- 1MaGBISE Research Laboratory, Department of Economics, Democritus University of Thrace, Komotini, Greece
- 2Department of Economics, Democritus University of Thrace, Komotini, Greece
The transition from linear to circular economic systems represents a critical step towards achieving global sustainability goals. At the core of this transformation lies the development of supply chain management that needs to deviate from the traditional “take-make-dispose” approach to one focused on resource efficiency, waste reduction, and material recycling. The use of Business Intelligence (BI) technologies has great potential to support this shift through enhanced decision-making and operational efficiency. This study adopts an assessment framework drawing upon an extensive review of literature, empirical analysis, and case studies from various industries. A theoretical concept map was created to evaluate the application of circular economy principles to supply chain management, highlighting the role of business intelligence technology. The findings highlight how BI tools (such as advanced analytics, predictive modeling, and real-time monitoring) enable organizations to identify inefficiencies, streamline operations, and optimize resource usage across the supply chain. BI contributes to circular economy practices in areas such as product life cycle management, manufacturing, and reverse logistics by facilitating material tracking, recycling, and reintegration into the value chain. This study analyzes the relevance of strong technology infrastructure, facilitatory policy and regulatory arrangements, and collaboration between industries in the development of circular supply chain models. In addition, this study recommends capacity building initiatives that would enable organizations to acquire skills and capabilities to employ business intelligence-based strategies. Finally, the study provides recommendations to businesses, policy makers, and stakeholders on how business intelligence can support circularization. The proposed framework offers practical application and contributes to the body of literature relating to the incorporation of circular economy concepts into supply chain management.
1 Introduction
During the past century, industrial and technological advancements, coupled with increased global trade over recent decades, have dramatically boosted economic advancement and human wellbeing (Dimitriou et al., 2024). This remarkable period of expansion has resulted in remarkable achievements in diverse sectors like healthcare, communication technology, and transportation that have greatly raised living standards and considerably reduced poverty rates at a global level. Despite this progress, it has come with drastic environmental consequences reflected through increased consumption of resources and degradation of natural environments (Dimitriou et al., 2024; Kok et al., 2013).
Following high-profile global climate conferences, namely the Paris Conference of 2015 and the Glasgow Summit of 2021, there has been increased global awareness of the environmental impacts linked to uncontrolled resource extraction. Current assessments show a 70% increase in raw material extraction, outcompeting the Earth’s regenerative capacity, thus highlighting the urgency for sustainable strategies (Circular Economy, 2022). Current resource consumption patterns are considered unsustainable, posing considerable threats to environmental stability and the prosperity of future generations. Today, there is huge consumption of natural resources at about 7.3 billion tons within the EU annually. The quantity of wastes produced is huge and stands at about 2.7 billion tons of wastes, including hazardous wastes estimated at 98 million tons per year, and food wastes estimated at 89 million tons yearly, meaning each European citizen generates 179 kg of food waste. It is concerning that merely 40% of this waste is either repurposed or recycled, with the majority unfortunately being deposited in landfills, thereby aggravating pollution and environmental damage. The planet faces substantial environmental issues, including climate change and the depletion of biodiversity, which present serious risks to health, ecosystems, and economies (Dimitriou and Karagkouni, 2022a). These issues are closely associated with unsustainable practices prevalent across multiple sectors, including transportation. Especially, the sector attracts much criticism due to the great amount of greenhouse gas emissions and resource usage contributed by transport operators. Such scrutiny shows that there is a need for transformational ways in which resources and emissions are managed.
A developing field within the framework of circular economy practices, especially pertinent to airport operations, is the reduction of food waste via recycling and biomass generation. The effective repurposing of food and agricultural by-products not only diminishes the quantity of waste directed to landfills but also aids in decreasing greenhouse gas emissions, as demonstrated by recent studies (Rashwan et al., 2023). By repurposing organic waste for bioenergy, such practices can contribute to carbon neutrality targets, aligning with circular economy principles aimed at waste minimization and resource recovery (Rashwan et al., 2023). Besides, the application of the circular economy approach to food waste management is considered to include extensive work in terms of mitigating climate change (Yang et al., 2022). The method addresses wide environmental issues, which include carbon emission reduction and conservation of resources important for airport sustainability.
Addressing these crucial challenges, institutional and European bodies emphasize the need to transition toward a circular economy. CE insists on reduction, reusing, and recycling of materials to create a closed-loop cycle that would reduce waste and preserve natural resources. This is considered one of the main ways to mitigate the threats of climate change and deterioration of the environment (Dimitriou and Karagkouni, 2022b). The aim of this paper is to explore the role of Business Intelligence tools in the transition towards a CE by airports. More specifically, this research investigates how predictive analytics, real-time monitoring, and every other potential use of BI tools contribute to resource efficiency, waste management, reduction of emission, and other activities that are linked with CE at an airport. This study presents, with a sample of European airports, the best practices driven by BI that can provide the insights airport operators, policy makers, and other key stakeholders need in their pursuit of greener and resilient aviation operations.
This research concerns the role of an airport operator in accelerating the supply chain towards a more circular economy. Being nodes in a network of international transports, airports hold an advantageous position vis-à-vis leading by example in introducing innovative approaches to sustainable management. Based on integrating circular economy principles within its management model and added value chain, an airport operator may significantly minimize the negative impact on the natural environment and contribute to reaching general sustainability objectives.
2 Research background
Aviation is a key sector in driving a transition to more sustainable business practices due to its significant environmental impacts. The aviation sector, including airports and airlines, is a significant producer of greenhouse gas emissions, resources used, and waste generated. It has become vital to tackle such issues of sustainability due to the unabated and rapid increase in global air traffic that amplifies environmental pressures and requires developing new approaches to mitigate such impacts. The dominant production pattern since the Industrial Revolution has been Linear Supply Chain (LSC)-type frameworks that are typified by the “take-make-dispose” approach that involves extraction of resources, transformation thereof into products, and their disposal. Supply chains have traditionally focused on keeping costs low and efficiency high while sidelining environmental considerations to a large degree, with significant depletion of resources, pollution, and emissions occurring (Rockström et al., 2009).
With the severe environmental consequences linked to linear resource management—marked by high greenhouse gas emissions and loss of biodiversity—there is a need to adopt an alternative economic model. The Circular Economy (CE) presents a new sustainable paradigm focused on resource efficiency, waste minimization, and recycling. Unlike the traditional linear approach, CE aims to restore natural ecosystems while maintaining the functionality and value of products and materials, thus reducing waste (MacArthur, 2013; Tsironis et al., 2023). The integration of CE concepts in the aviation industry, specifically in airport operations, is a vital development in meeting sustainability goals. As part of the global transport system, airports have substantial potential to lead by example in sustainability measures. Airports can mitigate environmental effects significantly through efficient waste management, energy consumption, and reduced emissions by adopting CE measures, thus making a valuable contribution to global efforts in sustainability.
2.1 Traditional supply chains
The dominant mode of production since the time of the Industrial Revolution has been through conventional supply chain models within the linear framework operating in the paradigm “take-make-dispose,” whereby resources are taken out, then converted into goods for use by consumers. The traditional focus of supply chains has conventionally fallen on reducing costs and enhancing efficiency, often in ways that are in total disregard to the environment in terms of the over-exploitation of natural resources and waste and pollution emissions. A result of such approach is the severe raw material extraction, high emissions associated with various industrial activities, as well as the exceeding of critical biophysical boundaries (Rockström et al., 2009).
Linear resource extraction and processing are responsible for half of the total greenhouse gas emissions and more than 90% of biodiversity loss and water stress. Given these severe environmental impacts, it is evident that adopting a new economic model and production systems is crucial (Popović et al., 2022). It automatically requires an overall change: shifting resource management to a sustainable methodology should be developed together with implanting circular strategies into the production system and supply chain management.
2.2 Emergence of circular economy
Besides this fact, grave environmental problems linked to the traditional linear industrial model have made the Circular Economy an advanced and feasible sustainable alternative. According to Khaw-ngern et al. (2021), the CE model is based on the principle of circularity in the product life cycle through resource efficiency, waste reduction, and recycling. Unlike the linear “take-make-dispose” paradigm, the circular economy is regenerative by design and aims to decouple economic growth from the consumption of finite resources (Ellen MacArthur Foundation, 2022).
The circular economy mimics natural systems by maximizing the use of resources, keeping products and materials in use for as long as possible, and recovering and regenerating them at the end of each useful life, thereby minimizing waste and pollution (Tsironis et al., 2023; MacArthur, 2013; Asian Development Bank, 2024). According to MacArthur (2013), the circular economy is “restorative by design” and seeks to maintain the utility and value of products, components, and materials at all times. The circular economy is based upon business models that replace the “end-of-life” concept with reducing, reusing, recycling, and recovering materials during the product distribution and consumption processes. This approach results in lower volumes of waste produced and discarded from both manufacturing and raw materials processes (Ginga et al., 2020). The CE extends beyond recycling and is founded on a restorative industrial system that treats waste as a resource (Ghosh, 2020). It comprises three principal activities: reducing the use of virgin raw materials, reusing already processed materials, and recycling waste (Burneo et al., 2020).
This model is built on three key principles:
1. Waste equals food: redefining the lifecycle of goods to minimize ecological impacts from manufacturing new products, promoting maintenance, reuse, refurbishment, and recycling within a closed loop to extend product lifespans.
