- 1Institute for the Advanced Study of Sustainability, United Nations University, Tokyo, Japan
- 2Department of Applied Physics, Osaka University, Ōsaka, Japan
Cities facing direct risks and vulnerabilities to get damaged and harmed due to natural disasters are increasingly expected to implement a distinctive and positive role in promoting resilience. Among many other factors, a key component in sustaining urban resilience is the cleanliness of the air, which contributes to achieving a blue sky. In this context, this Article aims to discuss the strategies grounded on the circular economy approach, which are influential in addressing various forms of air pollution. In our approach, we consider the manifold origins of air pollution, including factors such as rapid urbanization, waste generation, industrial and wastewater pollutants, vehicular emissions, and wildfires that pose a complex challenge. Our recommendations to deal with these challenges revolve around addressing them by embracing circular economy strategies, which is crucial for achieving clean air in urban areas. We discuss the importance of clean air for resilient cities and outline critical strategies for building resilience through circular economy practices. Aligning with the United Nations Sustainable Development Goals, this article sheds light on the vital role of the circular economy in realizing blue skies for resilient cities.
1 Introduction
The third United Nations (UN) World Conference on Disaster Risk Reduction (The United Nations Office for Disaster Risk Reduction (UNISDR), 2015) held in March 2015 adopted “The Sendai Framework for Disaster Risk Reduction 2015–2030” as an international guideline for disaster risk reduction in response to creating urban resiliency. The aim of the Framework was “the substantial reduction of disaster risk and losses in lives, livelihoods and health and in the economic, physical, social, cultural and environmental assets of persons, businesses, communities, and countries.” Later in the same year in September, UN adopted the 2030 Agenda for Sustainable Development under “Transforming our World: The 2030 Agenda for Sustainable Development” (United Nations, 2015). This agenda outlines 17 Sustainable Development Goals (SDGs) and 169 targets arranged around five pillars (5Ps): people, planet, prosperity, peace, and partnership, to put the world on a sustainable and resilient path while leaving no one behind. This interconnected framework addresses the three core dimensions of sustainable development: social, environmental, and economic. One of these goals, SDG 11 (Sustainable Cities and Communities), aims to make cities and human settlements inclusive, safe, resilient, and sustainable. Particularly, target 11.6, which articulates “By 2030, reduce the adverse per capita environmental impact of cities, including by paying special attention to air quality and municipal and other waste management”, focuses on environmental issues, including the importance of clean air, to make cities resilient and sustainable.
The concept of blue skies refers to the aspiration for clean, unpolluted air and the environmental and public health benefits that come with it. The World Health Organization (WHO) emphasizes the need for air quality improvements, highlighting that poor air quality is responsible for millions of premature deaths globally each year (World Health Organization, 2021). By promoting cleaner energy sources, reducing industrial emissions, and encouraging sustainable urban development, the concept of blue skies serves as both a vision and a target for policymakers and environmental advocates, which has important environmental and climate implications. The United Nations has recognized the importance of clean air through the International Day of Clean Air for Blue Skies, celebrated annually to raise awareness and encourage action towards reducing air pollution. As more cities and nations work to achieve blue skies, the integration of sustainable practices, such as adopting circular economy principles, becomes crucial in combating both air pollution and climate change (United Nations Environment Programme (UNEP), 2024a).
According to the Organisation for Economic Co-operation and Development, a global association, resilient cities are designed and constructed to keep their original purposes and support citizens’ health and happiness. They can also handle and bounce back from possible future shocks, stress, and difficulties. Cities can improve their capacity to flourish in the face of change and uncertainty and offer their residents safe, healthy, and sustainable environments by incorporating resilience into their design (Resilience City Network, 2024). Resilient cities focus on keeping their residents healthy and taking care of the environment by reducing risks and ensuring they are less vulnerable. Resilient cities are created based on being inclusive, eco-friendly, and ensuring efficient use of resources. Incorporating a variety of features and strategies, such as green infrastructure, renewable energy, efficient waste management, adequate public transportation, affordable and competent housing, disaster preparedness, and climate adaptation measures in a comprehensive and interconnected manner with cutting-edge technologies and novel approaches, is necessary to hypothesize a resilient city. While there are multidirectional factors that need to be considered for sustaining the urban resilience, one of the fundamental requirements to build a resilient city is to maintain a pollution-free environment, nurturing clean air and blue sky, which comes as result of controlling various sources of pollutants, including industrial, domestic, vehicular, and greenhouse emissions. Furthermore, clean air is not only essential for safeguarding public health but is also vital for enduring local economy in various ways, making it one of the most important parameters for gauging the urban resilience.
According to a UN report, the world’s population may rise by 2.5 billion by 2050, with two-thirds of people living in cities (United Nations Department of Economic and Social Affairs (UN DESA), 2022), where it is projected that 43 megacities with a population of at least 10 million each could exist worldwide by 2030, the majority of them being in developing nations (United Nations Department of Economic and Social Affairs (UN DESA), 2022). This is expected to lead to significant urbanization and urban population growth, which would place tremendous strain on a city’s resources, escalating existing issues and generating new ones that will harm the environment and people’s quality of life. Infrastructure development, waste management systems, energy consumption, and the demand for fossil fuels are all expected to be under tremendous pressure from anthropogenic activities. In addition, household activities, such as heating, cooling, and cooking, are expected to contribute to both indoor and outdoor pollution increasingly. These anticipated activities have a direct effect on urban air quality (Khajuria and Verma, 2024). Figure 1 illustrates some of the common causes that contribute to air pollution in an urban setting, leading to various environmental and human health issues and strongly affecting the resilience of the cities.
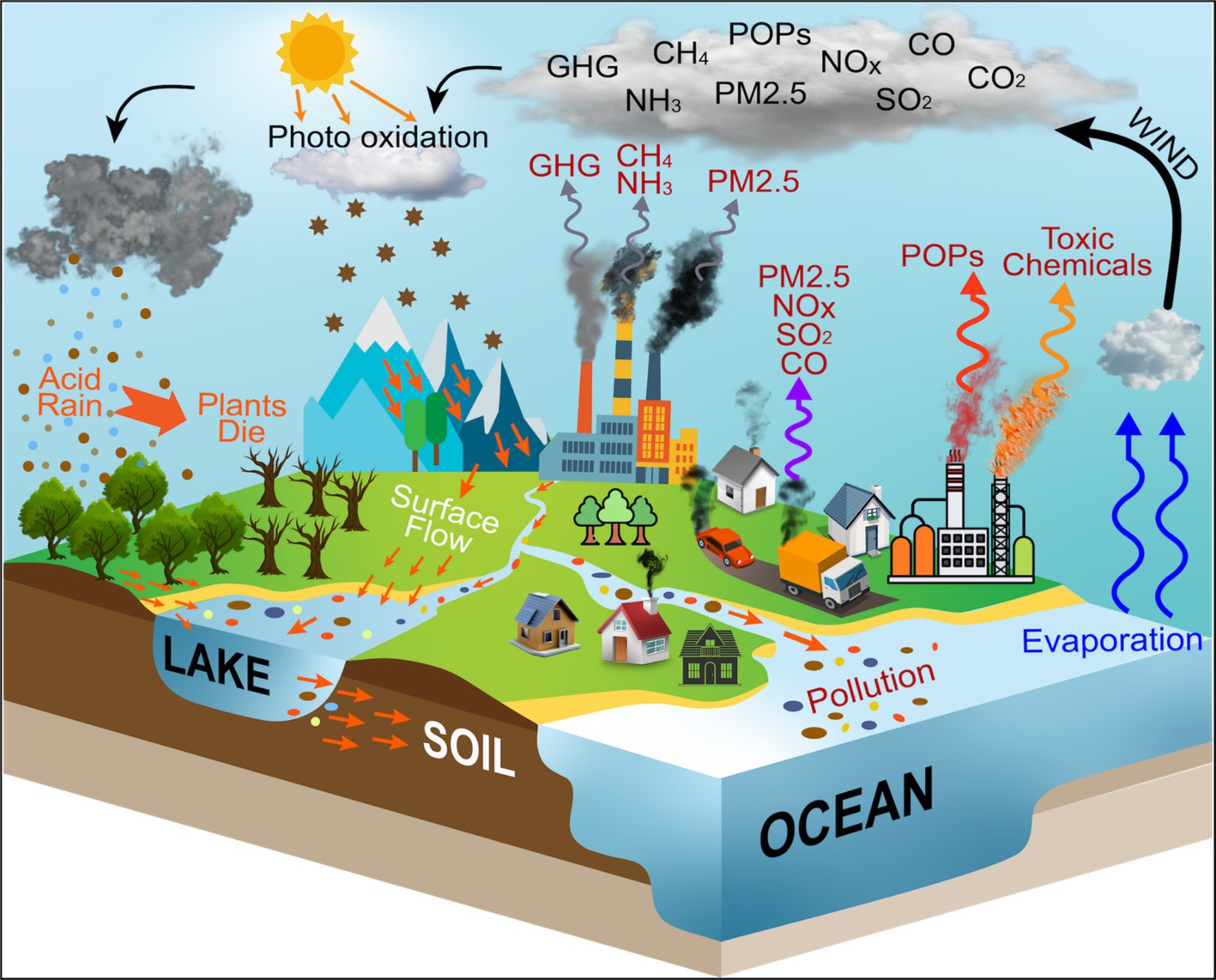
Figure 1. Presence of hazardous substances in the urban atmosphere and their origins. They produce harmful effects on air, waterbodies, soil, and forests and diminish the resilience of urban areas.
