers and lakes for recreational usage.
Additionally, flood protection was the second driver of river modifications. At the American River, while Sutter advised the migrants to settle on slightly higher ground, such as Sutter Fort, most preferred the areas with access to the river, despite its greater flood risk. Miner and merchant settlements were flooded as early as 1850 (Hayes, 2005). After this first destructive flood, Samuel Norris suggested that the settlement of Sacramento be rebuilt in an area free of floods, but the residents preferred to build a levee system. The levee system broke and overflowed in 1852 and 1853 and citizens rebuilt and raised the levees repeatedly. Between December 1861 and January 1862 four destructive floods reached Sacramento and broke the levees each time. Facing the realities of flood risk, residents decided to excavate a new channel for the American River far from the city and to close the natural channel. Other parts of the river were straightened to increase flow velocity during floods. Another approach was raising the level of buildings. Over the course of 15 years, they raised the entire downtown area (streets and buildings) as much as 3 m using the excavated debris from the new channel. However, accelerated erosion upstream due to mining activities caused the level of the riverbed to rise within the levee system as the sediments were not able to be deposited in the floodplain. Furthermore, the flow velocity increase within the levee system increased the potential of breakages in the levee. Ultimately, Sacramento was flooded again in 1867, 1875, and 1878 (NRC, 1995).
At the Isar, while extensive flood protection worked so far, the flood of 1813 killed 100 people and caused the collapse of the oldest and most iconic bridge in Munich (Rädlinger et al., 2012). It was the starting point for a regional flood concept based on intensive channelization and regulation. People wanted the “ugly gravel bars” to disappear (Wiebeking, 1811, p. 26 in Karl et al., 1998). The riverscape changed. The river was regulated, riparian forest and pioneer meadows dried off and in urban areas such as in Munich, large grasslands were established for recreational activities. The regulation of the river increased urbanization in many smaller cities such as Landshut, Freising, Bad tölz, and Wolfrathausen. The urban area of Munich doubed from 1854 to 1932. In 1899 the Isar flood completely destroyed channelization features, weirs, the Maximilian hydropower plant that was built in 1895, and the two main bridges (Rädlinger et al., 2012). To respond to this intense flood, the city government together with the local water agency started a large scale regulation of the Isar (1900–1905).
Improving navigation was not a reason for major riverine morphological change. At the American River, navigation was only possible during floods as the water depth was not sufficient and the roads were well-developed early on. At the Isar, navigation practices were adapted to river hydrology. In both cases the railroad rapidly became the more reliable, faster and less expensive means of transportation instead of industrial transport by boat.
Period 3: Intense Urbanization, Flood Protection and Energy Provision
The third period is characterized in both cases by intense urbanization except for some important parts of the riverine corridor as the flood risk was not fully “under control.” Riverine areas were further developed for recreation. During this period, dams were built to address hydro-meteorological hazard and by the end of this period, floods were considered as a contained risk. At the end of the Second World War (WWII), European and U.S. populations experienced both a demographic boom with a very high birthrate and lower mortality, along with migration from rural to urban areas. While in both cases, the depletion of green spaces was massive, low-lying area adjacent to the rivers were largely avoided due the occurrence of floods.
The American River became the land promoter's paradise. The San Juan Grant resulted in three main plots of land dedicated to new settlements along the American River: Orange Vale, Fair Oaks, and Carmichael (Hayes, 2005). After the Great Depression, World War One and World War Two, the population largely increased which led to the conversion of agricultural land into cities. Besides the expansion of the existing settlement, new settlements were created: River Park, Rancho Cordova, College Green, and Campus Commons. For example, in Rancho Cordova the population increased from essentially 0 to 28,500 inhabitants between 1950 and 1965 (Hayes, 2005). Farmers, business groups and bicycle groups united to request improvements to the road and bridge system. Downstream of the River Park and to the west, the Sacramento River Flood Control Project was developed in the early 20th century, featuring the Sutter and Yolo Bypasses, wide areas of floodplain set aside to convey the majority of flow during floods, now essential components for the flood management system (Serra-Llobet et al., 2018, 2022). Notably, the Yolo Bypass reduced flood risk for the City of Sacramento by diverting up to 14,000 m3/s of the Sacramento River flow, four times the capacity of the mainstem river channel as it passes Sacramento (Serra-Llobet et al., 2021). Existing levees were raised and more dams were built in the Sacramento River Basin. “By the middle of the twentieth century, what had begun as a disjointed assemblage of local levees became the State Plan of Flood Control (SPFC)” (Serra-Llobet et al., 2018). Folsom Dam was built on the American River from 1951 to 1955 at a cost of $29.5 million for the dam and $4.2 million for its hydroelectric powerhouse, which produces nearly 700 million kilowatt hours annually (Williams, 1993; Hayes, 2005). To even out flow fluctuations caused by hydropeaking releases from Folsom Dam, a second dam (Nimbus) was built downstream (Figure 6), and its impoundment is used to divert water to the Folsom South Canal, a 45-km-long aqueduct transferring water to agricultural areas to the south. In 1955 Sacramento narrowly escaped a large flood thanks to newly completed Folsom Dam and its flood storage capacity of 1.341 km3, preventing an estimated $20 million in flood damages.
The 1964 and 1986 floods exceeded the reservoir capacity and releases from the dam flooded low-lying neighborhoods (Peterson, 1993). In 1995, a broken spillway also caused flooding downstream (NRC 1995). In 2004 the Folsom Dam was raised by seven feet (Mishra et al., 2008). By 2005, the Sacramento Area Flood Control Agency had raised and strengthened about 85% of the levees at the lower American River. The flood risk was estimated to be fully contained. However, alterations to channel and floodplain geomorphology and to the flow regime resulted in impacts to anadromous fish and other native species (e.g., Anderson et al., 2006; Graf, 2006; Cox et al., 2008; Burke et al., 2009). Populations of adult, salmon (mostly chinook) decreased from 130,000 to 26,500 and ultimately to 11,000 from 1950 to 1990, respectively. To compensate for dam impacts on salmon, an artificial hatchery was built adjacent to Nimbus Dam in 1955. Soon after, five smaller hydropower plants were built on the Middle Fork and 11 on the South Fork. The sense of safety from floods created by the Yolo Bypass, Folsom Dam, and levees along the Lower American River encouraged developments in the floodplains, where houses were still at risk from floods that exceed the capacity of the structural measures to control flooding, a coupled human-natural interaction termed the “levee effect” (White et al., 2001).
