- 1Bren School of Environmental Science and Management, University of California, Santa Barbara, Santa Barbara, CA, United States
- 2Watershed Technology Inc. San Francisco, CA, United States
- 3CIRAIG, Polytechnique Montreal, Montreal, QC, Canada
- 4Quantitative Sustainability Assessment, Department of Environmental and Resource Engineering, Technical University of Denmark, Lyngby, Denmark
- 5School of Public Health, University of Nevada, Reno, NV, United States
- 6United Nations Environment Programme, UNEP, Marine and Fresh Water Branch, Ecosystems Division, UNEP India, New Delhi, India
- 7Carbon Minds GmbH, Cologne, Germany
- 8Institute for Technical Thermodynamics, RWTH Aachen University, Aachen, Germany
- 9United Nations Environment Programme, UNEP, Industry & Economy Division, Paris, France
With the increasing global concern over plastics' environmental and human health impacts, the urgency for effective regulatory measures is evident. The UN Environment Assembly's initiative to establish an international, legally binding instrument via the Intergovernmental Negotiating Committee (INC) on Plastic Pollution marks a significant step toward addressing this issue. However, the vast diversity of plastic types and their myriad applications present a complex challenge in pinpointing the most critical targets for regulation. This study builds on the existing body of literature to outline potential key criteria for identifying Polymers of Concern (PoC). We recommend a dual-focused definition of PoCs considering both (1) the type of the plastics and (2) their domain of applications based on the environmental and human health impacts throughout the polymer's life cycle. Recognizing the current gaps in our understanding of the full spectrum of plastics' impacts across their life cycles, we suggest adopting a precautionary approach that factors in the volume of plastics entering natural ecosystems alongside their life cycle impacts as reported in the literature. We then bring forward existing data on the assessment of some of the main polymer types and applications. We propose that policymakers examine a wide spectrum of strategies including not only bans and phaseouts but also economic incentives, innovation, and the redesign of plastic materials and products to mitigate the adverse impacts of PoCs. We further emphasize the importance of thoroughly assessing the feasibility, costs, and environmental, social and economic implications of alternative materials to avoid “regrettable substitution.” We conclude by identifying existing knowledge gaps and emphasizing the need for further research to refine the proposed criteria for identifying PoCs.
1 Introduction
The widespread use of synthetic and semi-synthetic polymers (“plastics” or “polymers” hereafter) has fundamentally transformed modern society since the 1950s (Geyer et al., 2017). The reliance on plastics has fuelled an over three-fold rise in plastic production from 1990 to 2015, reaching global annual production of over 400 million tons today (OECD, 2022). The largest production shares of polymers are represented by low-density (LDPE), linear low-density (LLDPE) and high-density polyethylene (HDPE), which, together, represent 24% of the total global annual production, followed by polypropylene (PP) (16%), and polyvinyl chloride (PVC) (11%), while major domains of application include packaging (31%), building and construction (14%), and automotive (14%) (OECD, 2022).
Projections indicate a continued upward trajectory in plastic production and consumption, with estimates pointing toward >1,200 million metric tons (Mt) per year by 2060 (OECD, 2022). Under the current trajectory, the cumulative production of plastics is expected to reach 33 billion metric tons by 2050 (Geyer, 2020; Persson et al., 2022). These projections underscore the urgent need for sustainable practices and policies to address the multi-dimensional challenges posed by plastics on human and planetary health.
The use of plastics across diverse applications has undeniably yielded economic benefits and improvements in human wellbeing (Andrady and Neal, 2009). Nonetheless, the mounting concerns on the environmental and health consequences of plastic utilization have captured considerable attention from policymakers, industries, scientists, and the broader public (UNEP, 2016; Nielsen et al., 2020; MacLeod et al., 2021). The concerns that have been raised encompass not only the impacts of plastics and its chemical constituents, but also its production and supply chain processes, end-of-life treatment, and degradation byproducts (Revel et al., 2018; Zheng and Suh, 2019; Lear et al., 2021; MacLeod et al., 2021; Turner and Filella, 2021).
One of the primary concerns is placed on the leakage of plastics to the environment (Thompson et al., 2009; Jambeck et al., 2015; Law, 2017; Napper and Thompson, 2023; UNEP, 2023c). It is estimated that aquatic ecosystem received as much as 19 to 23 Mt of plastic waste generated in a single year, 2016, which is set to grow to 53 Mt per year by 2030 (Borrelle, 2020). Another global estimate suggests that 6.2 Mt (confidence interval, CI: 2.0–20.4 Mt) of macroplastics and 3.0 Mt (CI: 1.5–5.2 Mt) of microplastics were lost to the environment in 2015 (Ryberg et al., 2019). Washing of synthetic garments alone is estimated to have emitted 5.6 Mt of synthetic microfibers to the environment between 1950 and 2016, while emissions to terrestrial systems are growing rapidly (Gavigan et al., 2020; Geyer et al., 2022). As a result, today, plastics are found even in the world's most remote environments such as Antarctica and Galápagos islands (Barnes et al., 2010; Muñoz-Pérez et al., 2023).
The presence and accumulation of plastic pollution in both natural and human environments pose a grave risk to ecosystems and human health (Beaumont et al., 2019). First, macroplastics emitted to the environment, such as abandoned or lost fishing nets and plastic bags, are reported to cause a variety of harms to the wildlife, through entrapment, entanglement or ingestion (Alexiadou et al., 2019; Barboza et al., 2019; Valente et al., 2020; Blettler and Mitchell, 2021). Second, the micro- and nano-plastics released and emitted from the use, fragmentation, and degradation of macroplastics in both the human and natural environment pose risks to human and ecosystem health (Teuten et al., 2009; Revel et al., 2018; Peng et al., 2020; Kumar et al., 2021; Sheraz et al., 2023). The conversion of macroplastics into smaller particles, especially nano-scale plastics, is known to substantially increase the uptake and absorption of plastics into biota (Shen et al., 2019). Additionally, the environmental emissions generated throughout the life cycle of plastics pose other risks to the environment and human health (Walker and Rothman, 2020). For example, forecasts suggest that plastic production and waste management could potentially contribute up to 6.5 gigatons of CO2-equivalent global greenhouse gas (GHG) emissions annually by 2050 (Zheng and Suh, 2019; Meys et al., 2021).
In addition, concerns have been raised due to the harmful chemicals used in plastics production. A wide range of substances used for plastics production to achieve certain properties such as flexibility, durability, and resistance to degradation. Studies have shown that some of these substances, which are often referred to as chemicals of concern, exhibit the potential to cause adverse effects to human health, including endocrine disruption, carcinogenicity, and ecological harm (Aurisano et al., 2021a; Scientists Coalition for an Effective Plastics Treaty, 2023; Wagner et al., 2024). At the root of the global problems associated with plastics today is the linear economy model of “take-make-dispose,” which is heavily dependent on fossil-based resources such as crude oil (Otto et al., 2015; Kätelhön et al., 2019; Meys et al., 2021; Bachmann et al., 2023; UNEP, 2023c). Plastics are projected to command 20% of global crude oil consumption by 2050 (WEF, 2016; UNEP, 2018). These trajectories underscore the urgent need for considering a reduction in primary plastic production, phasing out or phasing down certain plastics or plastics products that pose high risk to humans and the environment, a shift toward sustainable production and consumption, and the adoption of a circular economy approach to alleviate the environmental impact of plastics' life-cycles.
A wide spectrum of approaches to mitigating the impacts of plastics have been proposed, including the scaling of circular technologies (Ren et al., 2020; Meys et al., 2021; Stegmann et al., 2022; Bachmann et al., 2023; UNEP, 2023c), decarbonization of energy supply (Posen et al., 2017; Zheng and Suh, 2019; Meys et al., 2021), the use of renewable feedstock such as plastic waste, biomass, or captured CO2 (Shen et al., 2010; Kätelhön et al., 2019; Zheng and Suh, 2019; Meys et al., 2021; Lee et al., 2022; Raj et al., 2022; Stegmann et al., 2022; Bachmann et al., 2023), eliminating or reducing the use of chemicals and polymers of concern and problematic plastic products, minimizing waste generation (Idumah and Nwuzor, 2019; Okan et al., 2019; Chu et al., 2023), and disposal to the environment (Willis et al., 2018; Williams and Rangel-Buitrago, 2019; Schmaltz et al., 2020), economic incentives and behavioral change (Allison et al., 2022; Abiola et al., 2023), and improving reuse and recycling (Keane, 2007; Hopewell et al., 2009; Pacheco et al., 2012; Garcia and Robertson, 2017; Rahimi and García, 2017; Milios et al., 2018; Rosa et al., 2018; British Plastics Federation, 2021; Schwarz et al., 2021; Schyns and Shaver, 2021). Studies indicate that these approaches, in concert, have the potential to reduce plastics discarded to the environment, carbon and other pollutant emissions as well as the dependence of plastic products on fossil fuels throughout the life cycle of plastics (UNEP, 2018; Zheng and Suh, 2019; Meys et al., 2021; Bachmann et al., 2023).
In parallel, countries around the world have been enacting regulatory approaches to address plastic pollution at different stages of the plastic life cycle (UNEP, 2020, 2023c). According to Raubenheimer and Urho (2024), 33 countries have regulated one or more plastic polymers or monomers, and up to 141 countries have banned or restricted some form of plastic products. Many in the private sector have also taken the initiative of eliminating plastic packaging made of specific polymers, as part of the New Plastics Economy Global Commitment (Ellen MacArthur Foundation and UNEP, 2023).
This paper aims to establish the conceptual framework for identifying the criteria for polymers of concern as a means to prioritize the target areas that international efforts to tackle global plastics pollution can focus on. These criteria can help inform strategies to mitigate environmental and health impacts across the polymer life cycle, including substituting polymers of concern with suitable alternatives, promoting optimal technologies for polymer production and design practices promoting circularity, phasing down or phasing out polymers of most concern, as well as regulating certain applications to avoid the leakage of plastic to the environment (UNEP, 2023a). In addition, we aim to offer insights into research gaps and data needs for future research efforts.
2 Methods for identifying the criteria for polymers of concern
2.1 Existing literature
While there is currently no internationally agreed definition for polymers of concern, the concept, as well as some of the closely related concepts such as “chemicals of concern” and “products of concern,” have been widely discussed in both academic and regulatory contexts over the last two decades (Montes-Grajales et al., 2017; Aurisano et al., 2021a; Huang et al., 2022; Zimmermann et al., 2022; Scientists Coalition for an Effective Plastics Treaty, 2023).
The US Environmental Protection Agency (USEPA) defines products of concern based on their hazard properties and biodegradability as “compounds with high acute or chronic aquatic toxicity (L/E/IC50 ≤ 10 ppm or LOEC ≤ 1 ppm) and a slow rate of biodegradation (>28 days)” (US EPA, 2014). In addition, Section 5(b)(4) of the US Toxic Substances Control Act (TSCA) authorizes USEPA to maintain a list of chemical substances of which “manufacture, processing, distribution in commerce, use, or disposal, or any combination of such activities, presents or may present an unreasonable risk of injury to health or the environment” (US EPA, 2015a, p. 5).
The Corporate Sustainability Reporting Directive (CSRD) of the European Commission, disclosure requirement E2-5, “Substances of concern and substances of very high concern,” mandates the reporting companies to disclose the risks and opportunities related to the use, distribution and commercialization of the substances (European Commission, 2022). The European Commission defines the substances of concern and substances of very high concern using the criteria laid out under the Registration, Evaluation, Authorization, and Restriction of Chemicals (REACH) [Regulation (EC) No 1907/2006] Article 57, which are based largely on chemicals' intrinsic properties including carcinogenicity, mutagenicity, reproductive toxicity, persistence, bioaccumulation, endocrine disrupting properties, and their combinations (European Commission, 2006). The European Commission is also evaluating a proposal that seeks mandating registration of certain polymers under the REACH directive (Groh et al., 2023). The criteria for determining the EU REACH registration requirement are also relying heavily on the polymers' intrinsic properties such as molecular weight, surface activity, and hazard characteristics (Groh et al., 2023).
Defining polymers of concern based solely on the materials' intrinsic properties such as hazards, biodegradability under environmental conditions, and bioaccumulation characteristics, however, poses several challenges. First, literature suggests that non-plastic emissions from upstream and downstream plastic life-cycle processes contribute substantially to various environmental problems. The life-cycle greenhouse gas (GHG) emissions of plastics, for example, contribute to around 1.7–2 Gt CO2e emissions, which are much higher than for example the direct emissions from global aviation industry (Zheng and Suh, 2019; Cabernard et al., 2022; OECD, 2022). Studies also highlight regional and local emissions of Particulate Matter (PM), SO2 and NOx associated with plastics production in the regions that rely heavily on coal as the feedstock (Ren et al., 2020; Cabernard et al., 2022). Second, the usage pattern largely determines the nature of interactions between plastics and the environment, and therefore not only the types of polymer materials but also the context under which they are utilized should be considered as an important attribute in determining the polymers of concern.
