- 1Instituto de Macromoléculas Professora Eloisa Mano, Centro de Tecnologia, Universidade Federal do Rio de Janeiro, Rio de Janeiro, Brazil
- 2Petróleo Brasileiro S.A., Centro de Pesquisa, Centro de Pesquisas e Desenvolvimento Leopoldo, Rio de Janeiro, Brazil
- 3Escola Politécnica/Programa de Engenharia Ambiental, Centro de Tecnologia, Universidade Federal do Rio de Janeiro, Rio de Janeiro, Brazil
Introduction: Acrylonitrile-butadiene-styrene copolymer (ABS) and high impact polystyrene (HIPS) are plastics typically found in waste electrical and electronic equipment (WEEE or e-waste). As such, recycling is a beneficial method for reintroducing polymeric materials from electrical and electronic equipment to the same production cycle. This study aimed to investigate mechanical recycling of ABS and HIPS from WEEE reprocessed six times, totaling 24 cycles.
Methods: Reprocessing was performed by extrusion and test specimens were obtained by injection. The technical aspects assessed before and after reprocessing were mechanical properties (tensile, flexural and impact strength), thermogravimetric analysis (TGA), Fourier-transform infrared spectroscopy (FTIR) and melt flow index (MFI), compared with those of reprocessed virgin polymeric materials. The environmental aspect was evaluated by monitoring energy consumption and solid waste generation during each reprocessing cycle.
Results: Average energy consumption per extrusion and injection cycle was 0.7 and 2.0 kW/kg, respectively. There was a 30% increase in MFI from the first to sixth reprocessing cycle for virgin HIPS (5.5 g/10 min in 200°C and 5 kg), the largest among the polymers. The values for virgin ABS (6.0 g/10 min in 200°C and 5 kg) and ABS and HIPS from WEE increased by 11–15% from the first to sixth cycle. Impact strength was affected from the second reprocessing cycle onwards for ABS e-waste (14% decrease) and in the fifth cycle (16% decrease) for HIPS e-waste.
Discussion: The thermal, tensile and flexural properties of the virgin and post-consumer materials remained almost unchanged as the number of reprocessing cycles increased, indicating that these materials have the potential and properties for reintroduction into the same production cycle.
1. Introduction
Technological development has resulted in considerable growth in the production of new electrical and electronic equipment (EEE), defined as equipment that depends on an electric current or electromagnetic field to work properly. Cell phones, televisions, desktop computers, laptops, and tablets are just some examples of everyday electronic devices.
This accelerated growth leads to increased generation of waste electrical and electronic equipment (WEEE or e-waste). Plastics are increasingly used in EEE, which has lowered production costs and resulted in lighter products that are more durable and easily molded when compared to other traditional materials (Tansel, 2017). EEE typically contain around 10 to 30% plastic (Wang and Xu, 2014). High impact polystyrene (HIPS) and acrylonitrile-butadiene-styrene copolymer (ABS) are the most representative polymers in this market (Dimitrakakis et al., 2009; Wäger and Hischier, 2015; Hirayama and Saron, 2018; Teixeira et al., 2021).
Recycling is an excellent option for a more environmentally correct destination for post-consumer plastics (Ragaert et al., 2017) and those from EEE. Recovering these plastics is vital and urgent from a human health, environmental, social and economic perspective considering, for example, saving space in landfills and energy in the extraction and processing of virgin materials, transport, expansion of social programs with digital inclusion and job creation in the supply chain of maintenance, and recycling activities (Rodrigues, 2007; Menikpura et al., 2014; Campolina et al., 2017; Teixeira et al., 2021).
Plastic waste is considered a resource capable of replacing virgin materials (Huysveld et al., 2019), given the minimum technical requirements for the same application; or to be used in the manufacture of a product with properties that differ from those of the original material. Both cases involve minimal use of new resources (Xavier et al., 2021).
In general, reprocessing leads to polymer degradation and, consequently, loss of properties (Ha and Kim, 2012; Scaffaro et al., 2012). Brennan et al. (2002), Bai et al. (2007), Karahaliou and Tarantili (2009), Beigbeder et al. (2013), and Hirayama and Saron (2015, 2018) confirmed that degradation occurred during ABS recycling due to a deterioration in properties. The same occurred with HIPS (Hirayama and Saron, 2015, 2018).
In the light of the above, this study aimed to investigate the mechanical reprocessing of ABS and HIPS from e-waste compared with the processing of virgin material to assess the possibility of their reintroduction or not to the same production cycle.
2. Experimental
2.1. Materials
Virgin HIPS (HIPS825) was supplied by Innova and virgin ABS (AF3560) by Formosa Chemicals & Fibre Corporation (TAIRILAC®). Both virgin polymers are indicated for the production of EEE. The ABS and HIPS from WEEE were provided by two different recyclers, both located in the State Rio de Janeiro state (RJ), Brazil, and obtained from different types of e-waste:
• ABS from the rigid tablet casings.
• HIPS from computer screen casings and keyboards, washing machines, telephones, and cell phones
Initially, the WEEE samples were visually characterized. The ABS tablet casings were black and subsequently ground (Figure 1). A total of eight colors were identified for HIPS e-waste (black, ivory, gray, blue, red, pink, green, and yellow), which was manually sorted by color, as shown in Figure 1. All the characterization and processing equipment is located at the Instituto de Macromoleculas Professora Eloisa Mano at the Federal University of Rio de Janeiro, Brazil.
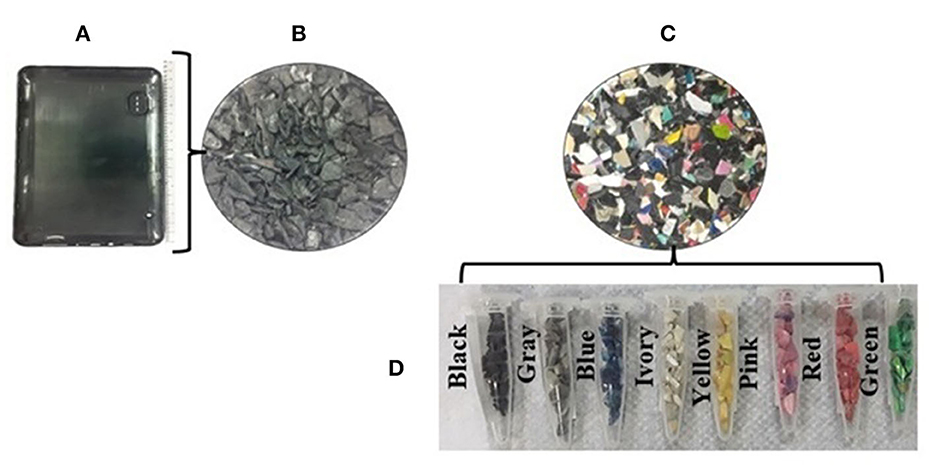
Figure 1. Polymers from WEEE: (A) ABS tablet casings; (B) ABS flakes; (C) HIPS flakes; (D) color sorted HIPS samples.
2.2. Characterization of the materials
The ABS from tablet casings was ground in a knife mill equipped with an approximately 9.0 mm mesh sieve, resulting in plastic pieces smaller than 2.0 mm, denominated ground flakes. All the ABS flakes were black.
HIPS was supplied already ground into different-colored flakes, with sizes ranging from 2 mm to larger than 5 mm. The flakes were manually separated by color into a 100 g sample to confirm that they were in fact HIPS and the percentage mass of each color was calculated. Each color was denominated as a subsample.
Next, ABS (virgin and post-consumer), virgin HIPS and the colored samples of HIPS e-waste were characterized in terms of their mechanical properties (tensile, flexural, and impact strength), thermogravimetric analysis (TGA), Fourier-transform infrared spectroscopy (FTIR), and melt flow index (MFI). Table 1 presents the codes and identification of the polymeric materials studied here.
2.2.1. Fourier-transform infrared spectroscopy
Fourier-transform infrared spectroscopy (FTIR) (Varian 3100 FT-IR Excalibur Series) was used to identify the functional groups in the polymers. Spectra were obtained using an attentuated total reflectance (ATR) accessory within a wavelength range of 4,000 to 600 cm−1.
2.2.2. Melt flow index
MFI values for the virgin and e-waste polymers were measured in accordance with ASTM standard D1238-13 (ASTM International, 2013), using 3 g of material analyzed at 200°C under a standard mass of 5.0 Kg in a Dynisco LMI 4003 melt flow indexer.
2.2.3. Thermal properties
The variation in the mass of the polymeric materials was analyzed by TGA (TA Instruments, Q500), using a temperature ramp of 10°C/min from 25 to 600 °C under a nitrogen atmosphere, with a flow rate of 20 mL/min. The samples weighed around 17 mg and were placed in an open ceramic container. Mass loss vs. temperature curves and their derivatives were analyzed by differential thermogravimetry (DTG).
Differential scanning calorimetry (DSC) (TA Instruments, Q1000) was performed to observe possible variations in glass transition temperature (Tg) and crystalline melting temperature (Tm). All the analyses were carried out under a nitrogen atmosphere. Two heating and one cooling cycle were conducted, both at a rate of 10°C/min between 25 and 250°C. The temperature readings from the second heating cycle onwards were considered, in order to erase the previous thermal history (first heating) since the materials analyzed were post-consumption.
2.3. Processing by (re)extrusion and injection molding to obtain test specimens
Both the virgin and e-waste polymers were extruded six times, that is, submitted to six reprocessing cycles. It is important to note that the HIPS waste samples, that is, all the different colored flakes, were extruded together to comply with the minimum amount needed for processing 6 times. The extruder used (Teck Tril DCT-20) has a production capacity of 10–20 kg/h and nine temperature zones, and the testing conditions were established based on recommendations in the technical specifications for the respective virgin polymers (Formosa Chemicals and Fibre Corporation, 2021; Innova, 2021), as shown in Table 2. The material was extruded in a continuous cylindrical filament, cooled in water at ambient temperature and then granulated. Approximately 1.0 kg of pellets were obtained per cycle for subsequent assessment of the properties of the reprocessed materials.
After extrustion, injection was carried out to obtain test speciments for the tensile, flexural, and impact strength tests, using an Arburg Allrounder injector with an 85 kg/h production capacity and five temperature zones. The injection profile used is presented in Table 2.