In Europe, during WWII, a massive migration from rural to urban areas took place and Munich's population reached almost 800,000 inhabitants. During the U.S. bombings in 1945, Munich lost 34% of its population and 45% of its buildings (Rädlinger et al., 2012). While many German cities used this as an opportunity to change the whole city plan and include major parks and natural areas in city planning (Zingraff-Hamed et al., 2017b), Munich decided to rebuild the city in the same way as before. Munich's strong industrial sector (e.g., Siemens and BMW, after World War II) helped to propel the city's population to over 1 million by 1957, both from expansion of the urban area but also densification.
At the Isar, the Sylvenstein Dam was built between 1954 and 1959 around 75 km upstream of Munich (Bäumler, 1988) flooding the village of Fall to store ~125 million cubic meters of water. One hundred twenty-two residents were relocated (Zingraff-Hamed and Egger, 2019). The reservoir was designed as a fjord-like lake fitting into the mountain landscape. It stores snowmelt and rainwater and delivers a constant year-round minimum flow through two small hydroelectric power plants with a 3.8 megawatt hour capacity (Goeb and Wirth, 1986). In 1990, the number of hydropower plants on the Isar reached 30. Old infrastructures were also updated. For example, the Walchensee-Kochelsee hydropower plant's mean annual hydroelectric production increased from 124 to 300 gigawatt hours. In response to increasing flood magnitudes from climate change the dam was raised by 3 m in 2001, and a relief tunnel built to reduce the bottleneck for flows going into the bottom outlet during a potential 1,000-year flood (Zingraff-Hamed et al., 2021). Today, the dam is 44 m high and 180 m long. The Loisach River, has a strong influence on flood flows (Schneeberger et al., 2021) while other tributaries are now controlled by impoundments.
Period 4: Increase of Environmental Concerns and Water Sharing
The fourth period does not have a clear timeframe, as its early stages started slowly as a response to the mismanagement observed in the second and third periods. The fourth and current stage is characterized by increasing influence of environmental legislation and environmental advocacy groups Environmental concerns grew in the third part of the twentieth century at both case study sites. On the American River, results of this new approach included in the establishment of the American River Parkway. While the concepts underlying the American River Parkway started taking shape in 1915, the implementation was possible only after the passing of the State Park Bond Act (1929). The parkway was designed by Frederick Law Olmsted Jr. in 1947 for the remaining floodplain, or the areas within the levee system. As most of the floodplain was under private ownership but still free of housing, the city council voted for the acquisition of 82 acres of land in 1950. While most of the land was donated, the city bought seven acres for $200,000 from the State Park Commission (Hayes, 2005). The objective was to create a park for recreational activities, especially since the completion of Folsom Dam in 1955 increased the pressure of urbanization on the floodplain. The park design benefited the civil society and recreationists. To build a parkway from Folsom Dam to the Sacramento River, further land acquisition was required. However, lack of funding delayed these acquisitions. In 1961, the Save the American River Association (SARA) started to actively inform the population and gain societal support for the project. It resulted in more funding from the Sacramento County Board of Supervisors, which voted for additional land acquisition for the Parkway in 1962, and a $12.6 million bond in 1972 (Hayes, 2005). The Natoma Company, a major landowner in the area, was an important supporter of the project. The parkway consisted of many smaller parks connected by bikeways and included boat launching points. In recognition of its recreational values, the lower American River has been designated a “recreational river” under both the California Wild and Scenic Rivers Act and the National Wild and Scenic Rivers Act. Since the 1950s, the Sacramento region has been torn by competition between water demand for agriculture and increasing municipal use, and the need for flows to support fish and other wildlife. This conflict was accentuated by droughts. In 1969, the Save the American River Association together with other NGOs successfully argued for higher minimal flows to benefit fish habitat and for recreational use. The California State Water Resources Control Board set higher minimum flows for most years with the expectation that storage in the basin would be increased by construction of the proposed Auburn Dam, but this dam was abandoned after an earthquake demonstrated that an active fault traversed the damsite (Duffield, 1980). In 1993, the Regional Water Forum was created to promote collaboration between the city of Sacramento, the County Office of Water Planning, environmental groups, business, building, agricultural and water districts, and community groups. In 1995, the Water Forum grew to include water managers in Placer and El Dorado Counties. Its two equally important objectives are to secure safe water supply for the region's economic health and to preserve fish, wildlife, recreation, and the landscape scenery of the American River. The enactment in 1968 of the Flood Insurance Act gave a tool to counties and cities to prevent further developments in high hazard areas through the implementation of the National Flood Insurance Program (NFIP). The city of Sacramento joined the NFIP in 1978 (National Academy of Sciences, 2013) and classified the American River Parkway as a “Regulatory Floodway,” an area of higher conveyance of water where developments are highly discouraged (although not totally forbidden in private properties). However, the issues over private property rights and public safety remain contested in the US (Klein, 2019).
In Europe, environmental concerns developed in the late 1960s and early 1970s and induced major changes in governance of rivers (Zingraff-Hamed et al., 2017a). Many EU Directives had been ratified to support the protection, conservation and restoration of hydrological systems, e.g., the EU Bathing Directive, the Water Framework Directive, the Nitrate Directive, and the Birds and Habitat Directive. The changes all happened at the river governance level. For instance, in 1970, the Bavarian State Ministry for the Environment and the Environmental Protection Department of Munich were created (Bäumler, 2019). In the early 1980s, public outcry in Bavaria about the extremely poor ecological condition and water quality of most of Germany's rivers became very strong. In 1984, Article 141 of the constitution was modified to define environmental and natural protection as a constitutional goal of the State. In 1987, the Bavarian water law was also changed to provide minimum flows in rivers. In 1993 the Isar Allianz, an advocacy group of NGOs of recreational users and environmental protection at the Isar was created (Sartori, 2010; Winiwarter et al., 2016). It demanded the restoration of the Isar at different locations, e.g., Mühltal and Munich. The restoration of the Isar River, labeled “New Life for the River Isar,” was implemented between 2000 and 2011 by the Bavarian Water Management Agency in Munich and the City of Munich. The 35 million Euro project targeted large riverine green spaces for recreational use and a high quality flood protection that supported habitat restoration. The Isar River restoration became an example of a nature-based solution with high quality standards established by civil society (RESTORE, 2013; Kiss, 2018).
New Challenges
Future challenges for the American River Parkway and the restored section of the Isar River in Munich have been identified through reviewing the case-specific publications produced in the last 10 years. Two main topics can be underscored: hydro-meteorological extremes and ecological crisis.