2. Use renewable resources: encouraging the use of renewable or waste-derived resources and energy, which can lead to job creation and reduced environmental impacts, including lower carbon emissions.
3. Increase resilience through material innovation: reducing raw material use and waste reproduction by facilitating the transition of materials from one manufacturing process to another, requiring collaboration among various companies and stakeholders within the circular economy system.
The CE aims for minimal consumption and extraction through the application of the 9Rs: refuse, rethink, redesign, reduce, re-use, repair, renew, recycle, and recover. This is based on the thought/idea that in nature, everything has value, waste is converted into a new source, and thus, the circle is complete (Korhonen et al., 2018).
Although CE was already expressed in the 1970s, the concept gained considerably more prominence and importance at the turn of the millennium in 2000. This is mainly because of the close associations that have since been made between CE and sustainable development according to Korhonen et al., 2018, and its associations with bio-economy and green economy domains at large according to D’Amato and Korhonen (2021). Despite the widespread recognition of the advantages associated with the shift toward a Circular Economy (CE), this transition remains in its nascent phases (Stucki et al., 2023). The movement toward CE presents a variety of challenges and hindrances, which encompass insufficient understanding, the absence of regulatory frameworks, and the necessity for extensive alterations in behavior among both consumers and enterprises (Zhang et al., 2023).
Nowadays, transitioning to a circular economy (CE) is improbable without incorporating digital technologies (DTs) such as the Internet of Things (IoT), simulation, and blockchain. These technologies offer new ways to optimize resource use, boost productivity (Kristoffersen et al., 2020), and improve supply chain agility (Oliveira-Dias et al., 2022).
2.3 Opportunities in CE
The circular economy has lately turned into a theme of high relevance for corporate strategies, as it grants firms competitive advantages, new profit opportunities, increased robustness, and solutions to urgent business challenges (Mishra et al., 2019; De Angelis, 2024; Saari et al., 2022). Operational and strategic advantages can be achieved by organizations on both microeconomic and macroeconomic levels through the adoption of CE principles (Dimitriou, 2022).
The theory of Competitive Advantage by Porter (1985) suggests that companies which embed principles of CE will be able to distinguish themselves with sustainable and resource-efficient processes and products. Improved market position and profitability result from differentiation. While Porter’s market-oriented approach is outside-in, focusing on external market factors, Barney (1991) takes an inside-out approach, considering a firm’s core competencies in efforts toward a unique market position. Barney defines competitive advantage as a firm’s ability to implement a value-creating strategy that competitors cannot duplicate. Combining resources and capabilities effectively is key to developing distinctive competencies.
Technological advancements play a vital role in the promotion of circular economy (CE) principles, markedly impacting systemic economic development and expansion (Popović et al., 2022). One prominent benefit of CE is its potential to diminish or eradicate greenhouse gas emissions associated with production by reducing the requirement for raw materials, energy, and waste creation (Baxter and Srisaeng, 2022). High material circularity in the supply chain will mean low demand for new resources, thus reducing the environmental impacts associated with the extraction and processing of raw materials. The circular economic model hence allows enterprises to implement regenerative manufacturing systems through closed-loop resources for economic viability with environmental sustainability. Circular modes of transport alone are able to radically reduce consumption of new materials, yielding much lower GHG emissions. According to Bleischwitz et al. (2022), the technology developed should be in such a way that it redesigns components and products at the end of their useful life, apart from developing a new material that is adaptable and recyclable with ease.
A Smart circular economy (SCE) is “an industrial system that uses Digital Technologies (DTs) to A Smart Circular Economy (SCE) is defined as “an industrial system that uses Digital Technologies (DTs) to provide intelligent functions for implementing value-added circular strategies” (Lobo et al., 2021, p. 1). In the context of airports, technological innovations are pivotal in enhancing sustainability. For instance, advanced sorting technologies and automated waste collection systems can greatly increase the efficiency and effectiveness of waste management programs. Specifically, the integration of robotics and AI in waste sorting has demonstrated significant potential in improving recycling rates and minimizing contamination levels (Ellen MacArthur Foundation, 2022).
2.4 The role of aviation
The principles of CE can be applied in various industries and sectors, including transportation. In particular, CO₂ emissions generated by transportation have risen by over 70 percent since 1990 and contribute more than 20 percent of the current worldwide CO₂ emissions from fossil fuel combustion today (Cokorilo, 2016). In addition, in 2022, carbon dioxide emissions from global transport rose by almost 5%, reflecting continued recovery from the unprecedented falls seen in the year 2020 due to the COVID-19 pandemic. According to Dimitriou and Sartzetaki (2022b), the same emissions are expected to grow significantly in the coming years.
Recent studies in transport and logistics reveal the importance of CE in enhancing supply chain sustainability. An investigation by Rashwan et al. (2023) and Yang et al. (2022), showed that the use of circular economy frameworks in the logistics industry reduced resource consumption because logistics companies close the loops by re-manufacturing components themselves. Approaches such as these in supply chains have been successful in reducing waste and aiding the recovery of resources; therefore, these examples become highly relevant to the aviation industry, where logistics and transport lie at its core. Building on this, the present study explores how the implementation of CE principles in supply chain practices at airports can better underpin resilient and sustainable aviation operation.
Nowadays, current research has started to delve into the application of the principles of CE in the aviation industry in depth, particularly on issues related to waste management, resource conservation, and emission reduction, including Tjahjono et al. (2023) and Castillo Malagón et al. (2024). Evidence shows that due to the extensive operation capacity, airports are one of the key players in the implementation of circular economy practices, mainly in the context of a closed-loop system for managing wastes and by-products of airport operations to reduce carbon footprint. For instance, studies have shown that those airports which apply CE models achieve minimal environmental impact through innovative ways of waste reuse, such as converting de-icing liquids into useful energy sources (Tjahjono et al., 2023).
It is also recognized in the current literature that, even though air travel is generally taken to be the greatest carbon emitter within the aviation industry, there is much potential for additional environmental benefits at airports that have not been explored yet. For example, airport terminals generate close to 6 million tons of passenger waste across the globe every year, most of which is always burnt or buried in landfills (Dimitriou and Sartzetaki, 2022a; Tjahjono et al., 2023). Additionally, ground operations conducted at airports account for approximately 2% of the emissions produced by air transportation. These factors underscore the necessity of tackling both emissions from flights and those from ground operations to attain thorough environmental sustainability within the aviation industry.
All the factors mentioned above become even more significant in view of the fact that aviation faces serious development. In particular, it is predicted by the International Civil Aviation Organization (ICAO Secretariat, 2022) that global air traffic will double in 2035, growing at an average annual growth rate of 4.4%. As the number of passengers grows, there will be a corresponding increase in waste and emissions, thereby requiring the implementation of innovative sustainability strategies (Celikel et al., 2022). This projected expansion within the aviation industry highlights the critical need for the integration of Circular Economy (CE) principles to alleviate its environmental effects.
To overcome such challenges, it is vital to adopt state-of-the-art technologies, ensure continuous innovation, and use skilled laborers (Celikel et al., 2022; cited in Castillo Malagón et al., 2024). Given the uninterrupted development of aircraft through modern technologies, specialists note that airports will play an ever more important role in contributing to sustainability and, especially, to the circular economy principles. That means that airports could actually get a grip on new practices and technologies which would result in reducing the environmental impact while air travel increases considerably in the near future.
Moreover, greater emphasis on sustainability with regards to supply chain management and operational practices is attributed to the fact that organizations are being asked to answer to and are responsible not only for solid economic performances but also for environmental and social impacts by substantial stakeholders (Walker et al., 2014), institutional frameworks, and regulatory mechanisms. Regulatory policies go a long way in encouraging the adoption of environmentally friendly processes for improvement at airports. Regulatory frameworks and incentives are a strong supporters of leveraging interest from the airport to invest in green infrastructure and technologies. The European Union, for example, has set ambitious goals toward reducing carbon emissions in the aviation sector through using sustainable aviation fuels and enhancing energy efficiency from airport operations through the European Commission (2021).
Such challenges have therefore propelled the European Commission into an all-inclusive European Green Deal for airports. The key proposal for reduction has been made concerning the emission from transport, added to smart and sustainable mobility. This is further evidenced by the “FIT FOR 55” package, which is a climate law and a legally binding target for the EU’s climate goal of at least a 55% reduction of emissions before 2030. It follows that the EU’s member states are now developing new legislation in order to reach this target and secure EU environmental sustainability by 2050.
This initiative focuses on several key areas: sustainability, such as the use of Sustainable Aviation Fuels (SAFs); smart operations, including the adoption of appropriate IT tools; multimodality, which involves integrating various transportation modes like rail and city center linkages; and other aspects of the built environment, such as improving energy efficiency. By prioritizing these areas, the initiative aims to significantly reduce the environmental footprint of airports and ports, fostering a more sustainable future for the transportation sector.
Furthermore, more than 270 airports in the ACI Europe network have committed to achieving net zero CO₂ emissions by 2050 under the initiative “Destination 2050,” representing 75.5% of European air passenger traffic based on 2019 levels. Nearly 50% of these airports have set even more ambitious targets to reach Net Zero by 2030. According to ACI Europe data, over 90 European airports have announced their intention to achieve net zero carbon emissions by 2030.
