- 1Department of Architecture and Built Environment, University of Nottingham Ningbo China, Ningbo, China
- 2Network for Education and Research on Peace and Sustainability (NERPS), Hiroshima University, Hiroshima, Japan
Floor Area Ratio, commonly known as FAR, is a primary planning metric in urbanism. It is commonly known as the ratio of accumulated built floor areas against the size of a site/plot. In recent years, China's rapid urban development has resulted in a substantial increase in FAR, particularly in residential areas. A notable shift from low-rise row housing typology to a more common high-rise residential unit is certainly a key factor behind the increase of energy use in many Chinese cities. This factor highlights the need for a sustainable energy planning strategy at the community level. As a novelty, this study reflects on the FAR changes in the context of China, and evaluates the impacts on energy use at the community scale. This study tests four FAR models of 1.0, 2.5, 3.0, and 4.0 and provides a computational modelling analysis, with a comparison analysis. FAR models of 1.0 and 4.0 are typical models of low-rise and mid-to-high rise, respectively. Findings suggest FAR 4.0 as the least efficient for energy planning while FAR 1.0 is the least economically viable model. The results support policy development on FAR monitory, and paradigm shifts for future urban development models, particularly for the small to medium-scale residential compounds in cities.
Introduction
The progressive urbanisation, while positive, also comes with distinct social, economic, environmental, and technological challenges (United Nations, 2014). From the urbanisation context, two major issues become imminent: (1) lack of information and uncertainty surrounding the development of newly formed or future urban zones; and (2) creating the opportunity to utilise sustainable design for form new cities and urban blocks. The latter is important in addressing and achieving urban sustainability in cities and city environments. Such consideration entails a thorough understanding of environmental impacts from urban development and rapid urbanisation growth. Thus, population increase and concentration in new residential zones affect the formation of policies and the local governance of the region (Wang et al., 2015). Such impacts include changes in urban land use patterns, urban development models, and the potential pathways sustainable planning and design are directed. Higher density, often mistaken with compact design, is a major indicator that suggests changes in land use policy, densification of cities, and redevelopment of older communities with new models of urban development. In particular, this applies to residential communities and compounds, where we see a growing trend of increasing urban energy demand and higher-level concentration of population, lack of green space, and high-density living environments.
One of the leading countries in the densification of urban environments is China, a country known for its rapid urbanisation rate in recent years and decades. The progressive urbanisation plans have also led to increasing energy consumption, climate change impacts, and policy and local governance challenges. Many Chinese metropolitan regions and cities have experienced and are still experiencing rapid economic growth and urbanisation; most of which are under significant development and redevelopment processes. As a result, this approach has led to the expansion of built-up areas in city environments, many of which doubled their built-up areas between the 1990s and 2000s. The same trend is reoccurring in small and medium scaled cities, since the start of the New-Type Urbanisation Plan (NUP) in 2014. This rapid change has occurred in the atmosphere of higher energy prices and the established linkage between GHG emissions and climate change (Que, 2007). Such key factors make the study of urban form and policy quite imperative, as both processes of urbanisation and sub-urbanisation directly link to the expansion of built-up areas and towards higher-level energy use and volume of greenhouse gas emissions in city environments (The World Bank., 2008). It is worth pointing out that energy consumption has not been the primary motivator for spatial planning, particularly in the context of China. In fact, in most cases, we see general neglect by relevant stakeholders, such as developers and planners (Cheshmehzangi et al., 2021). This urbanisation process is commonly led by five features of (i) rapid rural-to-urban migration; (ii) increased living standards; (iii) restructuring of the economy in response to integration in the global market and the conversion of high-density city cores to modern central business districts; (iv) change in transportation system from the public to private use; and (v) relocation of industries to economic zones (Cervero, 2001; Chan, 2007; Cheshmehzangi, 2016). These rationales consistently lack the motivation to include energy in spatial planning, let alone its relationships to considering urban form, urban layout, and energy consumption patterns in cities.
Globally, key sustainable development initiatives are growing in cities, which are increasingly influenced by policy (Roldán-Fontana et al., 2015). Among these initiatives, urban planning and spatial optimisation play important roles in reducing emissions and optimising energy performance at the urban level. The study of sustainability measures to mitigate GHG and optimise the energy use of cities is both multidisciplinary and a multi-sector effort. Therefore, a dynamic and integrated approach is needed to optimise land use and urban forms in cities (Pacheco-Torres et al., 2017). This should not only be driven from economic and social perspectives, but also from an environmental-energy oriented perspective. Hence, this study takes an insightful look into “urban form” in the form of spatial configuration and planning in the City of Ningbo in East China as a case study. This approach enables us to tackle current policy and practise paradigms, arguing against the recent and current trends of urban development. The City of Ningbo represents many cities in China, where increasing densification has led to major environmental and urban energy demand challenges. A similar trend is also seen in other developing countries, where densification and in-fill urban development are commonly practised without a robust planning framework and policies against higher-density urban living environments. In this research study, various Floor-Area-Ratios (FARs) testing will provide an overview of energy efficiency in residential layouts. This study investigates several spatial configurations and their impact on energy consumption at various FARs. In addition, a pre-assessment strategy is developed to help planners with clear directives or options to utilise FAR in urban planning from an environmental perspective compared to the economic perspective, which is usually the norm. The findings feed into the development of policies and practise paradigms related to urban development models and future densification plans in developing cities around the world.
In sum, this paper highlights the use of FAR as a viable indicator of energy efficiency while also recognising the complexity of the variables involved and the importance of the geographic and climatic contexts. When one considers the astounding volume of ongoing new residential development to be constructed across the globe in the next few decades (i.e., particularly in developing cities), we must have an empirically grounded grasp of more energy-efficient building configurations and spatial layouts. Also, such findings would help policy, and practical tools planners and developers could use to improve urban energy efficiency. The findings would reflect on community-level sustainable energy planning strategies, particularly for new residential compounds commonly built at a higher density, and/or are replace older low-density residential communities. Such changes require a more careful analytical view for future policy and practise adjustments and innovations.
Implications of FAR on Energy Planning
Originally, FAR was used as a zoning limit to hinder the number of people a building can hold; i.e., density or occupancy ratio (Cheshmehzangi, 2016). Historically, it was utilised in cities like New York, during the nineteenth century, to prevent tall buildings from blocking natural lighting and ventilation. Therefore, FAR simply refers to the division of a building's usable floor area by the site or land plot in which it sits (Joshi and Kono, 2009). According to Joshi and Kono (2009), in the urban context, negative externalities are associated with human living and development. These include population externality, building height externality, and other externalities (such as, landscape deterioration and racial tension). Historically, FAR addresses both population and building height externalities, such as insufficient sunlight exposure. Additionally, from the perspective of a commercial building, FAR is the preferable metric used and is continuously used in the planning sector. Other parameters have also been used to guide urban development, which include the surface to volume (S/V) ratio and surface coverage (SC) area (Bassam, 2002). However, FAR as a primary metric, explicitly removes the volume from the equation (Bassam, 2002). This is said to reward buildings with less floor-to-ceiling height. It is also because most building typologies (e.g., offices, schools, retail) do not require volume ratios of such and are not sold or leased on volume bases but rather based on usable floor area as the most important factor (Cheshmehzangi and Butters, 2016). In this respect, energy as a parameter has not been utilised as a precursor to utilise FAR in planning.
Further investigation also indicates its use as a “density regulation,” which means that cities impose plot size zoning and floor area regulations to control commercial and population activities. However, FAR as a regulation, cannot adjust or affect population density in an area, but it can control the available total floor place without controlling per capita floor space consumption. Thus, reducing FAR may not necessarily have an effect on reducing the number of residences unless a separate set of policies are developed for this particular factor (Cheshmehzangi and Butters, 2016). In a city like Ningbo, for instance, a policy of FAR of 3.0–5.0 is informed based on the contextual externalities such as, population control and economic progress (Bassam, 2002). So far, little research has explicitly utilised FAR as a parameter for energy analysis, particularly for residential compounds in places where higher urban density is introduced.
FAR Functionalities on Urban Form and the Built Environment
The use of FAR guided by the thermal and energy consumption consequences, has yet to be fully visualised and utilised as a working principle to set relevant policies and monitory FAR standards. This study draws from the aforementioned gap and takes a step further to develop a pre-assessment energy planning strategy to implement FAR as a planning metric that considers passive energy design and energy consumption in the urban residential compounds.
