- Environmental Studies Program, Science and Mathematics Department—Deree, The American College of Greece, Aghia Paraskevi, Greece
Green roofs have been proposed as a significant method of “renaturing” the city, mitigating the urban heat island effect and controlling storm water movement. Plant growth on green roofs affects the environmental performance of the roof. Thus, it is important to examine which parameters influence this growth. Green roofs in the Mediterranean region, due to the climatic specificities of the area, have been a challenge. What types of plants are appropriate, how green roofs should be constructed and other related topics have concerned scientists in the recent years. The aim of this pilot study was to explore the growth of five plants—Sedum sediforme, Drosanthemum floribundum and Lampranthus spectabilis, Medicago arborea, and Lavandula angustifolia—on a pilot Mediterranean green roof in relation to substrate depth, organic content of substrate and type of green roof (open and modular). Data were analyzed using multiple regression with Analysis of Variance. The results indicated that substrate depth, type of green roof and substrate organic content are not significantly important parameters affecting the growth of plants and cannot predict plant performance by themselves. A main insight arising from this study is that plant communities and interspecies relations should be examined more closely in future green roof research as they may affect the impact of selected parameters on plant growth on Mediterranean green roofs. Furthermore, although the type of the green roof—open or modular—could not be directly associated with plant performance, it may be worth examining its impact on plant community performance in future studies.
Introduction
Cities continue to grow and to attract an increasing number of people; at present, cities house more than half of the global human population. Contemporary cities violate nature and its laws, as they are made of concrete, are designed around the use of private cars and fossil fuels, with a high density of human population and human activities, importing resources for inhabitants' needs and used with a linear logic, generating much waste. The need to “renature” cities, find nature-based solutions to urban problems, and thus, make cities greener and more respectful of natural laws, has been recognized (e.g., European Commission, 2021). The concept of “biophilia” (Farr, 2008; Rogers, 2019)—love and contact with nature—is now considered a basic element of sustainable cities. Reconciliation ecology also promotes the idea of co-existence of humans with other species and anthropogenic habitats that support the existence of other species too (Francis, 2011).
Installing green roofs is a method for reintroducing nature into the cities and supporting the provision of its invaluable ecosystem services, as well as providing landscapes that are amenable to both humans and other species. The environmental benefits of green roofs have been the focus of many studies in the last decades (indicatively see Oberndorfer et al., 2007; Nagase and Dunnett, 2012; Berardi et al., 2014; Gong et al., 2021). These benefits include amongst others energy efficiency of buildings, storm water control, enhancement of microclimate and amelioration of the urban heat island effect, cleaner air, filtering water, increased biodiversity in cities, psychological benefits and aesthetic enhancement of urban landscapes. Each of these themes has attracted different levels of interest, while overall, researchers' interest in green roofs has increased over time (Liu et al., 2021).
As roofs are harsh environments, the selection of appropriate plants for different climates (Monterusso et al., 2005; Benvenuti and Bacci, 2010; Dvorak and Volder, 2010; Takahiro et al., 2010; Williams et al., 2010; MacIvor et al., 2011; Provenzano, 2011) has occupied researchers. Studies have shown that a combination of different species on a green roof improves resilience to stresses (Gedge and Kadas, 2005; Nagase and Dunnett, 2010).
Parameters, like irrigation (Maclvor et al., 2013; Carbone et al., 2014; Rowe et al., 2014; Vestrella et al., 2015a; Palermo et al., 2019; Zhang et al., 2021) and substrate, that influence plant growth have been significant research concerns in the recent years. The depth of the substrate has repeatedly been suggested as a main parameter influencing plant growth (Dunnett and Nolan, 2004; Durhman et al., 2007; Getter and Rowe, 2009; Rowe et al., 2012; Papafotiou et al., 2013). Nagase and Dunnett (2013) in their study of geophytes on extensive green roofs in the United Kingdom found that plants did better in terms of growth, flowering and survival rate in the deeper substrate of 10 cm. They hypothesized that this was due to better moisture retention and steadier temperatures. However, they also found that some plants, like different species of Tulipa, performed well at 5 cm substrate depth too without irrigation. Vangergrift et al. (2019) study showed that deeper substrates supported a larger variety of species, while irrigation was identified as critical for the maintenance of species diversity in the long term. Generally, different substrate depths – between 4 and 25 cm – support different vegetation forms from mosses and sedum to herbaceous plants and grasses (FLL, 2008; Ntoulas et al., 2017). Other studies, like Hawke's (2015) evaluation of different plants for use on green roofs, demonstrated that some plants did equally well in all tested growing substrate depths, while others that displayed differences in their growth ratings scored better in deeper depths. Other studies have demonstrated that other characteristics of the growing medium, like its organic content, nutrients or pH, are important (Nagase and Dunnett, 2011; Bates et al., 2013; Kotsiris et al., 2013; Thuring and Dunnett, 2014; Tassoula et al., 2015; Papafotiou et al., 2016; McAlister and Rott, 2019). Further studies have investigated the influence of different types of substrates (A'saf et al., 2020; Paraskevopoulou et al., 2021), or other parameters relating with the substrate like the role of bacteria in the soil (Xie et al., 2020; Wang et al., 2021), on plant performance, or on the functions of green roofs (Naranjo et al., 2020). Bates et al. (2013) investigated vegetation development on low-fertility growth substrates emulating brownfields and their responses to drought conditions. They noted that plants living in more fertile substrates and doing better when water is available are more vulnerable to drought disturbance. Young et al. (2014) demonstrated that substrate depth had no significant effect on plant growth under controlled temperature conditions, while they argued that substrate composition is significant for plant performance on green roofs and it should be carefully considered in green roof design. Some have investigated more specifically the type/source of organic component of the substrate and its influence on plant growth (Papafotiou et al., 2013).
Local climatic conditions influence the design considerations for green roofs. As green roofs are harsh environments in any case, the Mediterranean climate poses additional challenges for them due to its dry and hot summers. For this reason, only relatively recently modern green roofs started being installed in Mediterranean cities. In the same period, scientific interest in designing and establishing Mediterranean green roofs has increased (Benvenuti and Bacci, 2010; Provenzano, 2011; Caneva et al., 2013; Kotsiris et al., 2013; Papafotiou et al., 2013; Raimondo et al., 2015; Vestrella et al., 2015b). Relevant scientific literature has investigated which plants are more suited for Mediterranean green roofs and studies have generally shown that certain plant taxa and xerophytes or succulent plants are the most appropriate for them (e.g., Provenzano, 2011; Papafotiou et al., 2013; Tassoula et al., 2015). Some studies have examined and identified specific plant species (such as Sedum sediforme and other Sedum species, Lavandula angustifolia, Anthemis tinctoria, etc.) as most appropriate for green roofs in the Mediterranean climate (Nektarios et al., 2011; Kotsiris et al., 2012; Ondoño et al., 2016; Azenas et al., 2018, 2019). Vasl et al. (2017) examined the contribution of Sedum sediforme to the creation of biodiverse Mediterranean green roofs and concluded that the combination of sedum with annuals improved the performance of green roof functions. However, Nektarios et al. (2021), who investigated the appropriateness of seeded sedum species on a Mediterranean roof as well as the influence of substrate depth and irrigation regime on them, suggested that their use in the Mediterranean climate is not appropriate. Others (like Papafotiou et al., 2013; Ondoño et al., 2016) investigated the significance of substrate depth for plant growth on Mediterranean green roofs and have generally reiterated that deeper growing medium better promotes the establishment, growth, and survival of vegetation. Nevertheless, Papafotiou et al. (2013) indicated that other characteristics beyond the depth of the substrate (i.e., compost-amended substrate with sparse irrigation) can result in similar plant growth with that of deeper growing mediums. Water availability has been another issue that has been investigated in Mediterranean green roofs (Carbone et al., 2014; Palermo et al., 2019). Azenas et al. (2018) identify water availability as the main limiting factor for urban greening in Mediterranean climates and suggest that this should be a main criterion for the selection of plants for green roofs in these areas.