A crucial component of these efforts is the concept of the “green airport,” which aims to minimize environmental impact and achieve carbon neutrality by eliminating greenhouse gas emissions (González-Ruiz et al., 2017). Usually, airports create hazardous and non-hazardous solid wastes generated by activities on-site and from flight operations directly (Baxter et al., 2018a, 2018b). All of these wastes need to be managed through potential reuse, material recycling, recovery for energy, and land filling (Sarbassov et al., 2020). Green airports address waste management and other environmental impacts as crucial contributors to the development of the goals for the European Green Deal.
Although recent references indeed suggest that CE-associated practices have positive returns in a variety of industries, there is a considerable chasm concerning attention toward the principles of CE specifically for the aviation industry. This neglect becomes even more obvious in terms of those data-driven methodologies known as Business Intelligence and predictive analytics within the airport setting (Tjahjono et al., 2023; Rashwan et al., 2023). Furthermore, some of the specific environmental issues in aviation-such as high energy use and carbon emissions from ground operations-require adapted CE frameworks that have not yet been deeply discussed. The present paper tries to address those deficiencies by investigating the role of BI tools in supporting CE practices from airport operations, hence contributing to the emergence of hard data for the growing field of sustainable aviation.
3 Methodological approach
A flowchart is hereby presented that will fully represent the methodology, pointing out significant steps and processes taken in the context of this research (Figure 1). This flowchart sets the orderly structure through which the analysis of the role of Business Intelligence tools in enabling Circular Economy practices within airport operations has been performed. It starts with the selection and sampling of European airports, follows a structured data collection, content analysis using the 9Rs Circular Economy framework, and the use of Business Intelligence tools for assessment of sustainability indicators. Below is the flowchart of such steps, comparing at the end to develop best practices, barriers, and facilitators to implement principles of Circular Economy within airports.
A broad literature review was made in the first instance, which allowed presenting not only the theoretical framework but also the most relevant subjects regarding circular economy practices in supply chain management and the crossing point with Business Intelligence, based on the analysis of academic articles, reports from the industry, and relevant publications.
The following paper discusses some particular BI tools and their application in the context of the circular economy at airports. Predictive modeling can enable estimates of future wastes from historic data on passenger traffic, seasonal changes, and the general activities of an airport. Such forecasting of periods of high generation allows the airport authorities to make necessary changes in the scheduling of waste management in advance that may avoid overflows, further improve efficiencies in recycling, and smooth manpower allocation. Real-time monitoring tools integrated with IoT sensors can track resource use continuously, such as water and energy consumptions at the airport facilities. This provides real-time insight into immediately making necessary adjustments to minimize waste in consumptions, hence contributing to achieving energy efficiency goals.
This automatically implies that effective BI implementation within this arena must be surrounded with comprehensive and high-frequency data from every type of source. This would involve passenger traffic data, waste categorization metrics, energy consumption rate, emissions from ground operations, and water usage. This data comes from digital platforms, IoT-enabled devices, and historical records of airport operations. Energy consumption data, for instance, is key for predictive analytics to forecast peak demand and adjust resources for it, minimizing superfluous energy expenditure. Similarly, emissions data gathered on an interval basis can provide airports with trending information on their carbon footprint while prompting corrective measures in near real time to meet the Scope 1 and Scope 2 reduction targets. Data-driven insights will let airport managers make wiser decisions, raising the level of operational efficiency while aligning with the circular economy’s objectives of minimal consumption of resources and waste.
This research has studied the European airport sector with an emphasis on the crucial elements concerning CE practice adoption, which are necessary for the shift toward more sustainable operational methodologies. The sample used is European airports that have similar attributes in a unified regulatory context. These were selected based on their geographical locations and trends of traffic: all these airports were among major tourist destinations and transported between 10 and 30 million passengers in 2022. At least one airport was sampled in every one of the 10 European Union countries which had published a Sustainability Report for the year 2022. The needed information for the sampled airport is reflected in Table 1.
For this research, a purposive sampling approach was employed, with deliberate selection of European airports according to pre-defined criteria that were congruent with research objectives. The reason for using a purposive sampling lies in its suitability to qualitative and evaluation research studies that takes into account the deliberate selection of cases to create adequate and related information. Specifically, selected airports are based on their geographical coverage in Europe, annual passenger traffic between 10 and 30 million passengers, and documented commitment to sustainability through their publicly available sustainability reports. The research design developed here aimed to study those airports that represented a diverse range of medium-flow facilities with notable but manageable environmental issues that would allow for a close case study and thorough study of circular economy initiatives that would be implemented under similar conditions. The above-mentioned range excludes so-called mega-hubs that would skew results due to greater resources and regulatory imperatives, and also smaller airports where sustainability initiatives may still be in their infancy. The airports within this range demonstrate a blend of well-integrated sustainability practices influenced by regional regulatory frameworks, such as the EU Green Deal targets, yet reflecting operational dynamics suitable for benchmarking in the sector. These criteria ensured a meaningful comparison by focusing on airports that face substantial yet manageable environmental impacts, thereby reflecting realistic scenarios for implementing Circular Economy (CE) practices.
The methodological approach of the present study involved conducting extensive desk research to collect and assess already available information. Although the circular economy has emerged as a hot topic for research since the mid-2010s, academic papers related to the CE business practices adopted by airports remain distinctly limited. To bridge this gap, secondary data sources were utilized, such as sustainability reports published by airport operators (Geissdoerfer et al., 2017; Ghisellini et al., 2017).
The systematic content analysis approach is applied in this research based on the principles forwarded by Krippendorff (2013). In order to carry out a systematic scan of the sustainability reports, an extended codebook was prepared with four major categories: energy, emission, waste, and water management. These were further sub-coded; under the sub-code of emissions, for example, we had “Scope 1, 2, and 3 emissions,” while under waste, the sub-codes were sorted, recycled, and biologically treated waste. This is an iterative approach to coding, where preliminary coding was done on a subsample of reports as a means of developing and refining the codes themselves against emergent patterns and themes. This enhanced reliability further by double-coding the reports; that is to say, two different independent researchers coded a random sample of reports.
Through the provision of critical insights, this analysis seeks to furnish planners and decision-makers with significant information (Karagkouni and Dimitriou, 2022). This methodology presents planners and decision-makers with an extensive comprehension of airport management in tourist locales, emphasizing the advantages and pragmatic measures for incorporating Circular Economy principles into their operations.
To ensure a systematic application of circular economy (CE) principles, we applied the 9Rs framework—Refuse, Rethink, Reduce, Reuse, Repair, Refurbish, Remanufacture, Repurpose, and Recycle—as a structured lens through which to analyze airport operations. Each R principle was applied to relevant aspects of airport activities. By examining these operational activities through the 9Rs framework, the study highlights actionable steps that align with CE objectives.
Table 1 was developed to provide a structured overview of the environmental and operational metrics used to evaluate Circular Economy (CE) practices in airport operations. Data collection required assessing the sustainability reports of a representative group of European airports and coding the unique environmental management practices against the 9Rs framework. Each metric identified in Table 1 was chosen for its relevance to airport operations and for its ability to yield useful insights regarding CE practices. The chosen indicators to be analyzed were carefully selected to ensure full coverage of key dimensions of sustainability that have applicability to airport operations. The selection was guided by a wide-ranging review of current standards, academic literature, and best practices in the industry (Global Reporting Initiative, 2016; ACI Europe, 2022; Dimitriou and Karagkouni, 2022b; Dimitriou et al., 2024). Specifically, indicators chosen (emissions intensity, energy consumption, water consumption, and waste management) have good alignment with internationally accepted frameworks and standards, such as those adopted by the Global Reporting Initiative (GRI), Airport Carbon Accreditation (ACA), and those codified in airfield management standards of Airports Council International (ACI Europe, 2022; Global Reporting Initiative, 2016). Together, those indicators present a comprehensive and detailed evaluation of environmental performance in the aviation industry.
The choice of Scope 1, 2, and 3 emissions intensity is warranted based on the broad environmental issues relating to greenhouse gas emissions in the aviation industry, especially in regards to efforts intended to counter climate change (ACI Europe, 2022). Indicators regarding energy consumption which account for renewable energy consumption were selected based on the high-energy needs of operating airports; enhancing energy efficiency hence plays a paramount role in lessening environmental repercussions (Dimitriou and Karagkouni, 2022b). Measures of water consumption were included owing to their priority position in promoting sustainable resource allocation, especially as airports often leverage volumes of water comparable to what is used in small municipalities and therefore the urgency to implement meaningful water conservation policy (Global Reporting Initiative, 2016). Indicators of managing waste, i.e., rates of recycling, reuse, and diversion from the landfill, were selected to combat the varied character of waste handled by airports and thus the prominence of effective measures for managing waste toward circularity (González-Ruiz et al., 2017). Deliberate consideration of these measures guarantees the attainment of a set of inclusive as well as effectively applicable measures available for airports to gage and coordinate their shift toward more efficient functioning practice.
4 Depiction of airports’ elements linked to circular economy
This study’s evaluation approach is based on a review of airport Sustainability Reports and, more particularly, the identification of data about the incorporation of essential environmental management performance features in them supporting the transition to CE.
The review of airports’ environmental reports and plans revealed various methods of reporting their efforts to minimize environmental impacts. Specifically, in most cases there was not a common approach to address and present certain aspects cross reports, and quantitative data was often lacking. Moreover, rather than referring directly to the concept of CE, a company’s circularity is measured indirectly by identifying activities that ultimately make a company more circular or adhere to minimizing environmental externalities. The transition to a circular economy involves the prevention of waste, resource efficiency, and emissions reduction. Resources must be used far more efficiently so that we reduce the need to extract new resources. Products must last for as long as possible, be repaired, upgraded and reused. When products cannot be reused in their original state, waste must be recycled into new materials and used for new production.