2.4. Environmental monitoring of reprocessing
Energy consumption and solid waste generation were quantified in reprocessing to obtain an environmental monitoring of ABS and HIPS recycling. Energy consumption during extrusion and injection (Figure 2) was measured using a Wattmeter equipped with a current and voltage sensor. The device makes the calculation internally and sends the instantaneous power data collected to a database file under a certain periodicity (Becker Junior et al., 2017). All solid residues generated in the process (extrusion residues, purges, and burrs) were quantified on a precision scale.
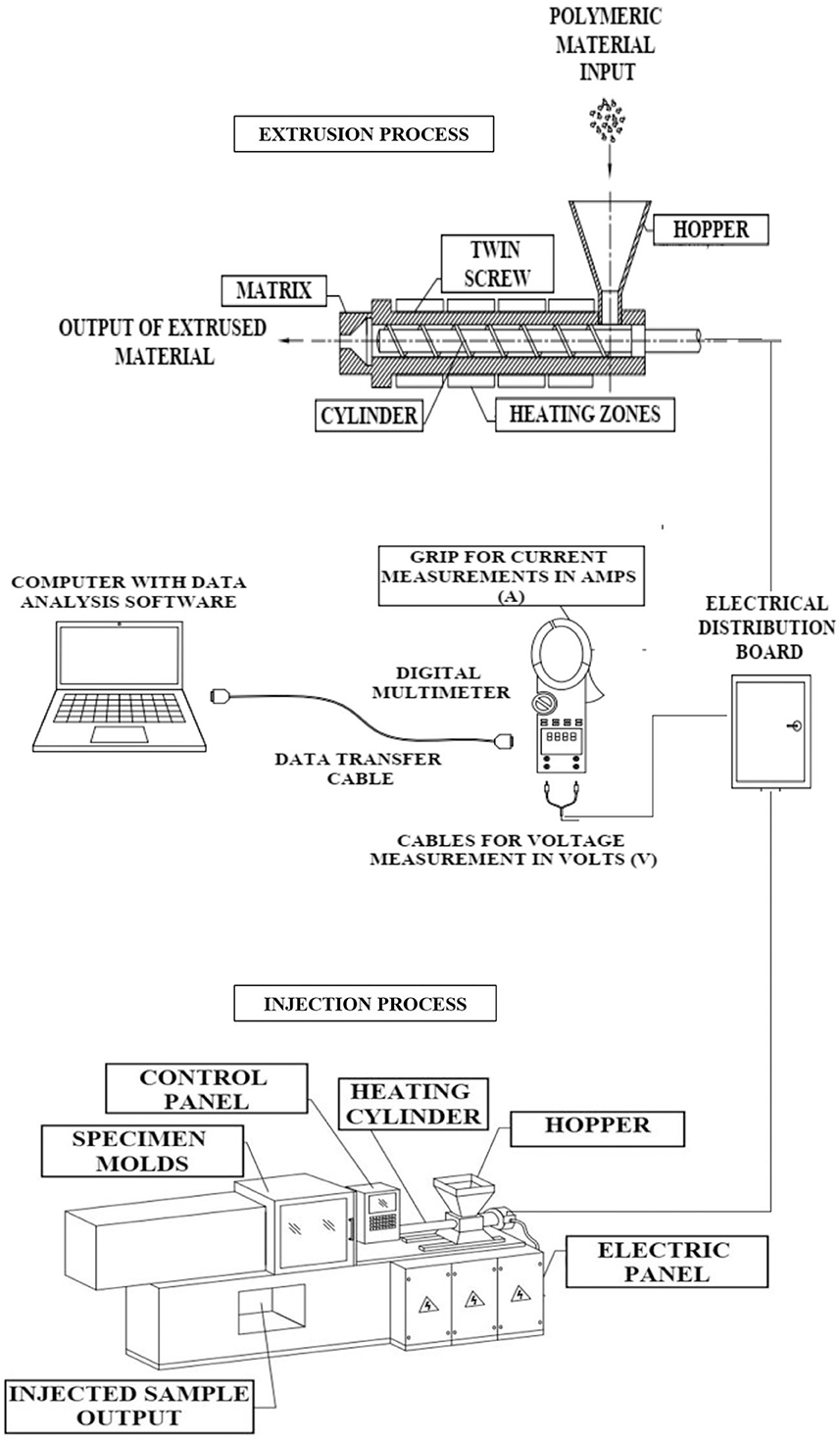
Figure 2. Scheme for measuring energy consumption in the process of extrusion and injection molding.
2.5. Assessment of the properties of the reprocessed polymeric materials
After reprocessing, the virgin and e-waste materials were analyzed by FTIR, TGA, DSC, and MFI following the same procedures used for the original raw materials. The mechanical properties (tensile, flexural, and impact strength) of the reprocessed materials were also evaluated, using injected test specimens of material.
Tensile testing (Emic DL3000) was performed in line with ASTM D638-10 (ASTM International, 2010b) on a universal testing machine, using type 1 test specimens (165 mm long, 12.6 mm wide, and 3.2 mm thick), a grip separation speed of 10 mm/min, maximum grip displacement of 90 cm and a 5 kN load cell.
Flexural strength testing (Emic DL3000) was tested according to ASTM D790-17 (ASTM International, 2017), with 52 cm between grips and test specimens measuring 127 mm long, 12 mm wide, and 3.2 mm thick. Six test specimens were used for each composition and testing was carried out on a universal testing machine.
IZOD impact strength testing (Resil Impactor Ceast) was conducted in accordance with ASTM D256-10 (ASTM International, 2010a), using a 2-joule pendulum and injected test specimens (63.5 × 12.7 × 3.0 mm) with a 2.54 mm notch and 0.25 mm notch radius.
3. Results and discussion
3.1. Quantification of the different HIPS and ABS waste
Visually, black, ivory, and gray HIPS e-waste predominated, which was confirmed via quantification and mass percentage assessment, as presented in Figure 3. Mass percent was calculated separately for HIPS and ABS. Colored HIPS % and black ABS % were calculated as a function of total HIPS and total ABS, respectively. These three colors accounted for 76% of the total mass of HIPS e-waste. Color sorting is a common procedure in the recycling industry for different colored products.
3.2. Characterization of the virgin and post-consumer polymers
The raw materials and different colored HIPS and ABS waste were characterized by TGA, DSC, FTIR and MFI.
3.2.1. Thermal analysis
Table 3 presents the TGA and DSC results for virgin and e-waste ABS and HIPS: the degradation onset temperature (Tonset), maximum degradation temperature (Tmax), and percentage of undegraded waste at 600°C obtained from the thermograms; and glass transition Temperature (Tg).
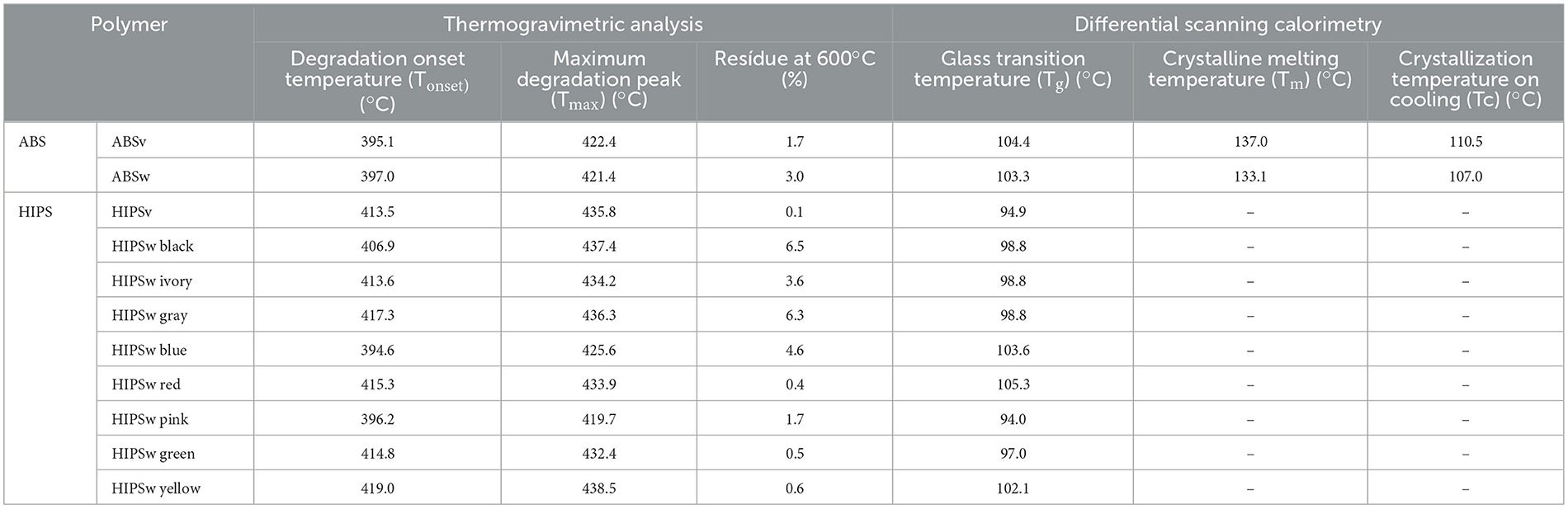
Table 3. Thermogravimetric analysis and differential scanning calorimetry of ABS and HIPS, virgin and from e-waste.
As expected, differences were observed in the thermal stability of virgin and post-consumer polymers. The variation in degradation temperatures for the different colored HIPSw was also expected. For HIPSw of the same grade, the different pigments or dyes interact differently with the polymer matrix, increasing or hampering their degradation under heating. According to Saron and Felisberti (2006), pigments and dyes have different chemical structures and interaction between them and the polymer alters its stability, potentially affecting its useful life and performance and even improving its thermal stability.
Differences in the onset and maximum degradation temperatures of ABSv and ABSw were very small. The value obtained for ABSw was 3.0%, higher than that of the virgin material but different from that of the virgin material with no pigment (ABSv), which was black.
The TGA results for the colored HIPSw samples indicated Tonset of 394 to 419°C and Tmax of 419 to 438°C, and 413 and 436°C for Tonset and Tmax of virgin HIPS, respectively.
