- 1Technical Services, Department of Clinical and Biomedical Sciences, University of Exeter, Exeter, United Kingdom
- 2RNA-Mediated Mechanisms of Disease Group, Department of Clinical and Biomedical Sciences, University of Exeter, Exeter, United Kingdom
Cell culture is a cornerstone of in vitro biological research. Whilst glassware was once commonplace in tissue culture facilities, in recent decades laboratories have moved towards a heavy reliance on single use plastics for routine procedures. Single use plastics allow for accessible, sterile, and often affordable equipment that comes at a high environmental cost. We developed a glassware preparation and cleaning process that allowed the comparison of “traditional” plastic-heavy, and adapted “sustainable,” cell culture practices, to empirically compare the sterility, viability, and proliferative capacity of cells cultured with differing techniques, by observing IL-6 production, morphology, and proliferation rate of cultured human pulmonary fibroblast cells. During which, we calculated the carbon footprint of traditional versus sustainable methods. We additionally endeavored to provide a realistic overview of the steps required to transition to more sustainable cell culture practices and make suggestions to ease the cost, labor, and time required to uptake similar practices in other laboratories. Cells cultured using reusable glassware did not show signs of contamination or stress compared to cells grown solely with plasticware, and glassware baked at 180°C for 120 min was sufficiently decontaminated and depyrogenated for culturing these cells. An individual researcher adopting the same methodology could reduce their carbon footprint by 105.92 kg of Carbon dioxide equivalent (CO2e) whilst also saving money (£408.78) over a 10-year period. We predict that these benefits would be greater if more researchers were to uptake these adapted practices. We intend for this paper to reassure researchers that viable, sterile, and sustainable routine cell culture can be achieved with little upfront cost to the researcher, with the prospective benefit of greatly reducing the cost to the environment. We additionally hope that increased uptake, and thus demand of more sustainable practices, encourages suppliers, policy makers, and funding bodies to make sustainable practices more accessible to individual researchers and institutions worldwide.
Introduction
Laboratories rely heavily on single use plastic. In 2015, researchers at the University of Exeter estimated that worldwide, the life sciences industries were projected to produce around 5.5 million tons of plastic waste annually; this corresponds to approximately 1.5% of the current total annual estimate (400.3 million tons) of global plastic waste, despite (in 2018) researchers only accounting for 0.15% of the world population (Featured news – Exeter scientists call for reduction in plastic lab waste – University of Exeter, 2015; Plastic waste worldwide – statistics & facts|Statista, 2024; Urbina et al., 2015; UNESCO Institute for Statistics, 2023). Currently, only ~15% of global plastic produced each year is collected for recycling; of which, just over half is successfully recycled, meaning ~6%, 24 million tons, is still disposed of (Plastic waste worldwide – statistics & facts|Statista, 2024; OECD, 2023). Plastic disposal methods vary depending on location, however the OEDC reported that in 2019 of worldwide plastic waste 22% was mismanaged or uncollected litter, 49% landfilled, 19% was incinerated, and only 9% successfully recycled (OECD, 2022). Further to the long-lasting environmental consequences of single use plastic, production and disposal contribute to CO2 emissions. Production accounts the largest proportion of the CO2 footprint of plastic products but the overall CO2 footprint is also influenced by disposal.
Although recycling some plastics can reduce their associated CO2 emissions, it is not an option for laboratory plastics. Common waste streams do not yet incorporate safe handling methods to allow the recycling of plastics that have been in contact with biological and chemical agents, meaning that plastic labware is often incinerated which further contributes to CO2 emissions (Ragazzi et al., 2023). Single use culture flasks, plates and serological pipettes are often made from polystyrene, which is less recyclable and generates more emissions (33%) than polypropylene – the material for conical centrifuge tubes (Ragazzi et al., 2023). Increased recycling and reuse of general laboratory plasticware have been shown to successfully reduce laboratory plastic waste and contribute to financial savings (Alves et al., 2021; Clancy et al., 2023), despite this, the infrastructure required to enable widescale implementation of recycling schemes is often inaccessible at first instance, minimizing the uptake.
Reducing CO2 emissions has been the focus of national and international climate goals and is an important factor of achieving climate neutrality by 2050 (2050 long-term strategy - European Commission, 2020). With laboratories accounting for a disproportionate amount of CO2 emissions, many scientists are taking action to reduce the environmental footprint research practices, demonstrating support and driving initiatives for sustainable, efficient laboratory environments (Durgan et al., 2023). Resources are available to support researchers in selecting sustainable practices. Whilst researchers are driving these grassroots changes, considering that most of these initiatives are voluntary, they add strain to already-high academic workloads, and are difficult to sustain through short-term contracts and high staff turnover. It therefore requires institutions to support these changes at a wider level, financially supporting such long-term initiatives and encouraging permanent staff into sustainability roles (Dobbelaere et al., 2022). Many universities have pledged to reduce their environmental impact across many areas, including reducing their CO2 emissions and phasing out single use plastic (LEAF-A New Approach to Achieving Laboratory Sustainability|Sustainability Exchange, 2024). A number of research institutions have joined accreditation schemes such as the Laboratory Efficiency Assessment Framework (LEAF), pioneered by sustainable UCL, which aim to reduce research related environmental impact by reducing plastic, water, energy, and general resource use (LEAF-A New Approach to Achieving Laboratory Sustainability|Sustainability Exchange, 2024; Net Zero Coalition|United Nations, 2024). Additional sustainability schemes include Green Impact, founded by students organizing for sustainability UK (SOS UK), and the non-profit organization My Green Lab. Helpful suggestions made by Alves et al. (2021) are applicable to wider practices, including chemical decontamination using Distel, rinsing, autoclaving, and re-capping of plastic tubes for reuse, saving on costs and incineration-emissions (Alves et al., 2021).
Some plasticware cannot safely be reused due to the risk of microbial contamination in tissue culture laboratories. The nutrients required to support eukaryotic cells also provide a favorable environment for a variety of bacteria and yeast which will thrive in a cell culture flask if given the opportunity, and single-use plastics come sealed, boasting a manufactures guarantee of sterility. Plastic serological pipettes are rendered non-sterile after a single use due to the need for the now-exposed external surfaces to be introduced to the internal, sterile, culture flask or reagent bottle, meaning that as soon as they are set down, they pose contamination risk. Their material, small aperture and filter also renders them unsuitable for sterilization and re-use.
Tissue culture plasticware is certified by the manufacturer to have low levels of endotoxins. Endotoxins consist of the heat-stable lipopolysaccharide (LPS) component, derived from bacterial cell walls. These are the most frequently encountered pyrogens in both the laboratory and in pharmaceutical manufacturing. Whilst autoclaving will destroy the bacteria themselves, endotoxins will remain. This can be problematic for cell culture facilities, as pyrogens may induce an inflammatory response in cells in culture, leading to oxidative stress, changes in proliferation, and changes in baseline cytokine production (Ryan, 2020; Nomura et al., 2017; Che et al., 2019). To reuse glassware for tissue culture, it must be sterilized, and endotoxins should be destroyed, termed depyrogenation. Dry heat sterilizes glassware and is more effective in destroying endotoxins than moist heat (Rashed et al., 2020). Recommended temperatures for dry heat sterilization and depyrogenation vary between 160°C and 250°C, with longer times needed at lower temperatures (Sandle, 2011).
Before plastic consumables became ubiquitous in tissue culture, glassware was routinely sterilized and reused, reintroducing these practices holds the potential to reduce plastic waste (Kilcoyne et al., 2022). In addition to landfill burden, CO2e outputs of material production, disposal, and reuse, as well as purchasing costs should be considered when deriving an alternative practice. The overall benefit of reducing single use plastic must be compared to the energy and resources required to reuse materials. Although the initial financial costs and CO2e footprint of glassware is typically higher than plasticware, additional ongoing costs and emissions are associated with its production and use (Farley and Nicolet, 2023).
To perform the comparison between traditional and sustainable cell culture practices in this study, we used Human primary pulmonary fibroblast (HPF) cells. HPFs can be used for a variety of applications such as the study of pulmonary toxicology, drug screening, and fibrosis (Phogat et al., 2023; Marwick et al., 2021). Recently, human primary cells cultured using phenotypically relevant culture systems have been increasingly recognized as advantageous. Exchanging fetal bovine serum (FBS) with human serum (HS) with the addition of cell-specific growth factors, can enhance the relevance of primary cell culture models, we therefore adopted a human-relevant methodology as derived by Bramwell et al. (2024).
Exchanging disposable plasticware for reusable glassware in cell culture is estimated to reduce CO2e footprints and single-use plastic disposal. However, if this argument alone were enough to encourage researchers to adapt their methods, single-use plastic would not remain commonplace. Lacking hands-on evidence of the successful re-introduction of glassware into routine cell-culture practices may be prevent the uptake of more sustainable cell culture practices. Additionally, concerns regarding cell viability, contamination of cultures, and changes in labor may deter users from implementing a change in tissue culture practices. We therefore set out to provide a candid and detailed account of the impact of implementing more sustainable culture practices for a tissue-culture user. We aimed to calculate the estimated CO2e footprint generated through obtaining and cleaning glassware compared with plasticware, compare the financial cost to the researcher, and evaluate any impact a change in practice caused on the proliferation rate, viability, and pro-inflammatory cytokine production of cells in culture.
Materials and equipment
Definitions
At the time of commencing this project, the comprehensive equipment required to depyrogenate glassware and utilize glass pipette tips were not yet available within our laboratory. We secured departmental funding to acquire this additional core equipment to perform this sustainable comparison. As the core equipment and subsequent start-up costs will vary from laboratory to laboratory, from this point we consider core equipment costs (oven and pipettes) separately from the replacement ‘consumables’ costs (glass bottles, lids, pipette tips, and cannisters).
During this study we consider:
‘traditional’, to refer to single-use plastic heavy cell culture methodology.
‘sustainable’, to refer to the exchange of glass bottles for 50 mL conical centrifuge tubes for aliquoting, the exchange of plastic 1 mL single-use plastic pipette tips for 1 mL glass pipette tips, and the exchange of 10 mL plastic serological pipettes for 10 mL glass pipette tips.
‘sustainable+’, to refer to the same exchanges made within the sustainable definition, with the additional re-use of plastic cell culture flasks three times.
Pipettes and pipette tips
For the purposes of comparison, a 10 mL Nichipet pipette (Nichiryo, Merck Life Sciences, Darmstadt, Germany) and 10 mL glass tips (1000–10,000 μL, Nichiryo, Merck Life Sciences, Darmstadt, Germany) were employed in parallel with a Pipetboy acu 2 (Integra, Fisher Scientific, Leicestershire, UK) and single-use plastic serological pipettes (10 mL, Sarstedt, Sarstedt Ltd., Leicester, UK). A 1 mL Nichipet pipette (Nicheryo, Merck Life Sciences, Darmstadt, Germany) and 1 mL glass tips (100–1,000 μL, Nichiryo, Merck Life Sciences, Darmstadt, Germany) were used alongside a 1 mL Pipet-Lite LTS pipette (Rainin, Mettler Toledo, Leicester, UK) and plastic pipette tips (1,000 μL, Rainin, Mettler Toledo, Leicester, UK).