Another novelty of the methodological framework developed is that it could become an important, available instrument for stakeholders, decision makers, planners, and managers in formulating regulatory policies and in strategic planning about investments in air transport infrastructure. Besides, the developed model makes it possible to evaluate the environmental performance at airports, putting in focus the critical aspects of environmental management that influence the trends in demand A review of the environmental reports and plans of airports showed that there are mixed approaches to reporting on efforts aimed at reducing environmental externalities from airport operations. More precisely, different subjects are emphasized and reported intermittently, with a noticeable scarcity of quantitative data in most of them. The majority of these airports follow an EMS such as EN-ISO 14001 and thus indicate that airports are committed to reducing environmental hazards and impacts; however quantitative information on specific measures and goals about their environmental performance or activities is considerably lacking in them (Dimitriou et al., 2024). Also, the measurement units are multiple and datasets incomplete most of the time, and many report on different time frames. A qualitative approach was thus considered appropriate for the study of airport performance in environmental management from which conclusions on its CE programs could be drawn.
During evaluation of airport sustainability reports, it was observed that quantitative information was at times reported at group level as opposed to being broken down to the level of individual airports, thus making it cumbersome to compare. For this purpose, a standard estimation procedure based on passenger traffic (PAX) volume was used to derive information at airport level. More specifically, group-level reported aggregate information was proportionately split up to individual airports based on their relative share of passenger traffic in that group. For instance, if an airport represented 20% of passenger traffic of a group, it was assigned 20% of group-reported totals of emissions, energy consumption, water consumption, or waste production. The proportional attribution principle was used systematically to all relevant information to enhance comparability and consistency in this context of research.
Table 2 provides a structured overview of key elements related to the Circular Economy practices at airports. It includes specific categories such as material issues; emissions, energy, water, and waste management. Each category encompasses various sub-elements crucial for sustainable operations and environmental impact reduction at airports. By focusing on these aspects, airports can implement strategies to enhance their sustainability practices and contribute to a more circular economy in the aviation industry.
By taking a closer look at the table it becomes evident that, although all airports dedicate a specific section of their reporting to the selected material issues, the elements presented vary significantly.
The study’s findings demonstrate that airports are applying CE principles systematically across key operational areas. For instance, under the “Reduce” principle, airports such as Helsinki have implemented advanced HVAC systems that adjust energy consumption based on occupancy, reducing unnecessary energy use. In waste management, the “Reuse” and “Recycle” principles are evident in the practices of Porto International Airport, where food waste is donated to local charities rather than discarded. Each of these examples illustrates the practical integration of the 9Rs framework, connecting CE principles directly to the resource efficiency and waste reduction strategies pursued by these airports. To further emphasize the application of CE principles, a table summarizing the 9Rs framework with corresponding examples from airport actions, reinforcing the consistent application of CE strategies, is presented below (Table 3). The environmental performance metrics of the selected airports, related to circular economy are analytically presented in Table 4.
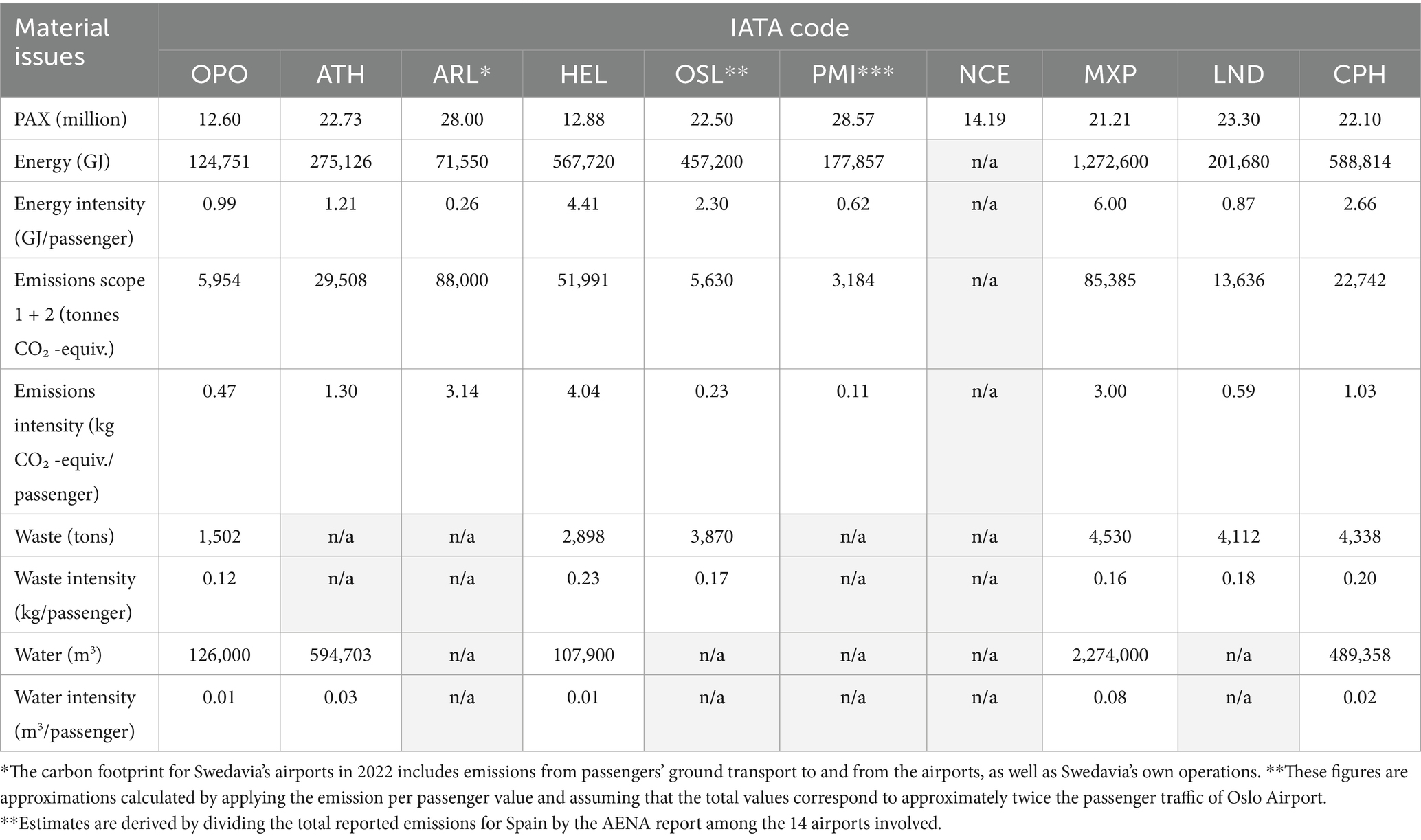
Table 4. Environmental performance metrics of airports related to Circular Economy (2022).
4.1 Emissions
At airports, GHG emissions come from gasoline and diesel for airport vehicles and ground support equipment (GSE), fossil fuels for electricity and heating, jet fuel for auxiliary power units (APUs) at gates, and other sources. Emissions are reported under Scopes 1, 2, 3. Scope 1 emissions refer to direct emissions from airport-owned or controlled sources, such as airport power plants burning fossil fuel, gasoline-powered vehicles, and diesel-fueled GSE. Scope 2 involve indirect emissions from purchased energy (electricity, heat, etc.). While Scope 3 emissions are those owned and controlled by airport tenants and other stakeholders. Airports can reduce GHG emissions and operating costs by implementing energy efficiency measures like improving insulation, purchasing renewable energy, installing compatible renewable energy systems, reducing energy consumption, monitoring HVAC system efficiency, and acquiring low or zero-emission vehicles and GSE. In our sample nearly all airports prioritize emissions reporting and emissions goal setting, consistently monitoring carbon footprints and setting greenhouse gas (GHG) emissions reduction targets. The only exception is Nice, which does not include all three elements.
4.2 Energy
Energy sources for civil airports include electricity, coal, natural gas, diesel, purchased heating, gasoline, and other fuels. “Energy management” refers to the process by which airports can evaluate and monitor their energy usage and implement strategies to reduce it. A key aspect of long-term sustainability, extensively discussed in the literature, is on-site renewable energy generation [Alba and Mañana, 2016; Clean Energy Finance Corporation (CEFC)]. Considering their large land areas, airports are excellent candidates for utilizing on-site renewable energy sources such as solar and wind.
Solar PV installations are one of the most common renewable energy considerations for airport facilities. These can be installed on roofs, parking garages, and large open space areas on the airport site. Since the solar photovoltaic systems ensure enough supply of clean energy, reliance on fossil fuels would be reduced and enhance the overall energy resiliency of the airport. On-site renewable energy systems, including solar photovoltaic (PV) installations, can be evaluated through several criteria, such as the proportion of energy requirements satisfied by on-site renewables and the exergy produced (Baxter et al., 2018b). In the dataset analyzed, 60% of the airports harness energy from solar park activities. As an example, in 2022, Athens International Airport generated 13,334 MWh of energy thanks to its photovoltaic systems, thus saving 5,600 tons of CO₂ equivalent.
