- 1International Business and Communication, Zuyd University of Applied Sciences, Maastricht, Netherlands
- 2Sustainable International Business Research Center, Domain of International Business and Communication, Zuyd University of Applied Sciences, Maastricht, Netherlands
- 3SKEMA Business School, Lille, France
- 4Commodity Risk Management Expertise Center (CORMEC), Marketing and Consumer Behavior Group, Wageningen University and Research, Wageningen, Netherlands
Responding to the call for circular transition, the Dutch and French governments aspire to achieve a fully circular economy by developing a transitional agenda in various sectors, including fashion and construction. The two countries are among the top 10 waste generators in the European Union (EU), while the two sectors—fashion and construction—are the largest polluters in the EU. The aim of this study, and its main contribution, is to harmonize circular design principles, which vary by sector into common types, and identify the circular design principle that balances the sustainability dimensions the most. This responds to research gaps that merely describe these design principles applicable to different sectors but which are also silent on which achieves sustainability balance. Using multicriteria decision analysis, selected case studies of companies in the two sectors and countries were scored and ranked according to environmental, economic, and social sustainability indicators. The case projects were selected based on the circular design principle that the enterprises were applying. These principles were standardized for the two sectors to come up with five distinct types, namely, design for (i) biobased materials, (ii) service/adaptability, (iii) disassembly, (iv) waste and material recovery, and (v) longevity. Three forms of triangulation were used to achieve reliability, validity, and equivalence of the findings: (i) data—by doubling the size of the sample cases to 40 establishments from 20, (ii) investigator—by having the authors score the projects separately, and (iii) method—by using two objective weighting methods in scoring the criteria. These techniques resulted in similar rankings of the cases in terms of triple bottom-line scores per design principle. Designing for biobased materials turned out to achieve the most balance. The case projects were also compared regarding performance in achieving the UN Sustainability Development Goals (SDGs), which companies use to integrate sustainability with business. Circularity in fashion and construction primarily targets responsible consumption, production, and climate action. A tertiary SDG was life on water and access to clean water for fashion and life on land and sustainable cities for construction.
1 Introduction
In fashion, a catwalk is a raised platform where models walk to display clothes in runway shows. A catwalk is a raised narrow walkway or bridge built for construction workers. The catwalk is not the only thing that binds the fashion and construction industries, as both sectors have lately been archetypes of the circular economy (CE). The Ellen MacArthur Foundation (EMF), which has trail-blazed the transition to CE over the past decades, identified 10 key sectors to be significantly impacted in the near term (Ellen MacArthur Foundation, 2020). Fashion and construction are among these 10 sectors that will be driven by regulation, innovation, and customer preferences for circularity. In response to the call for the transition to the circular economy, both the Dutch and French governments launched policy directives to navigate toward a total CE, particularly targeting construction and fashion. The choice of the two countries and sectors is justified because both countries are among the top 10 waste generators in the EU. As shown in Annex Figure 1, France is second after Germany in waste production, while The Netherlands ranked seventh, following Italy, Poland, Sweden, and Romania. Fashion and construction are also the biggest polluters in the EU. Fashion ranked second among industrial polluters, contributing 8–10% of carbon emissions, as shown in Annex Figure 2.1 This is higher than what aviation and shipping combined produce. Similarly, fashion contributes to enormous water consumption and accounts for about 20% of global clean water pollution.2 Regarding per tonnage of textile waste in 2020, France ranked third after Italy and Germany, while The Netherlands ranked fifth after Belgium (Annex Figure 3). Construction, in turn, ranked first in terms of waste generation among economic sectors, as shown in Annex Figure 4 at 36% (Eurostat, n.d.), with France ranking second after Germany and The Netherlands ranking third in construction waste (Annex Figure 5).
The main research question posed by this study is “Which circular design principle most balances the environmental, social, and economic dimensions of sustainability, or what is referred to as the sustainability triptych or triple bottom line (TBL)?” The study addresses research gaps in the circular economy about the existence of a universal set of circular design principles that could be applied to any sector. A literature survey on circular design principles points to distinct circular principles applied to fashion,3 and another one for construction.4 Another research gap is the impact of the design principles on achieving the sustainability balance in accordance with the triple bottom line model of Elkington (1994). Hence, this article aims to harmonize the circular design principles into general typologies applicable across sectors and will compare them in terms of sustainability balance. Such information will help policymakers frame directives on circularity that aim for balanced sustainability and guide businesses in their compliance.
The study uses multicriteria decisional analysis (MCDA) to evaluate case studies of enterprises. Cases were selected based on the “harmonized” circular design principles the projects are applying. The case companies will be scored using the sustainability indicators as criteria to argue the case for balanced sustainability. Scoring the indicators based on a checklist of qualitative descriptions is the best approach,5 given that the suggested sustainability and circularity indicators6 require a breakdown of products into their material components. Moreover, different scales are used to measure their impact (e.g., kilowatt hours in energy reduction versus liters of water consumption), physical measurements of which are often not available. The sectors will also be matched up to achieve industry-specific UN Sustainability Development Goals (SDGs), which companies use to show sustainability compliance. The following study will be organized into the following parts. First is a literature review that provides a theoretical framework for the study. This is followed by a description of the research methodology, a discussion of the findings, and a conclusion on the general implications of the results, including limitations and scope for future research.
2 Literature review
Available bibliometric and scientometric analysis of the literature on CE and sustainability has been relatively recent, with the majority of the research occurring only since 2015–2016, as cited in Torcatoru et al. (2022), Schöggl et al. (2022), Zapucioiu and Trica (2021), and Mustafa and Legyel (2022). A sustainability assessment of the circular economy has been found lacking, with a focus on material flow accounting that requires standardization and the absence of social issues in favor of more environmental focus. Moreover, Schöggl et al. (2022) observe that CE and SDG-related topics have been an emerging research theme, with climate and energy taking prominence over other social SDGs. Bocken et al. (2016) invoked the need to develop methods for assessing the three sustainability pillars for CE business models. In response to this significant research gap, the scope of this section had to be narrowed down to relevant topics needed for the research design. This literature review provides a theoretical background of (a) the origins of the circular economy, (b) the circularity design principles, (c) policy measures on circularity introduced by the Dutch and French governments affecting construction and fashion, (d) sustainability indicators on environmental, social and economic dimensions to be used in MCDA that will be performed in the research, and (e) the UN SDGs, which companies use to incorporate corporate social responsibility (CSR). These topics reviewed in the literature will frame the research design used in the study. A theory of CE is needed for a unified understanding, which leads to the design principles (independent variable) that are then scored in terms of sustainability dimensions on environmental, economic, and social impacts to determine the balance on TBL (dependent variable). Many of these design principles are also influenced by policy mandates, a moderating variable that frames what companies should do to become sustainable, and the SDGs which is a mediating or intervening variable that companies incorporate into their CSR strategies. Hence, this study hypothesizes that the type of circularity design principle applied by a company determines sustainability balance.
2.1 Origins of the circular economy
Pearce and Turner (1990) formally introduced the word circular economy in the second chapter of their book, describing the path for economic growth within ecological limits. The early academic writings on CE resembled those of closed-loop supply chains described in industrial ecology, although focusing more on technical and engineering than business perspectives. There is no universal definition of CE based on literature surveys of De Angelis (2018) and Sillanpää and Ncibi (2019), although a global definition of circularity awaits some consensus. Rizos et al. (2017) summarize multiple CE definitions and interpretations as found in Annex Table 1. The difficulty of finding a precise definition is attributed to the concept of CE being intertwined with sustainable development, CSR, and business models. De Angelis (2018) reconciled the link between CE and business models, where the latter was a crucial constituent. A circular business model combines notions of “value proposition, creation, delivery, and capture” within the EMF’s ReSOLVE framework. The acronym stands for regenerate, share, optimize, loop, virtualize, and exchange. According to Mendoza et al. (2017), the ReSOLVE framework is the leading CE tool to accelerate CE business opportunities. This lacks implementation guidance, resulting in their proposed iRESOLVE framework, where “i” stands for “implement.”
In De Angelis’ survey, common principles of CE are listed from originators in various disciplines of economics7—industrial ecology,8 biomimicry,9 architecture,10 and management.11 Meanwhile, the survey by Sillanpää and Ncibi (2019) denounces the limited attention to the social dimension and predominance of the environment to complete the triptych of sustainable development (economy-environment-social) and CSR’s three P’s (people, planet, and profit) found in existing definitions. Evident from the surveys of the origins of CE is that the circular economy is conveniently juxtaposed against the alternative linear economy that is often characterized as a “take-make-use-dispose” paradigm, as shown in Figure 1. Common among the different constructs of CE is the idea of “closing the loop,” which means the disposal stage at the end feeds back into the production system to create circularity. Thus, the final state of waste disposal is linked to the concept of circularity, as this waste is looped back.
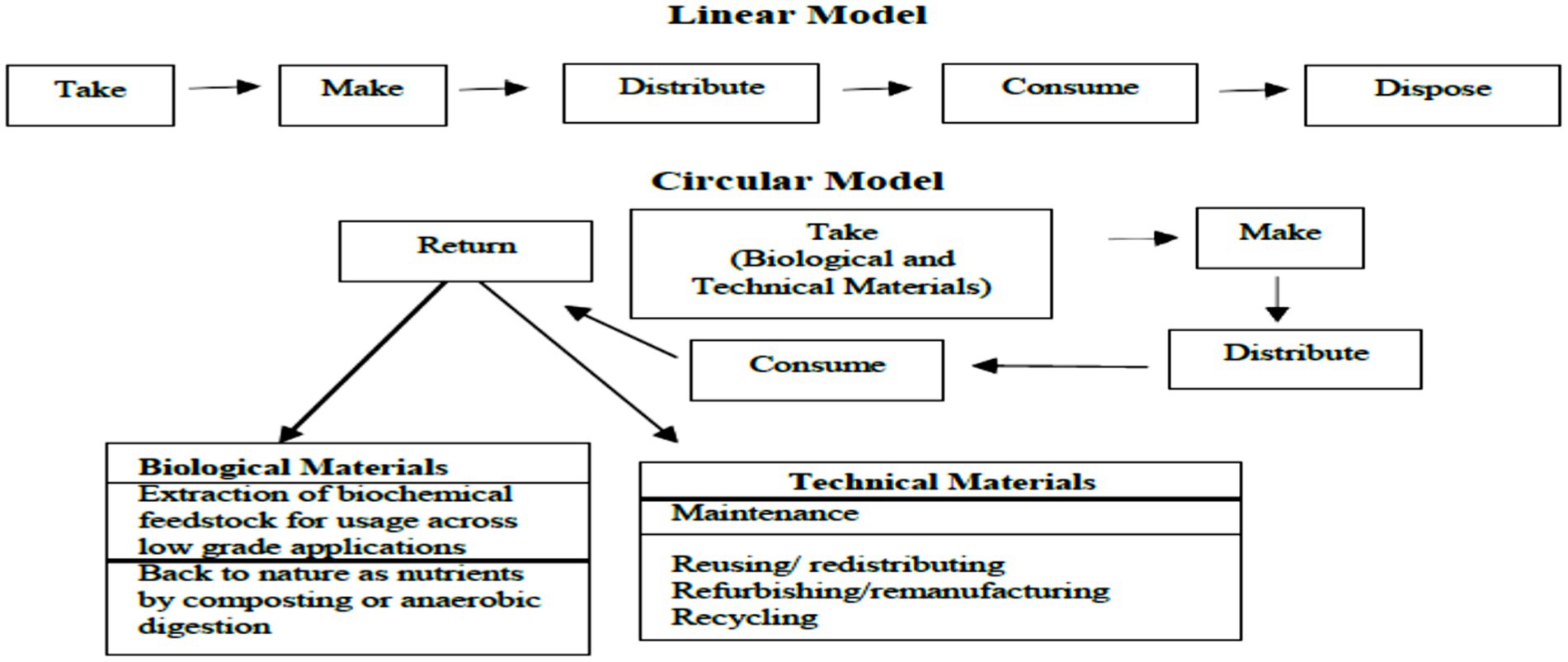
Figure 1. Circular versus linear economy. Source: adapted from De Angelis (2018, p.21).
In 2014, a Dutch politician, Lansink (1979), developed a waste hierarchy framework called Lansink’s Ladder, consisting of rungs (Figure 2) showing an order of preference of actions in reducing and managing waste. The ladder shows that prevention or avoidance through reducing, reusing, and recycling is preferable to disposing. Later used in the European Waste Frame Directive, the ladder was refined to incorporate circularity principles. Grant et al. (2017) developed a hierarchy of how waste is looped back that comprises the stages of circularity: reduce, reuse, recycle, recover, and dispose of, as shown in Figure 3. In this framework, waste prevention is preferable to waste disposal. Ghisellini et al. (2018), Kirchherr et al. (2017), and Pötting and Hanemaaijer (2018) extend the hierarchy to comprise the 10 R’s (refuse, rethink, reduce, reuse, repair, refurbish, remanufacture, repurpose, recycle, and recover)12 which is a more comprehensive paradigm, as illustrated in Figure 4.
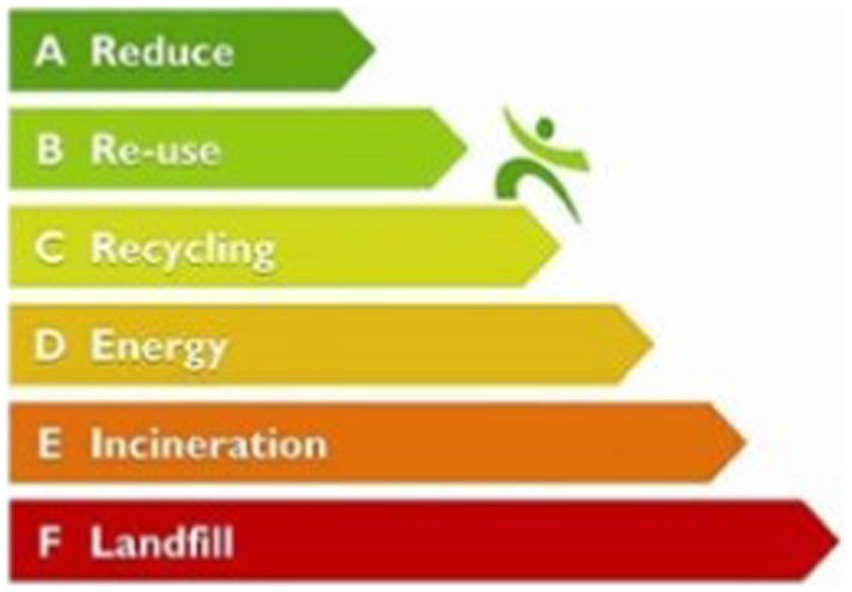
Figure 2. Lansink’s ladder. Source: Adapted from https://www.recycling.com
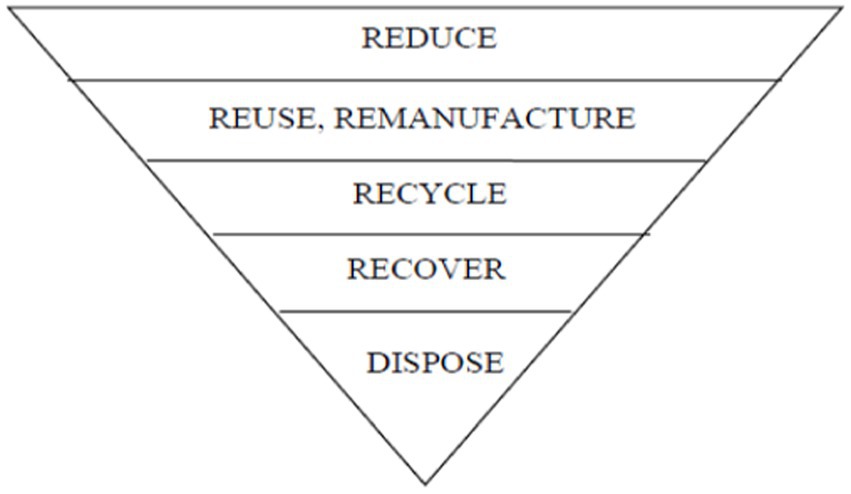
Figure 3. Hierarchy of waste management. Source: Adapted from Grant, Trautrims & Wong (2017, p. 183).
