- Leeds School of Architecture, Leeds Beckett University, Leeds, United Kingdom
The fast fashion industry’s rapid growth has led to about 92 million tons of textile waste each year and contributes 10% of global carbon emissions, which is expected to rise by 50% by 2030. This study examines a sustainable method for upcycling textile waste using the edible mushroom Pleurotus ostreatus. Thirteen fabric substrates, consisting of both natural and synthetic fibers, were cultivated on four growth media—malt extract agar (MEA), malt extract yeast agar (MYA), potato dextrose agar (PDA), and potato dextrose yeast agar (PDYA)—to assess their effectiveness in supporting mycelium growth, morphological characteristics, and biomass yield. Results showed that all fabric substrates supported mycelium growth, with MEA, MYA, and PDYA emerging as the most effective growth media. PDYA produced the highest biomass yield, highlighting its potential for upcycling applications. Statistical analysis demonstrated the significant effect of growth media on the lag and exponential growth phases, underscoring the importance of nutrient-rich environment conditions for optimized growth. This study confirms the viability of utilizing Pleurotus ostreatus mycelium for transforming textile waste, with the potential to provide an innovative and eco-friendly approach to reducing the fashion industry’s environmental footprint.
1 Introduction
Fast fashion has become the preferred business model for the fashion industry. This model relies on selling large volumes of clothing at low prices, significantly influencing how people consume and dispose of clothes (Bick et al., 2018). The fashion industry has been scrutinized for its environmentally damaging supply chain practices. Despite its significant environmental impact, the fashion industry continues to expand, driven by low-cost labor, mass production, and high consumer demand (Niinimäki et al., 2020). The environmental damage caused by the industry is extensive and well-documented, which has resulted in ongoing negative publicity. For example, Bick et al. (2018) reported that consumers worldwide purchased 80 billion tons of new clothing annually, which has led to the generation of approximately 92 million tons of textile waste globally. 85% of this waste is incinerated or disposed of in landfills (Niinimäki et al., 2020).
Moreover, the fashion industry currently accounts for 10% of global carbon emissions, a figure expected to rise by 50% by 2030 (Bick et al., 2018). Global water consumption is also significant, with material cultivation and manufacturing processes such as bleaching, dyeing, printing, and finishing accounting for approximately 79 trillion liters of water annually (Niinimäki et al., 2020). The fashion industry uses 60% of the world’s fiber production, with cotton being the most widely used natural fiber, which accounts for one-third of total fibers manufactured worldwide (Grishanov, 2011; Niinimäki et al., 2020; Voora et al., 2020). In addition, cotton production requires heavy pesticide use, with the sector responsible for applying 16% of insecticides and 6.8% of herbicides globally (Innovation Forum, 2015; Voora et al., 2020; Workman, 2019). Cotton has the highest water footprint of any fashion fiber, which accounts for 1,559 liters of freshwater per kilogram (Niinimäki et al., 2020). The rise of fast fashion has turned the fashion industry into a significant environmental concern, underscoring the urgent need for innovative practices and reforms to help mitigate its ecological impact.
The enormous amounts of textile waste the fashion industry generates globally present an opportunity to upcycle these fabrics. One promising material innovation with the potential to help the fashion industry reduce its waste problem is mycelium-based materials. This new material innovation has been used to develop a sustainable alternative to leather (Raman et al., 2022). It has demonstrated the potential to transform agricultural waste materials into eco-friendly solutions suitable for thermal and acoustic applications (Al-Qahtani et al., 2023). Although there is increasing interest in mycelium-based materials, few studies have examined their potential for upcycling textile waste. This study aims to address this gap by evaluating the morphogenesis of mycelium growth as a means to upcycle end-of-life textile waste from the fashion industry. In a study by Helberg et al. (2019), the authors examined the growth of Pleurotus ostreatus on various textile waste materials for vertically farming edible mushrooms. Globally, only 12% of materials used for clothing production are recycled (Igini, 2023). According to Beall (2020), the problem largely stems from the materials used for clothing and the inadequate technologies available for recycling them. In this study, the edible mushroom Pleurotus ostreatus (blue-grey oyster mushroom) is cultivated on 13 different fabric substrates to assess the impact of four different growth media on mycelium development, morphological characteristics, and dry biomass production.
2 Materials and methods
All materials and equipment used in the study were sterilized by autoclave and prepared aseptically in a laminar flow chamber. Mycelial spawn, Pleurotus ostreatus (strain M2191), was purchased from Urban Farm It Ltd., UK, as a mycelial mass on digested wheat grain sealed in a plastic bag with filter patches. The fabric materials were obtained from the BA(Hons) Fashion Design course at the School of Arts, Leeds Beckett University, UK. The fabric samples under examination were cotton gauze (F1); polyester chiffon (white) printing (F2); ice wool medium (white) (F3); calico light (F4); white poplin cotton Delphina shrunk (F5); Merz cotton natural jersey (F6); jersey silk Noil natural (tubular) (F7); ecru cotton jersey (tubular) (F8); 100% wool light grey (F9); 100% wool dark grey (F10); raised natural cotton (F11); white jersey cotton pimenta sweatshirt (F12); and 100% heavy denim natural cotton (F13). Fabric samples were chosen based on a combination of synthetic (polyester) and natural fibers (cotton, silk, and wool) commonly used to manufacture clothing. Figure 1 shows the laser-cut fabric samples designed to fit in 90 mm Petri dishes. The growth media used in the study were malt extract agar (MEA), malt extract yeast agar (MYA), potato dextrose agar (PDA), and potato dextrose yeast agar (PDYA). The agar powder and 90 mm Sterilin™ sterile Petri dishes were purchased from Beacon Hill Mushrooms Ltd., UK.
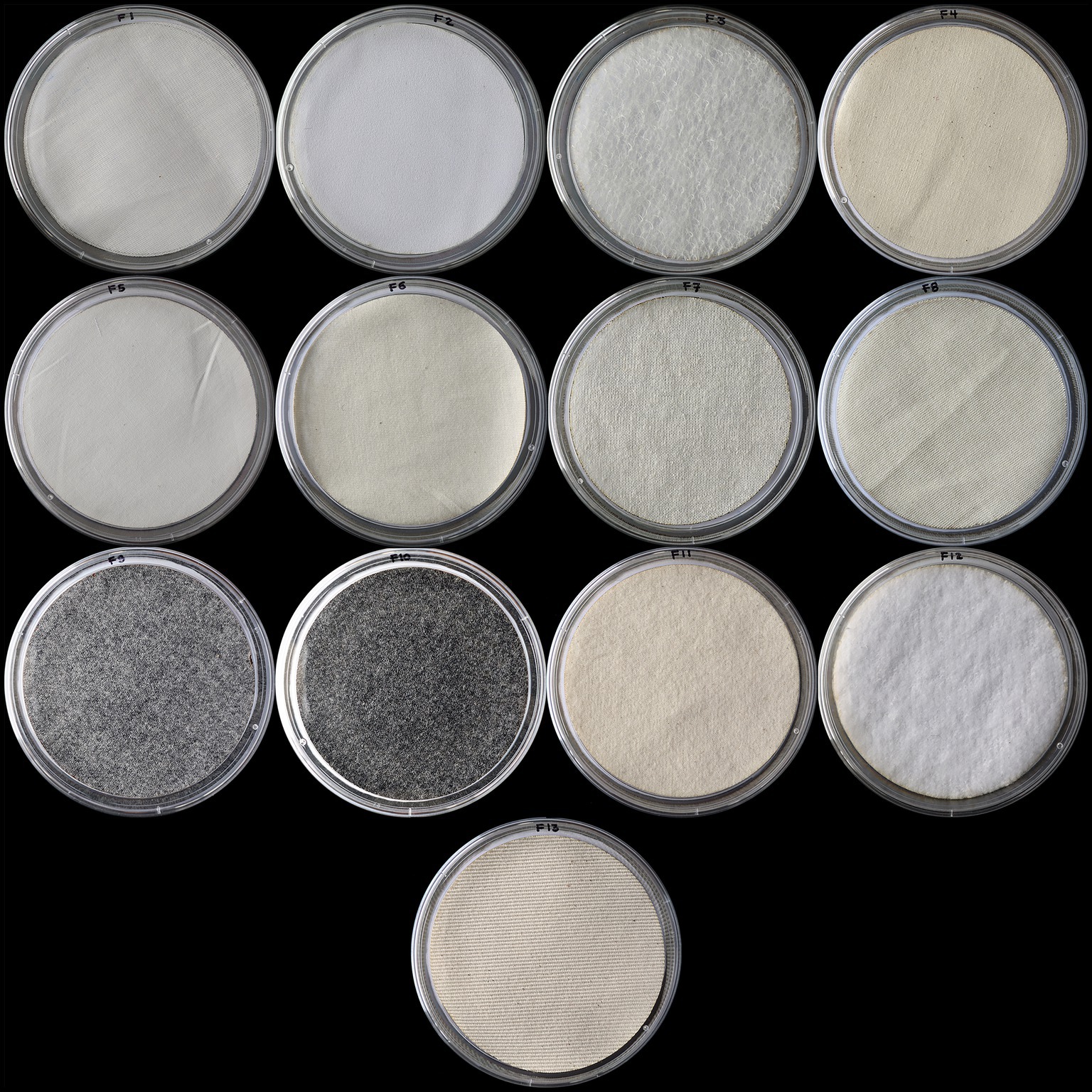
Figure 1. Fabric samples under examination, arranged from top left to bottom right with their corresponding labels: CX202 Cotton Gauze (F1), Polyester Chiffon White Printing (F2), Ice Wool Medium White (F3), Calico Light (F4), Poplin Cotton Delphina White Shrunk (F5), Merz Cotton Natural Jersey (F6), Jersey Silk Noil EF101 Natural Tubular (F7), Ecru Cotton Jersey Tubular (F8), 100% Wool Light Grey (F9), 100% Wool Dark Grey (F10), Raised Natural Cotton (F11), Jersey Cotton Pimenta Sweatshirt White (F12), and Denim 100% Natural Cotton Heavy (F13).