First, floods and droughts are natural events in mountain rivers but changing weather patterns have led to increased extremes. However, flood protective measures such as dikes and reservoirs are static engineering elements, whose rigidity precludes most adaptation capacity. As flood managers revise their estimates of the magnitude of design floods, such as the centennial flood, to account for climate change effects, there is an increasing need for solutions with greater adaptability to climate change. The urgent needs are in estimating maximum precipitation and resultant extreme runoff from the watershed (Mann et al., 2012; Ohara et al., 2012, 2017; Ishida et al., 2013, 2017; Schneeberger et al., 2021), as well as in estimating the effect of climate change on extreme precipitation within the catchment area (Jobst et al., 2012; Ishida et al., 2015; Wagner et al., 2017; Sultana and Choi, 2018). Research is also needed in high risk areas within downstream flood hazard zones (Kalyanapu et al., 2013). In particular, there is an urgent need for developing suitable mitigation and adaptation options for a more resilient urban infrastructure and to adapt to the identified growing hydro-meteorological risk. Dams and reservoir capacities are also being investigated (Yigzaw et al., 2013; Newman et al., 2014) as data used to design these infrastructures were from historical discharge or meteorological records without integrating changes in land-atmospheric and hydrological processes as a result of land-cover modification. Another source of concern is the sediment storage within the dam and within the levee system, which reduces the performance of the flood defense (Pender et al., 2014). Risks from drought are also a major concern at both case study sites (Lepley et al., 2020). Drought related wildfires damaged over 11 percent of the American River Parkway in 2021. Moreover, legitimacy of water rights have been questioned and more intense conflict for water may destabilize the water governance constellation (Schwarz, 2015). Dams have also been pointed out as source of ecological degradation.
Also, ecological degradation linked to the urban pressure is a core challenge for the urban river parks since the second period. Much of the current attention is focused on conflicts among uses, e.g., energy production, recreation, and nature conservation. The modifications of the river flow caused by upstream regulation by dams and water use (agriculture and power generation) have been thoroughly studied. In particular, the impacts of flow regulation on fisheries induced by hydro-electric production falls into this category (Cocherell et al., 2010, 2011; Sogard et al., 2012; Georgakakos et al., 2014). In this context, scientists call for flow hydrographs to the resemble more natural hydrological patterns. Most research has focused on economically relevant species like rainbow trout (Oncorhynchus mykiss) (Cocherell et al., 2010, 2011) and protected species (Hansen et al., 2015; Zingraff-Hamed et al., 2018a). These serve as examples to illustrate a problem that impacts the whole ecosystem and all the species included in it. Overall, an integrative assessment at the scale of the ecosystem and over the whole course of the river should be carried out to effectively support the decision-making. Even minimal alterations such as artificial daily pulse flows (e.g., for hydropeaking) may impact many species (Cocherell et al., 2010, 2011). Not only the minimal discharges but also the higher water temperature is a source of concern (Martinez et al., 2014). While increasing flow releases has been identified as a possible solution, this does not always solve the problem of too-high water temperatures and there may be too little water available. Other impacts of the dam on ecological processes include the trapping of sediments behind the dam, which can result in incision in the channel downstream, and reductions in flow downstream, with potentially severe ecological consequences (Wisheropp, 2018; Gabet and Miggins, 2020). Dams also influence the regional landscape through alterations in land use after the dam was built, which can influence microclimate and weather patterns (Woldemichael et al., 2012, 2014a,b). Finally, the impact of recreation on the ecological system is also a source of concern for the American River Parkway Foundation and the Green Space Management Department of the city of Munich and has also been partly investigated (Zingraff-Hamed et al., 2018b, 2022). In particular, the press and advocacy groups have denounced plastic litter from recreational users.
Discussion
The ex-post analysis of the case study sites show that urban rivers and related parks evolve within an uncertain process that occurs in a large context of human demographic, technological, economic and ethical changes. We found and described in the result sections, four main evolutionary periods characterized by different interests and challenges (Figures 6, 7). These are real-live illustrations of the constant evolutions of the socio-ecological system that were described first at the theoretical level by Ostrom (2009). Our study shows that the adaptation to societal and environmental changes requires major shifts in the focus of a decision-making processes at the intersections of three components: values, rules, and knowledge.
The driver of changes i further considered using the Value-Rules-Knowledge Framework developed by Gorddard et al. (2016). The Values-Rules-Knowledge (VRK) Framework is an established method to analyze the context of decisions. The approach considers three aspects influencing the decision-making process: (1) knowledge that includes scientific and technical theories, facts and information as well as tacit traditional and experiential knowledge; (2) values that are preferences and ethical considerations, such as principles that inform the preferences; and (3) rules governing the choice of management or design. The values, rules and knowledge can intersect at different levels. The balance within the value-knowledge-rules nexus differs between the periods identified (Figure 8).
The first period (Figures 6, 7) is characterized by a decision-making process based on traditional knowledge and values (Figure 8). Rather than technical knowledge, inherent experiential knowledge of the natural processes were associated with a higher value for human life and development of society to inform decision-making process. As a result, human settlements were preferentially located at slightly higher elevations, above most floods. The emerging importance of economic activity in the second period caused a change in the values, namely in the system of preferences and ethics. This encouraged the advancement of technical knowledge with the development of new procedures and infrastructure to intensively exploit a few ecosystem services with a high economical value (gold and energy). This shift caused a rupture between the socio-ecological values of the river system and led to ecosystem collapse (Hardin, 1968). New governance mechanisms came into play in the form of rules. As one of the three mechanisms to manage environmental issues at the governance level (strategic planning, education to increase value and knowledge, and regulation), regulation can be viewed as an emergency fix for past mismanagement (Zingraff-Hamed et al., 2018b). The third period was characterized by the increase of legislative support for a more sustainable development. This was accompanied by increased value placed on the environment by the public. The advocacy groups that demanded for more sustainable management of natural resources started to reestablish environmental values within the decision-making process and caused a shift to the fourth period. According to the Value-Rules-Knowledge Framework, the position represented by the intersection between the three core elements of the system is best suited to achieve adaptive management (Gorddard et al., 2016). Viewed in the framework of the socio-ecological system, Ostrom (2009) argued that the socio-ecological system tends to evolve toward a long-term balance between the ecological and the social. The case studies illustrate implementation of a more sustainable management of the urban river park through a circular evolution within the Value-Rules-Knowledge Framework. Initially, the river management relied mostly on traditional knowledge and values, then it became reliant on technical knowledge, then on stronger environmental rules, and recently on more environmental values. The importance of changing social values in the management of ecologicaly between the first and the second period is illustrated on the Lower American River by the confrontation of two cosmovisions of socio-ecological systems. Native Americans view nature through their belief systems, believing that “a river or water not only sustains life—it is sacred” (LaPier, 2017). For some, floods were seen as “acts of God” (White, 1945), for others, floods were regarded as an example of the unavoidable forces of nature and as such can be little controlled by humans (the deterministic view). However, the Gold Rush not only transformed the landscape, but also the demography of California, imposing Western attitudes toward the natural world which were shaped by different views of nature (Glacken, 1967). In the second and third periods, flood control became the new paradigm, consistent with the dominant view of human control over the environment (the anthropocentric view). The shift of social values toward a more environmental ethic in period 4 has been described in the literature, especially with respect to how people think about the environment (Mascia et al., 2003; Ives and Kendal, 2014). Further research is needed on how environmental outcomes depend on socio-political factors and how facts and knowledge influence the socio-political component of the decision making process. Indeed, the discipline of environmental management has been dominated by natural sciences, with less input from the social sciences, but to achieve sustainable development goals, the social component must be integrated into the thinking process as much as the ecological components (e.g., Chua et al., 2020).