The Tonset and Tmax values for ABSv and ABSw were 395 and 422°C and 397 and 421°C, respectively. All the degradation curves for HIPSw were similar, varying between 419.7 and 438.5°C, with 0.5–6.5% undegraded waste. It is important to underscore that, in addition to pigments, inert fillers are used in polymers to lower the cost of the final product and generally remain as residue in thermal degradation (Arráez et al., 2019).
DSC analyses were performed primarily to determine the glass transition temperature (Tg) of the virgin and post-consumer ABS and HIPS (Table 3). This property identifies the temperature at which the polymeric material changes from a glassy to a rubbery state. The mobility of molecular chains increases from Tg and the polymers become more elastic and flexible (Gregorova, 2013).
ABSv and ABSw values were similar, demonstrating the change from the glassy to rubbery state occurs at practically the same temperature for both the virgin and recycled polymers. Both polymers exhibited a slight endothermal peak at 137°C for ABSv and 133°C for ABSw, corresponding to the transition of acrylonitrile. Waheed et al. (2019) observed an endothermic peak close to 138°C for acrylonitrile in an ABS sample. A small exothermic crystallization peak was also observed at 110°C for ABSv and 107°C for ABSw during the cooling process.
The Tg of HIPSv was around 95°C and similar values are reported in the literature: 94°C by Vilaplana et al. (2007) and 95°C by Momanyi et al. (2019). The Tg of HIPSw varied from 94.0 to 103.6°C and it should be noted that all these samples, except for Pink HIPSw, obtained a higher Tg than that of HIPSv.
3.2.2. Fourier-transform infrared spectroscopy
FTIR was used to identify and characterize the functional groups present in the virgin and e-waste polymers. Table 4 presents the main FTIR transmittance bands in the ABSv and HIPSv copolymers. There was considerable similarity between the transmittance peaks of the two virgin polymers and the out-of-plane deformation bands at 3,025 cm−1 corresponding to C-H; the peaks at 1,600, 1,490, 700, and 760 cm−1 are attributed to C=C of the polystyrene ring and those at 966 and 911 cm−1 to aliphatic C=C, but more specifically to trans-1.4 and vinyl-1.2 corresponding to the polybutadiene phase. In addition to these bands, medium-intensity peaks were observed at 2,237 cm−1 only in the virgin ABS spectrum, corresponding to the nitrile group of the acrylonitrile. This peak is not present in HIPS. Similar results were reported for ABS in research by Kamelian et al. (2014).
In this analysis, there were similarities in the FTIR curves between e-waste and virgin ABS. A medium-intensity peak was observed at 2,240 cm−1, confirming the presence of acrylonitrile in ABSv and ABSw. There were also two peaks at around 1,723 and 1,770 cm−1 in the ABSw spectrum, corresponding to the carbonyl group (C=O), which may be related to the presence of an additive or possible degradation of post-consumer ABS (Tiganis et al., 2002), a material subject to wear during use and after disposal.
The FTIR results for the eight different colored HIPS e-waste samples showed that the main bands corresponding to polystyrene and polybutadiene are similar to those of virgin HIPS. However, all the HIPSw samples have small peaks at around 3,700 cm−1 that were not observed for HIPSv. Peaks at this wavelength are characteristic of hydroxyl group vibrations, possibly from a common additive in EEE. The hydroxyl peak is more evident in the black, ivory, gray, and blue HIPSw samples, which exhibited a larger amount of residue in TGA, that is, they likely contain more carbonate. Calcium carbonate (CaCO3) is a common additive in thermoplastics (Gorna et al., 2008). Thermal decomposition of CaCO3 occurs between 700 and 800°C (Karunadasa et al., 2019); as such, the additive is in a solid state at the final temperature used in TGA.
Blue HIPSw displayed peaks at 3,695 and 3,618 cm−1 corresponding to O-H stretching and at 1,435 and 874 cm−1 attributed to carbonate stretching. In addition to these bands, Silva et al. (2006) reported that bands at 1,106, 1,029, 1,010, and 913 cm−1 are characteristic of a blue pigment called azurite, whose molecular formula is Cu3(CO3)2(OH)2. For the black, ivory and red HIPSw samples, a transmittance peak was observed at 2,237 cm−1, corresponding to the nitrile group. However, unlike ABSv and ABSw, the intensity of the peaks on the HIPS e-waste curves is low and may be associated with the presence of dyes, pigments or ABS. The possible presence of nitrile could be from a HIPS/ABS blend, but this percentage could not be quantified. It is important to note that the HIPS was waste provided by a recycler that does not use techniques to identify the materials processed.
Silva et al. (2006) studied different pigments and dyes by FTIR and found that the Prussian Blue exhibited a band at around 2,083 cm−1 associated with nitrile. However, this band was not present in the spectra of the samples analyzed in the present study and as such, it was concluded that the nitrile peak (2,237 cm−1) may be attributed to the acrylonitrile in ABS.
3.2.3. Melt flow index
Figure 4 shows the MFI values of the virgin and ABS and HIPS e-waste. The melt flow index was measured to assess the reprocessing cycles. The MFI values obtained for ABSv and ABSw were 6.6 and 2.6 g/10 min. Scaffaro et al. (2012) recorded MFIs of 3.6 and 3.3 g/10 min for ABS from telephone waste and virgin polymeric material. ABSw appears to differ considerably from ABSv, both of which are used in EEE, demonstrating the diversity of this market. The virgin ABS polymers with MFI close to 2.6 g/10 min, such as ABS AF3560 (MFI = 6.0); ABS AG15A3 (MFI = 2.2); ABS AG15E3 (MFI = 2.1); ABS AG15E0 (MFI = 1.8), are used in different EEE applications, including calculators, telephones, and stereos, among others (Formosa Chemicals and Fibre Corporation, 2021).
The MFI of HIPS was 5.3 g/10 min, while the value recorded for HIPS e-waste, in which all the HIPS samples were combined, was 6.5 g/10 min (Figure 4). In general, the MFI of the HIPS e-waste samples showed greater homogeneity, despite their different origins. The different HIPS grades used in EEE include HIPS825 (MFI = 5.5), HIPS RC600 (MFI = 6.0), and HIPS RT441M (MFI = 6.5; Innova, 2021).
3.3. Processing the polymeric materials
ABSv, ABSw flakes smaller than 2.0 mm and HIPSv were fed into the extruder and subjected to six reprocessing cycles. The materials were then injected to obtain test specimens for the mechanical testing. The reprocessing of both virgin ABS and ABSw took approximately 20 min for 1 kg of each material; however, there were difficulties in feeding the post-consumer HIPS into the extruder. After characterization, the different colored e HIPS e-waste flakes were extruded together as received from the recycler, becoming a single material after extrustion, referred to in this study as HIPSw. The reason for extruding all the HIPS colors together was to have sufficient material to be reprocessed six times and save 1 kg from each cycle for the subsequent tests.
There was a blockage in the hopper during the first HIPSw extrusion cycle due to the size of the flakes (>5 mm). Larger flakes required greater force to rotate the screw. This lengthened the extrusion process because small amounts of the material had to be fed into the extruder manually, doubling the extrusion time to around 43 min per kilogram for the first cycle. Thus, it was concluded that for the extruder used in this study, flakes had to be smaller than 5 mm. Residence time for HIPS inside the extruder in this first cycle was longer than that of the other cycles.
It should also be noted that the laboratory-scale extruder used here is smaller than those found in recycling plants, meaning that limited flake size may not be an issue for recyclers. There were no feeding difficulties in HIPS reprocessing from the second extrusion cycle onwards, when 1 kg was processed in approximately 20 min, since in this phase the material was in pellet form.
The virgin HIPS pellets became yellower as the number of reprocessing cycles increased, particularly from the second cycle onwards (Figure 5). The color change may be a sign of polymer degradation (Luna et al., 2019).
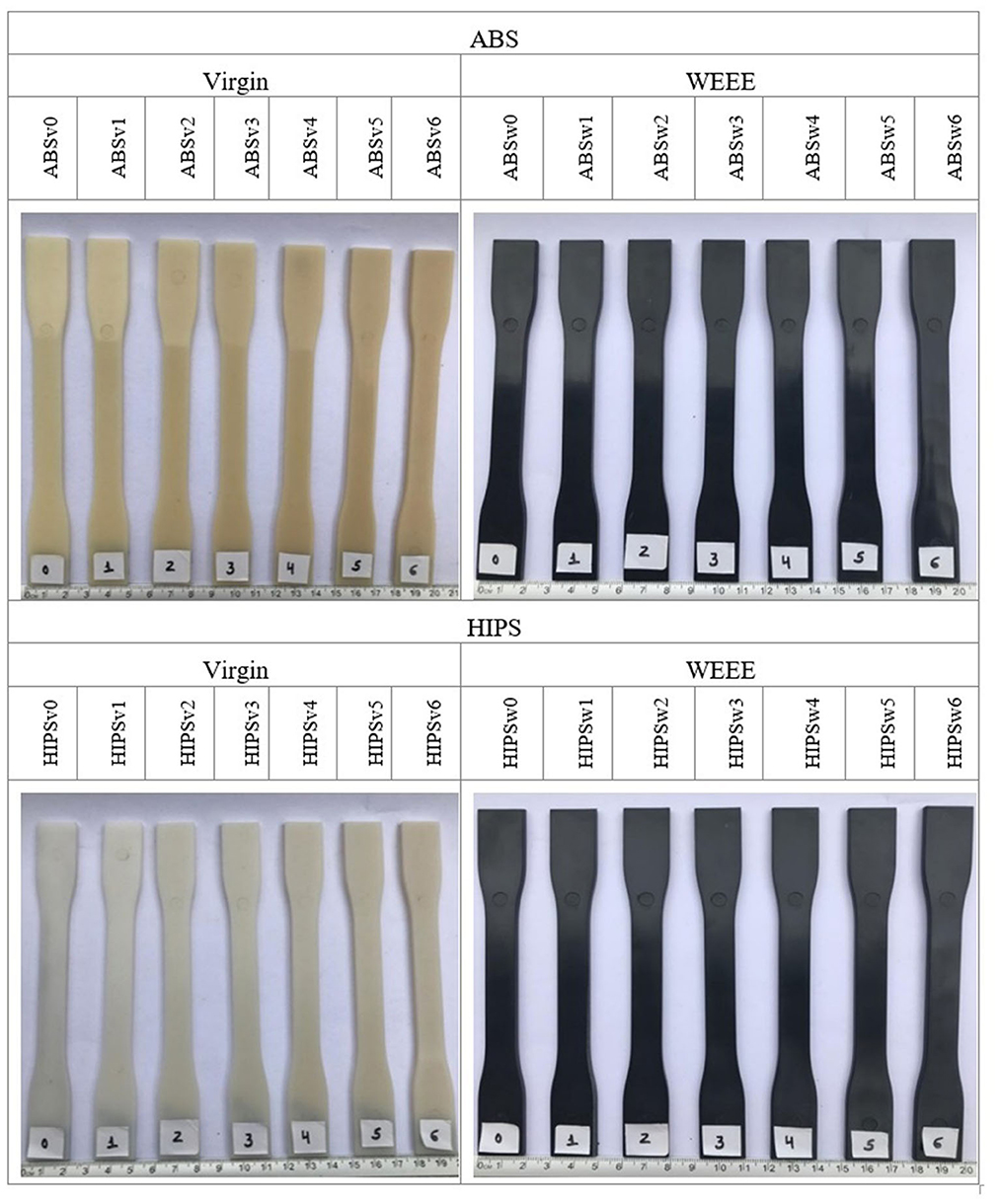
Figure 5. Test specimens for tensile testing obtained by injection of ABS and HIPS, virgin and from e-waste, reprocessed six times.
