- Department of Sociology and Anthropology, University of Fort Hare, Alice, South Africa
The rapid worldwide transition to electric vehicles (EVs), propelled by progress in lithium-ion battery (LIB) technology, brings opportunities and problems in sustainable development and resource management. This study examines how incorporating circular economy ideas and enhancing skills in EV LIB recycling can be a strategic approach to meeting the 2030 Agenda for Sustainable Development. The article explores the relationship between environmental sustainability, economic growth, and social fairness by studying EV battery recycling, the workforce skills gap, and the economic ramifications of a circular approach. Based on the existing literature, the study highlights the importance of circular economy practices in improving resource efficiency, decreasing environmental pollution, and supporting various Sustainable Development Goals (SDGs), especially those concerning responsible consumption and production (SDG 12), climate action (SDG 13), and industry, innovation, and infrastructure (SDG 9). The study highlights the significance of Education for Sustainable Development (ESD) in preparing the workforce with the essential skills to adapt to a more sustainable and circular economy. It also highlights significant obstacles in present recycling methods, such as technological limitations, legislative discrepancies, and the necessity for worldwide collaboration and standardization. The paper suggests practical policy suggestions and future research paths to improve the sustainability of EV battery recycling. The initiatives involve establishing global recycling standards, promoting circular economy models through incentives, boosting technological innovation, and facilitating international collaboration and knowledge exchange.
1 Introduction
The beginning of the 21st century has marked remarkable technical progress, with the electric vehicle (EV) industry leading this transformation. The lithium-ion battery (LIB) is crucial for advancing this growing industry because of its association with energy efficiency, high power density, and environmental sustainability (Placke et al., 2017; Fan et al., 2020). However, with the increasing use of EVs worldwide (International Energy Agency, 2023), the disposal of LIBs at the end of their life cycle is a significant challenge. This raises worries about resource scarcity, environmental contamination, and proper waste disposal. In this situation, the concepts of the circular economy and the necessity for skill development are crucial in dealing with these difficulties. The circular economy presents a sustainable alternative to the linear economy model by emphasizing waste reduction, product and material reuse, and natural system regeneration (Kirchherr et al., 2023). This method aims to reduce the environmental consequences of battery disposal (Goyal et al., 2023), utilize economic prospects, and promote sustainable growth (Godfrey, 2021).
The 2030 Agenda for Sustainable Development, unanimously agreed by all United Nations Member States in 2015, offers a common framework for promoting peace and prosperity for both people and the planet, both presently and in the future. The initiative’s core consists of the 17 Sustainable Development Goals (SDGs), which serve as a pressing call to action for all nations in a worldwide collaboration. They acknowledge that eradicating poverty and other forms of deprivation must be accompanied by initiatives that enhance health and education, diminish inequality, and stimulate economic growth. This should be done while addressing climate change and striving to conserve our oceans and forests. The recycling and re-manufacturing of EV LIBs support various SDGs, such as responsible consumption and production (SDG 12), climate action (SDG 13), and industry, innovation, and infrastructure (SDG 9) within the framework of the circular economy.
This article intends to investigate how incorporating circular economy principles and skill development can facilitate the recycling and re-manufacturing of EV LIBs to support the goals of the 2030 Agenda for Sustainable Development (Church and Wuennenberg, 2019). This review explores the opportunities and challenges of aligning EV LIB recycling with SDGs by analyzing the current landscape of EV battery recycling, identifying workforce skills gaps, and examining the economic implications of a circular approach. This article analyzed existing literature to suggest ways to improve EV battery recycling processes, promote policy changes, and emphasize the significance of education for sustainable development (ESD) in preparing a competent workforce to advance toward a more sustainable and circular economy. This introduction prepares for a thorough analysis of incorporating circular economy principles and skill development in EV LIB recycling, highlighting its importance for the 2030 Agenda for Sustainable Development. This study targets academic researchers, environmental science and engineering scholars, environmental consultants, sustainability advocates, educators, and trainers in sustainable development and engineering domains who want to enhance their knowledge of circular economy applications in the EV business. It is relevant for policymakers and industry stakeholders responsible for creating and enforcing sustainable standards and practices. The remainder of this paper is organized as follows: Section 2 outlines the material and methods. Section 3, the paper’s core, presents the results and discussions. Section 4 discusses the economic impacts of sustainable recycling practices. Following this, Section 5 offers policy recommendations and future research directions. Finally, Section 6 concludes the paper, summarizing the essential findings and reaffirming the role of circular economy and skill development in driving forward the 2030 Agenda for Sustainable Development objectives.
2 Materials and methods
This study uses an integrative review methodology to investigate how circular economy principles and skill development can be incorporated into the recycling and re-manufacturing of EV LIBs, explicitly focusing on meeting the 2030 Agenda for Sustainable Development (Church and Wuennenberg, 2019). The upcoming sections detail the research design and technique employed in this study.
2.1 Scope and selection
This study devised search phrases that combined specificity and breadth to encompass the multifaceted aspects of EV LIB recycling, circular economy, and skill development, thereby ensuring a comprehensive and inclusive literature capture. The search strings were constructed by integrating various keywords, including “EV LIB recycling” AND “circular economy principles” AND “skill enhancement”; “sustainable development goals” AND “battery recycling” AND “skill development”; “Technological Innovations” AND “EV Battery Recycling”; “EV LIB recycling” AND “circular economy principles” AND “economic impact”; Education” AND “EV Battery Recycling” AND “environment”; “EV LIB recycling” AND “circular economy principles” AND “policy.” Using Boolean operators to refine the search, the reasoning behind combining these terms was established. The operators “AND” and “OR” were utilized to ensure that the literature returned encompassed all the specified themes and to broaden the search to include all relevant studies by accounting for variations in terminology and synonyms. To ensure the inclusion of precise terms, phrases were encased in quotation marks. Wildcard characters were employed to represent unusual word endings and orthography to accommodate the global diversity of terminologies.
The search went beyond the academic databases Scopus, Web of Science, and Google Scholar to obtain a comprehensive range of pertinent insights and incorporated grey literature sources. In addition to technical documents from academic institutions and relevant governmental and non-governmental organizations, grey literature sources comprised conference proceedings, policy documents, industry reports, policy documents, theses, and dissertations. By incorporating grey literature, the review was more comprehensively strengthened by practical field insights, real-world implementations of circular economy strategies, and skill development in EV LIB recycling, as opposed to the publication bias towards positive and statistically significant results commonly observed in academic journals. The databases’ references and selected sources were cross-examined for content germane to the research objectives to identify supplementary works that the initial search may not have returned. To ensure the literature assessment was comprehensive and included seminal works in the field, a manual search was performed on the references of critical articles. Respecting the value of peer-reviewed research and the practical expertise of practitioners within the industry, incorporating grey literature offered a comprehensive perspective on the subject.
A comprehensive and systematic methodology was employed to search for and select relevant literature, which served as the basis for the subsequent thematic analysis. This enabled a thorough synthesis of data that accurately represents the present state of EV LIB recycling about skill development in line with the SDGs and a circular economy. The summary of the characteristics of the reviewed papers is shown in Figure 1.
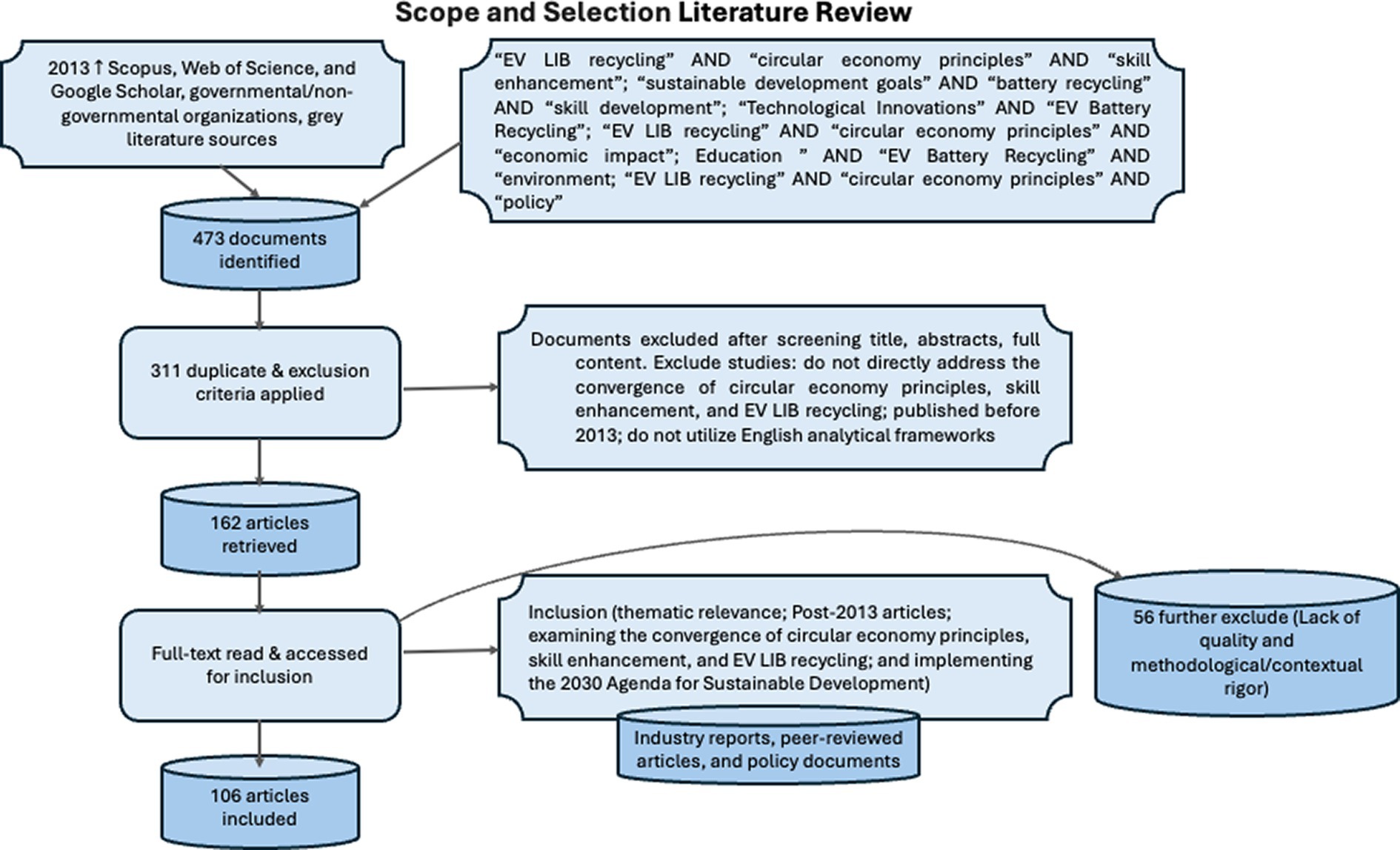
Figure 1. Integrative literature review methodology and selection method flowchart. Source: Authors.
2.2 Inclusion and exclusion criteria and literature search rationale
The purpose of developing the inclusion and exclusion criteria for this research was to guarantee that the chosen literature was both pertinent and of high quality. The review applied the following exclusion criteria:
• Studies that do not directly address the convergence of circular economy principles, skill enhancement, and EV LIB recycling help maintain the focus of the literature review on the specific topics of interest.