Recognizing these shortcomings, recent studies tend to use multiple criteria, both intrinsic and extrinsic to the chemicals in question, in determining chemicals of concern. Zimmermann et al. (2022), for example, identified the food contact chemicals of concern (FCCoCs) based not only on the chemical hazard, but also on usage pattern, and chemical production volume, among others (Zimmermann et al., 2022).
2.2 Potential criteria to be considered
Environmental and human health impacts of plastics are materialized throughout their life cycle, and it is therefore crucial to maintain the life-cycle perspective when developing the criteria for polymers of concern (Figure 1) (Rikhter et al., 2022). Emitted to the environment throughout the life-cycles of plastics are (i) macroplastics such as plastic bags and bottles as well as abandoned fishing nets and fishing gears, (ii) micro- and nano-plastics and associated chemicals (e.g., processing aids, plasticizers, colorants, non-intentionally added substances) through the normal use or wear-and-tear of plastic products such as plastic bottles and synthetic garments, and (iii) other, non-plastic constituent emissions including CO2, SO2, NOx, and particulate matter (PM) (Figure 1). In particular, it is important to note that not only the plastics and their chemical constituents, but also non-plastic emissions and wastes generated from the life cycle of plastics do contribute substantially to various environmental impacts including climate change, acidification and toxicological effects to humans and the ecosystem (Valavanidis et al., 2008; Koornneef et al., 2012; Ren et al., 2020; Cabernard et al., 2022; Rikhter et al., 2022). These primary pollutants generated throughout the life-cycle of plastics may directly affect the environment and human health. Furthermore, they may go through a variety of physicochemical and biological transformations including fragmentation, degradation, and leaching and may form secondary pollutants (Figure 1), while the mechanisms of such processes are yet to be fully understood (Teuten et al., 2009; Law, 2017; Ryberg et al., 2019; Chamas et al., 2020; Groh et al., 2023).
Based on the literature and legislative review, the following criteria to determine polymers of concern are proposed:
a) Health and safety issues by polymers;
b) Environmental impacts through emissions and resource use across the life cycle;
c) Circularity of polymers;
d) Leakage of plastics to the environment.
2.2.1 Health and safety issues of polymers
The materials and chemicals that constitute plastic materials themselves can directly affect the environment and human health. In general, there are two types of chemicals that are intentionally added to form plastic materials: (1) polymerized monomers that form the foundation of the polymer matrix, and (2) various chemicals that fulfill specific functions and deliver desired properties. The functions of additives include enhancing material flexibility (plasticizers), incorporating fire resistance (flame retardants), giving color (colorants), and preventing oxidation (antioxidants) (Maier et al., 2009). Other constituents are unintentionally added, such as transformation or degradation byproducts, and contaminants from plastics manufacturing processes (Geueke, 2018). Overall, approximately 16,000 chemicals are associated with plastics and related production processes across the numerous plastics applications in the building, food, cosmetics, and other industries (Aurisano et al., 2021b; Wiesinger et al., 2021; UNEP, 2023b; Wagner et al., 2024), many of which are of potential concern regarding human and environmental health (Aurisano et al., 2021a; Huang et al., 2022; Geueke et al., 2023). Human exposure to these potentially harmful substances can occur throughout the life cycles of plastic products, be that from direct consumer use or via production or disposal related emissions to the general environment.
Concerns around chemicals in plastics are associated with various chemical properties, including environmental persistence and mobility, bioaccumulation in ecological and human food chains, and various toxicity-related effects on humans and ecological organisms (e.g., carcinogenicity, reproductive and developmental effects, endocrine disruption, and specific organ and neurotoxicity). These properties are often used as criteria for identifying chemicals or groups of chemicals of concern in plastics. For example, the European Union's chemicals regulation, REACH (European Commission, 2006; Strempel et al., 2012; Wagner et al., 2024) considers mobility of the chemicals in addition to the Persistent, Bioaccumulative and Toxic (PBT) criteria. Specific chemicals in polymers that have raised a concern include certain monomers, flame retardants, UV light stabilizers, Per- and Polyfluorinated Substances (PFASs), phthalate plasticizers, bisphenols, certain ethoxylates, biocides (e.g., formaldehyde), metals and metalloids, polycyclic aromatic hydrocarbons, and non-intentionally added substances, such as dioxins and furans (UNEP, 2023b). Wagner et al. (2024) reports the state of science on the chemicals used in plastics and identifies 15 priority groups of chemicals of concern.
Impacts of these chemicals contribute to human and environmental health burden, which lead to substantial economic burden worldwide, through exposure to the chemicals along the full life cycle of plastics, including occupational exposure. For example, human disease burden in the U.S. attributable to plastic-related chemicals in 2018 led to costs of around 250 billion USD, mostly associated with exposure to PBDE, phthalates and PFASs (Trasande et al., 2024). All major polymers contain hundreds of chemicals of concern, but “rubber, polyurethanes, polycarbonates, and PVC are most likely to contain such compounds” (Wagner et al., 2024). While these criteria for chemicals of concern apply generally to all chemicals found in plastic polymers, additional criteria need to be defined to cover other concerning aspects around polymers not driven by their chemical constituents.
As plastic products can be used in a wide variety of consumer products (e.g., flooring, furniture, food packaging materials, personal care products, cleaning supplies), human exposure to its ingredient is ubiquitous with numerous studies focused on the resulting potential human health risks from these ingredients such as bisphenols and phthalates (Li and Suh, 2019). Among such ingredients, bisphenol-A (BPA) and di-2-ethylhexyl phthalate (DEHP) are the most well-studied due to their endocrine disrupting capabilities, which may cause series of reproductive and developmental toxic effects (Halden, 2010; Dodson et al., 2012; Katsikantami et al., 2016). In fact, public concerns for these two chemicals have risen to levels high enough for them to be banned or phased out from certain consumer products in the 21st century (European Commission, 2005; FDA., 2012). Nevertheless, challenges remain in the search of safer alternatives for these chemicals, as reports of concerns for their replacements have also surfaced (Bui et al., 2016; Winkler et al., 2022).
Additionally, micro- and nano-plastics released from the use and degradation of plastic products such as plastic water bottles have also been considered as an important emerging pollutant with many experiments showing potential toxic effects on the cellular, organ, and whole-body levels (Li et al., 2023; Qian et al., 2024). Consumer exposure to microplastics can happen via multiple pathways as plastics are used in various products—oral ingestion of food and water containing microplastics (WHO, 2019; Udovicki et al., 2022) and inhalation of suspended microplastics in the indoor air from synthetic fabrics and coating (Kacprzak and Tijing, 2022). These exposure pathways have led to confirmed presence of microplastics in human placenta, lung, brain, and arterial plaque (Ragusa et al., 2021; Chen et al., 2022; Kopatz et al., 2023; Marfella et al., 2024). Alarming research implicates the potential for congenital deformities and brain disease similar to Variant Creutzfeldt-Jakob or other prion diseases (Hollóczki and Gehrke, 2019; Windheim et al., 2022; Wang et al., 2023). While large scale epidemiological evidence linking microplastic exposure and adverse health outcomes is still absent, a recent study found higher risks for heart attack, stroke, and death are associated with the presence of micro- and nano-plastic found in carotid artery plaque of patients who underwent carotid endarterectomy (Marfella et al., 2024); another study suggests higher exposure is associated with inflammatory bowel disease (Yan et al., 2022).
Finally, the fragmentation and degradation of plastics in the environment may result in harmful secondary chemicals that adversely affect the ecosystem and human health, while our understanding of the degradation pathways, the fate, transport and exposure of the degradation byproducts, and their impacts are limited (Chamas et al., 2020; Croxatto Vega et al., 2021). It is also worth mentioning that despite bioplastics and plant-based plastics are often viewed as more sustainable alternatives, they may nevertheless exhibit similar toxicity of their petroleum-based counterparts (Zimmermann et al., 2020).
The effort to synthesize the studies on hazard characteristics of polymers is on-going. Senathirajah et al. (2022), for example, identified Polyvinyl Chloride (PVC), Polypropylene (PP), and Polystyrene (PS) as the polymers with highest risk of harms using 21 criteria and multi-criteria prioritization framework. The dominant sectors to which these polymers contributed were building and construction, packaging, consumer and household, and automotive sectors (Senathirajah et al., 2022).
2.2.2 Life-cycle environmental impacts
Life cycle assessment (LCA) is an approach to quantify the environmental impacts of products throughout their life cycles including raw material extraction, materials processing, manufacturing and synthesis, transportation, use and disposal (Guinée et al., 2002; ISO, 2006a; Finnveden et al., 2009). Feedstock types for plastics production include petroleum and natural gas, biomass, and captured CO2, while the majority of the plastics produced today (~99%) are still fossil-based (European Bioplastics, 2019; Ögmundarson et al., 2020). The ISO standards 14040 and 14044 serve as the cornerstone for a standardized methodology in conducting LCAs, ensuring uniformity and facilitating result comparison (ISO, 2006a,b). In essence, LCA encompasses the assessment of environmental impacts spanning from raw material generation to the final waste management, leading to recycling or disposal (Guinée et al., 2002; Finnveden et al., 2009).
Various life cycle stages contribute to the overall greenhouse gas (GHG) emissions of plastics (Zheng and Suh, 2019). Overall, GHG emissions are predominantly attributed to the resin production stage, accounting for 61%, followed by the conversion stage at 30%, and the end-of-life treatment stage at 9% (Zheng and Suh, 2019).
However, it needs to be noted that fugitive methane emissions from fossil fuel extraction have been poorly accounted for in the past, and recent studies suggest that improved measurement could significantly increase the climate change impact linked to fossil-fuel derived products such as the majority of synthetic polymers (Allen et al., 2021; Burns and Grubert, 2021). Chemical processes involved in transforming these feedstocks into plastic polymers also release GHGs, with refinery operations and steam cracking emitting notable amounts (Ren et al., 2008; Young et al., 2022). Additionally, the energy-intensive operations within the plastic production chain, from refining feedstocks to molding, result in substantial emissions, exacerbated by using fossil fuels for energy generation (Posen et al., 2017; Zheng and Suh, 2019; Meys et al., 2021).
In some cases, the precursors or chemical constituents of polymers emitted to the environment over their life-cycles may be harmful to the environment and human health. This is the case for products made of polystyrene (PS) releasing styrene (listed as “probably carcinogenic” by IARC), or emissions of vinyl chloride from the life cycle of PVC (Huff and Infante, 2011; Iizuka et al., 2020; Sun, 2023), both regulated by the US EPA as Hazardous Air Pollutants under the US Clean Air Act (US EPA, 2015b).
Other relevant emissions during the life cycle of polymers include SO2, NOx and particulate matter (PM) emissions (Ren et al., 2020; Cabernard et al., 2022). Several ozone depleting substances (ODSs) and a few hydrofluorocarbons (HFCs) controlled under the Montreal Protocol are used as feedstocks in plastics manufacture including, for example, polychlorotrifluoroethylene (PCTFE), polyvinylidene fluoride (PVDF), and polytetrafluoroethylene or Teflon (PTFE); possibly also polyvinyl fluoride (PVF); and polyvinyl chloride (PVC) (Andersen et al., 2021).
Furthermore, conventional incineration of plastics waste that contains chlorine or brominated flame retardants is known to lead to the release of highly toxic products of incomplete combustion and reformation byproducts such as furans and dioxins into the atmosphere, if no strict air pollution control technologies are applied (Wang et al., 2003; Buekens and Cen, 2011; Zhang et al., 2016).
Plastic materials and products discharged to the environment throughout their life cycles may also adversely affect the ecosystem. Species and ecosystems encounter plastic pollution in various ways, such as through ingestion, entanglement, smothering, and the transportation of invasive species (Gregory, 2009; Seif, 2018; Alexiadou et al., 2019; Høiberg et al., 2022). These forms of exposure can lead to adverse effects on individual organisms through physical pathways. Ingestion of plastic can block an organism's digestive tract or cause false satiation, impairing its ability to feed (Gregory, 2009; Seif, 2018; Alexiadou et al., 2019). This can lead to starvation, reduced fitness, and negative impacts on growth, reproduction, and predator avoidance, or even death. Ingesting plastic fragments can also cause internal injuries, increasing the risk of infection. Entanglement in plastic debris can result in death from starvation or injury, increased risk of infection, compromised mobility, behavioral changes, reduced growth, and impaired reproduction (Seif, 2018; Høiberg et al., 2022). These effects, which exclude toxic effects associated with associated chemicals or unpolymerized monomers, are caused by the physical effect of the plastic item and is mostly independent of the type of polymer constituting it, but rather dependent on the shape and strength of the item (Corella-Puertas et al., 2023).