Sustainable development, guided by the UN SDGs, represents a holistic approach to fostering economic growth, social well-being, and environmental protection, all contributing to urban resilience. The circular economy and technological advancements serve as crucial tools for encouraging the sustainable development of cities, helping to reduce air pollution and promoting economic growth. To effectively combat air pollution, businesses must innovate and devise efficient strategies that align with the principles of resource efficiency and the circular economy (Global Alliance on Circular Economy and Resource Efficiency (GACERE), 2024). These policies and technologies reduce environmental pressures on our planet while facilitating the establishment of resilient cities. By prioritizing energy efficiency and addressing urban air pollution, the circular economy fosters sustainable urban development and a blue sky through the responsible use of resources.
Additionally, inclusive and quality education can act as a catalyst in promoting the principles of the circular economy for clean air. Fostering awareness, innovation, and education support, the development of cities that can withstand and adapt to the challenges of the 21st century and beyond can be well-promoted. Education plays a pivotal role in linking the circular economy and the creation of resilient cities by equipping individuals with the knowledge, skills, and innovation needed to address urban sustainability challenges. It bridges gaps by promoting skill development in areas such as green technologies, which are essential for clean air and for strengthening urban resilience. By embedding circular economy concepts into curricula and community training, education helps cities mitigate and adapt to the growing pressures of pollution and resource scarcity, aligning with the goals of creating cleaner, more sustainable urban environments.
In line with the SDGs, this article discusses critical strategies for creating resilient cities, promoting the circular economy, and achieving clean air for resilient cities. It points out the important factors that affect urban air pollution and recommends strategies to overcome them by implementing the circular economy approach into core strategies. It also elucidates the power of education in raising awareness and understanding, promoting skill development, and driving innovation to achieve clean air and blue skies.
2 Clean air: a key strategy for building resilient cities
The ability of a city to bounce back from difficulties is affected by various things, like its buildings and roads, the urban infrastructure, the general facilities for the citizens, the people who live there, and its ability to adapt. A holistic approach is necessary to plan and construct a resilient city to address all aspects of urban life. One can take several approaches while designing a resilient city, as shown in Table 1. Cities can strengthen their capacity to recover and improve the general well-being of their residents by implementing a comprehensive and inclusive approach (Resilience City Network, 2024). However, any approach that one takes to build a resilient city must aim to achieve clean and pollution-free air. That includes adopting approaches that aim to minimize construction and industrial pollution, chemical and vehicular exhausts, greenhouse gas emissions, and the like. High levels of air pollution can undermine the resilience of urban areas by affecting public health, reducing the quality of life, and imposing heavy costs on healthcare systems (Bikis, 2023). Frequent increases in air pollution can also impact local economies in various ways, including the need for emergency measures such as driving bans and factory shutdowns, disrupting daily life and affecting economic activities. The C40 Clean Air Cities Declaration (C40 Cities, 2022) is a crucial component in the broader vision of building resilient cities, as it directly addresses the need for urban areas to safeguard public health, enhance the quality of life, and mitigate environmental risks through improved air quality. By committing to reduce emissions from key sources such as industrial activities, vehicles, and construction, cities that adhere to the C40 Clean Air Cities Declaration are not only tackling pollution but also fortifying their resilience against the cascading impacts of poor air quality on healthcare systems, infrastructure, and community well-being.
3 Crucial factors impeding the realization of clean air for resilient cities
3.1 Air pollution caused by greenhouse gas emissions
Greenhouse gases are substances found in the atmosphere of the Earth that trap heat, causing the greenhouse effect and ultimately warming the planet (Intergovernmental Panel on Climate Change (IPCC), 2014). By absorbing some of the heat radiated back from the Earth’s surface in the form of infrared radiation, these gases not only allow sunlight to enter the atmosphere but also help to keep the planet warm. However, an excessive buildup of these gases in the atmosphere can lead to climate change as they continue to store heat and increase atmospheric temperature. Maintaining a moderate amount of these gases in the atmosphere is a crucial strategy for keeping the atmosphere sufficiently warm without stepping beyond a particular temperature. Air pollution produced through fossil fuel combustion for energy production and various industrial exhausts dominantly contribute to the increased greenhouse effect by releasing significant amounts of greenhouse gases into the atmosphere. In addition, these gases have potentially disastrous effects, including rising sea levels, more frequent and severe natural disasters, and several health risks hindering the city’s resilience. Urban development must adopt practices that aim to produce low amounts of greenhouse gases and maintain cleaner air, which needs strategic planning while building a resilient city.
3.2 Air pollution caused by particulate matter
Tiny liquid droplets and solid particles suspended in the atmosphere are called “particulate matter,” or PM. These particles may contain a range of constituents, including metals, organic materials, soot, dust, and chemical pollutants. Based on their sources and the environment, particulate matter can have various properties. Their sizes also vary, with PM2.5 designating particles with a diameter of 2.5 micrometers or less, a size about 3% the diameter of human hair, which is small enough for them to penetrate human lungs and enter the bloodstream. These tiny particles, which come from various sources, including dust, industrial emissions, vehicle exhaust, wildfires, and combustion processes, have substantial environmental impacts, severely affecting the air quality. Increased PM2.5 concentration can contribute to poorer air quality, which can reduce visibility, cause haze and smog to form, and cause acid rain (Wu and Zhang (2018)). These particles are also harmful for human health and are one of the dominant causes of various respiratory problems, such as asthma, impairing lung function, and increasing cardiovascular risks, including heart attacks and strokes (Pope and Dockery, 2006), intensely affecting the resilience of cities. Strategies should be implemented to minimize PM2.5 and its exposure to the city’s residents.
3.3 Air pollution caused by Ammonia release
Ammonia (NH3) is a compound of nitrogen and hydrogen that can be released from both natural and human activities, contributing significantly to air pollutants. Although ammonia is crucial for various industrial productions, it poses significant environmental and health risks when present in high concentrations in the atmosphere, polluting the urban air. Furthermore, the atmospheric chemistry of ammonia is complex, as it can react with various atmospheric acids and produce particulate matter such as ammonium nitrate and ammonium sulfate, contributing to creating haze and affecting air quality (Xu et al., 2020). Its release, primarily from agricultural practices such as fertilizer application and livestock waste, as well as from industrial processes, poses a serious environmental and public health threat (Wyer et al., 2022). While certain natural processes, such as the breakdown of organic matter, microbial activity in the soil, and volcanic eruptions, are some of the primary sources of atmospheric ammonia, human activity is the leading cause of ammonia emissions, mainly through agricultural practices like raising livestock and applying fertilizer, ignoring proper procedure. Understanding the multifaceted impacts of increased atmospheric ammonia is essential for building resilient cities with clean skies and developing effective mitigation strategies.
3.4 Air pollution caused by vehicle exhaust
Vehicle exhaust emissions are a major contributor to urban air pollution, posing a serious threat to the environment and public health. These emissions jeopardize climate stability and diminish the overall resilience of urban areas, particularly in densely populated cities with heavy traffic. The negative impacts of such pollution extend beyond immediate health concerns, affecting the quality of life and the sustainability of urban ecosystems. These emissions consist of various pollutants, including PM2.5, nitrogen oxides, carbon monoxide, carbon dioxide, methane and volatile organic compounds (VOCs). The complex interaction of these pollutants with the urban environment and public health leads to a variety of challenges. While nitrogen oxides and VOCs contained in vehicle exhaust can react with sunlight to form ground-level ozone, smog, and acid rain, PM2.5 contributes to reduced visibility and can carry toxic organic compounds into the respiratory system (Gurjar et al., 2010). Prolonged exposure to high levels of vehicle exhaust is associated with a range of serious health issues, including chronic respiratory diseases, lung cancer, cardiovascular diseases, and asthma. It is crucial to implement practices that decrease these harmful components in vehicle exhaust.