Another viable solution for on-site renewable energy generation at airports is represented by wind energy. Wind farms and geothermal facilities are applied in only 10% of the reviewed cases. For instance, the geothermal infrastructures of Palma de Mallorca Airport generate 6,100 MWh per year for heating and 4,500 MWh for cooling; the Palma de Mallorca Airport applies enhanced HVAC for increasing energy efficiency. Aeana reports that the feasibility studies have been carried out regarding the use of geothermal energy in the Palma de Mallorca airport. The first forecasts obtained indicate the possibility of covering from 30 to 15% of the refrigeration and up to 100% of the heating.
Furthermore, they have said to continue doing so until 2026. London Stansted Airport generates 100% of its electricity from renewable sources and 74% of its total energy needed. Another good example is Helsinki Airport, of which 100% of its electricity consumption comes from wind power. Moreover, 100% of Palma de Mallorca Airport’s electricity procurement is of guaranteed renewable origin. No airports were reported to have biomass installations. 50% ran on SAF - Sustainable Aviation Fuel, that is to say, fuels from non-fossil sources used in commercial aviation today. That reduces CO₂ emissions by up to 80% as compared to traditional fuel. About 93% of the carbon footprint of airlines makes for all the Scope 1 and 2 aviation emissions, while airports comprise about 1%. The application of renewable systems for generating energy at airports decreases dependence on conventional sources of energy and raises energy efficiency levels, improving environmental conditions. Energy management practices applied in addition to the generation of renewable energy contribute much to the sustainable operation of all facilities serving civil aviation. Well-implemented energy management strategies contribute not only to helping airports reduce their operational costs but also help with environmental sustainability through the reduction of greenhouse gas emissions.
4.3 Waste
Implementation of CE practices widely points out that effective waste management is indeed a matter of primary concern. Waste at airports means all refuse that arises from various operations and activities related to the functionality of airports. It also covers waste from passengers, airfield operations, maintenance activities, and waste from construction and demolition works. Basically, the types of wastes generated are solid urban waste, non-hazardous waste, hazardous waste, municipal waste, compostable waste, and lavatory waste.
The exponentially growing challenges that face the air transport industry in solid waste management within airports are a significant environmental issue. The rise in passenger travel, combined with the increasing activities at and around an airport, contributes to increased rates and variance of wastes produced (Sebastian and Louis, 2021). In the absence of any standard rules or regulations on waste disposal, it has become a core concern for environmental management in the global air transport industry (Ravishankar and Christopher, 2022). On the other hand, effective airport waste management systems have already demonstrated the ability to reduce volumes over time across a number of different airports (Baxter et al., 2018a, 2018b; ICAO Secretariat, 2022).
The waste management hierarchy ranks the various types of waste disposal methods from the most to the least desirable. Airports have choices in how to manage collection, treatment, storage, and disposal. When these choices are considered and carried out beneficially, they can improve airport operations and minimize environmental impact. A successful waste management process includes the separation of solid and liquid waste, differentiation between hazardous and non-hazardous waste, and the implementation of waste reduction techniques. Additionally, strategies to enhance reuse, recycling, and reprocessing play a crucial role in this process (Baxter et al., 2018a, 2018b). Some airports have implemented waste-to-energy (WtE) systems. These systems help minimize the environmental impact of waste disposal by converting waste into energy. WtE involves generating and utilizing energy from treated solid wastes (Tayeh et al., 2021). This process has become a key tool in modern waste management and energy generation (Ahmadi et al., 2019). Furthermore, WtE supports a circular economy by reducing the volume of waste sent to landfills, thereby avoiding the least desirable disposal method (Moshood et al., 2022; Biron, 2020; Williams, 2013).
For example, Oslo Airport (OSL) recycles waste and transforms it into new materials or energy. OSL is a leading example of commitment to circular economy and waste management principles, with a dedicated section in their Annual Sustainability Report highlighting these achievements. They have set a target of zero unsorted waste by 2030.
In our sample, 40% of the airports reported handling hazardous waste. However, it is common practice for airports to sort their waste. For instance, London Heathrow Airport and Porto International Airport discharges zero waste to landfills. Evidence shows that in most cases, waste is recovered, repurposed, or recycled. Porto International Airport reports a waste recovery rate of 98%. Avinor, the operator of Oslo Airport, aims for zero waste by 2030. This vision involves halving the quantity of unsorted waste by 2025 and achieving 100% reuse or material recycling. They also target a 50% reduction in food waste per passenger by 2030 and increasing recycling/reuse rates in construction projects to a minimum of 70% by 2025.
Porto International Airport has a success story to share regarding food waste. By working with Refood, they donate uneaten meals to needy families every day, effectively fighting food waste. This initiative contributes to reducing food waste and reducing the greenhouse gas emissions associated with food waste, as well as having a social nature. In 2022, roughly 30,000 meals were donated, corresponding to 17,000 kilograms of food. Additionally, Helsinki airport recycles all clothes worn out in customer service tasks and some decommissioned work wear. These are flagships of the circular practice approach as they demonstrate in a remarkable manner an example of closing the loop. In 2022, Airpro at Helsinki Airport delivered 970 kg of textile waste to a closed loop, avoiding the burning of textile waste and reducing emissions by 970 kg CO₂. Water consumption was reduced by 509,250* liters by using recycled textiles instead of the corresponding amount of virgin cotton/polyester fiber.
4.4 Water
The reuse of water has been one of the main challenges of airport management, particularly in collecting rainwater for reuse in sanitary facilities, which is not an easy task in older airports. Its implementation and integration into different airports are being studied. Airports utilize water for various internal operations, including toilet flushing, food preparation, and HVAC systems, as well as external activities such as irrigation, aircraft and infrastructure washing, and maintenance. The water consumption at major airports is significant, often comparable to that of small and medium-sized cities (Dimitriou and Karagkouni, 2022a). While daily water consumption is a common metric for analyzing airport water usage, it does not provide a comprehensive picture of the types of water sources used or the management strategies that yield the best results (De Castro Carvalho et al., 2013; cited in Dimitriou and Karagkouni, 2022b).
Strategies include the assessment of water use at airports; the installation of water-efficient fixtures and fittings; the reduction of irrigation needs wherever possible; and use of non-potable sources of water, including rainwater, gray water and treated wastewater. It is reckoned that a high proportion of water use at airports is for applications that does not require potable water (Baxter et al., 2018a) Thus, airports implement water-saving measures such as efficient irrigation systems and water recycling in order to save water, protect local ecosystems, and safeguard resources. Besides, responsible guidelines about water use can be developed within the airport community in order to reduce water demand successfully, especially in regions experiencing drought, which improves the quality of life for locals (Zhu et al., 2004).
Some notable examples of water reuse are presented below. The Nice Côte d’Azur Airport (NCE) uses a warm water loop between the airport and the Haliotis water treatment plant, which collects heat from wastewater. This system provides heating in winter and air conditioning in summer, using renewable resources. Additionally, NCE invests in enhanced handling facilities and processes to mitigate the risk of groundwater and surface water pollution from de-icing operations and fuel handling equipment. Athens International Airport (ATH) recycles water through its industrial wastewater treatment facility and monitors surface water quality through an Online Monitoring System (OWMS). This system ensures that water discharge meets environmental standards before being released offsite. Helsinki Airport has implemented an underground wetland to manage extreme water flow and improve water quality in the Veromiehenkylänpuro brook. This pioneering structure in Finland helps mitigate the adverse impacts of de-icing and anti-icing agents and is rare at the European level.
In a similar direction, Helsinki Airport piloted the recycling of glycol, yielding promising outcomes. In this recycling process, used glycol-containing water collected from the asphalt is concentrated using advanced technology and purified for reuse. Additionally, the Nice Côte d’Azur water treatment plant collects heat from wastewater supplied by the towns around Nice. It collects heat from wastewater from showers, washing machines, and dishwashers of thousands of nice residents’ and uses it to heat the airport terminal. In summer, the system switches to air conditioning mode, collecting frigories from the Var’s particularly cold groundwater supply. The analysis reveals notable differences in emissions intensity and water usage per passenger among the airports studied. Higher emissions intensities observed at airports like Helsinki and Malpensa can be attributed to climatic factors necessitating extensive heating and energy-intensive operations, as well as high levels of international traffic that contribute to increased ground operations and longer aircraft taxi times. In contrast, airports such as Palma de Mallorca, which cater predominantly to seasonal tourism, exhibit lower emissions intensities due to operational efficiencies during peak travel seasons. Similarly, greater water usage per passenger at Malpensa and Nice Côte d’Azur can be linked to factors such as extensive landscaping and water-intensive cooling systems, highlighting areas where water recycling and conservation measures may reduce consumption. The following graph Figure 2 illustrates normalized environmental performance metrics across selected European airports, offering a clear comparative visualization of key sustainability indicators, including energy intensity, emissions intensity, waste intensity, and water intensity per passenger. Missing data points are marked clearly with zeros to highlight reporting deficiencies that occur often and the urgent call for increasing data openness. The visualization supports airport administrators and stakeholders to identify relative strengths, recognize best practices, and establish key areas to drive forward with airport sustainability.
To further contextualize the performance of environmental practices across these airports, we have plotted the current findings against industry benchmarks. For example, the ACA program sets a progressive target in terms of emissions intensity per passenger. ICAO benchmarks are that airports committed to environmental leadership would have below 1.5 kg CO₂ per passenger. With only 40% of the current sample reaching or surpassing this threshold, which calls for new means of reducing emissions, this certainly calls for more to be done. GRI 303 regarding water usage recommends per passenger water consumption reduction by adopting recycling and using sustainable supplies. Although 60% of airports have implemented certain measures of water reuse, the findings highlight further areas of potential improvement, particularly for those airports whose performances exceed the threshold of 0.05 m3/passenger. To provide a clear, actionable summary for practitioners, a table comparing the study’s environmental metrics with industry benchmarks and suggested improvements for each category is presented below (Table 5).