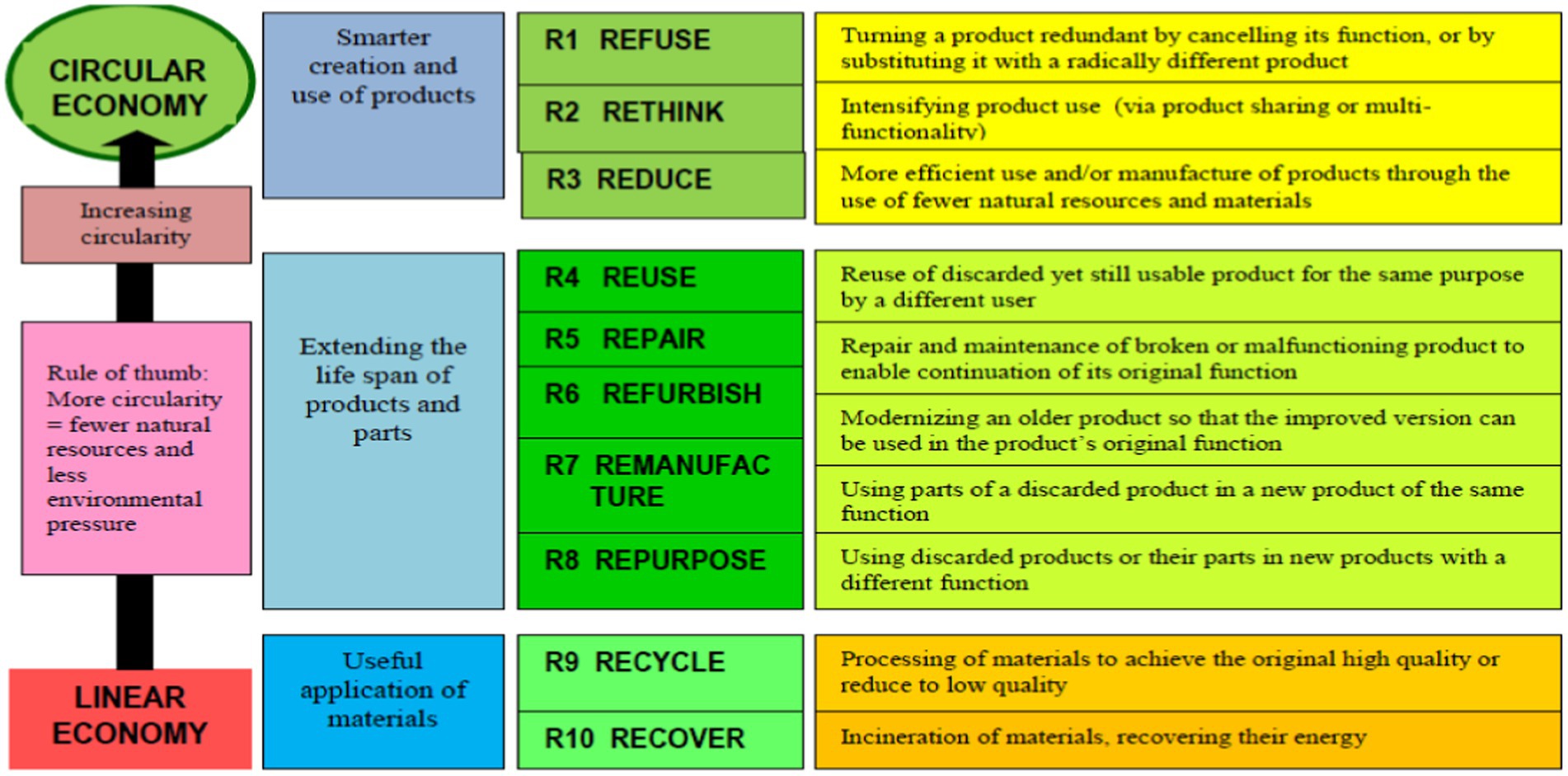
Figure 4. The 10R’s of the circular economy. Source: adapted from Pötting and Hanemaaijer (2018).
De Groene Zaak and Ethica (2015) amended this hierarchy and introduced a circularity ladder concept that probes into the environmental sustainability of different CE business models. A distinction is made between product life extension and sharing models and resource recovery business models. The former potentially reduces the amount of waste generated by slowing resource loops and funneling resource flows. The latter diverts existing waste toward material and energy recovery. The circularity ladder, as depicted in Figure 5, considers the environmental impact of the entire product life cycle, contrary to the end-of-life focus of the waste hierarchy model.
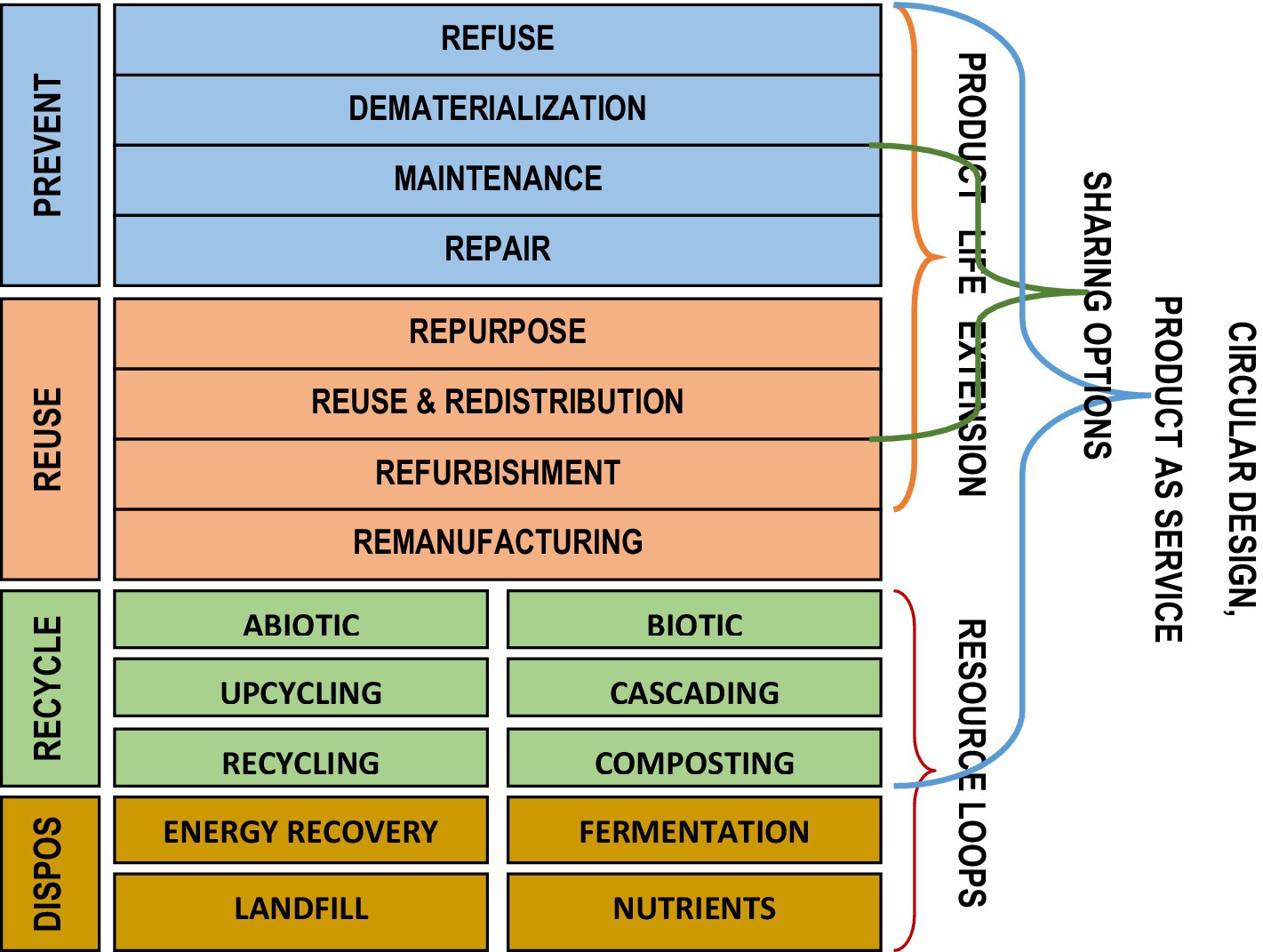
Figure 5. The circularity ladder. Source: adapted from De Groene Zaak (2015).
2.2 The circular design principles
The butterfly diagram based on Ellen MacArthur Foundation (2015) as applied to the construction sector is shown in Figure 6. This is the basis of circularity design principles adapted from the cradle-to-cradle (C2C) design philosophy of Braungart and McDonough (2002). C2C operates on the following three principles where everything: (i) is a resource for something else (i.e., waste of one system becomes food for another), and can be designed to be disassembled; (ii) is safely returned to the soil as biological nutrients; and (iii) is reutilized as materials for new products as technical nutrients. Hence, the two wings of the butterfly represent (a) the biological cycle (i.e., materials that can biodegrade and safely return nutrients to the soil and help regenerate nature) and (b) the technical cycle (i.e., products that are used as inputs rather than consumed).
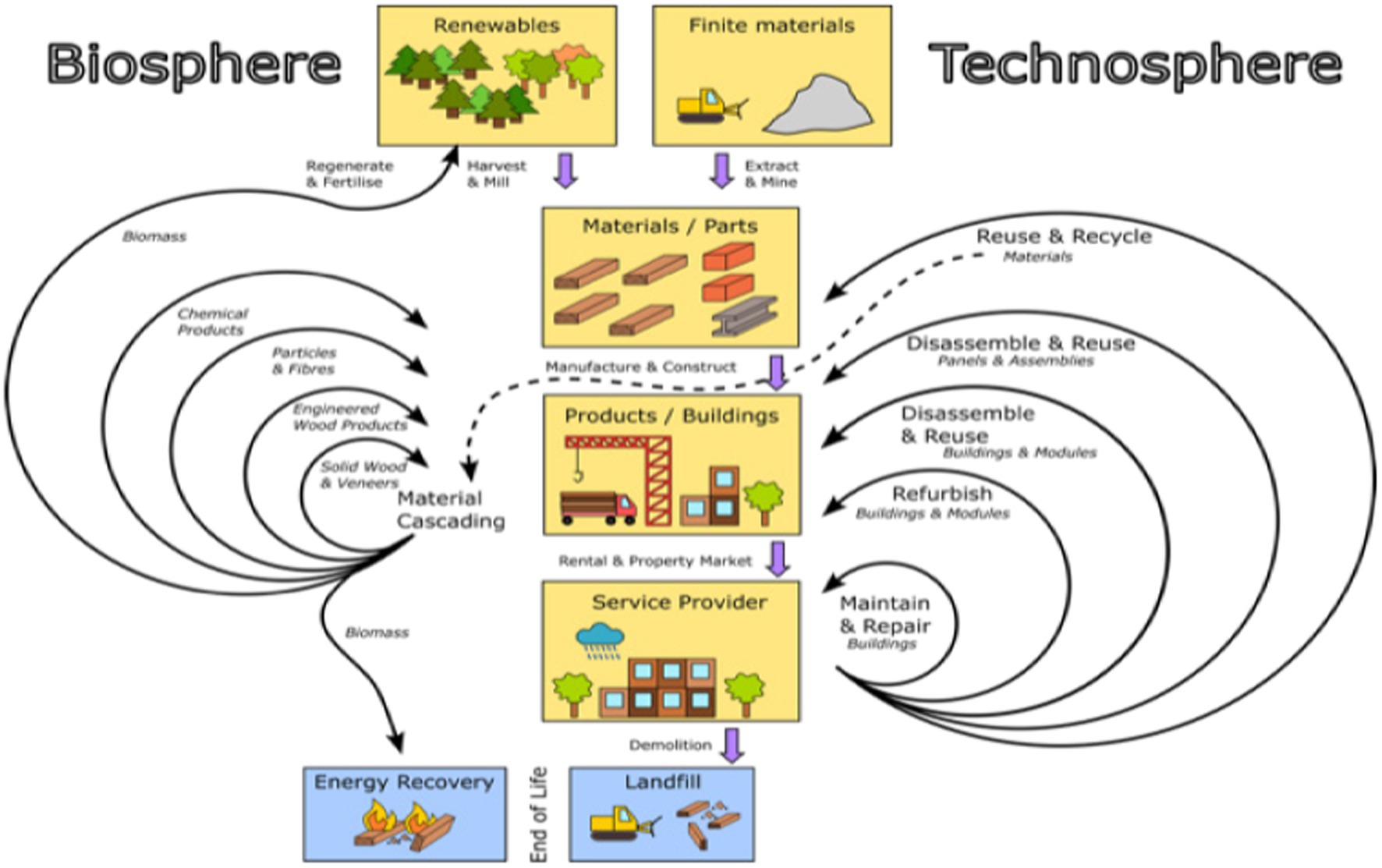
Figure 6. Butterfly diagram of the circular economy. Source: adapted from Ellen MacArthur Foundation and Granta Design (2015).
The biological cycle focuses on regeneration to help rebuild natural capital, such as managing farms, forests, and fisheries, through practices that improve biodiversity, air and water quality, and healthy and stable soils. Nutrients in organic waste streams are collected and returned to the soil by composting (microbial breakdown of organic waste with oxygen) and anaerobic digestion (without oxygen). The small inner loops called cascades refer to the use of organic by-products to make other materials (like textiles from pineapple or hemp fibers) or new products from waste (such as ketchup from overripe bananas or chutney from orange peels) or materials for animal feed (such as crop residues). When the products or materials can no longer be used, these enter the outer loops to be returned to the soil.
In the technical cycle, the inner loops of sharing, maintaining, reusing, refurbishing, and remanufacturing embody where the most value can be captured while retaining the product’s original value. In this cycle, there are cost savings to consumers and producers who use products already in circulation rather than investing in new ones. The outermost recycling loop is the last recourse as the product loses its embedded value and is reduced to its basic materials, which can be reprocessed to make new materials.
The circular design principles evolving from this butterfly framework were conceptualized by the Royal Society of Arts (RSA) in the UK in 2013 from cross-disciplinary consultations, workshops, and roundtables, as shown in Figure 7. Four design configurations were identified, namely design for (i) longevity, (ii) leasing or service, (iii) reuse in manufacture, and (iv) material recovery. Designing for longevity is about prolonging the life span of a product. This extension, through user actions of upgrade, fixing, and repairs, ensures continued workability or functionality of the product instead of being thrown away. Designing for leasing or service refers to a business model allowing people to share or lease products as an alternative to buying and owning. Producers and manufacturers are forced to increase the life and durability of materials since ownership stays with them. Designing for reuse in manufacture is ideally designed for disassembly such that the return of the product into the material stream reduces the producer’s risks. These risks are related to price volatility and even material availability through closed-loop systems where waste from one industry is used as raw material for another. Finally, designing for material recovery, which is the outermost loop, concerns the fastest-flowing products, such as fashion, packaging, or product parts that are fed into a recovery stream once they have completed usage.
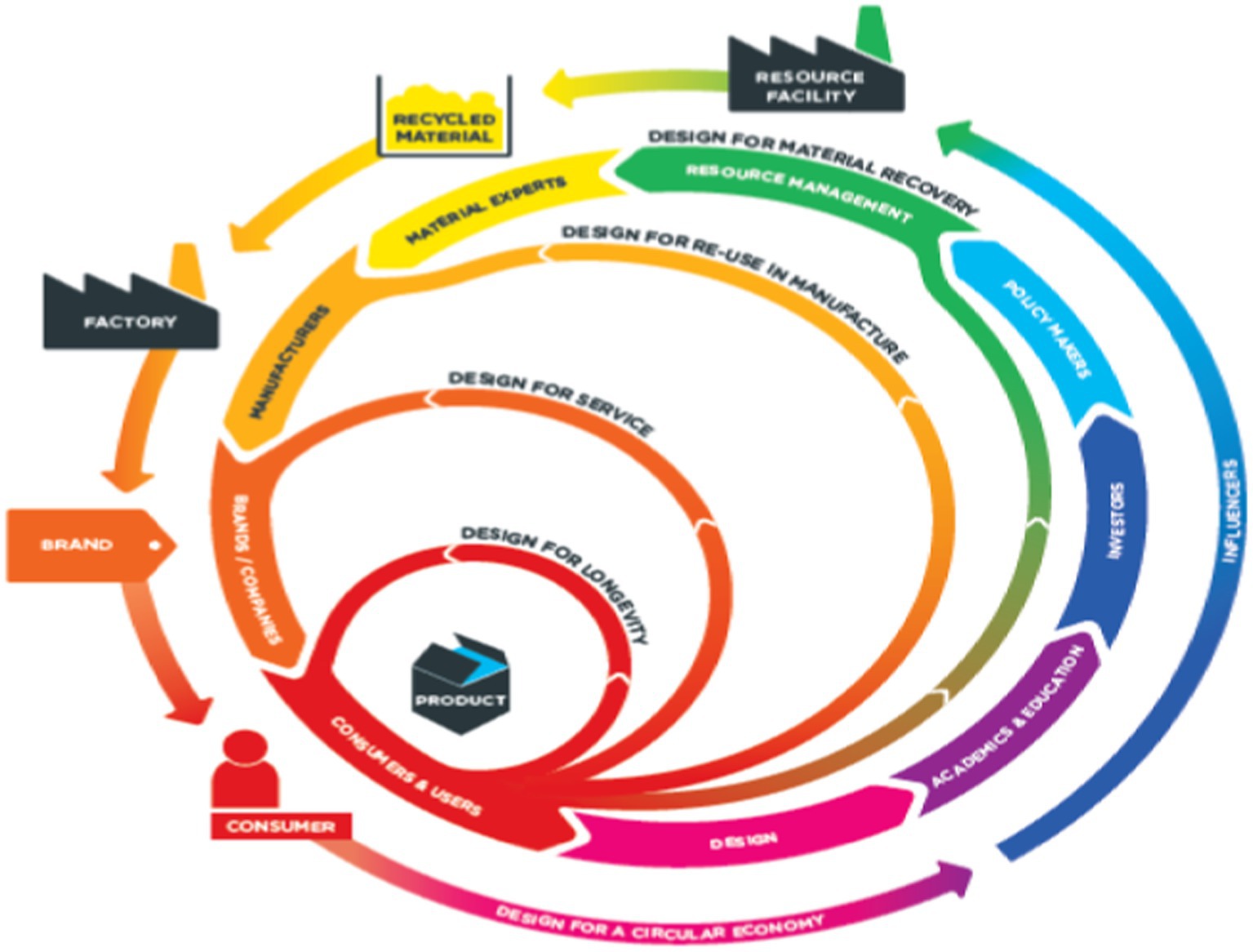
Figure 7. Design principles of the circular economy in the fashion industry. Source: RSA (2013, p. 34).
For the fashion industry, Tanttu et al. (2016) used the same design principles of the RSA, although Piller (2021) urges applying Bason’s four credos of design principles in circularly. This sector intends to detract from the planned obsolescence of cheaply priced fast fashion that encourages a throw-away culture. These four credos are (i) challenging the status quo; (ii) engaging and valuing consumers and employees in the fashion supply chain; (iii) iterating and scaling small innovative successes; and (iv) customizing concrete solutions (Bason, 2010).
Cheshire (2016) conceptualized a similar circular design model for buildings in the construction industry, as shown in Figure 8. The proposed circularity design principles in construction comprise (i) building in layers, (ii) designing out waste, (iii) designing for adaptability, (iv) designing for disassembly or deconstruction, and (v) selecting materials. These will allow a building to be more adaptable as its components can be reconfigured for repair or replacement. The circularity stages, in turn, were identified as retain, refit, refurbish, reclaim/reuse, remanufacture, and recycle/compost, which is most of the R’s of circularity. The inner three circles depict that retaining existing buildings is the most resource-efficient option, followed by refitting and refurbishing. The outermost circles pertain to building materials that could be reclaimed or remanufactured and only recycled or returned to nature as the final destination. The design principles associated with CE probes into the lifespans of the building components and the possibilities of dismantling them for later reuse.