3.5 Air pollution caused by persistent organic pollutants (POPs)
A class of harmful chemicals known as persistent organic pollutants (POPs) can persist in the environment for long periods. These pollutants are artificial organic substances that are toxic, bioaccumulated through the food cycle, and are highly persistent in the environment because they withstand natural process degradation. Pesticides, industrial chemicals, and unintended byproducts of specific industrial processes are just a few of the chemical substances that fall under the category of POPs (United Nations Environment Programme (UNEP), 2005). They are widely distributed because they can travel great distances by air, water, and migratory birds and are found globally, including in urban areas where their concentration can be particularly harmful. Because of their persistence, toxicity, and ability to travel great distances, POPs present severe risks to cities even far from their source locations. As they are mostly synthetic chemicals, POPs are typically found in industrial wastes, exhausts, and agricultural pesticide leaks because they are mostly synthetic chemicals (Khajuria and Verma, 2021). They can indirectly affect climate by changing atmospheric processes despite not directly contributing to the greenhouse effect like carbon dioxide and other greenhouse gases do. Certain POPs can accelerate the formation of tropospheric ozone, and cloud formation can be impacted by the presence of POPs in the atmosphere. POPs have been associated with a range of serious health issues, including cancer, hormone disruption, reproductive disorders, and immune system suppression (Guo et al., 2019). Due to their ability to persist in the environment and accumulate in the human body over time, even low levels of exposure can be dangerous, which calls for a greater concern while designing a resilient city.
3.6 Air pollution caused by industries
Industrialization has significantly shaped urban areas, fueling economic growth and creating employment opportunities. Yet it is like a double-edged sword, as the resilience of cities is threatened by industries in addition to the advantages they provide, not only because of air pollution but also because of land and water pollution. Hazardous substances and harmful emissions in the form of solids, liquids, and gases are frequently produced by industrial activities. The solid wastes are either dumped in landfills or bodies of water or burned outside, which frequently release toxic gases into the atmosphere, pollute the soil, and discharge liquids into water bodies (Hoornweg and Bhada-Tata, 2012). Even land and water pollutants frequently evaporate into the atmosphere and pollute the air. These pollutants may include greenhouse gases like carbon dioxide, nitrogen oxides, methane, and ammonia; airborne particles like smoke and dust; PM2.5 and POPs; and other airborne substances like smoke. Industrial air pollution significantly affects the resilience of urban areas by increasing incidences of respiratory and cardiovascular diseases among the citizens, levitating healthcare costs, reducing workforce productivity, and the need for extensive pollution control measures that can hinder economic growth (United Nations Environment Programme (UNEP), 2017). The rising healthcare demands lead to greater public and private expenditures on medical treatments, diverting resources away from other areas of economic development.
4 Circular economy and its contribution to cities’ resilience
In recent years, the global economic model has undergone a significant shift away from its traditional linear approach. The former method operated on a linear trajectory, where products moved in a straight line from production to consumption, and once utilized, resources were disposed of without consideration for recycling or reuse (Geissdoerfer et al., 2017). Known as the linear economy, this system involved extracting natural resources, converting them into products, and ultimately discarding them as waste (Stahel, 2016), leading to substantial waste generation. This continuous extraction of finite natural resources not only depletes them but also exacerbates their scarcity, raising concerns for the future (Ellen MacArthur Foundation, 2013). The disposal of various waste streams, such as plastic, electronic, construction, and industrial waste, further contaminates the land, water, and air, posing significant threats not only to environmental sustainability but also to urban resilience, as polluted environments can lead to health issues, decreased quality of life, and increased vulnerability to climate change impacts. This linear mindset, which was focused solely on economic growth and resource utilization, failed to address the escalating depletion of natural resources, the rising amount of waste, and the consequential environmental impacts (Geissdoerfer et al., 2017). Ultimately, the linear economy has proven to be a polluting system that disrupts the climate system, diminishes biodiversity, and destroys urban resilience.
In contrast, the concept of a circular economy has emerged as a climate-resilient alternative designed to minimize waste generation by maximizing resource efficiency. This approach creates a win–win situation where natural resources are saved, and waste generation is minimized while goods are produced (Kirchherr et al., 2017). In a circular economy, nearly all products are designed for reuse, repair, or remanufacture, thus extending their lifecycle and minimizing waste. Instead of following a linear trajectory from production to disposal, products circulate within a closed-loop system (Ellen MacArthur Foundation, 2013), where waste serves as a valuable raw material for new products. Figure 2 illustrates the distinction between linear and circular economy models. Transitioning to a circular economy presents a more sustainable and economically viable prospect compared to the linear model, as it emphasizes resource conservation through practices such as recycling, refurbishing, and reusing (Geissdoerfer et al., 2017). In fact, the concept of circular economy is based on ten Rs, which are discussed later. By embracing sustainable consumption habits and supporting initiatives (United Nations Environment Programme (UNEP), 2024b) such as urban farming and green infrastructure, consumers play a pivotal role in driving the circular economy forward. Urban farming not only helps reduce food miles and waste but also improves air quality by sequestering carbon and producing oxygen, contributing to blue skies. Additionally, implementing green infrastructure such as permeable pavements and green roofs—can reduce urban heat and enhance stormwater management, making cities more resilient. Ultimately, the transition to a well-functioning circular economy requires collective efforts from the government, industry, and consumers. This collective effort is essential for sharing the responsibility of reducing waste and improving resource efficiency. Governments can implement supportive policies, industries can design sustainable products, and consumers can adopt responsible consumption behaviors. Together, these efforts foster innovation and create new business opportunities while promoting environmental sustainability.
The concept of the circular economy has roots in various historical and cultural practices from around the world, even if it is not always called that (Kirchherr et al., 2023). Many traditions that promote sustainability, like recycling, reusing, and sharing, have developed independently in different cultures over time. Particularly, some countries including China and Germany, have been implementing practices and policies that resonate with circular economy principles, such as comprehensive waste management and recycling systems, for decades (Ogunmakinde, 2019). The Netherlands was one of the first countries in the world to integrate circular economy principles into their national strategy. In 2016, the Netherlands government launched a program aimed at developing a full circular economy by 2050 (Report of Netherlands, 2016), with intermediate targets for 2030. This government-led program is very comprehensive, focusing on sectors such as biomaterials, manufacturing, and construction, and it includes significant innovation and business incentives representing the commitment to shifting towards a circular economic model. Studies by Khajuria et al. (2022) underscore the significance of the circular economy in sustainable resource management, emphasizing the reuse, repair, and recycling of materials to minimize reliance on natural resources. These findings emphasize that the circular economy is the best course of action for advancing the UN 2030 Agenda for SDGs for urban resilience. The principles of a circular economy contribute to the resilience of cities by promoting resource efficiency, reducing vulnerability to supply chain disruptions, and boosting overall environmental sustainability (United Nations Environment Programme (UNEP), 2024b). By encouraging the development of local circular economies and integrating renewable energy sources, cities can reduce their ecological footprint while improving their capacity to withstand environmental challenges such as climate change and natural disasters (Yang et al., 2023). Additionally, the emphasis on circular design principles in urban planning and infrastructure development enables cities to optimize resource use, minimize waste generation, and create more livable, equitable, and resilient urban environments.
Moreover, a circular economy fosters innovation and collaboration across various sectors, inspiring the development of green technologies, sustainable business models, and circular supply chains. The principles of a circular economy can significantly mitigate air pollution, as they promote the use of cleaner production methods and encourage waste reduction strategies that limit harmful emissions. For example, by redesigning products for longevity and reparability, manufacturers can reduce the need for new raw materials, which often require energy-intensive extraction and processing, resulting in lower air pollutants. Additionally, public awareness and education initiatives play a crucial role in promoting the adoption of circular practices among consumers and communities, empowering individuals to make informed choices that support the transition to cleaner air and a more resilient society. By reimagining the way we produce, consume, and dispose of goods, a circular economy holds the potential to create cleaner, healthier environments and contribute to blue skies, ensuring a more sustainable future for the next generations.
4.1 The R-strategies for circular economy
According to the Netherlands Environmental Assessment Agency, there are ten R-strategies for action to a circular economy, which include refuse, rethink, reduce, reuse, repair, refurbish, remanufacture, repurpose, recycle, and recover (Potting et al., 2017).
These ten R-strategies, R0–R9, which fall under a hierarchy, are useful tools for visualizing and understanding the different stages of resource use and waste management in a circular economy. They are classified into three categories that demonstrate the length of the waste loop each represents (Table 2). The shorter the loop, the more sustainable the strategy. The three categories are:
1. Short loops that focus on smarter product use and manufacture: R0: Refuse, R1: Rethink, R2: Reduce.
2. Medium loops that focus on life extension strategies: R3: Reuse, R4: Repair, R5: Refurbish, R6: Remanufacture, R7: Repurpose.