The current decision-making process with the intersection of the three aspects of the Value-Rules-Knowledge will face emerging challenges. Almost all the research produced the last 10 years about our case study sites point out issues related to climate change, lost biodiversity, urban pressure, and some authors have challenged the continued existence of the dams. While the removals of dams in Europe and in the United States continue to accelerate because of demand for ecological restoration and for economic reasons (Grabowski et al., 2018), the dams on the American and the Isar Rivers perform important functions (e.g., flood protection, power generation and water supply) and are not likely candidates for removal anytime soon, despite the dams' evident impacts and rising ecological concerns. As in the second period, we are facing a situation in which economical value surpasses ecological value. Furthermore, the high level of urbanization of the floodplain after the construction of the dams makes it difficult to remove the dams and reestablish the flow of the river while protecting the people and their communities. However, some of examples of multi-benefit projects like nature based-solutions to reduce flood risk exist, such as the Bear River levee setback in California, but they are still rare (Serra-Llobet et al., 2022). The limitations of flood protection from dams and reservoirs are well-established in the scientific community. Most reservoirs are trapping sediment, which displaces water storage and reduces reservoir capacity (Randle et al., 2021). In addition, climate change induced increases in extreme weather events, making it more likely that storage capacities in some dams will be overwhelmed in the near future. For both the American and Isar River catchments, studies in both case study sites estimate an increase of extreme rain events (Ishida et al., 2015; Wagner et al., 2017). In 2001 the height of the Sylvenstein Dam was increased, and the Folsom Dam was also raised in 2007. To adapt to the growing hydro-meteorological risk, Folsom Dam is now also equipped with an auxiliary spillway.
Balancing the seasonal effects of climate change presents in both cases another challenge within the catchment. Both winter flooding and low flows frequency are projected to become more extreme (Sultana and Choi, 2018). As more precipitation falls as rain instead of snow, reduced snowmelt runoff in the summer will directly reduce power generation, while water and power demands will peak under most climate projections (Vicuña et al., 2011). Agriculture faces droping groundwater levels resulting from drainage and soil impermeabilization as well as greater competition with cities for water supplies. Strategies to keep rainwater in the soil and adaptation of agricultural practices will be need. However, the implementation of nature-based solutions [multi-benefit (social and ecological) projects] is slowed down by the limited knowledge regarding their effectiveness and lack of familiarity with many of the techniques on the part of decision makers (Lupp et al., 2021a; Solheim et al., 2021; Raška et al., 2022). To insure the central position within the Value-Rules-Knowledge framework, knowledge should be generated to support implementation. A decision context that is no longer effective may exclude potentially useful options such as nature-based solutions due to the fact that decision-makers do not consider them credible, legitimate, or important. Further research should explore the viability of such options to provide the decision-making process with robust knowledge and avoid a further shift to a rules-values based decision process.
Our investigation of the historical and emerging drivers of the design and management of urban river parks on the Isar River in Munich and the American River in Sacramento point to four distinct evolutionary periods driven by changing demographic, technological, economic and ethical settings. Most of the older cities of the world originated along rivers and because of economic advantages. We have “conquered” the wild and abundant rivers and we frequent them within parkways like we visit animals kept in a zoo (Hayes, 2005). Many cities have made the situation worse than the city of Sacramento and of Munich. Some have restricted rivers to a concrete channels as in the case of the city of Paris (France) or put them in underground tunnels as in the case of the city of Montréal (Canada). Sacramento and Munich try to at least preserve their rivers by setting aside parkways with crucial flood protection functions, ambitious wildlife heritage conservation, and increasing recreational use. But both cities still have more work to do. Water agencies, local authorities, NGOs and civil society have to stay vigilant (Hayes, 2005). Major challenges remain to be addressed: climate change, the biodiversity crisis, and urban pressure in floodplains. Decision-making processes should balance values, knowledge, and rules to achieve long-term resilience.
Data Availability Statement
The original contributions presented in the study are included in the article/supplementary material, further inquiries can be directed to the corresponding author.
Author Contributions
AZ-H: conceptualization, data curation, funding acquisition, methodology, resources, and writing original draft. AZ-H, AS-L, and GK: formal analysis, investigation, and validation, revision and editing. AZ-H and AS-L: visualization. All authors have read and agreed to the published version of the manuscript.
Funding
AZ-H has received funding from the European Union's Horizon 2020 research and innovation programme under grant agreement No. 776681. The research of AS-L was supported by the INRAE Post AgreenSkills Fund.
Conflict of Interest
The authors declare that the research was conducted in the absence of any commercial or financial relationships that could be construed as a potential conflict of interest.
The handling editor TR declared a shared parent affiliation with the author AZ-H at the time of review.
Publisher's Note
All claims expressed in this article are solely those of the authors and do not necessarily represent those of their affiliated organizations, or those of the publisher, the editors and the reviewers. Any product that may be evaluated in this article, or claim that may be made by its manufacturer, is not guaranteed or endorsed by the publisher.