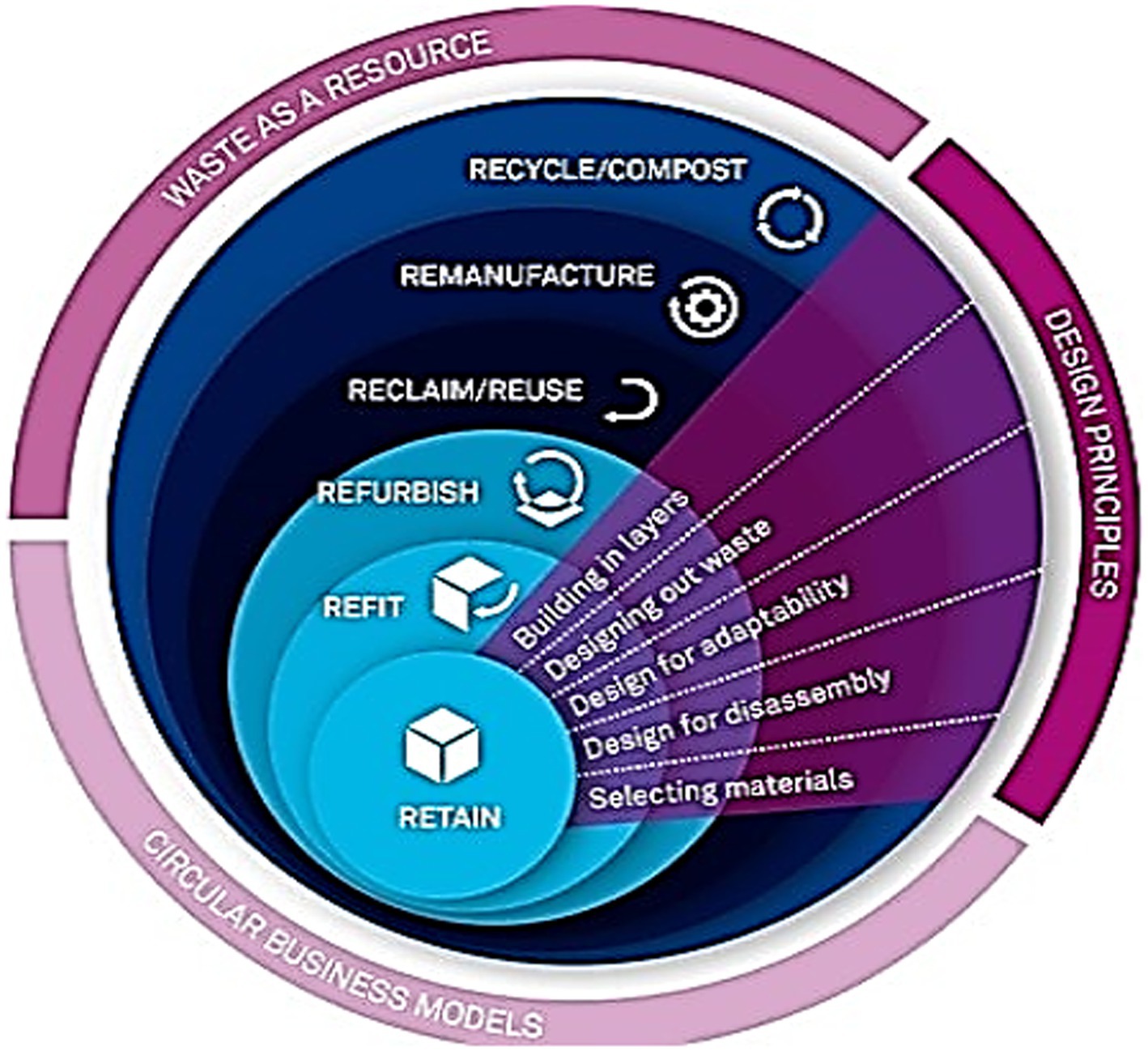
Figure 8. Circular design principles in the construction industry. Source: Cheshire (2016).
As described in Cheshire (2016), Mossberg (2018), and van Vliet (2018), the philosophy behind building in layers is partitioning building elements according to their different lifespans such that their independence allows different layers to be peeled off without damaging the adjoining layers. This is depicted in Figure 9, where a building structure is fragmented into six S’s. These represent the site, structure, skin (façade and roof), services, space plan, and stuff (Brand, 1994). The site and structure, independent of the skin, have the longest life span. Services are accessible, replaceable layers when required, and the space plan and stuff are the short-lived components. This principle thus combines designing for longevity and services in the RSA model.
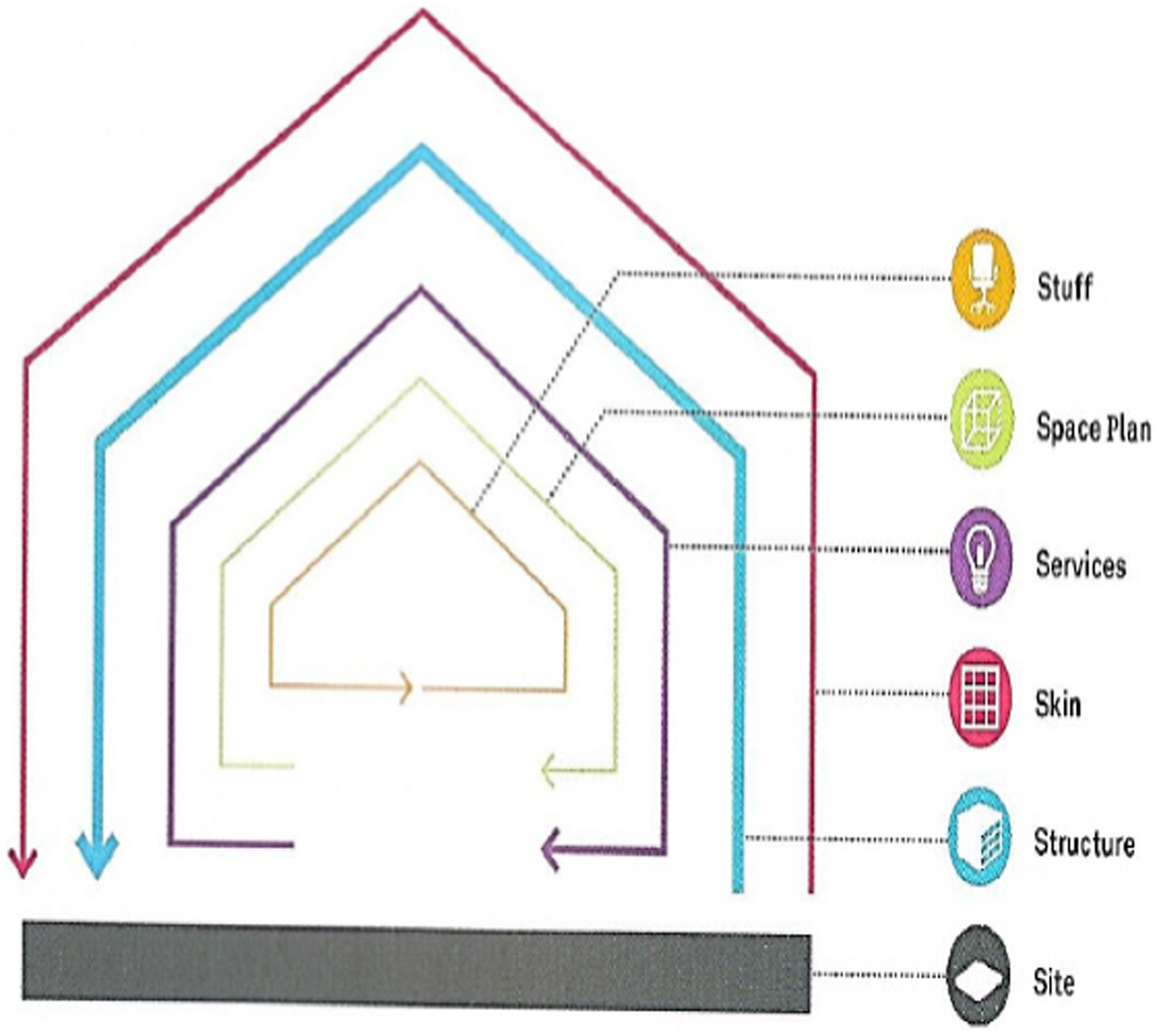
Figure 9. Building in layers. Source: Brand (1994).
Designing out waste employs lean design to explore waste reduction by reusing or recovering building components, producing off-site (i.e., prefabrication), materials optimization, waste efficient procurement, and deconstruction. Relevant factors cover embodied carbon content and water use. Designing for adaptability involves reconfiguring buildings during their lifetimes. This is achieved by adapting their layout to changing markets from residence to retail to office areas using multispace concepts and flexed floor-to-ceiling height requirements. In designing for disassembly, as intended, parts of the building can be moved or transferred to another site by dismounting in case is no longer available as intended. This means that buildings and materials can be valuable assets independent of their site. Their value could be prolonged with extractable materials deposited into a “materials bank.” Finally, selecting materials, called designing for regenerative resources, distinguishes between technical and biological materials. The latter can quickly be returned to nature, whereas the former is manufactured and is more challenging to recycle at the end of life. Based on these descriptions, the fashion design principle of designing for material recovery encompasses construction’s designing for disassembly, designing out waste and selecting materials while designing for adaptability matches with designing for longevity and services in the construction context. This shows the potential to harmonize these principles into basic typologies applicable across sectors, which is a research gap.
2.3 National policies on the circular economy
The national policies of The Netherlands and France on CE were both triggered by EU directives, particularly the Circular Economy Action Plan of 2020 as part of the European Green Deal. It aims to reduce pressure on natural resources and achieve the 2050 climate neutrality target. Both countries responded to the Extended Producer Responsibility (EPR) dictate for fashion and construction as part of the EU Waste Directive. This affected the fashion industry by making brands, producers, and retailers responsible for the end-of-life of sold garments aside from promoting traceability in the value chain. For construction, the use of natural materials such as wood and recycled building components, as well as off-site prefabrication, were pursued in both countries.
2.3.1 The Netherlands
In 2016, the Dutch government set out three goals for transforming to a complete CE by 2050. These goals are (i) to ensure more efficient production processes using fewer raw materials; (ii) to use sustainably-produced renewable (inexhaustible) and widely available raw materials that reduce dependence on fossil fuels; and (iii) to develop new production methods and design novel products that are circular.13 In 2017, a National Agreement on the Circular Economy was drafted by Dutch institutions14 and the National Government. By 2030, the government wants to halve raw materials usage and achieve full circularity by 2050. In 2017, 180 parties from both government and industry signed the Raw Materials Agreement (RMA) to ensure that the economy runs on renewable resources. The process conceptualizes at the design stage how raw materials in a product can be reused as a basis for a recycling economy. Subsequently, in 2018, the signatories to the RMA framed the transition agenda, focusing on five economically vital and environmentally burdensome sectors. These are Plastics, Consumer Goods (under which falls Textiles and Fashion), Manufacturing, Construction, and Biomass and Food. In 2019, the Dutch government presented its Circular Economy Implementation Program (CEIP), which translates the five transition sectoral agenda into projects for implementation between 2019 and 2023. For the textile sector, the EPR, on the heels of EU directives, was introduced to make producers responsible for the textiles they manufacture up to the time they are discarded as waste. In 2020, the Denim Deal was signed by producers, brand owners, and retailers to work together in the value chain to use recycled textiles in all denim products marketed in The Netherlands. For the construction sector, the Central Government Real Estate Agency and the Rijkswaterstaat15 should make their operations circular by 2030. It mandated that all government buildings constructed after 2018 should be energy-neutral and that recycled and recyclable materials and resources should be used in the built environment.
In 2021, the CEIP was updated to introduce three themes, namely, (1) movement higher on the R circularity ladder toward the front end of the chain; (2) systemic change from linear to CE; and (3) impact through focus on raw material flows (Hanemmaijer et al., 2021). The first theme requires circular reuse, sharing, lending, and repair strategies. Applied to construction, housing will primarily be built with renewable and biobased materials and components. These must be built in factories that are assembled on-site to shorten construction time, raise the quality of housing, and reduce emissions, waste, and transport. For textiles and fashion, consumers need to be encouraged to buy second-hand clothes and other consumer apparel. This would counteract the “convenience gap,” which makes it easier to purchase new products rather than used products. In the second theme, new business models, financing, and legislation on products and their use are required for the circular transition, especially removing financial barriers. The Dutch Central Bank developed a Sustainable Finance Platform along with Invest-NL, an open organization of impact investors, to fund circular initiatives, including creating metrics to assess circularity progress. Finally, the third theme enables management to track raw materials consumption at the product level and associated environmental impacts over their entire life cycle and throughout the supply chain. By 2030, the goal is to use 50% fewer primary resources such as metals, minerals, and fossil fuels.
2.3.2 France
In October 2017, the French Ministry for Ecological and Inclusive Transition developed the Circular Economy Roadmap France after 2 months of workshops with stakeholders and citizen contributions. The roadmap is a policy framework to build a national vision toward a total CE. It consists of sub-plans for better production, consumption, waste management, and mobilization of actors. The paramount goals are to (i) reduce consumption of French resources by 30 percent of GDP by 2030 from 2010 levels; (ii) reduce by 50% the quantities of non-hazardous waste sent to landfills in 2025 from 2010 levels; (iii) recycle plastics by 100% by 2025; (iv) avoid emission of 8 million additional tons of CO2 each year due to plastic recycling; and (v) create an additional 300,000 jobs in new professions (Ministry for an Ecological and Solidary Transition and Ministry for the Economy and Finance, 2018). The roadmap contains 50 measures intending to make France a 100% CE at different timelines. It comprises actions relating to (i) research and innovation; (ii) regulatory measures; (iii) resource efficiency and waste management; (iv) circular business models; (v) education and awareness; and (vi) collaboration among stakeholders and international cooperation.
The roadmap covers manufacturing and industry, construction and built environment, food and agriculture, energy and utilities, retail and consumer goods, and transport and mobility. Focusing on fashion, which falls under manufacturing and industry, and retail and consumer goods, the most relevant regulation is the EU EPR directive. Fashion brands and producers will be held accountable for the environmental impacts of their products, be responsible for the collection, recycling, or proper disposal of their products at the end of life, and fund collection and recycling initiatives. Implementing EPR in the fashion industry can help shift the responsibility for managing textile waste from consumers to producers. It encourages better product design, improves waste management systems, and increases stakeholder collaboration to address the environmental challenges associated with fashion production and consumption.
In designing for circular fashion, design principles should be aimed at durability, reparability, and recyclability (French Ministry of Ecological Transition and Solidarity, 2020). According to The Essentials for the CLF Industry (2020),16 France adopted in February 2020 the Anti-Waste and Circular Economy Law, part of which came into effect on January 2023. It forbids destroying unsold goods, most of which are fashion brands. Contemporaneously, brands and retailers selling in France are obligated to disclose their products’ environmental impact. For apparel and footwear, (i) labels should reveal the percentage of recycled material by weight; (ii) details about the future recyclability of the product; (iii) the presence of harmful or toxic substances; (iv) indication of microplastic shedding for garments containing more than 50% synthetic fibers; and (v) traceability whereabouts like country of origin for various stages of the manufacturing process apart from the finished garment. Time of compliance with the new regulation will vary by company size, with companies having a turnover of above €50 million and producing over 25,000 units immediately compliant. Those with turnover above €20 million and introducing more than 10,000 units have until January 2024 to comply. Those selling over €10 million with 10,000 units are liable until January 2025. With an EPR in place since 2009, prior to the EU, France targets the reuse of clothing from 40 to 60% by 2028 (Paris Good Fashion, 2023).
For construction and the built environment, the roadmap (i) endorses the adoption of sustainable construction practices and materials; (ii) promotes the reuse and recycling of construction waste; (iii) encourages the deconstruction and salvaging of materials from buildings; (iv) implements circular design principles in building projects; and (v) development of circular building components and systems. In recent years, there has been growing recognition of the environmental benefits of using wood as a construction material due to its renewable and low-carbon properties. One of the new French construction laws is that all public buildings should use 50% wood and timber as materials by 2030. This was inspired by the construction of the 2024 Paris Olympics complex, where organizers made a commitment that any building that rises more than eight storeys high will be built entirely from timber (Tansley, 2020; Crook, 2020; Global Construction Review, 2020).
2.4 Sustainability indicators
Sustainability indicators will be used as criteria to measure balanced sustainability in the case of companies. The Material Circularity Indicator project of the EMF developed a framework for circularity indicators based on material flows of products and components (Ellen MacArthur Foundation, 2015) alongside complementary indicators that measure risk and impact from transitioning to CE. Moraga et al. (2019) suggested a framework of circularity indicators that combined micro- and macrolevels and that are grouped based on CE strategies according to function, product, components, materials, and embodied energy. Framing indicators based on inputs and outputs turns out to be problematic as component parts of products are multiple, and some companies do not just produce a single but several products with multifunctionalities. Moreover, the impact indicators use different non-comparable scales such as kilowatt hours in energy reduction, liters of water consumed, or kilograms of waste produced. As these material components and physical impact metrics are often unavailable or not tracked by most companies, scoring cases based on a complete checklist of qualitative descriptions of sustainability indicators for the three sustainability dimensions is the more convenient approach. This approach was used in Dytianquin et al. (2021) and Dytianquin et al. (2023), which showed valid results.