Tubes and bottles
One hundred milliliter laboratory bottles (VWR, VWR, Leicestershire, UK) with heat resistant caps (Duran, VWR, Leicestershire, UK), or plastic 50 mL tubes (Sarstedt, Sarstedt Ltd., Leicester, UK), were used for the purposes of aliquoting reagents.
Oven and cannisters
Lidded stainless-steel beakers (Nickel Electro, Fisher Scientific, Leicestershire, UK) were used to store pipette tips. A dry heat oven, Sci Quip Oven-80HT (Sciquip, Sciquip Ltd., Shropshire, UK), was used for glassware sterilization and depyrogenation.
Startup costs
The total startup cost for core equipment and glass replacements for consumables was £2544.15. This includes the oven, pipettes, pipette tips, bottles, bottle lids, and cannisters. The breakdown for these was as follows: Sci Quip Oven-80HT £1,239, 100–1,000 mL pipette £409.78, 1,000–10000-ml pipette £450.54, 5 × 100 mL bottles £23.10, 5 × bottle lids £78,10 × 100–1,000 μL glass pipette tips £85.64, 10 × 100–1,000 μL glass pipette tips £101.44, 2000 mL cannister £83.69 and 1,000 mL cannister £72.96.
Methods
Definitions
As a reference point for both CO2e and financial calculations, we considered an individual researcher’s usage to be the number of iterations of passaging and subsequent requirement for glassware cleaning using the equipment obtained specifically for this project. This equates to an average of six consecutive passages, with ~6 interim media changes performed for one cell type in a month period.
Equipment cleaning sterilization and depyrogenation procedure
Glass pipette tips were submerged in 1% Virkon for 20 min then rinsed by submersion in distilled water to remove residue and collected in stainless steel cannisters. Glass bottles and lids were disinfected with Virkon, washed in a glasswasher (Miele P5883), and dried in a drying oven (SLS SLS5026). Once dry, the oven was used to heat all glassware at 180°C for 120 min to sterilize the glassware and with the view to destroy any remaining endotoxins. As seen in Figure 1.
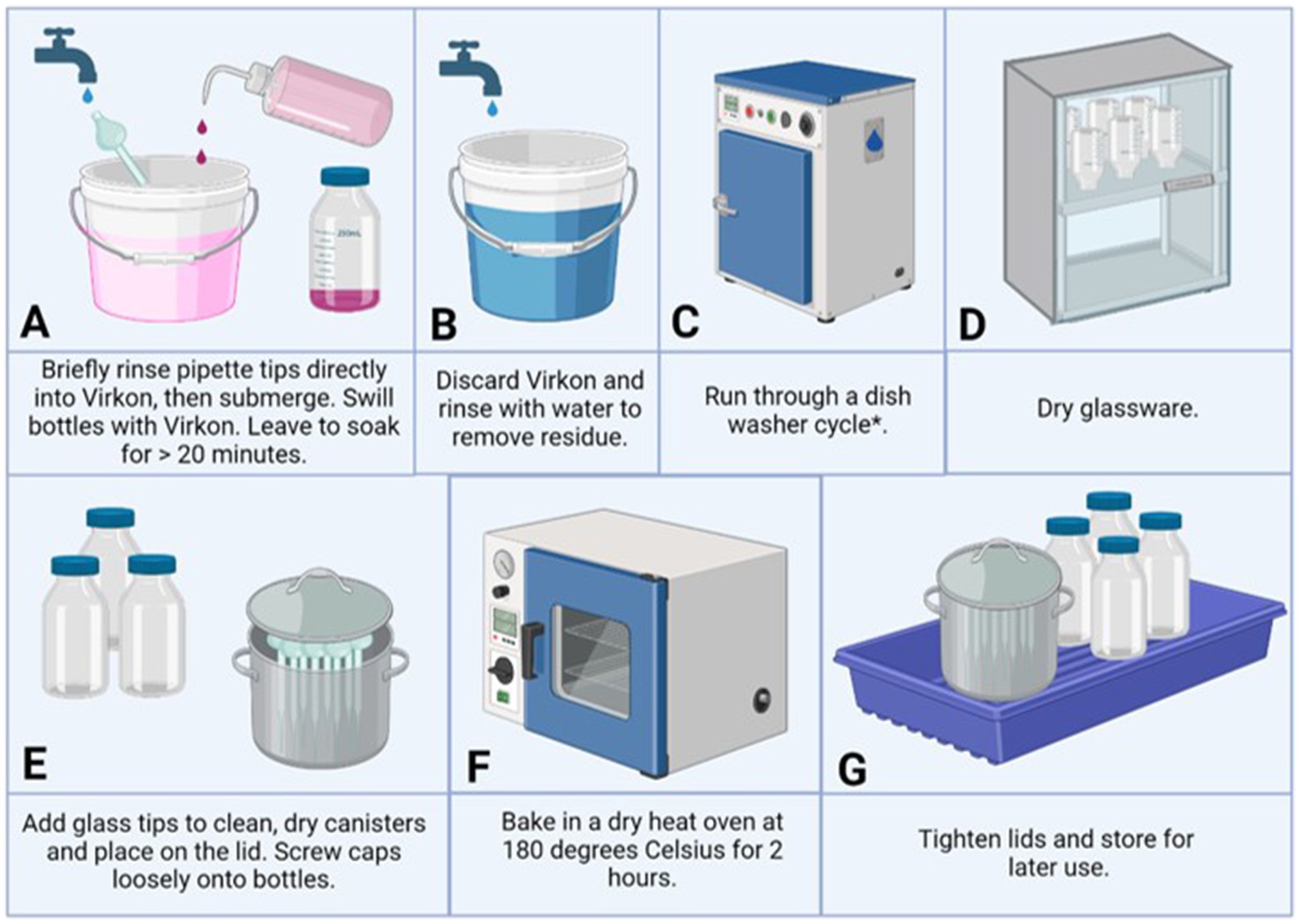
Figure 1. An overview of glassware cleaning, sterilization and depyrogenation process. Panels (A–G) demonstrate the steps taken to decontaminate (A), remove residue (B), clean (C), dry (D), prepare (E), depyrogenate (F), and store (G), glassware for reuse in cell culture practices. Created with BioRender.com. * Optional (glass pipettes were easier to hand wash).
Use of glass pipettes
Sterilized, closed cannisters containing glass pipette tips were only opened under sterile conditions. Tips were twisted onto the pipette by hand prior to use, ensuring that only the upper section of the bulb was handled. Users wore gloves, which were sprayed with 70% ethanol (EtOH) prior to any handling. After use, the pipette tip was removed via twisting, and cleaned. An overview of this process can be seen in Figure 2.
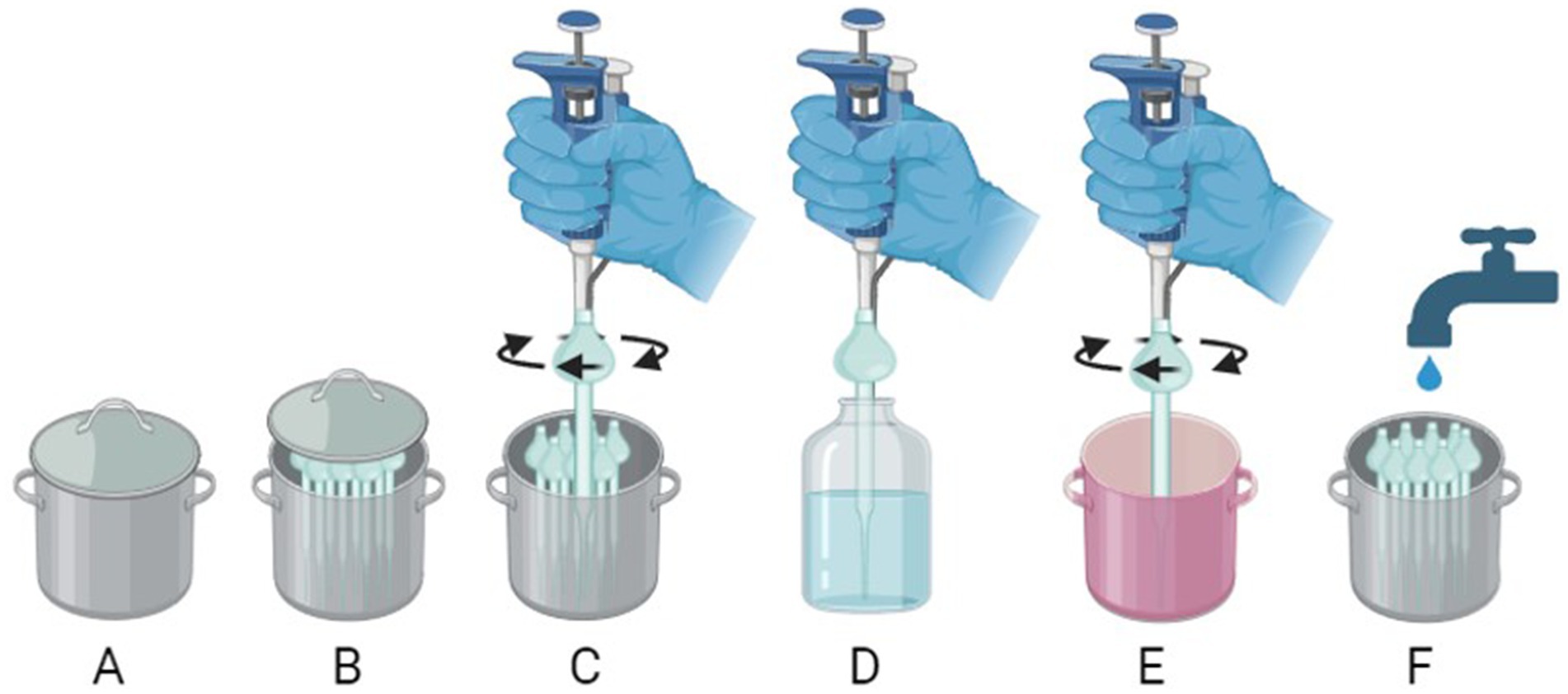
Figure 2. An overview of glass-pipette handling for sustainable cell culture. (A) Storage cannister containing pipette tips. (B) An open storage cannister revealing pre-prepared pipette tips (only to be opened under sterile conditions). (C) Applying the glass pipette tip to the pipette by twisting the opening of the tip over the silicone O-ring on the pipette (handling of the bulb may be required using gloved hands cleaned with 70% EtOH). (D) use of the pipette. (E) Removal of glass pipette tip by manual twisting and placement into Virkon. (F) Rinsing tips with water after decontamination. Created with BioRender.com.