This pilot study aims to investigate the influence of substrate depth, organic content in the substrate and the type of the green roof (i.e., modular or open extensive approaches to green roofs) on plant growth in a typical urban Green Roof (i.e., sometimes obstructed with structural elements) without any human interference (i.e., no pruning, replanting etc.) after the original set up, approximating real conditions as much as possible. For this reason, the green roof was left to evolve without any human interference for the whole duration (~2 years) of the experiment. The aim was to examine a green roof situation that is akin to a real-life, non-experimental site.
Five plants that are considered possible candidates for Mediterranean climates were selected. Our main research questions and the hypotheses we tested are:
1) What is the effect of substrate depth on plant growth in an open and closed (modular) green roof system?
Hypothesis: Increasing substrate depth will positively impact the growth of plants.
2) What is the effect of different levels of organic content on plant growth in a closed (modular) green roof system?
Hypothesis: Higher levels of organic content will positively impact the growth of plants.
3) Do selected plants grow better in an open or a closed (modular) green roof system?
Hypothesis: No such study has been identified in the literature; thus, we had no pre-conceived hypothesis for this question. An open system allows for slightly more space but is also subject to more loss of substrate.
Materials and Methods
The Experimental Site
The pilot green roof site was at Aghia Paraskevi, Greece (Lat 38° 00′ 16″ N Long 23° 49′ 59″ E) in the college campus of the American College of Greece, which is found at the Northeast side of the Hymettus Mountain at 270 m altitude, has a mild inclination and is exposed to north winds. As Figure 1 illustrates, it was located at the Deree building complex, on the 6th level of a 7-level step-hill building with a Northwest-Southeast axis. Thus, this is a roof that is generally sheltered from winds. The choice of the roof was limited by campus-specific restrictions.
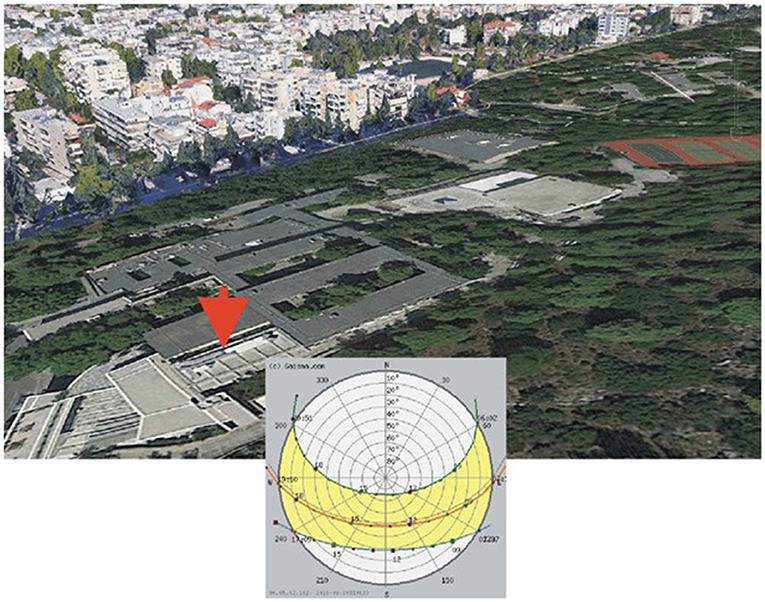
Figure 1. The experimental site location and movement of the sun. Source: Google maps and www.gaisma.com.
It was installed as a set of 3 plots (labeled A-C). Every plot had an area of 8 m2 (2 m width by 4 m length) and included 8 quadrats (labeled a-h) of 1 m2 each. Figure 2 below provides a graphic representation of the research site. Each plot represented a different set of conditions, addressing the research questions. One plot (A) was an open extensive system with different substrate depths, including 20, 15, 10, and 6 cm. Another one (B) was a modular system with different substrate depths too, i.e. 12, 10, 8, and 6 cm. The largest depth of the modular system (12 cm) was smaller than the largest one in the open system (20 cm) as deeper modular pieces are too heavy to move; however, we ensured to have 2 common depths (i.e. 6 and 10 cm) in the A and B plots and 10 cm depth in all the quadrats of the C plot for comparison purposes. The third one (C) was a modular system with all quadrats having the same substrate depth (10 cm) but different organic content: 10, 7.5, 5, and 2% or less (reflecting existing literature and practice in the Mediterranean region). The two adjacent quadrats (i.e. a-b, c-d, e-f, g-h) in each plot were identical in their design, meaning that each group of conditions has two replicates. Each of the 11 groups (labeled i-xi) represent a specific combination of parameters (i.e., organic content, substrate depth and type of green roof). For example, quadrats denoted as a(i) and b(i) are replicates. Each plot aimed to address one of the 3 main research questions of this experiment.
The green roof plots were constructed by the research team in accordance with the official green roof installation guidelines (FLL, 2008). For the open extensive system (System A), a wooden box of 8 m2 was constructed with 8 quadrats of 1 m2 surface each and the following commercially available layers were installed: water-proofing and root-barrier membrane, geotextile, drainage layer, geotextile, irrigation system and substrate. The two layers of geotextile that were used were different: the lower one was thicker in order to increase water retention, while the second one was thinner allowing root and water penetration. The modular systems (Systems B and C) had the same initial layers: water-proofing and a root-barrier membrane, geotextile and drainage. Modular pieces (i.e., geotextiled pillowcases filled with substrate) were placed on top of them. The modular pieces were commercially available in the local market and adjusted to the needs of the Mediterranean region. The substrate (used in the open and modular systems) was a patented product produced and distributed by Oikosteges (a Small & Medium Enterprise with extensive experience on green roofs). The substrate is mainly composed of multiple granulometries of perlite and contains ~2% organic matter, including mycorrhizae. The dry weight of each 1 m2 module was ~20 kg. An automatic irrigation system was also installed, common for all plots.
Weather, Soil Conditions, and Irrigation
At the location of the experiment, the typical Mediterranean climate (i.e., mild winters and hot dry summers) is experienced. Weather patterns were recorded for the whole period of the experiment, using data from the campus meteorological station.
The climatic conditions in the area during the experimental period (September 2016 – July 2018) are shown in Figure 3 below. The air temperature ranged from −2.9 to 42.1°C, and soil temperature from 13°C (in winter 2018) to 24.5°C (in summer 2018). The average humidity was 39.72% and the storm total ranged from 0 to 131.2 mm.