No yellowing was observed in the reprocessed ABSw and HIPSw samples (Figure 5) since both of these polymers were black. Reprocessed HIPSw remained an opaque black through all six extrusions, becoming slightly brighter after injection of the pellets, whereas ABS waste pellets were considerably brighter than the original material from the second cycle onwards. This greater bightness was also observed in the virgin ABS pellet, which naturally exhibited this characteristic before processing.
3.4. Environmental monitoring of the reprocessing cycles
Processing of the virgin and e-waste polymers was monitored by measuring electric energy consumption and the solid waste generated during the cycles. Table 5 shows the electric energy used during extrusion and injection, with around 0.7 kWh/kg consumed in each extrusion cycle for ABSv, ABSw and HIPSv, corresponding to 2.52 MJ/kg. This differs from the first HIPS e-waste extrusion cycle, which required more energy (5.47 MJ/kg). This is because of the feeding difficulties in this cycle due to flake size (>5 mm), with the resulting blockage doubling the molding time in relation to the remaining cycles, thereby increasing energy consumption.
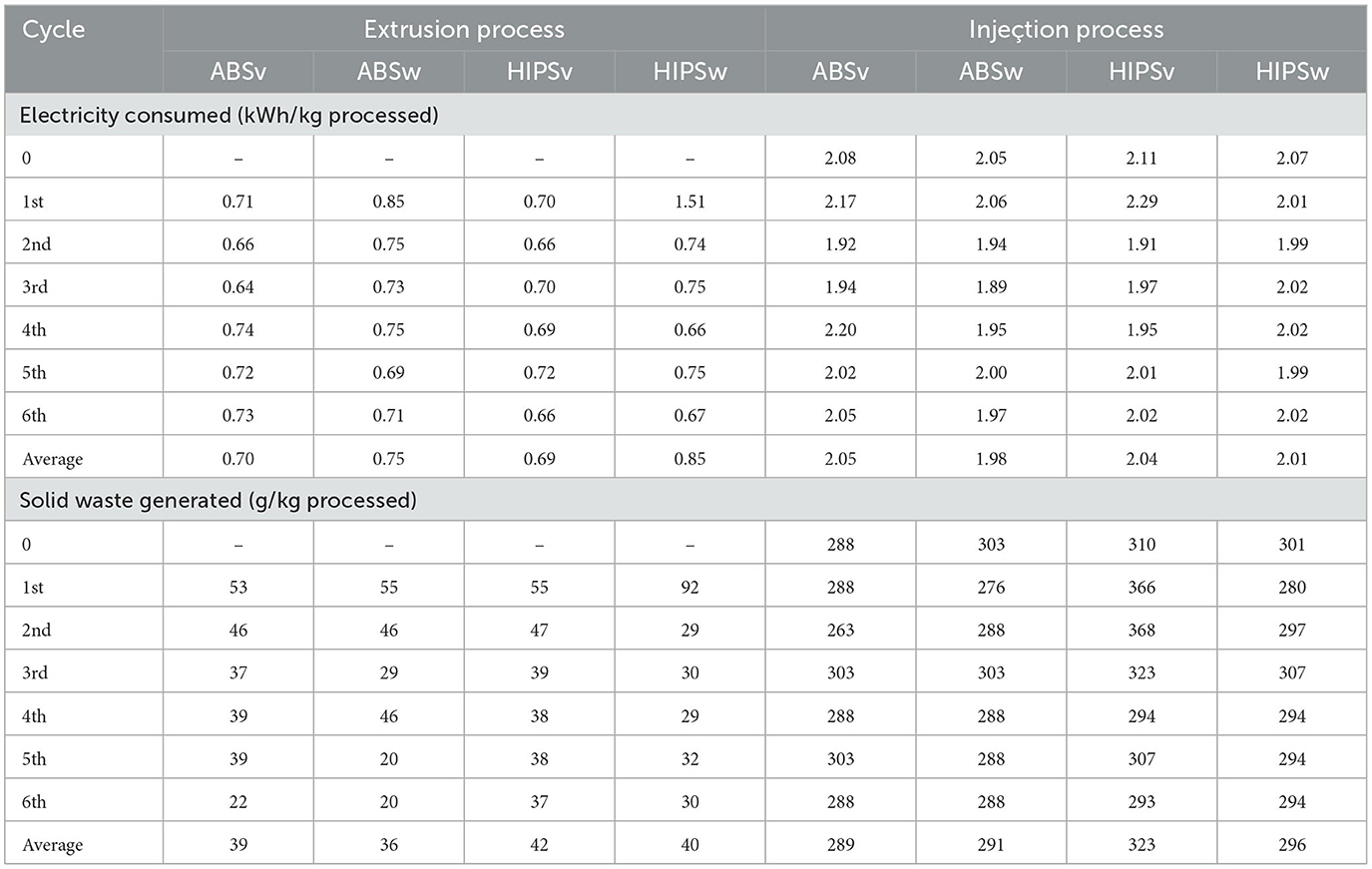
Table 5. Quantification of electric energy consumption and solid waste generation during the reprocessing of ABS and HIPS.
In a life cycle inventory (LCI), Campolina et al. (2017) found that extrusion of recycled ABS and HIPS consumed 6.76 and 7.03 MJ/kg, respectively. As shown in Table 5, injection consumed about 2.0 kWh/kg (7.0 MJ/kg) of energy, more than that used in extrusion, since the injector is more powerful (37.5 kW) than the extruder (5.5 kW). Becker Junior et al. (2017) reported the average energy consumption of eight injectors used to process polymeric materials such as polypropylene (PP) and ABS was 3.08 kWh/kg, regardless of the type of polymer. According to the authors, consumption varied from 1.25 to 5.38 kWh/kg depending on the production capacity, process settings, and mold types of the injector.
Table 5 also shows the solid waste generated during the six reprocessing cycles for each polymeric material. The injection process generated the most residue (average of 289 and 291 g/kg processed for ABSv and ABSw, respectively), while extrusion produced an average of 39 (ABSv) and 36 g/kg (ABSw). Similarly, a larger amount of waste was generated during HIPS injection (323 g/kg for HIPSv and 296 g/kg for HIPSw) than extrusion (42 g/kg for HIPSv and 40 g/kg for HIPSw). The waste produced in injection molding corresponds to runner and sprue generated in each reprocessing cycle. These results confirm waste generation in each injection or extrusion cycle and that this residue can be ground and reprocessed, remaining within the circular economy.
3.5. Assessment of properties of the reprocessed materials
3.5.1. Melt flow index
Table 6 shows the MFI values of ABSV and ABSW after each extrusion cycle. The MFI was also analyzed after injection and increased for both polymers with each successive cycle. Comparison of MFI values between cycles showed a very small percentage variation.
However, comparison between the first and sixth ABSv extrusion and extrusion + injection cycles showed an 11% increase in MFI, indicating that the virgin polymer was affected by reprocessing, likely due to the resulting degradation. There were no significant variations in MFI values between the extrusion + injection and extrusion processes considering standard deviations of 0.2 g/10 min per cycle.
Similar behavior was observed for ABSw, with a small variation (approximately 4%) across the six cycles. However, there was a 15% increase from the first to sixth extrusion cycle and approximately 10% for extrusion + injection (Table 6). Analysis of the standard deviations (0.2 g/10 min) after each cycle for extrusion and extrusion + injection also showed no variations, demonstrating that despite undergoing two types of processing, the MFI of ABSw shows that processing has little effect on the melt flow index ty of the material.
Comparison between the first and sixth HIPSv processing cycles showed an increase of approximately 30% for extrusion (Table 6) greater than that observed for the other polymers. The MFI of HIPSv submitted to extrusion + injection increased by 12.9% from the second to the third cycle, 8.6% from the third to the fourth, and 5.3% from the fifth to the sixth, reaching 27% from the first to the sixth cycle (Table 6).
Small MFI variations were observed for HIPSw, namely a 4% increase from the first to the second extrusion, 5% from the fifth to the sixth and 12% from the first to the sixth, and 6% for extrusion +injection (Table 6). An increase in MFI was also reported in other studies.
Comparison of MFI values for HIPSw extrusion + injection with those for extrusion revealed increases of 19, 13, 10, and 14% for the first, fourth, fifth and sixth cycles, respectively.
Santana and Manrich (2002) studied HIPS submitted to up to five reprocessing cycles and recorded a 12% rise in MFI in the first cycle, while the index remained constant at up to 3% after 5 cycles. According to the authors, this increase was the result of consecutive grinding and injection molding.
3.5.2. Thermogravimetric analysis
TGA curves and derivatives for virgin ABS and ABS e-waste reprocessed up to 6 times showed a well-defined decomposition stage. The results obtained for the initial decomposition temperatures (Tonset) of virgin ABS and WEEE reprocessed up to 6 times showed very similar decomposition temperatures, with 378 to 384°C for reprocessed ABSv and 381 to 386° for reprocessed ABSw. The Tonset values for ABSv were ABSv0 = 382°C; ABSv1 = 382°C; ABSv2 = 379°C; ABSv3 = 381°C; ABSv4 = 381°C; ABSv5 = 378°C; ABSv6 = 384°C, and for ABSw: ABSw0 = 384°C; ABSw1 = 386°C; ABSw2 = 382°C; ABSw3 = 382°C; ABSw4 = 381°C; ABSw5 = 381°C; and ABSw6 = 382°C.