3. Long loops that focus on creative material applications: R8: Recycle, R9: Recover.
5 Circular economy for blue skies
The ten R-strategies of the circular economy play crucial roles in creating pollution-free atmospheres and fostering blue skies by revolutionizing resource management practices and consumption patterns. By efficiently using materials and minimizing waste generation, the circular economy indirectly reduces the demand for new resource extraction and production processes. These processes are energy-intensive and polluting, and they often serve as major sources of atmospheric pollutants. Employing circular economy strategies results in lower emissions of GHGs and other pollutants, thereby helping to improve air quality. For example, by keeping materials in use for as long as possible and encouraging the repurposing of waste, circular economy practices directly reduce the waste sent to landfills and incineration facilities, both of which contribute to air pollution. Additionally, minimizing the use of natural resources through the circular economy decreases the demand for energy-intensive manufacturing and transportation processes, which are responsible for a significant share of air pollution and GHG emissions. Studies indicate that circular economy strategies in Europe could lead to a 56% reduction in CO₂ emissions by 2050, primarily due to decreased energy consumption in production processes (European Environment Agency (EEA), 2016). Cities can utilize and implement circular economy strategies to reduce air pollutants and promote a culture of resource efficiency and sustainable consumption, ultimately contributing to addressing the root causes of air pollution. However, it requires a deliberate combination of R-strategies tailored to each pollutant. While Refusing (R0) is essential for banning some highly hazardous and toxic materials altogether, Rethinking (R1) serves as the primary framework for new innovations. Additionally, Reduce (R2), Reuse (R3), Repair (R4), Recycle (R8), and Recover (R9) offer operational strategies applied across various urban sectors to minimize pollution at its source, extend resource lifecycles, and safely manage waste streams, ultimately contributing to improved urban air quality and enhanced resilience.
5.1 Circular economy strategies for industrial and construction waste management
Industrial waste poses severe environmental risks as it may contain pollutants that degrade air quality, including toxic gases, GHGs, and POPs. To prevent these waste-generated air pollutants from compromising air quality, it is essential to manage them carefully and strategically. Reducing the amount of waste sent to landfills or incineration is one of the key benefits of the circular economy for controlling air pollution. In this context, Rethink (R1), Reuse (R3), Repurpose (R7), Recycle (R8), and Recover (R9) strategies of the circular economy can be employed to mitigate waste-generated air pollution. The circular economy views waste as a valuable resource that can be recovered, reused, repurposed, and ultimately reused into the production cycle to create a closed-loop system rather than a burden. Waste production can be significantly reduced by rethinking product design with durability, repairability, and recyclability in mind (Kirchherr et al., 2017), which can help reduce toxic gas and POP emissions from industrial waste. Eco-design inspired by the circular economy further encourages the development of products that are more durable, repairable, and recyclable, focusing on reducing waste at the source. These strategies tackle the root cause of toxic emissions by minimizing waste generation and supporting more sustainable product life cycles. Therefore, embracing circular practices for managing industrial waste is essential to achieving the goal of blue skies.
On the other hand, the construction sector also presents several environmental threats that must be addressed to achieve clean air for resilient cities. Various construction-related activities, including extracting raw materials, excessive energy consumption, and debris waste production, contribute to these dangers. Approximately 60% of the world’s natural resources are consumed by industries, with a significant portion allocated to building materials and infrastructure development (World Economic Forum, 2022), making the construction industry a major contributor to air pollution. Additionally, the high demand for raw materials in construction can lead to overuse and biodiversity loss (World Economic Forum, 2022), ultimately impacting urban resilience. Proper management of construction waste is essential to prevent harm to the environment and human health, particularly through the Refuse (R0), Reduce (R2), Reuse (R3), and Repurpose (R7) strategies of the circular economy by avoiding certain materials in the construction process and reducing waste by reusing it directly or by recovering useful components from the waste. Circular construction can promote the use of recycled aggregates, reclaimed wood, and salvaged materials instead of relying solely on virgin resources materials. Implementing cleaner technologies, installing pollution control devices, adhering to stricter standards, and shifting to renewable energy sources during the planning of industrialization can be further effective in reducing industrial air pollution in urban areas. Consequently, adopting circular economy strategies for managing industrial and construction waste helps achieve blue sky goals and promotes the transition to a low-carbon economy.
5.2 Circular economy strategies for controlling air pollutants
As discussed earlier, the most significant pollutants in urban air include GHGs, PM2.5, ammonia, and POPs, all of which degrade air quality and hinder the achievement of a blue sky for resilient cities. Implementing a circular economy within urban settings presents a significant opportunity to mitigate these air pollutants, minimizing emissions while enhancing urban resilience through improved resource efficiency and waste reduction. Specific R-strategies can be adopted within a circular economy framework to effectively address decreasing GHGs, PM2.5, ammonia, and POPs in urban air, providing a systematic approach to resource management and waste reduction while directly addressing the sources of these pollutants. Mitigating these air pollutions is also closely linked to several SDGs. Table 3 summarizes the main pollutants found in urban air, their key sources, the circular economy strategies to address these contaminants, and the SDGs associated with them.
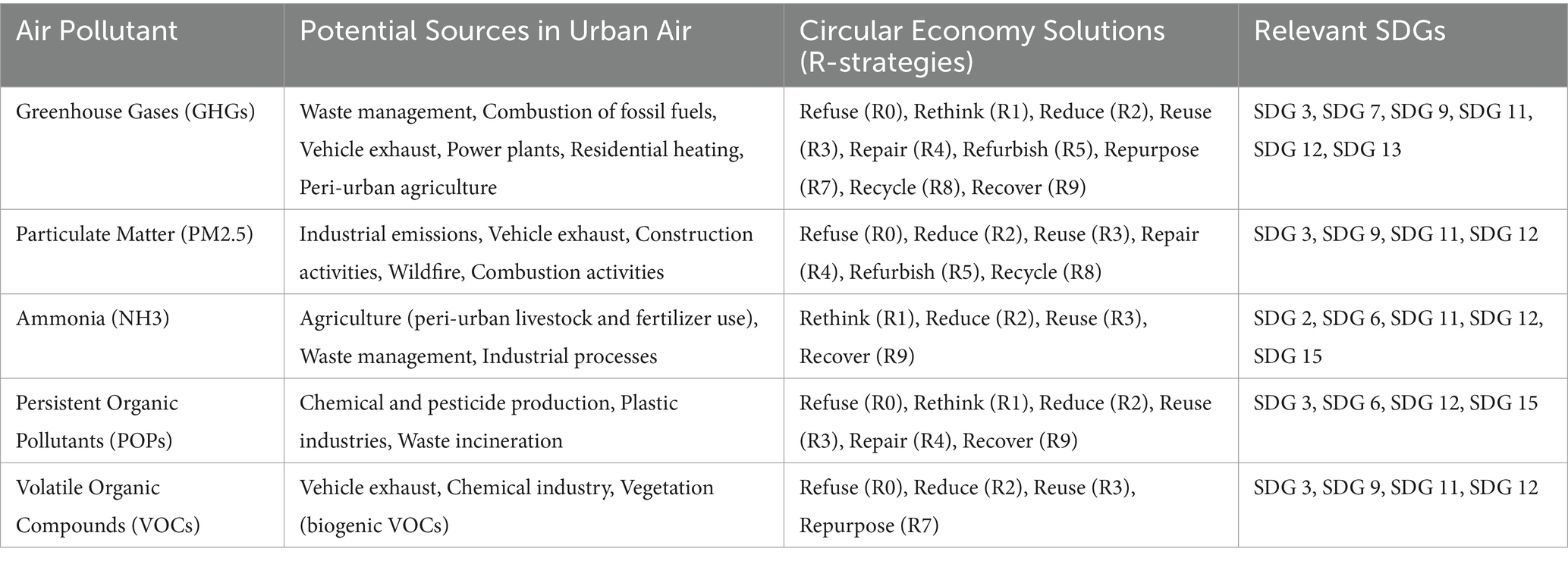
Table 3. Potential urban air pollutants, their key sources, the circular economy strategies to address these contaminants, and the associated Sustainable Development Goals (SDGs).
5.2.1 Greenhouse gases (GHGs)
Several R-strategies of the circular economy are essential for lowering urban GHG emissions. Rethinking (R1) is a fundamental strategy that necessitates a systemic shift in urban planning and infrastructure design to prioritize low-carbon solutions. This involves reimagining energy systems to favor renewable sources. The reducing strategy (R2) is directly relevant through energy efficiency measures in buildings and industries and by minimizing overall consumption. The strategies of reusing (R3) in transportation, including shared vehicle programs, and in construction, through the repurposing of building components, reduce the demand for new production and the associated GHG emissions. Recovering (R9) is also crucial for organic waste management, where capturing biogas from the anaerobic digestion of organic waste helps reduce methane emissions and generates renewable energy. Recycling (R8) supports material production, as using recycled materials in construction and manufacturing lessens the energy-intensive processes associated with virgin material extraction and processing. All these practices in planning a resilient city can reduce GHG emissions and contribute to a blue sky for urban resilience.
5.2.2 Particulate matter (PM2.5)
Mitigating PM2.5 pollution benefits significantly from the strategies of Refuse (R0), Reduce (R2), Reuse (R3), Repair (R4), and Recycle (R8). Refuse strategies target the sources of PM2.5 by preventing pollution at its origin, for instance, by refusing the use of highly polluting construction materials or processes and promoting cleaner industrial production methods. Reducing strategies are crucial, for example, in transportation, encouraging less reliance on private vehicles while promoting public transit, which decreases traffic-related PM2.5. In construction, reducing material usage through efficient design and minimizing demolition waste limits dust generation. Reuse and repair strategies in construction, by utilizing reclaimed materials and components and extending the lifespan of buildings, reduce the need for new construction and the associated dust and PM2.5 emissions. Recycling in construction and industry decreases the demand for virgin materials, often involving processes that release PM2.5. Adopting various circular economy strategies can help significantly reduce PM2.5 from the urban air.