• Studies published before 2013 ensure that the literature review captures the latest and most relevant advancements in EV LIB recycling, circular economy principles, and skill development, considering the rapid progressions in these areas over time.
• Studies that do not utilize English analytical frameworks or present substantiating evidence for their conclusions help ensure the reliability and validity of the findings included in the review.
The criteria for inclusion comprised various aspects, such as methodological rigor, thematic relevance, and temporal relevance. Thematic relevance constituted the initial criterion. Articles specifically examining the convergence of circular economy principles, skill enhancement, and EV LIB recycling were incorporated. This included research that examined recycling technologies, models of circular economy, the necessary skills for sustainable recycling practices, and how these factors contribute to attaining the SDGs. Furthermore, the significance of temporal relevance was underscored in light of the swift progressions in EV LIB technology and circular economy methodologies. The literature search was initiated in 2013 to encompass the latest and most relevant advancements. The following factors influenced these decisions:
• Post-2013, the substantial expansion of the EV market prompted a heightened focus on battery recycling.
• Implementing the 2030 Agenda for Sustainable Development in 2015 has impacted sustainability-related research and policy trajectory, encompassing initiatives such as EV LIB recycling and the circular economy.
• The past decade has witnessed significant technological advancements in battery design and recycling processes, rendering older studies less pertinent to the present circumstances.
This methodological rigor served as a criterion for assessing the dependability of the results. Priority was given to industry reports, peer-reviewed articles, and policy documents. Additionally, the methodology of the studies was evaluated, giving preference to those that utilized English analytical frameworks and presented substantiating evidence for their conclusions. Initially, 473 documents were identified. After screening titles, abstracts, and full content for relevance, rigor, quality, timeframe, language, and duplicates, 367 articles were overall excluded, leaving 106 articles to be included.
2.3 Thematic analysis
The data for this integrative review were analyzed using the qualitative thematic analysis technique, which identifies, examines, and reports patterns (themes) throughout a dataset. This methodology is especially well-suited for scientific investigations, which aim to produce knowledge by synthesizing the discoveries of diverse texts. This method was selected based on its effectiveness in capturing the intricacies of meaning in a textual dataset concerning circular economy practices and enhancing skills in EV LIB recycling. The data analysis process commenced with an extensive gathering of qualitative data from scholarly publications relevant to the recycling of EV batteries, the principles of a circular economy, the enhancement of skills, and the SDGs. The data was understood by engaging in multiple readings, during which preliminary thoughts were recorded. This action facilitated a profound comprehension of the completeness and scope of the material. The entire dataset was subjected to systematic classification, during which portions of the text pertinent to the research question were assigned codes. This procedure played a critical role in arranging the data into significant categories. The identification of potential themes ensued after the coding process. Collecting all pertinent data for each provisional theme allowed for assessing whether the data consistently substantiated each theme. Themes underwent iterative review, wherein they were evaluated for both their integrity and their aggregate contribution to the dataset. This entailed the creation of a thematic map to depict the interconnections among themes and the analytical procedure. The essence of the patterned response or meaning within the data was extracted by refining each theme. Subsequently, the themes were designated with names that effectively communicated their fundamental nature and content. In the concluding stage, the thematic analysis was incorporated into the overarching narrative of the review. By establishing connections between the themes of the research question and the literature review, a cohesive storyline was constructed that fulfilled the research objectives and contributed to the existing knowledge on the subject.
This study uses an integrative review methodology to analyze how circular economy and skill development can improve the sustainability of EV battery recycling. It adds to the discussion on sustainable development and provides practical insights for policymakers and researchers.
3 Results and discussions
3.1 Circular economy principles: a paradigm shift
In this paper, the circular economy is conceptualized as a transformative model that transitions from the linear take-make-dispose model to a regenerative, restorative, and sustainable approach. It is based on waste reduction, product reuse, and natural system restoration. In EV LIBs recycling, the circular economy involves a comprehensive reconsideration of battery life cycles, encompassing design, manufacturing, and end-of-life strategies, focusing on optimizing resource efficiency and sustainability. Figure 2 discusses the circular economy model applied to EV LIBs recycling.
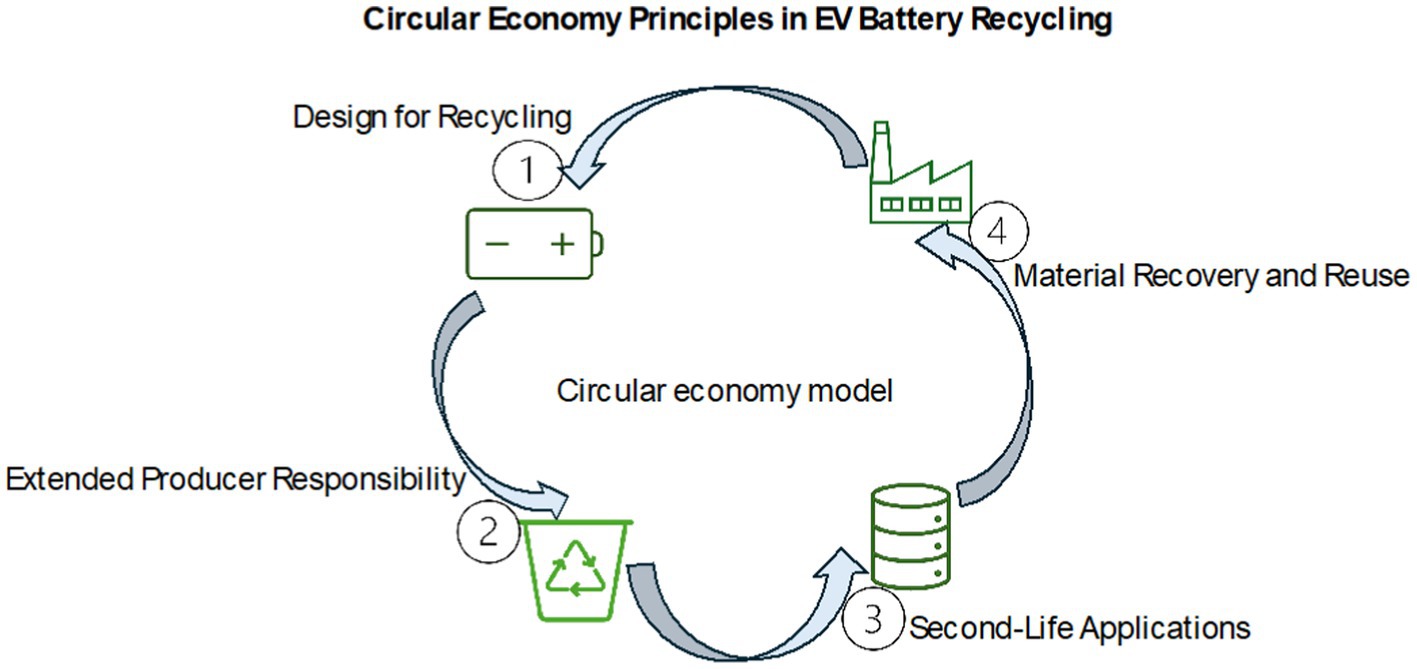
Figure 2. The key components of the circular economy of EV LIBs sustainable loop of reducing waste, reusing resources, and recycling materials. Source: Authors.
3.1.1 Key components of the circular economy EV lithium-ion battery recycling
The following mapping explains how each study element on EV LIBs aligns with the components of the circular economy.
• Design for Recycling: The implementation of circular economy principles starts at the battery design phase (Cusenza et al., 2019; Alamerew and Brissaud, 2020). Batteries are engineered for longevity, easy disassembly, and recyclability to effectively retrieve valuable materials like lithium, cobalt, and nickel (Hua et al., 2020; Zhang et al., 2018). This design concept helps reduce waste and minimizes the environmental impact of batteries throughout their lifespan.
• Extended Producer Responsibility (EPR): EPR schemes are crucial for implementing the circular economy in the EV battery sector. EPR laws incentivize manufacturers to be responsible for the whole lifecycle of their products, from production to disposal, which promotes the creation of more sustainable and recyclable batteries (Nash and Bosso, 2013; Dawson et al., 2021). This creates a market climate where sustainable design and recycling are essential components of business strategies.
• Second-Life Applications: Repurposing EV batteries into energy storage systems demonstrates maximizing product and material utilization. Repurposing batteries that are no longer suitable for vehicles extends their lifespan, delaying their disposal and decreasing the need for new raw materials (Chen et al., 2019; Abdelbaky et al., 2021).
• Material Recovery and Reuse: Advanced recycling technologies efficiently extract essential elements from used EV batteries (Jin et al., 2022; Neumann et al., 2022). This decreases dependence on extracting new resources and lessens the environmental and social effects of mining activities. Utilizing reclaimed materials in fresh battery manufacturing completes the cycle, reflecting the circular economy’s principle of material circulation (Gaines et al., 2018).
• Technological and Economic Challenges: The importance of continuous progress in overcoming obstacles to circular economy practices, such as constraints in existing recycling technology and economic feasibility (Gaines et al., 2018; Rakesh et al., 2023), is recognized.
3.1.2 Critique of circular economy principles and their application in sustainable EV battery recycling
By advocating for waste reduction and resource efficiency, applying circular economy principles to recycling EV batteries can significantly transform the industry (Baars et al., 2021; Hagelüken and Goldmann, 2022). Nevertheless, this application is not devoid of criticisms and obstacles. The present technological and economic viability of recycling processes is a pivotal concern. Although the tenet of preserving products and materials in service promotes refurbishment and remanufacturing as a means to prolong the life of batteries, not all batteries are recyclable to these procedures due to material deterioration and safety considerations (Gaines et al., 2018; Church and Wuennenberg, 2019; Mossali et al., 2020; Thompson et al., 2020; Costa et al., 2021). Moreover, eliminating waste and pollution through design frequently conflicts with the current methodologies employed in battery manufacturing and design, wherein recyclability is not consistently prioritized. The disparity above highlights a substantial obstacle to the ideal execution of the circular economy, given that the absence of uniformity in battery chemistries and designs complicates recycling endeavors, which may lead to an expansion of the ecological impact and compromise the sustainability objectives (Wang et al., 2023).
By incorporating circular economy principles into the recycling process of LIBs used in EVs, a proactive measure is taken to tackle the environmental issues that arise from the current EV growth. The present recycling techniques may be enhanced to attain a better level of circularity. Standardizing battery design is crucial for improving recycling and disassembly processes due to the necessity for consistency in battery designs. The absence of consistency in chemistries and designs hinders recycling efforts (Wang et al., 2023). Implementing modular designs would enhance the efficiency of material recovery. Moreover, it is essential to create and implement sophisticated recycling technology. Existing recycling technologies, such as pyrometallurgical and hydrometallurgical processes, are constrained by energy usage and material recovery rates. It is crucial to do more research on more effective direct recycling methods (Gaines et al., 2018). Digital tracking and traceability are essential for obtaining a greater level of circularity. Utilizing digital monitoring systems such as blockchain might improve transparency and efficiency in battery recycling, guaranteeing adherence to environmental regulations (Ni et al., 2021).