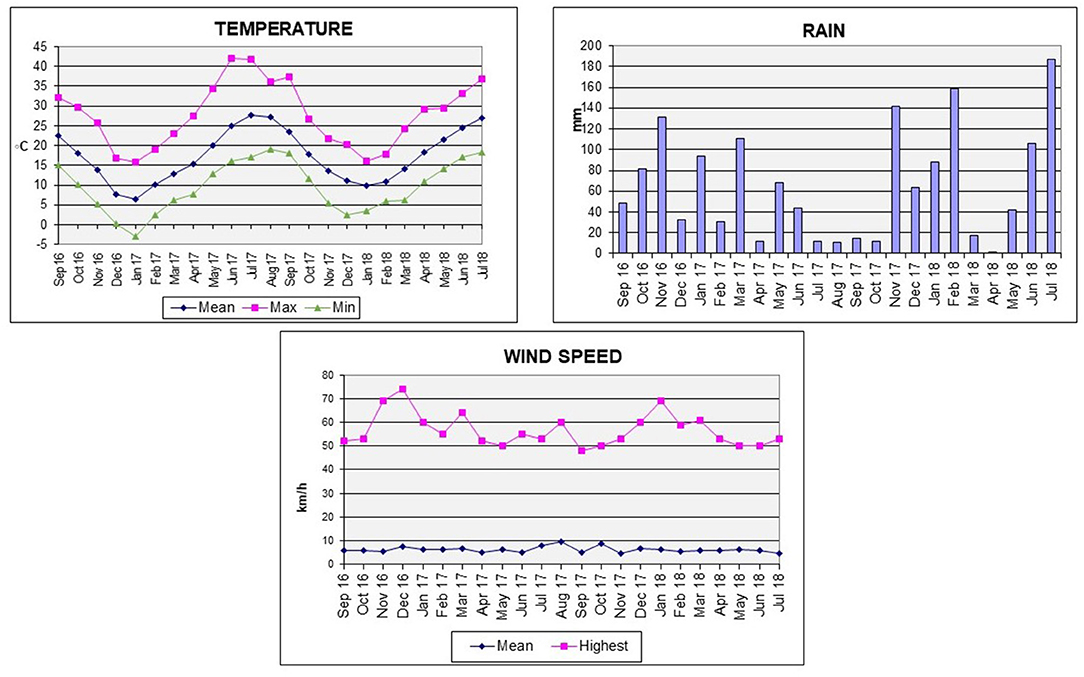
Figure 3. Air temperature, precipitation and wind speed for the experimental period. Source: NOANET Database (Ag. Paraskevi meteo station); http://meteosearch.meteo.gr/.
All plots and quadrats were subjected to the same irrigation plan. Given that each plant has different irrigation needs, we selected an average irrigation scheme to cover the needs of the selected plants. In the period June 2016 to end of January 2018 and 17 April 2018 to the end of the experiment, the plots were irrigated 15 min per day at 5:00 A.M., while in the period February 2018 to 16 April 2018, they were watered for 5 min per day at the same time. Given our irrigation system (i.e., size of pipes, length etc.), in the periods with the longer irrigation schedule, 250 ml of water were provided per day from each hole, while in the period with the shorter irrigation time, 80 ml. Plants were not irrigated for almost 2 weeks, in the first 2 weeks of April 2018, as the battery of the automatic irrigation system ran out.
Plants
Five plant species were selected to be tested on this green roof experiment, based on green roof practicioners' knowledge regarding plants that are considered appropriate for the Mediterranean green roofs and relevant literature. Three of them are succulent plants (Sedum sediforme, Drosanthemum floribundum and Lampranthus spectabilis) and two shrubs (Medicago arborea and Lavandula angustifolia). The same five plants were planted in each quadrat in the exact same arrangement. Table 1 below presents the taxonomy and main characteristics of the selected plant species.
Data Collection: Plant Growth
Data on plant height and two-dimensional width (longest called length) were collected approximately every 2 weeks for a period of 22 months, for the estimation of plant growth (like Monterusso et al., 2005). In total, data were collected in 34 different time points, leading to 816 data points. Height was measured starting from the bottom of the plants (soil top in the midst of the plant). Length was considered to be the longest distance between the two furthest ends of the plant's canopy. The width was the longest vertical line to the length. For the Open system - Plot A - (see Figure 2), width and length of plants were recorded even when they exceeded their wooden frames. Furthermore, observations regarding the plants' physical appearance (dry or dead), and flowering period, as well as other factors that affect plant growth such as presence of wild grass and flowers were also recorded.
Specifically, the physical appearance was recorded as Status (0: alive, 1: dry, and 2: dead) and as Absence (0: present, 1: absent). Flower denotes the presence (1) or absence (0) of flowers of the plants, while Grass denotes the presence (1) or absence (0) of wild flowers or grass.
Data Analysis
Based on the above-mentioned measurements, a Growth Index (GI) was calculated to assess plant growth, as the average of the three dimensions according to the following formula: GI = (H + W + L)/3, where H denotes the height parameter, W the width and L the length parameter. A qualitative assessment of the data was first performed, based on the graphical depictions of plant growth over time, followed by a basic statistical analysis aiming to assess the statistical significance of the parameters considered as well as any possible interactions between those. Data were analyzed using multiple linear regression with Analysis of Variance (ANOVA) to estimate the statistically significant parameters that affect the growth of plants as measured by GI. Pre-processing of the data was conducted. Correlation analysis was performed for pairwise comparisons of parameters to identify and exclude any correlated features from further analysis (Figure 4). The parameters Width, Length and Height were excluded for further analysis as highly correlated to GI (above than 0.8 by absolute value). Data were filtered to exclude correlated data and outlier data; particularly, three outliers were found based on the interquartile range values of the descriptors and they were all referring to one data point. A stepwise procedure was considered where independent descriptors were added to improve the fit of the model. The descriptors found to be statistically significant at a significance level of α = 0.05 or less, specifically: Species, Flower, Grass, Status, Absence, Plot, Irrigation water volume, Humidity (all of them having p < 0.0001), as well as Temperature (p = 0.00101), and Group (p = 0.0509). Interaction terms were also considered (adjusted R2 = 0.7923), depicting some statistically significant interactions between parameters, specifically between Species and Absence, Species and Status, Species and Temperature, Species and Group, Flower and Status (α = 0.05). Some of the interactions found statistically significant such as Species and Absence, serve as a good validation for our results as they are evident; thus, these are not further discussed as an analysis outcome. Two triple interaction terms were found to be statistically significant at α = 0.05: Species-Flower-Group (p = 0.001025), Species-Temperature-Status (p = 0.0262884). Detailed observational results are presented in the following section together with interaction plots for those parameters found to be statistically significant (p < 0.0001, α = 0.0001).
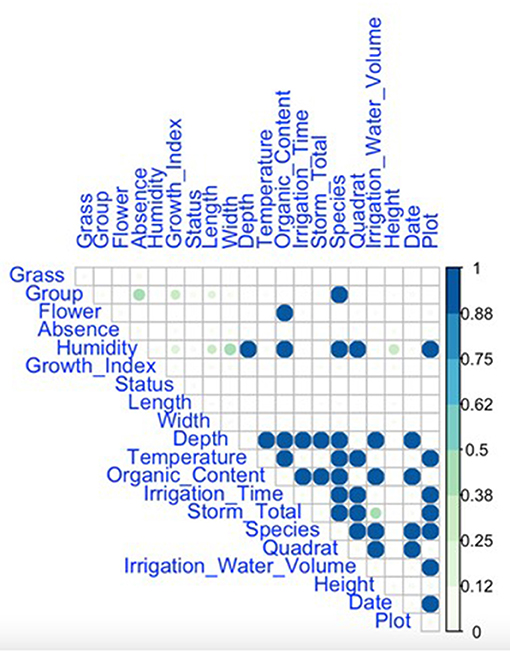
Figure 4. A pairwise correlation plot for all parameters considered in the study. The color and size of the circle denotes the magnitude of the correlation value. Highly correlated parameters were considered to be those with absolute correlation values >0.7. Source: Authors.