The agar solution was prepared by dissolving 40 g of MEA and MYA in 1,000 ml of distilled water and 50 g of PDA and PDYA in 1,000 ml of distilled water in 1-litre glass media bottles (Kimax® Kimble). Bottles were placed on a magnetic stirrer until the agar was fully dissolved and then sterilized. After sterilization, MEA, MYA, PDA, and PDYA were each poured into Petri dishes and allowed to solidify. The fabric samples were individually wrapped in aluminum foil and sterilized. After cooling to room temperature, they were carefully placed on the surface of each agar dish using sterile tweezers. The inoculum was cut from grain spawn using a sterile knife and placed at the approximate center of each fabric sample using sterile tweezers. Petri dishes were covered with lids and incubated upside-down in a Genlab incubator at 22°C to prevent condensation.
2.1 Mycelium growth
Photographic images were taken daily from Day 2 until the fabric samples were entirely covered with mycelium. The images were taken from a constant distance using a DSLR camera (Canon EOS 250D) and a Canon EFS 18–55 mm lens. The images were analyzed using ImageJ 1.53 k software (National Institutes of Health, Bethesda, Maryland, USA), and the daily growth area for mycelium growth was calculated to the nearest 0.00 mm2/day.
2.2 Mycelium morphological characteristics
Mycelium morphology was examined using photographic images taken on Day 22 and analyzed by visual inspection following the methods by Guadarrama-Mendoza et al. (2014) and Sobal et al. (2007). Morphological characteristics were assessed based on mycelium texture (cottony or floccose), density (low, regular, or high), color (white or white with yellowish areas), aerial hyphae (scarce, regular, or abundant), and mycelium growth (irregular or regular).
2.3 Mycelium dry biomass
Each fabric sample was carefully removed from Petri dishes on Day 22 using sterile tweezers and then dried in an Excalibur dehydrator (USA) at 50°C for 1 h to measure dry biomass. Samples were weighed before and after mycelium growth using an Accuweight digital laboratory scale, and the difference in weight was recorded in grams to the nearest 0.00 g.
2.4 Statistical
The data were analyzed, and graphs were generated using Microsoft Excel (v.16.9). A two-way ANOVA was used to evaluate the impact of growth media, MEA, MYA, PDA, and PDYA on the growth phases of mycelium development: lag, exponential, and stationary phases. A multivariate analysis of variance (MANOVA) was used to evaluate the combined effects of independent variables, mycelium density, texture, and aerial hyphae, on the dependent variables, growth and color. A one-way ANOVA and Tukey’s (HSD) post-hoc analysis (p < 0.01) were used to evaluate the effect of growth media MEA, MYA, PDA, and PDYA on dry biomass production.
3 Results
In the final study, 13 different fabric samples and four different growth media were used to produce fifty-two samples (n = 52) for examination. As shown in Figures 2–5, all samples exhibited mycelium growth; however, growth performance and mycelium morphological characteristics showed significant variation. Moreover, no microbial contamination was detected throughout the growth process. The observation aligns with previous studies that show the antimicrobial properties produced by the mycelium of Pleurotus ostreatus (Bruscato et al., 2019; Cilerdzic et al., 2015; Dundar et al., 2013).
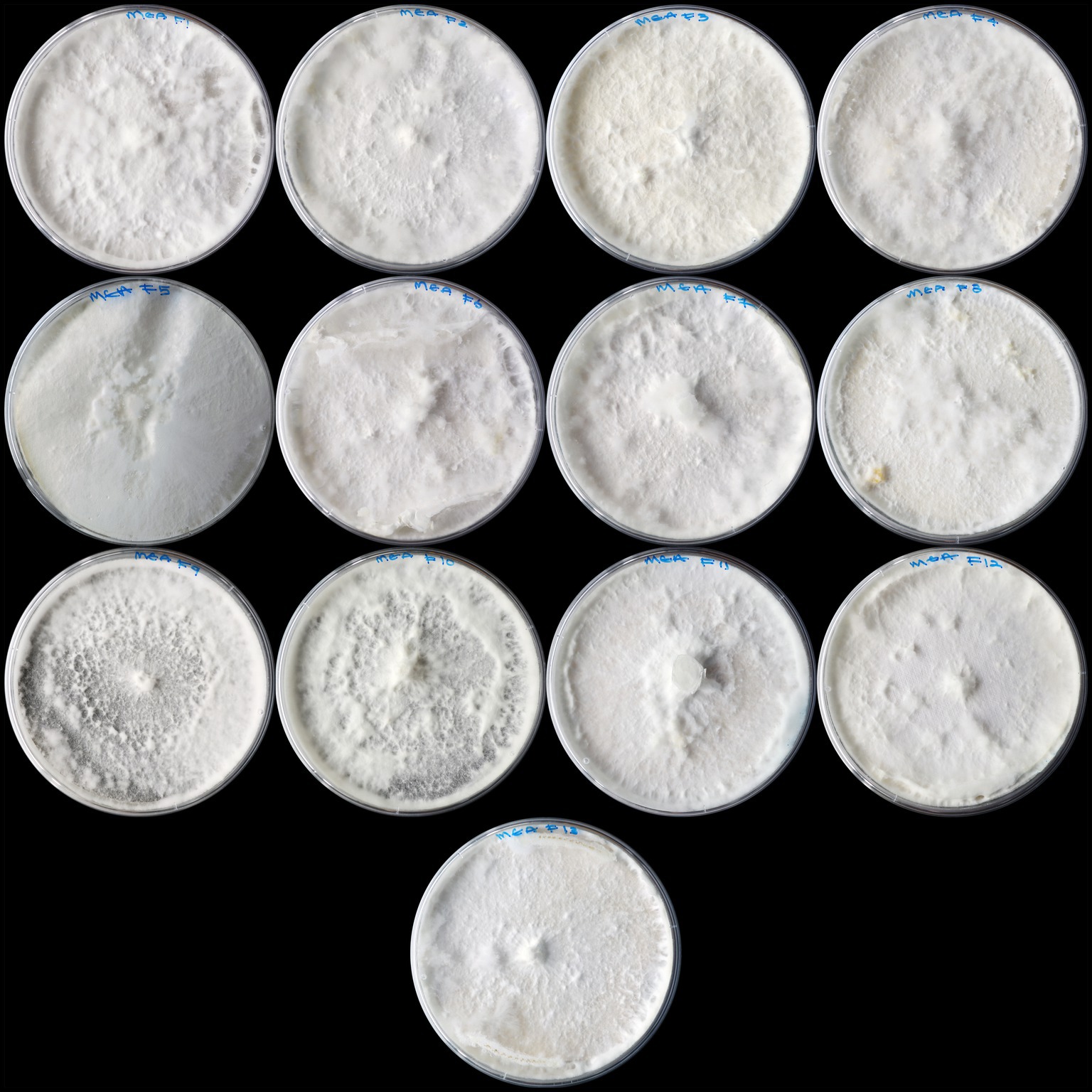
Figure 2. Shows the variation in the growth of Pleurotus ostreatus mycelium on malt extract agar (MEA) across different fabric substrates. The samples are arranged from top left to bottom right in the following order: F1-MEA, F2-MEA, F3-MEA, F4-MEA, F5-MEA, F6-MEA, F7-MEA, F8-MEA, F9-MEA, F10-MEA, F11-MEA, F12-MEA, and F13-MEA.
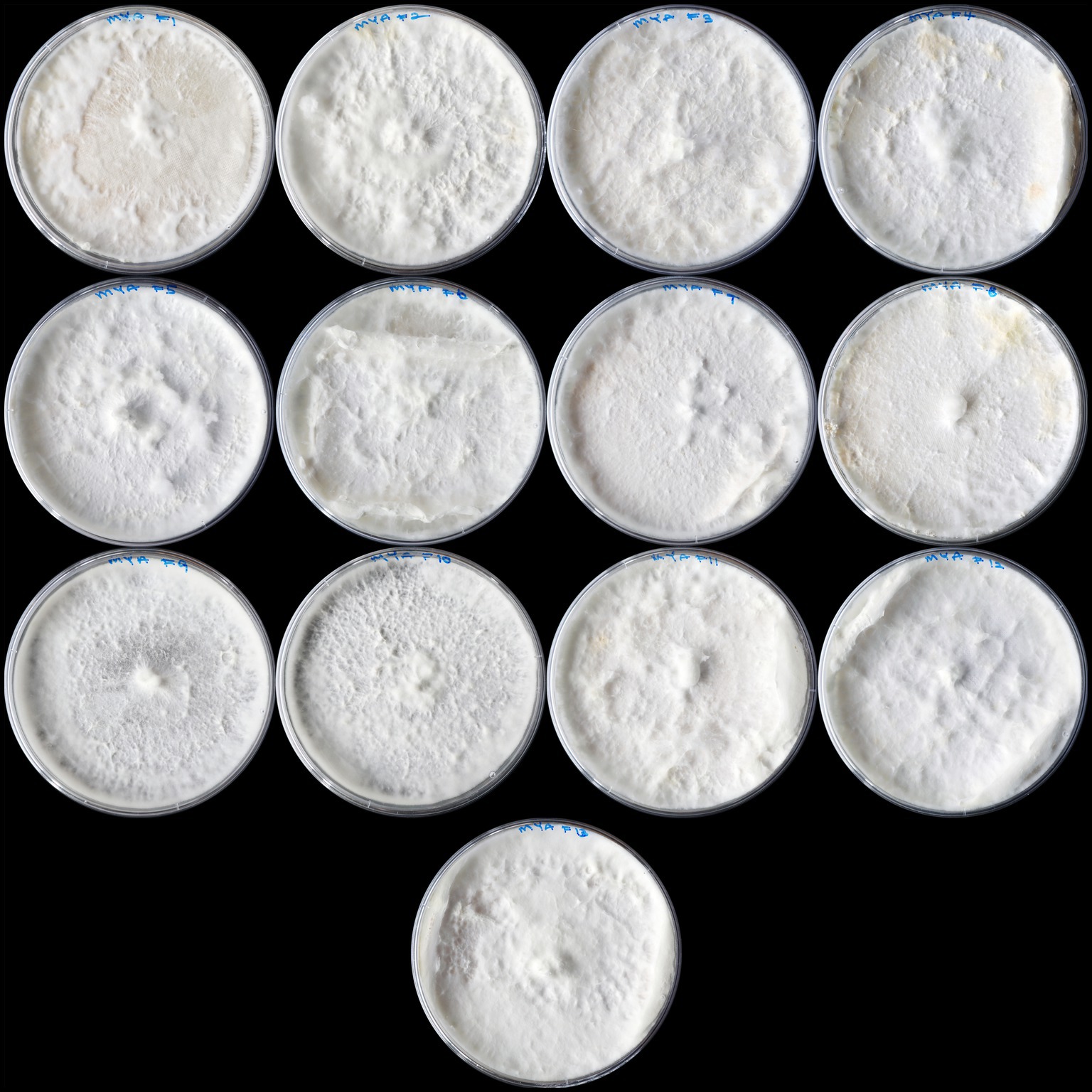
Figure 3. Shows the variation in the growth of Pleurotus ostreatus mycelium on malt yeast agar (MYA) across different fabric susstrates. The samples are arranged from top left to bottom right in the following order: F1-MYA, F2-MYA, F3-MYA, F4-MYA, F5-MYA, F6-MYA, F7-MYA, F8-MYA F9-MYA, F10-MYA, F11-MYA, F12-MYA, and F13-MYA.