With a large population in high dense city environments, optimal urban land use becomes necessary in countries like China. This factor is combined with three key aspects of the scarcity of arable land, increasing energy cost, and accumulation of environmental pollution comprised of water, air, and soil pollutions (Dawodu and Cheshmehzangi, 2016). It can be verified that FAR is at the forefront of community development and planning strategies (Holden and Norland, 2005; Aggarwal, 2006; Jabareen, 2006; Johansson, 2006; The World Bank., 2008). Consequently, such an approach can fortify two key principles of density and energy management and through better planning and consideration of FAR in practise. Hence, the assessment conducted in this study utilises FAR not only as a key planning metric, but also towards a directive tool for energy impact analysis and based on specific urban forms.
There is a clear relationship between urban form and its impacts on energy use from the existing literature. Since the oil crisis era of the early 1970s, studies of urban form shaped around issues of planning and energy consumption (LSE, 2011) and developed towards various comparative analysis, detailed design assessments and towards modelling in more recent years. A pioneer in this field is Steadman (1979), who theorised the relationship and implications of large-scale urban form in relation to energy implications. The study considered both line and blob formation with key research findings suggesting a variety of configurations and integrated models, including the argument that a combined model of high-density growth in line with transportation infrastructure is more efficient than a centralised dense growth. Originally, the consideration of urban form in research, especially during the era of the oil glut, has resulted in a stronger impact on transportation development (Owens, 1986, 1998). In fact, research by Newman and Kenworthy (1989) and Næss (2001) further solidified the impacts and high association of urban density on the use of gasoline. Results included increased density equalled reduced energy consumption by transport. Therefore, the closer people are to their jobs, the greater the vehicles' miles travelled are reduced and the reduction in freeway traffic and tailpipe emissions. More importantly, location is a critical factor in car dependency and energy consumptions (Newman and Kenworthy, 1989; Cervero, 1996; Banister et al., 1997).
Focused on urban geometry that involves factors like density and spatial configuration, Baker and Steemers' study (Baker and Steemers, 2003) concludes that this specific parameter affects solar radiation incident on building envelope, microclimate and ventilation, wind speed, and airflow patterns around buildings (also see Larivière and Lafrance, 1999). A further study by Yamaguchi et al. (2007) quantifies the effects of spatially planning cities and neighbourhoods. In addition, the key design parameter of orientation, and not only positioning, affects solar access wind speeds and airflow. On the other hand, by observing building configurations from a historical perspective, Gropius (1965), a German architect, investigated the concept of rational building layout that ensured daylighting and ventilation (dated back to the 1930s). The research led to three rules for parallel blocks layout, which considered four variables. These variables included population density on each block, the site area, the number of storeys, and the incidence of the angle of sunlight at the bottom of the building during the winter period. The three rules are as follows:
1) Within specific land area and solar incidence, the number of inhabitants increases as the number of building storeys increase;
2) On a specific solar incidence and number of residence, the size of the required site decreases with an increasing number of storeys;
3) On a specific site and a specific number of inhabitants, solar incidence reduces with an increase in the number of storeys.
Moreover, these rules led to observations by Gropius (1965), suggesting that buildings of 10–12 storeys were more energy-efficient than 3–4 storeys. Another specific argument from Gropius (1965) was that open spaces increase with block heights. However, when considering the scope of this research and context it involves, certain observations may or not be true. Firstly, climatic factors need to be considered, especially when building heating and cooling are required. Secondly, though surface or open space may increase with the height of building blocks, this does not consider the current population and rapid urbanisation in contexts like China. Therefore, this leads to smaller surface areas but with an increasing number of storeys in building heights. These two variables (i.e., population and land scarcity) make China's case of spatial configuration, density, and FAR particularly attention-grabbing for planning and policy measures. Furthermore, contemporary research on cities argue that density equals efficiency (Holden and Norland, 2005). However, research began to investigate the shortcomings of this logic, in which existing research highlight the inefficiencies of density on natural solar gains and ventilation (Compagnon, 2004; Johansson, 2006). For instance, the argument of compact form vs. dispersed urban form is debated (such as Mindali et al., 2004; Holden and Norland, 2005, and many others), suggesting density is equivalent to energy efficiency with its adverse effect on key aspects of solar gains, natural lighting, and ventilation (Hui, 2001; Mindali et al., 2004; Dawodu and Cheshmehzangi, 2016). This also suggests a trade-off between the two concepts and the possibility that density and efficiency are not necessarily causal (Larivière and Lafrance, 1999; Steemers, 2003).
As addressed in LSE's report (LSE, 2011), many findings in various research associated with studies of urban form and varied configurations have developed further with computational simulation studies and programmes that address spatial analysis and urban morphologies. Since the early 2000s, some of these programmes have contributed towards knowledge enhancement on the analysis of solar calculations and wind flow as well as calculations and modelling of energy demand and consumption in urban areas (Steemers, 2003; Ratti et al., 2005). Prior to those studies, scholars like Yannas (1994) studied a variety of housing typologies, including detached units and apartments, and observed up to 40% of heat savings by assessing various densities. Key conclusions of those studies suggest FAR 2.5 as a potential optimum density model for neighbourhood planning (Yannas, 1994), which was later taken into consideration for some European housing studies (LSE, 2011). Similarly, studies by Capeluto and Shaviv (2001) on a variety of densities, identified that FAR between 1.6 and 1.8 offers a reasonably suitable solar access to all buildings within a neighbourhood (Capeluto and Shaviv, 2001; Arboit et al., 2008; Dawodu and Cheshmehzangi, 2016). Subsequently, scholars like Montavon et al. (2004) modelled various building layouts and assessed the effects of urban layout on solar collectors across cities of Switzerland. Such evidence-based studies further developed towards the provision of urban planning guidelines on solar access that emphasised the importance of configuration of urban blocks, orientation, and energy-oriented aspects of urban form. These are identified as key factors for the context of China, where rapid urban development, particularly in two specific modes of redevelopment and infill development, has led to the substantial increase of average FARs in many Chinese cities. Such increase, especially since the early 2000s, reflects on what LSE (2011) has concluded in their research that China's urban land usage has progressively become a crucial factor to economic development, with little concerns over optimising associated aspects, such as energy consumption and thermal comfort. Such aspects can be simply addressed by better understanding FAR variations that apply to the overall planning and design of urban form. This study reflects this necessity and contributes to existing literature from the FAR perspective and towards sustainable energy planning strategies for residential compounds and future urban planning and design policies and practise paradigms.
Motivation and Aims
The literature review verifies that various researchers well-cover the investigation of urban configuration. Furthermore, the optimisation of urban configuration through computational modelling has made researchers take a closer look at methods of reducing heating and cooling demand in buildings and power generation. The utilisation of various parameters has been used to investigate the relationship that exists between spatial configurations and energy performance. Similarly, LSE's study (LSE, 2011) concludes that there is still a general lack of study on urban morphology and thermal performance. This study, however, investigates a specific aspect of urban form termed FAR, which is important as it quantifies and ascribes useful and implementable meaning to the utilisation of land area for development. One could argue that this parameter is synonymous with density; but FAR is utilised as a planning metric, and density is not. Further to this, the arguments are supported through a case by utilising a particular community setting in the City of Ningbo for this investigation. This will be addressed by the development of a pre-assessment strategy for optimisation of city planning and energy reduction techniques. In this respect, the study aims to connect FAR and energy efficiency factors in the built environment, using a residential compound as an example. Thus, the objectives of this study are:
a) to verify that FAR plays a significant role in building envelope energy consumption and identifying the indicators that affect such consumption. This would be validated with the use of pre-assessment software for modelling (i.e., EcoTect software).
b) to create a strategy to guide city planners in the pre-feasibility stage of urban development that does not just consider population distribution and commercialisation; and
c) energy optimisation, while using a quantifiable FAR metric as a primary catalyst for urban development.