Results
Plant Growth and Substrate Depth
Plots A (open) and B (modular) were set up to investigate the impact of substrate depth on the selected plants. Overall, observations suggested that different plants might have different behaviors in relation to substrate depth. In Supplementary Figure 1 of the supplement, growth curves for the plants in the highest and lowest depths of the open and modular systems are displayed, showing the Growth Index factor (y axis) against measurement (x axis). The statistical analysis demonstrated that substrate depth was not an important parameter affecting GI. The interaction plot of species, substrate depth and GI is depicted in Figure 5A. Observations from the analysis indicate that substrate depth did not impact the growth of D. floribundum. Due to the erratic growth and high mortality rate of S. sediforme, no clear association was identified with substrate depth and plant growth. It is important to mention that most of the individuals that managed to survive were in plot A at the deeper growing media. Whereas, the individuals that survived in plot B were in lower-depth substrates [Bc(vi), Bd(vi), Bh(viii)]. L. angustifolia showed high GI in intermediate depths (12 and 15 cm). L. spectabilis seemed to benefit from deeper growing media. Finally, there is not a clear connection between depth and GI for M. arborea.
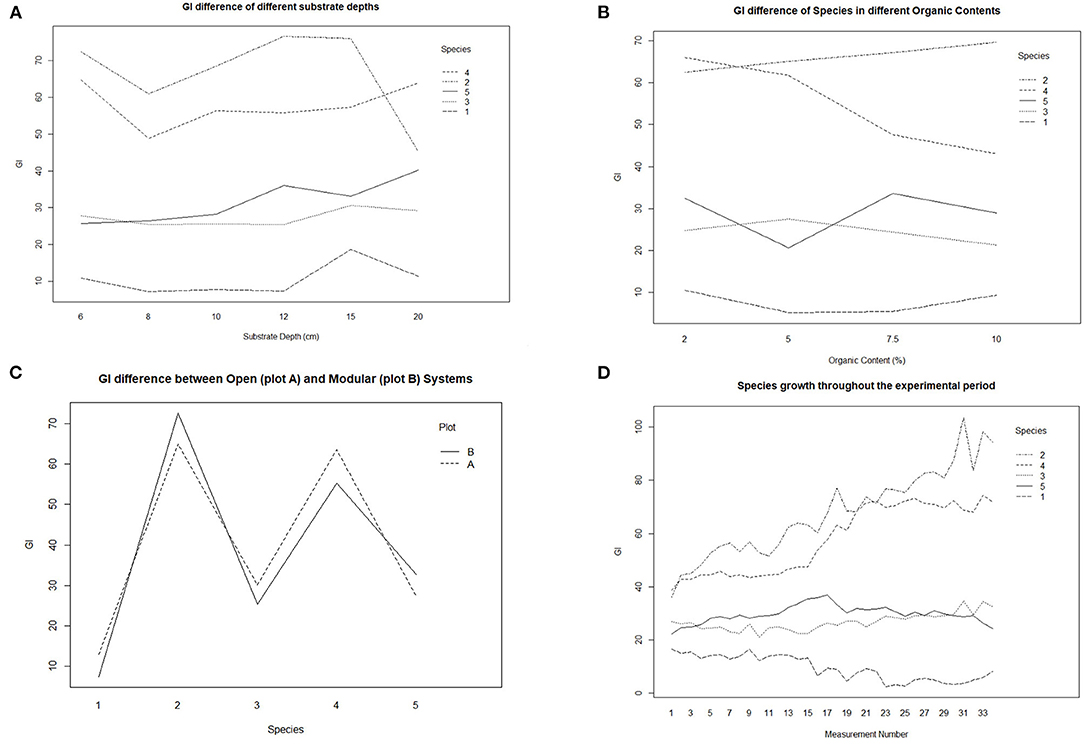
Figure 5. Interaction plots of species in relation to substrate depth, organic content, type of green roof system, and measurement number. (A) GI difference of different substrate depths, (B) GI difference of species in different organic contents, (C) Mean GI difference between Open (plot A) and Modular (plot B) Systems and (D) Species growth throughout the experimental period (Measurement numbers: 1-34); where plant species: 1: Sedum sediforme, 2: Medicago arborea, 3: Drosanthemum floribundum, 4: Lavandula angustifolia and 5: Lampranthus spectabilis. Source: Authors.
Plant Growth and Organic Content of the Substrate
Plot C was set up to study the impact of the composition of the substrate, especially in relation to organic content, on plant growth. Qualitative observations (shown in Supplementary Figure 2) and the statistical analysis demonstrated that organic content did not significantly affect the GI (see Figure 5B). M. arborea did better in substrates with higher organic content. S. sediforme has higher GI mean value at 2 and 10% organic content, while L. angustifolia exhibited the highest end growth at the quadrats with the lowest organic content.
Plant Growth and Type of Green Roof System
To compare the possible different patterns of growth in the two different systems (open and closed), the quadrats of plots A (open) and B (modular) were analyzed. Both the qualitative observations (shown in Supplementary Figure 3) and the statistical analysis found that the type of green roof system did not affect the GI. Based on Figure 5C some observations can be made for the specific species. Overall, M. arborea and L. spectabilis performed better in the modular system, whereas S. sediforme, D. floribundum, and L. angustifolia benefit more in the open system. Specifically, Sedum sediforme did better in the open system, especially at the deeper growing media, while the three plants that disappeared in the open system [Ae(iii), Af(iii), Ag(iv)] reappeared. S. sediforme struggled and more than half (5) died in the modular system. Additionally, L. spectabilis did much better in the modular system, where all except one plant survived and several doubled their size. In the open system, more than half of the L. spectabilis (5) plants died. This may be an indication of how this species reacts differently in different types of green roof systems (open and modular).
Plant Species
The growth of the different plant species throughout the experimental period is depicted in the interaction plot “Species growth throughout the experimental period” (see Figure 5D). Similarly, the GI of species among the different groups is depicted in Figure 6C.
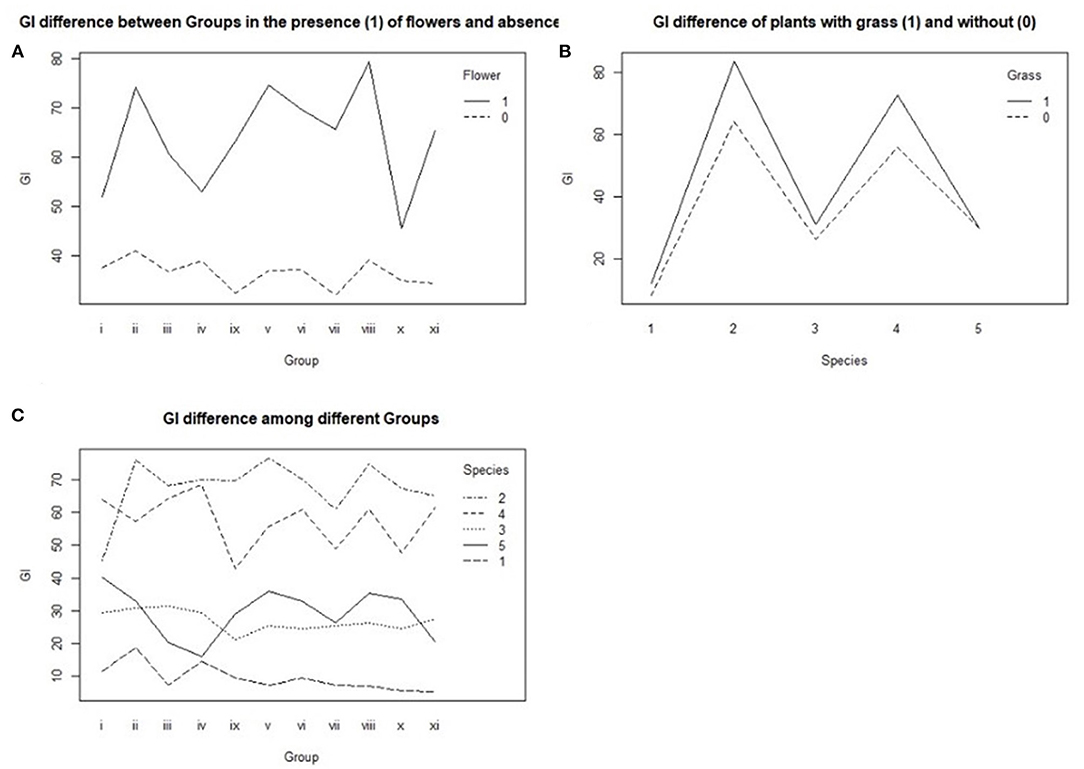
Figure 6. Interaction plots of Species in relation to Flower, Grass and Group. (A) GI difference between Groups (i-xi) in the presence (1) and absence (0) of Flowers, (B) GI difference of plants with Grass (1) and without (0) and (C) GI difference of Species among different Groups (i-xi); where plant species: 1: Sedum sediforme, 2: Medicago arborea, 3: Drosanthemum floribundum, 4: Lavandula angustifolia and 5: Lampranthus spectabilis. Source: Authors.