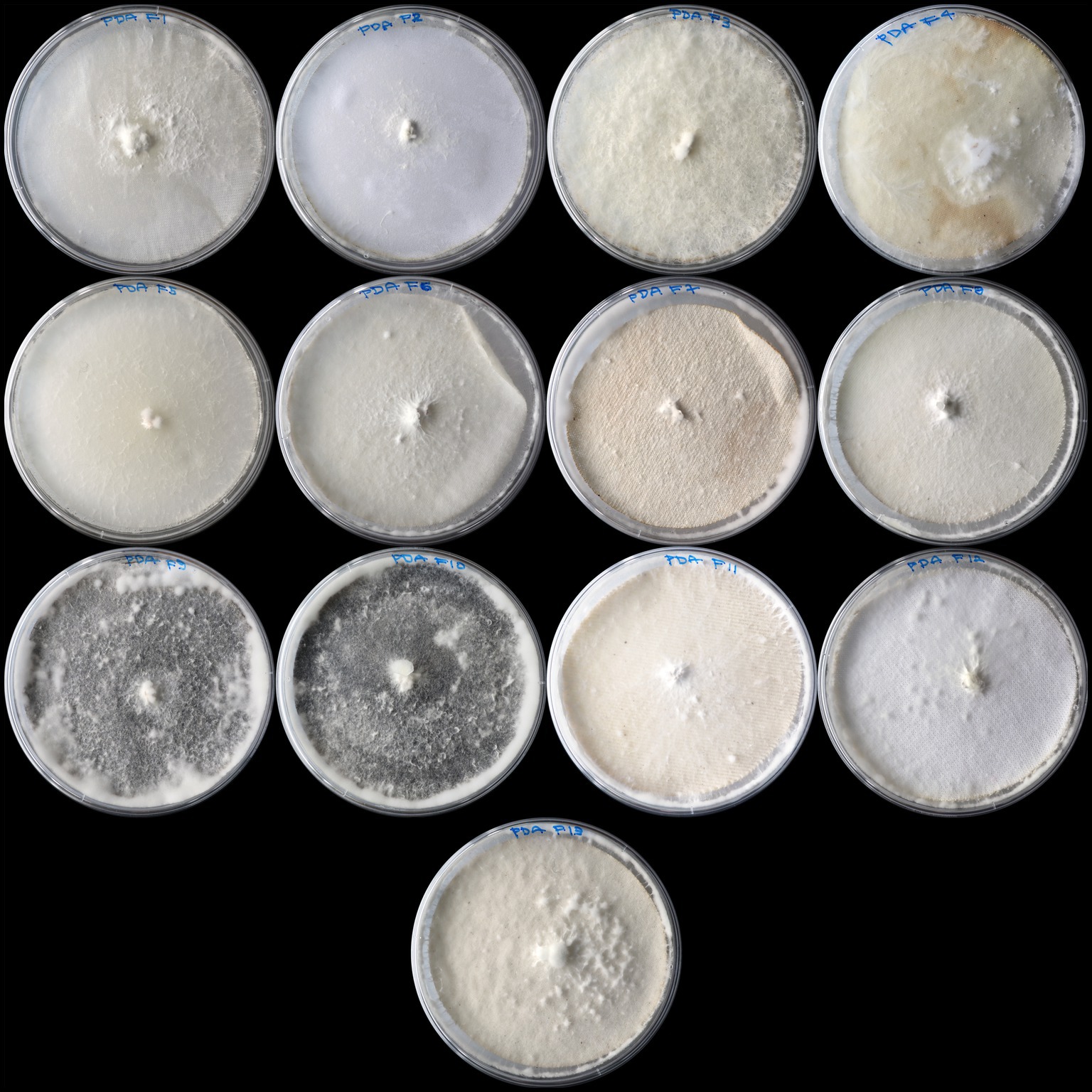
Figure 4. Shows the variation in the growth of Pleurotus ostreatus mycelium on potato dextrose agar (PDA) across different fabric susbstrates. The samples are arranged from top left to bottom right in the following order: F1-PDA, F2-PDA, F3-PDA, F4-PDA, F5-PDA, F6-PDA, F7-PDA, F8-PDA, F9-PDA, F10-PDA, F11-PDA, F12-PDA, and F13-PDA.
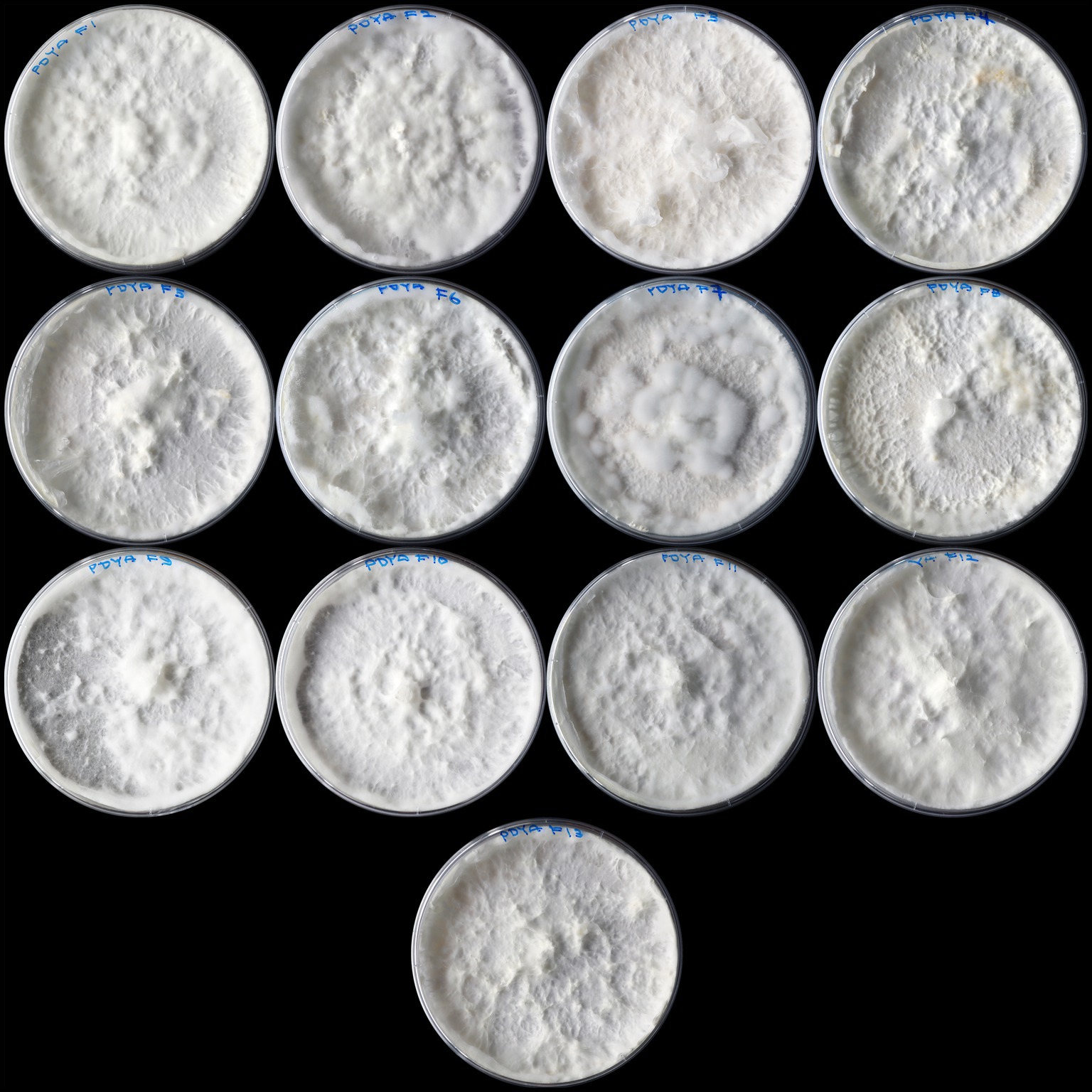
Figure 5. Shows the variation in the growth of Pleurotus ostreatus mycelium on potato dextrose yeast agar (PDYA) across different fabric substrates. The samples are arranged from top left to bottom right in the following order: F1-PDYA, F2-PDYA, F3-PDYA, F4-PDYA, F5-PDYA, F6-PDYA, F7-PDYA, F8-PDYA, F9-PDYA, F10-PDYA, F11-PDYA, F12-PDYA, and F13-PDYA.