Methodology and Case Study Research
The study is processed in four steps. The first is through case study research, which is the central part of the study. Selective pre-assessment research is conducted to develop a baseline with the consideration of geographical and climatic data, site analysis study, and demographic assessment. The latter includes a general evaluation of a residential compound's estimated size, urban layout, and population. The second step is to develop a representational model of the site, used as the baseline of the selected study area in the City of Ningbo, China. The third step is to develop several models of the site with different FARs and surface coverages. Using mainly the FAR variation, the study then focuses on the computational simulation of each model. The fourth step is an evaluation based on the simulated models, allowing us to do comparative analysis and study the energy performance and energy impact of each FAR model (see Figure 1). An additional model is then proposed to strengthen the case study research and analysis.
The research case study is situated in the City of Ningbo, which is located in the Southeast coastal part of China. The climate in Ningbo is categorised as a subtropical monsoon climate, which boasts warm and moist weather in summer, with cold winters (i.e., Hot Summer and Cold Winter—HSCW). Additionally, the location of the case study is in the immediate inner-city area of Ningbo, located next to one of the three main rivers of the city. The development is a mixture of low to mid-rise buildings and mid to high-rise buildings. This configuration goes against the common development sizes in China, which are predominantly high-rise residential compounds. The introduction of this combination is based on studies by Cheshmehzangi et al. (2016), who highlight the advantages of low to mid-rise buildings. The study points out that low to mid-rise typology provides shading for most of the housing units, is highly performative, and has reasonable density for the development area. This model has the potential to enhance ground spatial functionality and more land-use dedication to green spaces. High-rise buildings could simply claim higher provision of land-use (unless restricted with less surface coverage regulations) with no other specific functional advantage (Cheshmehzangi et al., 2016). Based on these observations, a mixed baseline model was created. Figures 2, 3 below show the case study location and baseline model.
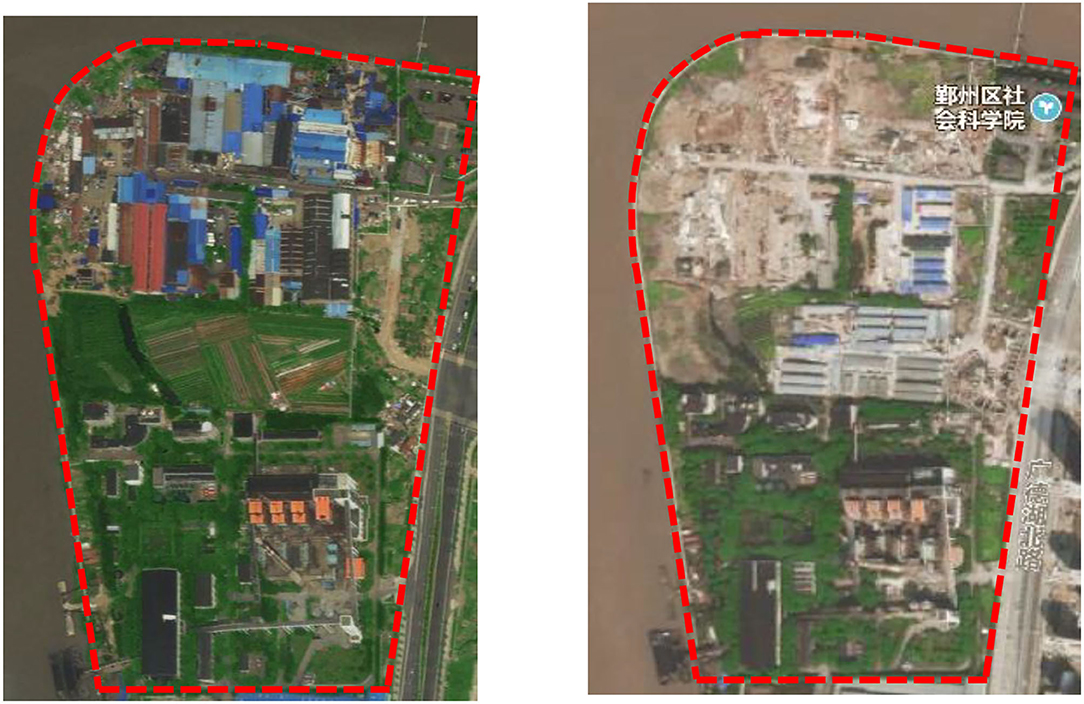
Figure 2. Plan of site area through Baidu Map (left: status in 2013 prior to redevelopment phase; right: status in 2017, which is now under construction as of 2021) (source: authors' own, extracted from Baidu local maps).
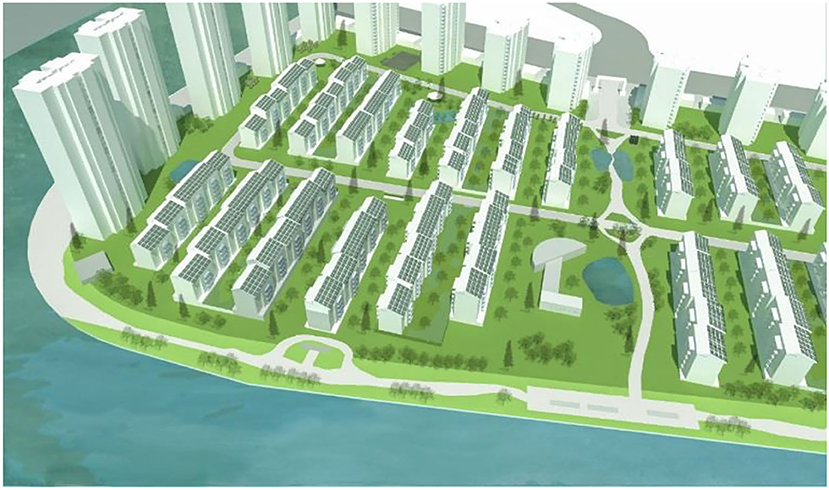
Figure 3. Proposed model of development based on the given criteria of the site for a low-rise model (source: authors' own).
The site has been previously occupied by three industrial units, (1) a small size steel company, (2) a thermoelectricity company, and (3) a concrete processing company. The northern edges of the site include several restaurants and food companies.
The study is based on various formation and spatial placement of building blocks to evaluate impacts on energy use patterns at the community level. This study uses only a typical row layout widely seen in most Chinese cities of the South of Yangtze River Zone as opposed to the perimeter blocks of European cities. The Chinese residential compounds follow the row housing layout of south-north orientation and are often combined with mid to high-rise typologies surrounded by a podium of commercial and retail use (Figure 3). This study explicitly studies this very common typology, in terms of its spatial configuration, design layout, and arrangement. Hence, the investigations here are to identify suitable FAR models as an example for an optimised energy design at the meso-scale. This is based on using FAR modelling of the site, reflecting on some of LSE's key findings (LSE, 2011) that argue in favour of FAR 1.5–2.5 as the most energy-efficient FAR models. Their studies provided this particular finding from a range of analyses conducted from a spatial planning perspective, which is also utilised here as the basis of FAR calculations and variations (Dawodu and Cheshmehzangi, 2016). However, this study focuses on a developing city context, where rapid urbanisation and increasing population adds to the spatial planning conundrum. In the literature and existing research, we see that the full extent of energy implications has not been fully investigated at the meso level. This study also provides a set of tested models for FARs of higher values, such as 3.0 and 4.0, where we see common practises of higher FAR planning. These FAR values are mid-to-higher FAR values in China and are common planning guidelines in cities like Ningbo. The lower FARs and much higher FARs are not tested intentionally to avoid repeating research that suggests lower energy performance of very low and very high FARs.
Based on the original baseline model, four various FAR configurations are also developed. These variations include FAR 1.0 as the current low to mid-rise typology, 2.5 as the highest recommended FAR by LSE's study (2011), 3.0 and 4.0 as two variations of higher FARs and associated with current planning practises in Chinese cities. These models are summarised in Figure 4. Except for FAR 4.0, the spatial arrangements are based on the development of useful floor areas in a given site. The models are typical examples of practises in the planning and design of residential compounds of such scale in China.
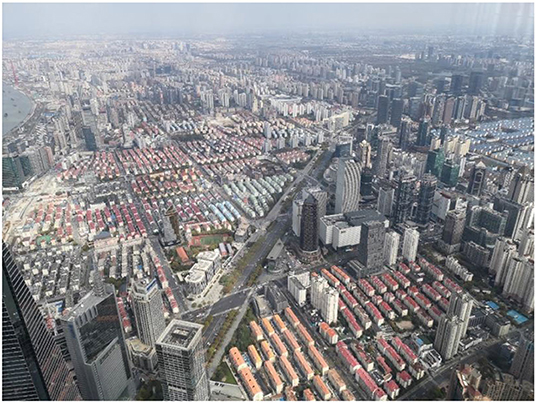
Figure 4. A typical arrangement of mid to high-rise housing compound with a podium of commercial/retail units on the edges and north-south orientation (source: authors' own).