Re-use of flasks
Sarstedt, Nümbrecht, Germany, 83.3911 were re-used for three consecutive passages. After cellular detachment, cells were removed and flasks were washed twice using 7 mL of dPBS, prior to re-seeding the passaged cells.
Cell type and culture conditions
HPFs were kindly provided by SENISCA which commercially sourced these cells from PromoCell, Heidelburg, Germany with ethical approval granted at source (catalogue number C-12360, lot number 433Z024). Cells were cultured in DMEM, 10% human serum (HS, H3667, Sigma Aldrich, Gillingham, UK), and 1 ng /ml of basic fibroblastic growth factor (bFGF), (HZ-1285, Proteintech, Manchester, UK). Cells were seeded at a density of 5.25 × 105 cells in a T75 flask.
Passaging procedure
Cells were washed in dPBS (Gibco, Fisher Scientific, Leicestershire, UK), and detached using 3 mL TrypLE (10,718,463, Gibco). Once detached, this was neutralized using 3 mL 10% human serum (HS, H3667, Sigma Aldrich) in dPBS, and the suspension was centrifuged at 500 × g for 3 min. Supernatant was discarded into Virkon, and cells were re-suspended in 1 mL of media prior to counting using a Denovix CellDrop™ and Acridine Orange - Propidium Iodide (AO/PI) viability stain (BT40039 and BT40017, Cambridge Bioscience), cells were split 1 in 3, 1/3 of the total cell were frozen down into two cryovials, in Cryo-SFM (C-29912, Promocell), and the remaining 1/3 were counted and subsequently 5.25 × 105 cells were re-seeded in a T75 flask.
The handling and equipment required to culture cells using traditional versus sustainable culture methods varied slightly. The steps taken for the preparation and cleaning of the equipment, as well as the amount of equipment required per passage according to the above passaging procedure is indicated in Table 1.
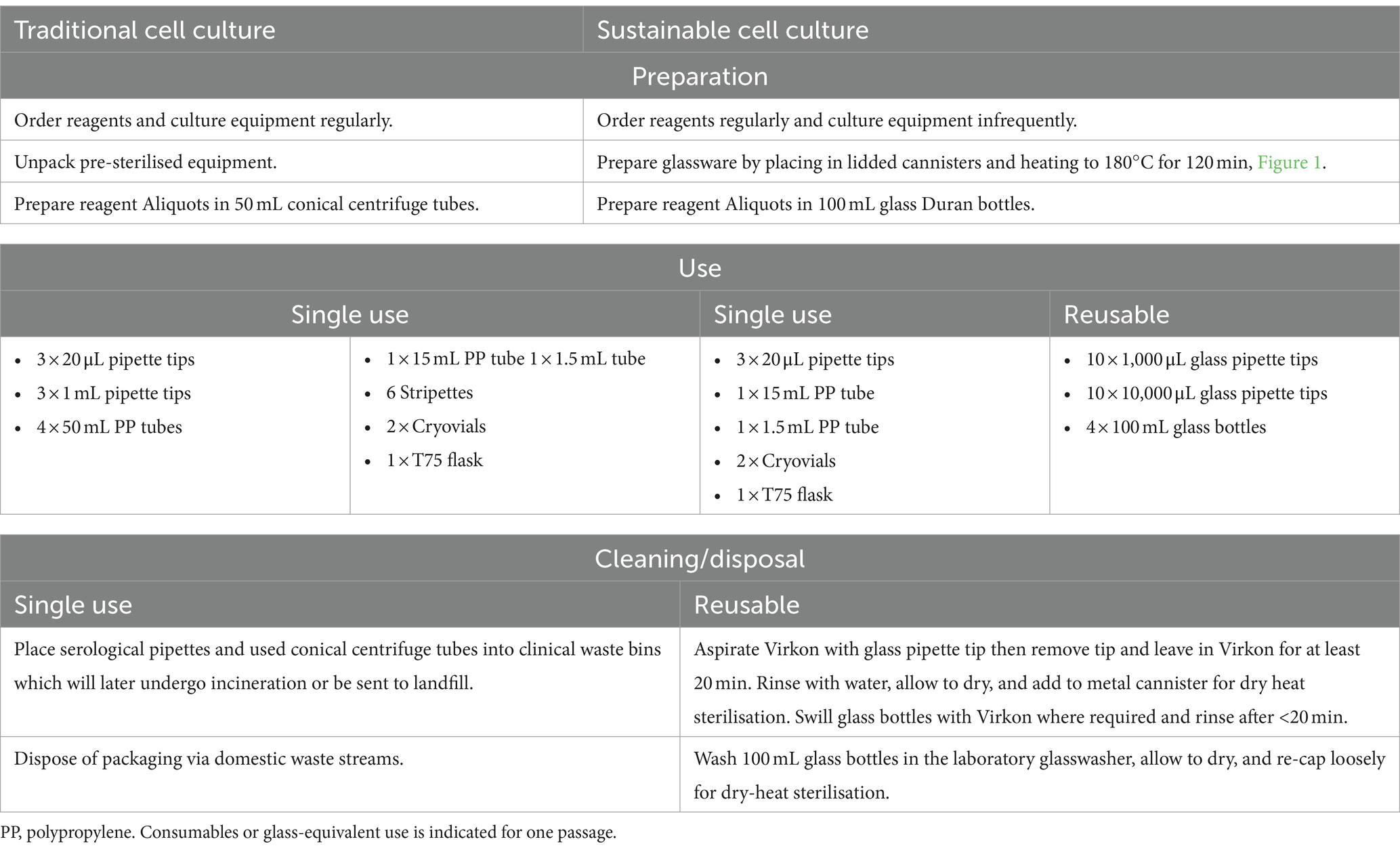
Table 1. A tabulated comparison of single and reusable equipment preparation, use, cleaning, and disposal, between the traditional and sustainable cell culture practices used in this paper.
Monitoring of cellular characteristics
Cells were routinely observed and imaged using a light microscope to check for visual signs of contamination or stress. We calculated population doubling time, average cell diameter, and viability at each passage from individual datapoints collected from seven consecutive passages to compare cells grown in traditional and sustainable culture conditions.
Cell viability
The percentage of viable cells was measured at passaging with a Denovix CellDrop™ and Acridine Orange - Propidium Iodide (AO/PI) viability stain (BT40039 and BT40017, Cambridge Bioscience).
Population doubling time
The number of viable cells per ml was measured at passaging with a Denovix CellDrop™ and Acridine Orange - Propidium Iodide (AO/PI) viability stain (BT40039 and BT40017, Cambridge Bioscience).
PDT = Population doubling time.
h = Hours between passages.
= Current cell count.
= Cell seeding density.
Cell size and morphology
Cells were imaged using a light microscope (Zeiss AxioCam ERC55 PrimoVert) to visually compare the morphology of cells cultured using glassware to cells cultured without using glassware. Cell diameter was measured with a Denovix CellDrop™
Contamination
Cultures were monitored closely for signs of contamination, including clouding of the media, paying close attention to the phenol red indicator, and microscopic evaluation at each media change and passage.
Inflammatory cytokine production
HPF cells cultured using traditional (single-use plastic heavy), sustainable (using glass pipettes instead of plastic serological pipettes, and Duran bottles instead of 50 mL conical centrifuge tubes), and sustainable+ (as with sustainable, with the additional re-use of plastic culture flasks) conditions. Media samples were taken upon changing media or passaging, every 3–5 days.
Production of IL-6 by HPF cultured using traditional and sustainable practices were quantified by sandwich ELISA, using commercially available Human IL-6 DuoSet ELISA, (R&D Systems, Abingdon, UK). Protocols were followed according to manufacturer’s instructions and compared to standard curves of recombinant human cytokines between the range of 9.38 and 600 pg./mL. Colorimetric development was determined spectrophotometrically by a PHERAstar microplate reader at 450 nm.
Reporting and statistics
A HPF culture was consecutively passaged using traditional, sustainable, or sustainable+ conditions and readouts were performed for seven consecutive passages. Datapoints were collected at each passage and considered as one biological replicate (n = 1). Cell PD time, viability, and size were calculated from one technical replicate, taken as a singular reading taken on the Denovix Cell drop, and IL-6 expression was calculated from 3 technical rep = licates from ELISA readouts.
Due to the small number of datapoints, non-parametric testing was performed on all occasions. A Mann–Whitney U test was conducted to compare traditional and sustainable culture for cell population doubling time, viability and size. Independent Mann–Whitney U tests were performed to firstly compare IL-6 production between traditional and sustainable methods to explore the impact of glassware on the cellular inflammatory response, and secondly to compare sustainable methods with sustainable+ methods, to explore the impact of single-use flasks with re-using a plastic culture flask up to three times. A p-value lower than 0.05 was considered to be statistically significant. Statistical tests and graphs were completed using Graphpad Prism version 10.0.3 for Windows (GraphPad Software, San Diego, California USA1).
CO2e footprint estimates
CO2e, or carbon dioxide equivalent, accounts for total greenhouse gases emitted, expressed in terms of the equivalent measurement of carbon dioxide. The estimated CO2 and cost saving scenarios presented in this paper were based on the usage, cleaning system, and equipment in place during this project. The number of glass and plastic consumables used were recorded over the 31-day duration of this study. These records were used to estimate the CO2e footprint of plastic consumables and glassware used during the study and to estimate differences in CO2e if glassware use continued and expanded to more researchers. The CO2e footprint of glass and plasticware production, as well as glassware reuse, was estimated using the UK government 2022 conversion factors (Department for Energy Security and Net Zero and Department for Business, Energy and Industrial Strategy, 2022). Electricity and water consumption values were based on figures provided in manufacturer equipment manuals and the consumption was scaled to the proportion of the load taken up by the equipment designated to this study.
To further compare the CO2e footprint of traditional culture to sustainable culture methods, the estimated CO2e emissions of a month of study conditions were extrapolated to a longer time scale. The CO2e footprint of sustainable culture was estimated at different points in time by adding the emissions from glassware production to total emissions from reuse, and plasticware production and incineration for the number of months. The estimated CO2e footprint of reuse includes water and electricity usage from pipette rinsing, dishwasher runs, and oven use The footprint of traditional culture was estimated as the emissions of plasticware production and incineration by month.