For smaller size particles (micro- and nano-plastics), the microplastic release potential and plastic degradation rate and density, which vary across polymers, are important parameters in quantifying their impacts on both human environments and natural ecosystems. The more microplastic release potential and the longer the particles are exposed to their environment, the higher the impact in, and to, that environment (Hurley and Nizzetto, 2018; Revel et al., 2018; Chamas et al., 2020; Peng et al., 2020; Kumar et al., 2021). The variability of these two parameters can make the impacts of the plastic emission vary from one of the least “toxic” substance to one comparable to the top 25% of substance toxicity as assessed in life cycle impact assessment (LCIA) (Corella-Puertas et al., 2023). However, these assessments are still incomplete and their high uncertainty representative of the limited data availability. Biodegradable polymers do not necessarily offer a better alternative, as very few alternatives degrade faster in the environments (Chamas et al., 2020). For larger size debris (macroplastics), such as strapping bands and ropes, size, and shape affect the potential impacts via entanglement (Gregory, 2009; Høiberg et al., 2022). Plastic bags can wrap animals around, causing lacerations, infections, and ultimately death (Derraik, 2002; Gall and Thompson, 2015). Abandoned, lost, or discarded plastic fishing gear, is considered as the main source of entanglements for many marine species (Johnson et al., 2005; Gregory, 2009; Stelfox et al., 2016; Hamilton and Baker, 2019; Høiberg et al., 2022). Macroplastics fragment into microplastics, hence their impact is additive (Woods et al., 2021).
Other impact pathways have been identified although not quantified yet; for example, drifting plastic items contribute to the invasive species problem, carrying species from one eco-region to another where they may not have any natural predators (Rech et al., 2018; García-Gómez et al., 2021). Plastic litter also play a role of vector of other pollutants, such as heavy metals, which are adsorbed to the plastic and thus increasingly ingested by animal species (Nguyen et al., 2023; Guo et al., 2025). Maquart et al. (2022) provide a comprehensive review of studies linking mismanaged plastic waste with the spread of infectious diseases, e.g., by generating suitable habitats for vectors of arthropod-borne diseases both directly (e.g., plastic cups or bottles) and indirectly (clogging drains and causing stagnant waters); or promoting waterborne diseases through generation of stagnant waters (Maquart et al., 2022). Bidashimwa (2023) also highlight the role of plastic pollution in the transmission of vector-borne infectious diseases (Bidashimwa, 2023). While this pathway is far less studied than the exposure to chemicals present in plastic, and it is difficult to quantify its overall effect on the burden on human health, the widespread presence of mismanaged plastic in areas with poor or non-existing plastic waste management could represent a significant impact on human health.
It is worth noting that the incidence for all these pathways is likely to be more closely affected by the likelihood of the polymer to leak into the environment than by any intrinsic properties of the polymer, with only density and biodegradation rate likely to be playing an effect as discussed. In this sense, the application of the polymer is likely to be a stronger determinant than its composition.
2.2.3 Circularity
Estimates indicate that only about 9% of plastics produced are being recycled globally, indicating a large room for improvements (Geyer et al., 2017; OECD, 2022). The limited circularity of plastics poses challenges at multiple fronts as studies show that circularity of plastics is crucial not only for resource conservation and waste minimization but also for achieving various environmental objectives within planetary boundaries (Schwarz et al., 2021; Bachmann et al., 2023; Chu et al., 2023; UNEP, 2023c). Analyses show that, for example, improving circularity plays an integral role in mitigating greenhouse gas emissions from plastics' life cycle (Zheng and Suh, 2019; Meys et al., 2021; Stegmann et al., 2022). A recent analysis also suggests that increasing recycling within a region can reduce the leakage of plastics to ocean environment, as a substantial portion of the post-consumer plastics exported is poorly managed (Bishop et al., 2020).
On the one hand, many thermoplastic polymers can be re-molded and re-shaped through heating while keeping their molecular structure, thereby being technically recyclable (Kulkarni, 2018; British Plastics Federation, 2021). Furthermore, progresses are being made in both mechanical and chemical recycling technologies (Rahimi and García, 2017; Kulkarni, 2018; Rosa et al., 2018; Marson et al., 2021; Schyns and Shaver, 2021; Lehr et al., 2022; Goevert, 2024). On the other hand, plastics are largely not recyclable in practice and at scale due to technological, behavioral, economic and social constraints. Ellen MacArthur Foundation, for example, defines being “recyclable in practice and at scale” as recycling “at least 30% of post-consumer rate […] achieved in multiple regions collectively representing at least 400 million inhabitants” (Ellen MacArthur Foundation, 2020). The ability to recycle plastics in practice at scale is determined not only by the intrinsic properties of plastics and additives, but also by various factors including socio-economic and regulatory drivers as well as the context in which plastics and plastics products are utilized and the ability to access the infrastructure needed for recycling (Hopewell et al., 2009; Mwanza and Mbohwa, 2017; Baldassarre et al., 2022; Fogt Jacobsen et al., 2022; Lee and Wong, 2023).
2.2.4 Risk of leakage
Despite the recent progresses, the fate and behavior of plastics emitted to the environment is still poorly understood (Zhu, 2021; Roebroek et al., 2022). Therefore, reducing the magnitude of plastics leakage to the environment as much as possible would be prudent, while our understanding of their behavior and impacts, including the fate, transport, exposure, and effects of plastics in the environment, matures.
Various factors affect the rate of plastics entering the environment. Lau et al. (2020) distinguish three plastic product categories to differentiate waste management fates: rigid monomaterial, flexible monomaterial, and multimaterial or multilayer (Lau et al., 2020). It is expected that polymers with very low density (such as XPS or EPS) may also be more prone to being blown into the environment, and thus contribute more to impacts related to presence of macroplastics in the environment. The Plastic Footprint Network identifies a series of factors that will influence the likelihood of a plastic item to end-up in the environment: the weight/density, the targeted use (whether “on-the-go” or at home), the residual value of the item (which will make it more or less likely to be picked up by waste pickers), the effectiveness of the local waste management system in place, and the environmental conditions (wind, proximity to a waterway, etc.) (Plastic Footprint Network, 2023). It is notable that the amount of plastics entering the environment depends not only on the type of plastics and their domain of application, but also on various geospatial and policy variables, including the effectiveness of waste management system in place, leading to a wide spatial variability (Meijer et al., 2021; OECD, 2022).
2.3 Proposed approach
2.3.1 Proposed method for identifying polymers of concern
First, it became clear that not only the type of polymer material but also the context of how the polymer in question is utilized, which determines not only the functionality of the polymer and its potential alternatives but also the magnitude and type of polymer emissions to human and natural environments and the nature of interactions between the polymer and its environments, is crucial in defining polymers of concern. A type of plastic used for rapidly discarded packaging, for example, does not affect the environment and human health the same way that the same type of plastic used as a building material does. As another comparison, polypropylene used in car bumpers poses little risk of microplastic shedding, while the same polymer used for car carpeting creates a higher risk of microplastic shedding and exposure in the environment of a small car interior, potentially impacting human health. Second, the literature clearly shows that the environmental and human health impacts of plastics are materialized through both plastic and non-plastic emissions throughout the life cycle, and therefore the overall life-cycle impacts of the polymer type and its application in combination should serve as the basis of determining the polymers of concern. Without the full life-cycle perspective, focusing on only one stage of the life cycle or one environmental problem may lead to a problem-shifting or regrettable substitutions (Fantke et al., 2020; Kouloumpis et al., 2020; Ren et al., 2020; Miller, 2022; Qadeer et al., 2022).
This general principle, however, has limitations in practice, because (1) our understanding of the life cycle environmental and human health impacts of polymers is limited due e.g., to data limitation, nascent stage of research, and unknown unknowns; (2) there are uncertainties in the measurements and models used for LCAs; and (3) the use of multiple impact categories in LCA often leads to a trade-off situation with no unequivocally superior or inferior choices. Some of these issues have been pointed out as a limitation of LCA when evaluating plastics and polymer-based materials (Plastic Soup Foundation, 2019; Miller, 2022; Tabuchi, 2022; Oberschelp et al., 2023).
In the presence of these limitations, the use of precautionary principles and a broader set of metrics, in addition to LCA-based metrics is necessary in defining polymers of concern (Foster et al., 2000; Kriebel et al., 2001). For example, minimizing the amount of plastics entering the human and natural environment targeting the most impactful plastic types, applications, and geographies, while our understanding of their environmental and human health impacts is still maturing, would be desirable. Wagner et al. (2024) also consider a broad range of criteria to identify polymers of concern, broadly grouped under “hazards” related to the polymers and the polymer's “compatibility with circularity.”
Finally, the availability and cost, as well as the life-cycle environmental consequences of the potential alternatives should be carefully evaluated to avoid “regrettable substitutions.”
Overall, determining polymers of concern should consider (1) the magnitude and severity of the impacts throughout the life cycle, (2) the volume of the plastics emitted to the human and natural environment, and (3) the availability and cost of alternatives, as well as the criticality of the service the particular polymer application renders.
From the following section, we attempt to map these criteria to high volume plastics based on the information and data available.
3 Mapping the concern criteria with high-volume plastics
Tables 1–4 attempt to map these criteria to high volume plastics based on the information and data available. Table 1 provides information for criterion 1 (health and safety issues) for high-volume polymers. The intention is to provide an initial appraisal of the issues reported in the literature rather than providing an exhaustive list.
LCAs of various polymers and their application types have been reported in the literature (Plastics Europe, 2013; Laurent et al., 2014; Rikhter et al., 2022). Harmonizing the differences in the assumptions, system boundaries, and underlying datasets used for those individual LCAs, however, is beyond the scope of this study. Therefore, we have used a recent dataset, cm.chemicals, which covers a wide range of plastic types and applications (Stellner et al., 2023). The life cycle inventory data from cm.chemicals are representative for the life cycle until the polymer is produced (i.e., cradle-to-gate) and are characterized using ReCiPe 2016 methodology for conventional impact categories (Table 2) (Huijbregts et al., 2017). The regional variation in feedstock, underlying energy mix, and uncertainties in raw data result in a large variability between minimum and maximum values (Table 2).
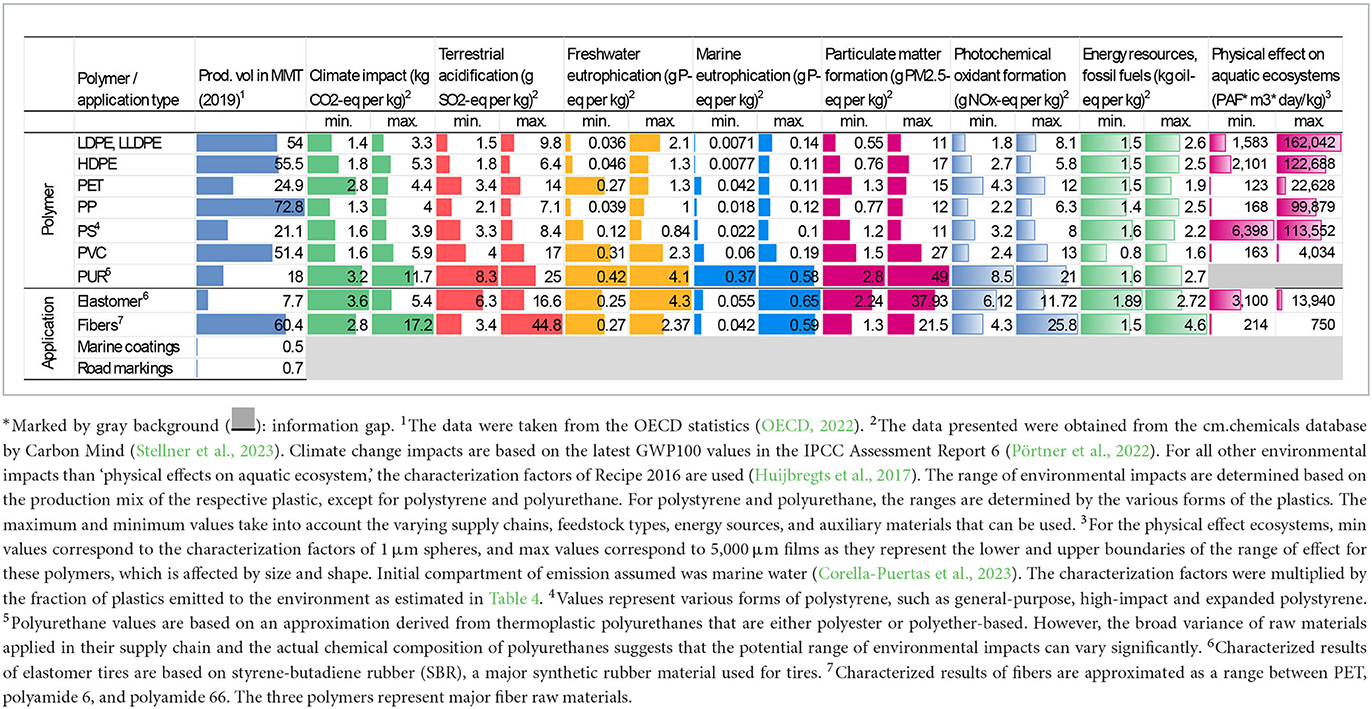
Table 2. Annual production volumes and characterized life-cycle impacts of major polymers and application types (criterion 2)*.