Considering the local planning specifications and regulation constraints, 30 floors are considered the maximum given height for residential towers of all models. In order to meet optimum FAR figures (Table 1), additional buildings were added for the model of FAR 4.0. For maintaining consistency in modelling scenarios, all parameters not related to form are kept constant in the simplest form. This is intentionally done to ensure comparison analysis is accurate between the developed models of various FARs. These parameters include data on geographical and topographical conditions, as well as climatic conditions that used the nearest possible weather station to the site location. The study's simulation work is conducted by Autodesk EcoTect 2011. These are done for indirect solar radiation simulation and assessment of heat loss and heat gain of block units. This leads to a simulation of average cooling and heating demands based on the overall usable floor area and per m2. The FAR variations are utilised for this purpose where it is possible to assess a wide range of energy-related factors. Models are kept with the same parameters for wall construction materials without considering detailed technological specifications, such as appliances and other energy supplies.
As EcoTect software is mainly effective as a computational simulation toolkit at the early design stage (Dawodu and Cheshmehzangi, 2016), relative differences are fully considered as part of design variations. Such variation is considered for the comparison of cooling and heating demands associated with the various spatial configuration at the meso-scale. Hence, these simplified modelling arrangements suggest related energy use as the relative values of the models. Therefore, these are merely utilised based on various urban forms that are linked to FAR variations and heights of those modelled residential units. Another major parameter studied here is indirect solar radiation. This is recognised as a solar radiation incident upon building walls (Dawodu and Cheshmehzangi, 2016). This parameter helps to evaluate energy use through FAR metrics. As a methodology, computational modelling approaches at the meso-scale are used as instructive tools for design and planning development (Cheshmehzangi, 2016). Nevertheless, we note the main modelling limitation, which needs is minimised simplification of the actual models with fixed parameters and constant conditions.
Results
The results of the study indicate indirect solar radiation as an important finding in terms of FAR variations. As indicated in Figure 5, a larger quantity of solar radiation is expected for a larger surface area of the building envelope, i.e., in the example of FAR 4.0. Evidently, this is associated with volume exposures that are much lower in the model of FAR 1.0 with the least radiation. Hence, height reduction in both cases of high-rise blocks and low-rise row housing units plays a major part in making such variation of FAR models (see Figure 4). On the other hand, direct solar radiation is assessed through EcoTect modelling of various FARs. This is recognised as radiation that accesses the internal areas of a building (Dawodu and Cheshmehzangi, 2016) while indirect solar radiation suggests an estimation of radiation in terms of energy absorbed by the building materials. Therefore, this links to the total usable floor area of a particular development, which is calculated per m2 in the computational models. Hence, the results suggest an increase of radiation coverage based on the increase in FAR, which again is associated to surface coverage increase per unit.
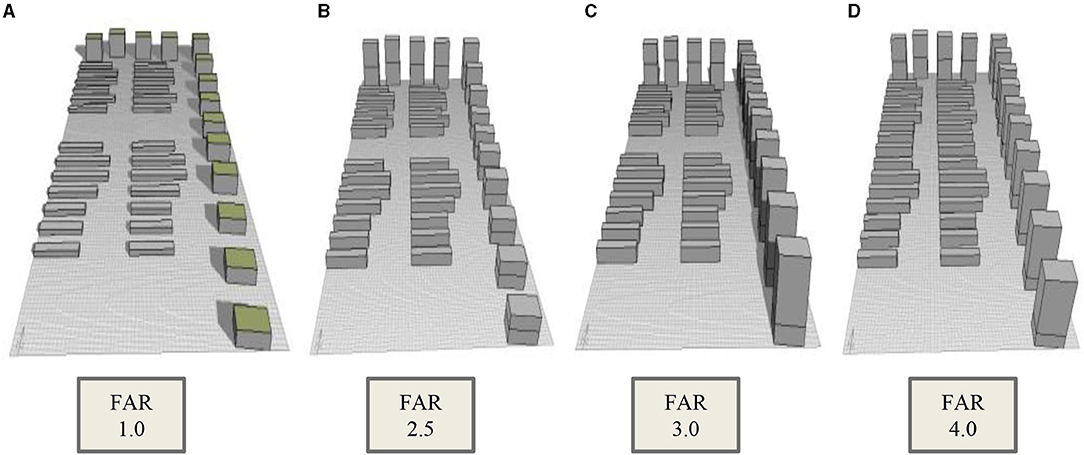
Figure 5. Left to right: (A) FAR 1.0 model; (B) FAR 2.5 model; (C) FAR 3.0 model; (D) FAR 4.0 model.
A key observation in this study is the similarity of radiation identified between FARs 2.5 and 3.0, with a slight difference in the heights of tower blocks. This is irrespective of FAR change at an insignificant rate of 0.5, which has a minimal impact on the overall building envelope energy consumption rates between the two FARs (i.e., 2.5 and 3.0). Also, based on comparative analysis of degree days, the results indicate FAR 4.0 with the highest heat gain of all, and equally with the highest heat loss that supersedes the overall heat gain figures (Figure 6). On the other hand, FAR 1.0 is the model identified with the lowest heat loss and heat gain rates. This result verifies climate as a significant factor in heat gain and heat loss variations. Furthermore, regarding the renewable energy aspect of energy planning of the site, the results also provide an overview for suggesting a specific type of development, particularly for the optimisation of solar thermal and PV panel use at the meso-scale planning. Hence, expanded assessment is conducted to first review heat gain and heat loss components, and then verify what factor impacts these variations through the analysis of FAR models.
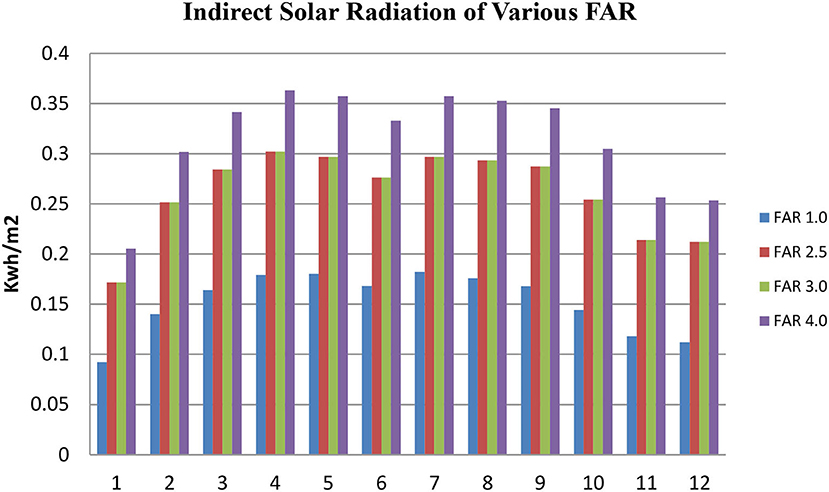
Figure 6. Indirect solar radiation for various FAR (y-axis: indirect solar radiation in Kwh/m2; x-axis: all months from January/1 to December/12).
As shown in Figure 7 above, FAR 1.0 is verified to have less ventilation and conduction losses than FAR 4.0. This result suggests the impact of height on the fabric losses, particularly when it couples with the climatic conditions of sub-tropical Ningbo. For validation purposes and confirming the results, a new FAR model 4.0 was developed with one major variation of height differences in comparison to the original model of FAR 4.0. In this regard, the height of tower blocks was reduced from 30 to 25 floors, but the FAR value was maintained by adding more low-rise row housing units. By increasing the overall unit density and reduction in heights of high-rise units, the new model offers an overall increase of surface coverage of the site (Figure 8).