3.1.3 Financing EV LIB recycling
Financing is crucial for the feasibility and sustainability of the EV LIBs recycling sector. Assessing the economic viability of recycling LIBs from EVs involves several factors, such as initial expenditures, operational expenses, and possible income from reclaimed materials. High initial investment expenses are crucial for setting up a recycling operation. The expenditures encompass site acquisition, facility building, and procurement of specialized recycling equipment. The recycling technology for LIBs is still developing, and the selection of recycling method—pyrometallurgical, hydrometallurgical, or direct physical recycling—can have a substantial impact on the initial capital needed (Zeng et al., 2014; Beaudet et al., 2020; Yu et al., 2021; Pražanová et al., 2022). Operational costs refer to the routine expenses associated with operating the recycling operation. The expenses encompass labor, energy usage, equipment upkeep, and adherence to environmental requirements. The recycling process efficiency and hazardous materials management significantly impact operational costs (Zeng et al., 2014; Yu et al., 2021).
The primary source of income in the LIBs recycling industry comes from selling recovered minerals, including lithium, cobalt, nickel, and other precious metals. The variable pricing of these commodities might affect the profitability of the recycling business. The possibility of remanufacturing and repurposing LIBs can offer alternative revenue streams by prolonging the batteries’ lifespan before they are ultimately recycled (Chen et al., 2019; Harper et al., 2019; Hua et al., 2020; Yu et al., 2021). Government subsidies and incentives are crucial in determining the economic viability of LIBs recycling. Financial assistance can mitigate the substantial upfront and ongoing expenses, promoting recycling technology (Sun et al., 2020). An economic analysis of recycling LIBs should also account for environmental benefits like decreased greenhouse gas emissions and preservation of natural resources. These benefits can have monetary value through carbon credits and lower raw material expenses (Yu et al., 2021; Luong et al., 2022; Pražanová et al., 2022; Hantanasirisakul and Sawangphruk, 2023). Financing the EV LIBs recycling company requires evaluating the initial investment, operational expenses, income from recovered materials, remanufacturing and repurposing possibilities, government incentives, and the environmental and economic advantages.
3.2 Circular economy practices for achieving SDGs
There is a growing recognition that the incorporation of circular economy principles into the recycling of EV LIBs is an essential element in the endeavor to achieve sustainable development, specifically about the SDGs 12 and 13 established by the United Nations (Quinteros-Condoretty et al., 2021).
3.2.1 Contribution to SDG 12: responsible consumption and production
Through EV battery recycling, circular economy practices contribute directly to SDG 12 by encouraging the efficient use of resources and reducing waste. The implementation of strategies such as prioritizing recyclability in battery design, extending their operational lifespan via second-life applications, and recovering valuable materials for reutilization contribute to the reduction of raw material demands and the mitigation of environmental consequences linked to battery manufacturing and disposal (Akram and Abdul-Kader, 2023). Furthermore, implementing EPR programs guarantees that producers bear liability for the complete life cycle of their goods, thereby cultivating an atmosphere of accountability and sustainability within the sector (Church and Wuennenberg, 2019; Maitre-Ekern, 2021).
3.2.2 Contribution to SDG 13: climate action
In addition to being a significant contributor to SDG 13, implementing circular economy practices in the recycling of EV batteries is vital in the fight against climate change. Battery recycling effectively mitigates greenhouse gas emissions by decreasing the reliance on energy-intensive extraction and refining of virgin materials, which are frequently linked to substantial carbon emissions (Church and Wuennenberg, 2019). Further reducing the carbon footprint of batteries, using recycled materials in producing new batteries is typically less energy-intensive than using virgin materials (Beaudet et al., 2020). Nonetheless, a complex relationship exists between circular economy practices and achieving the SDGs. Energy and resource consumption during the recycling process must be considered in addition to the environmental benefits of recycling (Farzadkia et al., 2021). Furthermore, the potential hindrance to the advancement of SDG 10 (Reduced Inequalities) may result from the global discrepancy in recycling infrastructure and technology.
3.3 Technological innovations in EV battery recycling
Technological innovation is burgeoning in the EV battery recycling sector, with machine learning (ML) and artificial intelligence (AI) emerging as critical efficiency and sustainability drivers. These cutting-edge technologies possess the capacity to profoundly influence the sector through the improvement of diverse facets of the recycling procedure.
3.3.1 AI and ML in sorting and separation
AI and ML are bringing about a significant transformation in EV battery recycling by facilitating the sifting and segregation of battery materials and components (Zanoletti et al., 2024). Sophisticated algorithms streamline and optimize procedures, thereby substantially augmenting precision and productivity. Sorting systems propelled by artificial intelligence, for instance, rapidly distinguish and categorize various battery chemistries, materials, and components, thereby increasing material recovery rates and decreasing reliance on manual labor. Concurrently, advancements in separation and recovery methodologies are fundamentally altering the sector. Conventional approaches frequently lead to the depletion of valuable resources and the pollution of the environment. In contrast, innovative hydrometallurgical techniques, including ion exchange and solvent extraction, provide a more environmentally sustainable and selective method for retrieving materials. Utilizing these sophisticated methodologies enables the retrieval of nickel, lithium, and cobalt from depleted batteries, consequently leading to enhanced material conservation and waste minimization (Pregowska et al., 2022).
3.3.2 Material recovery and quality control
For economic viability and sustainability, recovering high-quality materials is vital in EV battery recycling. The utilization of AI and ML is crucial in the improvement of material recovery. Through parameter adjustments and data analysis spanning multiple phases of the recycling process, these technologies aim to optimize the purity and yield of materials that have been recovered (Pregowska et al., 2022). Furthermore, AI and ML are implemented in the quality control process to verify that recycled materials satisfy the necessary criteria for re-utilization in the production of batteries. The implementation of direct recycling is transforming the industry. Their structural and electrochemical properties are preserved by directly disassembling exhausted batteries and recovering cathode materials. By circumventing the energy-intensive processes of material degradation and synthesis, direct recycling preserves resources while maintaining the performance attributes of the initial cathode materials. This methodology presents an environmentally conscious substitute for traditional recycling techniques.
3.3.3 Digitalization and traceability
Incorporating digital technologies, including blockchain, AI, ML, and the Internet of Things (IoT), significantly transforms the supply chain’s transparency and traceability for EV battery recycling (Chauhan et al., 2022). Using its decentralized ledger system, blockchain technology improves traceability and transparency by documenting each transaction and motion of recycled materials. This ensures the integrity of the process and promotes the implementation of a circular economy. Traceability is enhanced by machine learning algorithms, which monitor the movement of materials during the recycling process to ensure adherence to regulations and standards (Ni et al., 2021). IoT technology facilitates data-driven insights and real-time monitoring utilizing sensors that gather significant information regarding battery health, material composition, and process efficiency to enhance recycling operations. In its entirety, digitalization in the EV battery recycling sector fosters accountability, optimizes operations, and stimulates enhanced efficiency and sustainability.
3.3.4 Challenges and opportunities for technological innovations in EV battery recycling
Although digitalization presents encouraging prospects for improving the recycling of EV batteries, various obstacles must be confronted. The preservation of data privacy and security is of the utmost importance when integrating blockchain and IoT technologies (Hassan et al., 2019). Furthermore, substantial involvement and cooperation from various parties are necessary to incorporate these digital tools into the current recycling infrastructure. However, the opportunities that digitization offers are extensive. Digital technologies can significantly contribute to the expanded recycling of EV batteries and the conscientious fulfillment of the increasing need for recycled materials through traceability, efficiency, and sustainability enhancements. The implementation of digitalization in recycling procedures will play a crucial role in establishing a battery ecosystem that is both sustainable and circular as the EV market continues to grow.
3.4 The role of education for sustainable development (ESD) in EV battery recycling
ESD assumes a critical function in providing the labor force with the necessary competencies to navigate the forthcoming labor market in the recycling of EV batteries. ESD is an instructional methodology that prioritizes incorporating sustainability principles into learning and teaching (Sukiennik et al., 2021; Scalabrino et al., 2022). Its overarching objective is to enable individuals to develop the knowledge and judgment necessary to engage in responsible behavior that safeguards the environment, ensures economic sustainability, and promotes social justice. ESD is essential for creating a workforce capable of addressing the challenges and capitalizing on the opportunities associated with sustainable recycling practices within the context of the EV battery recycling industry.
3.4.1 ESD: a primer for future skills
With an emphasis on problem-solving, engagement in teaching and learning, and the acquisition of knowledge, abilities, attitudes, and values that are essential for creating a sustainable future, ESD endeavors to equip students with the necessary capabilities (Mochizuki and Bryan, 2015; Franco et al., 2019). A sustainability-integrated curriculum in EV battery recycling distinguishes ESD from traditional educational paradigms; by doing so, it equips students with the knowledge and understanding necessary to navigate the intricacies of contemporary recycling procedures and recognize the broader ramifications of their efforts on the environment and society.
3.4.2 Integration of sustainability in technical and vocational education
Technical and vocational education and training (TVET) institutes are crucial in executing ESD (UNESCO-UNEVOC, 2018), specifically in sectors such as EV battery recycling that demand specialized technical expertise. Including sustainability principles in TVET curricula guarantees that graduates possess technical expertise (Mutebi and Kiplagat, 2022) and comprehend the ecological and societal ramifications of battery recycling. An educational approach that considers all aspects of sustainability cultivates employees with the necessary skills to effectively implement recycling practices, recover resources efficiently, and reduce environmental harm.
3.4.3 Continuous professional development and lifelong learning
Current EV battery recycling sector employees must engage in continuous professional development (CPD) due to the swift technological progress in battery design and recycling methods. Continual education regarding the most recent recycling technologies, sustainability standards, and optimal approaches to environmental management can be obtained through CPD programs that prioritize ESD (Leicht et al., 2018). The provision of lifelong learning opportunities by ESD guarantees that the workforce maintains its ability to adjust and react to the ever-changing requirements of the sector.
3.4.4 Collaborative learning and multi-stakeholder engagement
ESD stresses the significance of multi-stakeholder engagement and collaborative learning when addressing complex sustainability challenges. This entails collaborations among academic institutions, governmental bodies, industry, and non-governmental organizations to devise and implement educational and training initiatives about the recycling of EV batteries. This form of collaboration guarantees that the educational material remains pertinent to the demands of the industry and worldwide sustainability objectives (Ayala-Orozco et al., 2018).
3.4.5 Critical thinking and problem-solving skills
Critical thinking and problem-solving abilities, which are crucial for tackling the technical and environmental obstacles in EV battery recycling, are fostered through ESD. ESD promotes the progression of sustainable recycling practices (Sukiennik et al., 2021) by motivating students to engage in critical thinking regarding the environmental consequences of various recycling techniques and to devise inventive approaches to minimize waste and extract materials.
3.4.6 Implementing ESD: challenges and opportunities
There are numerous obstacles to implementing ESD within the context of EV battery recycling education and training. To begin with, the educational standards and curricula are still in the developmental stages due to the nascent industry stage. This necessitates the cooperation of policymakers, academia, and industry to guarantee their continued relevance and applicability. In regions with limited resources, requiring specialized facilities and instruments to facilitate practical training could impede the availability of high-quality education in this domain. Nevertheless, these challenges also provide prospects for inventive thinking and cooperative efforts. As an illustration, digital learning tools such as virtual reality (VR) can replicate authentic recycling situations (Rocca et al., 2020), thereby offering training experiences that are both immersive and accessible. In addition, collaborations between recycling companies and academic institutions can facilitate internships and apprenticeships (Guerreschi et al., 2023), which provide students with hands-on experience and ensure that academic curricula align with the industry’s requirements.