5.2.3 Ammonia
Reducing ammonia emissions in urban areas primarily involves the strategies of Reduce (R2), Reuse (R3), and Recover (R9). Reducing the use of synthetic fertilizers in urban green spaces and peri-urban agriculture, a major source of ammonia, is essential. Promoting precision agriculture and optimizing fertilizer application directly decrease ammonia volatilization. Reuse strategies can be implemented by utilizing composted urban organic waste as a natural fertilizer rather than synthetic alternatives. Recovery strategies play a crucial role in wastewater treatment, where nutrient recovery technologies can extract nitrogen and phosphorus from wastewater, reducing ammonia release from sewage treatment plants while creating valuable fertilizer products, thus closing nutrient loops. Reducing ammonia in urban air is vital due to its harmful effects on public health, and implementing circular economy strategies can aid in achieving this goal towards a blue sky.
5.2.4 Persistent organic pollutants (POPs)
Addressing POPs pollution in urban air requires a strong emphasis on Refuse (R0), Reduce (R2), Reuse (R3), Repair (R4), Recycle (R8), and Recover (R9) strategies, focusing on prevention and safe management. Refuse is paramount, demanding the rejection of POPs in product design and manufacturing processes while opting for safer alternatives. Reducing overall consumption minimizes the production and potential release of products containing POPs. Reuse and Repair strategies extend product lifespans, delaying their entry into waste streams and lowering the demand for new products that might contain POPs. Recycling strategies must be implemented with care for materials containing POPs, requiring specialized processes to ensure that the toxic POPs are not released during recycling and are safely contained or destroyed. In some cases, Recovery strategies, such as specialized incineration with advanced emission controls, may be necessary for the safe disposal of waste streams containing POPs when recycling is not feasible, ensuring destruction under controlled conditions. Additionally, Rethinking (R1) is crucial to drive innovation in material science and product design to eliminate the use of POPs altogether and develop truly circular material flows that are inherently safe. Given their persistent and highly toxic nature, reducing POPs in urban air is vital for achieving blue skies and resilient cities.
5.3 Circular economy for alternative sources of clean energy
The circular economy plays a crucial role in promoting alternative sources of clean energy, thereby contributing to cleaner air and enhancing the resilience of urban areas. The shift towards a circular economy involves utilizing dissembled and recycled products for manufacturing renewable energy sources, such as wind turbines and solar panels. For instance, the use of recycled metals in solar panel production can reduce the environmental footprint associated with mining and refining raw materials, effectively minimizing energy consumption and greenhouse gas emissions (Heath et al., 2022). A shift to renewable energy sources for supplying electricity to homes and production activities reduces reliance on fossil fuels, which are some of the major contributors to air pollution and urban smog. By embracing a circular economy approach encourages the development of energy-efficient technologies and infrastructure, such as smart grids (Reindl et al., 2024), which further reduce the carbon footprint of cities. It aims to increase access to clean, renewable energy sources, which have unlimited energy potential and can help fight pollution and climate change. Clean energy can lessen the strain on natural resources, pave the way for cutting emissions to zero, spur sustainable economic growth and employment, and lower supply chain risk.
Clean energy covers a range of processes, including mining, the creation of materials, the production of technology, the transmission and distribution of energy, and the construction of supporting infrastructure. Technological advancements have also enabled the capture and reuse of waste heat from residential and industrial sources, creating new opportunities for energy recycling. This innovation not only enhances energy efficiency but also aligns with the principles of the circular economy, which advocates for resource recovery and waste minimization throughout the energy lifecycle. By scaling up the recycling of renewable energy technologies, thereby facilitating the achievement of net-zero emissions (Paul et al., 2020). Adopting a circular economy strategy for producing clean and green energy can help lessen the sector’s adverse environmental effects while fostering the resilience of urban areas in the long run. By optimizing energy consumption and reducing waste through recycling material, repurposing by-products, and redesigning for longevity, urban areas can achieve significant improvements in air quality, enhancing public health and overall urban resilience against environmental stresses and climate change impacts (Impact on Urban Health, 2020).
6 Importance of education of circular economy in achieving blue skies
The importance of education in the circular economy for promoting clean air is indisputable. It serves as a key driver for innovation and resilience in urban areas, essential for achieving the goals of blue skies. By investing in education, cities can equip their residents with the knowledge, skills, and attitudes needed to make informed decisions and take actions that contribute to a healthier environment and a more sustainable future. The connection between education in the circular economy and clean air plays a profound and multifaceted role in fostering blue skies and promoting resilient cities. This relationship underscores not only the intrinsic value of education in societal advancement but also its central role in environmental protection and urban resilience.
6.1 Awareness and understanding
Education plays a fundamental role in cultivating awareness of the interconnectedness between linear consumption patterns, waste generation, and urban air pollution. Proper education should raise awareness about the benefits of circular economy approaches, where individuals can understand that outdated linear economic models, which significantly contribute to air pollution through industrial emissions, transportation exhaust, and waste incineration, must be avoided. Specifically designed educational initiatives should clarify the principles of a circular economy, emphasizing closed-loop systems, resource efficiency, and the reduction of pollutants at their source. By sharing knowledge about the environmental impacts of conventional practices, education can foster a greater understanding of the necessity for transitioning to circular models, thereby driving demand for sustainable alternatives and reducing the acceptance of polluting practices. Integrating these topics into awareness campaigns, workshops, and community outreach programs can effectively convey the importance of the circular economy. Furthermore, incorporating these subjects into educational curricula across all levels, from primary education to higher education, ensures that individuals learn from a young age about their roles in environmental stewardship (Bennett et al., 2009). In addition to formal education, public engagement can deepen understanding of air quality issues, leading to community-driven activities for cleaner air (United Nations Educational, Scientific and Cultural Organization (UNESCO), 1999).
6.2 Skill development
The implementation of circular economy principles necessitates a skilled workforce good at designing, implementing, and managing sustainable systems, where education extends beyond theoretical knowledge as it serves as a crucial catalyst by employing multifaceted approaches, including skill development. These skills are essential for creating and executing solutions to complex challenges that hinder the cleanliness of urban air. Educational programs should focus on cultivating practical skills in waste management, recycling technologies, sustainable product design, and urban planning that aim toward reducing pollutants in urban air. Training initiatives centered on resource recovery, remanufacturing, and eco-innovation can provide individuals with the necessary expertise to develop circular solutions for achieving cleaner urban air. Additionally, education needs to encourage interdisciplinary collaboration, allowing professionals from various fields like engineering, design, and policy to unite in creating integrated solutions for reducing air pollution. Educational institutions can further facilitate partnerships with local businesses and government agencies to create programs that provide students with opportunities to engage in projects that directly contribute to reducing air pollution. By establishing a strong skill foundation, education enables communities to effectively execute circular economy strategies, resulting in significant decreases in air pollution to achieve blue skies for resilient cities (United Nations Educational, Scientific and Cultural Organization (UNESCO), 2015).
6.3 Behavioral change
Education with well-designed curricula of circular economy plays a crucial role in fostering behavioral changes that support the transition to a circular economy. Through quality education and engagement, individuals can develop more conscientiousness about their actions and their potential impact on the environment, including air quality. Educational programs, both in a classroom framework and in public awareness campaigns, can encourage individuals to adopt sustainable lifestyles that are aligned with the principles of the circular economy and promote sustainable consumption patterns to reduce their reliance on single-use products, adopt repair and reuse practices, and prioritize sustainable transportation options, all of which contribute to achieving cleaner urban air. By highlighting the health benefits of clean air and the environmental impacts of individual choices, education can inspire citizens to embrace eco-conscious lifestyles. Furthermore, education should tackle the social and cultural norms that preserve unsustainable practices, nurturing a collective sense of responsibility for environmental stewardship. These hands-on initiatives not only provide practical knowledge but also inspire individuals to become advocates for sustainability within their communities. By encouraging behavioral changes at both individual and community levels, education significantly reduces pollution from consumer activities and transportation (Mariam and Knuth, 2024).
6.4 Policy advocacy and implementation
Education empowers individuals and organizations to engage in policy advocacy and contribute to the development of effective environmental regulations, ensuring that efficient and equitable policies are upheld (United Nations Summit, 2023). These policies encourage renewable energy, reduce emissions, and promote sustainable urban growth. By providing a solid understanding of circular economy principles and their potential impact on air quality, education enhances the capacity of stakeholders to participate in public discourse and advocate for sustainable policies. Educational programs also equip policymakers and urban planners with the knowledge and tools necessary to implement circular economy strategies at municipal and regional levels. This includes developing incentives for sustainable businesses, establishing infrastructure for waste segregation and recycling, and integrating circular principles into urban planning initiatives. By fostering informed policy advocacy and implementation, education enables the creation of a regulatory environment that supports the transition to a circular economy and promotes clean urban air.