Maximum degradation temperatures (Tmax) for reprocessed ABSv and ABSw ranged from 402 to 410°C and 410 to 416°C, respectively, with the following values obtained for ABSv: ABSv0 = 409°C; ABSv1 = 409°C; ABSv2 = 407°C; ABSv3 = 408°C; ABSv4 = 408°C; ABSv5 = 402°C; and ABSv6 = 410°C, and for ABSw: ABSw0 = 415°C; ABSw1 = 417°C; ABSw2 = 412°C; ABSw3 = 412°C; ABSw4 = 411°C; ABSw5 = 411°C; and ABSw6 = 413°C.
The mass percentage of residue for the materials reprocessed at 600°C was 1% for all the ABSv samples and 4% for reprocessed ABSw materials. The higher value obtained for ABSw is likely due to the presence of inorganic material in its composition, such as pigments responsible for its black color.
TGA curves and derivatives for HIPSv and HIPSw reprocessed up to 6 times also indicated a decomposition stage. The Tonset of reprocessed HIPSv and HIPSw was 401–402°C and 395–396°C in all cycles, respectively. The maximum degradation temperatures for both HIPSv and HIPSw were 424°C-425°C. The mass percentage of residue at 600°C for HIPSv was 0.1–0.3% and around 1–3% for HIPSw. These findings indicate that both the virgin and WEEE polymeric materials showed good thermal stability, which remained practically unchanged even after six processing cycles.
3.5.3. Fourier-transform infrared spectroscopy
The FTIR results for ABSv, ABSw, HIPSv, and HIPS no reprocessed and reprocessed six times are shown in Figures 6A, B, respectively. The FTIR results for ABSv reprocessed six times (Figure 6A) demonstrated that the main bands corresponding to the virgin polymer remained unchanged, that is, there was no decline and no new bands emerged with successive cycles. Peaks corresponding to nitrile (Figure 6C) and butadiene (Figure 6D) in the FTIR curves for ABS e-waste are slightly smaller than those of ABSw0 and more distinct for ABSw6. Figures 6C, D show the height of the peaks, with the most distinct regions of the nitrile group (2,237 cm−1) and trans-1.4 and vinyl-1.2 corresponding to the polybutadiene phase (966 and 911 cm−1).
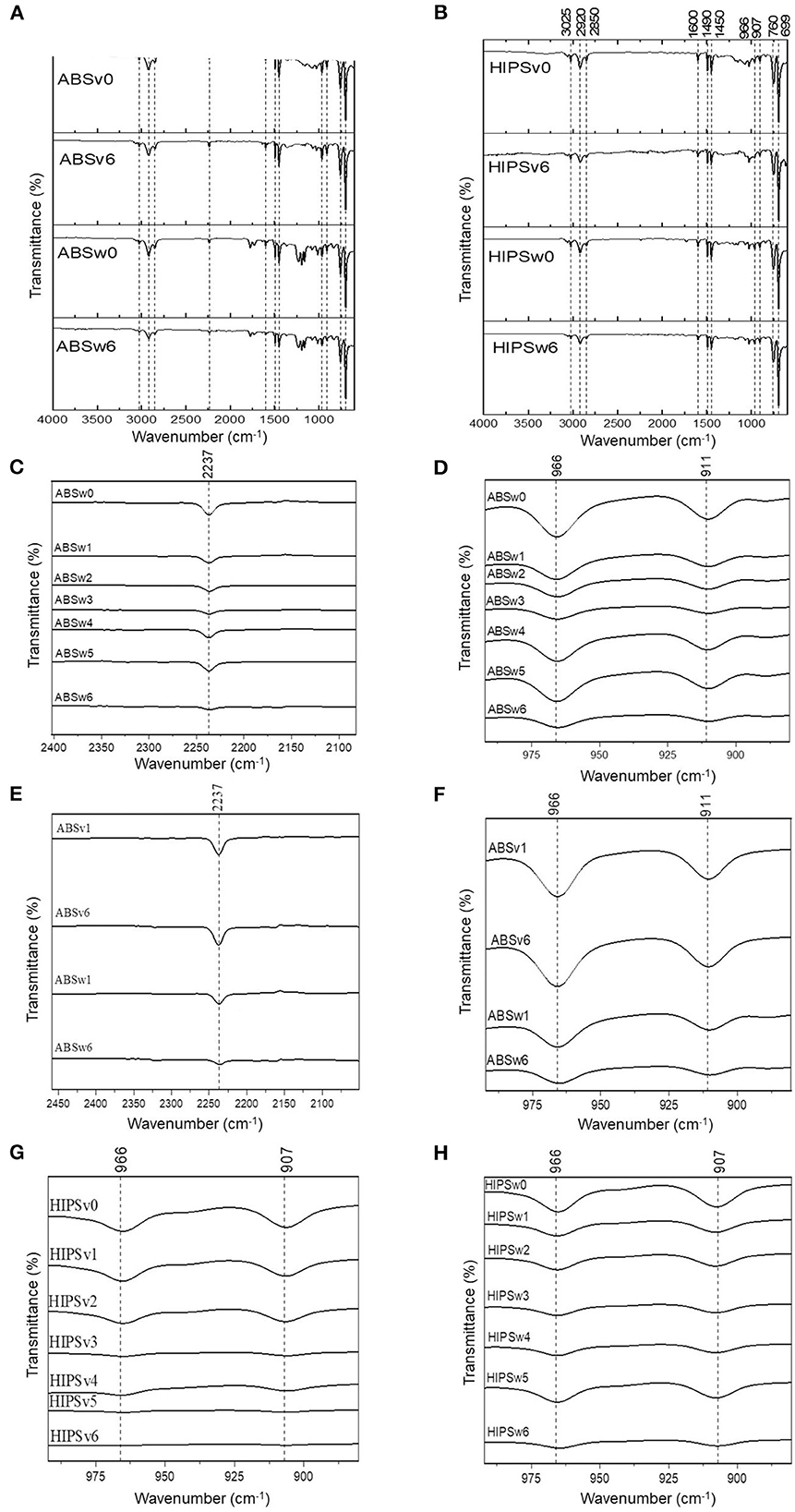
Figure 6. FTIR spectra for: (A) virgin and e-waste ABS no reprocessed and reprocessed six times; (B) virgin and e-waste HIPS no reprocessed and reprocessed six times; (C) ABS e-waste reprocessed six times in the nitrile regions; (D) ABS e-waste in the first and sixth reprocessing cycles for the butadiene regions; (E) Comparative FTIR spectra of virgin and e-waste ABS from the first and sixth cycles in the nitrile group region; (F) Comparative FTIR spectra of virgin and e-waste ABS from the first and sixth cycles in the butadiene group region; (G) regions corresponding to the butadiene group of reprocessed HIPSv; (H) regions corresponding to the butadiene group of reprocessed HIPSw.
Comparison of the first reprocessing cycle for ABSw and ABSv showed a slight decline in peaks corresponding to nitrile and butadiene. However, the decline is more evident in the sixth cycle, indicated by the peaks of the butadiene group and nitrile presented in Figures 6E, F, suggesting possible degradation of the butadiene phase. According to Ramesh et al. (2014), the microstructure of the polymer facilitates thermal degradation. The tertiary carbons in the polybutadiene phase of ABS are responsible for hydrogen abstraction by oxygen, and as such, thermal energy will activate hydrogen abstraction by the oxidation reaction to initiate and accelerate degradation.
Klein (2009) studied the effect of thermomechanical and photooxidative degradation of ABS and found that the reduction of C=C bonds in the elastomeric phase suggests possible degradation of the polymer, whose bands related to out-of-plane angular vibration are more sensitive than in-plane angular absorption of C=C bonds. Other authors (Luna et al., 2019) also reported that rubber degradation is associated with molecular scission, which results in smaller chains and a larger number of chain terminals and/or cross-linking, forming a highly bonded network.
For ABSw, degradation of the polybutadiene phase is related to molecular scission and not the generation of a network structure. This is corroborated by MFI analysis, with ABSw6 (MFI = 3.0 g/10 min) being more fluid than ABSw0 (MFI = 2.6 g/10 min) and therefore exhibiting a lower molar mass in the sixth cycle. Wang et al. (2015) found that the chemical structure of the styrene-acrylonitrile (SAN) phase of ABS remained almost unchanged after aging, which they attribute to the fact that thermooxidative aging occurs in the polybutadiene phase, or that ABS aging is due to the influence of physical aging on the SAN phase and oxidation of the polybutadiene phase.
The HIPSv spectra demonstrated a decrease in the peaks of the butadiene group (966 and 907 cm−1) from the third reprocessing cycle onwards. Figure 6G shows that the trans-1.4 and vinyl-1.2 peaks corresponding to the butadiene group only declined in cycle six.
Comparison between the first and sixth cycles for virgin HIPS and HIPS e-waste (Figure 6H) demonstrates that the virgin polymer tends to degrade more with reprocessing in the region of the butadiene group. This result can be analyzed in conjunction the MFI findings for HIPSv, whereby there was a greater increase in MFI in the first cycle, which may be a characteristic of decreased molar mass due to degradation.
3.5.4. Mechanical properties
The mechanical properties assessed in the reprocessed virgin and ABS and HIPS e-waste polymers were tensile, flexural, and impact strength.
3.5.4.1. Tensile strength
The stress vs. strain curves for virgin ABS and HIPS and reprocessed WEEE were typical of plastics and similar in shape. Both ABS and HIPS show linear behavior at the beginning of the curve, where there is a modulus of elasticity or Young's modulus (ε). As the polymer begins to experience strain, it transitions from elastic to plastic deformation at a point of maximum stress known as the yield point (θr), where yield point elongation (εr) can also be obtained. Strain continues until rupture (θy), when the curve slopes downward and elongation at break (εy) can be obtained. Table 7 presents the results for Young's modulus, stress and strain at Yield and break of virgin and ABS e-waste and virgin and HIPS e-waste.