Acknowledgments
The authors would like to thank their colleagues for their support and especially Joshua Huang for editing the paper and Marcelian Grace for helping designing some illustrations. AZ-H address best thanks to her colleague, Dr. Gerd Lupp and Prof. Pauleit and especially her project coordinator, Dr. Amy Oen for their support.
References
Anderson, E. P., Freeman, M. C., and Pringle, C. M. (2006). Ecological consequences of hydropower development in Central America: impacts of small dams and water diversion on neotropical stream fish assemblages. River Res. Applic. 22, 397–411. doi: 10.1002/rra.899
Bäumler, K. (1988). Heilige Kühe für Die Isar anmelken. Materialien Zur Novelle des Bayerischen Wassergesetzes 1987. Munich: Gemeinsamer Arbeitskreis Isar der CSU-Kreisverbände Freising, Schwabing und München Land.
Belletti, B., Garcia de Leaniz, C., Jones, J., Bizzi, S., Börger, L., Segura, G., et al. (2020). More than one million barriers fragment Europe's rivers. Nature 588, 436–441. doi: 10.1038/s41586-020-3005-2
Biggs, R., Schlüter, M., and Schoon, M. (2015). Principles for Building Resilience: Sustaining Ecosystem Services in Social-Ecological Systems. Cambridge: Cambridge University Press. doi: 10.1017/CBO9781316014240
Böhm, O., and Wetzel, K. F. (2006). Flood history of the danube tributaries lech and isar in the alpine foreland of Germany. Hydrol. Sci. J. 51, 784–798. doi: 10.1623/hysj.51.5.784
Brierley, G. J. (2020). “The socio-ecological river: socio-economic, cultural and environmental relations to river systems,” in Finding the Voice of the River, ed P. Pivot (Cham: Springer), 29–60. doi: 10.1007/978-3-030-27068-1_2
Burke, M., Jorde, K., and Buffington, J. M. (2009). Application of a hierarchical framework for assessing environmental impacts of dam operation: Changes in streamflow, bed mobility and recruitment of riparian trees in a western North American river. J. Environ. Manage. 90 (Suppl. 3), S224–S236. doi: 10.1016/j.jenvman.2008.07.022
Carvalho, L., Mackay, E. B., Cardoso, A. C., Baattrup-Pedersen, A., Birk, S., Blackstock, K. L., et al. (2019). Protecting and restoring Europe's waters: an analysis of the future development needs of the water framework directive. Sci. Total Environ. 658, 1228–1238. doi: 10.1016/j.scitotenv.2018.12.255
Chua, L., Harrison, M. E., Fair, H., Milne, S., Palmer, A., Rubis, J., et al. (2020). Conservation and the social sciences: beyond critique and co-optation. A case study from orangutan conservation. People Nat. 2, 42–60. doi: 10.1002/pan3.10072
Cocherell, S. A., Cocherell, D. E., Jones, G. J., Miranda, J. B., Thompson, L. C., Cech, J. J. Jr., et al. (2011). Rainbow trout Oncorhynchus mykiss energetic responsesto pulsed flows in the American River, California, assessed by electromyogram telemetry. Environ. Biol. Fishes 90, 29–41. doi: 10.1007/s10641-010-9714-x
Cocherell, S. A., Jones, G. J., Miranda, J. B., Cocherell, D. E., Cech, J. J. Jr., Thompson, L. C., et al. (2010). Distribution and movement of domestic rainbow trout, Oncorhynchus mykiss, during pulsed flows in the South Fork American River, California. Environ. Biol. Fishes 89, 105–116. doi: 10.1007/s10641-010-9701-2
Cox, N., Huff, H., Rahmeyer, W., Barfuss, S., Mifkovic, C., and Falvey, H. T. (2008). Model study of Submerged Tainter Gate Structure for Auxiliary Spillway at Folsom Dam C3–Association of Dam Safety Officials - Dam Safety 2008. Honolulu, HI: American Society of Civil Engineers.
Döring, N., and Binder, W. (2010). Die neue Isar/1-Renaturierung, Kulturelle Öffnung und Ideen-Fluß, Geschichtliches wie Literarisches. Munich: Buch & Media.
Duffield, J. W. (1980). Auburn Dam: a case study of Water polica and economics. JAWRA 16, 226–234. doi: 10.1111/j.1752-1688.1980.tb02383.x
Freistaat Bayern (1946). “Verfassung des freistaates bayern,” in BayVerf, ed F. Bayern (Munich: Freistaat Bayern).
Gabet, E. J., and Miggins, D. P. (2020). Minimal net incision of the northern Sierra Nevada (California, USA) since the eocene-early oligocene. Geology 48, 1023–1027. doi: 10.1130/G47902.1
Georgakakos, A. P., Yao, H., and Georgakakos, K. P. (2014). Ensemble streamflow prediction adjustment for upstream water use and regulation. J. Hydrol. 519, 2952–2966. doi: 10.1016/j.jhydrol.2014.06.044
Glacken, C. J. (1967). Traces on the Rhodian Shore. Berkeley, CA: University of California Press. doi: 10.1525/9780520343054
Goeb, H., and Wirth, H. (1986). Increasing the flow rate of the Isar - an additional measure to improve river water quality. GWF 127, 234–238.
Gorddard, R., Colloff, M. J., Wise, R. M., Ware, D., and Dunlop, M. (2016). Values, rules and knowledge: Adaptation as change in the decision context. Environ. Sci. Policy 57, 60–69. doi: 10.1016/j.envsci.2015.12.004
Grabowski, Z. J., Chang, H., and Granek, E. F. (2018). Fracturing dams, fractured data: empirical trends and characteristics of existing and removed dams in the United States. River Res. Applic. 34, 526–537. doi: 10.1002/rra.3283
Graf, W. L. (2006). Downstream hydrologic and geomorphic effects of large dams on American rivers. Geomorphology 79, 336–360. doi: 10.1016/j.geomorph.2006.06.022
Hansen, E. C., Scherer, R. D., White, G. C., Dickson, B. G., and Fleishman, E. (2015). Estimates of survival probability from two populations of giant gartersnakes in California's great central Valley. Copeia 103, 1026–1036. doi: 10.1643/CE-15-233
Hardin, G. (1968). The tragedy of the commons. Science 162, 1243–1248. doi: 10.1126/science.162.3859.1243
Hayes, P. J. (2005). The Lower American River. Prehistory to Parkway. Carmichael, CA: American River Natural History Association.