3.1 Mycelium growth
Table 1 shows samples ranked by their overall growth performance (based on mean growth rate and maximum growth area), peak growth, and the corresponding day it was achieved. The best-performing sample for mycelium growth was F6-MEA, which had an average growth rate of 818.24 mm2/day and a maximum growth area of 5,800.53 mm2. This was followed closely by F4-MYA (813.89 mm2/day, 5,800.53 mm2), and F3-MYA (813.88 mm2/day, 5,800.53 mm2). These samples exhibited superior growth performance compared to F1-PDA (354.81 mm2/day, 5,356.50 mm2), F7-PDA (318.23 mm2/day, 4,813.51 mm2), and F3-PDA (249.33 mm2/day, 4,020.37 mm2), which were the worst-performing samples for mycelium growth (Figure 6). Peak growth occurred between Days 4 and 9, with the highest proportion of samples (44.23%, n = 23) achieving peak growth on Day 8. The most significant increase in mycelium growth occurred in F13-MYA at 2,095.37 mm2 on Day 8. This was followed by F3-PDYA (1,847.02 mm2) and F3-MEA (1,813.76 mm2). Figure 7 shows the worst-performing samples for peak growth, which were F3-PDA, F7-PDA, and F5-PDA at 123.03 mm2, 265.71 mm2, and 302.13 mm2, respectively.
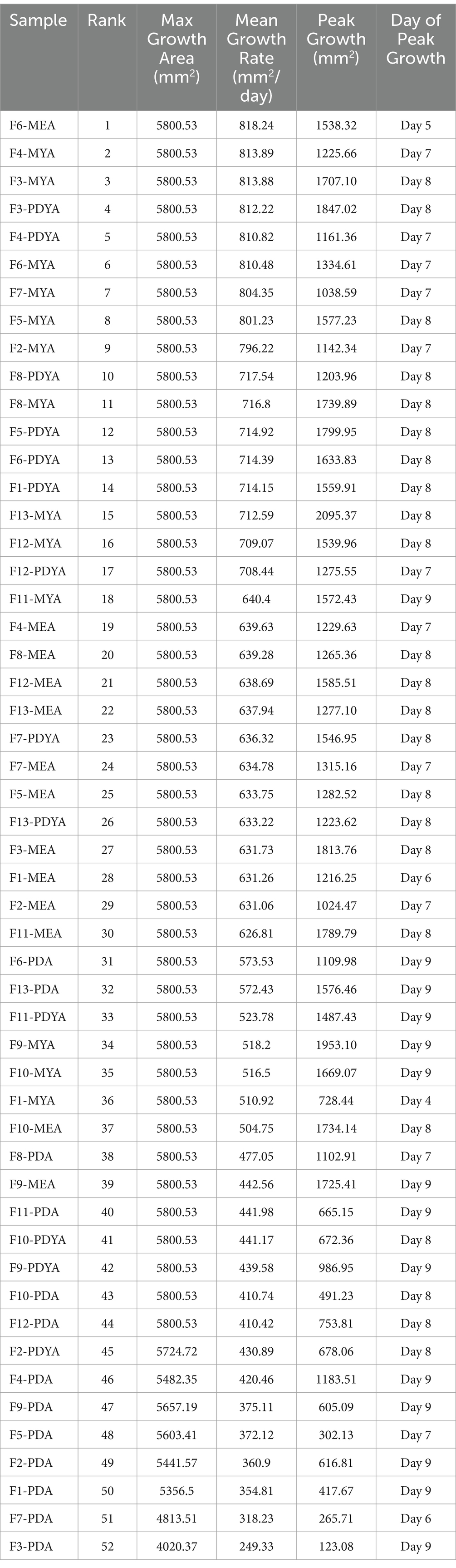
Table 1. Samples ranked by mycelium growth performance (based on their mean growth rate and maximum growth area), peak growth, and the corresponding day it was achieved.
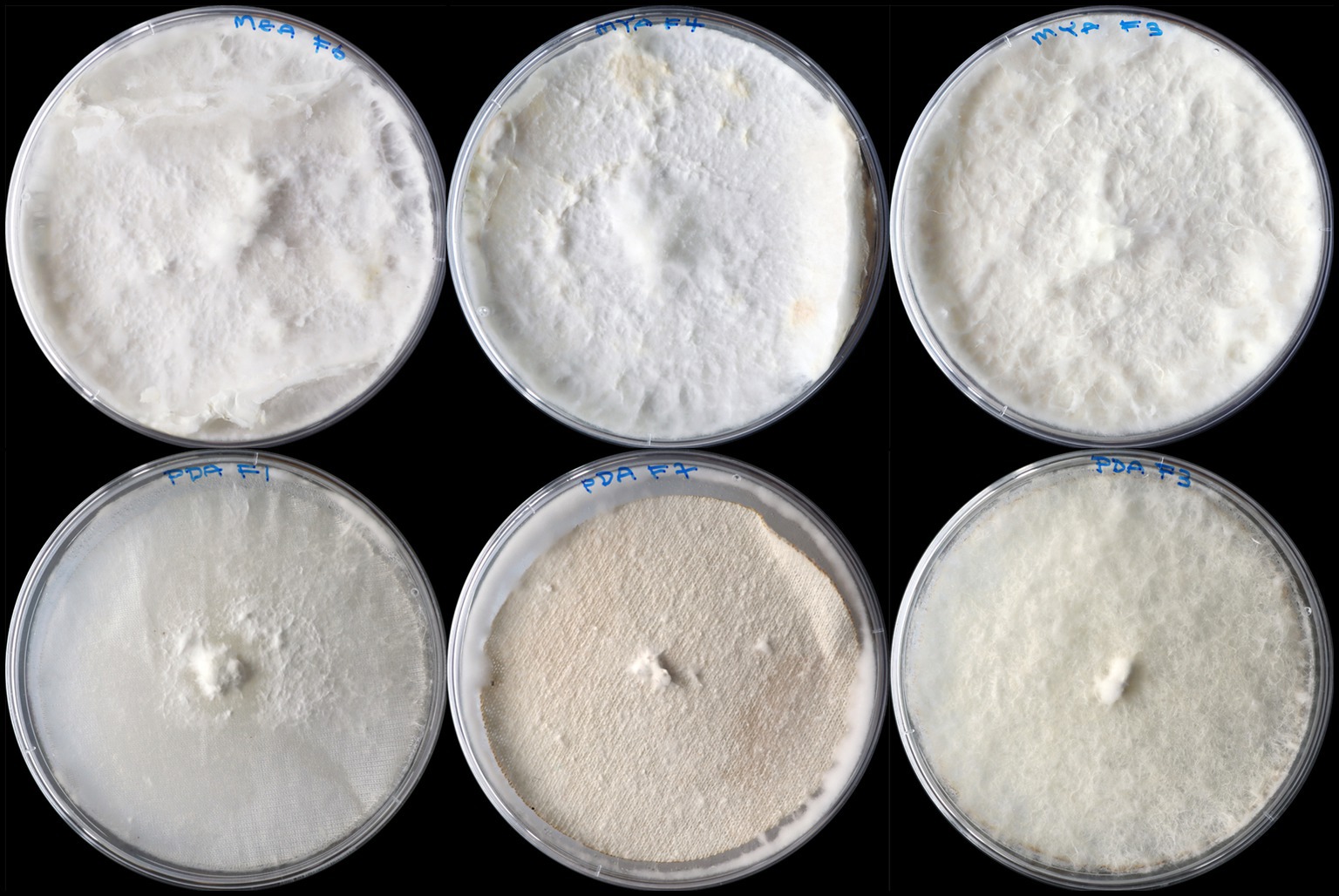
Figure 6. Pleurotus ostreatus mycelium growth after 22 days of incubation on different fabric substrates and growth media. Samples are arranged from top left to bottom right as follows: F6-MEA, F4-MYA, F3-MYA, F1-PDA, F7-PDA, and F3-PDA. The figure illustrates variations in mycelium colonization, highlighting differences in growth patterns influenced by substrate compostion and nutrient availability in the respective agar media.
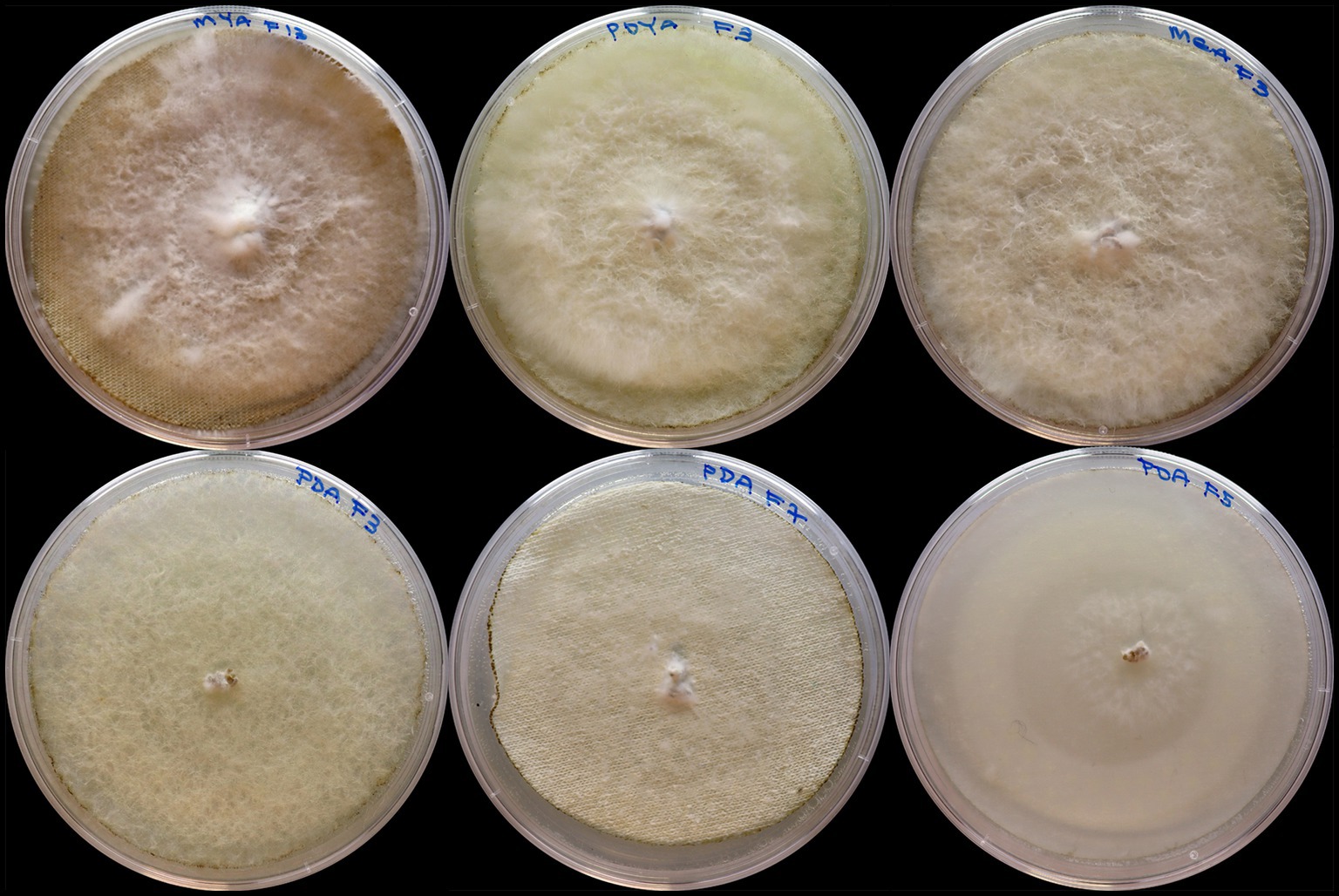
Figure 7. Peak growth of Pleurotus ostreatus after 8 days of incubation on different fabric substrates and growth media. Samples are arranged from top left to bottom right as follows: F13-MYA, F3-PDYA, F3-MEA, F3-PDA, F7-PDA, and F5-PDA.