Financial costs
The research costs, at the level of the individual researcher were calculated to include the direct cost of equipment including plastic consumables and glass alternatives. These were compared using the 2023 list prices of the materials purchased for this study. Due to the small proportion equipment used within this trial when compared to the vast number of researchers utilizing the same laboratory facilities, and the inherent variability of facilities and estates costs depending on the energy efficiency of equipment, laboratory, institution, and region, the utilities costs were not included within our calculations. Core equipment startup costs were considered separately to replacement “consumables” costs as detailed in the materials and equipment definitions section. Subsequently, replacement glass pipette tips, cannisters, bottles, bottle lids, and supporting plasticware were included in the costs calculation.
Results
Cellular characteristics
Human pulmonary fibroblasts (HPFs) were serially passaged for seven passages under two different conditions. Firstly, cells were cultured using traditional, single-use plastic-heavy methods. Secondly, cells were cultured using sustainable methods using re-usable glassware in exchange for plastic pipette tips and Duran bottles in exchange for 50 mL conical centrifuge tubes. Finally, cells were cultured using re-usable glassware and re-using the same plastic culture flask for multiple passages for comparison with traditional single-use culture for three consecutive passages. At each passage cell counts, cell viability, and cell size was recorded for traditional and sustainable culture methods. In addition, supernatant was collected, and images were taken for morphological comparison between cells cultured for only one passage per single-use flask and cells cultured for a second and third passage in a singular culture flask.
‘Traditionally’ and ‘sustainably’ cultured cells exhibited no differences in proliferation, size, or viability across the three passages. There was no significant difference in cell population doubling (PD) time between cells cultured with glassware compared with plasticware (p = 0.4848) as indicated in Figure 3A. Population doubling time for HPFs cultured with glass was 47.83 h (SEM 2.39) and 52.15 h (SEM 4.04) for HPFs cultured with plastic. There was also no significant difference in cell size, Figure 3B (p > 0.9999) or viability, Figure 3C (p = 0.5962). Additionally, the re-use of culture flasks up to three times led to no observable differences in cellular morphology when compared to single use, as seen in Figure 4.
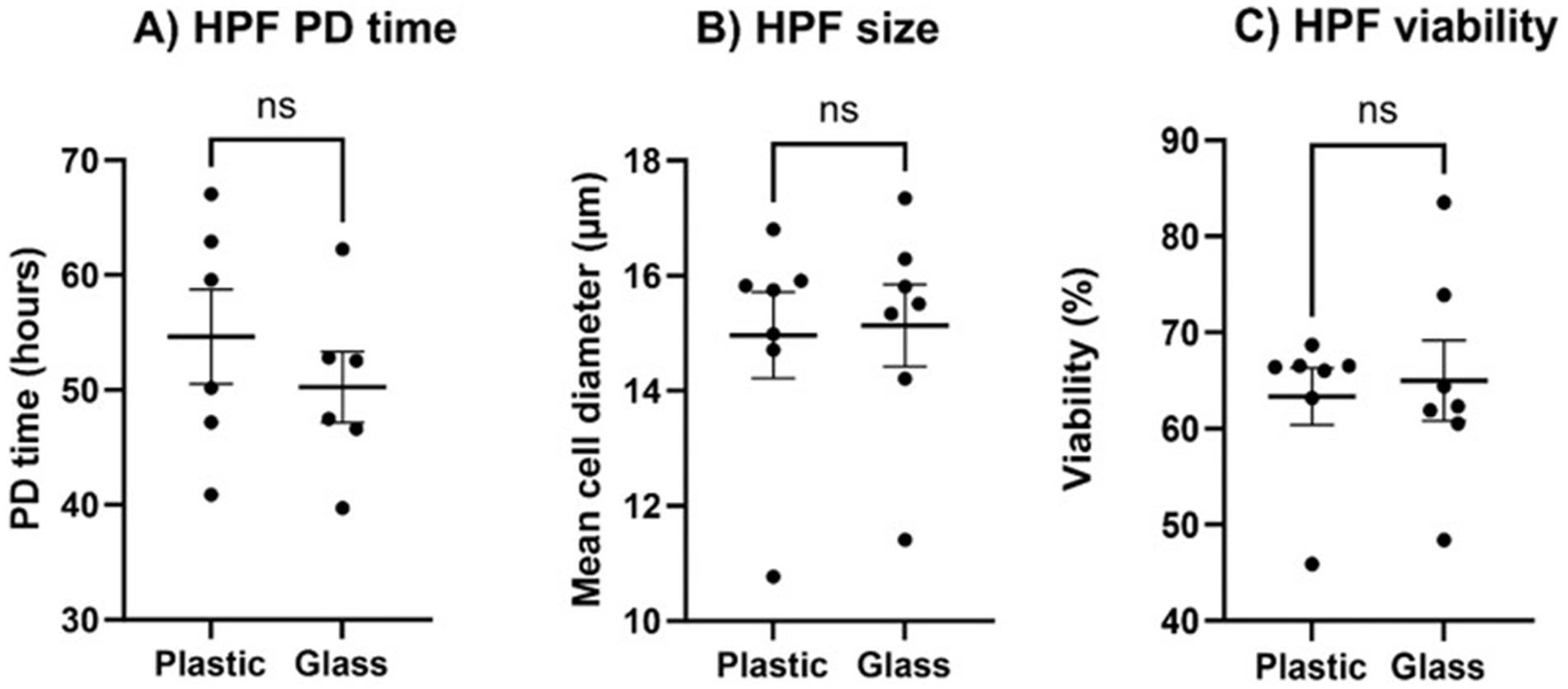
Figure 3. Key characteristics of Human Pulmonary Fibroblast (HPF) culture do not vary between cells cultured with plastic or reused glassware. (A) HPF population doubling (PD) time, (B) HPF mean cell diameter at passaging and (C) percentage of viable cells at passaging, were compared across seven consecutive passages, from p12 – p18. Each datapoint presented was calculated from a singular readout taken using a DeNovix CellDrop at each passage (n = 7). Statistical significance between cells cultured with plasticware and glassware was calculated using a Mann–Whitney U test, p-values <0.05 were considered to be statistically significant. Error bars denote the mean +/− standard error of the mean (SEM).
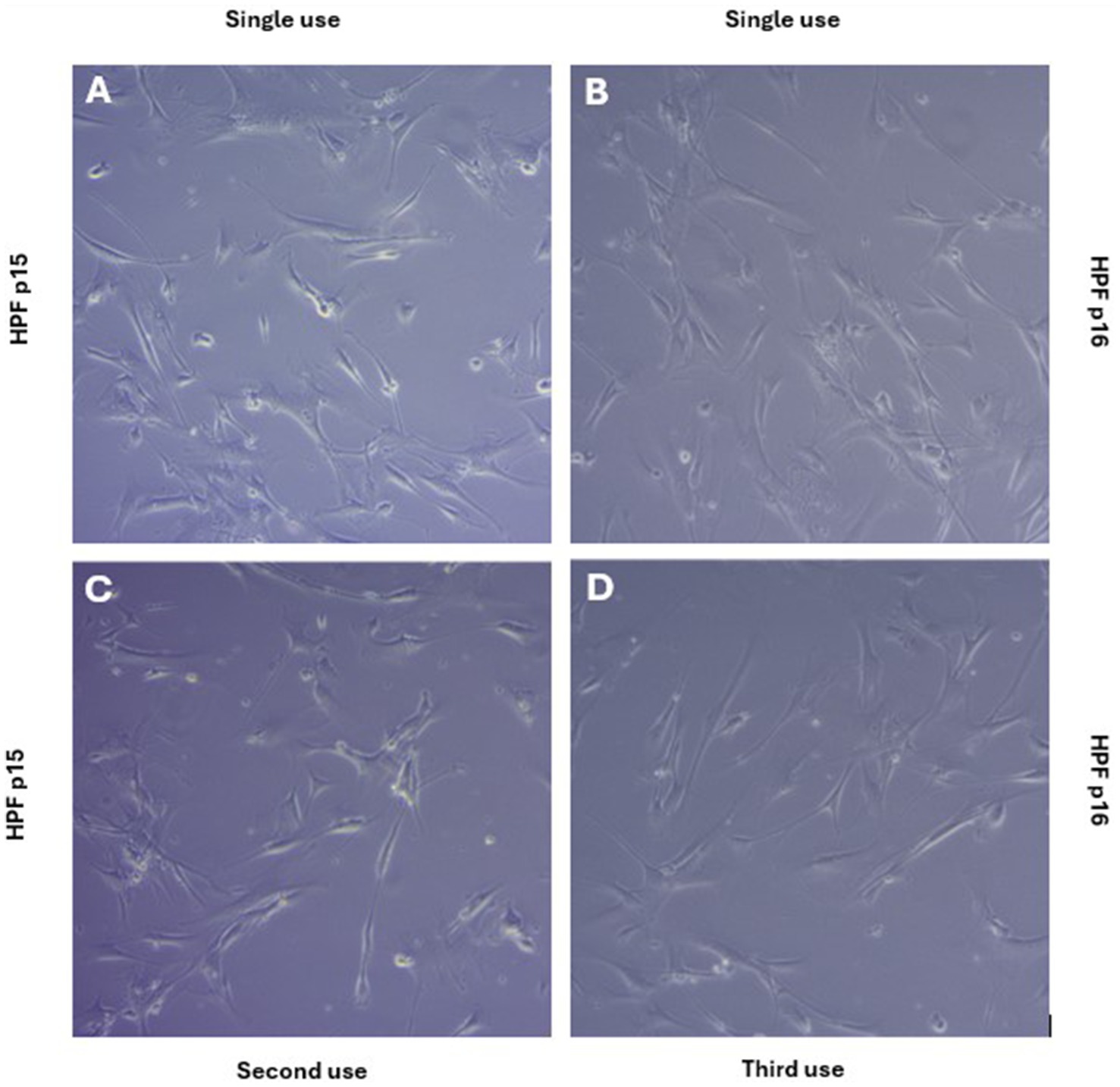
Figure 4. Human Pulmonary Fibroblasts (HPFs) are morphologically analogous when cultured in a new flask (A,B) or a reused flask (C,D) for at least three consecutive passages. Images were taken 2 days post-passaging (A,C, p15), (B,D, p16) at 100 × magnification. Contrast and colour balance was edited post-acquisition for presentation purposes.
Conditioned media collected from reused flasks was screened for IL-6 expression by ELISA. The mean concentration of IL-6 secretion did not vary significantly between HPFs cultured traditionally without glassware, nor sustainably with glassware (p = 0.9682), Figure 5. Additionally, IL-6 expression did not significantly differ between sustainable culture, and sustainable+ culture, which additionally included the reusing of a culture flask across three consecutive passages (p = 0.5135).