For the purposes of this experiment, it was considered that a plant did not survive (plant mortality) and was recorded as non-existent or “dead” when it had only dry stems and no leaves for three consecutive measurements (~1.5 months). We faced a challenge with S. sediforme, which on some occasions re-sprouted after several weeks. Specifically, S. sediforme had an unpredictable pattern of growth, mortality and disappearance. A significant number of S. sediforme plants died (50%). D. floribundum and L. angustifolia did well and no individual died or was removed throughout the sampling period. For the latter, one plant died after an attack to all lavender plants by a certain invasive insect in April 2018, while one plant disappeared probably by a human intervention. M. arborea proved very well-suited for this setting. All plants survived with the exception of Ab(i)2 which was removed probably by a human intervention before the first summer in 2017. Finally, high mortality (33%) was also observed among the L. spectabilis plants.
Throughout the sampling period and for all species dried (Status = 1), individuals that managed to survive were recorded. The statistical model constructed for this study showed, as expected, that the status of the plant (p < 2e-16) and mortality or absence (p < 2e-16) affected the GI, but also identified flowering (p < 2e-16) and grass presence (p = 1.18e-06) as statistically significant parameters affecting GI. Figures 6A,B show the interactions between Flower, Group, and GI as well as the interactions between Grass, Species, and GI, respectively.
It should be noted that S. sediforme never flowered during those 2 years and therefore there is no record for its flowering pattern. That sounds reasonable as these individuals were generally struggling for survival. Overall, the two main flowering periods of the green roof were from 11/2016 to 06/2017 and 02/2018 to 06/2018. More specifically, both M. arborea and D. floribundum did very well in our system. They displayed similar growth patterns irrespective of substrate depth or organic content. During the experiment, both plants flowered in the period from February or March to June. L. angustifolia flowered in the 1st year, from December 2016 to June 2017, and in the 2nd year, from October 2017 to March 2018. Finally, L. spectabilis plants flowered in April 2017, while in the 2nd year, only some individuals flowered. Overall, as it was expected the GI increased during the flowering periods as it is shown in Figure 6A. The presence of wildflowers or grass, denoted as Grass in Figure 6B, was found to be a statistically significant parameter (p = 1.18e-06) with higher GI and the flowering periods for all species. This may be related to seasonal climatic variations.
Discussion
It is worth starting our discussion by emphasizing that this irrigated green roof was intended to approximate a “natural” green roof as closely as possible. Thus, aside of the daily – same in all quadrats – irrigation, the only other human intervention was the hand-removal of the insects that attacked L. angustifolia plants in the spring periods of the experimental period. No pruning, mowing or replanting of disappeared individuals was performed.
A number of studies have identified substrate depth as an important parameter influencing plant growth on green roofs, with deeper growing media supporting higher plant growth (Kazemi and Monorko, 2017). This has also been supported by studies in Mediterranean climates as well (see Benvenuti and Bacci, 2010; Panayiotis et al., 2011; Papafotiou et al., 2013). However, our study suggests that substrate depth does not seem to have a clear impact on plant growth. Different plants may react differently to depth of growing media. The statistical analysis verified this observation as it did not identify depth as a significant parameter influencing plant growth. This observation agrees with a few other published studies (Nagase and Dunnett, 2013; Hawke's, 2015). There are indications that L. spectabilis benefits from deeper growing media. These findings are in accordance with other relevant scientific findings that show that some species can grow well on less deep growing media too. Durhman et al. (2007) state that although deeper substrates support greater plant growth in general, “in the shallowest depth of 2.5 cm, several species were observed to form stable communities.” Also, Nagase and Dunnett (2013) found that some dwarf geophytes could perform well on a substrate depth of 5 cm on an extensive roof without irrigation. Thus, based on the findings of this study, which are also supported by some previous literature, we propose that substrate depth as a parameter influencing plant growth on Green Roofs should be considered in relation with the plant species characteristics and physical parameters of the roof, like light (Getter et al., 2009) and water availability (see Table 1 above).
Specifically, for Lavandula angustifolia, our study demonstrated that substrate depth does not impact plant growth. This finding contradicts the results of the study of Kotsiris et al. (2012), which showed that lavender grows better in deeper depths and with more compost; however, they examined deeper growing media (30 cm and 20 cm) than we did. This issue requires further research, focusing on specific plants' (e.g., L. angustifolia) behavior at shallower depths, for a more conclusive comment.
Young et al. (2014) identified substrate composition as a significant parameter influencing plant growth, with growing media richer in organic matter supporting more plant growth. This is not supported by our study which suggests that the substrate's organic content is not an important parameter influencing plant growth. Our observations for the different species (i.e., S. sediforme and L. angustifolia did better in the substrates with less organic content, while M. arborea in the substrates with higher organic matter) may suggest that plant species and their needs, in connection with the plant community (e.g., interspecies competition; aboveground and below ground interactions) as indicated by Elhakeem et al. (2018), may mitigate the impact of the organic content of the substrate. This allegation agrees with Lundholm et al. (2014) findings that plant traits can be used to predict plant growth, and Elhakeem et al. (2018) suggestion that plant interactions influence plant performance. Similarly, Bates et al. (2015) showed that in their study of a brown field or biodiverse green roof, low organic content was “ideal,” and claimed that the most appropriate substrate organic content may vary with the green roof's water holding capacity.
The type of the Green Roof did not seem to significantly impact the growth of M. arborea, D. floribundum, and L. angustifolia as demonstrated by the statistical analysis and also indicated by qualitative observations. Based on the qualitative observations, the modular system seemed to better support plant growth for L. spectabilis (i.e., considerably better survival rate) and M. arborea (i.e., higher end growth). D. floribundum and L. angustifolia performed slightly better in the open system. Also, S. sediforme did better in the open system, especially in deeper growing media. These may be indications of how some species react differently in different types of green roof systems (open and modular), but they cannot be ascertained on the basis of this study. Further research is needed to examine to what extent the type of the Green Roof may affect plant growth and community development, and why.