These results indicate that the nutrient-rich environment of MEA, MYA, and PDYA was more favorable than PDA for supporting mycelium growth on the fabric samples. The results align with studies by Mahadevan and Shanmugasundaram (2018) and Muthu and Shanmugasundaram (2015), showing that malt extract offers complex carbohydrates and proteins that are quickly metabolized by Pleurotus ostreatus. Moreover, yeast extract in MYA and PDYA provide a rich source of vitamins and amino acids, significantly enhancing mycelium development and resulting in a greater growth rate and higher biomass production than PDA (Paswal et al., 2021). Figure 4 shows that PDA, while supportive of mycelium growth, lacked the nutritional complexity found in MEA, MYA, and PDYA. The findings suggest that PDA is not a practical option for upcycling applications and is ineffective in situations that demand rapid mycelium growth.
Figure 8 shows daily growth rates of F6-MEA (#1), F1-MEA (#28), F1-MYA (#36), and F1-PDA (#50). The samples were selected based on their growth performance ranking. F1-MEA had a sharp increase in growth on Day 4 at 693.23 mm2/day and peaked on Day 6 at 1,216.25 mm2/day. However, after Day 9, the growth rate declined until the entire fabric substrate was covered entirely with mycelium on Day 11. F6-MEA exhibited a similar trend, with a quick, steady increase in growth, reaching a peak of 1,538.32 mm2/day on Day 5, followed by a decrease in growth until it was fully covered by mycelium on Day 9. F1-MYA displayed a more gradual increase in growth, reaching its peak of 668.34 mm2/day on Day 8 and maintaining a steady rate until it was fully covered by mycelium growth on Day 13. F1-PDA exhibited the slowest growth rate, peaking at 1,108.97 mm2/day on Day 14, and had consistently low daily growth rates throughout its observation period of 22 days. The sample exhibited irregular floccose growth and did not fully colonize the fabric sample. The results indicate that the combination of cotton fabrics and the nutritional composition of MEA had an exemplary impact on the daily growth rate.
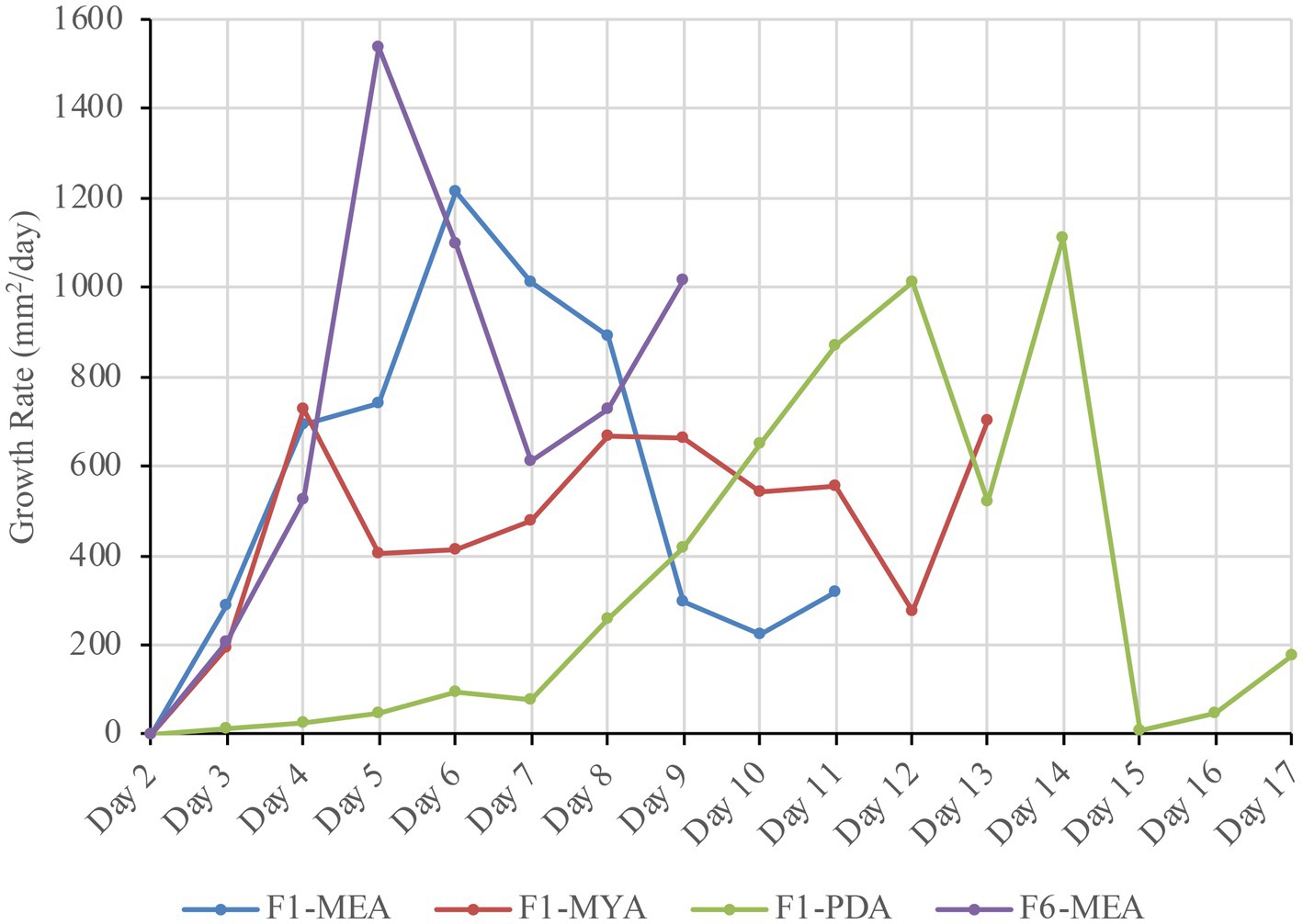
Figure 8. Comparison of the daily growth rate for F1-MEA, F6-MEA, F1-MYA, and F1-PDA, highlighting variations in colonization speed across different growth media.
As previously mentioned, the main aim of this study was to investigate whether end-of-life textile substrates could be upcycled from the fashion industry for mycelium-based materials. When mycelium is inoculated in a suitable growth media, growth progresses through three distinct growth phases: lag, exponential, and stationary (Jones et al., 2017). It is essential during growth to ensure optimal environmental conditions and abundant nutrients are available to maximize growth rate and yield. A two-way ANOVA was performed to evaluate the impact of growth media on the distinct phases of mycelium development: lag, exponential, and stationary. The analysis showed a significant main effect of growth media on the lag phase (F = 10.74, p < 0.001) and the exponential phase (F = 6.13, p < 0.01). However, no significant effect was observed for the stationary phase (F = 1.24, p = 0.30). These findings suggest that while the lag and exponential phases showed considerable variation across the four different growth media, the stationary phase remained relatively consistent among the samples. For example, samples grown on MYA and PDYA exhibited the shortest lag phases, averaging 0.2 days, which made them the best growth media for rapid mycelium growth.
Similarly, MEA had relatively short lag phases, averaging 0.5 days, which made it the second-best growth medium for quick mycelium production. The results indicate that MEA and PDYA are the most effective growth media for balanced growth across the growth phases. In contrast, PDA exhibited considerable extended lag and exponential phases, averaging 2.8 and 6.4 days, respectively (Figure 9). Although the results indicate that PDA was the least effective for mycelium growth performance, the growth media could be used for applications requiring prolonged active growth.