3.5 Economic growth and sustainable development
Creating a sustainable EV battery recycling sector demonstrates how creative environmental policies may create economic prosperity. This industry matches global environmental ideals and provides significant economic potential, including job creation, innovation, and market leadership. Below, I go over these potential economic benefits in depth, incorporating insights from current research and reports to present a whole perspective. Figure 3 provides a comparative analysis of the financial impacts of traditional and sustainable EV battery recycling. It outlines three key areas: job creation, resource security, and cost savings.
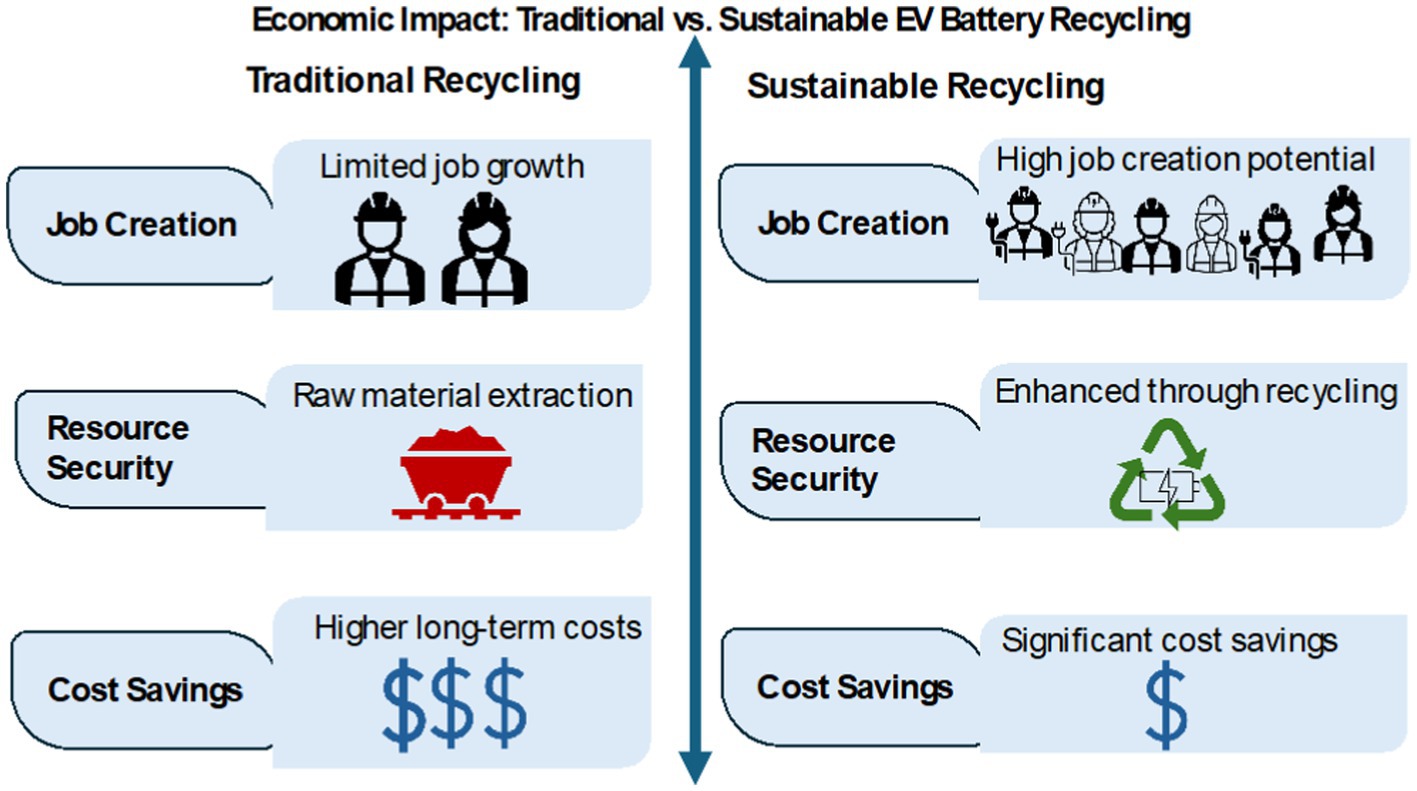
Figure 3. A comparison between traditional and sustainable recycling practices in terms of job creation, resource security, and cost savings associated with establishing a sustainable EV battery recycling industry. Source: Authors.
3.5.1 Job creation and workforce development
The EV battery recycling business is expected to produce many job opportunities along its value chain, including collecting, sorting, processing, and materials recovery. The specialized nature of these professions creates an excellent opportunity for workforce development through customized training programs that focus on skills required for sustainable recycling processes. According to the International Labour Organisation, the transition to a green economy could produce 24 million new employment globally by 2030, with sustainable businesses like EV battery recycling playing a critical part in this expansion (International Labour Organization, 2018). Furthermore, the expansion of the EV LIB recycling industry is predicted to spur job creation in a variety of sectors, contributing to economic growth and facilitating the shift to a green economy. Recycling activities benefit the economy by returning valuable materials, reducing dependency on imported goods, and promoting the development of associated businesses such as battery production and renewable energy. The expansion of the recycling industry is expected to boost overall GDP and establish a circular economy (UNIDO, 2019).
3.5.2 Resource security and cost savings
Establishing a sustainable recycling industry for EV batteries is paramount to improving resource security and attaining financial benefits. Recycling critical metals from end-of-life batteries, including lithium, cobalt, and nickel, can help the industry decrease its dependence on fundamental raw materials, most of which are obtained from geopolitically sensitive regions. This mitigates the fluctuations in the costs of primary materials and guarantees battery manufacturers a more reliable and consistent supply chain. Adopting circular economy models for the recycling of batteries has the potential to generate substantial economic advantages. The World Economic Forum, Ellen MacArthur Foundation, and McKinsey & Company (2016) estimate that by 2025, organizations could achieve annual cost savings of up to $1 trillion using decreased material expenditures. As the demand for critical metals increases and their natural reserves diminish, recycling is progressively gaining cost-effectiveness, rendering it a financially viable alternative over an extended period (Zhang et al., 2023). In addition, recycling reduces the ecological consequences associated with mining and waste disposal, resulting in financial benefits derived from healthcare expenditures, environmental restoration, and adherence to regulations (Sasmoko et al., 2022). Although recycling infrastructure and technology require a significant initial investment, the enduring advantages of resource security, price stability, and environmental sustainability more than compensate for these expenditures (Yang et al., 2023).
3.5.3 Stimulating innovation and technological advancement
EV battery recycling investments are anticipated to stimulate advancements in recycling processes and technologies, which may result in the identification of novel, sustainable materials for battery production and more effective recovery methods. The abovementioned emphasis on innovation enhances the industry’s sustainability while stimulating economic expansion by facilitating novel ventures and promoting research and development. The significance of innovation in guaranteeing the provision of vital materials for the green transition is underscored in the action plan on critical raw materials put forth by the European Commission (European Union, 2023).
3.5.4 Enhancing global competitiveness
Nations can be frontrunners in the burgeoning green economy by establishing resilient EV battery recycling capacities. Such leadership can potentially enhance global competitiveness throughout the battery value chain, encompassing energy storage solutions, manufacturing, and recycling. To support a low-carbon future, the WEF (2019) emphasizes the critical strategic significance of batteries and projects that a sustainable battery value chain may contribute $150 billion to the worldwide economy by 2030.
3.5.5 Market expansion and economic diversification
Establishing a sustainable industry for recycling EV batteries can generate economic diversification by expanding current and emerging fresh markets. The proliferation of novel offerings and solutions associated with sustainable battery technologies and recycled materials empowers enterprises to cater to the expanding consumer desire for ecologically conscious products. This, in turn, enhances the robustness of economies by diminishing their susceptibility to disruptions in conventional sectors. In addition, the expanding EV industry and the demand for energy storage solutions propel the worldwide LIB market to expand. The increasing need for these materials creates prospects for the recycling sector to provide essential resources, bolstering the battery industry’s sustainable expansion. Additionally, the volatility of critical metal prices, which can be affected by geopolitical factors, supply chain disruptions, and fluctuating demand, is mitigated by recycling LIBs. Recycling has the potential to reduce market volatility and guarantee price stability for battery manufacturers through the provision of a more consistent supply of these materials (Tankou et al., 2023).
3.5.6 Critical reflections and future directions: supporting battery recycling and local industrial development
A key challenge facing sustainable EV battery recycling is achieving social sustainability from a labor employment perspective. There is a need for research and development to support battery recycling and, simultaneously, to create local industrial development that counteracts social unsustainability from a labor employment perspective. Promoting local industrial development requires the collaboration of educational institutions, policymakers, and industry stakeholders to guarantee that battery recycling extends beyond economic and environmental benefits to include social equity (Reinhardt et al., 2019). In addition to fostering technological innovation, promoting local industrial development through research and development in EV battery recycling also addresses social sustainability by generating employment opportunities (Yu et al., 2021). Policies encouraging local industries’ expansion, such as tax incentives and subsidies for small and medium-sized enterprises (SMEs), can substantially reduce entry barriers and encourage industry involvement. Furthermore, providing state-of-the-art recycling facilities, efficient logistics networks, and establishing numerous local jobs can improve material recovery processes and reduce operational costs (Zeng et al., 2014). Incorporating ESD into technical and vocational education programs is imperative. Industry stakeholders can collaborate to develop training programs and apprenticeships that will equip the workforce with the necessary skills to fulfill the requirements of sustainable recycling practices (Wei et al., 2022). Community engagement initiatives can further guarantee that local populations benefit from these developments, promoting social equity and reducing unemployment (Hantanasirisakul and Sawangphruk, 2023).
3.6 Skill development for sustainable recycling practices
A concomitant alteration in the workforce’s skill set is imperative as the EV battery sector transitions to sustainable recycling and remanufacturing processes. An essential component of ensuring the long-term viability of the recycling industry, this integrative review scrutinizes the critical need for skill development in sustainable recycling procedures. It emphasizes the significance of such development in augmenting the effectiveness of these processes. Figure 4 illustrates the essential skills required for sustainable EV battery recycling. It depicts a flow of core competencies crucial in the industry: technical skills, safety knowledge, problem-solving abilities, and learning and adaptation.
3.6.1 Technological proficiency
Efficient EV battery recycling and remanufacturing necessitate implementing sophisticated chemical and mechanical techniques, material separation, and secure disassembly (Xiao et al., 2023). An individual in this sector must possess an extensive knowledge base encompassing battery chemistry, material science, and the technologies utilized in recycling procedures. Moreover, ongoing growth and adjustment are imperative as recycling technologies progress to sustain competitiveness and operational efficiency.
3.6.2 Environmental and safety standards
Implementing sustainable recycling practices is supported by rigorous safety and environmental regulations. Expertise in this domain guarantees that personnel can apply these criteria during recycling. These encompass the supervision of dangerous substances, the reduction of environmental pollution, and the guarantee of a secure work environment (Siqi et al., 2019). Compliance with regulations and establishing socially acceptable ecological management practices are fundamental requirements for the industry.
3.6.3 Analytical and decision-making skills
A comprehensive comprehension of material value, process efficacy, and environmental impact is necessary to make informed decisions regarding the recycling and remanufacturing of EV batteries. Employees must possess analytical abilities to evaluate the viability of recycling specific battery components, optimize material recovery rates, and make well-informed decisions regarding the end-of-life pathways for various battery types (El Jalbout and Keivanpour, 2024). This analytical capability contributes to the overarching strategic objective of optimizing recycling operations’ resource utilization while reducing carbon emissions.