This part of the study is quite original as we could not locate studies that compared modular and open Green Roof systems. The open system may allow the development of a more diverse bio-community potentially with both supportive and competitive relations, but it is also subject to more loss of particles through wind and easier evaporation of water.
Our experiment included three succulent plants – S. sediforme, D. floribundum and L. spectabilis – and two shrubs – M. arborea, which is a nitrogen fixer, and L. angustifolia. Low survival rate was observed for S. sediforme (50%) and L. spectabilis (33%). M. arborea and L. angustifolia proved more resilient. From these observations, we cannot conclude that S. sediforme and L. spectabilis species are inappropriate for Mediterranean green roofs; rather, we may need to consider the significance of other parameters like the community for S. sediforme. S. sediforme disappeared in large numbers in plots B and C (modular), while it did better in plot A (open system). In plot A, sedum plants reappeared after a period when they were considered as disappeared. This could be associated with inter-species competition, especially as it seems that sedum plants did better where other plants struggled. Furthermore, we had the opportunity to observe the impact of drought on our plants due to an accident with the automatic irrigation system, which left the plants without water for ~2 weeks in spring 2018, but they all recovered to their previous stage after reinstating the irrigation program and sustained their optimum growth.
Thus, we agree with Kazemi and Monorko (2017) who indicate that: “While it is obvious that growing media components, depth, and attributes affect plant performances in green roofs, the extent to which these components and their ratios affect vegetation in different climates is still unknown.” Further multi-criteria investigation is needed. Such an approach may also enhance our understanding regarding the influence of the type of green roof on plant performance. Furthermore, the influence of the plant community on different species' establishment and growth should be further examined (see Durhman et al., 2007; Lundholm et al., 2014).
Conclusion
This study aimed to contribute to the scientific basis of the practice and implementation of green roofs in the Mediterranean. It was conducted in the context of an educational setting; thus, there were both spatial constraints (in the selection of the roofs that could be used), and time constraints (in the data collection). A positive aspect of this study is that it provides a realistic green roof setting that can be found in Mediterranean urban environments and so enables useful lessons to be drawn for such contexts.
Based on our findings, it appears that substrate depth by itself can neither explain differences in plant growth nor predict plant performance on green roofs. Substrate depth may be an important factor in combination with other parameters (also indicated by Raimondo et al., 2015; Elhakeem et al., 2018; Vangergrift et al., 2019). Similarly, organic content is not appearing to affect the plant growth. However, plant species and their needs, as well as interspecies relations (Elhakeem et al., 2018) may mitigate the impact of the substrate organic content on their growth.
In addition, the type of green roof – open or modular – could not be associated with plant performance; some species in our experiment performed better in the open system (i.e., S. sediforme) and others grew better in the modular one (i.e., L. spectabilis). As this is an issue that has not been much explored in the scientific literature, an extensive study will be needed to investigate why and how the type of the green roof may be associated with plant performance.
Data Availability Statement
The original contributions presented in the study are included in the article/Supplementary Materials, further inquiries can be directed to the corresponding author/s.
Author Contributions
The research was designed by CM, AM, PP, and DG. Statistical analysis was performed by PS and GT. MK and PS were instrumental in data collection, processing, and presentation. The writing of the article was primarily done by PS and CM, with input from MK, PP, AM, GT, and DG. All authors significantly contributed to all aspects of the research process according to their expertise.
Funding
This research was possible with the help of many people, and especially: Andrew Clements (of Oikosteges) who provided inspiration, advice and practical knowledge at the research planning and installation of the research site; Thrassyvoulos Manios and Fenia Galliou who offered their expert advice at the research design phase; and Nikos Karahlis and Eirini Panteliodi who practically supported the research process at different stages. George Kontellas, Nephele Cauchi, Nikos Chanas, Jean-Philippe Grossetete, Xenia Vasilaki, Nikos Christodoulopoulos, Justen Biery, Nikos Kotsiopoulos, Zoe Eleni Moragli, and Nikos Synetos - students of the Environmental Studies Program of Deree - The American College of Greece - supported the actual field measurements. Finally, Deree – The American College of Greece funded this research. We are very thankful.
Conflict of Interest
The authors declare that the research was conducted in the absence of any commercial or financial relationships that could be construed as a potential conflict of interest.
Publisher's Note
All claims expressed in this article are solely those of the authors and do not necessarily represent those of their affiliated organizations, or those of the publisher, the editors and the reviewers. Any product that may be evaluated in this article, or claim that may be made by its manufacturer, is not guaranteed or endorsed by the publisher.
Supplementary Material
The Supplementary Material for this article can be found online at: https://www.frontiersin.org/articles/10.3389/frsc.2021.796441/full#supplementary-material
References
A'saf, T. S., Al-Ajlouni, G., Ayad, J. Y., Othman, Y. A., and Hilaire, R. S. (2020). Performance of six different soilless green roof substrates for the Mediterranean region. Sci. Total Environ. 730, 1–10. doi: 10.1016/j.scitotenv.2020.139182
Azenas, V., Janner, I., Medrano, H., and Gulías, J. (2018). Performance evaluation of five Mediterranean species to optimize ecosystem services of green roofs under water-limited conditions. J. Environ. Manage. 212, 236–247. doi: 10.1016/j.jenvman.2018.02.021
Azenas, V., Janner, I., Medrano, H., and Gulías, J. (2019). Evaluating the establishment performance of six native perennial Mediterranean species for use in extensive green roofs under water-limiting conditions. Urban Forestry Urban Green. 41, 158–169. doi: 10.1016/j.ufug.2019.04.002
Bates, A. J., Sadler, J. P., Grewell, R. B., and Mackay, R. (2015). Effects of varying organic matter content on the development of green roof vegetation: A six year experiment. Ecol. Eng. 82, 301–310. doi: 10.1016/j.ecoleng.2015.04.102
Bates, A. J., Sadler, J. P., and Mackay, R. (2013). Vegetation development over four years on two green roofs in the UK. Urban For. Urban Green 12, 98–108. doi: 10.1016/j.ufug.2012.12.003
Benvenuti, S., and Bacci, D. (2010). Initial agronomic performances of Mediterranean xerophytes in simulated dry green roofs. Urban Ecosyst 13, 349–363. doi: 10.1007/s11252-010-0124-9
Berardi, U., Ghaffarian-Hoseini, A. H., and Ghaffarian-Hoseini, A. (2014). State-of-the-art analysis of the environmental benefits of green roofs. Appl. Energy 115, 411–428. doi: 10.1016/j.apenergy.2013.10.047
Caneva, G., Kumbaric, A., Savo, V., and Casalini, R. (2013). Ecological approach in selecting extensive green roof plants: A data-set of Mediterranean plants. Plant Biosyst. 2, 374–383. doi: 10.1080/11263504.2013.819819
Carbone, M., Garofalo, G., Nigro, G., and Piro, P. (2014). A conceptual model for predicting hydraulic behaviour of a green roof. Proc. Eng. 70, 266–274. doi: 10.1016/j.proeng.2014.02.030
Dunnett, N., and Nolan, A. (2004). “The effect of substrate depth and supplementary watering on the growth of nine herbaceous perennials in a semi-extensive green roof,” in Proceedings of IC on Urban Horticulture (ISHS). doi: 10.17660/ActaHortic.2004.643.40
Durhman, A. K., Rowe, D. B., and Rugh, C. L. (2007). Effect of substrate depth on initialgrowth, coverage, and survival of 25 succulent green roof plant taxa. HortScience 42, 588–595. doi: 10.21273/HORTSCI.42.3.588
Dvorak, B., and Volder, A. (2010). Green roof vegetation for North American ecoregions: a literature review. Landsc. Urban Plan. 96, 197–213. doi: 10.1016/j.landurbplan.2010.04.009
Eggli, U. (2003). Illustrated Handbook of Succulent Plants: Crassulaceae (1st Edition). Berlin: Springer. doi: 10.1007/978-3-642-55874-0_1
Elhakeem, A., Markovic, D., Broberg, A., Anten, N. P. R., and Ninkovic, V. (2018). Aboveground mechanical stimuli affect belowground plant-plant communication. PLoS ONE 13:e0195646. doi: 10.1371/journal.pone.0195646
European Commission (2021). Nature-Based Solutions. Available online at: https://ec.europa.eu/info/research-and-innovation/research-area/environment/nature-based-solutions_en (accessed August 10, 2021).