A comparative analysis between airports with advanced CE implementations and those with limited CE adoption reveals distinct patterns in operational strategies, resource management, and environmental performance (Table 6). For instance, airports such as Oslo and Helsinki, which have incorporated robust CE practices, demonstrate higher recycling rates and lower emissions intensities per passenger compared to airports like Nice Côte d’Azur and Malpensa, which have not fully adopted CE frameworks. Key factors enabling CE adoption in these advanced airports include supportive regulatory frameworks, dedicated sustainability teams, and investments in data infrastructure for real-time resource tracking.
5 Discussion
Best practices observed among CE-leading airports include proactive engagement with CE principles through initiatives such as comprehensive waste sorting and recycling, electrification of ground support equipment, and installation of renewable energy sources like solar panels. Enablers of CE adoption include regulatory alignment with national sustainability goals, as seen in Scandinavian countries, where environmental regulations encourage circular practices. Additionally, organizational culture that prioritizes sustainability and collaboration with technology vendors for BI and IoT infrastructure are significant enablers. For example, Oslo Airport’s zero-waste target, achieved through collaborative partnerships, underscores the importance of cross-sector collaboration.
As it is evident in Table 4 Energy consumption per passenger varies significantly across airports, from 0.26 GJ/passenger at ARL to 5.64 GJ/passenger at MXP. This suggests that there are substantial differences in energy efficiency practices and infrastructure among these airports. Particularly the lowest value observed is the one of ARL (0.26 GJ/passenger x) while the highest the one of MXP (6 GJ/passenger x). Extreme values are observed in the cases of MPX and HEL, while the rest of the sample values are more closely aligned. The highest energy intensity at MXP (5.64 GJ/passenger) and HEL (4.41 GJ/passenger) indicates potential inefficiencies or higher energy demands that could be addressed to improve overall efficiency.
Emissions intensity per passenger ranges from 0.11 to 4.04 (kg CO₂ -equivalent/passenger), with an average value of 1.47. The lowest emissions intensity is at PMI (0.11 kg CO₂ -equivalent/passenger), and the highest is at HEL (4.04 kg CO₂ -equivalent/passenger). HEL, ARL and MXP show extreme values, whereas the other airports have more consistent emissions intensities. The wide range in emissions intensities suggests there is significant potential for many airports to adopt best practices from their lower-emitting counterparts.
To reduce emissions within airport operations, deploying real-time emissions monitoring tools and electrifying ground support equipment (GSE) is recommended. Airports can utilize BI tools to monitor Scope 1 emissions from vehicles and equipment in real time, adjusting operations to optimize fuel usage. Predictive analytics can be applied to identify peak times for emissions, allowing airports to proactively manage resource allocation. For instance, during high-traffic hours, electrified GSE can be prioritized for tasks such as baggage handling and aircraft servicing. Implementing renewable energy sources, like solar panels for terminal operations, can also reduce Scope 2 emissions while lowering operational costs.
Waste intensity per passenger ranges from 0.12 to 0.23 (kg/passenger), averaging 0.18 (kg/passenger). OPO has the lowest waste intensity (0.12 kg/passenger), while HEL has the highest (0.23 kg/passenger). The waste intensities of OPO and HEL are at the extremes, with the other airports showing more uniform values. Waste intensity per passenger is relatively consistent, averaging 0.18 kg/passenger, with values ranging from 0.12 kg/passenger at OPO to 0.23 kg/passenger at HEL. However, airports with higher waste intensities like HEL could explore more effective waste reduction and recycling programs. Given the close alignment of most airports, implementing standardized waste management practices could help reduce the higher values.
From the perspective of solid waste management, prediction analytics brings a number of great benefits: it allows forecasting waste generation based on predictive models for passenger flows and event schedules, optimizes collections and sorting. This will prevent landfills from overfilling, improving percentages of recyclable items and eventually depleting the reserves. A very good example is London Stansted, which has put into practice very effective predictive waste analytics for optimizing the positioning of recycling bins during periods of high traffic to ensure improved waste segregation, therefore enhancing recycling efficiency. Further investment in automated sorting technologies and waste-to-energy systems can improve these outcomes and support the goals of the circular economy with reduced environmental impacts.
Water use per passenger varies: MXP has 0.08 m3/passenger, which is much higher than the values in OPO and HEL, 0.01 m3/passenger. There is such a big difference that it underlines the possibility to realize water-saving measures for those airports where this indicator is higher. The minimum value for OPO and HEL is 0.01 m3/passenger, while for MXP it is 0.08 m3/passenger. The value for MXP is an outlier; the rest of the airports have quite similar values.
The recommendations are given on various paths of improvements that help practitioners attain low emissions and water usage intensities. Among these are investments by airports in electrified ground support equipment, increasing the use of renewable energy sources like solar and wind power, and installation of energy-efficient systems for lighting, HVAC, and de-icing operations, all of which can greatly reduce energy demand. For instance, those with high usage could be engineered to have an advanced water recycling mechanism and thus use rainwater harvesting methods to reduce dependency on potable water sources. Such would be an industry-leading practice like Oslo and Athens International, acting like a yardstick to those airports wanting to increase resource efficiency.
Examples are provided to bring out the pragmatic importance of such BI tools in existing airport operations. For instance, predictive models make use of passenger inflow data at Athens International Airport to work out logistical issues related to waste sorting on the spot so that timely collection and segregation increase the efficiency of recycling and reduce landfill contributions. There has been the deployment of real-time monitoring systems at London Stansted to track the energy consumption arising from HVAC, thus enabling immediate adjustment by occupancy and outdoor temperature, hence avoiding excess energy use. These examples show how BI tools are playing their part in resource efficiency improvements and circular economy adoptions within an airport context.
Modern airport sustainability strategies go beyond waste and emission management to the management of the life cycles of products and closed-loop systems. A good example is Oslo Airport, with its closed-loop water system that captures, treats, and reuses water used in de-icing processes, reducing its resource usage and environmental impact. Athens International Airport has also implemented lifecycle management for the electronic waste it produces, liaising with vendors to refurbish and repurpose parts to extend the life of the product and reduce the amount sent to landfills. These examples show how airports operationalize CE principles, applying theoretical models to lifecycle and closed-loop strategies in their operational practice.
To actively drive the practice of Circular Economy (CE), it is essential that airports take proactive roles in advocating inclusive regulatory schemes. Such endorsement can be heightened through coordinated initiatives with aviation industry associations like Airports Council International (ACI), together with collaborative approaches with government and worldwide environmental agencies (González-Ruiz et al., 2017; Dimitriou and Karagkouni, 2022b). Such collaborations can be used by airports to present fact-based evidence of effective implementations of CE to showcase tangible economic, environmental, and functional benefits that come with circular approaches (Popović et al., 2022; MacArthur, 2013).
Additionally, effective funding mechanisms play a vital role in supporting extensive circular economy (CE) projects in airports. In line with this imperative, airports should aggressively pursue different financial instruments such as public-private collaborations, grant-based schemes with a focus on sustainability that can be accessed through initiatives like those provided by the European Green Deal (European Commission, 2021), green bonds, and environmental investment vehicles with a focus on special sectors. Having a clear linkage between envisioned CE projects and current frameworks supporting sustainability increases their attractiveness to potential investors and policymakers (Dimitriou et al., 2025). Having specialized working groups handling long-term financial planning and securing funding can help boost an airport’s financial resilience to challenges and speed up efficient implementation of circular economy principles.
For airports in the early stages of CE adoption, several common barriers are apparent. Limited access to funding for initial technology investments, regulatory gaps, and organizational resistance to operational changes are primary obstacles. Airports like Nice Côte d’Azur and Malpensa cite financial constraints as a significant barrier to implementing large-scale CE initiatives. The other relevant barrier to effective implementation and monitoring of progress is the lack of standardized metrics that indicate performance on CE and inconsistency of data between departments. These barriers indicate that financing options and a common standard for CE may facilitate broader industry adoption.
The findings of this study offer significant practical suggestions for airport managers wishing to enhance their sustainability initiatives through the adoption of Circular Economy (CE) principles. Airport operators are advised to undertake strategic investments in renewable energy technologies, such as solar photovoltaic and wind power installations, that not only assist in fulfilling regulatory imperatives but also result in significant cost benefits and environmental gains. Additionally, the implementation of extensive recycling and waste minimization programs, as well as efficient water management systems, constitute actionable solutions that airports can immediately adopt to enhance their environmental performance. Despite these opportunities, there are many challenges that confront the effective implementation of CE. Budgetary limitations continue to pose a significant hindrance, often preventing crucial upfront investments in infrastructure and technological innovation. To overcome these constraints, airports should actively pursue diverse funding sources such as sustainability grants, green bonds, or public-private partnerships. Another apparent challenge is organizational resistance, often stemming from deep-rooted operational routines or limited awareness of the advantages of CE among employees. Overcoming this challenge requires targeted capacity-building initiatives, including training programs, workshops, and the creation of specialized teams with the mandate to guide and monitor CE initiatives.