2.4.1 Environmental impact
Singh et al. (2016) describe the standard tools used to assess the environmental impact of projects are environmental impact assessment (EIA), ecological footprint (EF), and life cycle assessment (LCA). Performed usually during the planning stage of a project, an predicts the projects, namely: the impact on the environment and outlines mitigation measures to eradicate adverse effects of the project on the environment, such as carbon or greenhouse gas (GHG) emissions, water use, energy demand, solid waste, exposure to hazardous and toxic materials, and other forms of pollution. In Yijun et al. (2011), CE theory is applied to EIA. On the other hand, EF, based on the Global Footprint Network (n.d.)17 was introduced in the 1990s to measure how much natural resources are consumed and how much waste is generated, hence accounting for the demand for and supply of natural resources. On the demand side, EF aggregates all productive areas for which consumers, society, or a given product competes, hence measuring the ecological assets required by users to produce the natural resources they consume and to absorb its waste, specifically carbon emissions. On the supply side, EF measures the biocapacity or productivity of ecological assets to absorb an economy’s waste. The third tool, LCA, is an international standardized methodology for accounting for the environmental footprint of a product or service within the requirements of ISO18 14040 and 14044, outlined in the OECD (2019). LCA involves data collection on inputs (resources) and outputs (emissions and waste) that constitute inventory analysis. These get translated into indicators of environmental, health, and resource availability impacts, leading to some quantification of the environmental load of products or services throughout their lifetime. Haupt and Hellweg (2019) developed a framework for measuring environmental sustainability in a CE that measures the environmental value retained by circular products through the different circularity stages. The list of environmental criteria or indicators for the selected projects and their respective definitions are shown in Annex Table 2.
2.4.2 Economic impact
Rizos et al. (2017) describe the economic effects of CE as generating employment in the recycling business. Caution, though, should be placed on net employment as there are also jobs that get displaced by CE, especially in resource-extractive sectors. Other economic effects cited in Hysa et al. (2020) are (i) trade effects in terms of exports of new recycled materials coupled with reduction of imports of primary materials; (ii) value chain multiplier effects in terms of input purchases and delivery channels as well as cross-value chain cooperation; (iii) changes in consumption usage and demand patterns; (iv) higher value-added and greater residual values at end of life; (v) increase in investments in innovations and new technologies related to recycling and remanufacturing; and (vi) savings on maintenance costs. While most circular projects are subsidized, the potential for self-financing is important for replicating similar CE projects; otherwise, these become reliant on the availability of subsidies. The economic indicators are found in Annex Table 3.
2.4.3 Social impact
Padilla-Rivera et al. (2020) surveyed how social aspects have been considered and incorporated in CE, and they suggested thematic areas in terms of labor practices and decency of work, human rights, society, and product responsibility. The social impact of CE concerns mainly stakeholder participation, as shown by Persson and Olander (2004) and Bal et al. (2013). Social impact assessments suggest other indicators relating to income equality, cultural diversity, involuntary settlement, gender and race disparities, social cohesion and inclusion, and preservation of cultural heritage, as outlined in Inter-American Development Bank (2018). The social life cycle assessment (S-LCA) by United Nations Environment Program (2009) also covers socioeconomic aspects along the life cycle of products and services that directly affect stakeholders positively or negatively. Many of these indicators, however, overlap with economic and environmental indicators such as local employment, supplier relationships, health, and consumer and worker safety. In this article, some overlapping indicators, such as employment, were used in the economic impact instead of social. The social indicators are listed in Annex Table 4.
2.5 The UN sustainable development goals, corporate social responsibility, and the triple bottom line
In September 2000, member states of the United Nations unanimously adopted the eight Millennium Development Goals (MDGs). These range from halving extreme poverty to combatting the spread of HIV/AIDS, providing universal primary education, improving maternal health, reducing child mortality, promoting gender equality by empowering women, and ensuring environmental sustainability with a specific deadline of 2015. While substantial progress was made in attaining these goals, many nations failed to meet the MDGs, such that calls for particular indicators and targets and how the goals are to be met and the financial interventions needed to achieve them have been raised (Sattertwaithe, 2012).
In 2015, the member states of the United Nations adopted the 2030 Agenda for Sustainable Development, providing a blueprint for shared peace and prosperity for the whole world. The 17 Sustainable Development Goals (SDGs) are at its core with 169 targets. This global agenda calls for partnerships to end poverty, reduce inequality, promote economic growth, mitigate climate change, and foster ecological integrity. The ethical foundation of the 17 SDGs is provided for by the Earth Charter (EC). Following the Earth Summit in Rio de Janeiro, Brazil, EC was a global initiative based on a worldwide multicultural and multisectoral consultation process and research on the values and principles of sustainability that took place between 1994 and 2000 (Rockefeller, 2015; Clugsston, 2011; Burford et al., 2013). The EC document consists of 4 pillars (i.e., respect and care for the community of life; ecological integrity; social and economic justice; and democracy, non-violence, and peace) with 16 principles.19 The SDGs can be traced to one of these principles, as shown in Figure 10.
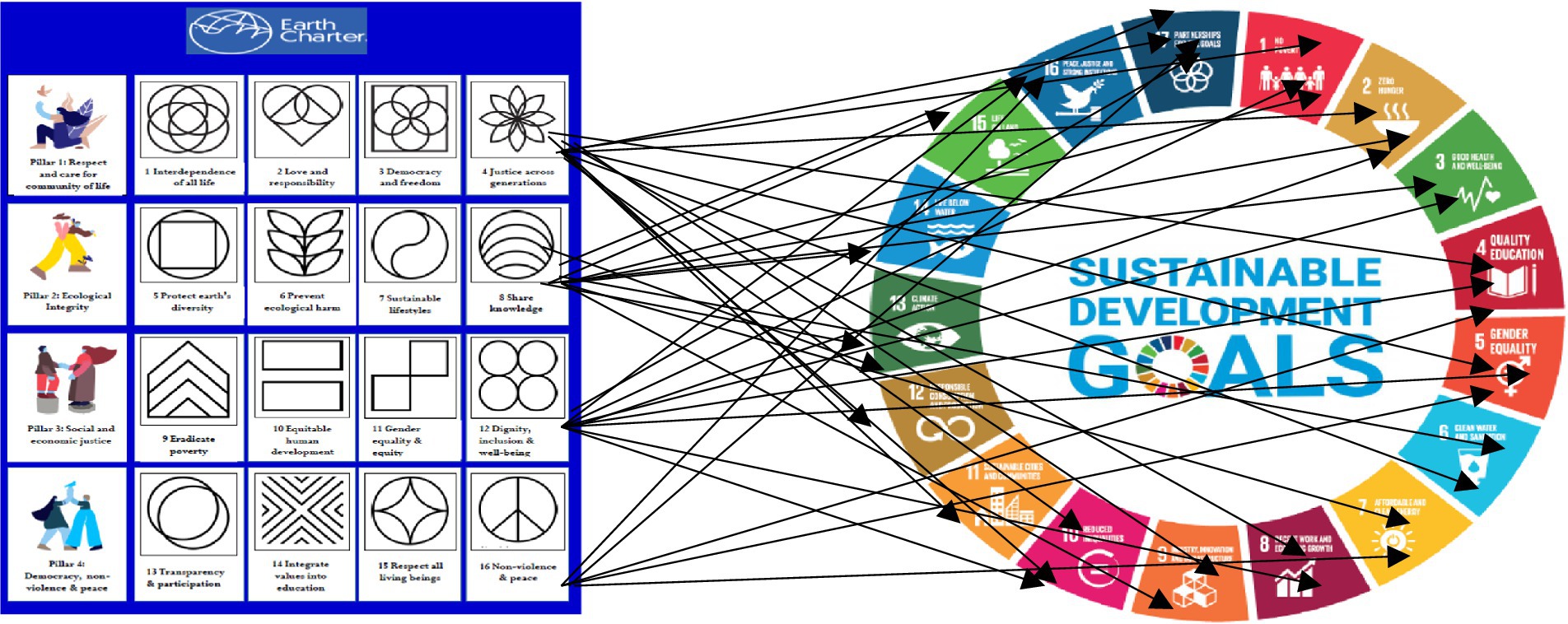
Figure 10. The earth charter and the UN SDGs. Source: author’s own, adapted from the Earth Charter and UN SDGs.
The emergence of the concept of sustainability compelled businesses to rethink their approach to measuring performance by considering social and environmental attributes. Stakeholders increasingly hold firms accountable for their social and environmental responsibilities (McLaren, 2004). Companies operate within an ecosystem of multiple stakeholders (Desai, 2018). Stakeholder demands drove companies to incorporate sustainability into their operations (Herremans et al., 2016). This obligation and accountability to society by businesses became known as corporate social responsibility (CSR). However, Marrewijk (2013) describes the confusion of the definitions of CSR with corporate sustainability. CSR covers company activities, which are voluntary and include social and environmental concerns in business operations and interactions with stakeholders. Corporate sustainability, in turn, is the goal of value creation, environmental management, sustainable production systems, and human capital management. He proposed a distinction between CSR and corporate sustainability based on the Finnish model (Figure 11). There has been a difference as well with sustainability relating to only the environment. At the same time, CSR is concerned with social aspects, although there has been a convergence later with the two terms becoming synonymous. Moreover, the word social in social responsibility tended to be misleading as it applied to social welfare issues rather than a more embracing concept of societal responsibility, which pertains to the company’s impact, relations with, and responsibilities to society (Marrewijk, 2013).
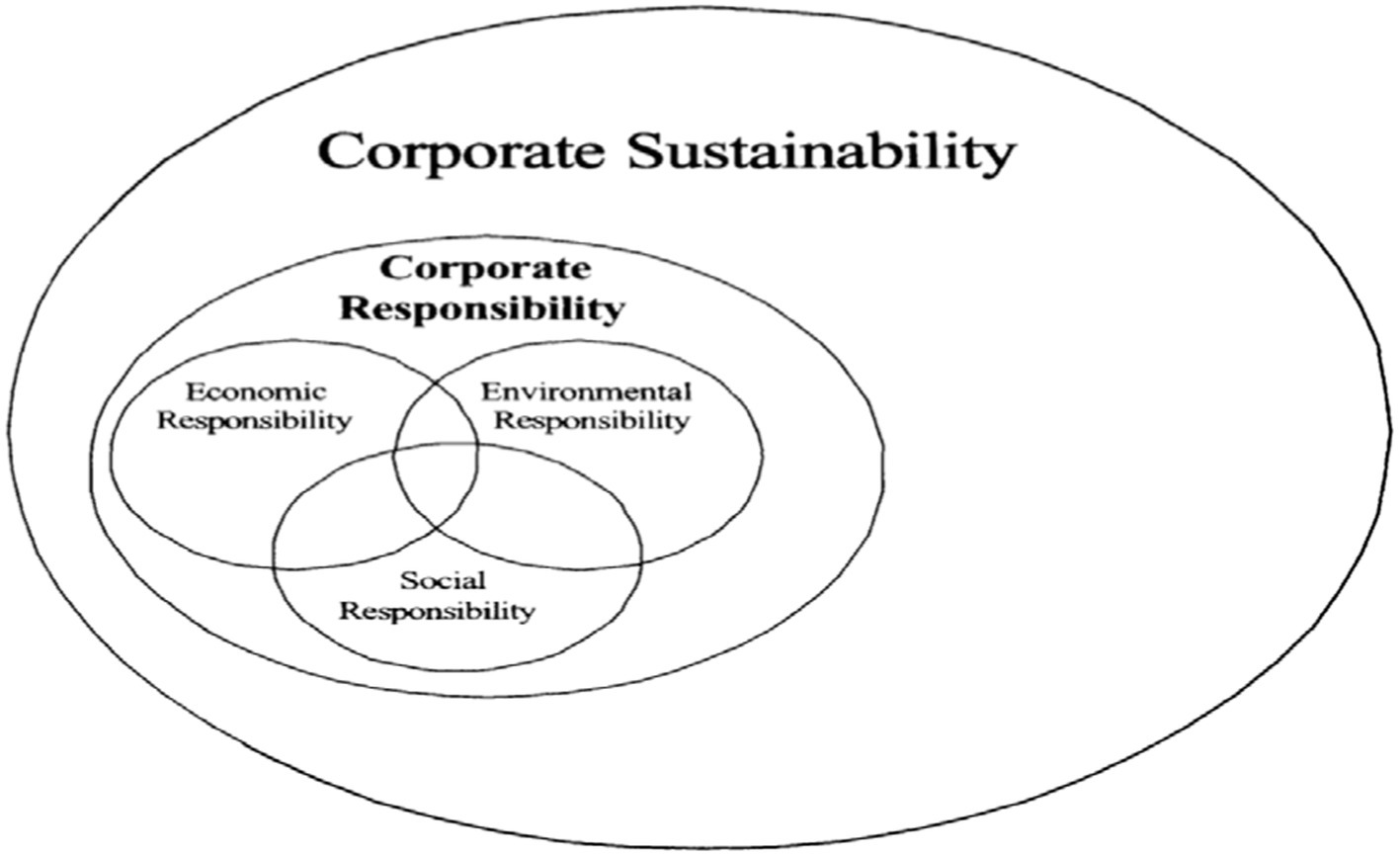
Figure 11. Finnish model of CSR and corporate social responsibility. Source: Linnanen and Panapanaan (2002) as cited in Marrewijk (2013).
One of the popular models of CSR that became its prototype is the triple bottom line (TBL). Elkington (1994) introduced the concept of TBL, where sustainability comprises three interconnected dimensions: economic prosperity, social equity, and environmental integrity. It is also known as the three P’s—for people, planet, and profit. Mulia et al. (2017) maintain that an appropriate balance exists between economic, environmental, and social outcomes. This balance is represented by the shaded intersection of overlapping circles or Venn diagrams of the three dimensions depicted in Figure 12. The TBL also encompasses the materiality indicators that govern integrated or sustainability reporting of companies in compliance with another EU directive, called the Corporate Sustainability Reporting Directive20 (CSRD). Companies must incorporate all three dimensions into their operations equally (Venkatraman and Nayak, 2015). Many companies strive to improve their performance regarding the sustainability triptych and use SDGs as a metric for CSR and corporate sustainability, considering its well-defined and time-bound targets. The integration of TBL and the SDGs can motivate companies to contribute to sustainable development not only in the country where they are based but also in the country where they operate internationally. Thus, it is important to classify SDGs according to the TBL dimensions, as shown in Figure 13, adapted from Singh and Rahman (2021). In their framework, there are definite overlaps with two sustainability dimensions covered by one SDG. The social dimension is exclusively covered in SDGs 1 (poverty), 2 (hunger), 3 (health), 4 (education), and 16 (peace, justice, and strong institutions). Three SDGs concern mostly the environment, i.e., 13 (climate action), 14 (life below water) and 15 (life on land). SDGs 9 (industry, innovation, and infrastructure) and 8 (decent work and economic growth) are purely economic. Four SDGs, namely, 5 (gender equality), 8 (decent work and economic growth), 10 (reduced inequalities), and 12 (responsible production and consumption) share social and economic dimensions. Two SDGs are environmentally and socially focused, i.e., SDGs 6 (clean water and sanitation) and 11 (sustainable cities and communities). Economic and environmental dimensions intersect in SDG 7 (affordable and clean energy). In SDG 17, all three dimensions are invoked. As the circular economy is intertwined with corporate sustainability and the TBL, the case projects covered in this study will also rate the projects in terms of their SDG affiliation.
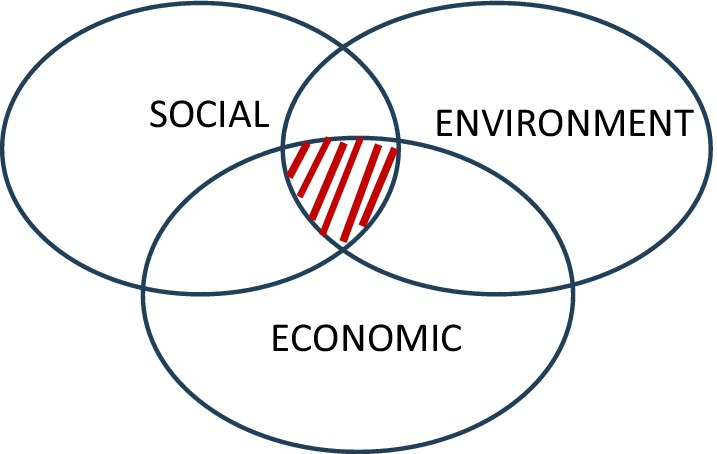
Figure 12. The triple bottom line and sustainability balance. Source: adapted from Elkington (1994).
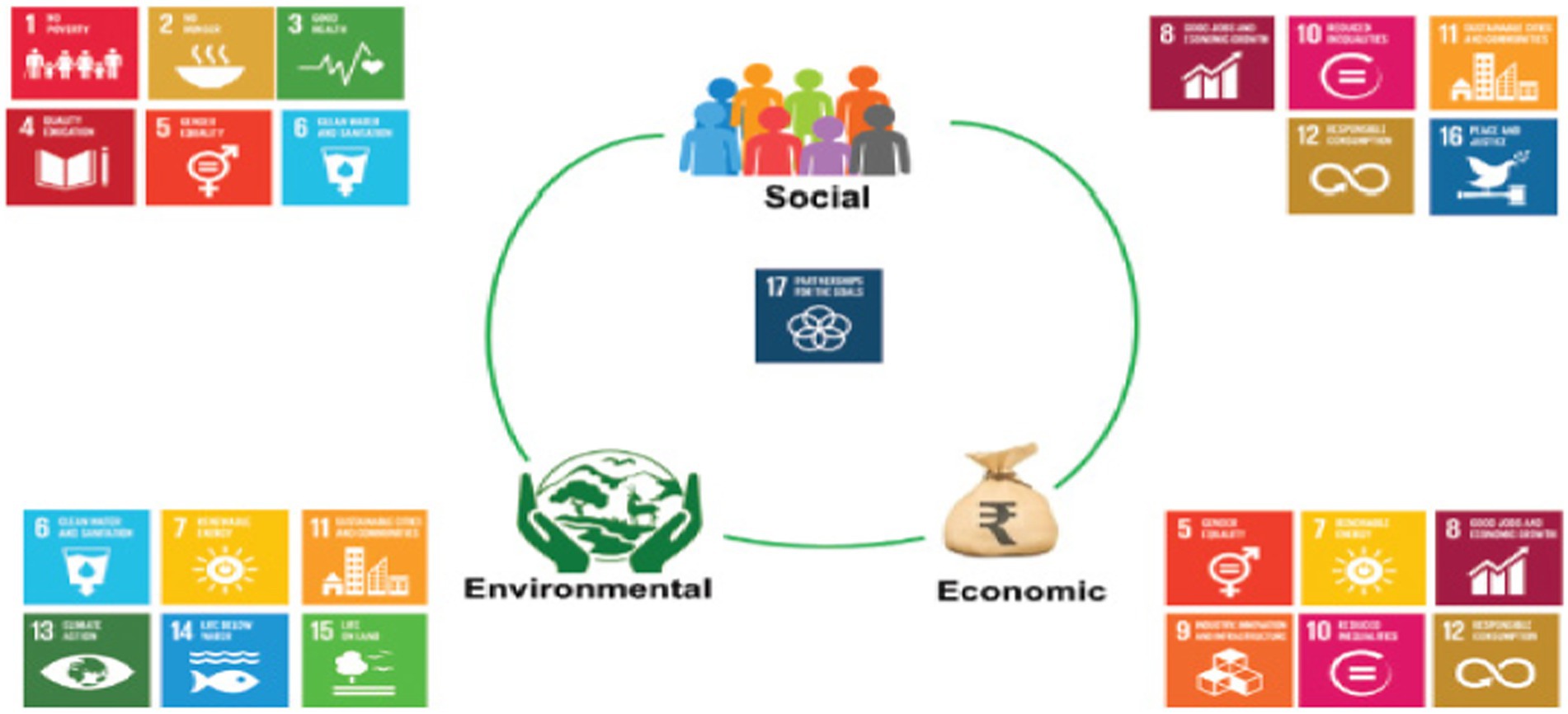
Figure 13. TBL-SDG framework. Source: adapted from Fonseca and Carvalho (2019) as cited in Singh and Rahman (2021).