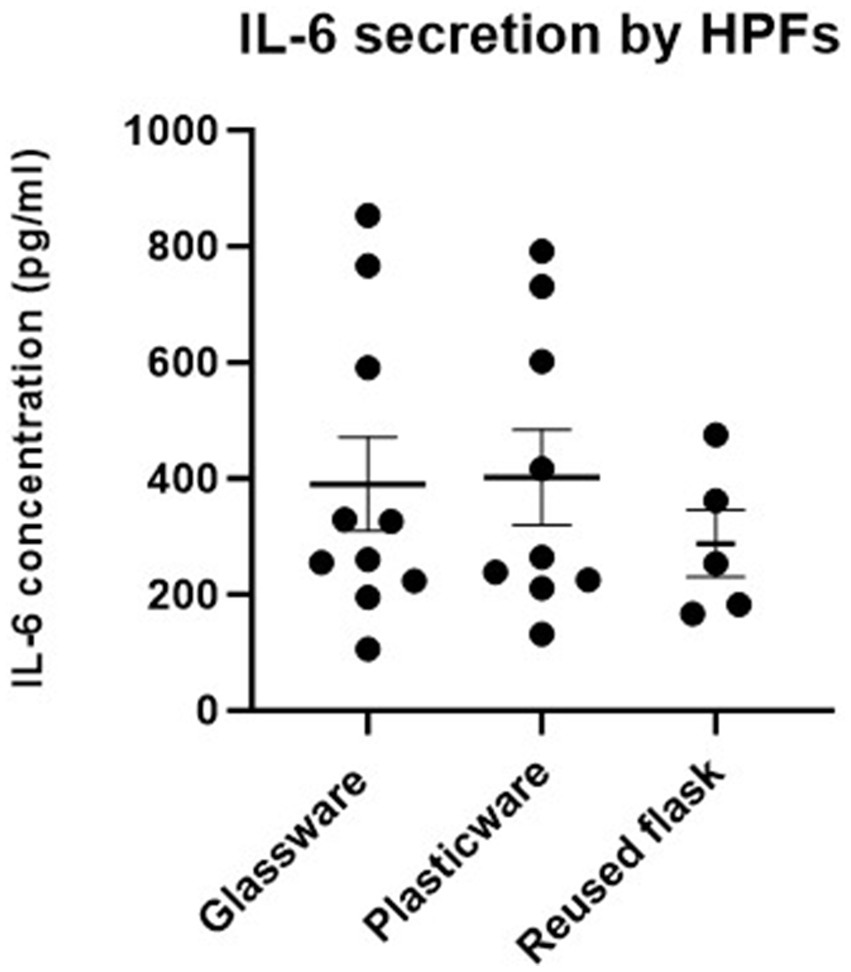
Figure 5. No significant difference in interleukin 6 secretion (IL-6) (used as a proxy for endotoxin exposure) was observed between human pulmonary fibroblasts (HPF) cultured using plasticware or glassware, nor between single-use flasks and re-used flasks. IL-6 concentration (pg/ml) was measured by sandwich ELISA, from conditioned media samples collected at, and once between, each passage, from cells cultured sustainably- using glassware (n = 7), traditionally- without glassware (n = 7), and sustainable+- with glassware and reused flasks (n = 5). Statistical significance was calculated between traditional and sustainable culture, and sustainable and sustainable+ culture using a Mann–Whitney U test. p-values <0.05 were considered as statistically significant. Error bars denote the mean +/− standard error of the mean (SEM).
Collectively, these data indicate that cellular phenotype and culture sterility are not compromised by the introduction of reusable culture ware.
CO2e footprints and projections
The CO2e footprints of culture conditions over this were estimated, considering the production of consumables, glassware reuse, and plastic incineration. These estimates were then used to compare the potential CO2e footprint overtime if culturing in the same conditions continued. During this study, glass pipettes were reused 5 times and glass bottles were reused 2 times, and culture flasks were reused three times.
The initial CO2e footprint for the production of the glassware used in sustainable culture was 8.63 kg, including the metal cannisters for pipette tips. The reuse of glassware and the production of accompanying single-use plastics were estimated to have a CO2e footprint of 3.16 kg per month. The production and incineration of plastic consumables required for traditional culture had a CO2e value of 4.11 kg per month.
If plastic pipettes were exchanged for glass pipettes but plastic tube use continued, the footprint of cell culture including glassware would become equal to cell culture with only single use plastic after 8 months, Figure 6A. If 50 mL plastic tubes were exchanged for glass bottles, it would take 42 months until the use of glassware led to a reduction in CO2e footprint, Figure 6B. The total CO2e footprint of traditional culture would surpass the CO2e footprint of the sustainable culture conditions after 10 months of culture, or 4 months if flasks were reused in addition to using glassware, Figure 6C. The CO2footprint of sustainable culture after 5 years is 21.79% lower than culturing with plastic for 5 years. This equates to 48.48 kg CO2e reduction for a single user.
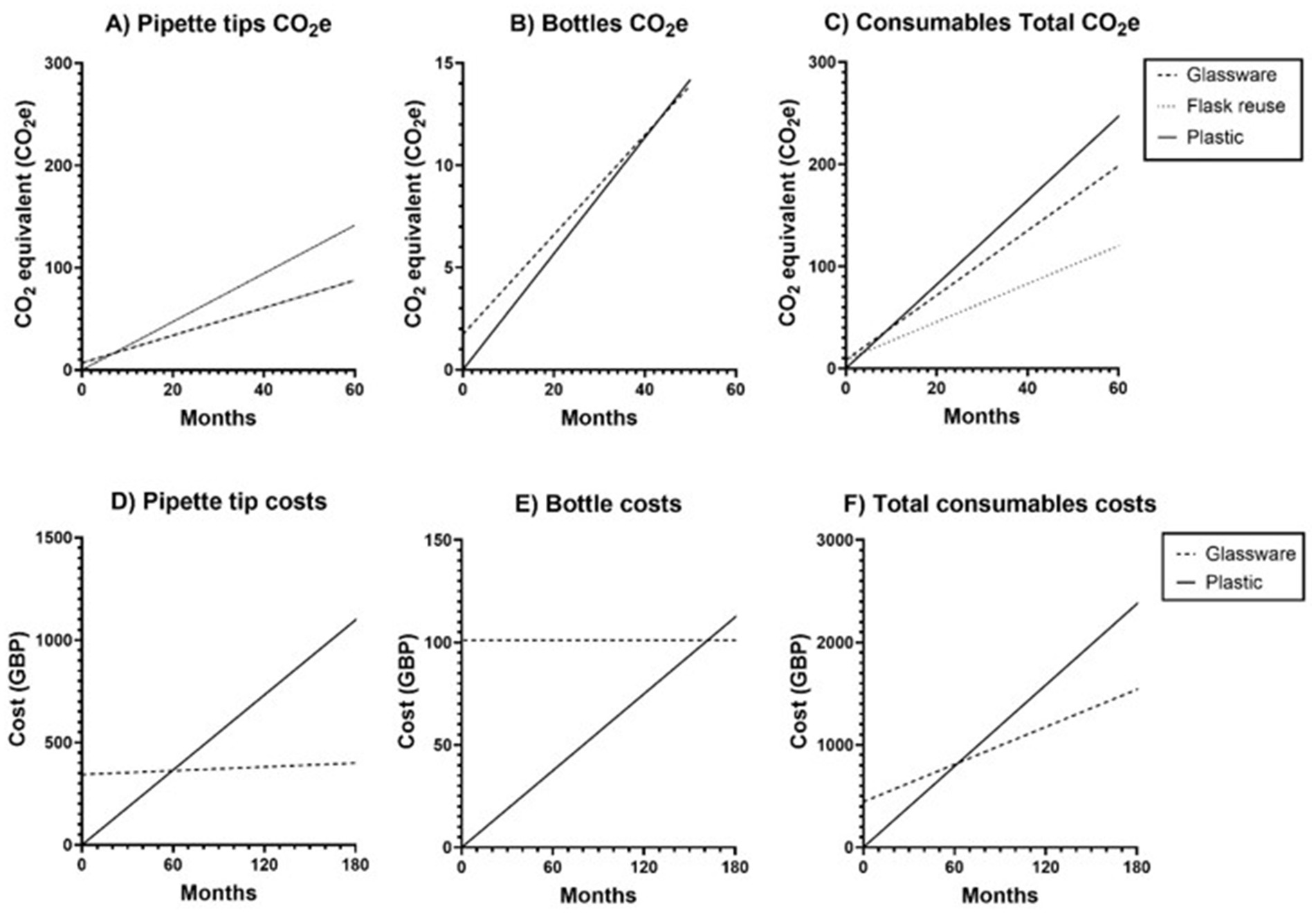
Figure 6. Over time, re-usable culture ware is more cost-effective and energy efficient than single use plasticware. Based on extrapolated usage from 1 month of human pulmonary fibroblast (HPF) culture, consisting of 6 passages and interim media changes, the CO2e and financial cost and of tissue culture plastic consumables and corresponding replacement glassware is displayed for 60 months and 180 months, respectively. This is broken down into the CO2e (A) and cost (B) of plastic pipette tips compared to the cost of 1,000 μL and 10,000 μL glass- and supporting 20 μL plastic- pipette tips, the CO2e (B) and cost (E) of 50 mL plastic conical centrifuge tubes compared with glass 100 mL bottles and heat-proof lids. Total costs are displayed in panels C and F, which demonstrate the total CO2e (C) and cost (F) of consumables used in traditional tissue culture compared with the glassware and consumables used in the sustainable culture conditions as per the methodology and equipment used within this study.
Financial costs
The cost of replacement “consumables” used in this project was £444.83 including ten 1,000 μL glass pipette tips, ten 10,000 μL glass pipette tips, cannisters, five 100 mL glass bottles and five heat resistant lids. The cost of plasticware used in sustainable culture over a month was £6.09 while the monthly cost of plasticware used in traditional culture was £13.21. Whilst the initial cost of glassware is higher than plasticware, the ongoing cost of plasticware is greater and costs equalise around the 5-year mark, making glassware cheaper beyond this point. If plastic disposable serological pipettes and 1 mL pipette tips were replaced with glass alternatives, the cost of glassware would be lower than if only plastic had been used after 60 months, Figure 6D. If plastic 50 mL tubes were exchanged for glass 100 mL bottles, it would take 162 months, Figure 6E. Substituting glassware for both tubes and pipettes would mean that the cost of only using plasticware would exceed the cost of glassware after 63 months, Figure 6F. Overall, if an individual used the same culture method with reusable glassware instead of culturing only with plasticware, £408.78 or 29.6% less would have been spent on consumables after 10 years.
Practical perspectives
Important findings from this study also included the experience, views, and narratives of the current and prospective users of the sustainable methodologies explored within this paper. Below we detail some of the concerns, challenges and successes experienced when implementing this adapted practice.
Implementing a new practice
• One concern amongst colleagues was whether changing to glassware would be time consuming and labour intensive. Whilst there is no disputing that single use plastic does require less preparation and prior planning, the hands-on time required to prepare glassware as a part of routine practice was unexpectedly minimal and the methodology used in this study required ~1 h of preparation time per week.