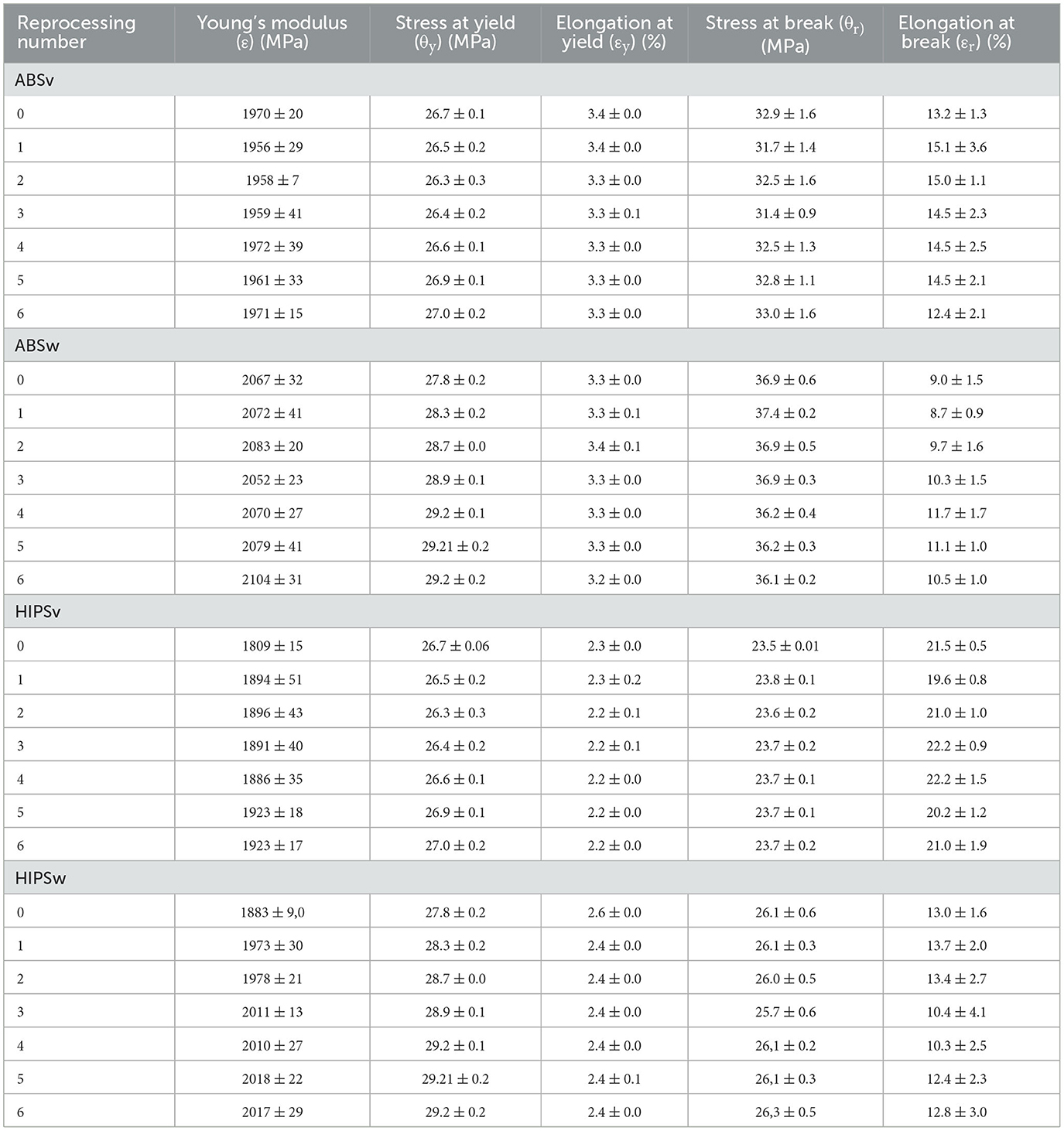
Table 7. Young's modulus, stress, and strain at yield and break of virgin and e-waste ABS and virgin and e-waste HIPS reprocessed six times.
For ABSv and ABSw, the yield point occurs at around 43 MPa, when strain is approximately 3%. The values obtained for the reprocessed materials were practically the same, indicating that the tensile properties of both polymers were almost unchanged after the six processing cycles. These findings corroborate those reported in the literature (Bai et al., 2007; Karahaliou and Tarantili, 2009; Beigbeder et al., 2013).
For ABSv and ABSw, the yield point occurs at around 43 MPa, when strain is approximately 3%. The values obtained for the reprocessed materials were practically the same, indicating that the tensile properties of both polymers were almost unchanged after the six processing cycles. These findings corroborate those reported in the literature (Bai et al., 2007; Karahaliou and Tarantili, 2009; Beigbeder et al., 2013).
The Young's modulus, elongation at break and rupture point of ABSw and ABSv differed considerably, corroborating the different grades of the materials. ABSw exhibited a Young's modulus greater than 2,050 MPa, rupture point of about 36 MPa and around 10% elongation at break. Elongation at break of ABSw is 42% lower than that of its virgin counterpart (ABSv), indicating that ABSw is a harder material and contains fillers, while ABSv has a higher modulus and is less tough and ductile. Toughness, which is related to impact strength, is lower in the e-waste than the virgin material, and was obtained from the area of the stress vs. strain curve. This area is smaller for ABSw than ABSv, indicating that the former is not as tough.
According to Beigbeder et al. (2013), degradation of the rubbery butadiene phase and the loss of small molecules such as monomers, heat stabilizers, functional molecules etc., are possible explanations for the inferior elongation at break results obtained for the recycled polymeric materials. However, in the present study, elongation at break for materials recycled one to six times was not inferior, but remained almost unchanged. Across 10 reprocessing cycles, Pérez et al. (2010) found that the Young's modulus and tensile strength of ABS increased slightly with the number of cycles, which they attributed to the SAN phase that is not affected.
Strain at the yield point is similar for HIPSv and HIPSw (Table 7) after each reprocessing cycle, at around 2%. Additionally, Young's modulus, the yield point and elongation at break remained almost unchanged across the HIPS reprocessing cycles. However, HIPSw differed from HIPSv in terms of the elastic modulus, rupture point and elongation at break, corroborating the different grades of the two materials. HIPSw obtained a Young's modulus greater than 2,000 MPa, rupture point of approximately 29 MPa and 13% elongation at break, likely due to the presence of fillers, indicating that this material is harder and not as tough.
3.5.4.2. Flexural strength
Flexural strength was analyzed for virgin and ABS and HIPS e-waste polymers submitted to six reprocessing cycles yielding the following data: flexural strength, elastic modulus and maximum flexural stress. Maximum flexural stress is the stress applied to the center of the test specimen needed to deform it.
For ABSv, elastic modulus and maximum flexural stress were almost unchanged across the six reprocessing cycles at around 2,500 and 70 MPa, respectively, as shown in Table 8. However, for ABSv, maximum flexural stress declined by approximately 6% from the third reprocessing cycle onwards, indicating that less force was needed from this point for deformation to occur under flexion.
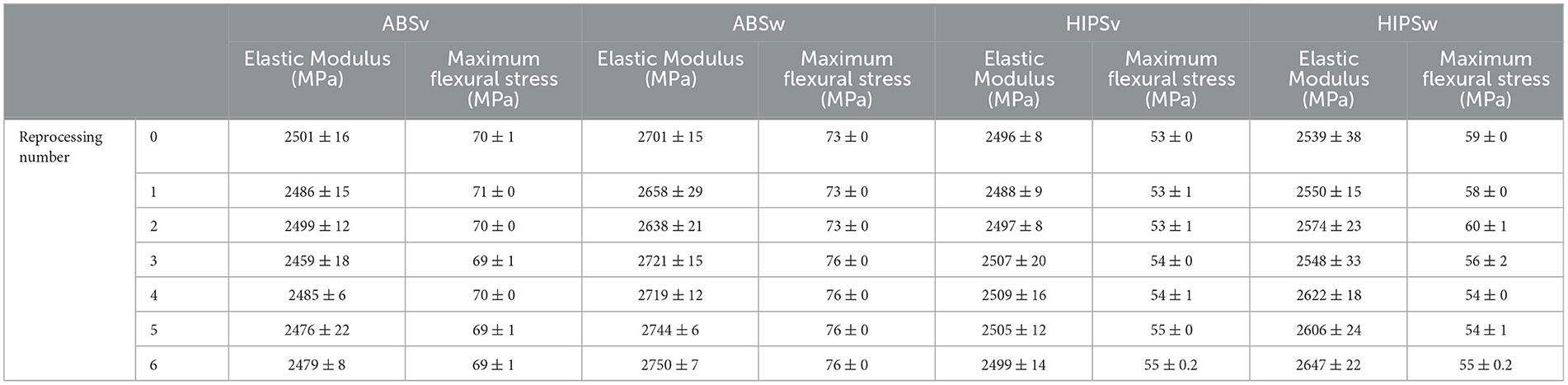
Table 8. Flexural properties of virgin and e-waste ABS and virgin and e-waste HIPS reprocessed six times.
Although there was almost no variation in elastic modulus and maximum flexural stress for ABSw until the second cycle, slight increases of 3, 4, and 4%, respectively, were observed from cycle three onwards. Greater maximum flexural stress was needed for ABSw from the third cycle, but declined from this cycle onwards for ABSv. The flexural modulus of ABSw was greater than that of ABSv. Rahimi et al. (2014) observed a 9% rise in the flexural modulus of ABS across five reprocessing stages, indicating that the stiffness of the polymer increased with the number of stages.
In HIPSv, elastic modulus, maximum flexural stress and flexural strength were almost the same after the six cycles (Table 8). The results demonstrate that flexural properties remained almost unaltered after six processing cycles.
For HIPSw, yield and flexural strength decreased by 7 and 6%, respectively, from the third cycle onwards. The modulus of reprocessed HIPSw was 6% higher than that of HIPSv. As observed in FTIR, bands corresponding to the butadiene group (C=C bonds) in both ABS and HIPS declined after reprocessing, suggesting polymer degradation. Degradation due to heat, light or other factors breaks the double bonds of the butadiene group, which have less energy, and can cause cross-link formation, reducing molecular mobility and, consequently, increasing the stiffness of the polymers with successive reprocessing cycles.