Education is essential for successfully implementing circular economy principles in urban environments, resulting in enhanced air quality. By fostering awareness, developing skills, promoting behavioral changes, and enabling informed policy advocacy, education empowers individuals and institutions to contribute to the development of sustainable, pollution-free cities. A comprehensive educational strategy that includes formal education, public awareness campaigns, and professional training is crucial for maximizing the circular economy’s potential in reducing urban air pollution and securing a healthier future for all. Cities that prioritize educational initiatives are more likely to evolve into resilient urban areas capable of addressing current and future environmental challenges. Well-designed quality education not only enlightens individuals but also paves the way for sustainable urban development and a cleaner, fairer world. Moreover, educational institutions can serve as catalysts for change by fostering collaborations between students, local governments, and non-profit organizations to create a unified voice advocating for sustainable policies (Kioupi and Voulvoulis, 2022).
7 Resilient urban air pollution, and the circular economy: towards the UN 2030 agenda for sustainable development
Approaches based on the principles of the circular economy can significantly improve the resilience of urban areas in terms of economy, society, and environment. A resilient city is characterized by its ability to anticipate, prepare for, respond to, and recover from adverse events while maintaining essential functions and fostering sustainable development. This concept is closely aligned with Sustainable Development Goal (SDG) 11, which aims to make cities and human settlements inclusive, safe, resilient, and sustainable (United Nations, 2015). However, the benefits of resilient cities extend beyond SDG 11, contributing significantly to other global goals, such as clean water and sanitation (SDG 6), affordable and clean energy (SDG 7), climate action (SDG 13), and broader socio-economic objectives, including poverty eradication (SDG 1), good health and well-being (SDG 3) and quality education (SDG 4).
Urban areas are primary sources of key air pollutants, including greenhouse gases (GHGs), particulate matter (PM2.5), ammonia (NH₃), and persistent organic pollutants (POPs), which originate from industrial activities, transportation, energy production, waste mismanagement, and agricultural emissions (World Health Organization, 2021). These pollutants lead to climate change and environmental degradation and highlighting the urgent need for integrated strategies to mitigate their effects. The circular economy is integral to building resilient cities, as it promotes sustainable resource management, waste reduction, and the regeneration of natural systems. It helps cities mitigate environmental impacts associated with urbanization, fosters economic growth, and enhances social inclusion, thereby addressing multiple SDGs concurrently. For example, by prioritizing waste minimization and resource efficiency, cities can support SDG 12, which advocates for responsible consumption and production patterns. Likewise, transitioning towards circular business models within urban systems can spur innovation in industry and infrastructure (SDG 9) while creating green jobs and enhancing economic opportunities (SDG 8) (International Energy Agency (IEA), 2021).
Persistent organic pollutants (POPs) pose another significant challenge for urban resilience, as they accumulate in the environment and pose long-term risks to ecosystems and human health. These pollutants originate from industrial chemicals, pesticides, and waste combustion, necessitating stricter regulations and the adoption of circular solutions, such as cleaner production technologies, extended producer responsibility schemes, and closed-loop material recovery (United Nations Environment Programme (UNEP), 2024b). By integrating these approaches into urban policies, cities can minimize hazardous emissions and support SDG 14 (life below water) and SDG 15 (life on land) by preventing contamination of natural ecosystems. Moreover, the transition to circular economies fosters multi-stakeholder partnerships (SDG 17), driving collaborative efforts between governments, industries, and communities to develop innovative and scalable solutions to urban pollution and climate resilience (Rockefeller Foundation, 2017). Similarly, addressing ammonia emissions from agricultural activities through sustainable farming practices, circular models, and nutrient recovery systems can contribute to improved air quality while supporting SDG 2 (zero hunger) and SDG 6 (clean water and sanitation) (Ellen MacArthur Foundation, 2019). As one can notice here, the concept of resilient cities, clean air, blue sky, and the integration of circular economy in achieving them are deeply interlinked with almost all SDGs, which are depicted in Figure 3. By prioritizing these strategies, cities can effectively prepare for and respond to contemporary challenges while simultaneously laying the groundwork for a stable and sustainable future. Building resilience in urban areas is about creating a sustainable foundation that supports all aspects of human development, paving the way for a stable and sustainable future in line with the UN’s 2030 Agenda.
8 Future perspective and conclusion
A blue sky, representing clean urban air, is not only a symbol of environmental health but also a foundational element for resilient cities capable of adapting to, withstanding, and recovering from environmental, economic, and social challenges while prioritizing the well-being of their residents. The connection between a blue sky and resilient cities stems from their mutual goal of fostering sustainable, livable urban spaces. Key contributors to urban air pollution that hinder the goal of a blue sky include various toxic gases such as ammonia and GHGs, as well as dust, particulate matter, POPs, and other hazardous chemicals. These pollutants originate from diverse sources, both natural and human-made, often stemming from carelessness and mismanagement related to urbanization, waste management, agricultural practices, transportation, and more, all of which require proper upgrades and regulation. Circular economy practices, which aim to minimize waste and maximize resource use by keeping products, materials, and components in circulation for as long as possible, can significantly reduce the generation of various pollutants and pave the way toward achieving a pollution-free blue sky. In this section, we present perspectives and recommendations for implementing circular economy practices to attain clean air in urban environments, focusing on developing resilient cities where we examine the major contributors to urban air pollution.
One significant contributor to air pollution is GHG emissions, which a circular economy framework can address through several key interventions. Firstly, transitioning to renewable energy sources is essential. Urban areas can foster distributed renewable energy generation, such as solar panels on rooftops and wind turbines in peri-urban areas, thereby reducing dependence on fossil fuel-based energy, a major source of greenhouse gases. Secondly, improving energy efficiency in buildings and infrastructure is crucial. Adopting circular design principles in construction, using recycled and locally sourced materials, and encouraging building retrofits for enhanced insulation and energy management can significantly reduce the carbon footprint of the built environment. Furthermore, promoting sustainable urban mobility through integrated public transportation systems and shared mobility services decreases reliance on private vehicles, another significant contributor to greenhouse gas emissions in cities. Waste management also plays an important role, where circular economy principles advocate for minimizing waste generation through source reduction, reuse, and comprehensive recycling programs. Organic waste, a major source of methane, a potent GHG, can be diverted from landfills through composting and anaerobic digestion, producing biogas for energy generation and nutrient-rich compost for urban agriculture.
Reducing PM2.5 concentrations in urban air, another major contributor to air pollution, requires addressing various urban sources such as construction, transportation, and industrial activities. In construction, adopting circular practices that emphasize material reuse and recycling can minimize dust generation and reduce the need for resource-intensive material production, which often includes processes that emit PM2.5. Utilizing recycled aggregates, reclaimed timber, and other secondary materials diminishes the environmental impact of construction and demolition. For industrial activities located within or near urban areas, implementing industrial symbiosis, where waste from one industry serves as a resource for another, can reduce pollution. Additionally, promoting cleaner production processes and incorporating advanced filtration technologies in industries can directly cut PM2.5 emissions. While the government can implement and enforce stricter air quality standards to decrease emissions from industrial sources and vehicles, educating citizens about the sources and health risks associated with PM2.5 can encourage behavioral changes, such as reducing car usage, wearing masks in high-risk areas, and transitioning to green energy solutions. To reduce exposure to PM2.5 and protect both human health and the environment, it is also crucial for the general public to be aware of and adhere to air quality regulations and guidelines.
An equally important contributor to air pollution, ammonia, often originates from agricultural activities and waste management processes in peri-urban areas. Urban agriculture can integrate closed-loop nutrient systems to mitigate ammonia emissions within a circular economy framework. This includes utilizing composted urban organic waste as fertilizer and applying precision agriculture techniques to optimize fertilizer use, thereby minimizing excess nitrogen that can volatilize as ammonia. Additionally, improving wastewater treatment processes to recover nutrients like nitrogen and phosphorus can reduce ammonia release from sewage treatment plants. Agricultural and industrial practices must also be strategically planned, and related policies need to be regulated to decrease ammonia emissions into the atmosphere. Using the appropriate amount of fertilizer at the right time and employing nitrification inhibitors, which slow down the conversion of ammonia into nitrate in the soil, can significantly reduce ammonia volatilization (Norton and Ouyang, 2019). Implementing low-emission livestock farming technologies is also effective in lowering atmospheric ammonia levels (Wyer et al., 2022). Industries that emit high levels of ammonia, such as petroleum refineries and chemical plants, should install ammonia scrubbers before releasing exhaust into the atmosphere. Collectively, these circular economy strategies address both the sources and processes contributing to elevated atmospheric ammonia levels, ultimately promoting cleaner air and a healthier ecosystem for blue skies.