3.7 Environmental impact assessment in EV battery recycling
Recycling batteries used in EVs is crucial for alleviating environmental consequences linked to their manufacturing and disposal. This is especially true about the conservation of natural resources and the reduction of carbon emissions. By their enhanced energy efficiency, advanced recycling procedures frequently achieve more significant carbon footprint reductions in battery production than the extraction and processing of virgin materials (Islam and Iyer-Raniga, 2022; Gutsch and Leker, 2024). Furthermore, as the recycling industry progresses, incorporating renewable energy sources into facilities further reduces the carbon intensity of operations, thereby contributing to an overall decrease in carbon emissions (Costa et al., 2021). According to lifecycle assessments (LCAs) conducted on recycled batteries, it is evident that emissions can be significantly diminished throughout their lifecycle, in contrast to batteries manufactured from virgin materials (Yang et al., 2024). This reduction can be attributed to the elimination of energy-intensive mining and processing operations.
3.7.1 Environmental impact of EV battery recycling: barriers
To guarantee the recycling process’s sustainability, the recycling of EV batteries poses difficult obstacles that must be overcome. The energy-intensive character of specific recycling processes, such as pyrometallurgical methods, is among the foremost obstacles. These techniques may counterbalance the environmental advantages of recycling; therefore, it is critical to advance energy-efficient technologies to optimize sustainability (Fan et al., 2020). Moreover, toxic substances, including electrolyte solvents and heavy metals, may be discharged during recycling. To mitigate these hazards and safeguard both workers and the environment, it is critical to enforce rigorous environmental regulations and safety protocols (Gaines et al., 2018). An additional obstacle pertains to the intricacy of battery chemistries and designs, which may impede the efficient recovery of materials and confound the recycling procedure. The effective resolution of the challenges currently confronting practices can be achieved by integrating energy-efficient technologies and developing advanced recycling methods (Doose et al., 2021). For instance, the implementation of human-machine hybrid modes and automation support facilitates enhanced safety protocols, reduced reliance on manual labor, and streamlined operations via the application of machine vision, AI, and robotics (Heath et al., 2022; Beghi et al., 2023; Villagrossi and Dinon, 2023). Utilizing labeling and monitoring systems guarantees the safe and efficient management of hazardous substances, thereby protecting workers throughout the recycling procedure.
Furthermore, implementing advanced sorting technologies, including sensor-based sorting and automated autonomous systems, significantly improves the precision of material separation, resulting in increased rates of recovery and decreased levels of waste (Zhu et al., 2021). By seamlessly incorporating recycling processes into the battery manufacturing supply chain, closed-loop recycling systems become crucial, promoting resource conservation and circularity. Furthermore, energy recovery systems, such as waste heat recovery and the integration of renewable energy sources, enhance energy utilization efficiency in recycling facilities, thereby reducing greenhouse gas emissions and consumption (Yu et al., 2022). By addressing current obstacles comprehensively, these solutions optimize environmental conservation efforts and the economic feasibility of recycling EV LIBs.
3.8 EV battery recycling in different regions
The challenges and opportunities of EV battery recycling vary significantly across regions, including developing countries, reflecting a complex landscape shaped by technological, regulatory, and economic factors.
3.8.1 Technological advances and recycling processes
Mechanical, hydrometallurgical, and pyrometallurgical processes present unique obstacles, including using hazardous substances, high energy consumption, and emissions. However, technological advancements are facilitating the development of recycling methods that are both more efficient and environmentally sustainable (Fan et al., 2020). Prominent nations in these technological advancements, including South Korea, Japan, China, and several European countries, employ sophisticated hydrometallurgical and pyrometallurgical methods to extract essential battery components. The environmental impact and efficiency of these processes differ.
3.8.2 Global regulatory frameworks
Globally, the regulatory environment about the recycling of EV batteries ranges considerably. Batteries are categorized as universal waste under the federal universal waste regulation of the United States Environmental Protection Agency (USEPA) (Curtis et al., 2021). In contrast, producer responsibility legislation is absent in Canada (Arnold, 2019); however, certain provinces have established recycling regulations. The European Union has implemented a Battery Directive to govern the procedures for gathering, recycling, processing, and eliminating batteries (Hoarau and Lorang, 2022). On the contrary, the NITI Aayog and Green Growth Equity Fund Technical Cooperation Facility (2022) revealed that India is implementing comprehensive regulations governing collecting and recycling all battery varieties, including LIBs.
3.8.3 Developed and developing countries: issues
The EV battery recycling sector in developed nations is enhanced by sophisticated recycling infrastructure that facilitates effective material retrieval and processing. Nevertheless, a substantial obstacle is presented by the exorbitant expenses linked to the establishment and upkeep of these facilities (Gaines et al., 2018). Robust regulatory frameworks in these areas promote and enforce environmentally and safety-conscious recycling practices, albeit with regional variations that may complicate international recycling initiatives (Harper et al., 2019). In addition, technological advancements that improve the sustainability and efficacy of recycling operations are more prevalent in developed nations’ research and development budgets (Beaudet et al., 2020).
Developing nations’ EV battery recycling sector encounters obstacles from inadequate recycling infrastructure (Jannesar Niri et al., 2024). These challenges hinder the Research Topic, separation, and processing of used batteries, reducing recycling rates and environmental contamination. In these regions, the informal recycling industry frequently provides marginalized communities with income opportunities while often failing to meet adequate safety and environmental standards. Developing nations can benefit from capacity-building initiatives and technology transfer to improve recycling infrastructure and processes. In this regard, international investment and collaboration are pivotal factors that provide support to these endeavors.
Promoting the EV battery recycling sector necessitates international cooperation, specifically concerning harmonizing recycling regulations and standards worldwide to guarantee uniformity and effectiveness in various geographical areas. It is imperative that developed and developing nations exchange information and best practices regarding EV battery recycling to surmount obstacles and capitalize on prospects. This includes the exchange of technical know-how, policy structures, and market analyses (WEF, 2023). In addition, establishing sustainable supply chains for recycled materials is crucial in pursuing a circular economy. This requires the cooperation of governments, manufacturers, and recyclers to guarantee the implementation of responsible recycling practices and ethical procurement.
Diverse regions present distinct challenges and opportunities regarding the recycling of EV batteries. Developed countries typically possess more developed infrastructure and regulatory frameworks, whereas developing countries encounter obstacles from inadequate infrastructure and informal recycling sectors (Ferronato and Torretta, 2019). To effectively leverage opportunities and confront these challenges, it is imperative to adopt a global perspective. Harnessing knowledge, promoting international cooperation, and coordinating standards are critical in pursuing sustainable recycling practices and facilitating the worldwide shift towards a circular economy.
3.9 Importance of circular economics relative to other industry considerations
Circular economics is a significant factor in the EV LIB sector, as it advocates for resource efficiency and sustainable business models. In stark contrast to linear economic models and conventional industry considerations such as swift technological adoption, immediate cost reductions, and competitive market dynamics, EV LIB offers financial and environmental benefits (Ahuja et al., 2020; Lander et al., 2021; Padmanabhan et al., 2024). Although technological advancements in the EV LIB industry frequently aim to enhance battery performance, durability, and safety (Liu et al., 2022; Roy et al., 2022; Ralls et al., 2023), they generally place product functionality above retrieving end-of-life resources (Christensen et al., 2021). On the other hand, the circular economy promotes the advancement of technologies that facilitate disassembly and material recovery (Ribeiro da Silva et al., 2023; Sánchez-García et al., 2024). This ensures that principles of reuse and recycling remain integrated throughout the lifecycle of resources, resulting in decreased waste and increased utility beyond their initial stages. However, this strategy is hindered by technological limitations, as existing recycling techniques might not completely extract all valuable components from discarded batteries (He et al., 2024; Ornes, 2024), resulting in inefficiencies and possible monetary setbacks.
The EV LIB industry is significantly impacted by short-term financial objectives motivated by competitive pricing strategies (IEA, 2024) and imminent supply and demand requirements (Jones et al., 2020; Jannesar Niri et al., 2024). These objectives frequently come at the detriment of long-term sustainability. Circular economics proposes a market framework in which products and materials are regarded as perpetual resources (Garcia-Saravia Ortiz-de-Montellano and van der Meer, 2022), which has the potential to reduce material costs and stabilize supply chains through the minimization of waste and consumption of basic materials. Nevertheless, establishing the essential infrastructure required for the successful execution of circular economy principles involves considerable initial expenditures (Nogueira et al., 2020; Godfrey, 2021), presenting a considerable economic obstacle, especially in underdeveloped areas. Moreover, regulatory compliance within the EV LIB industry predominantly pertains to fulfilling some legal requirements. It is occasionally perceived as an extra expense rather than a fundamental component of strategic deliberation. Circular economics proactively surpasses these benchmarks by implementing strategies forecasting forthcoming environmental regulations. This results in advancements in the administration of product lifecycles and end-of-life procedures. Adopting this proactive and integrative strategy ensures adherence to regulatory frameworks, which leverage them to catalyze more extensive environmental and economic transformations, promoting sustainable practices within the industry and bolstering conformance. Nonetheless, the absence of universally accepted worldwide regulations about battery recycling introduces an additional level of regulatory intricacy that may impede the worldwide execution of these methodologies, thereby impacting the industry’s capacity to expand and its integration with other nations (Prajapati, 2016; Melin et al., 2021; Lima et al., 2022).
3.10 Policy gaps and recommendations: a global perspective
A nuanced comprehension of global policies, their effectiveness, and areas requiring additional focus is necessary to integrate EV battery recycling into the 2030 Agenda for Sustainable Development. In addition to ensuring that the recycling industry contributes positively to the SDGs, these policies should seek to promote sustainable and efficient recycling practices.
3.10.1 Analysis of global existing policies
Diverse technological capacities, economic priorities, and environmental considerations are reflected in the substantial variation in recycling policies for EV batteries on a global scale (Kang et al., 2023). The Battery Directive promotes a circular economy within the European Union by minimizing the adverse environmental effects of batteries and accumulators (European Union, 2023). This directive establishes a standard for recycling efficacy by mandating battery Research Topic, recycling, and responsible disposal. Nonetheless, inadequate enforcement mechanisms and the absence of standardized parameters among member nations frequently impede the effectiveness of such policies.
On the contrary, nations such as China have enacted comprehensive battery recycling policies that extend to battery disposal and encompass the complete lifecycle of the batteries (Bej et al., n.d.). The oversight of EV manufacturers regarding the recycling of their products is mandated by regulations issued by the Ministry of Industry and Information Technology of China (Wei et al., 2022). These guidelines impose obligations on manufacturers to establish recycling channels and service outlets. Although these regulations signify a substantial advancement in sustainable battery recycling, obstacles persist regarding implementing technology, expanding recycling activities, and guaranteeing fair and impartial involvement throughout the sector.
Conversely, a comprehensive federal policy regarding the recycling of EV batteries is absent in the United States and Canada (Slattery et al., 2024). Such endeavors are predominantly propelled by private sector ingenuity and state-level regulations. The inconsistencies and inefficiencies that ensue from this fragmented approach to recycling demonstrate the absence of a unified national strategy in this regard.