Farr, D. (2008). Sustainable Urbanism: Urban Design With Nature. Hoboken, NJ: John Wiley & Sons, Inc.
FLL German Landscape Research, Development and Construction Society. (2008). Guidelines for the Planning, Construction and Maintenance of Green Roofing. Bonn.
Francis, R. A. (2011). Urban reconciliation ecology: The potential of living roofs and walls. J. Environ. Manage. 92, 1429–1437. doi: 10.1016/j.jenvman.2011.01.012
Gedge, D., and Kadas, G. (2005). Green roofs and biodiversity. Biologist 52, 161–169. Available online at: https://search-ebscohost-com.acg.idm.oclc.org/login.aspx?direct=true&db=a9h&AN=17821371&scope=site
Getter, K. L., and Rowe, D. B. (2009). Substrate depth influences sedum plant community on a green roof. HortScience 44, 401–407. doi: 10.21273/HORTSCI.44.2.401
Getter, K. L., Rowe, D. B., and Cregg, B. M. (2009). Solar radiation intensity influences extensive green roof plant communities. Urban Forestry Urban Greening 8, 269–281. doi: 10.1016/j.ufug.2009.06.005
Gong, Y., Zhang, X., Li, H., Zhang, X., He, S., and Miao, Y. (2021). A comparison of the growth status, rainfall retention and purification effects of four green roof plant species. J. Environ. Manage. 278, 1–10. doi: 10.1016/j.jenvman.2020.111451
Hawke, R. (2015). An evaluation study of plants for use on green roofs. Plant Evaluation Notes 38, 1–22. Available online at: https://www.chicagobotanic.org/downloads/planteval_notes/no38_greenroofplants.pdf
Huxley, A., Griffiths, M., and Levy, M. (1992). The New Royal Horticultural Society Dictionary of Gardening. London: MacMillan Press.
Kazemi, F., and Monorko, R. (2017). Review on the roles and effects of growing media on plant performance in green roofs in world climates. Urban Forestry Urban Greening 23, 13–26. doi: 10.1016/j.ufug.2017.02.006
Kotsiris, G., Nektarios, P. A., Ntoulas, N., and Kargas, G. (2013). An adaptive approach to intensive green roofs in the Mediterranean climatic region. Urban Forestry Urban Greening 12, 380–392. doi: 10.1016/j.ufug.2013.05.001
Kotsiris, G., Nektarios, P. A., and Paraskevopoulou, A. T. (2012). Lavandula angustifolia growth and physiology is affected by substrate type and depth when grown under Mediterranean semi-intensive green roof conditions. HortScience 47, 311–317. doi: 10.21273/HORTSCI.47.2.311
Liu, H., Kong, F., Yin, H., Middel, A., Zheng, X., Huang, J., et al. (2021). Impacts of green roofs on water, temperature, and air quality: A bibliometric review. Build. Environ. 196, 1–16. doi: 10.1016/j.buildenv.2021.107794
Lundholm, J., Heim, A., Tran, S., and Smith, T. (2014). Leaf and life history traits predict plant growth in a green roof ecosystem. PLoS ONE 9:e101395. doi: 10.1371/journal.pone.0101395
MacIvor, S. J., Ranalli, M. A., and Lundholm, J. (2011). Performance of dryland and wetland plant species on extensive green roofs. Ann. Bot. 107, 671–679. doi: 10.1093/aob/mcr007
Maclvor, J. S., Margolis, L., Puncher, C. L., and Carver Matthews, B. J. (2013). Decoupling factors affecting plant diversity and cover on extensive green roofs. J. Environ. Manage. 130, 297–305. doi: 10.1016/j.jenvman.2013.09.014
McAlister, R., and Rott, A. S. (2019). Upon the roof and down in the dirt: Differences in substrate properties (SOM, potassium, phosphorus and pH) and their relationships to each other between sedum and wildflower green roofs. PLoS ONE 14:e0225652. doi: 10.1371/journal.pone.0225652
Monterusso, M. A., Rowe, D. B., and Rugh, C. L. (2005). Establishment and persistence of Sedum spp. and Native Taxa for green roof applications. HortScience 40, 391–396. doi: 10.21273/HORTSCI.40.2.391
Nagase, A., and Dunnett, N. (2010). Drought tolerance in different vegetation types for extensive green roofs: Effects of watering and diversity. Landsc. Urban Plan. 97, 318–327. doi: 10.1016/j.landurbplan.2010.07.005
Nagase, A., and Dunnett, N. (2011). The relationship between percentage of organic matter in substrate and plant growth in extensive green roofs. Landsc. Urban Plan. 103, 230–236. doi: 10.1016/j.landurbplan.2011.07.012
Nagase, A., and Dunnett, N. (2012). Amount of water runoff from different vegetation types on extensive green roofs: Effects of plant species, diversity and plant structure. Landscape Urban Planning 104, 356–363. doi: 10.1016/j.landurbplan.2011.11.001
Nagase, A., and Dunnett, N. (2013). Performance of geophytes on extensive green roofs in the United Kingdom. Urban Forestry Urban Greening 12, 509–521 doi: 10.1016/j.ufug.2013.06.005
Naranjo, A., Colonia, A., Mesa, J., and Maury-Ramirez, A. (2020). Evaluation of semi-intensive green roofs with drainage layers made out of recycled and reused materials. Coatings 525, 1–19. doi: 10.3390/coatings10060525
National Center for Biotechnology Information NCBI (2021). Taxonomy Browser. Available online at: https://www.ncbi.nlm.nih.gov/Taxonomy/Browser/wwwtax.cgi (accessed February 28, 2021).
Nektarios, P. A., Amountzias, I., Kokkinou, I., and Ntoulas, N. (2011). Green roof substrate type and depth affect the growth of the native species Dianthus fruticosus under reduced irrigation regimes. HortScience 46, 1208–1216. doi: 10.21273/HORTSCI.46.8.1208
Nektarios, P. A., Kokkinou, I., and Ntoulas, N. (2021). The effects of substrate depth and irrigation regime, on seeded Sedum species grown on urban extensive green roof systems under semi-arid Mediterranean climatic conditions. J. Environ. Manage. 279, 1–13. doi: 10.1016/j.jenvman.2020.111607
Ntoulas, N., Nektarios, P. A., Kotopoulis, G., Paraskevas, I., and Ttooulou, T. (2017). Quality assessment of three warm-season turfgrassed growing indifferent substrate depths on shallow green roof systems. Urban Forestry Urban Greening 26, 163–168. doi: 10.1016/j.ufug.2017.03.005
Oberndorfer, E., Lundholm, J., Bass, B., Coffman, R. R., Doshi, H., Dunnett, N., et al. (2007). Green roofs as urban ecosystems: ecological structures, functions, and services. Bioscience 57, 823–833. doi: 10.1641/B571005
Ondoño, S., Martínez-Sánchez, J. J., and Moreno, J. L. (2016). The composition and depth of green roof substrates affect the growth of Silene vulgaris and Lagurus ovatus species and the C and N sequestration under two irrigation conditions. J. Environ. Manage. 166, 330–340. doi: 10.1016/j.jenvman.2015.08.045
Palermo, S. A., Turco, M., Principato, F., and Piro, P. (2019). Hydrological effectiveness of an extensive green roof in mediterranean climate. Water 11:1378. doi: 10.3390/w11071378
Panayiotis, N., Amountzias, I., Kokkinou, I., and Ntoulas, N. (2011). Green roof substrate type and depth affect the growth of the native species dianthus fruticosus under reduced irrigation regimens. HortScience 46, 1208–1216.