Ishida, K., Jang, S., Ohara, N., Kure, S., and Kavvas, M. L. (2013). “Physically based maximization of precipitation over American river watershed in California C3,” in World Environmental and Water Resources Congress 2013: Showcasing the Future—Proceedings of the 2013 Congress (Cincinnati, OH), 1198–1201. doi: 10.1061/9780784412947.117
Ishida, K., Kavvas, M. L., Ceyhan, S., Ohara, N., and Chen, Z. Q. (2015). “Climate change analysis on extreme precipitation over three watersheds in Northern California based on 137-year long-term dynamical downscaling C3,” in World Environmental and Water Resources Congress 2015: Floods, Droughts, and Ecosystems - Proceedings of the 2015 World Environmental and Water Resources Congress (Austin, TX), 983–988. doi: 10.1061/9780784479162.094
Ishida, K., Kavvas, M. L., and Chen, Z. Q. (2017). “Maximum precipitation estimation over American river watershed in Northern California under future climate conditions C3,” in World Environmental and Water Resources Congress 2017: Groundwater, Sustainability, and Hydro-Climate/Climate Change - Selected Papers from the World Environmental and Water Resources Congress 2017 (Buenos Aires), 367–373. doi: 10.1061/9780784480618.036
Ives, C. D., and Kendal, D. (2014). The role of social values in the management of ecological systems. J. Environ. Manage. 144, 67–72. doi: 10.1016/j.jenvman.2014.05.013
Jobst, A., Schmid, J., Ludwig, R., and Muerth, M. (2012). Impacts of Climate Change on the Water Management System on the Alpine Upper Isar River With Regards to Hydro Power Potential and Residual Flow. Vienna: EGU General Assembly.
Kalyanapu, A. J., Azad Hossain, A. K. M., Kim, J., Yigzaw, W., Hossain, F., and Shum, C. K. (2013). Toward a methodology to investigate the downstream flood hazards on the American river due to changes in probable maximum flood due to effects of artificial reservoir size and land-use/ land-cover patterns. Earth Interact. 17, 24. doi: 10.1175/2012EI000496.1
Karl, J., Binder, W., Gröbmaier, W., Hausmann, A., Hebauer, F., Jürging, P., et al. (1998). Die Isar - Ein Gebirgsfluß im Wandel der Zeiten. Munich: Verein zum Schutz der Bergwelt e.V. München.
Kelley, R. L. (1959). Gold vs grain: the hydraulic mining controversy in California's Sacramento Valley. Glendale, CA: AH Clark Co.
Kiss, B. (2018). Snapshot—Munich: The Restoration of the Isar River. 4. Available online at: https://naturvation.eu/sites/default/files/munich_snapshot.pdf (accessed March 17, 2019).
Klein, C. A. (2019). The national flood insurance program at fifty: how the fifth amendment takings doctrine skews federal flood policy. Geo. Environ. Law Rev. 31, 285. Available online at: https://scholarship.law.u?edu/facultypub/868/
Küster, H., von den Driesch, A., Tockner, C., Bill, H. C., Müller, N., Riegel, G., et al. (2011). Flusslandschaften im Wandel: Veränderung und weitere Entwicklung von Wildflusslandschaften am Beispiel des alpenbürtigen Lechs und der Isar Laufen/Salzach. Augsburg: Bayerische Akademie für Naturschutz und Landschaftspflege.
LaPier, R. R. (2017). Indigenous People Would Add, a River is More Than a “Person”—it is Also a Sacred Place. Available online at: https://theconversation.com/for-native-americans-a-river-is-more-than-a-person-it-is-also-a-sacred-place-85302 (accessed October 9, 2017).
Lepley, K., Touchan, R., Meko, D., Shamir, E., Graham, R., and Falk, D. (2020). A multi-century Sierra Nevada snowpack reconstruction modeled using upper-elevation coniferous tree rings (California, USA). Holocene 30, 1266–1278. doi: 10.1177/0959683620919972
Lupp, G., Huang, J. J., Zingraff-Hamed, A., Oen, A., Del Sepia, N., Martinelli, A., et al. (2021a). Stakeholder perceptions of nature-based solutions and their collaborative co-design and implementation processes in rural mountain areas—a case study from PHUSICOS. Front. Environ. Sci. 9, 678446. doi: 10.3389/fenvs.2021.678446
Lupp, G., Zingraff-Hamed, A., Huang, J. J., Oen, A., and Pauleit, S. (2021b). Living Labs—A Concept for Co-Designing Nature-Based Solutions. Sustainability 13(188). doi: 10.3390/su13010188
Mallach, N. (1997). Die Isar - Problemfluß oder Lösungsmodell? Laufen/Salzach: Bayerische Akademie für Naturschutz und Landschaftspflege.
Mann, H., Kavvas, M. L., Jang, S., Ohara, N., and Kure, S. (2012). Validation of extreme precipitation reconstructed by dynamical downscaling for the Upper Feather, Yuba, and American watersheds C3,” in World Environmental and Water Resources Congress 2012: Crossing Boundaries, Proceedings of the 2012 Congress (Albuquerque, NM), 2030–2035. doi: 10.1061/9780784412312.204
Martinez, V. I., Wells, S. A., and Addley, R. C. (2014). “Meeting temperature requirements for fisheries downstream of folsom reservoir, California C3,” in World Environmental and Water Resources Congress 2014: Water Without Borders - Proceedings of the 2014 World Environmental and Water Resources Congress (Portland, OR), 1081–1092. doi: 10.1061/9780784413548.108
Mascia, M. B., Brosius, J. P., Dobson, T. A., Forbes, B. C., Horowitz, L., McKean, M. A., et al. (2003). Conservation and the social sciences. Conserv. Biol. 17, 649–650. doi: 10.1046/j.1523-1739.2003.01738.x
Mishra, S., Barfuss, S. L., Rahmeyer, W. J., Huff, H., Smith, T., and Cox, N. (2008). “The Folsom Dam auxiliary spillway physical model study as a design tool C3,” in World Environmental and Water Resources Congress 2008: Ahupua'a - Proceedings of the World Environmental and Water Resources Congress 2008 (Honolulu, HI), 316. doi: 10.1061/40976(316)247
Morris, E., and von Sayler, S. (2019). Water Gold Soil—The American River. Berkeley, CA: Conveyor Editions.
National Academy of Sciences (2013). Levees and the National Flood Insurance Program: Improving Policies and Practices. Washignton, DC: National Research Council of the National Academies, The National Academy Press, 332.