• Considerations made prior to incorporating any new equipment into a lab included:
The lead time on equipment.
The local infrastructure including gaining relevant approvals including PAT testing and ensuring appropriate space for the incorporation of the oven.
Ensuring appropriate storage for glassware and facilities for the cleaning of equipment.
• Additional forward planning to ensure that sterile glassware is prepared in advance. It may take practice to develop an appropriate schedule to optimize turn-over.
• Finally, the use of glass pipettes required additional care and practice. These changes remain consistent with the dexterity and attention to detail already prevalent in cell culture practice, but as with any initial training, users may wish to first practice handling ahead of time, not during critical experimentation.
Costs and resourcing
• Suitable glassware suppliers were lacking. Even previously attainable basics, such as serological pipettes were unavailable which proved time consuming when trying to source appropriate alternatives to plastics.
• Whilst glass Duran bottles are inherently heat-resistant, the availability of heat-proof lids were scarce amongst suppliers and unexpectedly costly.
• We were initially surprised to discover that glass pipettes do not come with their own heat-proof storage boxes. Sourcing cannisters that would fit the dimensions of the glass pipette tips was challenging, but possible. Depending on purchasing requirements, inexpensive cookware may offer a suitable alternative.
• We hope that more suppliers will respond to increased demand resulting from sustainability initiatives by providing a wider variety of products with more competitive prices.
Sterility
• Contamination concerns are prevalent within the research community, and subsequently antibiotics are commonplace within routine cell culture. Adapting practice may be deemed to risk compromising aseptic technique.
• All handling practice, cleaning, preparation and experimentation was performed under antibiotic-free conditions by a tissue culture novice.
• No contaminations were identified during this study. We hope this reassures researchers that adopting these more sustainable practices will not pose any significant additional risk to the sterility of cultures.
Discussion
Summary
Here, we present a successful and more sustainable alternative method to traditional cell culture. By culturing human primary fibroblast cells (HPFs) in parallel, we demonstrate that sustainable culture has no negative impact on cell morphology, viability, or population doubling times, reassuring researchers of continuity of cell culture expansion and downstream experimental outcomes when including sustainable practices. We also indicate that dry-heat sterilization of glassware for 2 h at 180°C suitably sterilized and depyrogenated glassware for the culture of HPF cells, as indicated by the lack of contamination or IL-6 production by cells cultured using sustainable glassware. We estimate that it is possible to save 105.92 kg CO2 emissions and £408.78 over a 10-year period if just one researcher, culturing one cell type, were to alter their practices to these more sustainable methodologies, not to mention the reduced landfill burden, depending on laboratory waste streams.
With the UK’s goal to reduce its carbon emissions by 45% by 2030, and reach ‘Net Zero’ by 2050, there is an increased onus on the sustainability of corporations as well as the individual (GOV.UK, 2024). Considering that the life sciences industry contributes so substantially to global CO2 emissions, universities and healthcare settings have a responsibility to evaluate how to best implement practices that reduce this negative environmental impact. Small changes in routine practice, such as the re-introduction of reusable glassware into day-to-day use, hold the potential for a widescale reduction in landfill burden from waste generated in laboratory settings.
Environmental impact
The cost and energy saving potential, due to replacing single-use plastic with reusable glassware, is dependent on the energy rating and optimal loading of individual ovens and glass washers. Maximizing the efficiency of equipment cleaning and re-use is necessary to increase environmental benefits. In this scenario, the single user, and modest glassware in use was suboptimal and equated to approximately one full load. When considering the need for timely turn-over, partial (~½) loads were often run. The estimated CO2 emissions resulting from oven use were calculated proportionally, by considering the volume of the oven occupied for the glassware used for this project, and assuming the remainder of the oven was filled with additional equipment. Partial loading of the oven would inflate CO2 emissions (5.5 kg) when compared to full oven-loads (estimated 2.25 kg). Obtaining more equipment, increasing oven users, and careful planning of equipment use maximizes the use of the oven capacity. Capacity, therefore, can reduce the monthly CO2 emissions to less than that of single use plastic (4.1 kg), when compared with incomplete loads.
Water usage also contributes to emissions, as well as affecting existing supplies of clean water. Considerations must also be given to laboratory wastewater from sustainable practice cleaning routines, which if not correctly handled may also present an additional risk to the environment when considering varying local wastewater management (Choudri et al., 2020). The quantity of water needed to reuse glassware depends on the efficiency of laboratory dishwashers, as well as other equipment or processes for rinsing glassware. Disinfectants and cleaning detergents can also have a negative environmental impact through their production and disposal, particularly if used improperly. Bulk processing of glassware and selection of reagents based on environmental and health safety, as well as effectiveness, would minimize their contribution to the environmental impact of glassware reuse.
Financial impact
The cost of sustainable equipment, including pipettes, glass bottles, and bottle lids were higher than that of the plastic consumables needed for typical culture for 1 month. However, the total cost of typical culture over time would eventually exceed the cost of the reusable stand-ins for plastic consumables. If experimental load increased for sustainable culture, within reason, glassware could be turned over for reuse more frequently, minimizing the need for additional purchases, unlike in the case of single-use plastics.
The more users in each laboratory, the more rapidly start-up costs are covered by the savings made from reducing single-use plastic purchases. We envisage that increased uptake of such sustainable culture methods could pave the way for reduced initial costs to the research group by increasing institution acquisition of dry heat ovens. We also hope that increasing customer demand drives more suppliers to re-stock glass pipettes, which may enhance competitor demand for reusable laboratory glassware. Not accounted for in this study, is the additional cost of technician time that would likely be required for the efficient wide scale rule out of re-usable glassware laboratory wide. In this study, the cleaning and sterilization of glassware added approximately 1 h of work a week to the researcher. A bulk washing system support by a technician would help to ease time burden on researchers, particularly in the case of wider scale implementation. In addition to the cost of expanding technical support, the use of water, electricity, and disinfectant needed for glassware reuse would increase core facility costs. However, once the original materials are obtained, time savings will be made due to the reduced need for stock-takes, regular ordering. Additionally, in-house glassware will reduce the risk of supply issues and lengthy lead times, reducing overall experimental timeframes.
Research impact
The scope of exchanging plastics for glass within the laboratory will vary depending on application, such as cell culture, molecular investigation and proteins to ensure adequate data measurements (Goebel-Stengel et al., 2011). In this case, we could not source glass flasks for culturing cells and opted to trial reusing plastic flasks instead, experiencing success with reusing flasks at least three times for culturing HPFs. Plastic adherent cell culture flasks come pre-treated by the manufacture to reduce the natural hydrophobicity of polystyrene and promote cellular attachment (Polystyrene (PS) Labware-UK, 2024). If researchers were to trial glass flasks, coatings such as Poly-L-Lysine or collagen may be required to promote the attachment of adherent cells, the adequate removal of these coatings may prove challenging when washing glassware for reuse (Cultureware: From Glass to Plastic-Eppendorf Australia, 2019). One clear benefit of using reusable glassware compared within reusing polystyrene or polypropylene culture-ware is the potential to autoclave and bake glassware due to its resistance to higher temperatures. Polystyrene begins to deform at ~100°C, polypropylene at ~160°C (when commercial grade and not fully isotactic) and glass at >800°C (Rieger, 1996; Polypropylene, 2024; Boyi, 2024). Additionally, glassware is more physiochemically inert and therefore resistant to degradation. Impactful research, such as with the plasticiser Bisphenol A which mimics human oestrogen, has demonstrated the endocrine disruptive capacity of plasticisers, which cannot be mediated by everyday practice (Galloway et al., 2018). Furthermore, glass is more conductive relative to plastic. This can lead to more variation in temperature at the surface level of a culture vessel, however, glass implements are typically thicker which lead to similarities in general thermal conductivity. Development focus on coatings and methods for cell culture surface suitability would be beneficial to optimize culture options using glass surfaces (La Merrill et al., 2020; Yang et al., 2011; McManus and Sharifi, 2020).
Tissue culture impact
Despite antibiotic free culture conditions within this study, no contaminations were observed, demonstrating sterility of practice in a more sustainable context. Many laboratories unnecessarily use antibiotics and antimycotics for the routine culture of human cell lines. Fear of contamination and therefore perceived time and cost savings as a result are often drivers for this (Why Use Antibiotics in Cell Culture?, 2024). However, providing proper aseptic technique is used, contamination of antibiotic-free cell culture is unlikely, and routine freezing of cell stocks provide a less environmentally hazardous failsafe than consistent antibiotic use (Hanna et al., 2023). Antibiotics have been demonstrated to interfere with cellular biological processes and their misuse is frequently reported to drive antibiotic resistance; despite this knowledge their prophylactic use in cell culture is still frequent (Mancuso et al., 2021; Hassan and Ahmad, 2020).
To reduce variability in cell culture and produce relevant and reliable results it is important to ensure that cells are not exposed to stimulatory levels of endotoxins. Endotoxin contamination can significantly impact immune cell activity and may also impact mRNA and protein expression of other cell types that respond to pathogens. IL-6 is a multifunctional cytokine and endogenous pyrogen that plays a role in generating an immune response to endotoxins (Yap et al., 2022; Tanaka et al., 2014). Fibroblasts are capable of IL-6 production in response to LPS (Che et al., 2019). When considering glassware exposed to microbes, immune cells or precious stocks of alternate cell types, future users may wish to perform a confirmatory depyrogenation testing. If using a cytokine proxy (as with IL-6), users should consider the most appropriate reporter for their cell-type (Sandle, 2011; Chaiwut and Kasinrerk, 2022; Zhang et al., 2015). Multiple commercially available tests are available which can range from the deliberate introduction and subsequent confirmation of endotoxin destruction after employing the chosen depyrogenation method. In this case, monocytes as reporter cells and to produce IL-6 in the presence of endotoxin, this is then detected by ELISA and is known as the monocyte activation test (MAT) (Solati et al., 2022). To reduce variability in cell culture and produce relevant and reliable results it is important to ensure that cells are not exposed to stimulatory levels of endotoxins. Endotoxin contamination can significantly impact immune cell activity and may also impact mRNA and protein expression of other cell types that respond to pathogens. Limulus Amoebocyte Lysate (LaL) assay is a further, more direct method, whereby Limulus lysate will coagulate in the presence of LPS (Limulus Lysate Test - an overview|ScienceDirect Topics, 2005). Endotoxin challenge vials are also a useful tool and are introduced to the oven alongside glassware, a < three-log reduction in endotoxin units is considered as suitable depyrogenation (U.S. Department of Health and Human Services, 2004). If the current parameters are deemed insufficient, depyrogenation temperature and time could be increased, or additional methods including caustic rinsing may be used (Sandle, 2013).