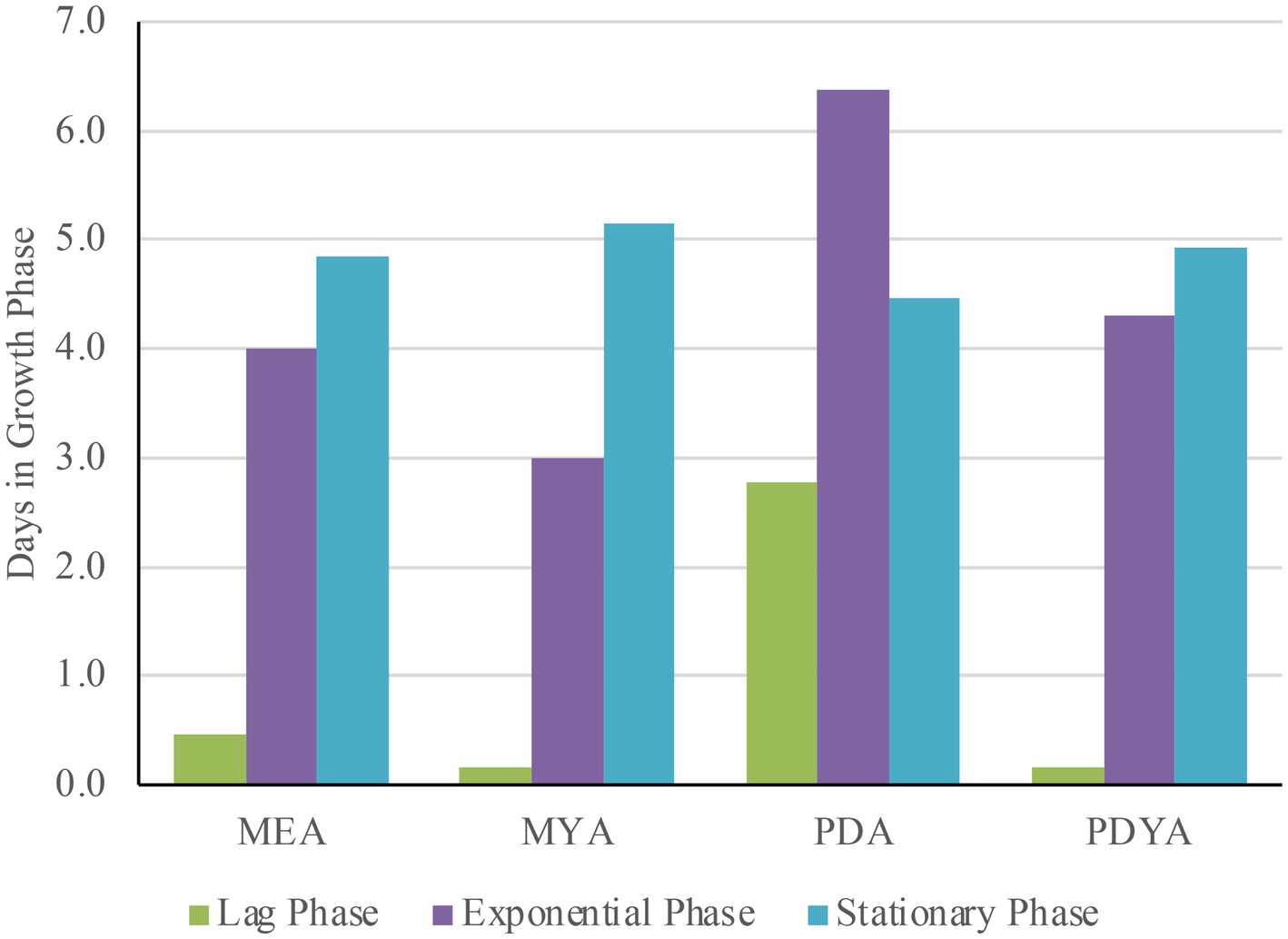
Figure 9. Average duration of the growth phases for Pleurotus ostreatus across growth media (MEA, MYA, PDA, and PDYA) shows variations in development progession influenced by nutrient composition.
Few studies have explored the use of mycelium for upcycling textile waste. However, several have evaluated the impact of different growth media on mycelium growth performance (Mahadevan and Shanmugasundaram, 2018; Muthu and Shanmugasundaram, 2015; Paswal et al., 2021). The significant influence of growth media on the lag and exponential phases of mycelium growth highlights the crucial role of nutrient composition. Growth media enriched with yeast extract (MYA and PDYA) facilitates a shorter lag phase, which supports faster mycelium growth. This finding is consistent with previous research, which shows that increased nitrogen and carbon availability can accelerate fungal metabolism and enzymatic activity, leading to quicker colonization (Jonathan and Fasidi, 2001; Naraian et al., 2009).
In contrast, the stationary phase showed no significant variation across different growth media, indicating that external nutrient availability has less influence once the mycelium reaches its maximum growth capacity. This stability in the stationary phase aligns with literature indicating that mycelium growth eventually plateaus due to substrate depletion and self-regulatory mechanisms (Sánchez, 2010). Overall, the results indicate that MEA, MYA, and PDYA are the optimal growth media for enhancing the colonization of Pleurotus ostreatus, which is a critical factor in optimizing mycelium-based upcycling of textile waste (Royse et al., 2017).
3.2 Mycelium morphological characteristics
Tables 2, 3 show mycelium morphological characteristics and frequency data for mycelium texture, density, color, aerial hyphae, and colony growth for the samples. The results show that 75%, n = 39 of samples had a fluffy, fibrous, cottony growth pattern, and 25%, n = 13 of the fungal colony was floccose, with loose, woolly tufts of hyphae. Mycelium density was relatively distributed, with 36.54% (n = 19) producing high and regular density each and 26.92% (n = 14) samples producing low density. The color of mycelium produced was predominantly white (84.62%, n = 44). However, as shown in Figure 10, samples F2-MYA, F4-MYA, F8-MYA, F11-MYA, F4-MEA, F8-MEA, F4-PDYA, and F8-PDYA produced mostly white-colored mycelium with patches of yellow areas after 12 days of incubation. This observation aligns with studies by Feldman et al. (2019), Margraf (1984), Tello et al. (2016), and Zhu et al. (2020), which showed that the mycelium of Pleurotus ostreatus produces yellow pigment. The findings highlight the need for further research to explore using these natural pigments in the fashion industry for dyeing, printing, and finishing applications.
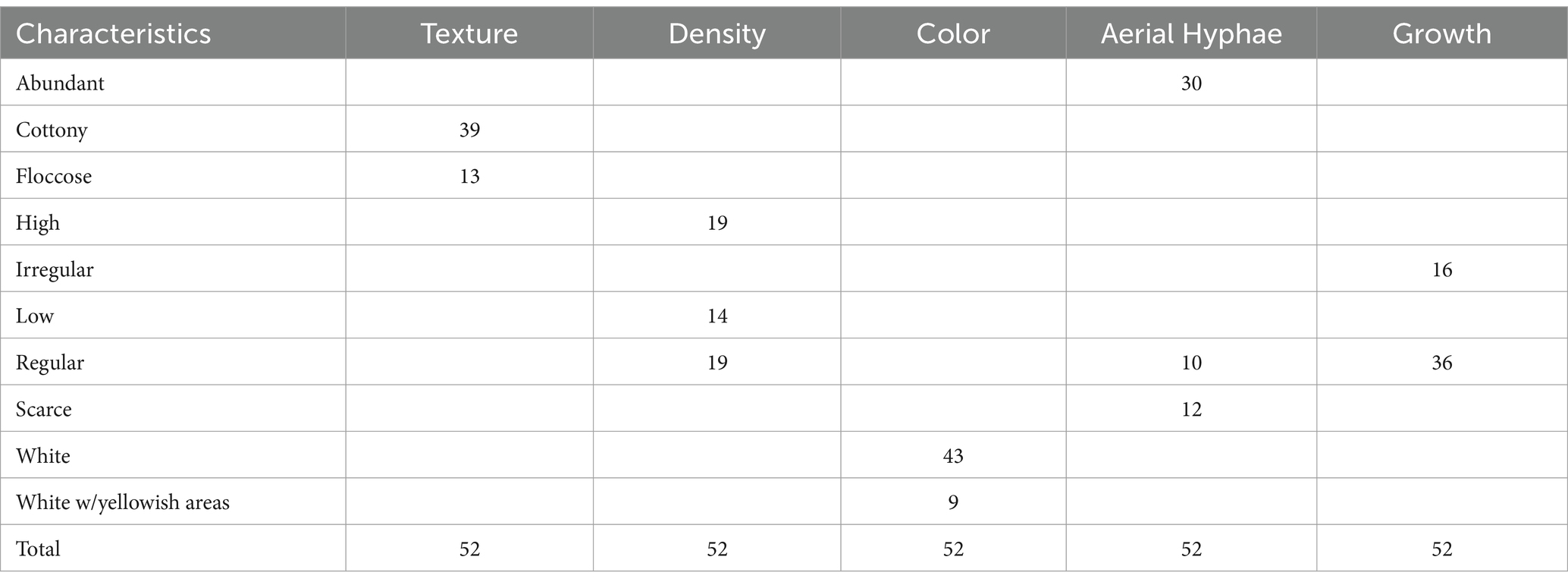
Table 3. Frequency data of morphological characteristics for fabric samples on MEA, MYA, PDA, and PDYA.