Overall, polyurethane (PUR) exhibits relatively large life-cycle environmental impacts per kg, while its volume of production is relatively small as compared to other types of polymers. Furthermore, PUR's main domains of application are generally more durable uses compared to other large-volume polymers. Characterized impacts for conventional impact categories of other polymer types are within similar ranges (Table 2). In terms of the physical impacts caused by the polymers emitted to the marine environment, we used the characterization factors developed by MariLCA project (Corella-Puertas et al., 2023), together with the estimated emissions to marine environment using Hoseini and Bond (2022) and OECD data (last columns in Table 2) (OECD, 2022). According to these estimates, polyethylene (LDPE, LLDPE, and HDPE) stood out as the major cause of physical impacts to aquatic ecosystem, followed by polystyrene (PS) and polypropylene (PP).
In addition, polymers are grouped by their main application types such as elastomer, fibers, marine coatings, and road markings (Table 2). The data shows that fibers are produced in high volume and their characterized impacts are sizable as compared to other applications, calling for an attention. These higher environmental impacts are mainly representative for the polyamide, a polymer used in, for instance, nylon. This polyamide supply chain includes several energy-intensive production steps to produce intermediate products such as caprolactam or adipic acid, which additionally lead to nitrous oxide emissions. These nitrous oxide emissions, in return, increase the overall environmental impacts of polyamide-based fibers.
Please note that the information provided on the environmental impacts of each polymer is not sufficient for comparative assertions regarding their overall sustainability or environmental impacts.
It should be noted that these datasets are far from complete or perfect. For example, conventional incineration of some plastics are known to generate toxic pollutants such as furans, dioxins, and polycyclic aromatic hydrocarbons (PAHs) due to incomplete combustion and the lack of adequate pollution control (Wang et al., 2003; Buekens and Cen, 2011; Zhang et al., 2016). However, allocating such pollutants to each input materials from a multi-input, multi-output process, such as incineration, is cumbersome, and existing databases often fall short in providing fully allocated emissions from such processes. Furthermore, LCA results and the main contributors of life-cycle impacts may widely vary depending on the domain of application and location of application, which affects various key aspects of plastics' life-cycle including underlying energy mix, waste management options, and feedstock choices. Therefore, the information presented in Table 2 should be interpreted with caution.
Table 3 provides an initial assessment of criterion 3 (circularity) for high-volume polymers and their main areas of application, based on available evidence of recycling “in practice and at scale” of different applications of each of the polymers (Ellen MacArthur Foundation., 2024). For many of these applications, alternatives are available as shown in the table, while the information on their costs and life-cycle environmental impacts is sparse; the intention here is to provide a sample of possible alternatives found in the literature rather than proposing them as definitive alternatives with superior environmental, technical and economic qualities; further assessments are needed for each to ensure affordability, safety and environmental and technical superiority, if any. Please note that the information provided about the environmental impacts of each polymer is not sufficient for comparative assertions regarding their overall sustainability or environmental impacts. It should also be noted that substantial information gaps remain to be filled in these tables.
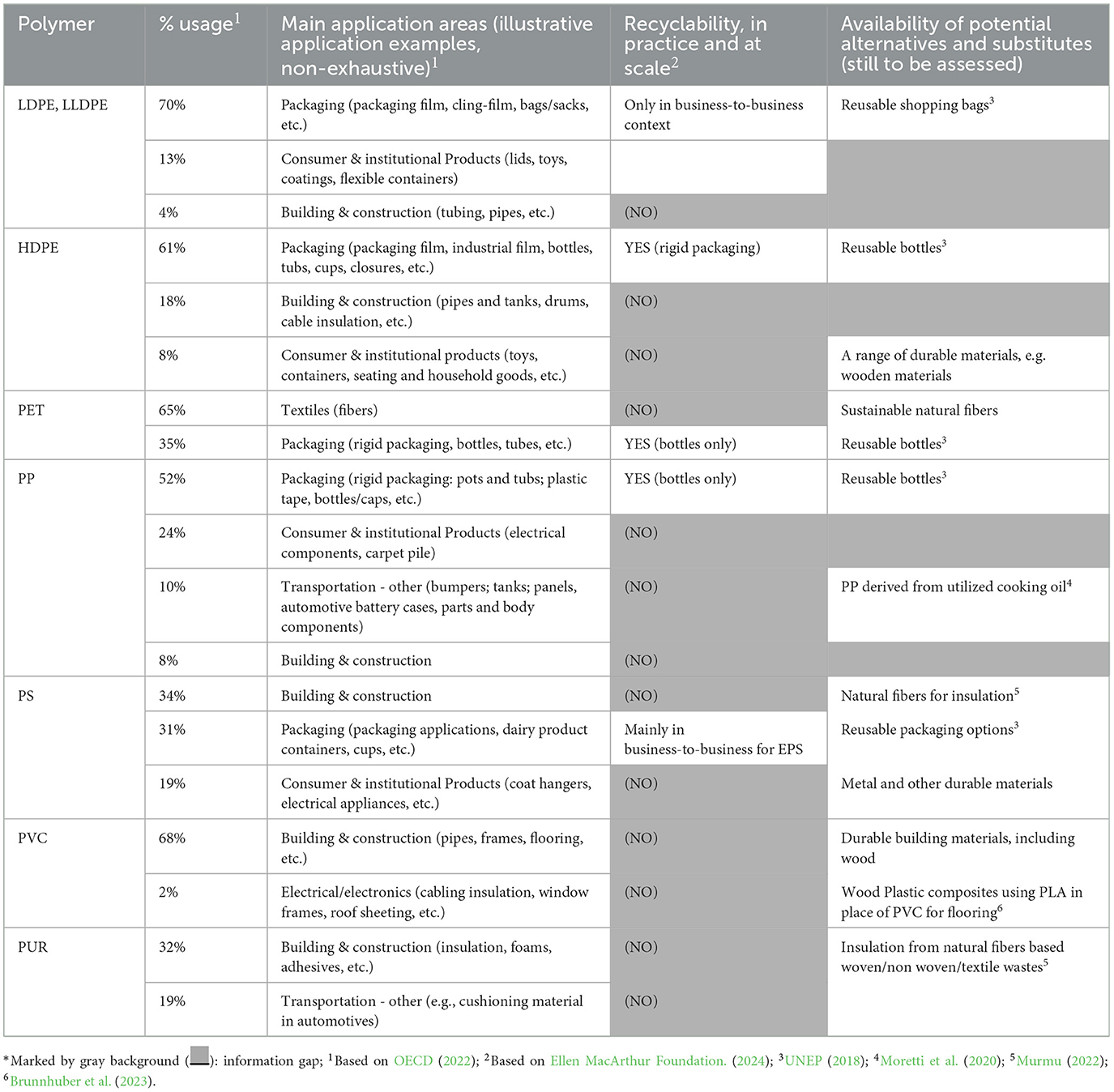
Table 3. Major polymer types, their main applications, their circularity (criterion 3), and sample of available potential alternatives*.
Table 4 shows the annual release of polymers to the environment based on Hoseini and Bond (2022) and OECD (2022). Among the polymer types, polyethylene (LDPE, LLDPE, and HDPE), PET and PP are identified as the key contributors, while fibers and elastomers release substantial amount of polymers to the environment among the major application types (Table 4).
It is notable that some of the major domains of application for large-volume polymers such as LDPE, LLDPE, HDPE, PET, PP, and PS are prone to pose the risk of leakage to the environment (Table 4). Packaging, for example, is the largest domain of application for LDPE, LLDPE, PET, PP, and PS, and their in-use lives are relatively short and the risk of leakage to the environment are reported to be high (Table 4).
4 Summary and conclusions
4.1 Summary of findings
In this study, the environmental and health impacts of polymers have been reviewed with the particular attention to the criteria that could be considered when identifying Polymers of Concern (PoC). Based on the literature reviewed, we identified (1) the health and safety issues associated with the chemicals used or incorporated in polymers and (2) the environmental and human health impacts from the pollutants generated throughout the life cycle of polymers as the main criteria for determining PoC. In addition, the importance of recycling in practice at scale in addressing multiple concerns around plastics and our limited knowledge and understanding of the behavior and impacts of the plastics in the environment and the uncertainties and data gaps in life cycle metrics and data warrant two additional criteria, (3) circularity, and (4) the volume of plastics entering the environment. The criteria are mapped to the available datasets highlighting the areas that need additional research.
The large variability in life-cycle impacts of polymers, which are often over one order of magnitude between minimum and maximum, suggests that not only the polymer types but also various underlying factors, such as the production technology used, underlying energy mix, and feedstock composition, determine the magnitude of these impacts. In addition, some polymers exhibit higher impacts than others, although not for all impact categories are presented in Table 1. For instance, PUR and PVC tend to have higher impacts than other polymers, except energy resources depletion in the case of PVC and physical effect on aquatic ecosystems for both polymers. In terms of the physical impacts to the aquatic ecosystem, polyethylene materials including LDPE, LLDPE, and HDPE stand out followed by PS and PP.
It is notable, however, that the information on environmental impacts per kg polymers does not offer a sufficient ground for comparing between polymers. For example, PUR and EPS exhibit different thermal insulation properties, so that comparing 1 kg PUR against 1 kg PS does not render a proper comparison when choosing the material for building insulation application.
Both elastomer and fiber applications of polymers are shown to generate substantial polymer emissions to the environment (Table 2). Large-volume emissions are associated with polyethylene materials (LDPE, LLDPE and HDPE), PET and PS (Table 2). However, the risk of leakage to the environment is often determined by the domain of application rather than the type of polymer (Table 4). This is also the case for the potential for recyclability (Table 3).
One important dimension worth noting is the uncertainty of these values. Freshwater eutrophication and Marine eutrophication categories, in particular, show 2–3 orders of magnitude difference between min and max. Physical effects on aquatic ecosystems show a similar issue. Some of them show 4 orders of magnitude difference, while PS only shows 2 orders of magnitude difference, making PP look worse than others when we look at the minimum impact values; as mentioned above this is likely caused by the extremely low density of many PS applications. While this uncertainty also indicates where future research efforts should be directed to, the current level of knowledge coupled with the precautionary principle already offer basis for regulating them on a start-and-strengthen approach.
4.2 Identification of the gaps and future works
The environmental and health impacts of plastics emitted to the environment are the subject of active research. The first sets of characterization factors for plastics emissions have been developed recently, while further refinements and expansions in characterization modeling are on-going (Woods et al., 2021; Lavoie et al., 2022; Maga et al., 2022; Corella-Puertas et al., 2023). The areas that need further research include:
• The method to quantify the impacts of plastics through e.g., entanglement, entrapment, and suffocation and integrating it into life cycle impact assessment (LCIA) methods for ecosystem health;
• Empirically backed models for the fate, transport, and effects of polymers emitted to human and natural environments including their fragmentation, degradation, leaching, and toxicological characteristics;
• Better quantitative evaluation of the micro- and nano-plastic release potential from plastic products, how their sizes and shapes affect human exposure potential to the polymers themselves as well as the associated chemicals embedded within, and the resulting human health impacts;
• Understanding the volume of plastics leaked to environment by region, plastic types and their applications;
• The effects that associated chemical, including fillers, colorants, plasticizers, and non-intentionally added substances have on the life-cycle environmental impacts of polymers;
• Understanding the composition of the additives/contaminants, which exist in the polymer matrix after the polymers' intended first use and while recycling, to limit the migration of non-intentionally added substances (NIAS).
• Emission factors of other air pollutants emitted when various plastic products are incinerated at their end of life and the associated human and ecosystem health impacts;
• The life-cycle environmental impacts, costs, and availability of substitutes and alternatives for plastics; and
• Allocation method based on physical causality for the emissions from multi-input process such as incineration.
4.3 Policy implications
Despite the limitations in the current knowledge and understanding as discussed in the previous section, urgent actions are needed to minimize further damages by polymers to the environment. To that end, the concept of polymers of concern (PoC) provides a focus for global regulatory efforts by highlighting intrinsic and extrinsic properties of the polymers that can be used to prioritize regulation on those that cause more concern.