3 Research gaps
The literature review points to several research gaps that this study will address. As described earlier, the study’s main premise is that the applied circularity design principle will determine the balance of the sustainability dimensions of environment, economic and social. Firstly, most of the literature21 on circular design principles surveyed in Section 2.2 merely describes principles that are specific to a sector (e.g., fashion or construction) or a particular R in the 10 R framework (e.g., mostly on recycling).22 Several archetypes23 of circular product design are outlined. Second, the question of which circular design principles lead to more balanced sustainability is not even discussed in the literature. Third, the use of circularity and sustainability indicators are not convenient as these: (i) mostly focus on the environment and economic issues and neglect the social aspect; (ii) require product breakdowns into their component material parts, and (iii) involve impact measurements that have different physical scales which are not often available and monitored by companies. Finally, the dominance of climate and energy SDGs among circular companies will be verified. This study will address these gaps by comparing two sectors (fashion and construction) where circularity is applied in two countries, France and The Netherlands. First, it unifies the types of circular design principles into homogenized categories that could apply to at least fashion and construction and, possibly, other sectors. Second, it identifies which circular design principle contributes more to balanced sustainability. The comparison also applies to two countries to determine if the link between circular design principles and sustainability balance is place or location specific, apart from being sector specific. Third, the TBL balance is measured by scoring a comprehensive qualitative blanket list of environmental, economic, and social indicators without material flows or physical units of impact metrics. Finally, evidence of which SDGs are commonly addressed in CE case companies will be probed.
4 Research methodology
The study applies multicriteria decision analysis (MCDA) on multiple case studies of enterprises involved in each design principle applied in the fashion and construction sectors in France and The Netherlands. The main objective of MCDA is to furnish decision-makers with tools that enable the selection of solutions to a multicriteria decision problem involving diverse criteria. The simplest and most popular MCDA method is the weighted sum (WSM) or simple additive weighting method, as Ginevicius and Podvezko (2005) call it. Triantaphyllou and Mann (1989) expresses the WSM as:
Where is the WSM score of the best alternative, N is the number of criteria, is the actual value of the ith alternative in terms of the jth criterion, and is the weight of importance of the jth criterion. Under the basic assumption of additive utility, the total value of each alternative equates to the sum of products given in the expression. This restriction in WSM is later modified in the weighted product method (WPM) where the main difference is multiplication instead of addition, making the calculation dimensionless by eliminating units of measure. Triantaphyllou and Mann (1989) further express the formula for WPM as:
Where N, , and are the same as in WSM and and are alternatives or options being considered by the decision maker. The values of will either be less than, equal to, or exceeding unity, where the latter case indicates that the alternative is superior to alternative . If all alternatives are lined up on this scaling, the best or superior alternative is the one that has the highest value for . Hence, instead of actual values, relative ones are used as in:
Where are actual values and are relative values.
In this study, the WSM approach is used inasmuch as the scoring system employs the same measurement units or cardinal values. In Triantaphyllou and Mann (1989), it was found that when converted into percentages, the WPM was inapplicable due to the occurrence of zeros and hence the problem of division by zeros. Hence, the WSM yielded the most reliable answer for choosing the most effective MCDA approach.
The MCDA will use the sustainability indicators for environmental, economic, and social dimensions presented in the literature review as criteria. This is applied to case projects chosen specifically for the circular design principle used in the case project. Rather than collect information, the case study approach investigates the embeddedness of a phenomenon, which, in this situation, is the circular design principles in their real-life context (Blumberg et al., 2014). The perspective taken from the case studies is positivist, following Eisenhardt (1989) and Yin (2009), which either starts from a phenomenon to develop a theory or confirm, extend, or challenge theories rather than adopt the reflective or interpretivistic research philosophy of Stake (1995) and Burawoy (1991).
Unlike other research methods, case studies allow the researcher to illuminate a phenomenon from multiple perspectives by detecting patterns and potential explanations that are unexpected to enable building or testing theories. Although not generalizable to a population, these can be generalizable to a theoretical disposition (Blumberg et al., 2014). The selection of cases is also not based on sampling logic but on replication logic, which implies that one will expect the same phenomenon to recur if similar conditions prevail or that the phenomenon changes when circumstances do not hold. Thus, the analysis combines pattern matching and quantitative methods, which, in this study, involve the rating and scoring of the projects using MCDA. The objective is to detect consistencies in the project ratings across the circularity design principles applied to fashion and construction in the two countries.
For purposes of this study, the circular design principles for the fashion and construction sectors, as introduced in the Literature Review, were harmonized into five design principles, as shown in Figure 14. These five circular design principles are (1) design for biobased materials (DfBMs); (2) design for service/adaptability (DfSA); (3) design for disassembly, reuse, or remanufacture (DfDRR); (4) design waste for material recovery (DfWMR); and (5) design for longevity (DfL). By harmonizing the design principles, patterns between the two sectors and countries can be compared.
4.1 Selection of cases
According to Seawright and Gerring (2008), case studies should serve the purpose of representing features of a population of cases that is broader or larger than the case itself. A truly representative case is easy to identify, especially for small-N populations, where randomization of sample cases could prove insufficient, and hence, purposive selection may be preferred. Purposive selection cannot entirely overcome non-representativeness, although the inherent unreliability of generalizing from small samples can be useful for inferential purposes. The authors suggested seven types of case selection techniques, namely, typical, diverse, extreme, deviant, most similar, and most different, depending on the hypothesized relationship between the dependent and independent variables and the researcher’s goals. In this study, the independent variable is the circularity design principle, while the dependent variable is the balance of sustainability dimensions. The goal is exploratory as there is no given hypothesis of which specific circular design principle achieves the most balance, which will only be revealed by the TBL scores.
The cases were selected deliberately based on project descriptions that match or contain the pertinent circular design principles. In this case, there is only a small N-population of companies applying a specific design principle. Data obtained were mainly secondary, based on company websites, sustainability journals, architectural digests, fashion and construction journals and reviews, and CSR and non-financial performance reports, where these are available. On web browser search functions, the circularity principle was named the country, sector, and company, for example, “design for disassembly fashion French company” or “Dutch Construction Company designing for longevity.” Some of the cases were named and were selected from published articles.24 In the event companies were identified from these sources, their websites were searched to confirm the circular design principle that the company is using. If searches do not return a website, then a deliberate search is made online, typing the keywords described above on the search browser. The websites of each case company are also indicated in the case company descriptions found in Annex Tables 5, 6 for fashion and construction in The Netherlands, respectively, and Annex Tables 7, 8 correspondingly for the two sectors in France. Similarly, the websites are listed in the “References” list. As explained below on triangulation of results for validity and reliability, data triangulation demanded two cases for each design principle; hence, two samples of cases for 5 design principles for 2 sectors for 2 countries, for a total of 40 cases selected for this study.
4.2 Ranking importance of sustainability indicators
To compare the selected case projects on the three dimensions of sustainability, a mixed approach of subjective and objective methods of MCDA was used. The mean weight method is the simplest way of weighting as it distributes the weights equally without any preference shown by the decision maker and is considered an objective method. Roszkowska (2013) states that when knowledge about the weights does not exist, then the weights are represented by a uniform probability density function where the expected value is centroid (center of mass) of the line with coordinates (½. ½) such that . The following weight formula is thus used called the mean weight:
where j = 1, 2…, n.
Again, as the number of criteria increases to 10 or more, the weights become diminutive. Equal weights are justified in this study since ranking the criteria by importance will vary depending on the circular design principle and will distort the comparability of the results. For instance, in the environmental impact, case projects using biotic materials would rank this criterion higher compared to designing for material recovery, where product life cycle extension would be heftier. Hence, each case project would apply different subjective weights on the criteria depending on the design principle, which are challenging to establish having no precedent. The subjective weighting of the criteria could also lead to biased results and difficulty in comparing the results across sectors and projects. Hence, for simplicity and objectivity, equal weights were applied to the sustainability criteria regardless of the circular design principle.
4.3 Scoring of cases on the sustainability indicators
The scoring, however, of the case projects followed the subjective method of direct rating or ranking for convenience and expediency, although a decision rule was applied in the scoring system. All indicators of each sustainability dimension are scored from 0 to 3, with 3 indicating the highest using a Likert scale. The higher the score, the more the project addresses the sustainability/circularity yardstick or indicator of the sustainability dimension based on company pronouncements. The scale allows some range of scoring that is not too close than if only 3 or 5 scores were used and will prevent the error of central tendency of choosing the middle score. While subjective, the scoring system used a decision rule, as shown in Table 1.
4.4 Determining the sustainability triple bottom line (TBL) ranking
The scores are then multiplied by the weights of the indicators for each dimension and then summed up to get the total score for the project for that sustainability dimension. The case company having the highest average weighted score for each dimension is ranked as first and chronologically down to the fifth rank for the case company that got the lowest average weighted score. In case of a tie, then the companies received the same rank. The next step is to calculate the average weighted total scores for economic, social, and environmental dimensions for a case project, applying equal weights of one-third for each dimension to represent the balance of the three dimensions. This means the sum of the weighted average scores of environmental, economic, and social impacts was divided by 3 (or what amounts to the same thing, multiplied by 1/3) to determine the total TBL score. The case companies and design principles are then ranked based on the TBL score from lowest to highest, with the lowest weighted average for TBL score ranked with a 1, indicating more balance in the three sustainability dimensions, and the highest with weaker balance ranked with a 5. After inspecting the ranks, the final rank goes to the case company that has the lowest average rank. Hence, a company that ranked 1 in all dimensions receives an overall final rank of 1, and so on. The lower the calculated average for rank, the more balanced the case project design is in attaining the three goals of sustainability and circularity, hence representing best practice.
4.5 Triangulating results for validity and reliability
Triangulation is a method used in research to strengthen the reliability, stability, and validity of research findings and prevent confirmation bias, which occurs when a researcher generates evidence for a preconceived opinion. Reliability is concerned with the accuracy and replicability of the research results. Stability is about the consistency of results with repeated measurements. The validity, in turn, is about the generalizability of the findings and transferability to other contexts, times, and samples, which in this case are other enterprises. Four general types of triangulation have been identified by Denzin (1978), namely: (i) data triangulation—using a variety of data sources over time, space, and population in a study; (ii) theory triangulation—using multiple theories or hypotheses in studying a phenomenon to ensure different perspectives; (iii) investigator triangulation—using more than one investigator or researcher or data analyst in the study; and (iv) methods triangulation—which is the use of multiple methods for studying a phenomenon. In this study, data, investigator, and methods of triangulation were used. For data triangulation, two samples of case projects for each circular design principle in the two sectors in both countries were used, hence doubling the size of the sample cases. The TBL scores of the two samples were then compared to confirm if an observed pattern emerged. For investigator triangulation, the authors or researchers also scored the projects separately and ended up with similar results when compared, with minor differences that did not affect the rankings. Hence, equivalence, where measures of different items for one construct taken by different people are similar, was also achieved.
In this study, methods triangulation was also employed by using other objective methods for weighting criteria for MCDA. While methods triangulation also involves collecting other means of data to support case studies such as interviews, surveys, or focus groups, time and resource constraints precluded the use of this triangulation technique, given the number of case projects used in the study. Moreover, companies would refer the researchers to their websites and impact reports as they have no time to do interviews or surveys. The alternative objective weighting method used for method triangulation is the CRiTIC method, which stands for CRiteria Importance Through Inter-criteria Correlation, which involves correlation analysis to reveal contrasts between criteria. A matrix of ’s is first created containing ideally the raw data of all n alternatives, although Zardari et al. (2015) use scores of the alternatives to comprise the matrix. The standard deviation of each vector of ’s is calculated to represent the contrast intensity of the corresponding criterion. A symmetric matrix of dimension is then created with rows and columns corresponding to each criterion. The correlation coefficients of each element of the matrix are denoted as is then calculated where the values denote the discordancy of the scores of a pair of criteria (i.e., the lower, the more discordant). Then, another matrix containing the ’s subtracted from unity is generated and then summed up to measure the conflict created by criterion j with another criterion k with respect to the decision situation defined by the rest of the criteria. This measure of conflict is denoted as:
The amount of information denoted by is then measured by multiplying each standard deviation calculated earlier ( ) by the conflict matrix, hence:
A higher value of signifies that more information is transmitted by the respective criterion, which is relatively important for decision-making. The objective weights for each criterion are finally derived by normalizing the resulting vector of ’s to unity, that is, . Hence, in this method, the weights are not equal but will vary depending on the co-variance between pairs of criteria.
5 Results and findings
5.1 Findings and analysis of TBL scores
Tables 2, 3 summarize the results of the MCDA conducted, showing the final weighted average TBL ranks of cases in both sectors and countries. A breakdown of the calculations for each sustainability dimension is provided in Annex Tables 9–12 for both sectors in the two countries. Data triangulation was performed by looking at two samples of case companies, hence doubling the sample size from 20 for each sector in each country or a total of 40 cases all in all.
After tabulation of the weighted average scores for each sustainability dimension and averaging the rankings, what stands out is that the rankings for the cases for each design principle in the two sectors for both countries are consistent and almost identical. The rankings for design for biobased materials, design for service/adaptability, and design for disassembly consistently bagged the top 3 ranks in terms of TBL scores, respectively. In contrast, both design for waste and material recovery and design for longevity scored lower, with rankings interchanging between the fourth and fifth places.
Designing for biobased materials ranked first in the overall TBL scores in both sectors and countries. This can be attributed to the higher economic impact due to the value chain effects further upstream to farmers and suppliers of these eco-based materials. Aside from job creation in the source sectors of biobased materials, there are associated multiplier effects further downstream that create employment and networks of stakeholders, as shown in Figure 15. These economic gains are augmented by the environmental impact of waste reduction, energy, and water conservation, and extended product life spans. The social impact gravitates more toward stakeholder participation, community engagement, and preservation of cultural heritage, regional inequality, human rights pertinent to labor conditions, and social inclusion.
Designing for service and adaptability ranked second in overall TBL scores as the new business model of leasing or renting in fashion or even in construction, as shown in Figure 16, puts the responsibility of product maintenance on the company and not the end customer. This allows the company to comply with EPR regulations for both sectors in the two countries, which are strict in their enforcement. The economic benefits accrue through subscription payments or rental income for financial self-sufficiency, change in production and consumption patterns, business creation, and employment generation. Meanwhile, the social benefits arise from the membership formation of loyal users that promote social cohesion and social capital formation. Adaptability gives the end customer a choice of extending or terminating the service in the case of fashion or expanding or reversing space used in the case of construction, hence creating new uses of the product that create new businesses and employment.
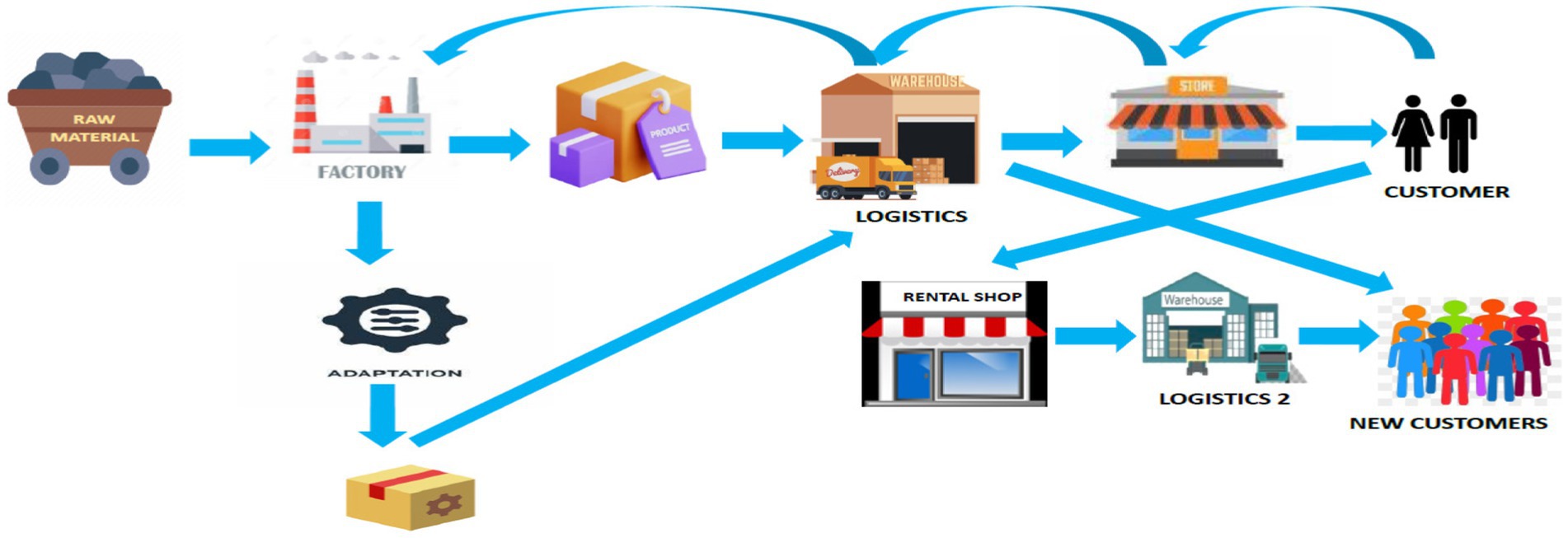
Figure 16. Value chain effects of designing for service/adaptability. Source: adapted from RSA (2013).