3.10.2 Policy gaps
• Lack of Global Standards: In the absence of globally recognized standards for EV battery recycling, there is a significant policy gap. This discrepancy impedes the efficacy and efficiency of recycling endeavors (March et al., 2022; Slattery et al., 2024), thereby adding complexity to the exchange of recycled materials and implementing optimal methodologies.
• Insufficient Incentives for Circular Economy Models: Although certain regions do provide incentives for recycling, there is a lack of comprehensive global policies that endorse circular economy models (United Nations, n.d.), including battery leasing and battery-as-a-service.
• Inadequate Support for Technology Innovation: Frequently, current policies fail to fund research and development initiatives about recycling technologies adequately (Kang et al., 2023), thereby impeding progress in the realm of sustainable and effective recycling practices.
3.10.3 Government policies and practical steps for implementing circular economy in EV LIB recycling
By operationalizing circular economy principles in the recycling of EV LIBs, policies can aid the transition to a more resource-efficient and sustainable industry. As depicted in Figure 5, the recommendations outlined herein present a step-by-step systematic, attainable approach to surmounting obstacles and exploiting prospects within the circular economy framework based on the findings made during this review.
‘Step 1: Enforce Legislative Framework and Regulatory Compliance.’ Governments must develop and enforce a comprehensive legislative framework and regulatory compliance mechanisms to implement circular economy principles effectively in the EV LIB recycling industry. This includes creating and enforcing EPR regulations that require manufacturers to take responsibility for the entire lifecycle of their batteries—from design through to disposal. This legislation should incentivize the production of sustainable, recyclable batteries and penalize non-compliance. Implement regulations that promote uniform battery designs that facilitate easy disassembly and recycling. Standards should encourage using materials that enhance the battery’s longevity and are easier to recycle.
‘Step 2: Leverage Technological Innovation.’ The government should leverage AI and ML to enhance the precision and efficiency of sorting and separation processes in battery recycling and foster advancements in recycling technologies within the EV LIB recycling industry. Support the development and deployment of AI-driven technologies through funding and policy incentives. Promote the adoption of environmentally friendly and efficient hydrometallurgical techniques, such as ion exchange and solvent extraction, to improve the recovery of nickel, lithium, and cobalt from depleted batteries. Implement digital technologies like blockchain and IoT to enhance the transparency and traceability of the recycling process. Develop regulations that ensure these technologies’ safe and ethical use, addressing data privacy and security concerns.
‘Step 3: Integrate ESD into Curricula.’ Implementing ESD-focused technical and vocational education programs to equip students with the knowledge and skills necessary to engage in sustainable recycling practices is a practical and viable approach to surmounting the obstacles associated with EV LIB recycling. This entails establishing collaborations between academic establishments and the recycling sector to ensure that training programs meet the industry’s demands. Furthermore, it is recommended that policymakers institute ongoing professional development initiatives to ensure that the current workforce remains well-informed regarding the most recent advancements in recycling technologies and sustainability benchmarks.
‘Step 4: Implement Economic Incentives and Financial Support.’ To accelerate the adoption of circular economy practices in the EV LIB recycling industry, it is crucial to implement a range of economic incentives and financial support mechanisms. This includes providing tax incentives and subsidies to companies that invest in circular economy technologies and processes, such as reduced tax rates for engaging in sustainable practices and subsidies for developing new recycling technologies. Additionally, targeted support for SMEs is essential, including grants for upgrading equipment and bonuses to achieve specific recycling rates. Such financial measures are designed to lower the entry barriers for adopting sustainable practices and encourage more involvement in the circular economy, ultimately fostering a more sustainable industry.
‘Step 5: Boost the efficacy of the recycling industry.’ To bolster the efficacy of the recycling industry, it is crucial to focus on education and workforce development. Establishing robust training programs for workers is essential to equip them with the necessary skills to operate new recycling technologies and comply with environmental regulations effectively. Additionally, launching public awareness campaigns is vital to educate the broader community about the benefits of battery recycling and to engage both individuals and businesses in recycling initiatives. These educational efforts enhance operational efficiency by ensuring a skilled workforce and fostering a culture of sustainability through increased public participation in recycling programs.
‘Step 6: Conduct Lifecycle Assessments (LCAs).’ Perform exhaustive Life Cycle Assessments (LCAs) to assess the ecological ramifications of diverse recycling methodologies. This evaluation aims to contrast the carbon footprint and resource consumption of battery recycling with that of virgin materials. The results of this study should serve as a guide to enhancing the environmental efficacy of recycling technologies. Encourage the implementation of energy-efficient recycling techniques, including hydrometallurgical and pyrometallurgical processes, to reduce greenhouse gas emissions and energy consumption. Incorporate rigorous protocols and technologies to manage and mitigate hazardous waste generated during recycling effectively. These encompass filtration and containment mechanisms that guarantee the secure processing of hazardous substances, such as heavy metals and electrolyte solvents, without compromising the well-being of the environment or human beings.
‘Step 7: Promote Infrastructure Development.’ To enhance the recycling infrastructure for EV LIBs, promoting investment in state-of-the-art recycling facilities equipped to handle large volumes of batteries and recover a high percentage of materials is essential. Alongside this, developing and supporting robust logistics networks is crucial. These networks should be designed to facilitate the efficient Research Topic and transportation of spent batteries to recycling centers, ensuring that materials are processed promptly and effectively. By prioritizing facility upgrades and logistical improvements, the industry can significantly boost its capacity to manage waste and recover valuable materials, contributing to a more sustainable and efficient recycling system.
‘Step 8: Foster Stakeholder Engagement and International Cooperation.’ Fostering stakeholder engagement and international cooperation is essential to address the complexities of battery recycling. Creating forums and working groups that include diverse representatives from industry, government, academia, and non-profits will facilitate the discussion of challenges, sharing of best practices, and coordination of efforts nationally and internationally. Additionally, engaging in international agreements is crucial to tackling global challenges associated with battery recycling, such as managing the movement of hazardous materials and regulating the global trade of secondary raw materials. This comprehensive approach ensures that various perspectives are considered and solutions are robust and globally applicable, enhancing the effectiveness of recycling strategies worldwide.
4 Conclusion
By integrating circular economy principles and improving proficiency in recycling LIBs for EVs, a feasible strategy can be established to achieve the goals outlined in the 2030 Agenda for Sustainable Development. Circular economy principles—including waste-free design, the reuse of materials and products, and the regeneration of natural systems—define a paradigm shift in the LIB recycling industry. This review emphasizes the critical significance of these practices in fostering resource efficiency, reducing environmental damage, and advancing economic development. The development of skills is essential for the implementation of sustainable recycling practices. Within this framework, Education for Sustainable Development enables individuals to make meaningful contributions to the cause of sustainable recycling by incorporating sustainability principles into technical education and encouraging continuous learning. Additionally, recycling sustainable EV batteries has significant economic ramifications, such as creating jobs, promoting innovation, and expanding markets. To fully actualize these financial benefits, substantial investments in recycling infrastructure, regulatory uncertainty, and technological obstacles must be overcome. Advancements in these areas are impeded by policy gaps, including inadequate incentives for circular economy models and the absence of universally recognized standards.
Certain limitations are highlighted in the study, including the restricted scope of the literature review, which exclusively contains articles published after 2013. This omission fails to consider essential foundational research. Supplementing the qualitative methodology of the study with supplementary quantitative data would enable a more comprehensive examination of the economic and environmental impacts of circular economy activities. The study does not thoroughly examine the ethical ramifications of labor rights, community engagement, and social equity, notwithstanding its reference to specific social factors. Future research should prioritize exploring sustainable business models, innovative recycling and battery design approaches, and the social and economic ramifications in developed and developing countries. Nonetheless, this study makes a valuable contribution to advancing sustainable and efficient battery recycling processes that align with the goals outlined in the 2030 Agenda for Sustainable Development by focusing on the areas mentioned above.
Author contributions
BC: Conceptualization, Data curation, Formal analysis, Funding acquisition, Investigation, Methodology, Project administration, Resources, Writing – original draft, Writing – review & editing.
Funding
The author(s) declare financial support was received for the research, authorship, and/or publication of this article. This work was supported by the National Research Foundation (NRF) Reference/Grant Number: PSTD2204133322. We also acknowledge the Govan Mbeki Research and Development Centre (GMRDC) at the University of Fort Hare South Africa, and the National Institute for the Humanities and Social Sciences (NIHSS) for their financial support for this study.
Conflict of interest
The author declares that the research was conducted in the absence of any commercial or financial relationships that could be construed as a potential conflict of interest.
Publisher's note
All claims expressed in this article are solely those of the authors and do not necessarily represent those of their affiliated organizations, or those of the publisher, the editors and the reviewers. Any product that may be evaluated in this article, or claim that may be made by its manufacturer, is not guaranteed or endorsed by the publisher.
References
Abdelbaky, M., Peeters, J. R., and Dewulf, W. (2021). On the influence of second use, future battery technologies, and battery lifetime on the maximum recycled content of future electric vehicle batteries in Europe. Waste Manag. 125, 1–9. doi: 10.1016/j.wasman.2021.02.032
Ahuja, J., Dawson, L., and Lee, R. (2020). A circular economy for electric vehicle batteries: driving the change. J. Property Plann. Environ. Law 12, 235–250. doi: 10.1108/JPPEL-02-2020-0011
Akram, M. N., and Abdul-Kader, W. (2023). Sustainable development goals and end-of-life electric vehicle battery: literature review. Batteries 9:353. doi: 10.3390/batteries9070353
Alamerew, Y. A., and Brissaud, D. (2020). Modelling reverse supply chain through system dynamics for realizing the transition towards the circular economy: a case study on electric vehicle batteries. J. Clean. Prod. 254:120025. doi: 10.1016/j.jclepro.2020.120025
Ayala-Orozco, B., Rosell, J. A., Merçon, J., Bueno, I., Alatorre-Frenk, G., Langle-Flores, A., et al. (2018). Challenges and strategies in place-based multi-stakeholder collaboration for sustainability: learning from experiences in the global south. Sustainability (Switzerland) 10:3217. doi: 10.3390/su10093217
Baars, J., Domenech, T., Bleischwitz, R., and Eric Melin, H. (2021). Circular economy strategies for electric vehicle batteries reduce raw material reliance. Nat. Sustain. 4, 71–79. doi: 10.1038/s41893-020-00607-0
Beaudet, A., Larouche, F., Amouzegar, K., Bouchard, P., and Zaghib, K. (2020). Key challenges and opportunities for recycling electric vehicle battery materials. Sustainability (Switzerland) 12:5837. doi: 10.3390/su12145837
Beghi, M., Braghin, F., and Roveda, L. (2023). Enhancing disassembly practices for electric vehicle battery packs: a narrative comprehensive review. Designs 7:109. doi: 10.3390/designs7050109
Bej, S., Zhimomi, T., Hochfeld, C., Riehle, E.-B., Rather, Z. B., Rammohan, M., et al. (n.d.). International review on recycling ecosystem of electric vehicle batteries. Available at: https://changing-transport.org/wp-content/uploads/GIZ-Battery-Recycling-Report.pdf
Chauhan, C., Parida, V., and Dhir, A. (2022). Linking circular economy and digitalisation technologies: a systematic literature review of past achievements and future promises. Technol. Forecast. Soc. Chang. 177:121508. doi: 10.1016/j.techfore.2022.121508
Chen, M., Ma, X., Chen, B., Arsenault, R., Karlson, P., Simon, N., et al. (2019). Recycling end-of-life electric vehicle lithium-ion batteries. Joule 3, 2622–2646. doi: 10.1016/j.joule.2019.09.014
Christensen, P. A., Anderson, P. A., Harper, G. D. J., Lambert, S. M., Mrozik, W., Rajaeifar, M. A., et al. (2021). Risk management over the life cycle of lithium-ion batteries in electric vehicles. Renew. Sust. Energ. Rev. 148:111240. doi: 10.1016/j.rser.2021.111240
Church, C., and Wuennenberg, L. (2019). Sustainability and second life: The case for cobalt and lithium recycling.