Another dominant air pollutant, POPs, poses a complex challenge due to their persistent presence in the environment and associated health risks. A POP-free environment is essential for a resilient city, which requires implementing legislative measures, adopting pollution prevention techniques, establishing monitoring and surveillance systems, and executing public awareness campaigns (United Nations Environment Programme (UNEP), 2024b). To minimize the amount of POPs released into the environment, it is crucial to properly handle, store, and dispose of products containing POPs (Muir and Howard, 2006). Employing advanced air and water filtration technologies in industrial processes to capture and destroy POPs before they are released into the environment will help reduce POP emissions into the atmosphere (Trojanowicz, 2020). A circular economy approach to reducing POPs emphasizes preventing both their generation and release throughout product lifecycles, starting with product design. It highlights the importance of eliminating POPs in manufacturing processes and selecting safer alternatives. Promoting product longevity and reuse decreases the demand for new products, thereby minimizing the overall production of items that may contain POPs. Advanced waste treatment technologies, such as specialized incineration or chemical treatment processes, are essential for safely managing waste streams that contain POPs. Industrial symbiosis can also play a role by facilitating the exchange of materials and byproducts to reduce the generation of POPs and encourage the use of safer substitutes. By implementing these measures, cities can lower the presence of POPs in the air and mitigate their adverse effects, thus enhancing urban resilience, safeguarding public health, and supporting clean air initiatives towards urban resilience.
Furthermore, vehicle exhaust can be a significant source of air pollution, particularly in densely populated urban areas. Along with various GHGs and PM2.5, it also contains nitrogen oxides, carbon oxides, methane, and VOCs. Adopting circular economy strategies, designing vehicles with recyclable materials, implementing remanufacturing processes, and encouraging the use of electric or hybrid engines that rely on renewable energy can greatly reduce both emissions generated during manufacturing and those released during vehicle operation. Additionally, sustainable resource management in fuel production, such as utilizing biofuels or green hydrogen, further aids this transition. By embracing these strategies, cities can enhance air quality, improve public health, and increase their resilience against the negative impacts of vehicle exhaust emissions on urban air.
In conclusion, this article discussed the significance of clean air and blue skies in designing resilient cities, supported by the 2030 Agenda for Sustainable Development, which emphasizes the crucial relationship between the circular economy and urban resilience. Cities prioritizing resilience must adopt the circular economy concept to transform into resilient urban areas. Strategies aimed at reducing greenhouse gas emissions, particulate matter, ammonia, and other harmful compounds such as persistent organic pollutants, alongside industrial pollution and vehicle exhaust, are vital for attaining clean air and blue skies. Innovations in industrial infrastructure and planning, technological advancements, sustainable transportation options, and well-designed efficient waste management policies, coupled with the implementation of circular economy approaches, are imperative. Furthermore, prioritizing education in all relevant fields empowers citizens to engage in sustainable practices and contribute to building resilient cities. Achieving blue skies and emphasizing sustainable urban development are essential for effectively addressing future crises and protecting the well-being of urban populations. Beyond reaching the goal of blue skies, the numerous benefits of circular economy practices include reduced environmental impact, resource preservation, economic growth, job creation, and enhanced capacity to manage resource scarcity. Transitioning from the traditional linear model to a circular economy can foster a sustainable and regenerative economic system that acknowledges the planet’s limits and ensures long-term prosperity for both present and future generations. Many UN-SDGs align well with the circular economy approach towards building resilient cities, assisting our efforts to create a better future and enhance the world for all.
Data availability statement
The original contributions presented in the study are included in the article/supplementary material, further inquiries can be directed to the corresponding author.
Author contributions
AK: Conceptualization, Data curation, Formal analysis, Investigation, Methodology, Resources, Supervision, Validation, Visualization, Writing – original draft, Writing – review & editing. PV: Conceptualization, Data curation, Formal analysis, Investigation, Methodology, Resources, Supervision, Validation, Visualization, Writing – original draft, Writing – review & editing.
Funding
The author(s) declare that no financial support was received for the research and/or publication of this article.
Conflict of interest
The authors declare that the research was conducted in the absence of any commercial or financial relationships that could be construed as a potential conflict of interest.
Publisher’s note
All claims expressed in this article are solely those of the authors and do not necessarily represent those of their affiliated organizations, or those of the publisher, the editors and the reviewers. Any product that may be evaluated in this article, or claim that may be made by its manufacturer, is not guaranteed or endorsed by the publisher.
References
Bennett, E. M., Peterson, G. D., and Gordon, L. J. (2009). Understanding relationships among multiple ecosystem services. Ecol. Lett. 12, 1394–1404. doi: 10.1111/j.1461-0248.2009.01387.x
Bikis, A. (2023). Urban air pollution and greenness in relation to public health. J. Environ. Public Health 2023, 1–18. doi: 10.1155/2023/8516622
C40 Cities. (2022). C40 Clean Air Cities Declaration. Available online at: https://www.c40.org/wp-content/uploads/2022/02/C40-Clean-Air-Cities-Declaration_Public-progress-report_Feb-2022.pdf (Accessed March 28, 2025).
Ellen MacArthur Foundation. (2013). Towards the circular economy: economic and business rationale for an accelerated transition. Available online at: https://www.ellenmacarthurfoundation.org/towards-the-circular-economy-vol-1-an-economic-and-business-rationale-for-an (Accessed March 28, 2025).
Ellen MacArthur Foundation. (2019). Cities and circular economy for food. Available online at: https://emf.thirdlight.com/file/24/K6LOnIrKMZq-8vK6HoTK6iyBra/Cities%20and%20circular%20economy%20for%20food.pdf (Accessed March 28, 2025)
European Environment Agency (EEA). (2016). Circular economy in Europe – Developing the knowledge base. Available online at: https://www.eea.europa.eu/publications/circular-economy-in-europe (Accessed March 28, 2025).
Geissdoerfer, M., Savaget, P., Bocken, N., and Hultink, E. (2017). The circular economy – a new sustainability paradigm? J. Clean. Prod. 143, 757–768. doi: 10.1016/j.jclepro.2016.12.048
Global Alliance on Circular Economy and Resource Efficiency (GACERE). (2024). Circular Economy, Pollution and Chemicals- Working Paper. Available online at: https://www.unido.org/sites/default/files/unido-publications/2024-07/GACERE-Working-paper-Circular-Economy-Pollution.pdf (Accessed March 28, 2025).
Guo, W., Pan, B., Sakkiah, S., Yavas, G., Ge, W., Zou, W., et al. (2019). Persistent organic pollutants in food: contamination sources, health effects and detection methods. Int. J. Environ. Res. Public Health 16:4361. doi: 10.3390/ijerph16224361
Gurjar, B. R., Jain, A., Sharma, A., Agarwal, A., Gupta, P., Nagpure, A. S., et al. (2010). Human health risks in megacities due to air pollution. Atmos. Environ. 44, 4606–4613. doi: 10.1016/j.atmosenv.2010.08.011
Heath, G., Dwarakanath, R., Silvana, O., Leroy, W., Taylor, C., Dev, M., et al. (2022). Environmental and circular economy implications of solar energy in a decarbonized U.S. grid. Golden, CO: National Renewable Energy Laboratory. NREL/TP-6A20-80818. Available online at: https://www.nrel.gov/docs/fy22osti/80818.pdf (Accessed March 28, 2025).
Hoornweg, D., and Bhada-Tata, P. (2012). What a waste: A global review of solid waste management. World Bank. Available online at: https://hdl.handle.net/10986/17388 (Accessed March 28, 2025).
Impact on Urban Health. (2020). Newsletter- Wha are the causes of air pollution in inner cities – and how bad is it? Available online at: https://urbanhealth.org.uk/insights/reports/what-are-the-causes-of-air-pollution-in-inner-cities-and-how-bad-is-it (Accessed May 7, 2024).
Intergovernmental Panel on Climate Change (IPCC). (2014). Climate change 2014: Synthesis report. Contribution of Working Groups I, II and III to the Fifth Assessment Report of the Intergovernmental Panel on Climate Change [Core Writing Team, R.K. Pachauri and L.A. Meyer (eds.)]. IPCC, Geneva, Switzerland, 151 pp.
International Energy Agency (IEA). (2021). Net zero by 2050 – a roadmap for the global energy sector. Licence CC BY 4.0. Available online at: https://iea.blob.core.windows.net/assets/deebef5d-0c34-4539-9d0c-10b13d840027/NetZeroby2050-ARoadmapfortheGlobalEnergySector_CORR.pdf (Accessed March 28, 2025).
Khajuria, A., and Verma, P. (2021). “Chapter 13: United Nations sustainable development goals: a future free from persistent organic pollutants and other toxic Chemicals for all” in Book: Book persistent organic pollutants in the environment. Edition1st ed (Boca Raton, Florida: CRC Press), 17.