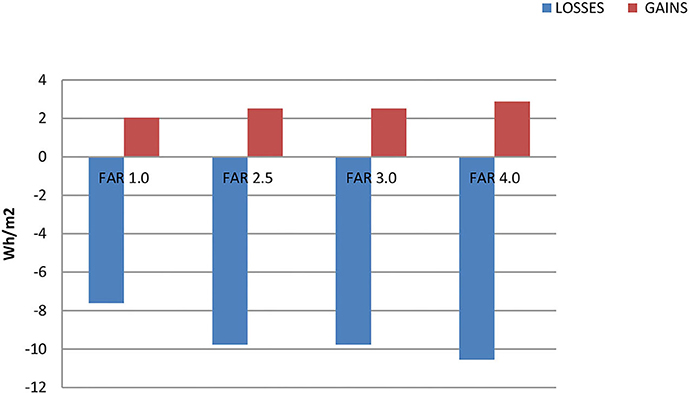
Figure 7. Annual Energy gains and Losses based on FAR (y-axis: annual energy losses and gains in Wh/m2; x-axis: FAR variations).
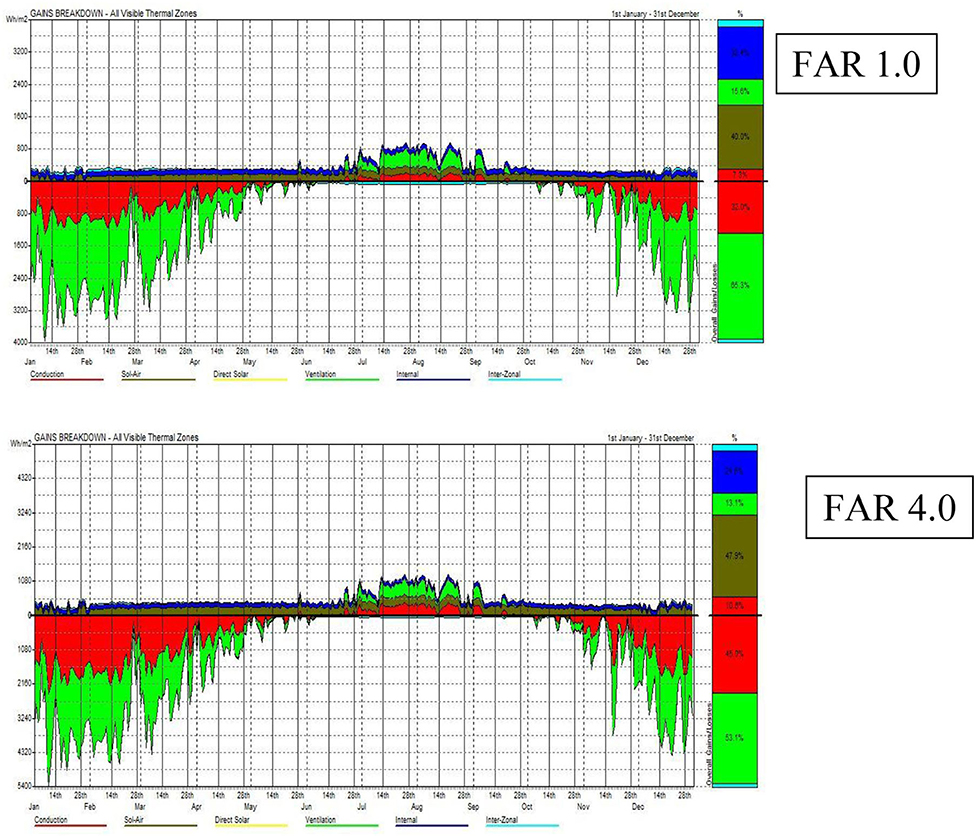
Figure 8. Passive building energy gains and losses breakdown for FAR 1.0 (top) and FAR 4.0 (below). Red, conduction; dark green, sol-air; yellow, direct solar; light green, ventilation; dark blue, internal; light blue, inter-zonal.
Based on the new assessment of this new model of FAR 4.0 (i.e., 2nd model of FAR), the heat loss is reduced by 8% while the overall heat gain is reduced by 7%. This verifies the earlier observation point from the comparative analysis of various FAR models that higher buildings provide for more losses and less heat gain. Thus, in a context like Ningbo, building heights could play a significant part in reducing ventilation and thermal losses. This is more evident when buildings require heating for a substantial time of the year (see Figures 6, 9). This factor affects energy use and associated costs, including environmental and financial factors of the site. In this regard, FAR 4.0 indicates the highest thermal gain in terms of indirect solar radiation. Similarly, this model also suggests the highest value of heat loss, suggesting more energy use on heating in comparison to cooling. Therefore, the findings from the analysis of various FARs suggests FAR 1.0 as the most energy-efficient model, but is not practically viable for rapidly urbanising contexts such as Ningbo or similar cities facing such rapid development.
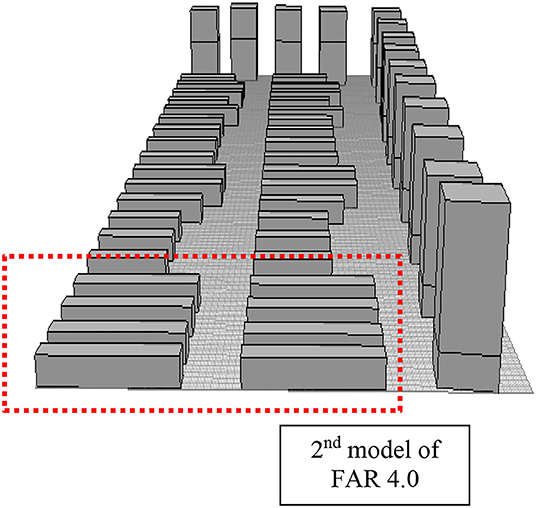
Figure 9. Second FAR 4.0 model. Reduced height of towers by five floors. Added eight more row housing blocks of up to six-storey at the south section (in red-dotted box).
Discussions
Energy Impact of Physical Parameters and Microclimate
Based on the results, we verify that other physical parameters should be considered, which has been primarily used to assess spatial configuration on energy impact. Martin and March (1972) and Ratti et al. (2005) identify the importance of S/V ratio as an indicator for compactness of buildings and can be used to determine heat loss within a building. Although the beauty of Martin and March's approach owed much to its generality; thus, it is widely accepted that the question “which urban forms make the best use of land?” cannot have an absolute answer. There are too many parameters to consider, each of which will affect the results. As stated by Oke (1988, p. 103), there are “almost infinite combinations of different climatic contexts, urban geometries, climate variables and design objectives. Obviously there is no single solution, i.e., no universally optimum geometry.” He then argues that fact should not be seen as a barrier, as we need to seek for general guidelines to respond to special needs and situations (Oke, 1988). Despite this, there are predominant urban types that are associated with certain climate types, such as the courtyard type in the hot-arid climate. Somewhat similar caveats, as in studies by Ratti et al. (2005) and Cheshmehzangi and Butters (2016), verify specific housing typologies for specific contexts. Even if these facts are acknowledged, it does not mean that we cannot sort out better from worse urban forms. In any case, we have to make choices; agnosticism about urban form is not an option.
To elaborate further, surface-to-volume (S/V) ratio means the ratio of the building envelope (external facades and roof) to the entire volume of that building (LSE, 2011). Minimising the S/V ratio leads to heat loss during the cold season. Still, it may increase consumption due to artificial lighting due to the minimisation of facades exposed to solar rays. Essentially, a smaller surface area means more heat gain/loss through the building blocks (Bassam, 2002). Hence, small S/V ratios imply minimum heat gains and losses, as it has been assessed and verified in this research study. According to Bassam (2002), in hot and dry climates, S/V should be low to minimise heat gains; and in a cold climate, it should also be low to minimise losses. In warm and humid climates, the primary concern is creating space for ventilation, which may not necessarily mean minimising the S/V ratio. Therefore, we can argue that to minimise heat transfer, a building envelope should be as compact as possible; hence, tending towards a cube shape (Bassam, 2002). However, the complex interrelationships of numerous variables makes this generalisation not as accurate. Bassam (2002) concludes that using the S/V ratio is not a correct indicator for complex building configurations due to complex relationships in an urban setting.