In our study, HPF cells cultured using glassware did not significantly increase IL-6 production when compared to their guaranteed endotoxin-free plastic counterparts. We consider this to be a good initial indication that baking the glassware for 2 h at 180°C is sufficient to degrade endotoxins. We avoided higher temperatures which may damage bottle lids and minimized baking time to 2 h to reduce environmental impact.
Wider implementation of sustainable laboratory practices
When repurposing these methods, it is important for researchers to carefully consider individual requirements for each assay type. For example, when storing reagents intended for use with RNA and DNA, which is sensitive to degradation by environmental enzymes, researchers may wish to explore further preparation of glassware such as with commercially available decontaminants such as RNAse-Zap and DNAse-Zap. At present, directly exchanging single use plastic pipette tips and 1.5 mL tubes for glassware may be inappropriate in the case of sample handling, due to an increased risk cross-contamination. Thorough, carefully validated decontamination protocols would need to be derived and applied to guarantee no cross-contamination in the case of patient samples. Additionally, depending on what is being stored within a vessel, specific decontamination and cleaning protocols may be required to safely and appropriately clean glassware that has previously contained hazardous reagents. Alternatively, some glassware may become reserved for the storage of a specific chemical to prevent the need for frequent cleaning.
Additional, more broadly applicable steps can be easily implemented to promote more sustainable practices. These could include adding additional waste streams to separate out recyclables that have not been in contact with hazardous samples or reagents, using refillable tip boxes and appropriately sized reagent vessels, minimizing the usage of gloves, and signing up to a glove recycling scheme. In addition to this, shopping wisely, by only ordering what you need; rather than bulk buying for discounts, keeping an inventory of consumables already on site, ordering equipment and reagents with a lower carbon footprint, i.e., from more local suppliers, and from companies that are mindful of their packaging and shipping practices, can have a substantial impact on CO2e emissions. Research Laboratories also require 3–10 times more energy per m2 than academic spaces, which can be reduced directly by increasing the temperature of ULT freezers from-80°C to-70°C for energy savings of ~28%, transferring DNA samples to-200C freezers with no risk of denaturing and ensuring that samples and reagents are stored only as long as necessary (U.S. EPA and U.S. Department of Energy Office of Federal Energy Management Programs, 2008; Farley et al., 2015; Wu et al., 2009). Further suggestions to improve laboratory sustainability are available in the publications by Durgan et al. (2023), Dobbelaere et al. (2022), and Greever et al. (2020).
A note to policy makers and suppliers
With wider uptake, it is small changes like those presented in this paper that may significantly reduce both CO2 emissions and the landfill burden generated by research laboratories worldwide. It should be noted that mobilizing funds for sustainable start-up equipment may be additionally difficult for those on short-term research contracts, as often with early career researchers. The upfront financial costs and concerns regarding increased time expenditure may still act as barriers for widescale uptake.
To overcome this, institutions could ensure that the basic infrastructure and core equipment is made is available to support researchers to make this sustainable transition. Included within this, we recommend that institutions consider the dedication of technician time to support glassware cleaning and equipment maintenance, enabling such practices to become routine. Additionally, small grants to obtain reusable equipment would make these practices more accessible on a wider laboratory scale.
In fast paced environments, it is important that researchers are aware of the negative impact of the single use culture and aware of suitable alternative practices that: (a) are little extra time burden (b) have no long-term additional financial cost and (c) do not compromise sterility of practice. Incentivizing the uptake of sustainable initiatives as well as reporting individual and laboratory-wide statistics on CO2e and landfill burden may further help to persuade researchers to adapt their practices. The ‘concordat for the environmental sustainability of research and innovation practice’ demonstrates the shared ambition its signatories at UK research institutions to ensure the future design and practice of UK research and innovation is environmentally sustainable (Concordat for the Environmental Sustainability of Research and Innovation Practice, 2023).
Making sustainability a stipulation for access to grants from certain funding bodies may further encourage the transition to sustainable culture practices. An example of this is Cancer research UK, who have implemented the requirement for Lead and Joint Lead applicants research groups to hold ‘LEAF’ or ‘My Green Lab’ certifications at the silver level or an equivalent scheme at the time of grant submission from January 2026 (Cancer Research UK, 2024). Accountability, Consistency and Transparency (ACT), environmental impact labelling, endorsed by ‘My Green Lab’, is a helpful way for researchers to identify the sustainability of laboratory products (ACT Home, 2024).
It would be additionally helpful if suppliers could promote and provide a broader repertoire of reusable equipment at more competitive prices to improve the accessibility of sustainable labware.
Conclusion
To challenge the use of unsustainable plasticware as default and to encourage researchers to practice more sustainable alternatives, we have empirically demonstrated the suitability and sterility of re-introducing glassware into routine cell culture. Additionally, we discuss the current challenges including sourcing equipment, upfront cost, and adapting laboratory protocols, and make recommendations to researchers, suppliers, and policy makers to ease the transition back to more sustainable practices.
In a side-by-side comparison, we observed no change in cell proliferation, viability, pro-inflammatory cytokine production, or contamination in HPFs cultured with dry heat sterilized glassware compared to cells cultured with typical single use plasticware. Incorporating glassware into an established method required little increased hands-on time, providing prior planning was used, moreover, it is calculated to result in a long-term reduction in both spending and emissions. Increased uptake of sustainable practices would improve efficiency by maximizing use of open space and optimizing turnover, as well as hopefully improving the commercial availability of glassware once more, due to increased demand.
The practices outlined in this paper are just one way in which laboratories can begin to reduce the environmental impact of their activities without having to compromise sterility or experimental reproducibility.
Data availability statement
The raw data supporting the conclusions of this article will be made available by the authors, without undue reservation.
Ethics statement
Ethical approval was not required for the studies on humans in accordance with the local legislation and institutional requirements because only commercially available established cell lines were used. Ethical approval was not required for the studies on animals in accordance with the local legislation and institutional requirements because only commercially available established cell lines were used.
Author contributions
ET: Data curation, Formal analysis, Investigation, Writing – original draft, Writing – review & editing. MD: Conceptualization, Funding acquisition, Methodology, Writing – review & editing. BS: Writing – review & editing, Resources. SG: Conceptualization, Formal analysis, Funding acquisition, Methodology, Resources, Supervision, Writing – original draft, Writing – review & editing.
Funding
The author(s) declare that financial support was received for the research, authorship, and/or publication of this article. University of Exeter Department of Clinical and Biomedical Sciences Small Equipment Grant – designed to improve infrastructure and provision within the UOE medical school laboratories which was awarded to make progress towards more sustainable laboratory practices.
Acknowledgments
The authors wish to acknowledge Jonathan Mill and Jonathan Brown for making small equipment grant funding available through the Department of Clinical and Biomedical Sciences, which enabled the purchase of the oven and glassware used within this project. The authors also wish to thank Adam Thompson for facilitating the incorporation of this equipment into our laboratory. This research was supported by the National Institute for Health and Care Research (NIHR) Exeter Biomedical Research Centre (BRC).
Conflict of interest
The authors declare that the research was conducted in the absence of any commercial or financial relationships that could be construed as a potential conflict of interest.
Publisher’s note
All claims expressed in this article are solely those of the authors and do not necessarily represent those of their affiliated organizations, or those of the publisher, the editors and the reviewers. Any product that may be evaluated in this article, or claim that may be made by its manufacturer, is not guaranteed or endorsed by the publisher.
Author disclaimer
The views expressed are those of the author(s) and not necessarily those of the NIHR or the Department of Health and Social Care.
Footnotes
References
2050 long-term strategy - European Commission . (2020) Available at: https://climate.ec.europa.eu/eu-action/climate-strategies-targets/2050-long-term-strategy_en (Accessed April 12, 2024).
ACT Home . (2024). Available at: https://act.mygreenlab.org/ (Accessed August 18, 2024).
Alves, J., Sargison, F. A., Stawarz, H., Fox, W. B., Huete, S. G., Hassan, A., et al. (2021). A case report: insights into reducing plastic waste in a microbiology laboratory. Access Microbiol. 3:173. doi: 10.1099/acmi.0.000173
Boyi, Chen F. . (2024). Exploring Glass Melting Points: What You Need to Know. Available at: https://www.boyiprototyping.com/materials-guide/glass-melting-point/ (Accessed August 16, 2024).
Bramwell, L, Gould, S, Davies, M, McMullan, C, Trusler, E, and Harries, H. (2024). An evaluation of the replacement of animal-derived biomaterials in human primary cell culture. Alternatives to laboratory animals: ATLA. Available at: https://pubmed.ncbi.nlm.nih.gov/39121342/ (Accessed August 10, 2024).
Cancer Research UK . (2024). Environmental sustainability in research. Available at: https://www.cancerresearchuk.org/funding-for-researchers/applying-for-funding/policies-that-affect-your-grant/environmental-sustainability-in-research (Accessed August 16, 2024).
Chaiwut, R., and Kasinrerk, W. (2022). Very low concentration of lipopolysaccharide can induce the production of various cytokines and chemokines in human primary monocytes. BMC. Res. Notes 15:42. doi: 10.1186/s13104-022-05941-4
Che, K. F., Sun, J., and Linden, A. (2019). Pharmacological modulation of endotoxin-induced release of IL-26 in human primary lung fibroblasts. Front. Pharmacol. 10:956. doi: 10.3389/fphar.2019.00956
Choudri, B. S., Al-Awadhi, T., Charabi, Y., and Al-Nasiri, N. (2020). Wastewater treatment, reuse, and disposal-associated effects on environment and health. Wastewater. Environ. Res. 92, 1595–1602. doi: 10.1002/wer.1406
Clancy, M., Wade, I. S., and Young, J. J. (2023). Facile methods for reusing laboratory plastic in developmental biology experiments. Differentiation 130, 1–6. doi: 10.1016/j.diff.2022.11.001
Concordat for the Environmental Sustainability of Research and Innovation Practice . (2023). Available at: https://wellcome.org/who-we-are/positions-and-statements/environmental-sustainability-concordat (Accessed August 16, 2024).
Cultureware: From Glass to Plastic-Eppendorf Australia . (2019). Available at: https://www.eppendorf.com/au-en/knowledge-hub/method-applications/cell-biology-knowledge/cultureware-from-glass-to-plastic/ (Accessed August 16, 2024).
Department for Energy Security and Net Zero and Department for Business, Energy and Industrial Strategy . (2022). Greenhouse gas reporting: conversion factors 2022. Available at: https://www.gov.uk/government/publications/greenhouse-gas-reporting-conversion-factors-2022 (Accessed April 12, 2024).