Papafotiou, M., Pergialioti, N., Tassoula, L., Massas, I., and Kargas, G. (2013). Growth of native aromatic xerophytes in an extensive mediterranean green roof as affected by substrate type and depth and irrigation frequency. HortScience 48, 1327–1333. doi: 10.21273/HORTSCI.48.10.1327
Papafotiou, M., Tassoula, L., Liakopoulos, G., and Kargas, G. (2016). Effect of substrate type and irrigation frequency on growth of mediterranean xerophytes at extensive green roofs. Acta Hortic. 1108, 309–316. doi: 10.17660/ActaHortic.2016.1108.41
Paraskevopoulou, A. T., Zafeiriou, S., and Londra, P. A. (2021). Plant growth of Atriplex portulacoides affected by irrigation amount and substrate type in an extensive green roof system. Ecol. Eng. 165, 1–11. doi: 10.1016/j.ecoleng.2021.106223
Provenzano, M. E. (2011). Plant Agronomic Performance and Selection for Green Roofs Under Mediterranean and Maritime Climate Conditions. [dissertation thesis]. Università degli studi della Tuscia.
Raimondo, F., Trifilo, P., Lo Gollo, M. A., Andri, S., Savi, T., and Nardini, A. (2015). Plant performance on Mediterranean green roofs: Interaction of species-specific hydraulic strategies and substrate water relations. AoB Plants 7:plv007. doi: 10.1093/aobpla/plv007
Rogers, K. (2019). Biophilia Hypothesis. Encyclopædia Britannica Online. Available online at: https://www.britannica.com/explore/savingearth/the-biophilia-hypothesis
Rowe, D. B., Getter, K. L., and Durhman, A. K. (2012). Effect of green roof media depth on Crassulacean plant succession over seven years. Landscape Urban Plan. 104, 310–319. doi: 10.1016/j.landurbplan.2011.11.010
Rowe, D. B., Kolp, M. R., Greer, S. E., and Getter, K. L. (2014). Comparison of irrigation efficiency and plant health of overhead, drip, and sub-irrigation for extensive green roofs. Ecol. Eng. 64, 306–313. doi: 10.1016/j.ecoleng.2013.12.052
Takahiro, S., Michio, K., Yuichi, U., and Noboru, I. (2010). Evaluation of growth and green coverage of ten ornamental species for planting as urban rooftop greening. J. Japan. Soc. Horticultural Sci. 79, 69–76. doi: 10.2503/jjshs1.79.69
Tassoula, L., Papafotiou, M., Liakopoulos, G., and Kargas, G. (2015). Growth of the native xerophyte Convolvulus cneorum L. on an extensive mediterranean green roof under different substrate types and irrigation regimens. HortScience 50, 1118–1124. doi: 10.21273/HORTSCI.50.7.1118
Thuring, C. E., and Dunnett, N. (2014). Vegetation composition of old extensive green roofs (from 1980s Germany). Ecol. Proces. 3:4. doi: 10.1186/2192-1709-3-4
United States Department of Agriculture (2020). Plants Database. Available online at: https://plants.usda.gov/java/ (accessed November 15, 2020).
Vangergrift, D. A., Rowe, D. B., Cregg, B. M., and Liang, D. (2019). Effect of substrate depth on plant community development on a Michigan green roof. Ecol. Eng. 138, 264–273. doi: 10.1016/j.ecoleng.2019.07.032
Vasl, A., Shalom, H., Kadas, G. J., and Blaustein, L. (2017). Sedum – Annual plant interactiosn on green roofs: Facilitation, competition and exclusion. Ecol. Eng. 108, 318–329. doi: 10.1016/j.ecoleng.2017.07.034
Vestrella, A., Save, R., and Biel, C. (2015a). An experimental study in simulated greenroof in mediterranean climate. J. Agri. Sci. 7, 95–111. doi: 10.5539/jas.v7n4p95
Vestrella, A., Save, R., and Biel, C. (2015b). Performance of two simulated green roofs in the mediterranean area. J. Agri. Sci. 7, 59–75. doi: 10.5539/jas.v7n11p59
Wang, H., Zhang, K., Gan, L., Liu, J., and Mei, G. (2021). Expansive soil-biochar-root-water-bacteria interaction: Investigation on crack development, water management and plant growth in green infrastructure. Int. J. Damage Mech. 30, 595–617. doi: 10.1177/1056789520974416
Williams, N. S. G., Hughes, R. E., Jones, N. M., Bradbury, D. A., and Rayner, J. P. (2010). “The performance of native and exotic species for extensive green roofs in Melbourne, Australia (Acta Hort. 881),” in Procedings of the IInd International Conf. on Landscape and Urban Hort. doi: 10.17660/ActaHortic.2010.881.113
Xie, L., Lehvavirta, S., and Valkonen, J. P. T. (2020). Case study: Planting methods and beneficial substrate microbes effect on the growth of vegetated roof plants in Finland. Urban Forestry Urban Green. 53, 1–12. doi: 10.1016/j.ufug.2020.126722
Young, T., Cameron, D. D., Sorrill, J., Edwards, T., and Phoenix, G. K. (2014). Importance of different components of green roof substrate on plant growth and physiological performance. Urban Forestry Urban Green. 3, 507–516. doi: 10.1016/j.ufug.2014.04.007
Keywords: Mediterranean green roof, plant growth, substrate depth, organic content, modular and open system, sustainable cities, succulents
Citation: Marouli C, Savvidou P, Koutsokali M, Papadopoulou P, Misseyanni A, Tsiliki G and Georgas D (2022) Plant Growth on a Mediterranean Green Roof: A Pilot Study on Influence of Substrate Depth, Substrate Composition, and Type of Green Roof. Front. Sustain. Cities 3:796441. doi: 10.3389/frsc.2021.796441
Received: 16 October 2021; Accepted: 08 December 2021;
Published: 21 January 2022.
Edited by:
Fanhua Kong, Nanjing University, ChinaReviewed by:
Sisay E. Debele, University of Surrey, United KingdomYuan Shi, The Chinese University of Hong Kong, Hong Kong SAR, China
Copyright © 2022 Marouli, Savvidou, Koutsokali, Papadopoulou, Misseyanni, Tsiliki and Georgas. This is an open-access article distributed under the terms of the Creative Commons Attribution License (CC BY). The use, distribution or reproduction in other forums is permitted, provided the original author(s) and the copyright owner(s) are credited and that the original publication in this journal is cited, in accordance with accepted academic practice. No use, distribution or reproduction is permitted which does not comply with these terms.
*Correspondence: Christina Marouli, Y21hcm91bGkmI3gwMDA0MDthY2cuZWR1
†These authors have contributed equally to this work