Neumann, A., Gabel, G., Gröbmaier, W., Kolbinger, A., Kraier, W., Krolo, M., et al. (2011). Flusslandschaf Isar im Wandel der Zeit. Augsburg: Bayerisches Landesamt für Umwelt.
Newman, E., Forrest, M., Jaramillo, C., Kegel, K., and Wisniewski, J. (2014). “Rock mechanics considerations in the design of folsom auxiliary spillway approach channel C3,” in 48th US Rock Mechanics/Geomechanics Symposium 2014 (Minneapolis, MN), 1790–1796.
Ohara, N., Kavvas, M. L., Anderson, M. L., Chen, Z. Q., and Ishida, K. (2017). Characterization of extreme storm events using a numerical model-based precipitation maximization procedure in the feather, Yuba, and American river watersheds in California. J. Hydrometeorol. 18, 1413–1423. doi: 10.1175/JHM-D-15-0232.1
Ohara, N., Kavvas, M. L., Kure, S., Chen, Z. Q., Jang, S., and Tan, E. (2012). “Maximization of precipitation for the 1997 storm event over American river watershed, California using a regional atmospheric model C3,” in World Environmental and Water Resources Congress 2012: Crossing Boundaries, Proceedings of the 2012 Congress (Albuquerque, NM), 1909–1916. doi: 10.1061/9780784412312.191
Ostrom, E. (2009). A general framework for analyzing sustainability of social-ecological systems. Sciences 325, 419–422. doi: 10.1126/science.1172133
Pender, D., Haynes, H., Pender, G., and Shvidchenko, A. (2014). “The influence of flood sequencing on the morphology and bed composition of the American River, California, USA C3,” in Proceedings of the International Conference on Fluvial Hydraulics, River Flow (Lausanne), 2297–2303. doi: 10.1201/b17133-306
Peterson, D. (1993). “Levee breach inundation study—Sacramento County, California C3,” in Proceedings—National Conference on Hydraulic Engineering (San Francisco, CA), 833–835.
Rädlinger, C., Hafner, K., Junge, M., and Nebl, A. (2012). Geschichte der Isar in München. Munich: Schiermeier.
Randle, T., Morris, G. L., Tullos, D. D., Weirich, F. H., Kondolf, G. M., Moriasi, D. N., et al. (2021). Sustaining United States reservoir storage capacity: Need for a new paradigm. J. Hydrol. 602, 126686. doi: 10.1016/j.jhydrol.2021.126686
Raška, P., Bezak, N., Ferreira, C. S. S., Kalantari, Z., Banasik, K., Bertola, M., et al. (2022). Identifying barriers for nature-based solutions in flood risk management: an interdisciplinary overview using expert community approach. J. Environ. Manage. 310, 114725. doi: 10.1016/j.jenvman.2022.114725
RESTORE (2013). “Isar river, Munich,” in River by Design—Rethinking Development and River Restoration, ed ECRR (Bristol: Environment Agency, Horizon House), 38–39.
Sartori, R. (2010). Die neue Isar/2—Renaturierung, kulturelle Öffnung und Ideen-Fluß, Geschichtliches wie Literarisches. Munich: Buch & Media.
Schellman, G. (1990). Fluvial geomorphodynamics on the younger Quaternary of the Lower Isar and the bordering Danube Valley. Dusseldorfer Geograp. Schriften 29. Available online at: https://www.researchgate.net/publication/307216201_Fluvial_geomorphodynamics_on_the_younger_Quaternary_of_the_Lower_Isar_and_the_bordering_Danube_Valley
Scheuermann, K. (1998). Die Isar—ein gebirgfluss im wandel der zeiten. Jahrbuch Verein Schutz Bergwelt 63, 1–131.
Schneeberger, K., Bellinger, J., Samm, M., von Stackelberg, M., and Lang, T. (2021). Flood pattern in the Isar river catchment. Hydrol. Wasserbewirtschaftung 65, 89–101. doi: 10.5675/HyWa_2021.3_1
Schwarz, A. M. (2015). California central valley water rights in a changing climate. San Franc. Estuary Watershed Sci. 13, 2. doi: 10.15447/sfews.2015v13iss2art2
Serra-Llobet, A., Jähnig, S. C., Geist, J., Kondolf, G. M., Damm, C., Scholz, M., et al. (2022). Restoring rivers and floodplains for habitat and flood risk reduction: experiences in multi-benefit floodplain management from California and Germany. Front. Environ. Sci. 9, 778568. doi: 10.3389/fenvs.2021.778568
Serra-Llobet, A., Kondolf, G. M., Magdaleno, F., and Keenan-Jones, D. (2021). Flood diversions and bypasses: benefits and challenges. WIREs Water 9, e1562. doi: 10.1002/wat2.1562
Serra-Llobet, A., Kondolf, G. M., Schaefer, K., and Nicholson, S. (2018). Managing Flood Risk: Innovative Approaches from Big Floodplain Rivers and Urban Streams. Cham: Palgrave Macmillan, 43–73. doi: 10.1007/978-3-319-71673-2
Sogard, S. M., Merz, J. E., Satterthwaite, W. H., Beakes, M. P., Swank, D. R., Collins, E. M., et al. (2012). Contrasts in habitat characteristics and life history patterns of Oncorhynchus mykiss in California's central coast and Central Valley. Trans. Am. Fish. Soc. 141, 747–760. doi: 10.1080/00028487.2012.675902
Solheim, A., Capobianco, V., Oen, A., Kalsnes, B., Wullf-Knutsen, T., Olsen, M., et al. (2021). Implementing nature-based solutions in rural landscapes: barriers experienced in the PHUSICOS project. Sustainability 13, 1461. doi: 10.3390/su13031461
Sultana, R., and Choi, M. (2018). Sensitivity of streamflow response in the snow-dominated Sierra Nevada watershed using projected CMIP5 data. J. Hydrol. Eng. 23, 1640. doi: 10.1061/(ASCE)HE.1943-5584.0001640
Swindle, L. J. (2000). The History of the Gold Discoveries in the Northern Mine's of California's Mother Lode Gold Belt. Victoria, BC: Trafford.