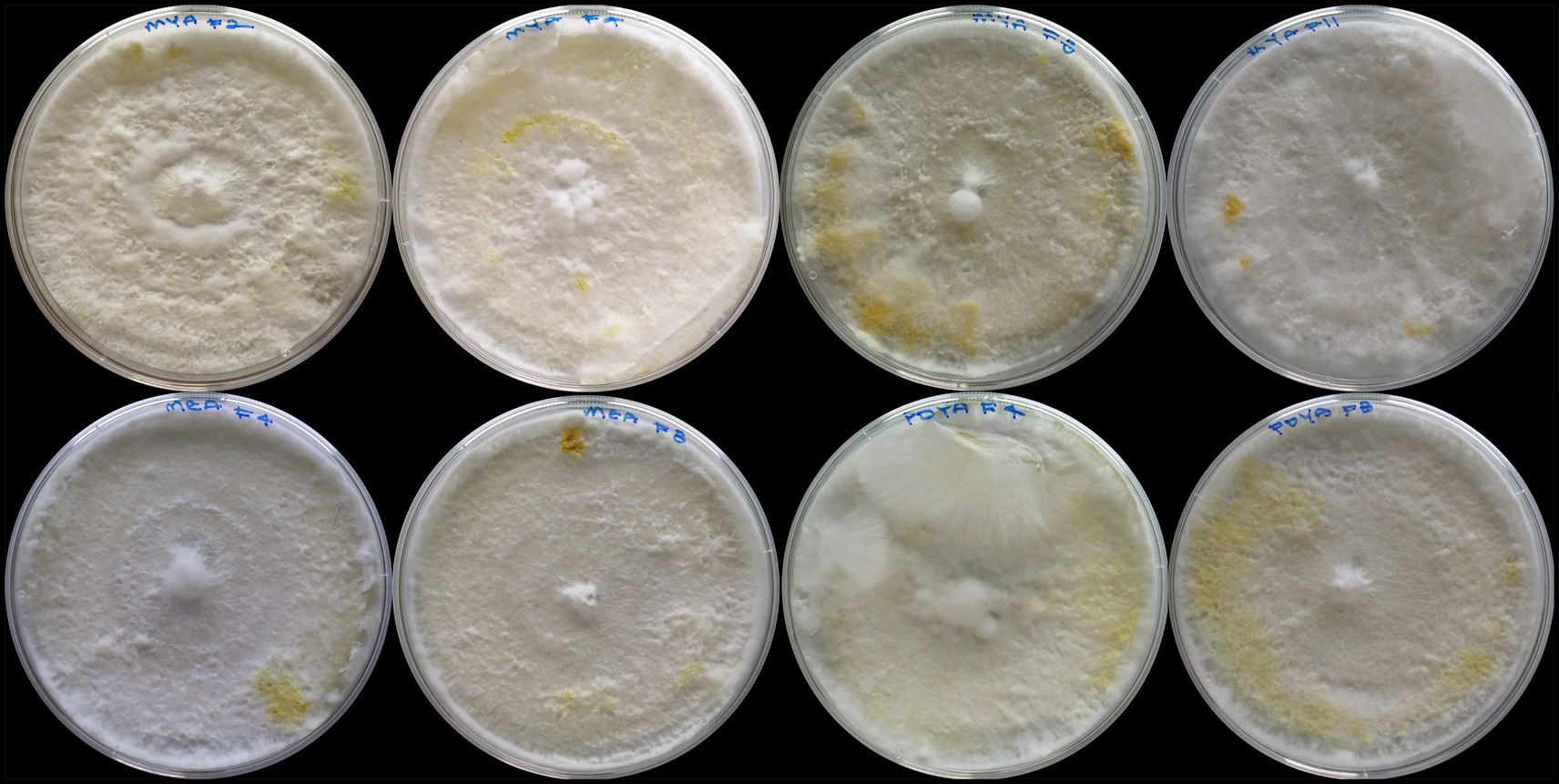
Figure 10. Yellow pigmentation of Pleurotus ostreatus was observed after 12 days of incubation on various fabric substrates and growth media Samples are arranged from top left to bottom right as follows: F2-MYA, F4-MYA, F8-MYA, F11-MYA, F4-MEA, F8-MEA, F4-PDYA, and F8-PDYA. The figure highlights pigmentation differences influenced by substrate composition and nutrient availability.
The multivariate analysis of variance (MANOVA) revealed that mycelium density had no significant influence on growth or color [Wilks’ Λ = 0.950, F (4, 44) = 0.58, p = 0.679], indicating it is not a vital determinant of these characteristics when considered alongside other variables. However, mycelium texture had a significant effect on both growth and color [Wilks’ Λ = 0.799, F (4, 44) = 2.77, p = 0.039], suggesting that texture (cottony or floccose) is a vital determinant of mycelium development. Aerial hyphae did not show a statistically significant effect [Wilks’ Λ = 0.750, F (8, 88) = 1.70, p = 0.108]. However, the p-value approached significance, suggesting that further research could reveal its impact under different fabric substrates and nutrient environments. In summary, mycelium texture emerged as a critical factor in determining growth and color, with density and aerial hyphae having no significance. These findings emphasize the importance of texture in optimizing mycelium growth, particularly for upcycling applications that require consistent mycelium growth and appearance.
3.3 Mycelium dry biomass
The one-way ANOVA analysis to assess the effect of MEA, MYA, PDA, and PDYA on dry mycelium biomass revealed a significant effect of the four different growth media on mycelium dry biomass, F (3, 16) = 60.51, p < 0.001. The post hoc Tukey HSD tests indicated that PDYA (M = 0.32 g, SD = 0.07) had significantly higher biomass compared to MEA (M = 0.10 g, SD = 0.05), MYA (M = 0.11 g, SD = 0.07), and PDA (M = 0.04 g, SD = 0.03), p < 0.001, respectively, (Figure 11). Additionally, there was a significant difference between PDA and PDYA (p < 0.05). These results indicate that PDYA significantly outperformed the other growth media for optimal mycelium growth (p < 0.01) and was best for mycelium biomass production. PDA consistently produced the lowest biomass yield compared to other media types (p < 0.01). Moreover, yeast extract provides a source of vitamins and amino acids that significantly improve and enhance mycelium biomass yield.
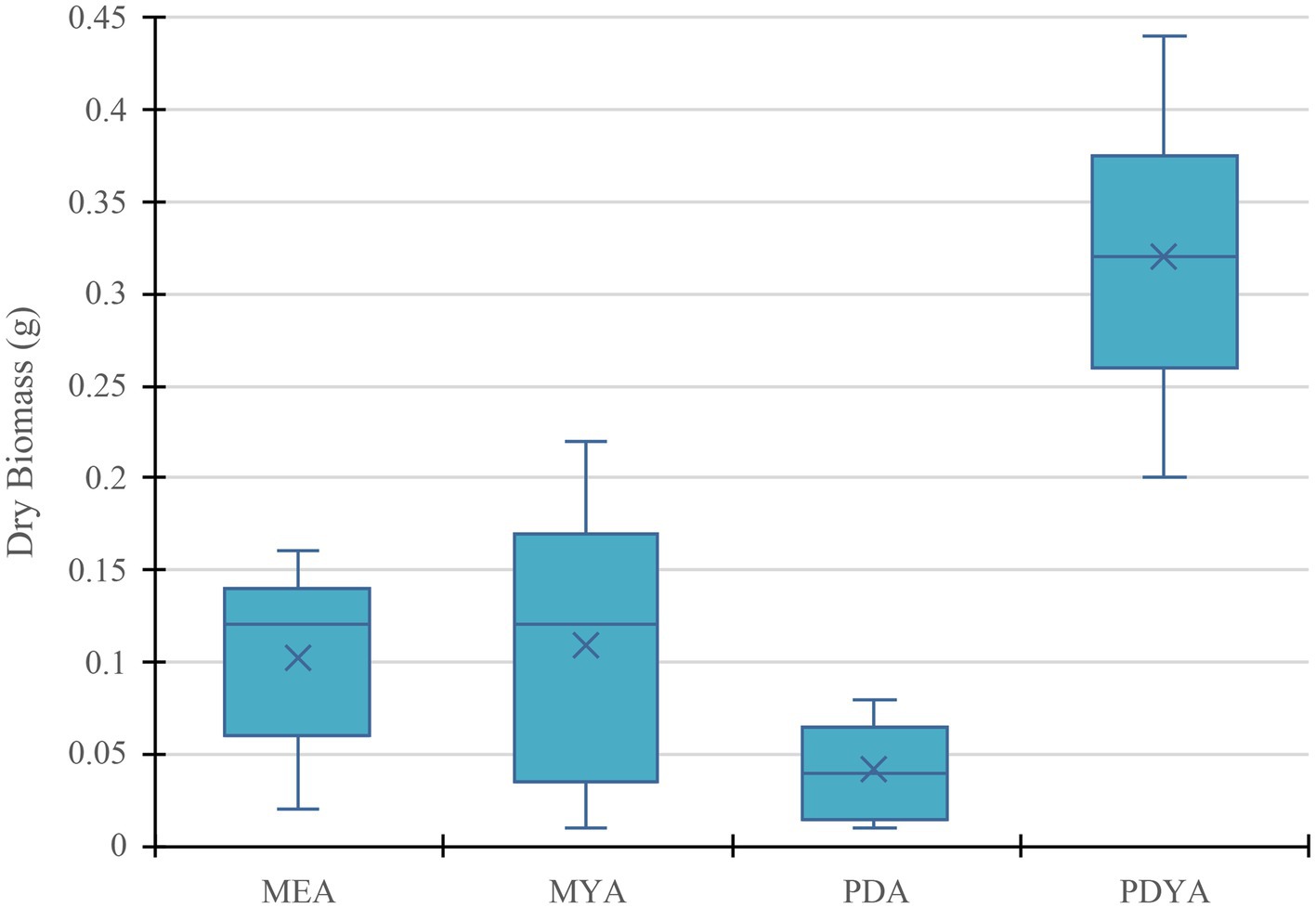
Figure 11. Dry mycelium biomass production of Pleurotus ostreatus across different growth media (MEA, MYA, PDA, and PDYA), illustrating variations in biomass yield influenced by nutrient composition and substrate compatibility.
4 Discussion
The findings of this study underscore the environmental challenges the fashion industry poses, particularly its contribution to global carbon emissions, water consumption, and textile waste generation. Fast fashion’s rapid turnover of inexpensive garments has significantly impacted consumption patterns, resulting in unsustainable disposal practices (Bick et al., 2018; Niinimäki et al., 2020). Addressing these issues requires innovative solutions, such as mycelium-based materials, which offer promising avenues for sustainable development and waste reduction in the fashion sector.
This study assessed the potential of upcycling end-of-life textile substrates using Pleurotus ostreatus cultivated on various fabric types and growth media. The results demonstrated that all fabric substrates supported mycelium growth, with significant variation in growth rates, morphological characteristics, and biomass yield. MEA, MYA, and PDYA emerged as the most effective growth media, providing the most favorable nutrient environments that supported rapid and robust mycelium development. PDYA exhibited superior performance in the production of mycelium biomass, aligning with previous research indicating that yeast extract enhances mycelium growth (Paswal et al., 2021).
The statistical analysis revealed a significant impact of growth media on the lag and exponential phases of mycelium growth but not on the stationary phase, suggesting that optimizing early growth conditions is critical for efficient mycelium cultivation. Additionally, the significant variation in mycelium texture, growth pattern, and color indicates that the nutritional composition of the fabric substrates and growth media can influence these morphological traits, highlighting their importance for upcycling applications.