We recommend policymakers to examine not only hazard-based criteria but also life-cycle-based criteria in the context of polymer type and the domain of applications. We believe that regulatory targets should be defined by the polymer types and corresponding domain of applications that most adversely affect the environment and human health throughout the life cycle. However, given the presence of uncertainties and information gaps, we believe that additional criteria should be considered following the precautionary principle including the volume of polymer emissions to the environment. We believe that cost, availability and environmental impacts of polymer alternatives should be carefully examined before a ban or phasing down of a polymer application is implemented.
It is also important to consider local and regional capacities (i.e., analytical facilities, institutional setup etc.) when regulating polymers (as well as chemicals or products). For example, if restrictions on certain polymers are imposed only in some regions, those polymers may be diverted to the regions with less capacity to monitor, assess or regulate them.
In addition, there is a need for harmonized information disclosure and reporting and international cooperation. In particular, policymakers should consider labeling and information disclosure on chemical constituents and associated chemicals used in plastics and plastics products.
Lastly, we recognize the challenges of phasing out a polymer or chemical (Ujaczki et al., 2022), and therefore we believe that it is important for policy makers to consider the whole suite of approaches to mitigating the adverse impacts of plastics, not just ban and phase-out. They include, but are not limited to: (1) economic incentives / disincentives to correct the market failure that makes environmentally worse products appear cheaper (e.g., regulation to favor reusable over single-use products); (2) alternative design of product applications (such as single-material bottles or shoes for easier recycling or mandating the one-stroke peelable labels on PET bottle), (3) regulatory and economic incentives for better collection and recycling of end-of-life plastics, (4) intercepting plastics in major riverine that feed plastics to ocean, and (5) alternative disposal system for wastewater treatment facilities.
Author's note
A shorter version of this document will be published as a Policy Brief by the United Nations Environmental Programme (UNEP). The current article is intended to provide the full context and references in support of the Policy Brief . The views expressed in this article are those of the authors and do not necessarily reflect those of the United Nations Environment Programme.
Author contributions
SS: Conceptualization, Formal analysis, Funding acquisition, Investigation, Methodology, Project administration, Supervision, Visualization, Writing – original draft, Writing – review & editing. A-MB: Conceptualization, Formal analysis, Investigation, Methodology, Writing – original draft, Writing – review & editing. PF: Conceptualization, Formal analysis, Investigation, Methodology, Writing – original draft, Writing – review & editing. DL: Conceptualization, Formal analysis, Investigation, Methodology, Writing – original draft, Writing – review & editing. DM: Conceptualization, Formal analysis, Investigation, Methodology, Writing – original draft, Writing – review & editing. RM: Conceptualization, Formal analysis, Investigation, Methodology, Writing – original draft, Writing – review & editing. LM: Conceptualization, Formal analysis, Funding acquisition, Investigation, Methodology, Project administration, Resources, Supervision, Writing – original draft, Writing – review & editing.
Funding
The author(s) declare financial support was received for the research, authorship, and/or publication of this article. PF was supported by the Partnership for the Assessment of Risks from Chemicals (PARC) project (grant 101057014) funded under the European Union's Horizon Europe Research and Innovation program.
Conflict of interest
SS was employed by Watershed Technology Inc. RM was employed by Carbon Minds GmbH.
The remaining authors declare that the research was conducted in the absence of any commercial or financial relationships that could be construed as a potential conflict of interest.
The author(s) declared that they were an editorial board member of Frontiers, at the time of submission. This had no impact on the peer review process and the final decision.
Publisher's note
All claims expressed in this article are solely those of the authors and do not necessarily represent those of their affiliated organizations, or those of the publisher, the editors and the reviewers. Any product that may be evaluated in this article, or claim that may be made by its manufacturer, is not guaranteed or endorsed by the publisher.
References
Abiola, B. A., Visser, M., and Daniels, R. C. (2023). Addressing plastic bags consumption crises through store monetary and non-monetary interventions in South Africa. Front. Sustain. 3:968886. doi: 10.3389/frsus.2022.968886
Alexiadou, P., Foskolos, I., and Frantzis, A. (2019). Ingestion of macroplastics by odontocetes of the Greek Seas, Eastern Mediterranean: Often deadly! Mar. Pollut. Bull. 146, 67–75. doi: 10.1016/j.marpolbul.2019.05.055
Allen, D. T., Chen, Q., and Dunn, J. B. (2021). Consistent Metrics Needed for Quantifying Methane Emissions from Upstream Oil and Gas Operations. Environ. Sci. Technol. Lett. 8, 345–349. doi: 10.1021/acs.estlett.0c00907
Allison, A. L., Purkiss, D., Lorencatto, F., Miodownik, M., and Michie, S. (2022). Improving compostable plastic disposal: an application of the Behaviour Change Wheel intervention development method. Front. Sustain. 3:968152. doi: 10.3389/frsus.2022.968152
Andersen, S. O., Gao, S., Carvalho, S., Ferris, T., Gonzalez, M., Sherman, N. J., et al. (2021). Narrowing feedstock exemptions under the Montreal Protocol has multiple environmental benefits. Proc. Natl. Acad. Sci. 118:e2022668118. doi: 10.1073/pnas.2022668118
Andrady, A. L., and Neal, M. A. (2009). Applications and societal benefits of plastics. Philos Trans. R Soc. B Biol. Sci. 364, 1977–1984. doi: 10.1098/rstb.2008.0304
Aurisano, N., Huang, L., i Canals, L. M., Jolliet, O., and Fantke, P. (2021a). Chemicals of concern in plastic toys. Environ. Int. 146, 106194. doi: 10.1016/j.envint.2020.106194
Aurisano, N., Weber, R., and Fantke, P. (2021b). Enabling a circular economy for chemicals in plastics. Curr. Opin. Green Sustain. Chem. 31, 100513. doi: 10.1016/j.cogsc.2021.100513
Bachmann, M., Zibunas, C., Hartmann, J., Tulus, V., Suh, S., Guillén-Gosálbez, G., et al. (2023). Towards circular plastics within planetary boundaries. Nat. Sustain. 6, 599–610. doi: 10.1038/s41893-022-01054-9
Baldassarre, B., Maury, T., Mathieux, F., Garbarino, E., Antonopoulos, I., and Sala, S. (2022). Drivers and barriers to the circular economy transition: the case of recycled plastics in the automotive sector in the European Union. Procedia CIRP 105, 37–42. doi: 10.1016/j.procir.2022.02.007
Barboza, L. G. A., Cózar, A., Gimenez, B. C., Barros, T. L., Kershaw, P. J., and Guilhermino, L. (2019). “Chapter 17 - Macroplastics Pollution in the Marine Environment,” in World Seas: An Environmental Evaluation (Second Edition), ed. C. Sheppard (London: Academic Press). doi: 10.1016/B978-0-12-805052-1.00019-X
Barnes, D. K. A., Walters, A., and Gonçalves, L. (2010). Macroplastics at sea around Antarctica. Mar. Environ. Res. 70, 250–252. doi: 10.1016/j.marenvres.2010.05.006
Beaumont, N. J., Aanesen, M., Austen, M. C., Börger, T., Clark, J. R., Cole, M., et al. (2019). Global ecological, social and economic impacts of marine plastic. Mar. Pollut. Bull. 142, 189–195. doi: 10.1016/j.marpolbul.2019.03.022
Bidashimwa, D, Hoke, T, Huynh, T. B, Narkpitaks, N, Priyonugroho, K, and Ha, T. T. (2023). Plastic pollution: how can the global health community fight the growing problem? BMJ Glob. Health 8:e012140. doi: 10.1136/bmjgh-2023-012140
Bishop, G., Styles, D., and Lens, P. N. L. (2020). Recycling of European plastic is a pathway for plastic debris in the ocean. Environ. Int. 142:105893. doi: 10.1016/j.envint.2020.105893
Blettler, M. C. M., and Mitchell, C. (2021). Dangerous traps: Macroplastic encounters affecting freshwater and terrestrial wildlife. Sci. Total Environ. 798:149317. doi: 10.1016/j.scitotenv.2021.149317
Borrelle, S. B, Ringma, J, Law, K. L, Monnahan, C. C, Lebreton, L, and McGivern, A. (2020). Predicted growth in plastic waste exceeds efforts to mitigate plastic pollution. Science 369, 1515–1518. doi: 10.1126/science.aba3656
British Plastics Federation (2021). Plastic Recycling. Available online at: https://www.bpf.co.uk/sustainability/plastics_recycling.aspx (accessed February 8, 2023).
Brunnhuber, N., Windsperger, A., Perdomo Echenique, E. A., and Hesser, F. (2023). “Implementing ecodesign during product development: an ex-ante life cycle assessment of wood-plastic composites,” in Progress in Life Cycle Assessment 2021, eds. F. Hesser, I. Kral, G. Obersteiner (Cham: Springer International Publishing). doi: 10.1007/978-3-031-29294-1_3
Buekens, A., and Cen, K. (2011). Waste incineration, PVC, and dioxins. J. Mater. Cycles Waste Manag. 13, 190–197. doi: 10.1007/s10163-011-0018-9
Bui, T. T., Giovanoulis, G., Cousins, A. P., Magnér, J., Cousins, I. T., and de Wit, C. A. (2016). Human exposure, hazard and risk of alternative plasticizers to phthalate esters. Sci. Total Environ. 541, 451–467. doi: 10.1016/j.scitotenv.2015.09.036
Burns, D., and Grubert, E. (2021). Attribution of production-stage methane emissions to assess spatial variability in the climate intensity of US natural gas consumption. Environ. Res. Lett. 16:044059. doi: 10.1088/1748-9326/abef33
Cabernard, L., Pfister, S., Oberschelp, C., and Hellweg, S. (2022). Growing environmental footprInt. of plastics driven by coal combustion. Nat. Sustain. 5, 139–148. doi: 10.1038/s41893-021-00807-2
Chamas, A., Moon, H., Zheng, J., Qiu, Y., Tabassum, T., Jang, J. H., et al. (2020). Degradation rates of plastics in the environment. ACS Sustain. Chem. Eng. 8, 3494–3511. doi: 10.1021/acssuschemeng.9b06635
Chen, Q., Gao, J., Yu, H., Su, H., Yang, Y., Cao, Y., et al. (2022). An emerging role of microplastics in the etiology of lung ground glass nodules. Environ. Sci. Eur. 34:25. doi: 10.1186/s12302-022-00605-3
Chu, J., Zhou, Y., Cai, Y., Wang, X., Li, C., and Liu, Q. (2023). Flows and waste reduction strategies of PE, PP, and PET plastics under plastic limit order in China. Resour. Conserv. Recycl. 188:106668. doi: 10.1016/j.resconrec.2022.106668
Corella-Puertas, E., Hajjar, C., Lavoie, J., and Boulay, A.-M. (2023). MarILCA characterization factors for microplastic impacts in life cycle assessment: Physical effects on biota from emissions to aquatic environments. J. Clean Prod. 418:138197. doi: 10.1016/j.jclepro.2023.138197
Croxatto Vega, G., Gross, A., and Birkved, M. (2021). The impacts of plastic products on air pollution - A simulation study for advanced life cycle inventories of plastics covering secondary microplastic production. Sustain. Prod. Consum. 28, 848–865. doi: 10.1016/j.spc.2021.07.008
Derraik, J. G. B. (2002). The pollution of the marine environment by plastic debris: a review. Mar. Pollut. Bull. 44, 842–852. doi: 10.1016/S0025-326X(02)00220-5
Dodson, R. E., Nishioka, M., Standley, L. J., Perovich, L. J., Brody, J. G., and Rudel, R. A. (2012). Endocrine disruptors and asthma-associated chemicals in consumer products. Environ. Health Perspect. 120, 935–943. doi: 10.1289/ehp.1104052
Duan, J., Bolan, N., Li, Y., Ding, S., Atugoda, T., Vithanage, M., et al. (2021). Weathering of microplastics and interaction with other coexisting constituents in terrestrial and aquatic environments. Water Res. 196, 117011. doi: 10.1016/j.watres.2021.117011
Ellen MacArthur Foundation (2020). Global Commitment - Definitions and Framework | Shared by New Plastics Economy.
Ellen MacArthur Foundation (2024). 2024 Recycling Rate Survey results summary. The Isle of Wight, UK. Available online at: https://emf.thirdlight.com/link/6bnvju827y0v-rr0pqw/@/
European Commission (2005). Directive 2005/84/EC of the European Parliament and of the Council of 14 December 2005 amending for the 22nd time Council Directive 76/769/EEC on the approximation of the laws, regulations and administrative provisions of the Member States relating to restrictions on the marketing and use of certain dangerous substances and preparations (phthalates in toys and childcare articles).