Designing for disassembly scored third in the two sectors in the two countries in overall TBL, although interchanging in ranking in some case projects that design for waste and material recovery and that design for longevity. The environmental impact is strong as nothing goes to waste, and the materials can be recovered to make new products, such as reusing the fabrics, zippers, and buttons in fashion or reusing the building materials that are dismounted in the case of construction. Hence, this design principle scores high in product life cycle extension and solid waste reduction. Some form of repurposing, remanufacturing, or upcycling is taking place that not only extends the life cycle of the products but transforms them into new products. The economic and social impacts are midway as the recovered materials after deconstruction or disassembly can be resold, hence leading to business creation, job creation, and technological innovations. Socially, there is stakeholder involvement and community engagement, development of social capital and social cohesion with networks involved in deconstruction, mainly from the downstream users. The value chain effects of designing for disassembly are pictured in Figure 17.
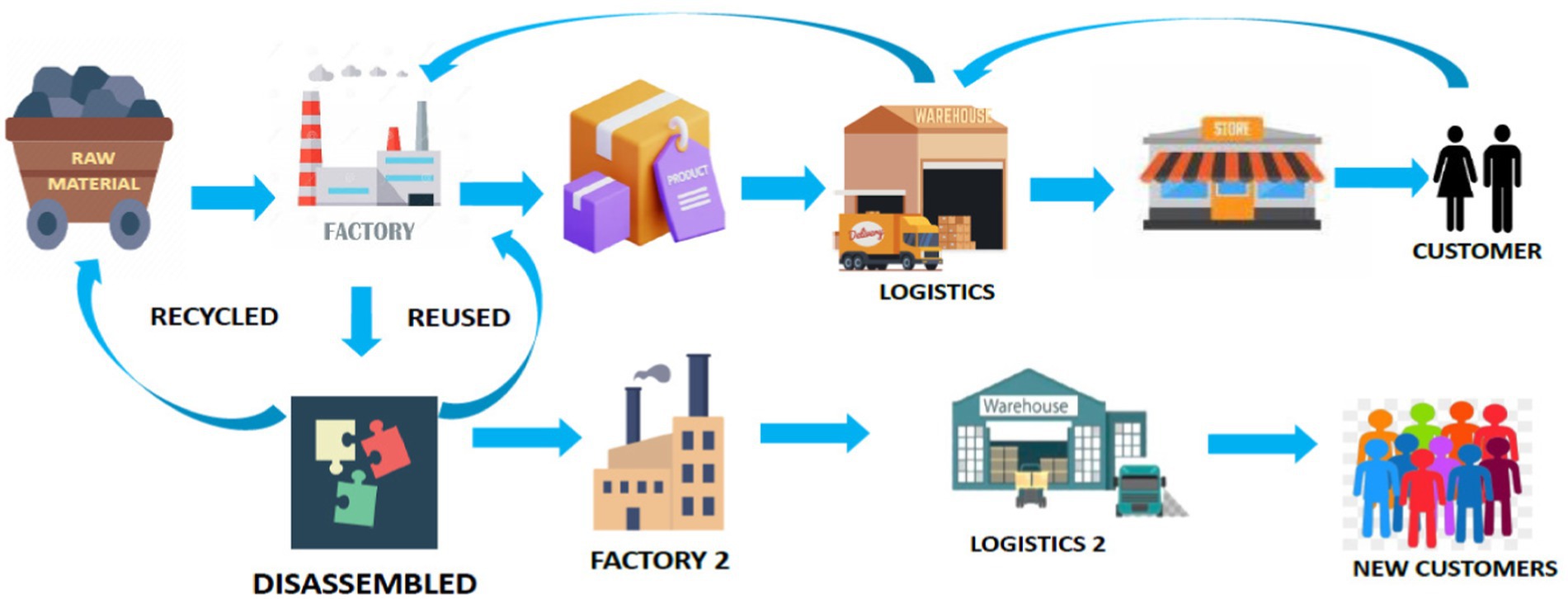
Figure 17. Value chain effects of designing for disassembly. Source: adapted from RSA (2013).
Designing for waste and material recovery alternated between the fourth and fifth spots in overall TBL scores as the social impact is relatively low, with only the end user disposing of the product in refuse bins from which sorting of recoverable materials is done by a third party. If a collective or eco-organization for waste collection exists, the social score is boosted by stakeholder collaboration and community engagement. The environmental impact is high because of waste reduction and extended product life cycles of recovered or discarded materials. The economic impact can also be high with business creation and employment generation for third parties collecting, sorting, and cleaning discarded waste. This is augmented by some technological investment required in terms of sorting and transforming the waste back to recoverable raw materials like yarn in fashion and recycled concrete and building materials in construction. The value chain effects, as shown in Figure 18, depend on whether recycled or upcycled materials are used as inputs by other companies for reselling to new customers.
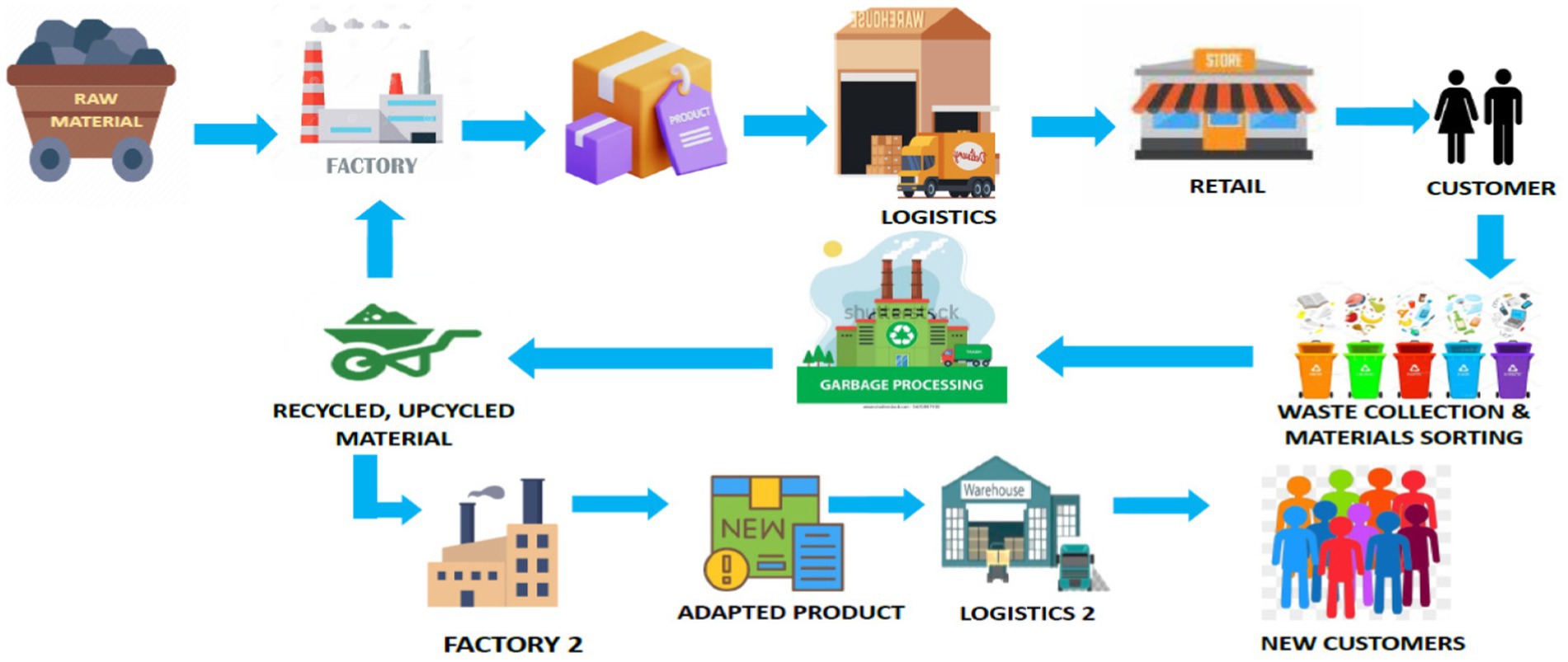
Figure 18. Value chain effects of designing for waste and material recovery. Source: adapted from RSA (2013).
Finally, designing for longevity also teetered between the lowest two ranks in both sectors and countries due to the low score on the social dimension, as the end customer, as depicted in Figure 18, is the only stakeholder involved in the value chain. Her role entails merely an extension of the product life cycle offered to the customer through sharing (rentals) or reselling platforms and repair services, which also reduces waste. There is some element, though, of stakeholder involvement through design co-creation with the company, be it for fashion or construction. In some cases where impact reports are present, there is a strong social impact regarding human rights and labor conditions in the value chain (factories), as well as social inclusion, cohesion, and social capital formation through networks of “green” customers or women entrepreneurs. The economic impact is through business creation with auxiliary jobs created and financial self-sufficiency of these new sharing or reselling platforms that change production and consumption patterns. Environmental scores are high for product life cycle extension, solid waste reduction, and associated implicit reductions in carbon footprints, and use of energy and water resources in sharing and repairing products compared to first-time purchasing (Figure 19).
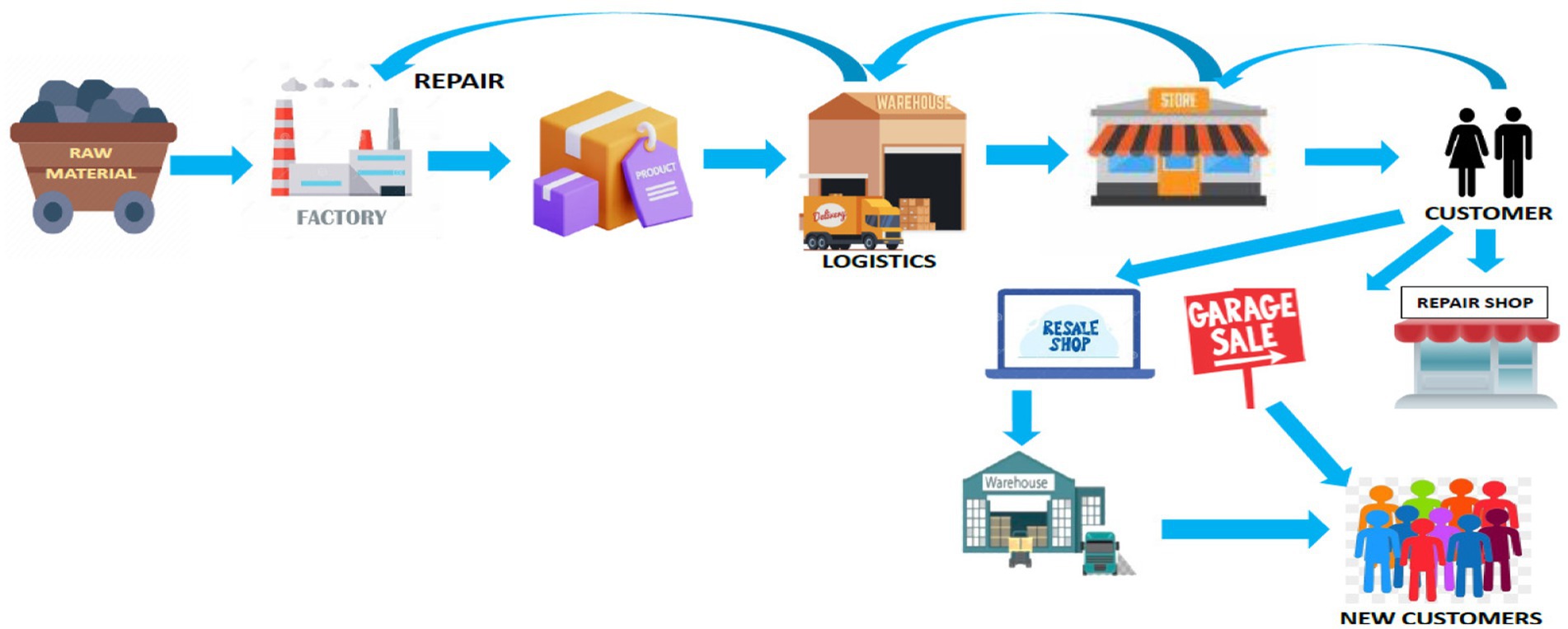
Figure 19. Value chain effects of designing for longevity. Source: adapted from RSA (2013).
5.2 Findings of method triangulation
While the scoring of the indicators was subjective, it was shown in a previous study (see Dytianquin et al., 2023) that the rankings of projects did not change much between subjective and objective criteria for weighting. This was also confirmed in this study where the sustainability indicators for each dimension were first equally weighted and then triangulated using the CRiTIC method using statistical techniques for determining the weights. Instead of assuming equal weights for the criteria, correlation analysis and standard deviations were calculated to determine the weights as described in Section 3.4. The weights of the criteria varied for the sectors, countries, and cases. In construction for both countries, higher weights were accorded to the use of biotic materials, solid waste reduction, energy consumption, carbon emissions, and land use for the environmental dimension. In the economic sphere, an increase in investments in technology, savings on maintenance costs, higher residual values, employment creation, and financial self-sufficiency carried heavier weights in the two countries for both sectors. Finally, the weights for social criteria were more significant in the two countries for regional inequality, human capital formation, and social cohesion, with gender equality, and human rights (labor conditions) emerging as well as more important in the French cases. The results of the CRiTIC method of weighting the criteria as an alternative to equal weighting used in the study are shown in Annex Tables 13, 14 for Dutch and French fashion sample cases, respectively, and Annex Tables 15, 16 for Dutch and French construction sample cases. The results confirm the earlier findings, showing design for biotic materials as ranking first in terms of overall TBL score, followed by design for service/adaptability. However, design for waste and material recovery replaced design for disassembly for third place, which now took penultimate ranking, while design for longevity ranked lowest in the TBL scores.
5.3 Findings on the SDGs
The SDGs, which were both explicit in the sustainability reports or company websites and implicit in the case descriptions, were also identified. As for the SDGs, Table 4 for Dutch companies and Table 5 for French companies in both sectors similarly show a pattern in SDG pronouncements. The transition to circularity in both sectors involves SDG 12 on responsible consumption and production. This is followed by SDG 13 on climate action for most of the case companies, regardless of design principles in both fashion and construction. A tertiary SDG is SDG 14, about life on water for fashion, and SDG 15, about life on land for construction. This is aptly so as fashion is a vast water consumer and polluter, whereas construction is land-intensive. Other SDGs varied between the two sectors, with SDG 8 on decent work and economic growth, SDG 6 on clean water and sanitation, and SDG 9 on industry, innovation, and infrastructure appearing as goals for fashion. For construction, SDG 7 on affordable and clean energy, SDG 6 on clean water and sanitation, and SDG 11 on sustainable cities and communities emerged as targets as well for the companies. A handful of fashion case companies pursue social inclusion by promoting gender equality (SDG 5) in the workplace through women empowerment. Construction companies, similarly, promote social inclusion by ensuring accessible layouts to people with disabilities, hence alluding to reducing inequalities (SDG 10).
6 Discussion
The main research question in this study is to determine if circularity design principles are linked to the balance of the sustainability triptych or triple bottom line of environment, economic, and social dimensions. It also explores if this association is specific to a sector or a country. In the study, two industries—fashion and construction in two countries—The Netherlands and France, using case companies selected specifically for the circular design principle they represent were rated according to the TBL indicators. The design principles for each sector were harmonized into five types: a design for biobased materials, service/adaptability, disassembly, waste and material recovery, and longevity.
The general findings show that for both fashion and construction in the Dutch and French cases, the design principles skew toward the environmental dimension. Still, economic and social dimensions are also emergent and can dominate in certain design principles, such as designing biobased materials for economic effects and designing for service/adaptability, longevity, and waste and material recovery for social impacts. The TBL scores and ranking for the design principles in both sectors in both countries reveal identical patterns. The balance for TBL was pronounced more in the design for biobased materials due to the involvement of the entire value chain further upstream, such as suppliers and farmers of these materials. This leads to more multiplier effects on the economy regarding employment and business creation and technological innovations. The social element is also vital in terms of networks and partnerships among stakeholders, from the suppliers to the architects, builders, designers, manufacturers, logisticians, and final consumers.
All design principles have the environment as a priority, with design for biobased materials having the most impact due to the regenerative nature of the materials used, followed by reducing carbon footprints, energy, and water usage. Design for longevity for fashion and design for waste and material recovery for construction have environmental impacts related to extended life cycles and waste reduction mainly confined to the end-users of the value chain, who are the consumers. The economic impact is also high for designing for service/adaptability and product longevity due to financial self-sufficiency arising from new business models that charge subscriptions and rental payments for leasing, sharing, and reselling platforms. This also leads to environmental benefits of lower maintenance costs and protracted life cycles of materials. More social impact is observed for cases involving design for service/adaptability, design for disassembly, and design for waste and material recovery due to (i) collaboration among stakeholders, (ii) formation of human capital with knowledge dissemination conducted through workshops and training for downstream participants in the value chain, and (iii) formation of social networks such as collector’s club for fashion and materials bank for construction.