These findings contribute to the growing body of research on mycelium-based materials by demonstrating the feasibility of using mycelium for upcycling textile waste. However, further studies are needed to explore material development, the scalability of this method, and the potential for integrating mycelium-based technologies at the end-of-life stage of fashion products. This approach could transform textiles into new biomaterials rather than allowing them to contribute to waste. While the findings demonstrate that mycelium can be used for textile waste upcycling, scaling this process to an industrial level presents several challenges. Large-scale production requires cost-effective nutrient sources, optimized cultivation conditions, and significant investments in infrastructure to ensure consistent quality and yield. Regulatory considerations must be addressed before commercial adoption, including material certification and safety compliance. Recognizing these practical challenges is essential for translating laboratory results into viable solutions for industry use. Developing mycelium-based technologies could help the fashion industry transition towards more sustainable practices, thereby reducing its environmental impact by repurposing textile waste and minimizing resource consumption. Future research should also explore the natural pigmentation of mycelium as an eco-friendly alternative to dyeing, printing, and finishing, adding further value to the sustainable transformation of the fashion industry.
5 Conclusion
In conclusion, this study provides a foundation for using mycelium-based technologies as a sustainable solution for textile waste management. The promising results indicate that with further development, mycelium-based materials could significantly help mitigate the environmental impacts associated with fast fashion, contributing to a more sustainable and circular economy in the fashion industry.
Data availability statement
The datasets presented in this study can be found in online repositories. The names of the repository/repositories and accession number(s) can be found at: https://data.mendeley.com/preview/49vgv5s5t7?a=05527345-151e-4f49-bfe2-e6a12e7750d0MendeleyData.
Author contributions
IF: Conceptualization, Data curation, Formal analysis, Funding acquisition, Investigation, Methodology, Project administration, Resources, Software, Supervision, Validation, Visualization, Writing – original draft, Writing – review & editing.
Funding
The author(s) declare that financial support was received for the research and/or publication of this article. The author wishes to acknowledge the financial support from Research England and the Leeds School of Arts, Leeds Beckett University.
Acknowledgments
The author wishes to acknowledge the technical support provided by Lynda Howarth and Patricia Hewitt for their invaluable technical expertise on fabrics and their applications for clothing.
Conflict of interest
The author declares that the research was conducted in the absence of any commercial or financial relationships that could be construed as a potential conflict of interest.
Generative AI statement
The author(s) declare that Gen AI was used in the creation of this manuscript. It was used to correct grammar and to verify the Excel data.
Publisher’s note
All claims expressed in this article are solely those of the authors and do not necessarily represent those of their affiliated organizations, or those of the publisher, the editors and the reviewers. Any product that may be evaluated in this article, or claim that may be made by its manufacturer, is not guaranteed or endorsed by the publisher.
References
Al-Qahtani, S., Koç, M., and Isaifan, R. J. (2023). Mycelium-based thermal insulation for domestic cooling footprint reduction: a review. Sustainability 15:3217. doi: 10.3390/su151713217
Beall, A. (2020). Why clothes are so hard to recycle - BBC future. Available at: https://www.bbc.com/future/article/20200710-why-clothes-are-so-hard-to-recycle (Accessed September 26, 2024).
Bick, R., Halsey, E., and Ekenga, C. C. (2018). The global environmental injustice of fast fashion. Environ. Health 17:92. doi: 10.1186/s12940-018-0433-7
Bruscato, C., Malvessi, E., Brandalise, R. N., and Camassola, M. (2019). High performance of macrofungi in the production of mycelium-based biofoams using sawdust — sustainable technology for waste reduction. J. Clean. Prod. 234, 225–232. doi: 10.1016/j.jclepro.2019.06.150
Cilerdzic, J., Stajic, M., Vukojevic, J., Milovanovic, I., and Muzgonja, N. (2015). Antioxidant and antifungal potential of Pleurotus ostreatus and agrocybe cylindracea basidiocarps and mycelia. Curr. Pharm. Biotechnol. 16, 179–186. doi: 10.2174/1389201015666141202152023
Dundar, A., Okumus, V., Ozdemir, S., and Yildiz, A. (2013). Antioxidant properties of cultured mycelia from four pleurotus species produced in submerged medium. Int. J. Food Prop. 16, 1105–1116. doi: 10.1080/10942912.2011.576793
Feldman, D., Amedi, N., Carmeli, S., Yarden, O., and Hadar, Y. (2019). Manipulating the expression of small-secreted protein 1 (Ssp1) alters patterns of development and metabolism in the white-rot fungus Pleurotus ostreatus. Appl. Environ. Microbiol. 85, e00761–e00719. doi: 10.1128/AEM.00761-19
Grishanov, S. (2011). Structure and properties of textile materials. Handb. Textile Indust. Dyeing 1, 28–63. doi: 10.1533/9780857093974.1.28
Guadarrama-Mendoza, P. C., Valencia del Toro, G., Ramírez-Carrillo, R., Robles-Martínez, F., Yáñez-Fernández, J., Garín-Aguilar, M. E., et al. (2014). Morphology and mycelial growth rate of Pleurotus spp. strains from the Mexican mixtec region. Braz. J. Microbiol. 45:861–872. doi: 10.1590/S1517-83822014000300016
Helberg, J., Klöcker, M., Sabantina, L., Storck, J. L., Böttjer, R., Brockhagen, B., et al. (2019). Growth of Pleurotus ostreatus on different textile materials for vertical farming. Materials 12:2270. doi: 10.3390/ma12142270
Igini, M. (2023). 10 concerning fast fashion waste statistics. Earth.Org. Available online at: https://earth.org/statistics-about-fast-fashion-waste/ (Accessed September 26, 2024).
Innovation Forum. (2015). Sustainable and ethical cotton sourcing management briefing | PDF. Available online at: https://www.slideshare.net/slideshow/if-cotton-report-final/45177401 (Accessed September 26, 2024).
Jonathan, S. G., and Fasidi, I. O. (2001). Effect of carbon, nitrogen, and mineral sources on growth of Psathyerella atroumbonata (Pegler), a Nigerian edible mushroom. Available online at: www.elsevier.com/locate/foodchem (Accessed September 26, 2024).
Jones, M., Huynh, T., Dekiwadia, C., Daver, F., and John, S. (2017). Mycelium composites: a review of engineering characteristics and growth kinetics. J. Bionanosci. 11, 241–257. doi: 10.1166/jbns.2017.1440
Mahadevan, K., and Shanmugasundaram, K. (2018). Comparative effect of different culture media on mycelial growth performance of Pleurotus sapidus. J. Pharm. Phytochem. 7, 874–878.
Margraf, W. (1984). “Orange/yellow pigments in the basidiomycete Pleurotus ostreatus (Jacq. ex. Fr.) Kummer” in Blue light effects in biological systems. ed. H. Senger (Berlin Heidelberg: Springer), 55–59.
Muthu, N., and Shanmugasundaram, K. (2015). Effect of five different culture media on mycelial growth of Agrocybe Aegerita. Int. J. Pharm. Sci. Res. 6:5193. doi: 10.13040/IJPSR.0975-8232.6(12).5193-97
Naraian, R., Sahu, R. K., Kumar, S., Garg, S. K., Singh, C. S., and Kanaujia, R. S. (2009). Influence of different nitrogen rich supplements during cultivation of Pleurotus florida on corn cob substrate. Environmentalist 29, 1–7. doi: 10.1007/s10669-008-9174-4
Niinimäki, K., Peters, G., Dahlbo, H., Perry, P., Rissanen, T., and Gwilt, A. (2020). The environmental price of fast fashion. Nat. Rev. Earth Environ. 1, 189–200. doi: 10.1038/s43017-020-0039-9
Paswal, S., Chatha, J., Gupta, A., Gupta, I. V., Mahajan, I. S., Gupta, V., et al. (2021). Evaluation of different culture media for in vitro mycelial growth of different strains of shiitake mushroom. Pharma Innov. J. 10, 962–965.
Raman, J., Kim, D. S., Kim, H. S., Oh, D. S., and Shin, H. J. (2022). Mycofabrication of mycelium-based leather from Brown-rot Fungi. J. Fungi 8:317. doi: 10.3390/jof8030317
Royse, D. J., Baars, J., and Tan, Q. (2017). “Current overview of mushroom production in the world,” in Edible and medicinal mushrooms: Technology and applications. eds. D. C. Zied and A. Pardo-Giménez (John Wiley & Sons), 5–13.
Sobal, M., Martínez-Carrera, D., Morales, P., and Roussos, S. (2007). Classical characterization of mushroom genetic resources from temperate and tropical regions of Mexico. Micologia Aplicada Internacional. 19, 15–23. Available at: https://www.documentation.ird.fr/hor/fdi:010056278
Sánchez, C. (2010). Cultivation of Pleurotus ostreatus and other edible mushrooms. Appl. Microbiol. Biotechnol. 85, 1321–1337. doi: 10.1007/s00253-009-2343-7
Tello, I., Montiel, E., Romero, O., Nava, E., and Leon, I. (2016). Comparative mycelial growth of Pleurotus djamor and Pleurotus ostreatus in culture media. J. Pure Appl. Microbiol. 10, 2563–2568. doi: 10.22207/JPAM.10.4.11
Voora, V., Larrea, C., Bermudez, S., and Baliño, S. (2020). Global market report: Cotton sustainable commodities marketplace series 2019.
Workman, D. (2019). Cotton exports by country 2023. Available online at: https://www.worldstopexports.com/cotton-exports-by-country/ (Accessed September 26, 2024).
Keywords: textile waste, upcycling, mycelium, Pleurotus ostreatus , circular economy
Citation: Fletcher IA (2025) Evaluating Pleurotus ostreatus growth for upcycling textile waste from the fashion industry. Front. Sustain. 6:1522527. doi: 10.3389/frsus.2025.1522527
Edited by:
Souad El Hajjaji, Mohammed V University, MoroccoReviewed by:
Ivan Ruziak, Technical University of Zvolen, SlovakiaNajoua Labjar, Mohammed V University, Morocco
Copyright © 2025 Fletcher. This is an open-access article distributed under the terms of the Creative Commons Attribution License (CC BY). The use, distribution or reproduction in other forums is permitted, provided the original author(s) and the copyright owner(s) are credited and that the original publication in this journal is cited, in accordance with accepted academic practice. No use, distribution or reproduction is permitted which does not comply with these terms.
*Correspondence: Ian A. Fletcher, aWFmbGV0Y2hlckBpY2xvdWQuY29t
†Present address: Ian A. Fletcher, Florida Agricultural and Mechanical University, Tallahassee, FL, United States