In this regard, if S/V and surface area are debatable indicators to use when looking at the complex relationships of the spatial configuration of buildings and its related energy impact, it is for best to identify which indicator should be utilised. Studies executed by Yang et al. (2012) utilise and evaluate parameters, such as block height, thermal performance, material conductivity, and surface albedo, which influence the thermal environment on courtyard in Beijing. Their study factored in both winter and summer periods, and the conclusion was that block height was observed to be the most important factor. Similar to this FAR comparison study, and from a microclimate perspective, solar radiation is identified as the most important microclimate parameter. When investigating this further, i.e., considering urban blocks in terms of courtyard, streets, and squares, it is important to note the height of buildings and street width (Johansson, 2006) as a key aspects in planning and design.
From the analysis, the effects of parameters, such as height, width, volume, and area, have clearly shown to have energy-related effects when considering urban form and configuration. From a microclimate perspective, ventilation, and solar radiation are key components that affect how spatial building configurations would contribute to cooling and heating. Thus, by emphasising solar access and design, we can argue in favour of parameters that direct building performance, i.e., density, orientation, and street layout. These parameters (also including building shapes, density, and site layout), if optimised, could ideally achieve an overall level of zero energy consumption (Sanaieian et al., 2014).
Several scholars have thoroughly investigated the field of solar access on urban blocks and its impacts on building performance (Johansson, 2006; Sanaieian et al., 2014; Ignatius et al., 2015). Several strategies have been developed to optimise or maximise solar access either for daylight or renewables; and minimise energy demand in buildings (Hachem et al., 2011a). Studies by Hachem et al. (2011a,b) have highlighted points on density and how to maximise solar access and identify the best place to avoid shading and benefits of street shapes. Further studies by Ghosh and Vale (2006) investigated the geometric form and the solar potential. Their study, executed in southern Sweden, concludes that urban morphology has significant impact on solar energy utilisation with further results indicating that sunlight access decreased by 10–75%, and when urban blocks are surrounded by other buildings. A similar scenario was tested and verified in this comparative study through shading variances of the same row-type housing layout.
As regards ventilation, airflow around buildings significantly affects energy use in buildings. Other factors that affect ventilation are the climatic conditions, which consider wind speed and wind direction. These can be tested separately and more accurately using different tools and computational software (Cheshmehzangi, 2016). Apart from microclimatic factors, the arrangement of adjacent buildings in relation to wind direction also strongly affects natural ventilation (Sanaieian et al., 2014). In their study, Bady et al. (2011) utilised a wind tunnel to investigate wind pressures on cubic buildings located within a high-density urban area. Four different geometries were analysed with varying directions of wind. Their study concluded that wind directions and building configurations significantly impact on natural ventilation within an urban area. This becomes an expedient factor when considering having a high-rise building, high densities, and compact configuration, which are predominantly utilised in urban China (Cheshmehzangi et al., 2010, 2016). Similarly, what has been tested here is the correlation between building height and density against the combination of surface coverage and density, where again FAR variations play a significant role in making a difference for better energy planning, including both productions and consumptions.
Utilisation of Floor Area Ratio for Community Planning and Design
Literature and the results from the simulation of various FARs in this study indicate the role that energy plays in land use. It is evident that passive energy and energy consumption of buildings as externalities can no longer be ignored in practise. To build on the results from this study in a practical form, it is vital to further provide a strategy that can be used by planners and policymakers and to decide best practises that suit specific development, either refurbishing/in-fill development or expanding a city. Figure 10 shows the prerequisite condition, which involves the context of the country, city, or neighbourhood in question. Generally, four pillars of sustainability govern how the built environment functions since the Brundtland report (Spangenberg et al., 2002; Dawodu et al., 2016). It is crucial to understand what a specific region is going through in terms of sustainability focus (Dawodu et al., 2016). Once that has been established, the “priority phase” (Phase 1—see Figure 10) is introduced. The addition here is the consideration of energy externality, which literature and the Ningbo case study results in previous validations as this affects height and density of development and translates to the energy consumed depending on the spatiality of design is the prerogative for establishing a particular FAR. Based on the context of the region, the percentage of consideration of each externality would vary. Still, the argument is to involve “energy externality” as a key player in urban planning and design.
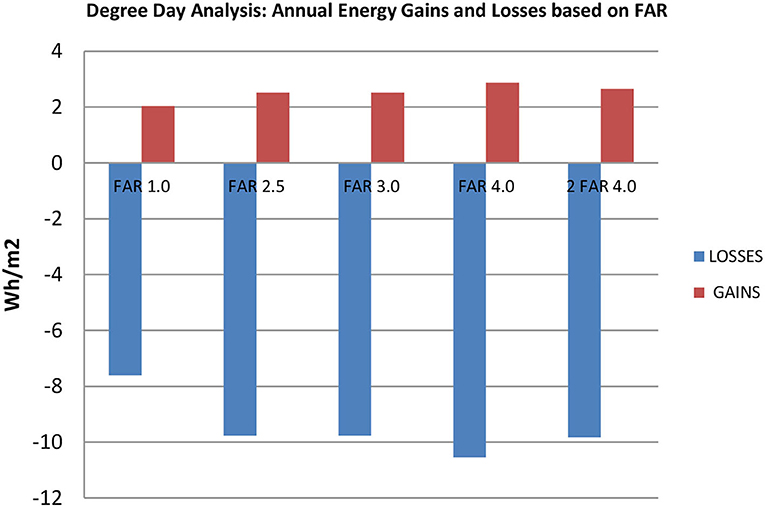
Figure 10. Degree day analysis—annual energy gains and losses based on FAR (y-axis: annual energy gains and losses in Wh/m2; x-axis: FAR variations, including second model of FAR 4.0).
Enhancing Methodology, Policy, and Practise Paradigms
The next phase (Phase 2: “methodology phase:—see Figure 11) involves utilising various computer simulation tools to predict the interaction of microclimate with the development and the energy implications. In addition, other parameters can be calculated; i.e., the number of floors for commercial and residential purposes and the optimal number of people to be housed. Finding the balance in the three considered externalities is essential and again depends on the prerequisite conditions.
Other important variables are policies and regulations that exist within the region. These policies usually depend on how flexible or rigid they can be to determine the degree of freedom a developer has to operate. However, from the results achieved in this study, one can see that the higher buildings are more prone to heat losses, particularly in colder environments/conditions. The energy externality can create an incentive to form a cap, i.e., stop building height at a point where it would be inefficient to build higher due to the sheer amount of thermal losses and the energy required to maintain thermal comfort. A sound example of this is the consideration of all priorities and methodology phase that leads to the development of various configurations, which would possess different FARs, similar to this study. At this point, critical analysis has to be done to illustrate the strength and weaknesses of each suggested FAR (Phase 3: “Data Analysis and Recommendation Phase” — see Figure 10). This leads to the final aspect of the “stakeholder(s) phase” (Phase 4—see Figure 10), in which optimum FARs are presented, depending on the stakeholders involved in making the selection. However, this selection is not arbitrary and is based on informed design considerations of prerequisites, priorities, methodology, and data analysis phase. This would ensure that stakeholders are not biassed in their decision, thereby selecting their options based on functionality that spans across all three externalities.
The importance of this strategy is that private developers can use it as a procedure and methodology to present to a potential client. It can also be used and enforced by policymakers as guidelines to ensure that all aspects of sustainability, particularly the energy aspect, are considered at the early stages of planning and design. In this study, the literature has shown this endeavour without using this methodology or similar considerations. It has been argued that it could be an otherwise commercial and socially dominated task. Therefore, it is argued that such use can be introduced and expanded in the development of other tools to enforce the environmental aspect of sustainability that is often easily neglected in these scenarios.
From a design perspective, the human dimension is given favourable by many urban designers and planners, as the “human scale” development, rather than the alienating high rise and skyscraper districts that are springing up all over the globe. Therefore, the study reinforces the arguments on medium-density urban fabric that is designed around walking and transit (as in Jeff Speck's Walkability City, Speck, 2018) or associated with particular patterns of urban design for living environments (as in Mehaffy and Salingaros, 2015; and Cheshmehzangi and Butters, 2016). It also verified factors concerning issues of climate change and energy matters that are addressed by Calthorpe (2013) in defining urban living environments. Accumulating these major works comes to the central argument of this paper, which is to suggest an energy-efficient urban form that can also, at the same time, be a more human-scaled and functional urban form.