Additionally, lack of integrated and consistent information systems impedes evaluation and management of sustainability performance and may hamper adoption of a circular economy (Sartzetaki et al., 2023). Prioritization of setting up advanced information analytical systems together with consistent sustainability reporting indicators by airport management can enhance effective collection of data, increase openness, and enhance decision-making. Adoption of recommended pragmatic approaches while facing such issues proactively can help operators of airports navigate complexity in adopting a circular economy effectively and thus increase their business operations sustainability and competitive edge.
This paper develops theoretical understanding of CE for the aviation industry, particularly focusing on how BI tools facilitate closed-loop and data-driven practices of CE. The findings strengthen the applicability of the 9Rs framework to airport operations and illustrate ways in which data analytics can make the principles of CE tangible within an industrial context traditionally tied to very high resource consumption. It also contributes to the theory of sustainable supply chain management by showing how BI can be extended for key environmental metrics, such as emissions and waste. This may bring an important theoretical contribution to fill some gaps in the current literature on the CE and indicate specifically in the aviation context that switching toward circularity needs data-driven practices.
6 Conclusion
This study provides a comprehensive analysis of likely pathways and executable strategies for airports moving toward Circular Economy (CE)-aligned operations, focusing mostly on sustainability and resource efficiency. The study outlines that significant environmental and operational benefits can be achieved through strategic investments in renewable energy projects, such as photovoltaic and wind power projects. These green and sustainable initiatives not only help airports meet regulatory requirements but also deliver significant reductions in greenhouse gas emissions and lower operational costs. Furthermore, the study highlights the indispensable importance of sound energy management policies, such as the use of energy-efficient HVAC systems, integration of renewable energy sources into airport infrastructures, and implementation of advanced IoT-equipped sensors. Those airports that have been able to integrate these technologies, as observed in the case of Helsinki and Palma de Mallorca, reflect significant energy efficiency improvements that are commensurate with measurable reductions in total energy intensity.
Implementation of efficient waste management practices forms a critical part of the shift toward Circular Economy (CE) strategies. This analysis outlines the benefits that emanate from high-performance recycling and smart waste-to-energy initiatives, illustrated by Oslo and Porto airports’ achievement of high waste recovery levels coupled with remarkable reductions in environmental impact associated with waste. The management of water resources through avenues like rainwater harvesting, recycling of wastewater, and high-performance irrigation systems has also been evidenced to record improved sustainability outcomes. Airports like Nice Côte d’Azur and Helsinki have successfully embraced these technologies and achieved high rates of water saving. Significantly, the adoption of Business Intelligence (BI) technologies has emerged as a fundamental driver toward increasing resource efficiency and sustainability performance. The use of real-time analytics, predictive models, and Internet of Things (IoT)-based systems allows airports to efficiently monitor, analyze, and optimize their use of resources, making significant contributions to the improvement of their sustainability performance. The findings clearly reveal that airports with superior BI capabilities reflect better environmental performance, especially regarding emissions reduction and waste management.
The research recommends that airport authorities make strategic investments in renewable energy infrastructure, adopt holistic waste recycling and management systems, and utilize advanced water conservation technologies. In addition, organizational structures should include specialized sustainability teams, extensive employee training programs, and the deliberate incorporation of advanced business intelligence solutions. Policymakers are urged to develop dedicated funding mechanisms to support initial capital investments in sustainable airport infrastructures and technologies. The development of standardized sustainability metrics, as well as the promotion of open data-sharing platforms, is a key step toward realizing consistent performance measurement and benchmarking. Capacity-building initiatives, with a focus on skills development and organizational readiness, are also essential for overcoming barriers to the adoption of a circular economy. Future research should focus on longitudinal studies of circular economy initiatives to evaluate their long-term effectiveness and sustainability outcomes. Comparative international research would greatly enhance the understanding of best practices and areas for improvement across different contexts. Additionally, the analysis of advanced business intelligence applications and predictive analytics technologies is expected to provide insightful information on their ability to optimize resource management and accelerate sustainability transitions in airport operations.
Data availability statement
The original contributions presented in the study are included in the article, further inquiries can be directed to the corresponding author.
Author contributions
MS: Conceptualization, Formal analysis, Investigation, Methodology, Resources, Validation, Writing – original draft, Writing – review & editing. TK: Data curation, Formal analysis, Investigation, Methodology, Visualization, Writing – original draft. AK: Data curation, Formal analysis, Investigation, Methodology, Visualization, Writing – original draft. ED: Project administration, Resources, Supervision, Validation, Writing – review & editing. DD: Conceptualization, Formal analysis, Methodology, Project administration, Supervision, Validation, Visualization, Writing – review & editing.
Funding
The author(s) declare that no financial support was received for the research and/or publication of this article.
Conflict of interest
The authors declare that the research was conducted in the absence of any commercial or financial relationships that could be construed as a potential conflict of interest.
Generative AI statement
The authors declare that no Gen AI was used in the creation of this manuscript.
Publisher’s note
All claims expressed in this article are solely those of the authors and do not necessarily represent those of their affiliated organizations, or those of the publisher, the editors and the reviewers. Any product that may be evaluated in this article, or claim that may be made by its manufacturer, is not guaranteed or endorsed by the publisher.
References
ACI Europe. (2022). Airport carbon accreditation: a framework for achieving carbon neutrality in airports. Available online at: https://www.airportcarbonaccreditation.org (Accessed July 16, 2024).
Ahmadi, A., et al. (2019). Benefits and limitations of waste-to-energy conversion in Iran. Renewable Energy Research and Applications. 1. doi: 10.22044/rera.2019.8666.1007
Alba, S., and Mañana, M. (2016). Energy research in airports: a review. Energies 9:349. doi: 10.3390/en9050349
Asian Development Bank (2024). Asia's journey to a circular economy: A guide for policy makers and business leaders. Mandaluyong: Asian Development Bank.
Barney, J. (1991). Firm resources and sustained competitive advantage. Journal of Management, 17, 99–120. doi: 10.1177/014920639101700108
Baxter, G., and Srisaeng, P. (2022). Optimizing airport sustainable waste management from the use of waste-to-energy technology and circular economy principles: the case of London Gatwick airport. International Journal for Traffic and Transport Engineering (IJTTE). 12. 176–185. doi: 10.7708/ijtte2022.12(2).03
Baxter, G., Srisaeng, P., and Wild, G. (2018a). An assessment of airport sustainability, part 1—waste Management at Copenhagen Airport. Resources 7:21. doi: 10.3390/resources7010021
Baxter, G., Srisaeng, P., and Wild, G. (2018b). An assessment of airport sustainability, part 2—energy Management at Copenhagen Airport. Resources 7:22. doi: 10.3390/resources7010022
Biron, M. (2020). “Industrial waste as a resource” in Waste plastics: Upgrading, recycling, and other paths to add value. eds. A. K. Panda and R. K. Singh (Cham: Springer), 1–20.
Bleischwitz, R., et al. (2022). The circular economy in China: Achievements, challenges and potential implications for decarbonisation, Resources, Conservation and Recycling. 183. doi: 10.1016/j.resconrec.2022.106350
Burneo, K., Cruz, N., and Molina, A. (2020). Circular economy in emerging markets: insights from Latin America. J. Clean. Prod. 260:120714. doi: 10.1016/j.jclepro.2020.120714
Castillo Malagón, R., Cruz Reyes, M. A., and Romero Saldaña, R. S. (2024). Circular economy: a technological innovation strategy for sustainability in air transport. Mercados y Negocios 25, 31–52. doi: 10.32870/myn.vi52.7726
Celikel, A., Rötger, T., and Casas, G. (2022). Study – assessment of the environmental sustainability status in the aviation maintenance and production organisation (M&P) domain. Cologne: The European Union Aviation Safety Agency.
Circular Economy. (2022). The circularity gap report 2022. Circle Economy. Available online at: https://www.circularity-gap.world/ (Accessed July 16, 2024).
Cokorilo, O. (2016). “Environmental issues for aircraft operations at airports” in Transportation research Procedia. ed. M. Gastaldi (Amsterdam: Elsevier).
D’Amato, D., and Korhonen, J. (2021). Integrating the green economy, circular economy and bioeconomy in a strategic sustainability framework. Ecol. Econ. 188:107143. doi: 10.1016/j.ecolecon.2021.107143
De Angelis, R. (2024). Circular economy business models as progressive business models: Evidence from circular start-ups. Business Strategy and the Environment, 33, 6303–6314. doi: 10.1002/bse.3821
De Castro Carvalho, F., Duarte, B., and Lima, P. (2013). Water Management in Airports: current practices and future trends. J. Environ. Manag. 119, 20–28. doi: 10.1016/j.jenvman.2013.01.021
Dimitriou, D. (2022). Corporate ethics: an arbitration of philosophical concepts and market practices. CONATUS Int. J. Philos. 7, 33–60. doi: 10.12681/cjp.29864
Dimitriou, D., and Karagkouni, A. (2022a). Assortment of airports’ sustainability strategy: a comprehensiveness analysis framework. Int. J. Sust. 14:4217. doi: 10.3390/su14074217
Dimitriou, D., and Karagkouni, A. (2022b). ‘Airports’ sustainability strategy: evaluation framework upon environmental awareness. Front. Sust. 3:880718. doi: 10.3389/frsus.2022.880718
Dimitriou, D., and Sartzetaki, M. (2022a). Modified fuzzy TOPSIS assessment framework for defining large transport enterprises business value. Operational Res. 22, 6037–6053. doi: 10.1007/s12351-022-00719-9
Dimitriou, D., and Sartzetaki, M. (2022b). Criticality of a regional airport development to mitigate covid-19 economic effects. Int. J. Case Stu. Transport. Policy 10, 581–590. doi: 10.1016/j.cstp.2022.01.018
Dimitriou, D., Sartzetaki, M., and Karagkouni, A. (2024). Managing airport corporate performance: Leveraging business intelligence and sustainable transition. Amsterdam: Elsevier.