European Commission (2022). Directive (EU) 2022/2464 of the European Parliament and of the Council of 14 December 2022 amending Regulation (EU) No 537/2014, Directive 2004/109/EC, Directive 2006/43/EC and Directive 2013/34/EU, as regards corporate sustainability reporting (Text with EEA relevance).
Fantke, P., Huang, L., Overcash, M., Griffing, E., and Jolliet, O. (2020). Life cycle based alternatives assessment (LCAA) for chemical substitution. Green Chem. 22, 6008–6024. doi: 10.1039/D0GC01544J
Finnveden, G., Hauschild, M. Z., Ekvall, T., Guinée, J., Heijungs, R., Hellweg, S., et al. (2009). Recent developments in life cycle assessment. J. Environ. Manage. 91, 1–21. doi: 10.1016/j.jenvman.2009.06.018
Fogt Jacobsen, L., Pedersen, S., and Thøgersen, J. (2022). Drivers of and barriers to consumers' plastic packaging waste avoidance and recycling – A systematic literature review. Waste Manag. 141, 63–78. doi: 10.1016/j.wasman.2022.01.021
Foster, K. R., Vecchia, P., and Repacholi, M. H. (2000). Science and the precautionary principle. Science 288, 979–981. doi: 10.1126/science.288.5468.979
Gall, S. C., and Thompson, R. C. (2015). The impact of debris on marine life. Mar. Pollut. Bull. 92, 170–179. doi: 10.1016/j.marpolbul.2014.12.041
Garcia, J. M., and Robertson, M. L. (2017). The future of plastics recycling. Science 358, 870–872. doi: 10.1126/science.aaq0324
García-Gómez, J. C., Garrigós, M., and Garrigós, J. (2021). Plastic as a vector of dispersion for marine species with invasive potential: A review. Front. Ecol. Evol. 9:629756. doi: 10.3389/fevo.2021.629756
Gavigan, J., Kefela, T., Macadam-Somer, I., Suh, S., and Geyer, R. (2020). Synthetic microfiber emissions to land rival those to waterbodies and are growing. PLoS ONE 15:e0237839. doi: 10.1371/journal.pone.0237839
Geueke, B., Groh, K. J., Maffini, M. V., Martin, O. V., Boucher, J. M., Chiang, Y.-T., et al. (2023). Systematic evidence on migrating and extractable food contact chemicals: most chemicals detected in food contact materials are not listed for use. Crit. Rev. Food Sci. Nutr. 63, 9425–9435. doi: 10.1080/10408398.2022.2067828
Geyer, R. (2020). “Chapter 2 - Production, use, and fate of synthetic polymers,” in Plastic Waste and Recycling, ed. T. M. Letcher (New York: Academic Press). doi: 10.1016/B978-0-12-817880-5.00002-5
Geyer, R., Gavigan, J., Jackson, A. M., Saccomanno, V. R., Suh, S., and Gleason, M. G. (2022). Quantity and fate of synthetic microfiber emissions from apparel washing in California and strategies for their reduction. Environ. Pollut. 298:118835. doi: 10.1016/j.envpol.2022.118835
Geyer, R., Jambeck, J. R., and Law, K. L. (2017). Production, use, and fate of all plastics ever made. Sci. Adv. 3:e1700782. doi: 10.1126/sciadv.1700782
Goevert, D. (2024). The value of different recycling technologies for waste rubber tires in the circular economy—A review. Front. Sustain. 4:1282805. doi: 10.3389/frsus.2023.1282805
Gregory, M. R. (2009). Environmental implications of plastic debris in marine settings—entanglement, ingestion, smothering, hangers-on, hitch-hiking and alien invasions. Philos Trans. R Soc. B Biol. Sci. 364, 2013–2025. doi: 10.1098/rstb.2008.0265
Groh, K. J., Arp, H. P. H., MacLeod, M., and Wang, Z. (2023). Assessing and managing environmental hazards of polymers: historical development, science advances and policy options. Environ. Sci. Proc. Impacts 25, 10–25. doi: 10.1039/D2EM00386D
Guinée, J. B., Gorrée, M., Heijungs, R., Huppes, G., Kleijn, R., de Koning, A., et al. (2002). Handbook on Life Cycle Assessment: Operational Guide to the ISO Standards. Cham: Springer Science & Business Media. doi: 10.1007/0-306-48055-7
Guo, Z., Tang, X., Wang, W., Luo, Z., Zeng, Y., Zhou, N., et al. (2025). The photo-based treatment technology simultaneously removes resistant bacteria and resistant genes from wastewater. J. Environ. Sci. 148, 243–262. doi: 10.1016/j.jes.2024.01.005
Halden, R. U. (2010). Plastics and health risks. Annu. Rev. Public Health 31, 179–194. doi: 10.1146/annurev.publhealth.012809.103714
Hamilton, S., and Baker, G. B. (2019). Technical mitigation to reduce marine mammal bycatch and entanglement in commercial fishing gear: lessons learnt and future directions. Rev. Fish Biol. Fish 29, 223–247. doi: 10.1007/s11160-019-09550-6
Heath, R. (2017). “Isocyanate-based polymers: polyurethanes, polyureas, polyisocyanurates, and their Copolymers,” in Brydson's Plastics Materials (Butterworth: Heinemann), 799–835.
Høiberg, M. A., Woods, J. S., and Verones, F. (2022). Global distribution of potential impact hotspots for marine plastic debris entanglement. Ecol. Indic. 135:108509. doi: 10.1016/j.ecolind.2021.108509
Hollóczki, O., and Gehrke, S. (2019). Nanoplastics can change the secondary structure of proteins. Sci. Rep. 9:16013. doi: 10.1038/s41598-019-52495-w
Hopewell, J., Dvorak, R., and Kosior, E. (2009). Plastics recycling: challenges and opportunities. Philos Trans. R Soc. B Biol. Sci. 364, 2115–2126. doi: 10.1098/rstb.2008.0311
Hoseini, M., and Bond, T. (2022). Predicting the global environmental distribution of plastic polymers. Environ. Pollut. 300:118966. doi: 10.1016/j.envpol.2022.118966
Huang, L., Fantke, P., Ritscher, A., and Jolliet, O. (2022). Chemicals of concern in building materials: a high-throughput screening. J. Hazard. Mater. 424:127574. doi: 10.1016/j.jhazmat.2021.127574
Huff, J., and Infante, P. F. (2011). Styrene exposure and risk of cancer. Mutagenesis 26, 583–584. doi: 10.1093/mutage/ger033
Huijbregts, M. A., Steinmann, Z. J., Elshout, P. M., Stam, G., Verones, F., Vieira, M., et al. (2017). ReCiPe2016: a harmonised life cycle impact assessment method at midpoInt. and endpoInt. level. Int. J. Life Cycle Assess 22, 138–147. doi: 10.1007/s11367-016-1246-y
Hurley, R. R., and Nizzetto, L. (2018). Fate and occurrence of micro (nano) plastics in soils: Knowledge gaps and possible risks. Curr. Opin. Environ. Sci. Health 1, 6–11. doi: 10.1016/j.coesh.2017.10.006
Idumah, C. I., and Nwuzor, I. C. (2019). Novel trends in plastic waste management. SN Appl. Sci. 1:1402. doi: 10.1007/s42452-019-1468-2
Iizuka, A., Mizukoshi, A., Noguchi, M., and Yamasaki, A. (2020). Emission fluxes of styrene monomers and other chemicals for products containing expanded polystyrene beads. PLoS ONE 15:e0239458. doi: 10.1371/journal.pone.0239458
ISO (2006a). ISO 14040: Environmental management — Life cycle assessment — Principles and framework. Geneva: International Standards Organization.
ISO (2006b). ISO 14044: Environmental management — Life cycle assessment — Requirements and guidelines. Geneva: International Standards Organization.
Jambeck, J. R., Geyer, R., Wilcox, C., Siegler, T. R., Perryman, M., Andrady, A., et al. (2015). Plastic waste inputs from land into the ocean. Science 347, 768–771. doi: 10.1126/science.1260352
Jiao, M., Cao, S., Ren, L., and Li, R. (2021). Analysis of composite microplastics in sediment using 3D Raman spectroscopy and imaging method. J. Hazar. Mater. Adv. 3:100016. doi: 10.1016/j.hazadv.2021.100016
Johnson, A., Salvador, G., Kenney, J., Robbins, J., Kraus, S. D., Landry, S., et al. (2005). Fishing gear involved in entanglements of right and Humpback Whales. Mar. Mammal. Sci. 21, 635–645. doi: 10.1111/j.1748-7692.2005.tb01256.x
Kacprzak, S., and Tijing, L. D. (2022). Microplastics in indoor environment: Sources, mitigation and fate. J. Environ. Chem. Eng. 10:107359. doi: 10.1016/j.jece.2022.107359
Kätelhön, A., Meys, R., Deutz, S., Suh, S., and Bardow, A. (2019). Climate change mitigation potential of carbon capture and utilization in the chemical industry. Proc. Natl. Acad. Sci. 116, 11187–11194. doi: 10.1073/pnas.1821029116
Katsikantami, I., Sifakis, S., Tzatzarakis, M. N., Vakonaki, E., Kalantzi, O. I., Tsatsakis, A. M., et al. (2016). A global assessment of phthalates burden and related links to health effects. Environ. Int. 97, 212–236. doi: 10.1016/j.envint.2016.09.013
Keane, M. A. (2007). Catalytic conversion of waste plastics: focus on waste PVC. J. Chem. Technol. BioTechnol. 82, 787–795. doi: 10.1002/jctb.1757
Koornneef, J., Ramírez, A., Turkenburg, W., and Faaij, A. (2012). The environmental impact and risk assessment of CO2 capture, transport and storage – An evaluation of the knowledge base. Prog. Energy Combust. Sci. 38, 62–86. doi: 10.1016/j.pecs.2011.05.002
Kopatz, V., Wen, K., Kovács, T., Keimowitz, A. S., Pichler, V., Widder, J., et al. (2023). Micro- and nanoplastics breach the blood–brain barrier (BBB): biomolecular corona's role revealed. Nanomaterials 13:1404. doi: 10.3390/nano13081404
Kouloumpis, V., Pell, R. S., Correa-Cano, M. E., and Yan, X. (2020). Potential trade-offs between eliminating plastics and mitigating climate change: an LCA perspective on Polyethylene Terephthalate (PET) bottles in Cornwall. Sci. Total Environ. 727:138681. doi: 10.1016/j.scitotenv.2020.138681
Kriebel, D., Tickner, J., Epstein, P., Lemons, J., Levins, R., Loechler, E. L., et al. (2001). The precautionary principle in environmental science. Environ. Health Perspect. 109, 871–876. doi: 10.1289/ehp.01109871
Kulkarni, G. S. (2018). “1 - Introduction to Polymer and Their Recycling Techniques,” in Recycling of Polyurethane Foams, eds. S. Thomas, A. V. Rane, K. Kanny (Loncon: William Andrew Publishing). doi: 10.1016/B978-0-323-51133-9.00001-2
Kumar, M., Chen, H., Sarsaiya, S., Qin, S., Liu, H., Awasthi, M. K., et al. (2021). Current research trends on micro- and nano-plastics as an emerging threat to global environment: a review. J. Hazard Mater. 409, 124967. doi: 10.1016/j.jhazmat.2020.124967
Lau, W. W. Y., Shiran, Y., Bailey, R. M., Cook, E., Stuchtey, M. R., Koskella, J., et al. (2020). Evaluating scenarios toward zero plastic pollution. Science 369, 1455–1461. doi: 10.1126/science.aba9475
Laurent, A., Bakas, I., Clavreul, J., Bernstad, A., Niero, M., Gentil, E., et al. (2014). Review of LCA studies of solid waste management systems – Part I: lessons learned and perspectives. Waste Manag. 34, 573–588. doi: 10.1016/j.wasman.2013.10.045
Lavoie, J., Boulay, A.-M., and Bulle, C. (2022). Aquatic micro- and nano-plastics in life cycle assessment: development of an effect factor for the quantification of their physical impact on biota. J. Ind. Ecol. 26, 2123–2135. doi: 10.1111/jiec.13140
Law, K. L. (2017). Plastics in the marine environment. Annu. Rev. Mar. Sci. 9, 205–229. doi: 10.1146/annurev-marine-010816-060409
Lear, G., Kingsbury, J. M., Franchini, S., Gambarini, V., Maday, S. D. M., Wallbank, J. A., et al. (2021). Plastics and the microbiome: impacts and solutions. Environ. Microbiome 16:2. doi: 10.1186/s40793-020-00371-w
Lee, K., Jing, Y., Wang, Y., and Yan, N. (2022). A unified view on catalytic conversion of biomass and waste plastics. Nat. Rev. Chem. 6, 635–652. doi: 10.1038/s41570-022-00411-8
Lee, K. J. L., and Wong, S. F. (2023). Comparative environmental and socioeconomic assessment on mixed plastic waste management: a Singapore case study. Sci. Total Environ. 893:164884. doi: 10.1016/j.scitotenv.2023.164884
Lehr, A. L., Heider, K. L., Aboagye, E. A., Chea, J. D., Stengel, J. P., Benavides, P. T., et al. (2022). Design of solvent-assisted plastics recycling: Integrated economics and environmental impacts analysis. Front. Sustain. 3:989720. doi: 10.3389/frsus.2022.989720
Li, D., and Suh, S. (2019). Health risks of chemicals in consumer products: a review. Environ. Int. 123, 580–587. doi: 10.1016/j.envint.2018.12.033
Li, Y., Tao, L., Wang, Q., Wang, F., Li, G., and Song, M. (2023). Potential health impact of microplastics: a review of environmental distribution, human exposure, and toxic effects. Environ. Health 1, 249–257. doi: 10.1021/envhealth.3c00052
MacLeod, M., Arp, H. P. H., Tekman, M. B., and Jahnke, A. (2021). The global threat from plastic pollution. Science 373, 61–65. doi: 10.1126/science.abg5433
Maga, D., Galafton, C., Blömer, J., Thonemann, N., Özdamar, A., and Bertling, J. (2022). Methodology to address potential impacts of plastic emissions in life cycle assessment. Int. J. Life Cycle Assess 27, 469–491. doi: 10.1007/s11367-022-02040-1
Maier, R., Zweifel, H., and Schiller, M. (2009). Plastics Additives Handbook. New Tork: Hanser Publications.