Vicuña, S., Dracup, J. A., and Dale, L. (2011). Climate change impacts on two high-elevation hydropower systems in California. Clim. Change 109 (Suppl. 1), 151–169. doi: 10.1007/s10584-011-0301-8
Wagner, T., Themessl, M., Schuppel, A., Gobiet, A., Stigler, H., and Birk, S. (2017). Impacts of climate change on stream flow and hydro power generation in the Alpine region. Environ. Earth Sci. 76, 4. doi: 10.1007/s12665-016-6318-6
Walsh, C. J., Roy, A. H., Feminella, J. W., Cottingham, P. D., Groffman, P. M., and Morgan, R. P. (2005). The urban stream syndrome: current knowledge and the search for a cure. J. North Am. Benthol. Soc. 24, 706–723. doi: 10.1899/04-028.1
Wantzen, K. M., Alves, C. B. M., Badiane, S. D., Bala, R., Blettler, M., Callisto, M., et al. (2019). Urban stream and wetland restoration in the global South—a DPSIR analysis. Sustainability 11, 4975. doi: 10.3390/su11184975
Wantzen, K. M., Ballouche, A., Longuet, I., Bao, I., Bocoum, H., Ciss,é, L., et al. (2016). River culture: an eco-social approach to mitigate the biological and cultural diversity crisis in riverscapes. Ecohydrol. Hydrobiol. 16, 7–18. doi: 10.1016/j.ecohyd.2015.12.003
White, G., Kates, R., and Burton, I. (2001). Knowing better and losing even more: the use of knowledge in hazards management. Glob. Environ. Change B Environ. Haz. 3, 81–92. doi: 10.3763/ehaz.2001.0308
White, G. F. (1945). Human Adjustment to Floods. Research Paper 29. Chicago, IL: Department of Geography, University of Chicago.
Williams, P. B. (1993). “Assessing the true value of flood control reservoirs: the experience of Folsom dam in the February 1986 flood C3,” in Proceedings—National Conference on Hydraulic Engineering (San Francisco, CA), 1969–1974.
Winiwarter, V., Haidvogl, G., and Bürkner, M. (2016). The rise and fall of Munich's early modern water network: a tale of prowess and power Core Springer Nature. doi: 10.1007/s12685-016-0173-y
Wisheropp, P. (2018). “Camp Sacramento erosion control project-−5 years later C3-−2018,” in IECA Annual Conference and Expo. 2018-February. Long Beach, CA.
Woldemichael, A. T., Hossain, F., Pielke Sr, R., and Beltrán-Przekurat, A. (2012). Understanding the impact of dam-triggered land use/land cover change on the modification of extreme precipitation. Water Resourc. Res. 48, W09547. doi: 10.1029/2011WR011684
Woldemichael, A. T., Hossain, F., and Pielke, R. (2014a). Evaluation of surface properties and atmospheric disturbances caused by post-dam alterations of land use/land cover. Hydrol. Earth Syst. Sci. 18, 3711–3732. doi: 10.5194/hess-18-3711-2014
Woldemichael, A. T., Hossain, F., and Pielke, R. (2014b). Impacts of postdam land use/land cover changes on modification of extreme precipitation in contrasting hydroclimate and terrain features. J. Hydrometeorol. 15, 777–800. doi: 10.1175/JHM-D-13-085.1
Yigzaw, W., Hossain, F., and Kalyanapu, A. (2013). Comparison of PMP-driven probable maximum floods with flood magnitudes due to increasingly urbanized catchment: the case of American river watershed. Earth Interact. 17, 1–15. doi: 10.1175/2012EI000497.1
Zingraff-Hamed, A., Bala, R., Bonnefond, M., Bonthoux, S., Cao, Y., Greulich, S., et al. (2020). Urban human-river encounter sites: how to re-establish contact between humans and nature in cities? Sustainability 13, 2864. doi: 10.3390/su13052864
Zingraff-Hamed, A., and Egger, G. (2019). Isar. Rivers of the Alps—Diversity in Nature and Culture (Vienna: Haupt Verlag), 512.
Zingraff-Hamed, A., George, F. N., Lupp, G., and Pauleit, S. (2022). Effects of recreational use on restored urban floodplain vegetation in urban areas. Urban For. Urban Green. 67, 127444. doi: 10.1016/j.ufug.2021.127444
Zingraff-Hamed, A., Greulich, S., Wantzen, K. M., and Pauleit, S. (2017a). Societal drivers of European water governance: a comparison of urban river restoration practices in France and Germany. Sustainability 9, 206. doi: 10.3390/w9030206
Zingraff-Hamed, A., Greulich, S., Wantzen, K. M., and Pauleit, S. (2017b). Societal drivers of European water governance: a comparison of urban river restoration practices in France and Germany. Water 9, 206.
Zingraff-Hamed, A., Lupp, G., Bäumler, K., Huang, J., and Pauleit, S. (2021). “The Isar river: social pride as a driver of river restoration,” in River Culture—Life as a Dance to the Rhythm of the Waters, ed K. M. Wantzen (Paris: UNESCO publishing).
Zingraff-Hamed, A., Martin, J., Lupp, G., Linnerooth-Bayer, J., and Pauleit, S. (2019). Designing a resilient waterscape using a living lab and catalyzing polycentric governance. Landsc. Archit. Front. 7, 12–31. doi: 10.15302/J-LAF-1-020003
Zingraff-Hamed, A., Noack, M., Greulich, S., Schwarzwälder, K., Pauleit, S., and Wantzen, K. M. (2018a). Model-Based evaluation of the effects of river discharge modulations on physical fish habitat quality. Water 10, 374. doi: 10.3390/w10040374
Keywords: urban river restoration, flood risk, nature-based solutions, urban biodiversity, recreation, climate change
Citation: Zingraff-Hamed A, Serra-Llobet A and Kondolf GM (2022) The Social, Economic, and Ecological Drivers of Planning and Management of Urban River Parks. Front. Sustain. Cities 4:907044. doi: 10.3389/frsc.2022.907044
Received: 29 March 2022; Accepted: 19 May 2022;
Published: 15 August 2022.
Edited by:
Thomas Rötzer, Technical University of Munich, GermanyReviewed by:
Balqis Mohamed Rehan, Putra Malaysia University, MalaysiaAbubakr Muhammad, Lahore University of Management Sciences, Pakistan
Copyright © 2022 Zingraff-Hamed, Serra-Llobet and Kondolf. This is an open-access article distributed under the terms of the Creative Commons Attribution License (CC BY). The use, distribution or reproduction in other forums is permitted, provided the original author(s) and the copyright owner(s) are credited and that the original publication in this journal is cited, in accordance with accepted academic practice. No use, distribution or reproduction is permitted which does not comply with these terms.
*Correspondence: Aude Zingraff-Hamed, YXVkZS56aW5ncmFmZi1oYW1lZEB0dW0uZGU=