A second major finding from the results is the emergence of new circular business models apart from closed-loop or cradle-to-cradle production systems. In design for biobased materials, this involves produce-on-demand or producing only when consumer demand has been quantified and confirmed to prevent overstocking. There is also inclusive sourcing with farmer and supplier connections and logistics traceability, including fair pricing. In design for service/ adaptability, the product-as-a-service model has been the dominant model. This entails the resale and rental of second-hand or used clothing and household furniture/utilities/building materials, and cooperative ownership or shared ownership among end users. In design for disassembly or deconstruction, re-materialization is developed where new products are created and upcycled from discarded or waste materials. Examples are old curtains used as fabrics for fashion and old windows or doors as panels for construction. In design for waste and material recovery, the materials passport bank for construction and collection depots or bins for fashion have appeared. Finally, design for longevity witnessed the occurrence of the physical-to-virtual model with the emergence of digital apps for end users, supported by online reselling or rental platforms and do-it-yourself repair services on social media.
Regarding SDGs, fashion and construction targets primarily SDG 12 on responsible consumption and production, followed by SDG 13 on climate action. A tertiary SDG that is common is SDG 14, or life on water for fashion because of microplastics released by certain fabrics, as well as SDG 6 on clean water and sanitation because of the water intensity of textile production. For construction, it is SDG 15, or life on land (biodiversity), as well as SDG 11 on sustainable cities and communities that prevail as construction is land-intensive. Other SDGs that the cases covered to varying extents are SDG 9 on industry, innovation, and infrastructure for both sectors due to the development of circular technologies, and SDG 8 on decent work and economic growth in fashion due to labor intensity of the industry, and SDG 7 on affordable energy for construction.
For SDG 12 on responsible consumption and production, the main obstacle arising from the cases is the lack of demand or consumer acceptance for wearing second-hand or used clothing and using recycled building materials. This may constrain producers’ financing and scale up possibilities for circular production systems. There is also a need to build collaboration in the value proposition, creation, delivery, and capture of circular products and to comprehend the ecosystem of these elements of circular and sustainable business models. This collaboration necessitates the coordination of innovative activities that develop new materials from recycled waste or new materials from biotic resources, and the sourcing of investments and financing for the circular technologies that are created.
7 Conclusion
In the transition to circularity, the catwalks of fashion and construction in The Netherlands and France traversed the same routes, primarily because of the policy nudge by their governments. This policy thrust complemented and activated the design principles, which was a moderating variable given the type of circular design as the independent variable and the balance of the TBL as the dependent variable. For instance, the French law requiring all new public buildings to be built from at least 50% timber and wood or other natural materials beginning in 2022 encouraged the widespread application of designing for bio-based materials. The Dutch law that all textiles must be recycled or sustainably produced by 2050 and the EPR in the EU requiring producers to be responsible for the entirety of the life cycle of their products enhanced the increasing application of design for waste and material recovery and design for longevity. The study revealed that designing for biobased materials showed the strongest balance because of the broader supply chain effects upstream and downstream, whereas in the latter, other circular design principles for waste and material recovery and longevity were confined mostly to downstream partners. This answers the research question that the type of circularity design principles adopted by a company determines sustainability balance. As two sectors in two countries were analyzed, the second research question as to whether the link between the circularity design principle and the TBL balance is sector or country-specific proves otherwise. Hence, there is a strong parallelism between this association across sectors and countries. Regarding SDGs, both fashion and construction industries unanimously addressed the issue of responsible consumption and production, followed by climate action. A tertiary SDG was life on water and clean water for fashion, given its water usage intensity. In contrast, construction rightly focused on life on land and sustainable cities and communities, where building activities frequently transpire.
7.1 Limitations and areas for further research
The main contribution of this study to the CE literature is the identification of five typologies of circular design principles that were applied to two sectors. This contrasts with existing studies describing circular design principles that are sector specific.25 These homogenized circular design principles could probably apply to other sectors such as food and agriculture, electronics, health and hospitality, and tourism that are also applying circularity strategies. Another contribution is the link the study made between sustainability balance and the circularity design principle that is used. In this study, case companies that designed for biobased materials were found to score higher in addressing TBL. None of the existing studies, as discussed in the literature review, explored the association between the type of circular design principle and balanced sustainability.
The fact that no primary data collection was used in this research and mainly access to secondary data was recoursed limits the generalizability of the findings. It is possible that more recent cases may dispute the findings as many of the design principles combine elements of using biotic materials with deconstruction and waste and material recovery. Hence, over time, some design principles will have overlapping elements. Moreover, the case studies were only snapshots of what transpired during a certain period, so the long-term impact on sustainability is not considered. Finally, only one case per design principle was selected, which could introduce bias into the findings, although this was triangulated by taking a second sample.
As scope for further improvement, more case selections, such as 3–5 per design principle per country, can be taken to ensure consistency of the findings. Cases from the same sectors of fashion and construction in other EU countries, such as Italy, Spain, Portugal, Greece, Germany, Sweden, and Denmark, might prove supportive of this study’s results. Comparison with cases from non-EU countries such as in the Americas, Asia, Africa and Oceania may also disclose striking similarities or differences. Finally, the types of companies, whether start-ups, small and medium enterprises, or large companies as having a bearing on the design principles, may be worth exploring.
Data availability statement
The datasets presented in this study can be found in online repositories. The names of the repository/repositories and accession number(s) can be found in the article/Supplementary material.
Author contributions
ND: Conceptualization, Data curation, Formal analysis, Funding acquisition, Investigation, Methodology, Project administration, Resources, Software, Supervision, Validation, Visualization, Writing – original draft, Writing – review & editing. SP: Data curation, Formal analysis, Investigation, Methodology, Resources, Writing – review & editing. NK: Resources, Supervision, Writing – review & editing, Funding acquisition.
Funding
The author(s) declare that financial support was received for the research, authorship, and/or publication of this article. Funding was only used to pay for the publication fee from Zuyd University Sustainable International Business Research Center.
Conflict of interest
The authors declare that the research was conducted in the absence of any commercial or financial relationships that could be construed as a potential conflict of interest.
The author(s) declared that they were an editorial board member of Frontiers, at the time of submission. This had no impact on the peer review process and the final decision.
Publisher’s note
All claims expressed in this article are solely those of the authors and do not necessarily represent those of their affiliated organizations, or those of the publisher, the editors and the reviewers. Any product that may be evaluated in this article, or claim that may be made by its manufacturer, is not guaranteed or endorsed by the publisher.
Supplementary material
The Supplementary material for this article can be found online at: https://www.frontiersin.org/articles/10.3389/frsus.2024.1456302/full#supplementary-material
Footnotes
1. ^See also Kerr and Landry (2017), Filho et al. (2022), EU Parliament (2022), Niinimäki et al. (2020).
2. ^See EU Parliament (2022), Niinimäki et al. (2020).
3. ^See RSA (2013), Henninger et al. (2022), Niinimaki (2018, 2011), Niinimaki and Hassi (2011).
4. ^See Cheshire (2016), Brand (1994), CE100 (2015).
5. ^See Dytianquin et al. (2023).
6. ^See Ellen MacArthur Foundation and Granta Design (2015), Moraga et al. (2019).
7. ^See Boulding (1966), Mäler (1974), Tietenberg (1984), Pearce and Turner (1990), Daly (1992).
8. ^See Frosch and Gallopoulos (1989), Allenby (1992), Bringezu (2003), Wells and Seitz (2005), Chertow (2000), Linton et al. (2007), Lifset and Boons (2012), Bloomsma and Brennan (2017).
9. ^The study of innovative solutions in nature and natural processes. See Benyus (2002), Kennedy (2007), Marshall and Lozeva (2009), Habib (2011), Green et al. (2015), Das et al. (2015).
10. ^See Stahel (1986), Lyle (1994), McDonough and Braungart (2002).
11. ^See Lovins et al. (1999), Hawken et al. (2000), Pauli (2010).
12. ^Refuse is to buy less or reject buying products that are not green for consumers or to reject the use of new raw materials for producers. Reduce means buying fewer products and using fewer resources. Reuse involves the use of a product or some parts that can be shared. Refurbish is restoring a product to good condition but not comparable to brand new. Repair is fixing a fault that made the product inoperable. Remanufacture is replacing a worn, non-functional product or component to a “like new” or “better than new” condition; hence, it is analogous to upcycling. Recycle refers to product recovery, where waste is separated into materials that may be reprocessed or fitted into new products. Recover is creating energy from waste that cannot be reused or recycled, and hence incinerated or disposed off in a landfill.
13. ^https://www.government.nl/topics/circular-economy/
14. ^VNO-NCW (Confederation of Netherlands Industry and Employers) and MKB-Nederland (representing small and medium-sized enterprises); FNV, Federatie Nederlandse Vakbeweging (Dutch Federation of Trade Unions); VCP, Vakcentrale voor Professionals (Trade Union Federation for highly educated professionals); Stichting Natuur and Milieu (Nature and Environment Foundation); VNG, Vereniging Nederlandse Gemeenten (Association of Netherlands Municipalities); IPO, Interprovinciaal Overleg (Association of Provincial Authorities); and Unie van Waterschappen (Association of Dutch Regional Water Authorities).
15. ^Department of Waterways and Public Works.
16. ^Clothing, Linen and Footwear.
17. ^see https://www.footprintnetwork.org/
18. ^International Organization for Standardization.
19. ^The 16 principles are (1) Interdependence of life, (2) love and responsibility, (3) democracy and freedom, (4) justice across generations, (5) protect earth’s diversity, (6) prevent ecological harm, (7) sustainable lifestyles, (8) share knowledge, (9) eradicate poverty, (10) equitable human development, (11) gender equality, (12) dignity, inclusion and wellbeing, (13) transparency and participation, (14) integrate values into education, (15) respect all living beings, and (16) non-violence and peace.
20. ^In January 2023, the EU CSRD went into force, which mandates companies to report social and environmental of their operations, This directive applies to all large companies and selected small and medium enterprises (SMEs) as well as non-EU companies that generate more than €150 million in the EU market (https://fiannce.ec.europa.eu/).
21. ^See RSA (2013), Henninger et al. (2022), Niinimaki (2018, 2011), Niinimaki and Hassi (2011), Tanttu et al. (2016), Cheshire (2016), Brand (1994), Bakker et al. (2014), Sumter et al. (2020), Balkenende et al. (2017), Bocken et al. (2016), Ghisellini et al. (2016).
22. ^See Schöggl et al. (2022).
23. ^See Bocken et al. (2016).
24. ^For Dutch fashion: Hekkert et al. (2021), Maas and Joshi (2021), Snoek (2017), Netherlands Enterprise Agency and Holland Circular Spot (2020), Reike et al. (2023), Transition Agenda (2018a); for Dutch construction: De Graaf and Schuitemaker (2022), Amory (2019), Dytianquin et al. (2023), and Transition Agenda (2018b); for French fashion: Guyot (2023), Ehrig et al. (2022), French Ministry of Ecological Transition and Solidarity (2020), Ellen MacArthur Foundation (2021, 2024), www.circularstories.org; and for French construction: Doussolin and Bittencourt (2022), Diemer et al. (2022), Dytianquin et al. (2023), CE100 (2015), Gravagnuolo et al. (2019), Derbal et al. (2017), www.climate-kic.org.
25. ^See RSA (2013), Tanttu et al. (2016), Cheshire (2016).
References
Allenby, B. R. (1992). Achieving sustainable development through industrial ecology. Int. Environ. Aff. 4, 56–68.
Amory, J. (2019). A Guidance Tool for Circular Building Design. Delft: Delft University of Technology, Architecture, and the Built Environment.
Bakker, C. A., den Hollander, M. C., vanHinte, E., and Zijlstra, Y. (2014). Products that last: Product Design for Circular Business Models. Delft University of Technology.
Bal, M., Bryde, D., Fearon, D., and Ochieng, E. (2013). Stakeholder engagement: achieving sustainability in the construction sector. Sustain. For. 2013, 695–710. doi: 10.3390/su5020695
Balkenende, R., Bocken, N., and Bakker, C. (2017). Design for Circular Economy. Chapter 36 in Egenhoefer, R.B. Routledge Handbook of Sustainable Design. doi: 10.4324/9781315625508
Bason, C. (2010). Leading public sector innovation: Co-creating for a better society. 1st Edn. Bristol: Bristol University Press.
Benyus, J. (2002). Biomimicry: innovation inspired by nature. 2nd Edn. New York, NY: Harper Perennial.
Bloomsma, F., and Brennan, G. (2017). The emergence of circular economy: a new framing around prolonging resource productivity. J. Ind. Ecol. 21, 603–614. doi: 10.1111/jiec.12603
Blumberg, B., Cooper, D., and Schindler, P. (2014). Business Research Methods. 4th Edn. London: McGraw Hill, 303–318.
Bocken, N., de Pauw, I., Bakker, C., and van der Grinten, B. (2016). Product design and business model strategies for a circular economy. Journal of Industrial and Production Engineering, 33, 308–320, doi: 10.1080/21681015.2016.1172124
Boulding, K. (1966). “The economics of the coming spaceship earth” in Environmental quality in a growing economy. ed. H. Jarett (Baltimore, MD: Johns Hopkins University Press), 3–14.
Braungart, M., and McDonough, W. (2002). Cradle to Cradle: Remarking the Way We Make Things. New York, NY: North Point Press.
Bringezu, S. (2003). “Industrial ecology and material flow analysis” in Perspectives on industrial ecology. eds. D. Bourg and S. Erkman (Sheffield: Greenleaf).
Burford, G., Hoover, E., Velasco, I., Janoušková, S., Jimenez, A., Piggot, G., et al. (2013). Bringing the “missing pillar” into sustainable development goals: towards intersubjective value-based indicators. Sustain. For. 5, 3035–3059. doi: 10.3390/su5073035
CE100 (2015). Circularity in the Built Environment Case Studies: A compilation of case studies from CE100, April. Ellen MacArthur Foundation. Available at: https://build360.ie/wp-content/uploads//2023/01//EMF_casestudies.pdf (Accessed April 30, 2023).
Chertow, M. (2000). Industrial symbiosis: literature and taxonomy. Ann. Rev. Energ. Environ. 25, 313–337. doi: 10.1146/annurev.energy.25.1.313
Cheshire, D. (2016). Building Revolutions: Applying the Circular Economy to the Built Environment. London: Riba Publishing.
Clugsston, R. (2011). Ethical framework for a sustainable world. J. Educ. Sust. Dev. 5:207. doi: 10.1177/097340821100500207
Crook, L. (2020). French Buildings to be Built With 50 percent wood. Available at: https://www.dezeen.com/ (Accessed May 24, 2024).
Daly, H. E. (1992). Allocation, distribution, and scale: towards an economics that is efficient, just, and sustainable. Ecol. Econ. 6, 185–193. doi: 10.1016/0921-8009(92)90024-M
Das, S., Bhowmick, M., Chattopadhyay, S. K., and Basak, S. (2015). Application of biomimicry in textiles. Curr. Sci. 109, 893–901. doi: 10.18520/cs/v109/i5/893-901
De Graaf, I. M., and Schuitemaker, N. (2022). Circular Buildings: Constructing a Sustainable Future. Brochure. Holland Circular Hotspot, the Transition Team Circular Construction Economy, Arcadis and the Netherlands’ ministries of the Interior and Kingdom Relations, and Infrastructure and Water Management.
De Groene Zaak,, and Ethica, (2015). Boosting circular design for a circular economy. Wrocław: ECKP.
Denzin, N. K. (1978). The research act: A theoretical introduction to sociological methods. 2nd Edn. New York, NY: McGraw Hill.
Derbal, R., Lucas, A., Brachelet, F., Defer, D., and Antczak, E. (2017). Rehafutur Insitu instrumentation for a comprehensive building analysis. Energ. Proc. 139, 301–306. doi: 10.1016/j.egypro.2017.11.212
Desai, V. M. (2018). Collaborative stakeholder engagement: an integration between theories of organizational legitimacy and learning. Acad. Manag. J. 61, 220–244. doi: 10.5465/amj.2016.0315
Diemer, A., Nedelciu, C. E., Morales, M. E., Batisse, C., and Cantuarias-Villessuzanne, C. (2022). Waste management and circular economy in the French building and construction sector. Front. Sust. 3:840091. doi: 10.3389/frsus.2022.8400
Doussolin, J. P., and Bittencourt, M. (2022). How effective is the construction sector in promoting the circular economy in Brazil and France? A waste input-output analysis. Struct. Chang. Econ. Dyn. 60, 47–58. doi: 10.1016/j.strueco.2021.10.009
Dytianquin, N., Gregersen-Hermans, J., Kalogeras, N., Ritzen, M., and Van Oorschot, J. (2021). Circularity in selected EU countries: the case of construction and demolition industries. IOP Conf. Series Earth Environ. Sci. 855:012017. doi: 10.1088/1755-1315/855/1/012017
Dytianquin, N., Kalogeras, N., van Oorschot, J., and Abujidi, N. (2023). Circularity in the construction and demolition industry: comparing weighting methods for multi-criteria decision analysis. Front. Sust. 4:1115865. doi: 10.3389/frsus.2023.1115865
Ehrig, B., Warcschum, M., Baddou, F., Minutella, D., and Dittmar, F. (2022). The Kearney CFC 2022 Report: Are Fashion Brans Ramping up Their Circularity Game? Available at: https://www.kearney.com/documents/291362523/291372000/ (August 26, 2023).
Elkington, J. (1994). Towards the sustainable corporation win-win-win business strategies for sustainable development. Calif. Manag. Rev. 36, 90–100.
Ellen MacArthur Foundation (2015). Towards a Circular Economy: Business Rationale for an Accelerated Transition. Available at: https://tinyurl.com/zt8fhxw (Accessed May 23, 2023).
Ellen MacArthur Foundation (2020). Financing the Circular Economy: Capturing the Opportunity. Cowes: Ellen MacArthur Foundation (EMF).
Ellen MacArthur Foundation (2021). Circular Design for Fashion. Cowes: Ellen MacArthur Foundation (EMF).