Maquart, P.-O., Froehlich, Y., and Boyer, S. (2022). Plastic pollution and infectious diseases. Lancet Planet Health 6:e842–e845. doi: 10.1016/S2542-5196(22)00198-X
Marfella, R., Prattichizzo, F., Sardu, C., Fulgenzi, G., Graciotti, L., Spadoni, T., et al. (2024). Microplastics and nanoplastics in atheromas and cardiovascular events. N Engl. J. Med. 390, 900–910. doi: 10.1056/NEJMoa2309822
Marson, A., Masiero, M., Modesti, M., Scipioni, A., and Manzardo, A. (2021). Life cycle assessment of polyurethane foams from polyols obtained through chemical recycling. ACS Omega 6, 1718–1724. doi: 10.1021/acsomega.0c05844
Meijer, L. J. J., van Emmerik, T., van der Ent, R., Schmidt, C., and Lebreton, L. (2021). More than 1000 rivers account for 80% of global riverine plastic emissions into the ocean. Sci. Adv. 7:eaaz5803. doi: 10.1126/sciadv.aaz5803
Meys, R., Kätelhön, A., Bachmann, M., Winter, B., Zibunas, C., Suh, S., et al. (2021). Achieving net-zero greenhouse gas emission plastics by a circular carbon economy. Science 374, 71–76. doi: 10.1126/science.abg9853
Milios, L., Christensen, L. H., McKinnon, D., Christensen, C., Rasch, M. K., and Eriksen, M. H. (2018). Plastic recycling in the Nordics: a value chain market analysis. Waste Manag. 76, 180–189. doi: 10.1016/j.wasman.2018.03.034
Miller, S. A. (2022). The capabilities and deficiencies of life cycle assessment to address the plastic problem. Front. Sustain. 3:1007060. doi: 10.3389/frsus.2022.1007060
Montes-Grajales, D., Fennix-Agudelo, M., and Miranda-Castro, W. (2017). Occurrence of personal care products as emerging chemicals of concern in water resources: a review. Sci. Total Environ. 595, 601–614. doi: 10.1016/j.scitotenv.2017.03.286
Moretti, C., Junginger, M., and Shen, L. (2020). Environmental life cycle assessment of polypropylene made from used cooking oil. Resour. Conserv. Recycl. 157:104750. doi: 10.1016/j.resconrec.2020.104750
Muñoz-Pérez, J. P., Lewbart, G. A., Alarcón-Ruales, D., Skehel, A., Cobos, E., Rivera, R., et al. (2023). Galápagos and the plastic problem. Front. Sustain. 4:1091516. doi: 10.3389/frsus.2023.1091516
Murmu, S. B. (2022). Alternatives derived from renewable natural fibre to replace conventional polyurethane rigid foam insulation. Clean Eng. Technol. 8:100513. doi: 10.1016/j.clet.2022.100513
Mwanza, B. G., and Mbohwa, C. (2017). Drivers to sustainable plastic solid waste recycling: a review. Procedia Manuf. 8, 649–656. doi: 10.1016/j.promfg.2017.02.083
Napper, I. E., and Thompson, R. C. (2023). Plastics and the environment. Annu. Rev. Environ. Resour. 48, 55–79. doi: 10.1146/annurev-environ-112522-072642
Nguyen, H. T., Lee, Y. K., Kwon, J.-H., and Hur, J. (2023). Microplastic biofilms in water treatment systems: Fate and risks of pathogenic bacteria, antibiotic-resistant bacteria, and antibiotic resistance genes. Sci. Total Environ. 892:164523. doi: 10.1016/j.scitotenv.2023.164523
Nielsen, T. D., Hasselbalch, J., Holmberg, K., and Stripple, J. (2020). Politics and the plastic crisis: A review throughout the plastic life cycle. WIREs Energy Environ. 9:e360. doi: 10.1002/wene.360
Oberschelp, C., Hellweg, S., Bradford, E., Pfister, S., Huo, J., and Wang, Z. (2023). Poor data and outdated methods sabotage the decarbonization efforts of the chemical industry. Working paper. doi: 10.26434/chemrxiv-2023-8c86t
O'Brien, S., Okoffo, E. D., O'Brien, J. W., Ribeiro, F., Wang, X., Wright, S. L., et al. (2020). Airborne emissions of microplastic fibres from domestic laundry dryers. Sci. Total Environ. 747, 141175. doi: 10.1016/j.scitotenv.2020.141175
OECD (2022). Global Plastics Outlook: Economic Drivers, Environmental Impacts and Policy Options. Paris: Organisation for Economic Co-operation and Development.
Ögmundarson, Ó., Herrgård, M. J., Forster, J., Hauschild, M. Z., and Fantke, P. (2020). Addressing environmental sustainability of biochemicals. Nat. Sustain. 3, 167–174. doi: 10.1038/s41893-019-0442-8
Okan, M., Aydin, H. M., and Barsbay, M. (2019). Current approaches to waste polymer utilization and minimization: a review. J. Chem. Technol. BioTechnol. 94, 8–21. doi: 10.1002/jctb.5778
Otto, A., Grube, T., Schiebahn, S., and Stolten, D. (2015). Closing the loop: captured CO 2 as a feedstock in the chemical industry. Energy Environ. Sci. 8, 3283–3297. doi: 10.1039/C5EE02591E
Pacheco, E. B. A. V., Ronchetti, L. M., and Masanet, E. (2012). An overview of plastic recycling in Rio de Janeiro. Resour. Conserv. Recycl. 60, 140–146. doi: 10.1016/j.resconrec.2011.12.010
Peng, L., Fu, D., Qi, H., Lan, C. Q., Yu, H., and Ge, C. (2020). Micro- and nano-plastics in marine environment: Source, distribution and threats — a review. Sci. Total Environ. 698, 134254. doi: 10.1016/j.scitotenv.2019.134254
Persson, L., Carney Almroth, B. M., Collins, C. D., Cornell, S., De Wit, C. A., Diamond, M. L., et al. (2022). Outside the safe operating space of the planetary boundary for novel entities. Environ. Sci. Technol. 56, 1510–1521. doi: 10.1021/acs.est.1c04158
Plastic Soup Foundation (2019). The plastic industry abuses lifecycle analysis (LCA) in communication surrounding plastic pollution. Available online at: https://www.plasticsoupfoundation.org/en/2019/10/the-plastic-industry-abuses-lifecycle-analysis-lca-in-communication-surrounding-plastic-pollution/ (accessed February 12, 2024).
Plastics Europe (2013). Plastics Europe LCA database. Available online at: http://www.plasticseurope.org/plastics-sustainability/eco-profiles/browse-by-family.aspx (accessed April 18, 2024).
Pörtner, H. O., Roberts, D. C., Adams, H., Adler, C., Aldunce, P., Ali, E., et al. (2022). Climate change 2022: impacts, adaptation and vulnerability. IPCC.
Posen, I. D., Jaramillo, P., Landis, A. E., and Griffin, W. M. (2017). Greenhouse gas mitigation for U.S. plastics production: energy first, feedstocks later. Environ. Res. Lett. 12, 034024. doi: 10.1088/1748-9326/aa60a7
Qadeer, A., Kirsten, K. L., Ajmal, Z., Jiang, X., and Zhao, X. (2022). Alternative plasticizers as emerging global environmental and health threat: another regrettable substitution? Environ. Sci. Technol. 56, 1482–1488. doi: 10.1021/acs.est.1c08365
Qian, N., Gao, X., Lang, X., Deng, H., Bratu, T. M., Chen, Q., et al. (2024). Rapid single-particle chemical imaging of nanoplastics by SRS microscopy. Proc. Natl. Acad. Sci. U S A 121:e2300582121. doi: 10.1073/pnas.2300582121
Ragusa, A., Svelato, A., Santacroce, C., Catalano, P., Notarstefano, V., Carnevali, O., et al. (2021). Plasticenta: First evidence of microplastics in human placenta. Environ. Int. 146:106274. doi: 10.1016/j.envint.2020.106274
Rahimi, A., and García, J. M. (2017). Chemical recycling of waste plastics for new materials production. Nat. Rev. Chem. 1, 1–11. doi: 10.1038/s41570-017-0046
Raj, T., Chandrasekhar, K., Naresh Kumar, A., and Kim, S.-H. (2022). Lignocellulosic biomass as renewable feedstock for biodegradable and recyclable plastics production: A sustainable approach. Renew. Sustain. Energy Rev. 158:112130. doi: 10.1016/j.rser.2022.112130
Raubenheimer, K., and Urho, N. (2024). Global criteria to address problematic, unnecessary and avoidable plastic products. Copenhagen: Nordisk Ministerråd. doi: 10.6027/temanord2024-508
Rech, S., Salmina, S., Borrell Pichs, Y. J., and García-Vazquez, E. (2018). Dispersal of alien invasive species on anthropogenic litter from European mariculture areas. Mar. Pollut. Bull. 131, 10–16. doi: 10.1016/j.marpolbul.2018.03.038
Ren, T., Patel, M. K., and Blok, K. (2008). Steam cracking and methane to olefins: Energy use, CO2 emissions and production costs. Energy 33, 817–833. doi: 10.1016/j.energy.2008.01.002
Ren, Y., Shi, L., Bardow, A., Geyer, R., and Suh, S. (2020). Life-cycle environmental implications of China's ban on post-consumer plastics import. Resour. Conserv. Recycl. 156:104699. doi: 10.1016/j.resconrec.2020.104699
Revel, M., Châtel, A., and Mouneyrac, C. (2018). Micro(nano)plastics: A threat to human health? Curr. Opin. Environ. Sci. Health 1, 17–23. doi: 10.1016/j.coesh.2017.10.003
Rikhter, P., Dinc, I., Zhang, Y., Jiang, T., Miyashiro, B., Walsh, S., et al. (2022). Life Cycle Environmental Impacts of Plastics: A Review. Technical report.
Roebroek, C. T. J., Laufkötter, C., González-Fernández, D., and van Emmerik, T. (2022). The quest for the missing plastics: large uncertainties in river plastic export into the sea. Environ. Pollut. 312:119948. doi: 10.1016/j.envpol.2022.119948
Rosa, A. D. L., Blanco, I., Banatao, D. R., Pastine, S. J., Björklund, A., and Cicala, G. (2018). Innovative chemical process for recycling thermosets cured with recyclamines® by converting bio-epoxy composites in reusable thermoplastic—An LCA Study. Materials 11:353. doi: 10.3390/ma11030353
Ryberg, M. W., Hauschild, M. Z., Wang, F., Averous-Monnery, S., and Laurent, A. (2019). Global environmental losses of plastics across their value chains. Resour. Conserv. Recycl. 151:104459. doi: 10.1016/j.resconrec.2019.104459
Schmaltz, E., Melvin, E. C., Diana, Z., Gunady, E. F., Rittschof, D., Somarelli, J. A., et al. (2020). Plastic pollution solutions: emerging technologies to prevent and collect marine plastic pollution. Environ. Int. 144:106067. doi: 10.1016/j.envint.2020.106067
Schwarz, A. E., Ligthart, T. N., Bizarro, D. G., De Wild, P., Vreugdenhil, B., and van Harmelen, T. (2021). Plastic recycling in a circular economy; determining environmental performance through an LCA matrix model approach. Waste Manag. 121, 331–342. doi: 10.1016/j.wasman.2020.12.020
Schyns, Z. O., and Shaver, M. P. (2021). Mechanical recycling of packaging plastics: a review. Macromol. Rapid Commun. 42:2000415. doi: 10.1002/marc.202000415
Scientists Coalition for an Effective Plastics Treaty (2023). Policy Brief: Role of chemicals and polymers of concern in the global plastics treaty. Working paper.