Dobbelaere, J., Heidelberger, J. B., and Borgermann, N. (2022). Achieving sustainable transformation in science – green grassroots groups need nurturing from the top. J. Cell Sci. 135. doi: 10.1242/jcs.259645
Durgan, J., Rodríguez-Martínez, M., and Rouse, B. (2023). Green labs: a guide to developing sustainable science in your organization 101, 289–301. doi: 10.1111/imcb.12624
Farley, M, McTeir, B, Arnott, A, and Evans, A. Efficient ULT freezer storage. The University of Edinburgh: Social Responsibility & Sustainability. (2015)
Farley, M., and Nicolet, B. P. (2023). Re-use of laboratory utensils reduces CO2 equivalent footprint and running costs. PLoS One 18:e0283697. doi: 10.1371/journal.pone.0283697A
Featured news – Exeter scientists call for reduction in plastic lab waste – University of Exeter . (2015). 3, 177–183. Available at: https://news-archive.exeter.ac.uk/featurednews/title_488903_en.html (Accessed April 12, 2024).
Galloway, T. S., Baglin, N., Lee, B. P., Kocur, A. L., Shepherd, M. H., Steele, A. M., et al. (2018). An engaged research study to assess the effect of a ‘real-world’ dietary intervention on urinary bisphenol a (BPA) levels in teenagers. BMJ Open 8:e018742. doi: 10.1136/bmjopen-2017-018742
Goebel-Stengel, M., Stengel, A., Taché, Y., Joseph, R., and Reeve, J. (2011). The importance of using the optimal plastic and glassware in studies involving peptides. Anal. Biochem. 414, 38–46. doi: 10.1016/j.ab.2011.02.009
GOV.UK . Environment Agency: reaching net zero. (2024). Available at: https://www.gov.uk/government/publications/environment-agency-reaching-net-zero (Accessed May 30, 2024).
Greever, C., Ramirez-Aguilar, K., and Connelly, J. (2020). Connections between laboratory research and climate change: what scientists and policy makers can do to reduce environmental impacts. FEBS Lett. 594, 3077–3239. doi: 10.1002/1873-3468.13932
Hanna, N., Tamhankar, A. J., and Stålsby, L. C. (2023). Antibiotic concentrations and antibiotic resistance in aquatic environments of the WHO Western Pacific and South-East Asia regions: a systematic review and probabilistic environmental hazard assessment. The Lancet Planetary Health. 7, e45–e54. doi: 10.1016/S2542-5196(22)00254-6
Hassan, S. N., and Ahmad, F. (2020). The relevance of antibiotic supplements in mammalian cell cultures: towards a paradigm shift. GMJ. 62, 224–230. doi: 10.4274/gulhane.galenos.2020.871
Kilcoyne, J., Bogan, Y., Duffy, C., and Hollowell, T. (2022). Reducing environmental impacts of marine biotoxin monitoring: a laboratory report. PLOS Sustain Transform 1:e0000001. doi: 10.1371/journal.pstr.0000001
La Merrill, M. A., Vandenberg, L. N., Smith, M. T., Goodson, W., Browne, P., Patisaul, H. B., et al. (2020). Consensus on the key characteristics of endocrine-disrupting chemicals as a basis for hazard identification. Nat. Rev. Endocrinol. 16, 45–57. doi: 10.1038/s41574-019-0273-8
LEAF-A New Approach to Achieving Laboratory Sustainability|Sustainability Exchange . (2024). Available at: https://www.sustainabilityexchange.ac.uk/leaf_a_new_approach_to_achieving_laboratory_sus (Accessed April 12, 2024).
Limulus Lysate Test - an overview|ScienceDirect Topics . (2005). Available at: https://www.sciencedirect.com/topics/medicine-and-dentistry/limulus-lysate-test (Accessed May 31, 2024).
Mancuso, G., Midiri, A., Gerace, E., and Biondo, C. (2021). Bacterial antibiotic resistance: the Most critical pathogens. Pathogens. 10:1310. doi: 10.3390/pathogens10101310
Marwick, J. A., Elliott, R. J. R., Longden, J., Makda, A., Hirani, N., Dhaliwal, K., et al. (2021). Application of a high-content screening assay utilizing primary human lung fibroblasts to identify Antifibrotic drugs for rapid repurposing in COVID-19 patients. SLAS Discovery. 26, 1091–1106. doi: 10.1177/24725552211019405
McManus, J. M., and Sharifi, N. (2020). Structure-dependent retention of steroid hormones by common laboratory materials. J. Steroid Biochem. Mol. Biol. 198:105572. doi: 10.1016/j.jsbmb.2019.105572
Net Zero Coalition|United Nations . (2024). Available at: https://www.un.org/en/climatechange/net-zero-coalition (Accessed May 14, 2024).
Nomura, Y., Fukui, C., Morishita, Y., and Haishima, Y. (2017). A biological study establishing the endotoxin limit for in vitro proliferation of human mesenchymal stem cells. Regenerative Therapy. 7, 45–51. doi: 10.1016/j.reth.2017.08.004
OECD . (2022). Plastic pollution is growing relentlessly as waste management and recycling fall short, says OECD. Available at: https://www.oecd.org/environment/plastic-pollution-is-growing-relentlessly-as-waste-management-and-recycling-fall-short.htm (Accessed April 12, 2024).
OECD . (2023). Towards eliminating plastic pollution by 2040 a policy scenario analysis interim findings. (2023) Available at: https://www.oecd-ilibrary.org/environment/global-plastics-outlook_de747aef-en (Accessed April 10, 2024).
Phogat, S., Thiam, F., Al Yazeedi, S., Abokor, F. A., and Osei, E. T. (2023). 3D in vitro hydrogel models to study the human lung extracellular matrix and fibroblast function. Respir. Res. 24:242. doi: 10.1186/s12931-023-02548-6
Plastic waste worldwide – statistics & facts|Statista (2024). Available at: https://www.statista.com/topics/5401/global-plastic-waste/#topicOverview (Accessed April 10, 2024).
Polypropylene . (2024). Available at: https://www.bpf.co.uk/plastipedia/polymers/PP.aspx (Accessed August 16, 2024)
Polystyrene (PS) Labware-UK . (2024). Available at: https://www.thermofisher.com/uk/en/home/life-science/lab-plasticware-supplies/plastic-material-selection/polystyrene-ps-labware.html (Accessed August 16, 2024).
Ragazzi, I., Farley, M., Jeffery, K., and Butnar, I. (2023). Using life cycle assessments to guide reduction in the carbon footprint of single-use lab consumables. PLoS Sustain Transform 2:e0000080. doi: 10.1371/journal.pstr.0000080
Rashed, A., El-Katatny, M., Hetta, A., and Hashem, Z. (2020). Validation of moist and dry heat processes used for sterilization and depyrogenation during ampoules manufacturing. J. Adv. Biomed. Pharmaceut. Sci. doi: 10.21608/jabps.2020.27282.1083
Rieger, J. (1996). The glass transition temperature of polystyrene. J. Therm. Anal. 46, 965–972. doi: 10.1007/BF01983614
Sandle, T. (2013). A comparative study of different methods for endotoxin destruction. Available at: https://www.americanpharmaceuticalreview.com/Featured-Articles/148858-A-Comparative-Study-of-Different-Methods-for-Endotoxin-Destruction/ (Accessed May 30, 2024).
Sandle, T. (2011). A practical approach to depyrogenation studies using bacterial endotoxin. Journal of GXP Compliance. 15, 90–96.
Solati, S., Zhang, T., and Timman, S. (2022). The monocyte activation test detects potentiated cytokine release resulting from the synergistic effect of endotoxin and non-endotoxin pyrogens. Innate Immun. 28, 130–137. doi: 10.1177/17534259221097948
Tanaka, T., Narazaki, M., and Kishimoto, T. (2014). IL-6 in inflammation, immunity, and disease. Cold Spring Harbor Perspect Biol. 6. doi: 10.1101/cshperspect.a016295
U.S. Department of Health and Human Services . (2004). Guidance for industry sterile drug products produced by aseptic processing current good manufacturing practice (Accessed May 30, 2024).
U.S. EPA and U.S. Department of Energy Office of Federal Energy Management Programs . (2008). Laboratories for the 21st Century: An Introduction to low–energy design. Available at: http://www.labs21century.gov (Accessed June 7, 2024).
UNESCO Institute for Statistics . (2023). Bulk data download service. Available at: https://uis.unesco.org/bdds (Accessed June 7, 2024).
Urbina, M. A., Watts, A. J. R., and Reardon, E. E. (2015). Labs should cut plastic waste too. Nature 528:479. doi: 10.1038/528479c
Why Use Antibiotics in Cell Culture? . (2024). Available at: https://www.sigmaaldrich.com/GB/en/technical-documents/technical-article/cell-culture-and-cell-culture-analysis/mammalian-cell-culture/antibiotics-in-cell-culture (Accessed May 10, 2024).
Wu, J., Cunanan, J., Kim, L., Kulatunga, T., Huang, C., and Anekella, B. (2009). SeraCare Life Sciences.
Yang, C. Z., Yaniger, S. I., Jordan, V. C., Klein, D. J., and Bittner, G. D. (2011). Most plastic products release estrogenic chemicals: a potential health problem that can be solved. Environ. Health Perspect. 119, 989–996. doi: 10.1289/ehp.1003220
Yap, J. M. G., Ueda, T., Kanemitsu, Y., Takeda, N., Fukumitsu, K., Fukuda, S., et al. (2022). Human lung fibroblasts exhibit induced inflammation memory via increased IL6 gene expression and release. Front. Immunol. 13:921728. doi: 10.3389/fimmu.2022.921728
Keywords: sustainable, reusable, leaf, cellculture, tissueculture, sustaianability, glassware, depyrogenation
Citation: Trusler EC, Davies M, Spurrier B and Gould SJ (2024) Reusable glassware for routine cell culture—a sterile, sustainable and affordable alternative to single-use plastics. Front. Sustain. 5:1447236. doi: 10.3389/frsus.2024.1447236
Edited by:
Joana Correia Prata, Cooperativa de Ensino Superior Politécnico e Universitário, PortugalReviewed by:
Joana Magalhaes, Universidade do Porto, PortugalAna Rita Ferreira, University of Aveiro, Portugal
Copyright © 2024 Trusler, Davies, Spurrier and Gould. This is an open-access article distributed under the terms of the Creative Commons Attribution License (CC BY). The use, distribution or reproduction in other forums is permitted, provided the original author(s) and the copyright owner(s) are credited and that the original publication in this journal is cited, in accordance with accepted academic practice. No use, distribution or reproduction is permitted which does not comply with these terms.
*Correspondence: Samantha J. Gould, S.Gould3@exeter.ac.uk
Emily C. Trusler, https://orcid.org/0009-0008-9485-0204
Merlin Davies, https://orcid.org/0000-0002-6951-9762
Benjamin Spurrier, https://orcid.org/0009-0000-1793-3307
Samantha J. Gould, https://orcid.org/0000-0001-9634-5772