Dimitriou, D., Sartzetaki, M., and Karagkouni, A. (2025). Airport landside area planning: an activity-based methodology for seasonal airports. Transp. Res. Proc. 82, 1167–1184. doi: 10.1016/j.trpro.2024.12.119
Ellen MacArthur Foundation (2022). Completing the picture: how the circular economy tackles climate change. England: Ellen MacArthur Foundation.
European Commission. (2021). Sustainable aviation fuels (saf) and other alternative fuels used for aviation. Available at: https://alternative-fuels-observatory.ec.europa.eu/transport-mode/aviation/general-information-and-context
Geissdoerfer, M., Savaget, P., Bocken, N., and Jan Hultink, E. (2017). The circular economy – a new sustainability paradigm?, Journal of Cleaner Production. 143, 757–768. doi: 10.1016/j.jclepro.2016.12.048
Ghisellini, P., Cialani, C., and Ulgiati, S. (2017). A review on circular economy: the expected transition to a balanced interplay of environmental and economic systems. Journal of Cleaner Production. 114, 11–32, doi: 10.1016/j.jclepro.2015.09.007
Ginga, C. P., Ongpeng, J. M. C., and Daly, M. (2020). Circular economy on construction and demolition waste: a literature review on material recovery and production. Materials 13:2970. doi: 10.3390/ma13132970
Global Reporting Initiative (2016). GRI 303: Water and effluents 2016. Amsterdam: Global Reporting Initiative Standards.
González-Ruiz, R., Sáez-Martínez, F. J., and Díaz-García, C. (2017). Green airports: the role of inter-organizational networks in the development of sustainability capabilities. J. Clean. Prod. 164, 410–419. doi: 10.1016/j.jclepro.2017.06.218
Karagkouni, A., and Dimitriou, D. (2022). ‘Sustainability performance appraisal for airports serving tourist islands’. Sustainability, 14: 13363. doi: 10.3390/su142013363
Khaw-ngern, K., Peuchthonglang, P., Klomkul, L., and Khaw-ngern, C. (2021). The 9Rs strategies for the circular economy 3.0. Psychol. Educ. J. 58, 1440–1446. doi: 10.17762/pae.v58i1.926
Kok, R., Balkenende, A. R., and Kremer, D. (2013). “Product design strategies for a circular economy: design experiences in practice,” in Proceedings of the 19th International Conference on Engineering Design (ICED13), Seoul, Korea, August 19–22.
Korhonen, J., Honkasalo, A., and Seppälä, J. (2018). Circular economy: the concept and its limitations. Ecol. Econ. 143, 37–46. doi: 10.1016/j.ecolecon.2017.06.041
Kristoffersen, E., Blomsma, F., Mikalef, P., and Li, J. (2020). The smart circular economy: a digital-enabled circular strategies framework for manufacturing companies. J. Bus. Res. 120, 241–261. doi: 10.1016/j.jbusres.2020.07.044
Lobo, A, Hofmann Trevisan, A, Liu, Q, et al. (2021). “Barriers to transitioning towards smart circular economy: A systematic literature review,” in SDM-2021 8th International Conference on Sustainable Design and Manufacturing, 16-17 September 2021, Split, Croatia. doi: 10.1007/978-981-16-6128-0_24
MacArthur, E. (2013). Towards the circular economy: Economic and business rationale for an accelerated transition. New York, NY: Ellen MacArthur Foundation.
Mishra, R., Hopkinson, P., and Tidridge, G. (2019). Implementing circular economy principles in supply chains: a framework for business model innovation. J. Ind. Ecol. 23, 496–507. doi: 10.1111/jiec.12759
Moshood, A., Olawale, M., and Temitope, R. (2022). Waste‐to‐energy nexus: An overview of technologies and implementation for sustainable development. Cleaner Energy Systems. 3. doi: 10.1016/j.cles.2022.100034
Oliveira-Dias, J., Pimentel, P., and Martins, P. (2022). The role of digital Technologies in the Circular Economy Transition. Resour. Conserv. Recycl. 177:105973. doi: 10.1016/j.resconrec.2021.105973
Popović, A., Ivanović-Djukić, M., and Milijić, A. (2022). Assessment of the impact of circular economy competitiveness and innovation on European economic growth. Eur. J. Appl. Econ. 19, 57–70. doi: 10.5937/EJAE19-39057
Porter, M. E. (1985).The competitive advantage: creating and sustaining superior performance. NY: Free Press. (Republished with a new introduction, 1998).
Rashwan, A. K., Bai, H., Osman, A. I., Eltohamy, K. M., Chen, Z., Younis, H. A., et al. (2023). Recycling food and agriculture by-products to mitigate climate change: a review. Environ. Chem. Lett. 21, 3351–3375. doi: 10.1007/s10311-023-01639-6
Ravishankar, B., and Christopher, B. (2022). Environmental threats: exploring waste management in the indian aviation sector. ECS Transactions. 107. 10811–10819. doi: 10.1149/10701.10811ecst
Rockström, J., Steffen, W., Noone, K., Persson, Å., Chapin, F. S. III, Lambin, E., et al. (2009). A safe operating space for humanity. Nature 461, 472–475. doi: 10.1038/461472a
Saari, U. A., et al. (2022). “The influence of circular economy implementation on competitiveness in manufacturing companies,” 2022 IEEE 28th International Conference on Engineering, Technology and Innovation (ICE/ITMC) & 31st International Association For Management of Technology (IAMOT) Joint Conference, Nancy, France, 2022, 1–8. doi: 10.1109/ICE/ITMC-IAMOT55089.2022.10033154
Sarbassov, Y., Kalisz, S., and Valle, J. (2020). Waste management practices at airports: a review. Sustain. For. 12:380. doi: 10.3390/su12010380
Sartzetaki, M., Karagkouni, A., and Dimitriou, D. (2023). A conceptual framework for developing intelligent services (a platform) for transport enterprises: the designation of key drivers for action. Electronics 12:4690. doi: 10.3390/electronics12224690
Sebastian, R. M., and Louis, J. (2021). Understanding waste Management at Airports: a study on current practices and challenges based on literature review. Renew. Sust. Energ. Rev. 147:111229. doi: 10.1016/j.rser.2021.111229
Stucki, T., Woerter, M., and Loumeau, N. (2023). Clearing the fog: How circular economy transition can be measured at the company level. Journal of Environmental Management. 326:Part B. doi: 10.1016/j.jenvman.2022.116749
Tayeh, B., et al. (2021). Performance of sustainable concrete containing different types of recycled plastic, Journal of Cleaner Production. 328. doi: 10.1016/j.jclepro.2021.129517
Tjahjono, M., Ünal, E., and Tran, T. H. (2023). The circular economy transformation of airports: an alternative model for retail waste management. Sustain. For. 15:3860. doi: 10.3390/su15043860
Tsironis, G., Karagkouni, A., Dimitriou, D., and Tsagarakis, K. P. (2023). Mapping sustainable practices and concepts in the transportation ecosystem for the EU-27 countries, based on LinkedIn company profiles. Front. Sust. 4:1268575. doi: 10.3389/frsus.2023.1268575
Walker, P. H., Seuring, P. S., Sarkis, J., and Klassen, R. (2014). Sustainable operations management: recent trends and future directions. Int. J. Oper. Prod. Manag. 34:5. doi: 10.1108/IJOPM-12-2013-0557
Yang, M., Chen, L., Wang, J., Msigwa, G., Osman, A. I., Fawzy, S., et al. (2022). Circular economy strategies for combating climate change and other environmental issues. Environ. Chem. Lett. 21, 55–80. doi: 10.1007/s10311-022-01499-6
Zhang, M., Zhang, D., and Yang, Y. (2023). Green bond and trade openness effects on sustainable business practices in natural resource markets. Resources Policy. 86:Part A. doi: 10.1016/j.resourpol.2023.104188
Keywords: circular economy, sustainable development, airport operators, business intelligence, environmental sustainability, sustainable strategies
Citation: Sartzetaki M, Karampini T, Karagkouni A, Drimpetas E and Dimitriou D (2025) Circular economy strategies in supply chain management: an evaluation framework for airport operators. Front. Sustain. 6:1558706. doi: 10.3389/frsus.2025.1558706
Edited by:
Giacomo Di Foggia, University of Milano-Bicocca, ItalyReviewed by:
Dimitrios Paparas, Harper Adams University, United KingdomLorenzo Zanolo, University of Milano-Bicocca, Italy
Copyright © 2025 Sartzetaki, Karampini, Karagkouni, Drimpetas and Dimitriou. This is an open-access article distributed under the terms of the Creative Commons Attribution License (CC BY). The use, distribution or reproduction in other forums is permitted, provided the original author(s) and the copyright owner(s) are credited and that the original publication in this journal is cited, in accordance with accepted academic practice. No use, distribution or reproduction is permitted which does not comply with these terms.
*Correspondence: Aristi Karagkouni, YXJrYXJhZ2tAZWNvbi5kdXRoLmdy