To further discuss these key points, China is selected as the context of this paper, where the high-rise typology is already a major changing pattern of urban landscapes in cities (Cheshmehzangi, 2018). In such context, planning and design decisions are mainly based on the development of many tall buildings in geometrical rows, particularly north-south orientation due to local policy requirements of China. As a result, the existing evidence indicates there are some considerations of natural lighting and ventilation—but not enough—and energy use considerations are still very minimal compared to other urban design control measures. In such cases, energy use is neglected in favour of a simple building configuration/design strategy that is to maximise the number of units while minimising the overall building costs. This then ended with a preference for high-rises positioned in superblocks where a larger population can be accommodated with a smaller building footprint (Jabareen, 2006; Cheshmehzangi and Butters, 2016). In most cases, the building footprint or surface coverage of the site is lower than 30% of the overall block, hence, rationalising an approach for higher residential units. Taken into consideration as a major planning pitfall in China, Calthorpe (2013) highlights the mistake of building high-density sprawl, i.e., towers sitting amid a hostile and unwalkable ground level of busy arterials or unused spaces (Cheshmehzangi and Butters, 2016) that may seem green yet ineffective for social uses. Therefore, the geometrical configuration and row-type planning are developed through regulations in place on particular urban design metrics that may be recognised as the easiest form for long-term energy efficiency. Yet, contemporary design interventions show some differences in energy use and performance, particularly in the higher towers of dense compounds.
Conclusions
The results from various FAR modelling inform future directions for verification of density, building heights, and urban forms. The findings imply the strong contextual nature of FAR. Thus, the energy characteristics of FAR would vary amongst continents and countries at a larger scale and regions and cities at a relatively smaller scale. Secondly, there is a clear relationship between FAR, energy production, and energy consumption. For instance, wider surface areas and higher buildings allow for more indirect solar radiation, which is linked to higher gains. However, if buildings were too short, this would affect radiation coverage and the productivity of solar energy technologies, such as solar facades. In addition, based on the findings, we can verify that the 0.5 FAR variation needs to be investigated further. Nonetheless, current results indicate that when using FAR, an additional 0.5 FAR would bear no significant loss but would have business/economic advantages by increasing usable floor area (e.g., FAR 4.0 would be a better option compared to FAR 3.5). The results also suggest that an optimum height can be gauged in terms of energy losses and gains; in other words, the higher the building, the more thermal losses. This would be a good precursor in developing policies and strategies for land use using FAR variations.
Another investigation involves the combination of FAR with solar energy production to optimise not only energy consumption but also energy production. Ultimately, it is up to the developer working with existing laws on height and FAR variation in order to find which design best suits a specific development at a particular location. By developing a step-by-step process, this study has provided the means by which to include energy as an “externality” and to be utilised when planning under the guidance of FAR calculations. It also shows that FAR is a viable indicator to be utilised as a benchmark in passive energy design for the built environment, and as a policy implementation guideline and strategy.
Limitations and Future Research
The study is limited to a particular urban form and perhaps the most popular configuration that is seen across many Chinese cities. Although it is a limitation, it also enables us to focus on a range of FAR options and not to examine more complex building form variations that seem to be less applicable to the context of China. The applications are also relevant to other developing cities in other contexts and with similar climatic conditions. However, as Cheshmehzangi and Butters (2016) suggested other models of building form and complex design can suggest better results that may eventually alter the current design and planning trends of residential compounds in China. Hence, future research should definitely follow up with additional studies and attempts at replication, suggesting energy planning directions and design alternatives that can revise the current policies and practises. In sum, this study considers energy consumption in the FAR specification to assess how the current trend of increasing FARs leads to the increase of urban energy demand. Thus, fundamental externalities associated with such energy consumption increase can translate into a component of FAR restrictions. Finally, this study should enable future research to consider energy planning strategies more comprehensively and towards shifts in policy and practise paradigms.
Data Availability Statement
The original contributions presented in the study are included in the article/supplementary material, further inquiries can be directed to the corresponding author/s.
Author Contributions
Both authors have worked jointly for conceptualisation, data analysis, data validation, and writing up the study.
Funding
The funding is provided by National Natural Science Foundation of China (NSFC), grant numbers 71850410544, 71950410760, and 72050410358.
Conflict of Interest
The authors declare that the research was conducted in the absence of any commercial or financial relationships that could be construed as a potential conflict of interest.
Publisher's Note
All claims expressed in this article are solely those of the authors and do not necessarily represent those of their affiliated organizations, or those of the publisher, the editors and the reviewers. Any product that may be evaluated in this article, or claim that may be made by its manufacturer, is not guaranteed or endorsed by the publisher.
Acknowledgments
The authors acknowledge The Ministry of Education, Culture, Sports, Science and Technology (MEXT), Japan.
References
Aggarwal, R. (2006). “Energy Design Strategies for City-centers: An Evaluation,” in 23rd Conference on Passive and Low Energy Architecture (Geneva).
Arboit, M., Diblasi, A., Fernandezllano, J., and Derosa, C. (2008). Assessing the solar potential of low-density urban environments in Andean cities with desert climates: the case of the city of Mendoza, in Argentina. Renew. Energy. 33, 1733–1748. doi: 10.1016/j.renene.2007.11.007
Bady, M., Kato, S., Takahashi, T., and Huang, H. (2011). Experimental investigations of the indoor natural ventilation for different building configurations and incidences. Build. Environ. 46, 65–74. doi: 10.1016/j.buildenv.2010.07.001
Baker, N., and Steemers, K. (2003). Energy and Environment in Architecture: A Technical Design Guide. Milton Park; New York, NY: Taylor & Francis.
Banister, D., Watson, S., and Wood, C. (1997). Sustainable cities, transport, energy and urban form. Environ. Plann. B Plann. Des. 24, 125–143. doi: 10.1068/b240125
Bassam, B. (2002). “Building form as an option for enhancing the indoor thermal condition,” in Building Physics 2002-6th Nordic Symposium, Session 18: Indoor Environment 2 (Trondheim), 759–766.
Capeluto, I., and Shaviv, E. (2001). On the use of ‘solar volume' for determining the urban fabric, Solar Energy 70, 275–280. doi: 10.1016/S0038-092X(00)00088-8
Cervero, R. (1996). Jobs housing balancing revisited. J. Am. Plann. Assoc. 62, 492–511. doi: 10.1080/01944369608975714
Cervero, R. (2001). Efficient urbanisation: economic performance and the shape of the metropolis. Urb. Stud. 38, 1651–1671. doi: 10.1080/00420980120084804
Chan, K. (2007). Misconceptions and complexities in the study of chinese cities: definitions, statistics, and implications. Eur. Geograp. Econ. 48, 383–412. doi: 10.2747/1538-7216.48.4.383
Cheshmehzangi, A. (2016). Multi-spatial environmental performance evaluation towards integrated urban design: a procedural approach with computational simulations. J. Clean. Product. 139, 1085–1093. doi: 10.1016/j.jclepro.2016.08.151
Cheshmehzangi, A. (2018). The changing urban landscape of chinese cities: positive and negative impacts of urban design controls on contemporary urban housing. Sustainability 10:2839. doi: 10.3390/su10082839
Cheshmehzangi, A., and Butters, C. (2016). Chinese urban residential blocks: towards improved environmental and living qualities. Urb. Des. Int. 22, 219–235. doi: 10.1057/s41289-016-0013-9
Cheshmehzangi, A., Dawodu, A., and Butters, C. (2016). “Greening the urban housing: the impact of green infrastructure on household energy-use reductions for cooling,” in The 5th Workshop on EU-Asia Relations (Hong Kong).
Cheshmehzangi, A., Dawodu, A., and Sharifi, A. (2021). Sustainable Urbanism in China. New York: Routledge. doi: 10.4324/9781003027126
Cheshmehzangi, A., Zhu, Y., and Li, B. (2010). “Integrated urban design approach: sustainability for urban design,” in Proceedings for ICRM, 5th International Conference for Responsive Manufacturing (Ningbo).