Ellen MacArthur Foundation (2024). Examples of circular economy in the fashion industry. Available at: https://www.ellenmacarthurfoundation.org/topics/fashion/examples (Accessed July 25, 2024)
Ellen MacArthur Foundation and Granta Design (2015). Circularity indicators - An approach to measuring circularity. Methodology 20, 1–98. doi: 10.1016/j.giq.2006.04.004
EU Parliament (2022). The Impact of Textile Production and Waste on the Environment (Infographic). Available at: https://www.europarl.europa.eu/news/ (Accessed April 28, 2023).
Filho, W. L., Perry, P., Heim, H., Dinis, M. A. P., Moda, H., Ebhuoma, E., et al. (2022). An overview of the contribution of the textiles sector to climate change. Front. Environ. Sci. 10:973102. doi: 10.3389/fenvs.2022.973102
Fonseca, L., and Carvalho, F. (2019). The reporting of SDGs by quality, environmental, and occupational health, and safety-certified organizations. Sustain. For. 11:5797. doi: 10.3390/su11205797
French Ministry of Ecological Transition and Solidarity (2020). France's Anti-waste and Circular Economy Law. Available at: https://circulareconomy.europa.eu/platform/en/strategies/french-act-law-against-waste-and-circular-economy (Accessed May 16, 2023).
Ghisellini, P., Cialani, C., and Ulgiati, S. (2016). A review on circular economy: the expected transition to a balanced interplay of environmental and economic systems. J. Clean. Prod. 114, 11–32. doi: 10.1016/j.jclepro.2015.09.007
Ghisellini, P., Ripa, M., and Ulgiati, S. (2018). Exploring environmental and economic costs and benefits of a circular economy approach to the construction and demolition sector. A literature review. J. Clean. Prod. 178, 618–643. doi: 10.1016/j.jclepro.2017.11.207
Ginevicius, R., and Podvezko, V. (2005). Objective and subjective approaches to determining the criterion weight in multicriteria models. Proc. Int. Conf. RelStat. Trans. Telecommun. 6, 133–137.
Global Construction Review (2020). New French buildings must be made 50% from wood. Available at: www.globalconstructionreview.com (Accessed July 16, 2024).
Global Footprint Network (n.d.). Available at: https://www.footprintnetwork.org/ (Accessed June 15, 2024).
Grant, D., Trautrims, A., and Wong, C. Y. (2017). Sustainable Logistics and Supply Chain Management. 2nd Edn. London: Kogan Page Ltd.
Gravagnuolo, A., De Angelis, R., and Iodice, S. (2019). Circular economy strategies in the historic built environment: cultural heritage adaptive reuse. Proc. STS Conf. 2019, 121–144. doi: 10.3217/978-3-85125-668-0-08
Green, D. W., Lee, J. M., and Jung, H. S. (2015). Marine structural biomaterials in medical biomimicry. Tissue Eng. Part B Rev. 21, 438–450. doi: 10.1089/ten.teb.2015.0055
Guyot, O. (2023). Strengths and Margins of CSR Progress of French Fashion Brands according to Paris Good Fashion and Climate Chance. Fashion Network. Available at: https://fr.fashionnetwork.com/ (Accessed July 28, 2023).
Habib, M. K. (2011). Biomimetics: innovations and robotics. Int. J. Mech. Manuf. Syst. 4, 113–134. doi: 10.1504/IJMMS.2011.039263
Hanemmaijer, A., Kishna, M., Brink, H., Koch, A., Prins, A., and Rood, T. (2021). Netherlands Integral Circular Economy Report 2021, English Summary. The Hague: PBL Netherlands Environmental Assessment Agency.
Haupt, M., and Hellweg, S. (2019). Measuring the environmental sustainability of a circular economy. Environ. Sust. Indic. 1:100005. doi: 10.1016/j.indic.2019.100005
Hawken, P., Lovins, A., and Lovins, L. (2000). Natural Capitalism; The Next Industrial Revolution. London: Earthscan.
Hekkert, M., Reike, D., Rainville, A., and Negro, S. (2021). Transition to Circular Textiles in the Netherlands: An innovation systems analysis. Utrecht: Copernicus Institute of Sustainable Development. PowerPoint Slides. Utrecht University.
Henninger, C., Niinimaki, K., Blasquez Cano, M., and Jones, C. (2022). “Design for a Circular Economy” in Sustainable Fashion Management (London: Routledge).
Herremans, I., Nazari, J., and Mahmoudian, F. (2016). Stakeholder relationships, engagement and sustainability reporting. J. Bus. Ethics 138, 417–435. doi: 10.1007/s10551-015-2634-0
Hysa, E., Kruja, A., Ur Rehman, N., and Laurenti, R. (2020). Circular economy innovation and environmental sustainability impact on economic growth: an integrated model for sustainable development. Sustain. For. 2020:4831. doi: 10.3390/su12124831
Inter-American Development Bank (2018). Social impact assessment: integrating social issues in development projects, IDB Series on Environmental and Social Risk and Opportunity. Washington, DC: Inter-American Development Bank (IDB).
Kennedy, S. (2007). Biomimicry.bimimetics: general principles and practical examples The Science Creative Quarterly. Available at: https://www.scq.ubc.ca/biomimicrybimimetics-general-principles-and-practical-examples/ (Accessed December 10, 2020).
Kerr, J., and Landry, J. (2017). Pulse of the Fashion Industry. Boston, MA: Global Fashion Agenda and the Boston Consulting Group.
Kirchherr, J., Reike, D., and Hekkert, M. (2017). Conceptualizing the circular economy: An analysis of 114 definitions. Res. Conserv. Recycling 127, 221–232. doi: 10.1016/j.resconrec.2017.09.005
Lifset, R., and Boons, F. (2012). “Industrial ecology: Business management in a material world” in The Oxford handbook of business and natural environment. eds. P. Bansal and A. Hoffman (Oxford: Oxford University Press), 311–326.
Linnanen, L., and Panapanaan, V. (2002). Roadmapping CSR in Finnish Companies. Helsinki: Helsinki University of Technology.
Linton, J., Klassen, R., and Jayaramman, V. (2007). Sustainable supply chains: An introduction. J. Operations Manage. 25, 1075–1082. doi: 10.1016/j.jom.2007.01.012
Lovins, A., Lovins, I., and Hawken, P. (1999). A road map for natural capitalism. Harv. Bus. Rev. 77, 145–158.
Lyle, J. T. (1994). Regenerative design for sustainable development New York, NY: John Wiley and Sons.
Maas, K., and Joshi, S. (2021). Clothes the Circle: A Case Study Report. Final Evaluation of ‘Ómzet met Impact’ Programme. Rotterdam: Erasmus University Business School.
Mäler, K. G. (1974). Environmental economics: a theoretical inquiry. Baltimore: Johns Hopkins University Press.
Marrewijk, M. (2013). “Concepts and Definitions of CSR and corporate sustainability: Between Agency and Communion” in Journal of Business Ethics. Advances in Business Ethics Research. eds. A. Michalos and D. Poff, vol. 2 (Dordrecht: Springer).
Marshall, A., and Lozeva, S. (2009). Questioning the theory and practice of biomimicry. Int. J. Design Nat. Ecodyn. 4, 1–10. doi: 10.2495/DNE-V4-N1-1-10
McDonough, W., and Braungart, M. (2002). Cradle to cradle: Remaking the way we make things. New York, NY: North Point Press.
McLaren, D. (2004). Global stakeholders: corporate accountability and investor engagement. Corp. Gov. Internat. Rev. 12:360. doi: 10.1111/j.1467-8683.2004.00360.x
Mendoza, J. M., Sharmina, M., Gallego-Schmid, A., Heyes, G., and Azapagic, A. (2017). Integrating back casting and eco-design for the circular economy: the BECE framework. J. Ind. Ecol. 21, 526–544. doi: 10.1111/jiec.12590
Ministry for an Ecological and Solidary Transition and Ministry for the Economy and Finance (2018). Roadmap for the Circular Economy. Available at: www.ecologique-solidaire.gouv.fr (Accessed May 12, 2023).
Moraga, G., Huysveld, S., Mathieux, F., Blengini, G. A., Alqerts, L., Van Acker, K., et al. (2019). Circular economy indicators: what do they measure? Res. Conserv. Recycling 146, 452–461. doi: 10.1016/j.resconrec.2019.03.045
Mossberg, M. (2018). Evaluation of Circular Economy design principles by Material Flow Analysis. Master Thesis. Gothenburg: Chalmers University of Technology.
Mulia, P., Behura, A., and Kar, S. (2017). Corporate Environmental Sustainability for a Sustainable Future. Problems of Social Development 12, 69-77. Available at: https://ssrn.com. (Accessed May 26, 2024).
Mustafa, O. M. A., and Legyel, P. (2022). Circular economy: a bibliometric mapping. J. Agric. Inf. 13, 36–45. doi: 10.17700/jai.2022.13.1.650
Netherlands Enterprise Agency and Holland Circular Spot (2020). From Linear to Circular in the Textile and Apparel Industries. Available at: https://hollandcircularhotspot.nl/wp-content/uploads/2020/09/3811_Brochure-Circular-Economy-and-textile_KG2.pdf (Accessed Jube 6, 2024).
Niinimaki, K., and Hassi, L. (2011). Emerging design strategies in sustainable production and consumption of clothing and textiles. J. Clean. Prod. 19, 1876–1883. doi: 10.1016/j.jclepro.2011.04.020
Niinimäki, K., Peters, G., Dahlbo, H., Perry, P., Rissanen, T., and Gwilt, A. (2020). The environmental price of fashion. Nat. Rev. Earth Environ. 1:39. doi: 10.1038/s43017-020-0039-9
OECD (2019). Building Models for the Circular Economy: Opportunities and Challenges for Policy. Paris: OECD Publishing.
Padilla-Rivera, A., Russo-Garrido, S., and Merveille, N. (2020). Addressing the social aspects of a circular economy: a systematic literature review. Sustain. For. 12, 1–17. doi: 10.3390/su12197912
Paris Good Fashion (2023). France has laid down the law on sustainability: What does it mean for fashion? Available at: https://parisgoodfashion.fr/ (Accessed August 10, 2024).
Pauli, G. (2010). The blue economy: 10 years, 100 innovations, 100 million jobs. Taos, NM: Paradigm Publications.
Pearce, D. W., and Turner, K. T. (1990). Economics of natural resources and the environment. Baltimore, MD: Johns Hopkins University Press.
Persson, U., and Olander, S. (2004). Methods to estimate stakeholder views of sustainability for construction projects. The 21st Conference on Passive and Low Energy Architecture. 19-22 September 2004. The Netherlands: Technische Universiteit Eindhoven.
Piller, L. (2021). Bason’s 4 credos of design thinking may frame an alternative, circular and sustainable approach to the way we design, produce and use clothes. Acad. Lett. 24:2720. doi: 10.20935/AL2720
Pötting, J., and Hanemaaijer, A. (2018). Circular economy: what we want to know and can measure. Framework and baseline assessment for monitoring the progress of the circular economy in the Netherlands. Dutch: Policy Report PBL Netherlands Environmental Assessment Agency.
Quantis (2018). Measuring Fashion: Environmental Impact of the Global Apparel and Footwear Industries Study. Available at: https://quantis.com/ (Accessed April 12, 2023).
Reike, D., Hekkert, M., and Negro, S. (2023). Understanding circular economy transitions: The case of circular textiles. Bus. Strateg. Environ. 32, 1032–1058. doi: 10.1002/bse.3114
Rizos, V., Tuokko, K., and Behrens, A. (2017). The circular economy: A review of definitions, processes, and impact. Center for European Policy Studies (CEPS) Research Report No. 2017/8. Available at: www.ceps.eu. (Accessed November 20, 2023).
Roszkowska, E. (2013). Rank ordering criteria weighting methods—a comparative overview. Optim. Stu. Ekonomiczne NR 5:2013.
RSA (2013). Investigating the Role of Design in the Circular Economy. The Great Recovery Redesigning the Future Report. Available at: www.greatrecovery.org.uk (Accessed July 15, 2024).
Sattertwaithe, D. (2012). Beyond the Millennium Development Goals. Eco-Business. Available at: https://www.eco-business.com/ (Accessed May 22, 2023).
Sauvé, S., Bernard, S., and Sloan, P. (2016). Environmental sciences, sustainable development, and circular economy: alternative concepts for trans-disciplinary research. Environ. Dev. 17, 48–56. doi: 10.1016/j.envdev.2015.09.002
Schöggl, J.-P., Stumpf, L., and Baumgartner, R. (2022). The narrative of sustainability and circular economy-A longitudinal review of two decades of research. Conserv. Recycl. 163:105073. doi: 10.1016/j.resconrec.2020.105073
Seawright, J., and Gerring, J. (2008). Case selection techniques in case study research. Polit. Res. Q. 61, 294–308. doi: 10.1177/1065912907313077
Sillanpää, M., and Ncibi, C. (2019). The Circular Economy: Case Studies about the Transition from the Linear Economy. Amsterdam: Elsevier.
Singh, A. P., and Rahman, Z. (2021). Integrating corporate sustainability and sustainable development goals: towards a multi-stakeholder framework. Cogent Bus. Manage. 8:1985686. doi: 10.1080/23311975.2021.1985686
Singh, A., Sharma, B., Gaurav, N., and Singh, N. (2016). Environmental impact assessment as a tool to achieve the sustainable development. IMPACT 4, 143–156.
Snoek, S. (2017). Circular Economy in the Textile Industry: Transition theory in Dutch start-ups towards a circular economy. MSc. Thesis Climate Studies. Wageningen: Wageningen University.
Stahel, W. R. (1986). Product life as a variable: the notion of utilization. Sci. Public Policy 13, 185–193. doi: 10.1093/spp/13.4.185
Sumter, D., de Koning, J., Bakker, C., and Balkenende, R. (2020). Circular Economy Competencies for Design. Sustainability. 12:1561. doi: 10.3390/su12041561
Su, B., Heshmati, A., Geng, Y., and Yu, X. (2013). A review of the circular economy in China: moving from rhetoric to implementation. J. Clean. Prod. 42, 215–227. doi: 10.1016/j.jclepro.2012.11.020
Tansley, E. (2020). France Unveils New 50% Timber Sustainability Law. Available at: www.twinfm.com (Accessed March 10, 2020).
Tanttu, M., Kohtala, C., and Niinimäki, K. (2016). Can Design-Driven Material Innovation Also Drive Circularity?. Available at: www.trash2cash.eu (Accessed March 10, 2020).
The Essentials for the CLF Industry (2020). French law on Fighting Waste and on the Circular Economy. Available at: https://refashion.fr/on (Accessed August 24, 2020).
Tietenberg, T. (1984). Environmental and natural resource economics. Northbrook, IL: Scott Foresman and Company.
Torcatoru, C., Savescu, D., and Repanovici, A. (2022). Literature review by scientometric methods on the impact of the circular economy on sustainable industrial products. Sustain. For. 2022:5084. doi: 10.3390/su14095084
Transition Agenda (2018a). The transition towards a Circular Goods Economy. Available at: https://hollandcircularhotspot.nl/wp-content/uploads/2018/06/TRANSITION-AGENDA-CONSUMER-GOODS_EN.pdf (Accessed July 25, 2024).
Transition Agenda (2018b). Circular Construction Economy. Available at: https://circulairebouweconomie.nl/wp-content/uploads/2019/09/Circular-Construction-Economy-1.pdf (Accessed June 24, 2024).
Triantaphyllou, E., and Mann, S. (1989). An examination of the effectiveness of multi-dimensional decision-making methods: a decision-making paradox. Decis. Support. Syst. 5, 303–312.
United Nations Environment Program (2009). Guidelines for social life cycle assessments of products. Paris, France: Office of the Director, UNEP Division of Technology, Industry and Economics.
Van Vliet, M. M. (2018). Disassembling the steps towards Building Circularity: Reinventing the Building Disassembly assessment method in the Building Circularity indicator. Eindhoven: Eindhoven University of Technology.
Venkatraman, S., and Nayak, R. R. (2015). Corporate sustainability: an IS approach for integrating triple bottom line elements. Soc. Resp. J. 11, 482–501. doi: 10.1108/SRJ-11-2013-0136
Wells, P., and Seitz, M. (2005). Business models and closed loop chains: A typology. Supply Chain Manage. Int. J. 10, 249–251. doi: 10.1108/13598540510612712
Yijun, J., Ying, H., and Xuhong, S. (2011). Applying circular economy theory in Environmental Impact Assessment. 2010 International Conference on Biology, Environment and Chemistry. IPCBEE Singapore: IACSIT Press
Zapucioiu, L. F., and Trica, C. L. (2021). The Circular Economy in the Context of Sustainable Development ―A Bibliometric Analysis. Conference Paper Competitiveness of Agro-Food and Environmental Economy. doi: 10.24818/CAFEE/2021/10/06
Zardari, N. H., Ahmed, K., Shirazi, S. M., and Yusof, Z. B. (2015). Weighting Methods and their Effects on Multi-Criteria Decision-Making Model Outcomes in Water Resources Management. Cham: Springer Verlag.
Appendix
Websites of case companies.
Keywords: circular design, triple bottom line, SDGs, balanced sustainability, fashion, construction
Citation: Dytianquin N, Paindavoine S and Kalogeras N (2024) Going circular with what we wear and how we build: parallelisms between the Dutch and French catwalks of fashion and construction. Front. Sustain. 5:1456302. doi: 10.3389/frsus.2024.1456302
Edited by:
Ioannis Kostakis, Harokopio University, GreeceReviewed by:
Dimitra Papadaki, National and Kapodistrian University of Athens, GreeceVasilis Nikou, Harokopio University, Greece
Giacomo Di Foggia, University of Milano-Bicocca, Italy
Copyright © 2024 Dytianquin, Paindavoine and Kalogeras. This is an open-access article distributed under the terms of the Creative Commons Attribution License (CC BY). The use, distribution or reproduction in other forums is permitted, provided the original author(s) and the copyright owner(s) are credited and that the original publication in this journal is cited, in accordance with accepted academic practice. No use, distribution or reproduction is permitted which does not comply with these terms.
*Correspondence: Norman Dytianquin, bm9ybWFuLmR5dGlhbnF1aW5AenV5ZC5ubA==; bm9ybWFuLmR5dGlhbnF1aW5AZ21haWwuY29t