Costa, C. M., Barbosa, J. C., Gonçalves, R., Castro, H., Campo, F. J. D., and Lanceros-Méndez, S. (2021). Recycling and environmental issues of lithium-ion batteries: advances, challenges and opportunities. Energy Storage Mater. 37, 433–465. doi: 10.1016/j.ensm.2021.02.032
Curtis, T. L., Smith, L., Buchanan, H., and Heath, G. (2021). A circular economy for Lithium-ion batteries used in Mobile and stationary energy storage: Drivers, barriers, enablers, and U.S. Policy Considerations. Available at: www.nrel.gov/publications.
Cusenza, M. A., Guarino, F., Longo, S., Ferraro, M., and Cellura, M. (2019). Energy and environmental benefits of circular economy strategies: the case study of reusing used batteries from electric vehicles. J. Energy Storage 25:100845. doi: 10.1016/j.est.2019.100845
Dawson, L., Ahuja, J., and Lee, R. (2021). Steering extended producer responsibility for electric vehicle batteries. Environ. Law Rev 23, 128–143. doi: 10.1177/14614529211006069
Doose, S., Mayer, J. K., Michalowski, P., and Kwade, A. (2021). Challenges in ecofriendly battery recycling and closed material cycles: a perspective on future lithium battery generations. Metals 11, 1–17. doi: 10.3390/met11020291
El Jalbout, S., and Keivanpour, S. (2024). Development of a body of knowledge for design for disassembly and recycling of high-tech products: a case study on lithium-ion batteries. J. Ind. Prod. Eng. 41, 19–39. doi: 10.1080/21681015.2023.2262467
European Union. (2023). Regulation of the European Parliament and of the council concerning batteries and waste batteries.
Fan, E., Li, L. W. F., Wang, Z., Lin, J., Huang, Y., Yao, Y., et al. (2020). Sustainable recycling technology for Li-ion batteries and beyond: challenges and future prospects. Chem. Rev. 120, 7020–7063. doi: 10.1021/acs.chemrev.9b00535
Farzadkia, M., Mahvi, A. H., Norouzian Baghani, A., Sorooshian, A., Delikhoon, M., Sheikhi, R., et al. (2021). Municipal solid waste recycling: impacts on energy savings and air pollution. J. Air Waste Manag. Assoc. 71, 737–753. doi: 10.1080/10962247.2021.1883770
Ferronato, N., and Torretta, V. (2019). Waste mismanagement in developing countries: a review of global issues. Int. J. Environ. Res. Public Health 16. doi: 10.3390/ijerph16061060
Franco, I., Saito, O., Vaughter, P., Whereat, J., Kanie, N., and Takemoto, K. (2019). Higher education for sustainable development: actioning the global goals in policy, curriculum and practice. Sustain. Sci. 14, 1621–1642. doi: 10.1007/s11625-018-0628-4
Gaines, L., Richa, K., and Spangenberger, J. (2018). Key issues for Li-ion battery recycling. MRS Energy Sustain. Rev. J. 5, 1–14. doi: 10.1557/mre.2018.13
Garcia-Saravia Ortiz-de-Montellano, C., and van der Meer, Y. (2022). A theoretical framework for circular processes and circular impacts through a comprehensive review of indicators. Global J. Flexible Syst. Manage. 23, 291–314. doi: 10.1007/s40171-022-00300-5
Goyal, M., Singh, K., and Bhatnagar, N. (2023). Circular economy conceptualization for lithium-ion batteries-material procurement and disposal process. Chem. Eng. Sci. 281:119080. doi: 10.1016/j.ces.2023.119080
Guerreschi, A., Piras, L., and Heck, F. (2023). Barriers to efficient knowledge transfer for a holistic circular economy: insights towards green job developments and training for young professionals. Youth 3, 553–578. doi: 10.3390/youth3020038
Gutsch, M., and Leker, J. (2024). Costs, carbon footprint, and environmental impacts of lithium-ion batteries – from cathode active material synthesis to cell manufacturing and recycling. Appl. Energy 353:122132. doi: 10.1016/j.apenergy.2023.122132
Hagelüken, C., and Goldmann, D. (2022). Recycling and circular economy—towards a closed loop for metals in emerging clean technologies. Miner. Econ. 35, 539–562. doi: 10.1007/s13563-022-00319-1
Hantanasirisakul, K., and Sawangphruk, M. (2023). Sustainable reuse and recycling of spent Li-ion batteries from electric vehicles: chemical, environmental, and economical perspectives. Glob. Chall. 7. doi: 10.1002/gch2.202200212
Harper, G., Sommerville, R., Kendrick, E., Driscoll, L., Slater, P., Stolkin, R., et al. (2019). Recycling lithium-ion batteries from electric vehicles. Nature 575, 75–86. doi: 10.1038/s41586-019-1682-5
Hassan, M. U., Rehmani, M. H., and Chen, J. (2019). Privacy preservation in blockchain based IoT systems: integration issues, prospects, challenges, and future research directions. Futur. Gener. Comput. Syst. 97, 512–529. doi: 10.1016/j.future.2019.02.060
He, B., Zheng, H., Tang, K., Xi, P., Li, M., Wei, L., et al. (2024). A comprehensive review of Lithium-ion battery (LiB) recycling technologies and industrial market trend insights. Recycling 9. doi: 10.3390/recycling9010009
Heath, G. A., Ravikumar, D., Hansen, B., and Kupets, E. (2022). A critical review of the circular economy for lithium-ion batteries and photovoltaic modules–status, challenges, and opportunities. J Air Waste Manag Assoc 72, 478–539. doi: 10.1080/10962247.2022.2068878
Hoarau, Q., and Lorang, E. (2022). An assessment of the European regulation on battery recycling for electric vehicles. Energy Policy 162:112770. doi: 10.1016/j.enpol.2021.112770
Hua, Y., Zhou, S., Huang, Y., Liu, X., Ling, H., Zhou, X., et al. (2020). Sustainable value chain of retired lithium-ion batteries for electric vehicles. J. Power Sources 478:228753. doi: 10.1016/j.jpowsour.2020.228753
IEA. (2024). Global EV outlook 2024: Moving towards increased affordability. Available at: www.iea.org
International Energy Agency. (2023). Global EV outlook 2023: Catching up with climate ambitions. Available at: www.iea.org
International Labour Organization. (2018). World employment and social outlook 2018: Greening with jobs.
Islam, M. T., and Iyer-Raniga, U. (2022). Lithium-ion battery recycling in the circular economy: a review. Recycling 7. doi: 10.3390/recycling7030033
Jannesar Niri, A., Poelzer, G. A., Zhang, S. E., Rosenkranz, J., Pettersson, M., and Ghorbani, Y. (2024). Sustainability challenges throughout the electric vehicle battery value chain. Renewable Sustainable Energy Rev. 191:114176. doi: 10.1016/j.rser.2023.114176
Jin, S., Mu, D., Lu, Z., Li, R., Liu, Z., Wang, Y., et al. (2022). A comprehensive review on the recycling of spent lithium-ion batteries: urgent status and technology advances. J. Cleaner Prod. 340:130535. doi: 10.1016/j.jclepro.2022.130535
Jones, B., Elliott, R. J. R., and Nguyen-Tien, V. (2020). The EV revolution: the road ahead for critical raw materials demand. Appl. Energy 280:115072. doi: 10.1016/j.apenergy.2020.115072
Kang, Z., Huang, Z., Peng, Q., Shi, Z., Xiao, H., Yin, R., et al. (2023). Recycling technologies, policies, prospects, and challenges for spent batteries. iScience 26:108072. doi: 10.1016/j.isci.2023.108072
Kirchherr, J., Yang, N. H. N., Schulze-Spüntrup, F., Heerink, M. J., and Hartley, K. (2023). Conceptualizing the circular economy (revisited): an analysis of 221 definitions. Resour. Conserv. Recycl. 194:1–32. doi: 10.1016/j.resconrec.2023.107001
Lander, L., Cleaver, T., Rajaeifar, M. A., Nguyen-Tien, V., Elliott, R. J. R., Heidrich, O., et al. (2021). Financial viability of electric vehicle lithium-ion battery recycling. IScience 24:102787. doi: 10.1016/j.isci.2021.102787
Leicht, A., Heiss, J., and Byun, W. J.UNESCO (2018). Issues and trends in education for sustainable development. France: UNESCO Publishing.
Lima, M. C. C., Pontes, L. P., Vasconcelos, A. S. M., de Araujo Silva Junior, W., and Wu, K. (2022). Economic aspects for recycling of used Lithium-ion batteries from electric vehicles. Energies 15, 1–19. doi: 10.3390/en15062203
Liu, W., Placke, T., and Chau, K. T. (2022). Overview of batteries and battery management for electric vehicles. Energy Rep. 8, 4058–4084. doi: 10.1016/j.egyr.2022.03.016
Luong, J. H. T., Tran, C., and Ton-That, D. (2022). A paradox over Electric vehicles, Mining of Lithium for Car batteries. Energies 15, 1–25. doi: 10.3390/en15217997
Maitre-Ekern, E. (2021). Re-thinking producer responsibility for a sustainable circular economy from extended producer responsibility to pre-market producer responsibility. J. Clean. Prod. 286:125454. doi: 10.1016/j.jclepro.2020.125454
March, A., Salam, S., Evans, T., Hilton, J., Fletcher, S., Foster, P., et al. (2022). A global review of plastics policies to support improved decision making and public accountability.
Melin, H. E., Rajaeifar, M. A., Ku, A. Y., Kendall, A., Harper, G., and Heidrich, O. (2021). Global implications of the EU battery regulation. Science 373, 384–387. doi: 10.1126/science.abh1416
Mochizuki, Y., and Bryan, A. (2015). Climate change education in the context of education for sustainable development: rationale and principles. J. Educ. Sustain. Dev. 9, 4–26. doi: 10.1177/0973408215569109
Mossali, E., Picone, N., Gentilini, L., Rodrìguez, O., Pérez, J. M., and Colledani, M. (2020). Lithium-ion batteries towards circular economy: a literature review of opportunities and issues of recycling treatments. J. Environ. Manag. 264:110500. doi: 10.1016/j.jenvman.2020.110500
Mutebi, R., and Kiplagat, H. (2022). TVET response to global challenges of sustainable development. Afr. J. Res. Math. Sci. Technol. Educ. 7, 447–456. doi: 10.2022/ajest.v7i1.783
Nash, J., and Bosso, C. (2013). Extended producer responsibility in the United States: full speed ahead? J. Ind. Ecol. 17, 175–185. doi: 10.1111/j.1530-9290.2012.00572.x
Neumann, J., Petranikova, M., Meeus, M., Gamarra, J. D., Younesi, R., Winter, M., et al. (2022). Recycling of Lithium-ion batteries—current state of the art, circular economy, and next generation recycling. Adv. Energy Mater. 12, 1–26. doi: 10.1002/aenm.202102917
Ni, D., Xiao, Z., and Lim, M. K. (2021). Machine learning in recycling business: an investigation of its practicality, benefits and future trends. Soft. Comput. 25, 7907–7927. doi: 10.1007/s00500-021-05579-7
NITI Aayog and Green Growth Equity Fund Technical Cooperation Facility. (2022). Advanced chemistry cell battery reuse and recycling market in India.