3.5.4.3. Impact strength
Table 9 shows the impact strength of reprocessed virgin and ABS e-waste. The impact strength of virgin ABS remained practically unchanged across all six cycles at approximately 115 J/m. For ABSw, there was a decline of around 14% by the second cycle, but the property remained almost the same from the third to sixth cycle considering the standard deviations. ABS and ABSv e-waste are polymers of different grades and have distinct characteristics, with the former exhibiting lower impact strength than the latter, making it more brittle. In other words, its ability to absorb energy under the load of the pendulum is lower than that of the virgin material, a finding also corroborated by tensile testing, whereby ABSw showed less elongation at break, indicating that it is less ductile.
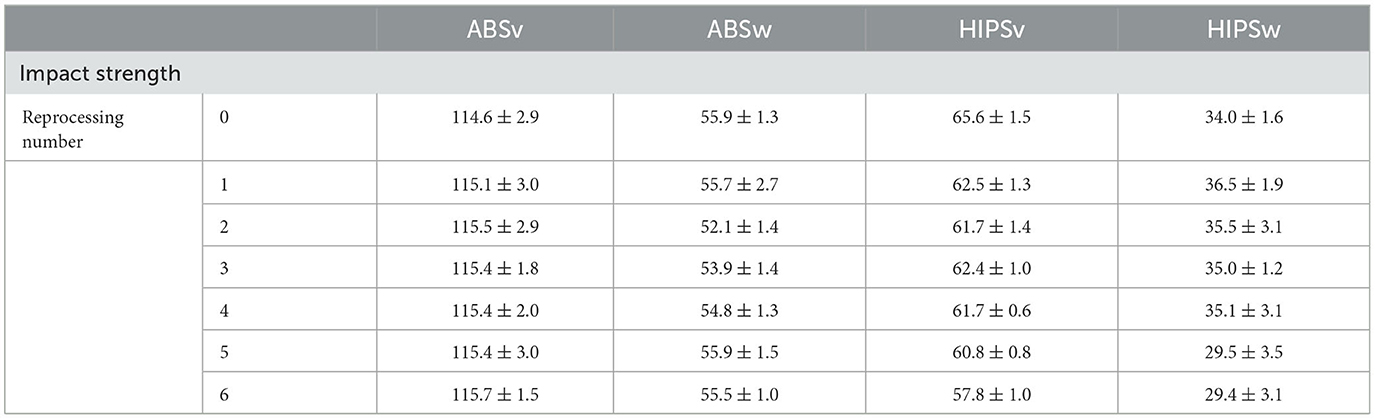
Table 9. Impact strength of virgin and e-waste ABS and virgin and e-waste HIPS reprocessed six times.
Rahimi et al. (2014) observed a 24% decrease in impact strength from the first to the fifth reprocessing cycle in ABS. Salari and Ranjbar (2008), also studied the impact strength of ABS reprocessed five times and found a 14% decrease in the first cycle, which they attributed to its smaller molar mass, lower entanglement density of the SAN matrix and polybutadiene degradation. Rahimi et al. (2014), reported that some additives used in ABS production improve its impact strength, so that their reduced efficacy during reprocessing may be another effect of this process.
Table 9 shows the impact strength of reprocessed virgin and HIPS e-waste. The impact strength of HIPSv remains almost the same (61 J/m) until cycle five and then declines by around 4% to approximately 58 J/m in cycle six. HIPSw exhibited impact strength of 35 J/m until the fourth reprocessing cycle, followed by a 16% reduction to about 29 J/m from cycle four to six. While both ABS and HIPS contain rubber, HIPS e-waste likely has a smaller rubber phase than its virgin counterpart, as confirmed by FTIR, in which the height of peaks corresponding to the butadiene group declined from the fifth reprocessing cycle onwards, suggesting lower impact strength. In the present study, recycled HIPS did not exhibit the degradation reported in the literature (Hirayama and Saron, 2015), indicating that the remolding conditions used were ideal for maintaining the properties of the polymer.
4. Conclusion
It was concluded that ABS and HIPS e-waste can be recycled in up to six cycles with no significant loss of mechanical, thermal or flow properties. There was no significant change in the technical aspects of ABS and HIPS e-waste over six reprocessing cycles, as observed in thermogravimetric analyses.
The tensile properties of all the polymeric materials studied here (ABSv, ABSw, HIPSv, and HIPSw) remained unchanged after six reprocessing cycles.
The melt flow index of virgin ABS increased by 11% from the first to the sixth extrusion cycle, but remained practically unchanged across six extrusion + injection cycles. For ABS e-waste, only impact strength was affected, showing a 14% decrease in cycle two, but then remained unchanged until cycle six.
Melt flow index was the property most affected in virgin HIPS across successive reprocessing cycles, increasing by around 30% from cycle one to six, unlike the behavior observed for HIPS e-waste. However, the impact strength of HIPS e-waste only changed from cycle five onwards, with a 16% reduction, lower than that reported in the literature.
Virgin ABS and HIPS exhibited greater stability than their e-waste counterparts; however, it is important to underscore that WEEE polymers have previously undergone molding and maintain their good properties across six reprocessing cycles after becoming waste, as observed in this study.
With respect to the environmental aspect, energy consumption in the first extrusion cycle for HIPSw was 12% greater than that of the other five cycles. This is because the larger flake size (>5 mm) in cycle one made it difficult to feed HIPSw into the extruder. Comparison between extrusion and injection showed that the latter consumed 143% more energy, since the injector used is more powerful than the extruder. Injection generates a larger amount of solid waste, including runner and sprue, which can be ground and recycled again, representing an additional benefit.
As such, recycled polymer e-waste such as ABS and HIPS show considerable potential for returning to the same production cycle. Nevertheless, products made with ABSw and HIPSw can also be considered in an open-loop recycling approach. Thus, it is important to pursue more sustainable alternatives for increasing the useful life of plastic waste from electrical and electronic equipment to comply with the circular economy.
Data availability statement
The original contributions presented in the study are included in the article/supplementary material, further inquiries can be directed to the corresponding author.
Author contributions
All authors contributed equally to data collection and writing the manuscript. All authors contributed to the article and approved the submitted version.
Funding
The results of this study were obtained as part of the Master's degree of FT, who received a grant from Coordination for the Improvement of Higher Education Personnel (CAPES).
Acknowledgments
The authors are grateful to the Coordination for the Improvement of Higher Education Personnel (CAPES) for the grant awarded to Lumirca Del Valle Espinoza León, the Research Support Foundation of Rio de Janeiro State (FAPERJ), and the National Council for Scientific and Technological Development (CNPq).
Conflict of interest
The authors declare that the research was conducted in the absence of any commercial or financial relationships that could be construed as a potential conflict of interest.
Publisher's note
All claims expressed in this article are solely those of the authors and do not necessarily represent those of their affiliated organizations, or those of the publisher, the editors and the reviewers. Any product that may be evaluated in this article, or claim that may be made by its manufacturer, is not guaranteed or endorsed by the publisher.
References
Arráez, F. J., Arnal, M. L., and Müller, A. J. (2019). Thermal degradation of high-impact polystyrene with pro-oxidant additives. Polym. Bull. 76, 1489–1515. doi: 10.1007/s00289-018-2453-4
ASTM International (2010a). D638-10. Standard Test Method for Tensile Properties of Plastics. doi: 10.1520/D.0638-10.1
ASTM International (2010b). D256-10. Standard Test Methods for Determining the Izod Pendulum Impact Resistance of Plastics. doi: 10.1520/D.0256-10
ASTM International (2013). D1238-13. Test Method for Melt Flow Rates of Thermoplastics by Extrusion Plastometer. doi: 10.1520/D.1238-13
ASTM International (2017). D790-17. Standard Test Methods for Flexural Properties of Unreinforced and Reinforced Plastics and Electrical Insulating Materials.
Bai, X., Isaac, D. H., and Smith, K. (2007). Reprocessing acrylonitrile-butadiene-styrene plastics: structure-property relationships. Polym. Eng. Sci. 47, 120–130. doi: 10.1002/pen.20681
Becker Junior, A. M., Dias, R. O., Chiumento, G., Motta, F. L. U. L., Almeida, L. G. O., Kabe, Y. H. O., et al. (2017). Desenvolvimento de base de dados ambiental para a cadeia de transformação de plástico no Brasil: primeiros avanços. Rev. Latino-Americana em Avaliação do Ciclo Vida 1, 48–62. doi: 10.18225/lalca.v1iespecial.3072
Beigbeder, J., Perrin, D., Mascaro, J. F., and Lopez-Cuesta, J. M. (2013). Study of the physico-chemical properties of recycled polymers from waste electrical and electronic equipment (WEEE) sorted by high resolution near infrared devices. Resour. Conserv. Recycl. 78, 105–114. doi: 10.1016/j.resconrec.2013.07.006
Bokria, J. G., and Schlick, S. (2002). Spatial effects in the photodegradation of poly(acrylonitrile-butadiene-styrene): a study by ATR-FTIR. Polymer 43, 3239–3246. doi: 10.1016/S0032-3861(02)00152-0
Brennan, L. B., Isaac, D. H., and Arnold, J. C. (2002). Recycling of acrylonitrile-butadiene-styrene and high-impact polystyrene from waste computer equipment. J. Appl. Polym. Sci. 86, 572–578. doi: 10.1002/app.10833
Campolina, J. M., Sigrist, C. S. L., de Paiva, J. M. F., Nunes, A. O., and da Moris, S. V. A. (2017). A study on the environmental aspects of WEEE plastic recycling in a Brazilian company. Int. J. Life Cycle Assess. 22, 1957–1968. doi: 10.1007/s11367-017-1282-2
Dimitrakakis, E., Janz, A., Bilitewski, B., and Gidarakos, E. (2009). Small WEEE: determining recyclables and hazardous substances in plastics. J. Hazard. Mater. 161, 913–919. doi: 10.1016/j.jhazmat.2008.04.054
Formosa Chemicals Fibre Corporation (2021). Products. Available online at: https://en.fcfc-plastics.com/product.htm (acessed January 10, 2021).