Seif, S, Provencher, J. F, Avery-Gomm, S, Daoust, P-Y, Mallory, M. L, and Smith, P. A. (2018). Plastic and non-plastic debris ingestion in three gull species feeding in an urban landfill environment. Arch. Environ. Contam. Toxicol. 74, 349–360. doi: 10.1007/s00244-017-0492-8
Senathirajah, K., Kemp, A., Saaristo, M., Ishizuka, S., and Palanisami, T. (2022). Polymer prioritization framework: a novel multi-criteria framework for source mapping and characterizing the environmental risk of plastic polymers. J. Hazard. Mater. 429:128330. doi: 10.1016/j.jhazmat.2022.128330
Shen, L., Worrell, E., and Patel, M. (2010). Present and future development in plastics from biomass. Biofuels Bioprod. Bioref. 4, 25–40. doi: 10.1002/bbb.189
Shen, M., Zhang, Y., Zhu, Y., Song, B., Zeng, G., Hu, D., et al. (2019). Recent advances in toxicological research of nanoplastics in the environment: a review. Environ. Pollut. 252, 511–521. doi: 10.1016/j.envpol.2019.05.102
Sheraz, M., Kim, J., and Kim, J. (2023). Nano/microplastics in indoor air: A critical review of synthesis routes for toxicity testing and preventative measure strategies. Proc. Saf. Environ. Prot 180, 274–304. doi: 10.1016/j.psep.2023.10.002
Stegmann, P., Daioglou, V., Londo, M., van Vuuren, D. P., and Junginger, M. (2022). Plastic futures and their CO2 emissions. Nature 612, 272–276. doi: 10.1038/s41586-022-05422-5
Stelfox, M., Hudgins, J., and Sweet, M. (2016). A review of ghost gear entanglement amongst marine mammals, reptiles and elasmobranchs. Mar. Pollut. Bull. 111, 6–17. doi: 10.1016/j.marpolbul.2016.06.034
Stellner, L., Kalousdian, A., Kätelhön, A., et al. (2023). Methodology of cm.chemicals Database. Version 2.00. Cologne, Germany: Carbon Minds GmbH.
Strempel, S., Scheringer, M., Ng, C. A., and Hungerbühler, K. (2012). Screening for PBT Chemicals among the “Existing” and “New” Chemicals of the EU. Environ. Sci. Technol. 46, 5680–5687. doi: 10.1021/es3002713
Sun, W. (2023). The devastating health consequences of the ohio derailment: a closer look at the effects of vinyl chloride spill. Int. J. Environ. Res. Public Health 20:5032. doi: 10.3390/ijerph20065032
Teuten, E. L., Saquing, J. M., Knappe, D. R. U., Barlaz, M. A., Jonsson, S., Björn, A., et al. (2009). Transport and release of chemicals from plastics to the environment and to wildlife. Philos Trans. R Soc. B Biol. Sci. 364, 2027–2045. doi: 10.1098/rstb.2008.0284
Thompson, R. C., Moore, C. J., vom Saal, F. S., and Swan, S. H. (2009). Plastics, the environment and human health: current consensus and future trends. Philos Trans. R Soc. B Biol. Sci. 364, 2153–2166. doi: 10.1098/rstb.2009.0053
Tibbetts, J., Krause, S., Lynch, I., and Sambrook Smith, G. H. (2018). Abundance, distribution, and drivers of microplastic contamination in urban river environments. Water 10:1597. doi: 10.3390/w10111597
Trasande, L., Krithivasan, R., Park, K., et al. (2024). Chemicals used in plastic materials: an estimate of the attributable disease burden and costs in the United States. J. Endocr. Soc. 8:bvad163. doi: 10.1210/jendso/bvad163
Turner, A., and Filella, M. (2021). Hazardous metal additives in plastics and their environmental impacts. Environ. Int. 156:106622. doi: 10.1016/j.envint.2021.106622
Udovicki, B., Andjelkovic, M., Cirkovic-Velickovic, T., and Rajkovic, A. (2022). Microplastics in food: scoping review on health effects, occurrence, and human exposure. Int. J. Food Contam. 9:7. doi: 10.1186/s40550-022-00093-6
Ujaczki, É., Stark-Rogel, V., Olbrich, M., Fuetsch, M., and Backmann, J. (2022). Experiences and consequences of phasing out substances of concern in a multinational healthcare company. Environ. Sci. Eur. 34:101. doi: 10.1186/s12302-022-00678-0
UNEP (2016). Marine Plastic Debris and Microplastics: Global Lessons and Research to Inspire Action and Guide Policy Change.
UNEP (2020). Tackling Plastic Pollution: Legislative Guide for the Regulation of Single-Use Plastic Products.
UNEP (2023a). Revised draft text of the international legally binding instrument on plastic pollution, including in the marine environment [Advance]. 2023 UNEP/PP/INC.4/3.
UNEP (2023c). Turning off the Tap: How the world can end plastic pollution and create a circular economy.
US EPA (2015b). Initial List of Hazardous Air Pollutants with Modifications. Available online at: https://www.epa.gov/haps/initial-list-hazardous-air-pollutants-modifications (accessed April 18, 2024).
Valavanidis, A., Iliopoulos, N., Gotsis, G., and Fiotakis, K. (2008). Persistent free radicals, heavy metals and PAHs generated in particulate soot emissions and residue ash from controlled combustion of common types of plastic. J. Hazard Mater. 156, 277–284. doi: 10.1016/j.jhazmat.2007.12.019
Valente, T., Scacco, U., and Matiddi, M. (2020). Macro-litter ingestion in deep-water habitats: is an underestimation occurring? Environ. Res. 186:109556. doi: 10.1016/j.envres.2020.109556
Wagner, M., Monclús, L., Arp, H. P. H., et al. (2024). State of the science on plastic chemicals - Identifying and addressing chemicals and polymers of concern. New York: Zenodo.
Walker, S., and Rothman, R. (2020). Life cycle assessment of bio-based and fossil-based plastic: a review. J. Clean Prod. 261:121158. doi: 10.1016/j.jclepro.2020.121158
Wang, C., Tang, J., Yu, H., Wang, Y., Li, H., Xu, S., et al. (2022). Microplastic pollution in the soil environment: Characteristics, influencing factors, and risks. Sustainability 14:13405. doi: 10.3390/su142013405
Wang, L.-C., Lee, W.-J., Lee, W.-S., Chang-Chien, G.-P., and Tsai, P.-J. (2003). Effect of chlorine content in feeding wastes of incineration on the emission of polychlorinated dibenzo-p-dioxins/dibenzofurans. Sci. Total Environ. 302, 185–198. doi: 10.1016/S0048-9697(02)00306-6
Wang, M., Rücklin, M., Poelmann, R. E., de Mooij, C. L., Fokkema, M., Lamers, G. E. M., et al. (2023). Nanoplastics causes extensive congenital malformations during embryonic development by passively targeting neural crest cells. Environ. Int. 173:107865. doi: 10.1016/j.envint.2023.107865
WEF (2016). The New Plastics Economy: Rethinking the Future of Plastics. World Economic Forum, Ellen MacArthur Foundation and McKinsey & Company.
Wiesinger, H., Wang, Z., and Hellweg, S. (2021). Deep dive into plastic monomers, additives, and processing aids. Environ. Sci. Technol. 55, 9339–9351. doi: 10.1021/acs.est.1c00976
Williams, A. T., and Rangel-Buitrago, N. (2019). Marine litter: solutions for a major environmental problem. J. Coast Res. 35, 648–663. doi: 10.2112/JCOASTRES-D-18-00096.1
Willis, K., Maureaud, C., Wilcox, C., and Hardesty, B. D. (2018). How successful are waste abatement campaigns and government policies at reducing plastic waste into the marine environment? Mar. Policy 96, 243–249. doi: 10.1016/j.marpol.2017.11.037
Windheim, J., Colombo, L., Battajni, N. C., Russo, L., Cagnotto, A., Diomede, L., et al. (2022). Micro- and nanoplastics' effects on protein folding and amyloidosis. Int. J. Mol. Sci. 23:10329. doi: 10.3390/ijms231810329
Winkler, J., Liu, P., Phong, K., Hinrichs, J. H., Ataii, N., Williams, K., et al. (2022). Bisphenol A replacement chemicals, BPF and BPS, induce protumorigenic changes in human mammary gland organoid morphology and proteome. Proc. Natl. Acad. Sci. 119:e2115308119. doi: 10.1073/pnas.2115308119
Woods, J. S., Verones, F., Jolliet, O., et al. (2021). A framework for the assessment of marine litter impacts in life cycle impact assessment. Ecol. Indic. 129:107918. doi: 10.1016/j.ecolind.2021.107918
Yan, Z., Liu, Y., Zhang, T., Zhang, F., Ren, H., and Zhang, Y. (2022). Analysis of microplastics in human feces reveals a correlation between fecal microplastics and inflammatory bowel disease Status. Environ. Sci. Technol. 56, 414–421. doi: 10.1021/acs.est.1c03924
Young, B., Hawkins, T. R., Chiquelin, C., Sun, P., Gracida-Alvarez, U. R., and Elgowainy, A. (2022). Environmental life cycle assessment of olefins and by-product hydrogen from steam cracking of natural gas liquids, naphtha, and gas oil. J. Clean Prod. 359:131884. doi: 10.1016/j.jclepro.2022.131884
Yuan, Z., Nag, R., and Cummins, E. (2022). Human health concerns regarding microplastics in the aquatic environment-from marine to food systems. Sci. Tot. Environ. 823:153730. doi: 10.1016/j.scitotenv.2022.153730
Zhang, K., Hamidian, A. H., Tubić, A., Zhang, Y., Fang, J. K., Wu, C., et al. (2021). Understanding plastic degradation and microplastic formation in the environment: a review. Environ. Pollut. 274:116554. doi: 10.1016/j.envpol.2021.116554
Zhang, M., Buekens, A., and Li, X. (2016). Brominated flame retardants and the formation of dioxins and furans in fires and combustion. J. Hazard Mater. 304, 26–39. doi: 10.1016/j.jhazmat.2015.10.014
Zheng, J., and Suh, S. (2019). Strategies to reduce the global carbon footprInt. of plastics. Nat. Clim. Change 9, 374–378. doi: 10.1038/s41558-019-0459-z
Zhu, X. (2021). The plastic cycle – an unknown branch of the carbon cycle. Front. Mar. Sci. 7:609243. doi: 10.3389/fmars.2020.609243
Zimmermann, L., Dombrowski, A., Völker, C., and Wagner, M. (2020). Are bioplastics and plant-based materials safer than conventional plastics? In vitro toxicity and chemical composition. Environ. Int. 145:106066. doi: 10.1016/j.envint.2020.106066
Keywords: life cycle assessment, polymers of concern, precautionary principle, regrettable substitution, plastic pollution
Citation: Suh S, Boulay A-M, Fantke P, Li D, Menon D, Meys R and Milà i Canals L (2024) Conceptual framework for identifying polymers of concern. Front. Sustain. 5:1399431. doi: 10.3389/frsus.2024.1399431
Received: 11 March 2024; Accepted: 16 April 2024;
Published: 23 April 2024.
Edited by:
Shabbir H. Gheewala, King Mongkut's University of Technology Thonburi, ThailandReviewed by:
Jorge Emmanuel, Silliman University, PhilippinesBethanie Carney Almroth, University of Gothenburg, Sweden
Copyright © 2024 Suh, Boulay, Fantke, Li, Menon, Meys and Milà i Canals. This is an open-access article distributed under the terms of the Creative Commons Attribution License (CC BY). The use, distribution or reproduction in other forums is permitted, provided the original author(s) and the copyright owner(s) are credited and that the original publication in this journal is cited, in accordance with accepted academic practice. No use, distribution or reproduction is permitted which does not comply with these terms.
*Correspondence: Sangwon Suh, suh@bren.ucsb.edu
†ORCID: Sangwon Suh orcid.org/0000-0001-8290-6276
Anne-Marie Boulay orcid.org/0000-0003-1518-7931
Peter Fantke orcid.org/0000-0001-7148-6982
Dingsheng Li orcid.org/0000-0002-8432-4023
Dilip Menon orcid.org/0009-0001-1578-0770
Raoul Meys orcid.org/0000-0002-0336-9714
Llorenç Milà i Canals orcid.org/0000-0001-9109-9736