Nogueira, A., Ashton, W., Teixeira, C., Lyon, E., and Pereira, J. (2020). Infrastructuring the circular economy. Energies 13, 1–24. doi: 10.3390/en13071805
Ornes, S. (2024). How to recycle an EV battery. Proc. Natl. Acad. Sci. USA 121:e2400520121. doi: 10.1073/pnas.2400520121
Padmanabhan, S., Joel, C., Mahalingam, S., Deepak, J. R., Kumar, T. V., and Raj, D. (2024). An overview of the need for circular economy on electric vehicle batteries. Nat. Environ. Pollut. Technol. 23, 183–191. doi: 10.46488/NEPT.2024.v23i01.014
Placke, T., Kloepsch, R., Dühnen, S., and Winter, M. (2017). Lithium ion, lithium metal, and alternative rechargeable battery technologies: the odyssey for high energy density. J. Solid State Electrochem. 21, 1939–1964. doi: 10.1007/s10008-017-3610-7
Prajapati, S. (2016). Lead acid battery recycling in India. IOSR J. Electr. Electron. Eng. 11, 99–101. doi: 10.9790/1676-111199101
Pražanová, A., Knap, V., and Stroe, D. I. (2022). Literature review, recycling of Lithium-ion batteries from electric vehicles, part I: recycling technology. Energies 15, 1–29. doi: 10.3390/en15031086
Pregowska, A., Osial, M., and Urbańska, W. (2022). The application of artificial intelligence in the effective battery life cycle in the closed circular economy model—a perspective. Recycling 7, 1–17. doi: 10.3390/recycling7060081
Quinteros-Condoretty, A. R., Golroudbary, S. R., Albareda, L., Barbiellini, B., and Soyer, A. (2021). Impact of circular design of lithium-ion batteries on supply of lithium for electric cars towards a sustainable mobility and energy transition. Procedia CIRP 100, 73–78. doi: 10.1016/j.procir.2021.05.012
Rakesh, C., Harika, A., Chahuan, N., Sharma, N., Zabibah, R. S., and Nagpal, A. (2023). Towards a circular economy: challenges and opportunities for recycling and re-manufacturing of materials and components. E3S Web Conf. 430, 1–11. doi: 10.1051/e3sconf/202343001129
Ralls, A. M., Leong, K., Clayton, J., Fuelling, P., Mercer, C., Navarro, V., et al. (2023). The role of Lithium-ion batteries in the growing trend of electric vehicles. Materials 16, 1–34. doi: 10.3390/ma16176063
Reinhardt, R., Christodoulou, I., Gassó-Domingo, S., and Amante García, B. (2019). Towards sustainable business models for electric vehicle battery second use: a critical review. J. Environ. Manag. 245, 432–446. doi: 10.1016/j.jenvman.2019.05.095
Ribeiro da Silva, E., Lohmer, J., Rohla, M., and Angelis, J. (2023). Unleashing the circular economy in the electric vehicle battery supply chain: a case study on data sharing and blockchain potential. Resour. Conserv. Recycl. 193:106969. doi: 10.1016/j.resconrec.2023.106969
Rocca, R., Rosa, P., Sassanelli, C., Fumagalli, L., and Terzi, S. (2020). Integrating virtual reality and digital twin in circular economy practices: a laboratory application case. Sustainability (Switzerland) 12, 1–27. doi: 10.3390/su12062286
Roy, H., Roy, B. N., Hasanuzzaman, M., Islam, M. S., Abdel-Khalik, A. S., Hamad, M. S., et al. (2022). Global advancements and current challenges of electric vehicle batteries and their prospects: a comprehensive review. Sustain. For. 14, 1–30. doi: 10.3390/su142416684
Sánchez-García, E., Martínez-Falcó, J., Marco-Lajara, B., and Manresa-Marhuenda, E. (2024). Revolutionizing the circular economy through new technologies: a new era of sustainable progress. Environ. Technol. Innov. 33:103509. doi: 10.1016/j.eti.2023.103509
Sasmoko, Z. K., Malik, M., Awan, U., Handayani, W., Jabor, M. K., and Asif, M. (2022). Environmental effects of bio-waste recycling on industrial circular economy and eco-sustainability. Recycling 7, 1–21. doi: 10.3390/recycling7040060
Scalabrino, C., Navarrete Salvador, A., and Oliva Martínez, J. M. (2022). A theoretical framework to address education for sustainability for an earlier transition to a just, low carbon and circular economy. Environ. Educ. Res. 28, 735–766. doi: 10.1080/13504622.2022.2031899
Siqi, Z., Guangming, L., Wenzhi, H., Juwen, H., and Haochen, Z. (2019). Recovery methods and regulation status of waste lithium-ion batteries in China: a mini review. Waste Manage. Res. 37, 1142–1152. doi: 10.1177/0734242X19857130
Slattery, M., Dunn, J., and Kendall, A. (2024). Charting the electric vehicle battery reuse and recycling network in North America. Waste Manag. 174, 76–87. doi: 10.1016/j.wasman.2023.11.018
Sukiennik, M., Zybała, K., Fuksa, D., and Kęsek, M. (2021). The role of universities in sustainable development and circular economy strategies. Energies 14, 1–18. doi: 10.3390/en14175365
Sun, B., Su, X., Wang, D., Zhang, L., Liu, Y., Yang, Y., et al. (2020). Economic analysis of lithium-ion batteries recycled from electric vehicles for secondary use in power load peak shaving in China. J. Clean. Prod. 276:123327. doi: 10.1016/j.jclepro.2020.123327
Tankou, A., Bieker, G., and Hall, D. (2023). Scaling up reuse and recycling of electric vehicle batteries: Assessing challenges and policy approaches. Available at: www.theicct.orgcommunications@theicct.org
Thompson, D. L., Hartley, J. M., Lambert, S. M., Shiref, M., Harper, G. D. J., Kendrick, E., et al. (2020). The importance of design in lithium ion battery recycling-a critical review. Green Chem. 22, 7585–7603. doi: 10.1039/d0gc02745f
UNESCO-UNEVOC (2018). Greening technical and vocational education and training: A practical guide for institutions. France: United Nations Educational, Scientific and Cultural Organisation.
United Nations. (n.d.). New economics for sustainable development: circular economy. Available at: https://www.un.org/sites/un2.un.org/files/circular_economy_14_march.pdf
Villagrossi, E., and Dinon, T. (2023). Robotics for electric vehicles battery packs disassembly towards sustainable remanufacturing. J. Remanuf. 13, 355–379. doi: 10.1007/s13243-023-00134-z
Wang, M., Liu, K., Yu, J., Zhang, Q., Zhang, Y., Valix, M., et al. (2023). Challenges in recycling spent Lithium-ion batteries: spotlight on Polyvinylidene fluoride removal. Glob. Chall. 7, 1–18. doi: 10.1002/gch2.202200237
WEF. (2019). A vision for a sustainable battery value chain in 2030: Unlocking the full potential to power sustainable development and climate change mitigation. www.weforum.org
Wei, L., Wang, C., and Li, Y. (2022). Governance strategies for end-of-life electric vehicle battery recycling in China: a tripartite evolutionary game analysis. Front. Environ. Sci. 10, 1–14. doi: 10.3389/fenvs.2022.1071688
World Economic Forum, Ellen MacArthur Foundation, and McKinsey & Company. (2016). The new plastics economy — Rethinking the future of plastics. Available at: http://www.ellenmacarthurfoundation.org/publications
Xiao, J., Jiang, C., and Wang, B. (2023). A review on dynamic recycling of electric vehicle battery: disassembly and echelon utilization. Batteries 9, 1–25. doi: 10.3390/batteries9010057
Yang, M., Chen, L., Wang, J., Msigwa, G., Osman, A. I., Fawzy, S., et al. (2023). Circular economy strategies for combating climate change and other environmental issues. Environ. Chem. Lett. 21, 55–80. doi: 10.1007/s10311-022-01499-6
Yang, H., Hu, X., Zhang, G., Dou, B., Cui, G., Yang, Q., et al. (2024). Life cycle assessment of secondary use and physical recycling of lithium-ion batteries retired from electric vehicles in China. Waste Manag. 178, 168–175. doi: 10.1016/j.wasman.2024.02.034
Yu, M., Bai, B., Xiong, S., and Liao, X. (2021). Evaluating environmental impacts and economic performance of remanufacturing electric vehicle lithium-ion batteries. J. Clean. Prod. 321:128935. doi: 10.1016/j.jclepro.2021.128935
Yu, X., Li, W., Gupta, V., Gao, H., Tran, D., Sarwar, S., et al. (2022). Current challenges in efficient Lithium-ion batteries’ recycling: a perspective. Global Chall. 6, 1–10. doi: 10.1002/gch2.202200099
Zanoletti, A., Carena, E., Ferrara, C., and Bontempi, E. (2024). A review of Lithium-ion battery recycling: technologies, sustainability, and open issues. Batteries 10, 1–30. doi: 10.3390/batteries10010038
Zeng, X., Li, J., and Singh, N. (2014). Recycling of spent lithium-ion battery: a critical review. Crit. Rev. Environ. Sci. Technol. 44, 1129–1165. doi: 10.1080/10643389.2013.763578
Zhang, W., Xu, C., He, W., Li, G., and Huang, J. (2018). A review on management of spent lithium ion batteries and strategy for resource recycling of all components from them. Waste Manage. Res. 36, 99–112. doi: 10.1177/0734242X17744655
Zhang, C., Yan, J., and You, F. (2023). Critical metal requirement for clean energy transition: a quantitative review on the case of transportation electrification. Adv. Appl. Energy 9:100116. doi: 10.1016/j.adapen.2022.100116
Keywords: circular economy, EV battery recycling, sustainable development goals, lithium-ion batteries, education for sustainable development, resource efficiency, workforce skills development, environmental sustainability
Citation: Chigbu BI (2024) Advancing sustainable development through circular economy and skill development in EV lithium-ion battery recycling: a comprehensive review. Front. Sustain. 5:1409498. doi: 10.3389/frsus.2024.1409498
Edited by:
Idiano D'Adamo, Sapienza University of Rome, ItalyReviewed by:
Maria Angela Butturi, University of Modena and Reggio Emilia, ItalyXiang Gao, Shanghai Business School, China
Copyright © 2024 Chigbu. This is an open-access article distributed under the terms of the Creative Commons Attribution License (CC BY). The use, distribution or reproduction in other forums is permitted, provided the original author(s) and the copyright owner(s) are credited and that the original publication in this journal is cited, in accordance with accepted academic practice. No use, distribution or reproduction is permitted which does not comply with these terms.
*Correspondence: Bianca Ifeoma Chigbu, bianca7h@yahoo.com