Khajuria, A., Atienza, V. A., Chavanich, S., Henning, W., Islam, I., Kral, U., et al. (2022). Accelerating circular economy solutions to achieve the 2030 agenda for sustainable development goals. Circ. Econ. 1:100001. doi: 10.1016/j.cec.2022.100001
Khajuria, A., and Verma, P. (2024). Chapter 1: Clean air for resilient cities- an important director for UN SDGs. Book: Clean air for blue skies- an understanding of air pollution concerns and its abatement (New Delhi: K. K. Publications), 1–22.
Kioupi, V., and Voulvoulis, N. (2022). Education for sustainable development as the catalyst for local transitions toward the sustainable development goals. Front. Sustain. 3:889904. doi: 10.3389/frsus.2022.889904
Kirchherr, J., Reike, D., and Hekkert, M. (2017). Conceptualizing the circular economy: an analysis of 114 definitions. Resour. Conserv. Recycl. 127, 221–232. doi: 10.1016/j.resconrec.2017.09.005
Kirchherr, J., Yang, N. N.-H., Schulze-Spüntrup, F., Heerink, M. J., and Hartley, K. (2023). Conceptualizing the circular economy (revisited): an analysis of 221 definitions. Resour. Conserv. Recycl. 194, 107001–103449. doi: 10.1016/j.resconrec.2023.107001
Mariam, M., and Knuth, M. (2024). Circular economy by engaging in the community. Department of Horticultural Sciences at North Carolina State University. Available online at: https://sustainabloom.org/wp-content/uploads/2024/04/9.ConsumerGuide_CircularEconomies.pdf (Accessed March 28, 2025).
Muir, D. C. G., and Howard, P. H. (2006). Are there other persistent organic pollutants? A challenge for environmental chemists. Environ. Sci. Technol. 40, 7157–7166. doi: 10.1021/es061677a
Norton, J., and Ouyang, Y. (2019). Controls and adaptive Management of Nitrification in agricultural soils. Front. Microbiol. 10:1931. doi: 10.3389/fmicb.2019.01931
Ogunmakinde, O. E. (2019). A review of circular economy development models in China, Germany and Japan. Recycling 4:27. doi: 10.3390/recycling4030027
Paul, D., Jensen, P. P., and Anne, P. M. V. (2020). Highlighting the need to embed circular economy in low carbon infrastructure decommissioning: the case of offshore wind. Sustain. Prod. Consump. 24, 266–280. doi: 10.1016/j.spc.2020.07.012
Pope, C. A. III, and Dockery, D. W. (2006). Health effects of fine particulate air pollution: lines that connect. J. Air Waste Manage. Assoc. 56, 709–742. doi: 10.1080/10473289.2006.10464485
Potting, J., Hekkert, M., Worrell, E., and Janemaaijer, A. (2017). Circular economy: Measuring innovation in the product chain. Available online at: https://www.pbl.nl/sites/default/files/downloads/pbl-2016-circular-economy-measuring-innovation-in-product-chains-2544.pdf (Accessed March 28, 2025).
Reindl, K., Dalhammar, C., and Brodén, E. (2024). Circular economy integration in smart grids: a Nexus for sustainability. Circ. Econ. Sust. 4, 2119–2145. doi: 10.1007/s43615-024-00375-5
Report of Netherlands (2016). A Circular Economy in the Netherlands by 2050. Available online at: https://circulareconomy.europa.eu/platform/sites/default/files/17037circulaireeconomie_en.pdf (Accessed May 5, 2024).
Resilience City Network. (2024). Resilience practice: A guide to updating resilience strategies for success 2024.
Rockefeller Foundation. (2017). 100 Resilient Cities. Available online at: https://www.rockefellerfoundation.org (Accessed March 28, 2025).
The United Nations Office for Disaster Risk Reduction (UNISDR). (2015). Adopted the Sendai Framework for Disaster Risk Reduction 2015–2030. Available online at: https://www.undrr.org/media/16176/download?startDownload=20240505 (Accessed May 5, 2024).
Trojanowicz, M. (2020). Removal of persistent organic pollutants (POPs) from waters and wastewaters by the use of ionizing radiation. Sci. Total Environ. 718:134425. doi: 10.1016/j.scitotenv.2019.134425
United Nations. (2015). Transforming our world: the 2030 Agenda for Sustainable Development Available online at: https://sustainabledevelopment.un.org/content/documents/21252030%20Agenda%20for%20Sustainable%20Development%20web.pdf (Accessed March 28, 2025).
United Nations Department of Economic and Social Affairs (UN DESA). (2022). World population prospects 2022. Available online at: https://www.un.org/development/desa/pd/sites/www.un.org.development.desa.pd/files/wpp2022_summary_of_results.pdf (Accessed March 28, 2025).
United Nations Educational, Scientific and Cultural Organization (UNESCO). (1999). Educating for a sustainable future: public awareness and understanding: the fuel for change. Available online at: https://unesdoc.unesco.org/ark:/48223/pf0000117162 (Accessed March 28, 2025).
United Nations Educational, Scientific and Cultural Organization (UNESCO). (2015). Not just hot air- putting climate education into practice.
United Nations Environment Programme (UNEP). (2005). Ridding the world of POPs: a guide to the Stockholm convention on persistent organic pollutants. Available online at: https://chm.pops.int/portals/0/repository/chm-general/unep-pops-chm-guid-ridding.english.pdf (Accessed March 28, 2025).
United Nations Environment Programme (UNEP). (2017). Towards a pollution-free planet background report. United Nations Environment Programme, Nairobi, Kenya. Available online at: https://wedocs.unep.org/bitstream/handle/20.500.11822/21800/UNEA_towardspollution_long%20version_Web.pdf?isAllowed=y&sequence=1 (Accessed March 28, 2025).
United Nations Environment Programme (UNEP). (2024a). The 2024 International of Clean air blue skies. A practical guide for individuals, cities, governments, visual identity guidelines | version 2024 schools & universities, businesses, civil society. https://www.cleanairblueskies.org/ (Accessed March 28, 2025).
United Nations Environment Programme (UNEP). (2024b). Global waste management outlook 2024: beyond an age of waste – turning rubbish into a resource. Nairobi. Available online at: https://wedocs.unep.org/20.500.11822/44939 (Accessed March 28, 2025).
United Nations Summit (2023). Report on the 2022 Transforming Education Summit Convened by the UN Secretary-General. Available online at: https://www.un.org/sites/un2.un.org/files/report_on_the_2022_transforming_education_summit.pdf (Accessed March 28, 2025).
World Economic Forum. (2022). How can the building materials sector tackle biodiversity loss? Available online at: https://www.weforum.org/agenda/2022/10/cop27-how-building-materials-sector-tackle-biodiversity-loss/ (Accessed March 28, 2025).
World Health Organization. (2021). WHO global air quality guidelines. Particulate matter (PM2.5 and PM10), ozone, nitrogen dioxide, sulfur dioxide and carbon monoxide. Geneva: World Health Organization; 2021. Licence: CC BY-NC-SA 3.0 IGO.
Wu, W., and Zhang, Y. (2018). (2018). Effects of particulate matter (PM2.5) and associated acidity on ecosystem functioning: response of leaf litter breakdown. Environ. Sci. Pollut. Res. 25, 30720–30727. doi: 10.1007/s11356-018-2922-1
Wyer, K. E., David, B., Kelleghan, D. B., Blanes-Vidal, V., Schauberger, G., and Curran, T. P. (2022). Ammonia emissions from agriculture and their contribution to fine particulate matter: a review of implications for human health. J. Environ. Manag. 323, 0301–4797. doi: 10.1016/j.jenvman.2022.116285
Xu, J., Chen, J., Zhao, N., Wang, G., Yu, G., Li, H., et al. (2020). Importance of gas-particle partitioning of ammonia in haze formation in the rural agricultural environment. Atmos. Chem. Phys. 20, 7259–7269. doi: 10.5194/acp-20-7259-2020
Keywords: circular economy, blue skies, clean air, resilient cities, 2030 Agenda for Sustainable Development Goals
Citation: Khajuria A and Verma P (2025) Circular economy for “blue skies” in building resilient cities—towards the UN 2030 Agenda for Sustainable Development Goals. Front. Sustain. 6:1479452. doi: 10.3389/frsus.2025.1479452
Edited by:
Desta Mebratu, Stellenbosch University, South AfricaReviewed by:
Kiriaki M. Keramitsoglou, Democritus University of Thrace, GreeceCopyright © 2025 Khajuria and Verma. This is an open-access article distributed under the terms of the Creative Commons Attribution License (CC BY). The use, distribution or reproduction in other forums is permitted, provided the original author(s) and the copyright owner(s) are credited and that the original publication in this journal is cited, in accordance with accepted academic practice. No use, distribution or reproduction is permitted which does not comply with these terms.
*Correspondence: Anupam Khajuria, a2hhanVyaWFAdW51LmVkdQ==; Prabhat Verma, dmVybWFAYXAuZW5nLm9zYWthLXUuYWMuanA=