Gorna, K., Hund, M., Vučak, M., Gröhn, F., and Wegner, G. (2008). Amorphous calcium carbonate in form of spherical nanosized particles and its application as fillers for polymers. Mater. Sci. Eng. A 477, 217–225. doi: 10.1016/j.msea.2007.05.045
Gregorova, A. (2013). “Application of Differential Scanning Calorimetry to the characterization of biopolymers,” in Application of Calorimetry in a Wide Context - Applications of Calorimetry in a Wide Context - 4 Differential Scanning Calorimetry, Isothermal Titration Calorimetry and Microcalorimetry, Chapter 1, ed A. A. Elkordy (IntechOpen), 3–20. doi: 10.5772/53822
Ha, K. H., and Kim, M. S. (2012). Application to refrigerator plastics by mechanical recycling from polypropylene in waste-appliances. Mater. Des. 34, 252–257. doi: 10.1016/j.matdes.2011.08.014
Hirayama, D., and Saron, C. (2015). Characterisation of recycled acrylonitrile-butadiene-styrene and high-impact polystyrene from waste computer equipment in Brazil. Waste Manag. Res. 33, 543–549. doi: 10.1177/0734242X15584845
Hirayama, D., and Saron, C. (2018). Morphologic and mechanical properties of blends from recycled acrylonitrile-butadiene-styrene and high-impact polystyrene. Polymer 135, 271–278. doi: 10.1016/j.polymer.2017.12.038
Huysveld, S., Hubo, S., Ragaert, K., and Dewulf, J. (2019). Advancing circular economy benefit indicators and application on open-loop recycling of mixed and contaminated plastic waste fractions. J. Clean. Prod. 211, 1–13. doi: 10.1016/j.jclepro.2018.11.110
Innova (2021). Fichas técnicas. Available online at: https://www.innova.com.br/nossos-produtos/ (accessed January 10, 2021).
Kamelian, F. S., Mousavi, S. M., Ahmadpour, A., and Ghaffarian, V. (2014). Preparation of acrylonitrile-butadiene-styrene membrane: investigation of solvent/nonsolvent type and additive concentration. Korean J. Chem. Eng. 31, 1399–1404. doi: 10.1007/s11814-014-0068-5
Karahaliou, E. K., and Tarantili, P. A. (2009). Stability of ABS compounds subjected to repeated cycles of extrusion processing. Polym. Eng. Sci. 49, 2269–2275. doi: 10.1002/pen.21480
Karunadasa, K. S. P., Manoratne, C. H., Pitawala, H. M. T. G. A., and Rajapakse, R. M. G. (2019). Thermal decomposition of calcium carbonate (calcite polymorph) as examined by in-situ high-temperature X-ray powder diffraction. J. Phys. Chem. Solids 134, 21–28. doi: 10.1016/j.jpcs.2019.05.023
Klein, F. R. (2009). Estudo da degradação térmomecânica e fotooxidativa de poli(acrilonitrila-butadieno-estireno) para fins de reciclagem primária. Florianópolis: Dissertação de Mestrado do Programa de Pós-Graduação em Ciência e Engenharia de Materiais da Universidade Federal de Santa Catarina
Luna, C. B. B., Gomes, F. B. C., Siqueira, D. D., and Araújo, E. M. (2019). Influence of thermo-oxidative aging on the mechanical and yellowing properties of polystyrene blends with recycled styrene-butadiene rubber (SBR). Rev. Mater. 24, 1–13. doi: 10.1590/s1517-707620190003.0729
Menikpura, S. N. M., Santo, A., and Hotta, Y. (2014). Assessing the climate co-benefits from Waste Electrical and Electronic Equipment (WEEE) recycling in Japan. J. Clean. Prod. 74, 183–190. doi: 10.1016/j.jclepro.2014.03.040
Momanyi, J., Herzog, M., and Muchiri, P. (2019). Analysis of thermomechanical properties of selected class of recycled thermoplastic materials based on their applications. Recycling 4, 1–16. doi: 10.3390/recycling4030033
Pérez, J. M., Vilas, J. L., Laza, J. M., Arnáiz, S., Mijangos, F., Bilbao, E., et al. (2010). Effect of reprocessing and accelerated weathering on ABS properties. J. Polym. Environ. 18, 71–78. doi: 10.1007/s10924-009-0154-7
Ragaert, K., Delva, L., and Van Geem, K. (2017). Mechanical and chemical recycling of solid plastic waste. Waste Manag. 69, 24–58. doi: 10.1016/j.wasman.2017.07.044
Rahimi, M., Esfahanian, M., and Moradi, M. (2014). Effect of reprocessing on shrinkage and mechanical properties of ABS and investigating the proper blend of virgin and recycled ABS in injection molding. J. Mater. Process. Technol. 214, 2359–2365. doi: 10.1016/j.jmatprotec.2014.04.028
Ramesh, V., Biswal, M., Mohanty, S., and Nayak, S. K. (2014). Recycling of engineering plastics from waste electrical and electronic equipments: influence of virgin polycarbonate and impact modifier on the final performance of blends. Waste Manag. Res. 32, 379–388. doi: 10.1177/0734242X14528404
Rodrigues, A. C. (2007). Impactos socioambientais dos resíduos de equipamentos elétricos e eletrônicos : estudo da cadeia pós-consumo no Brasil (Dissertation/Master's thesis). Universidade Metodista de Piracicaba, Piracicaba, Brazil.
Salari, D., and Ranjbar, H. (2008). Study on the recycling of ABS resins: simulation of reprocessing and thermo-oxidation. Iran. Polym. J. 17, 599–610.
Santana, R. C., and Manrich, S. (2002). Studies on thermo-mechanical properties of post-consumer high impact polystyrene in five reprocessing steps. Prog. Rubber Plast. Recycl. Technol. 18, 99–110. doi: 10.1177/147776060201800202
Saron, C., and Felisberti, M. I. (2006). Ação de colorantes na degradação e estabilização de polímeros. Quim. Nova 29, 124–128. doi: 10.1590/S0100-40422006000100022
Scaffaro, R., Botta, L., and Di Benedetto, G. (2012). Physical properties of virgin-recycled ABS blends: effect of post-consumer content and of reprocessing cycles. Eur. Polym. J. 48, 637–648. doi: 10.1016/j.eurpolymj.2011.12.018
Silva, C. E., Silva, L. P., Edwards, H. G. M., and De Oliveira, L. F. C. (2006). Diffuse reflection FTIR spectral database of dyes and pigments. Anal. Bioanal. Chem. 386, 2183–2191. doi: 10.1007/s00216-006-0865-8
Silverstein, R. M., Webster, F. X., Kiemle, D. J., and Bryce, D. L. (2005). Spectrometric Identification of Organic Compounds. New York, NY: John Wiley and Sons.
Tansel, B. (2017). From electronic consumer products to e-wastes: global outlook, waste quantities, recycling challenges. Environ. Int. 98, 35–45. doi: 10.1016/j.envint.2016.10.002
Teixeira, F. S. M., Peres, A. C. C., Gomes, T. S., Visconte, L. L. Y., and Pacheco, E. B. A. V. (2021). A review on the applicability of life cycle assessment to evaluate the technical and environmental properties of waste electrical and electronic equipment. J. Polym. Environ. 29, 1333–1349. doi: 10.1007/s10924-020-01966-7
Thanh Truc, N. T., and Lee, B. K. (2017). Selective separation of ABS/PC containing BFRs from ABSs mixture of WEEE by developing hydrophilicity with ZnO coating under microwave treatment. J. Hazard. Mater. 329, 84–91. doi: 10.1016/j.jhazmat.2017.01.027
Tiganis, B. E., Burn, L. S., Davis, P., and Hill, A. J. (2002). Thermal degradation of acrylonitrile-butadiene-styrene (ABS) blends. Polym. Degrad. Stab. 76, 425–434. doi: 10.1016/S0141-3910(02)00045-9
Vilaplana, F., Ribes-Greus, A., and Karlsson, S. (2007). Analytical strategies for the quality assessment of recycled high-impact polystyrene: a combination of thermal analysis, vibrational spectroscopy, and chromatography. Anal. Chim. Acta 604, 18–28. doi: 10.1016/j.aca.2007.04.046
Wäger, P. A., and Hischier, R. (2015). Life cycle assessment of post-consumer plastics production from waste electrical and electronic equipment (WEEE) treatment residues in a Central European plastics recycling plant. Sci. Tot. Environ. 529, 158–167. doi: 10.1016/j.scitotenv.2015.05.043
Waheed, S., Cabot, J. M., Smejkal, P., Farajikhah, S., Sayyar, S., Innis, P. C., et al. (2019). Three-dimensional printing of abrasive, hard, and thermally conductive synthetic microdiamond-polymer composite using low-cost fused deposition modeling printer. ACS Appl. Mater. Interfaces 11, 4353–4363. doi: 10.1021/acsami.8b18232
Wang, J., Li, Y., Song, J., He, M., Song, J., and Xia, K. (2015). Recycling of acrylonitrile-butadiene-styrene (ABS) copolymers from waste electrical and electronic equipment (WEEE), through using an epoxy-based chain extender. Polym. Degrad. Stab. 112, 167–174. doi: 10.1016/j.polymdegradstab.2014.12.025
Wang, R., and Xu, Z. (2014). Recycling of non-metallic fractions from waste electrical and electronic equipment (WEEE): a review. Waste Manag. 34, 1455–1469. doi: 10.1016/j.wasman.2014.03.004
Keywords: waste electrical and electronic equipment, WEEE, ABS, HIPS, reprocessing, recycling
Citation: Teixeira FdSM, Peres ACdC and Pacheco EBAV (2023) Mechanical recycling of acrylonitrile-butadiene-styrene copolymer and high impact polystyrene from waste electrical and electronic equipment to comply with the circular economy. Front. Sustain. 4:1203457. doi: 10.3389/frsus.2023.1203457
Received: 10 April 2023; Accepted: 29 May 2023;
Published: 12 June 2023.
Edited by:
Md. Shahruk Nur-A-Tomal, Monash University, AustraliaReviewed by:
Antonella Patti, University of Catania, ItalySandro Donnini Mancini, São Paulo State University, Brazil
Copyright © 2023 Teixeira, Peres and Pacheco. This is an open-access article distributed under the terms of the Creative Commons Attribution License (CC BY). The use, distribution or reproduction in other forums is permitted, provided the original author(s) and the copyright owner(s) are credited and that the original publication in this journal is cited, in accordance with accepted academic practice. No use, distribution or reproduction is permitted which does not comply with these terms.
*Correspondence: Flávia da Silva Müller Teixeira, flavia.smt@ima.ufrj.br; Elen Beatriz Acordi Vasques Pacheco, elen@ima.ufrj.br