Compagnon, R. (2004). Solar and daylight availability in the urban fabric. Energy Build. 36, 321–328. doi: 10.1016/j.enbuild.2004.01.009
Dawodu, A., Akinwolemiwa, B., and Cheshmehzangi, A. (2016). A conceptual re-visualization of the adoption and utilisation of the pillars of sustainability in the development of neighbourhood sustainability assessment tools. Sustain. Cities Soc. 28, 398–410. doi: 10.1016/j.scs.2016.11.001
Dawodu, A., and Cheshmehzangi, A. (2016). Impact of Floor Area Ratio (FAR) on energy consumption at meso scale in China: case study of Ningbo. Energy Proc. https://www.sciencedirect.com/science/journal/18766102/105/supp/C 105, 3449–3455. doi: 10.1016/j.egypro.2017.03.789
Ghosh, S., and Vale, R. (2006). The potential for solar energy use in a New Zealand residential neighbourhood: a case study considering the effect on CO2 emissions and the possible benefits of changing roof form. Aust. J. Environ. Manage. 13, 216–25. doi: 10.1080/14486563.2006.10648689
Hachem, C., Athienitis, A., and Fazio, P. (2011a). Parametric investigation of geometric form effects on solar potential of housing units. Solar Energy 85, 1864–1877. doi: 10.1016/j.solener.2011.04.027
Hachem, C., Athienitis, A., and Fazio, P. (2011b). Investigation of solar potential of housing units in different neighborhood designs. Energy Build. 43, 2262–2273. doi: 10.1016/j.enbuild.2011.05.008
Holden, E., and Norland, I. T. (2005). Three Challenges for the Compact City as a Sustainable Urban Form: Household Consumption of Energy and Transport in Eight Residential Areas in the Greater Oslo Region. London: Routledge. doi: 10.1080/00420980500332064
Hui, S. (2001). Low energy building design in high density urban cities. Renew. Energy 24, 627–640. doi: 10.1016/S0960-1481(01)00049-0
Ignatius, M., Wong, N. H., and Jusuf, S. K. (2015). Urban microclimate analysis with consideration of local ambient temperature, external heat gain, urban ventilation, and outdoor thermal comfort in the tropics. Sustain. Cities Soc. 19, 121–135. doi: 10.1016/j.scs.2015.07.016
Jabareen, Y. (2006). Sustainable Urban Form: Their Topologies Models and Concepts. Department of Urban Studies, Dissertation at Massachusetts Institute of Technology. doi: 10.1177/0739456X05285119
Johansson, E. (2006). Influence of urban geometry on outdoor thermal comfort in a hot dry climate: a study in Fez, Morocco. Build. Environ. 41,1326–1338. doi: 10.1016/j.buildenv.2005.05.022
Joshi, K. K., and Kono, T. (2009). Optimisation of floor area ratio regulation in a growing city. Region. Sci. Urban Econ. 39, 502–511. doi: 10.1016/j.regsciurbeco.2009.02.001
Larivière, I., and Lafrance, G. (1999). Modelling the electricity consumption of cities: effect of urban density. Energy Econ. 21, 53–66. doi: 10.1016/S0140-9883(98)00007-3
LSE (2011). Cities and Energy Urban Morphology and Heat Energy Demand. Project Report Document. London School of Economics.
Martin, L., and March, L. (1972). Urban Space and Structures. Cambridge, UK: Cambridge University Press.
Mehaffy, M. W., and Salingaros, N. A. (2015). Design for a Living Planet: Settlement, Science, and the Human Future. Portland: Sustasis Foundation.
Mindali, O., Raveh, A., and Salomon, I. (2004). Urban density and energy consumption: a new look at old statistics. Transport. Res. A Policy Pract. 38, 143–162. doi: 10.1016/j.tra.2003.10.004
Montavon, M., Scartezzini, J. L., and Compagnon, R. (2004). “Comparison of the solar energy utilisation potential of different urban environments,” in Plea 2004 Proceedings. The 21st Conference on Passive and Low Energy Architecture. eds M. H. De Wit (Eindhoven: University of Technology), 1733–1748.
Næss, P. (2001). Urban planning and sustainable development. Eur. Plann. Stud. 9, 503–524. doi: 10.1080/09654310120049871
Newman, P., and Kenworthy, J. (1989). Gasoline consumption and cities - a comparison of United- States cities with a global survey. J. Am. Plann. Assoc. 55, 24–37. doi: 10.1080/01944368908975398
Oke, T. R. (1988). Street design and urban canopy layer climate. Energy Build. 11, 103–113. doi: 10.1016/0378-7788(88)90026-6
Owens, S. (1998). Urban transport and land use policies in east and west: learning from experience? Int. J. Environ. Pollut. 10, 104–125 doi: 10.1504/IJEP.1998.002233
Pacheco-Torres, R., Roldán, J., Gago, E. J., and Ordóñez, J. (2017). Assessing the relationship between urban planning options and carbon emissions at the use stage of new urbanized areas: a case study in a warm climate location. Energy Build. 136, 73–85. doi: 10.1016/j.enbuild.2016.11.055
Ratti, C., Baker, N., and Steemers, K. (2005). Energy consumption and urban texture. Energy Build. 37, 762–776. doi: 10.1016/j.enbuild.2004.10.010
Roldán-Fontana, J., Pacheco-Torres, R., Jadraque-Gago, E., and Ordóñez, J. (2015). Optimisation of CO2 emissions in the design phases of urban planning, based on geometric characteristics: a case study of a low-density urban area in Spain. Sustain. Sci. 12, 65–85. doi: 10.1007/s11625-015-0342-4
Sanaieian, H., Tenpierik, M., van den Linden, K., Mehdizadeh Seraj, F., and Mofidi Shemrani, S. M. (2014). Review of the impact of urban block form on thermal performance, solar access and ventilation. Renew. Sustain. Energy Rev. 38, 551–560. doi: 10.1016/j.rser.2014.06.007
Spangenberg, J. H., Pfahl, S., and Deller, K. (2002). Towards indicators for institutional sustainability: lessons from an analysis of Agenda 21. Ecol. Indic. 2, 61–77. doi: 10.1016/S1470-160X(02)00050-X
Speck, J. (2018). Walkable City Rules: 101 Steps to Making Better Places. Washington DC: Island Press. doi: 10.5822/978-1-61091-899-2
Steadman, P. (1979). “Energy and patterns of land use,” in Energy Conservation Through Building Design, ed. D. Watson (New York: McGraw-Hill), 246–260.
Steemers, K. (2003). Energy and the city: density, buildings and transport. Energy Build. 35, 3–14. doi: 10.1016/S0378-7788(02)00075-0
The World Bank. (2008). The Spatial Growth of Metropolitan Cities in China: Issues and Options in Urban Land Use. Report Document.
Wang, S. C., Fang, C., Wang, Y., Huang, Y., and Ma, H. (2015). Quantifying the relationship between urban development intensity and carbon dioxide emissions using a panel data analysis. Ecol. Indic. 49, 121–131. doi: 10.1016/j.ecolind.2014.10.004
Yamaguchi, Y., Shimoda, Y., and Mizuno, M. (2007). Transition to a sustainable urban energy system from a long-term perspective: case study in a Japanese business district. Energy Build. 39, 1–12. doi: 10.1016/j.enbuild.2006.03.031
Yang, X., Li, Y., and Yang, L. (2012). Predicting and understanding temporal 3D exterior surface temperature distribution in an ideal courtyard. Build. Environ. 57, 38–48. doi: 10.1016/j.buildenv.2012.03.022
Keywords: floor area ratio, FAR, sustainable urbanism, energy planning, energy, solar, urban design, residential community planning
Citation: Cheshmehzangi A and Dawodu A (2021) Towards a Sustainable Energy Planning Strategy: The Utilisation of Floor Area Ratio for Residential Community Planning and Design in China. Front. Sustain. Cities 3:687895. doi: 10.3389/frsc.2021.687895
Received: 30 March 2021; Accepted: 18 August 2021;
Published: 08 September 2021.
Edited by:
Jin Yang, China University of Geosciences, ChinaReviewed by:
Sujit Sikder, Leibniz Institute for Ecological Urban and Regional Development (IOER), GermanyTrivess Moore, RMIT University, Australia
Copyright © 2021 Cheshmehzangi and Dawodu. This is an open-access article distributed under the terms of the Creative Commons Attribution License (CC BY). The use, distribution or reproduction in other forums is permitted, provided the original author(s) and the copyright owner(s) are credited and that the original publication in this journal is cited, in accordance with accepted academic practice. No use, distribution or reproduction is permitted which does not comply with these terms.
*Correspondence: Ali Cheshmehzangi, YWxpLmNoZXNobWVoemFuZ2lAbm90dGluZ2hhbS5lZHUuY24=