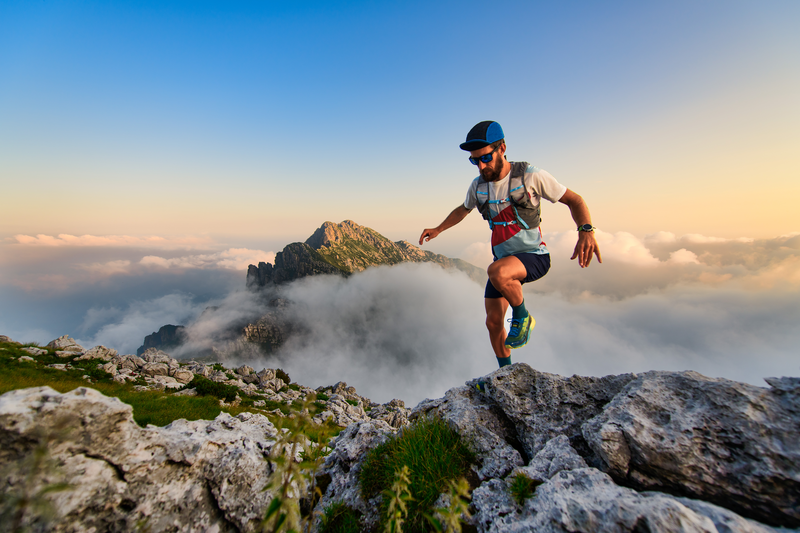
94% of researchers rate our articles as excellent or good
Learn more about the work of our research integrity team to safeguard the quality of each article we publish.
Find out more
REVIEW article
Front. Sustain. , 15 February 2022
Sec. Sustainable Chemical Process Design
Volume 3 - 2022 | https://doi.org/10.3389/frsus.2022.826190
Xylitol, a natural compound classified as a sugar alcohol, is found diversely in fruits and vegetables in small quantities. Commercial production of xylitol has expanded due to its health benefits and wide applications as an alternative sweetener in food and pharmaceutical products. Production of xylitol on large scale is industrially being achieved by the chemical method. However, the biotechnological method offers the possibilities of lowered cost and energy compared to the chemical methods. It involves the conversion of xylose to xylitol by microbes or enzymes which is environmentally safe. This review highlights the prospects of the biotechnological method of xylitol production. Various microorganisms that have been used to produce xylitol, the bioprocess parameters, and genetic modifications to increase xylitol yield have been reviewed. In addition, the applications, benefits, and safety concerns to health have been discussed.
Xylitol is one of the naturally occurring pentitols (five-carbon sugar alcohol) with a molecular formula of C5H12O5. It is a white crystalline sugar, commercially used as an artificial sweetener in the food and pharmaceutical industries. Xylitol has a lesser calorific value (2.4 cal/g) compared to sucrose (4.0 cal/g) but has a relative sweetness almost equal to sucrose (Chen et al., 2010; Tiefenbacher, 2017). The xylitol metabolism is independent of insulin; hence it has been used as a safe sucrose substitute for patients with diabetes. In the human gastrointestinal tract, 50–75% ingested xylitol is not absorbed (Rehman et al., 2013). Owing to its anti-cariogenic properties, it has been used in the production of chewing gums and toothpaste. Many studies show the role of xylitol to reduce the incidence of respiratory and middle ear infections. Xylitol increases the absorption of calcium thereby helps in combating osteoporosis (Mussatto, 2012).
In 1891, a German and French chemist concurrently discovered xylitol (Rehman et al., 2016). Several years later, xylitol crystals were purified successfully, and it was used widely as an alternative to sugar during World War II, to meet the severe shortage faced during the war (Rehman et al., 2013). Xylitol was recognized as a potent sweetener after the discovery of its property of not elevating blood sugar levels. Countries like Germany, Japan, and Italy started to use xylitol as a part of the diabetic diet. Later in the 1970s, the dental benefits of xylitol came into light after the “Turku Sugar Studies” (Janakiram et al., 2017). Since 1975, various xylitol substituted products were introduced globally.
Xylitol is naturally present in little quantity in diverse vegetables and fruits. Some of the fruits containing xylitol are strawberry, raspberry, banana, yellow plums, and vegetables containing xylitol include cauliflower, spinach, carrot, onion, white mushroom, eggplant, lettuce, and pumpkin. It is also found in hardwood trees like birch and beechwood and in husks and stalks of the plants (Chen et al., 2010). Humans and animals produce small quantities of xylitol as well, during the metabolism of glucose. On average, an adult human produces 5 to 15 g of xylitol per day (Rehman et al., 2016). Since the amount of xylitol present in these natural sources is low, its extraction from these sources is inefficient. Currently, the chemical method of xylitol production is being used commercially to meet the xylitol demand. In this chemical process, xylose extracted from the lignocellulosic biomass undergoes catalytic hydrogenation to produce xylitol. Alternatively, to reduce the high production costs, biotechnological methods of xylitol production from lignocellulosic biomass can be employed (Mathew et al., 2018). There is an increasing interest in commercializing biotechnological methods for the sustainable production of xylitol.
In this review, the production of xylitol has been discussed, with the main focus on the biotechnological process. Although different microorganisms and enzymes have been studied for xylitol production, there is an utmost need for exploring engineered strains for enhanced xylitol production. Hence this review focuses on the microbial and enzymatic production of xylitol and highlights the metabolic engineering of microorganisms as well. Furthermore, the applications of xylitol in the food and pharmaceutical industries, its health benefits, and safety concerns have been summarized.
Industrial production of xylitol is carried out by a chemical hydrogenation process where Raney nickel is used as a catalyst to convert xylose from hemicellulosic hydrolysate to xylitol. It involves five steps as follows: (1) Hydrolysis of the lignocellulosic biomass by acid to get monomeric sugars; (2) Treatment of hydrolysate to purify xylose; (3) Catalytic hydrogenation (usually carried out at a temperature of 353–413 K); (4) Xylitol purification; (5) Xylitol crystallization (Arcaño et al., 2020). By employing the catalytic hydrogenation method, about 50–60% of xylan from the hydrolysate can be converted to xylitol (Naidu et al., 2018). Other catalytic systems with Ruthenium, a noble metal, have also been proven to be effective in the hydrogenation of xylose to xylitol (Baudel et al., 2005; Yadav et al., 2012; Mishra et al., 2013). Recently, a catalytic formulation where noble metals have been replaced by non-noble metals like cobalt supported on silica is a better alternative catalyst (Audemar et al., 2020). However, the dependence of the chemical mode of synthesis seems to be non-sustainable and costly which prompted for biological routes of conversion of sugars to xylitol.
The biotechnological conversion of xylose to xylitol is carried out by microorganisms that produce enzymes for xylose metabolism. The substrate, xylose, is obtained from the hemicellulose-rich fraction of lignocellulosic biomass such as wood, agricultural wastes, or aquatic weeds (Sindhu et al., 2017; Espinoza-Acosta, 2020). Yeast is the predominant microorganism that can utilize xylose and ferment it to xylitol. Certain bacteria and filamentous fungi are also known to ferment xylose. In addition to microbial fermentation, enzymes have been used to produce xylitol as well. Furthermore, genetically engineered strains are being developed to improve xylitol yield to meet the industrial requirements (Xu et al., 2019).
The chemical method of xylitol production involves high pressure and temperature. It requires high energy and it is labor-intensive as well (Chen, 2015). Though the raw material for xylitol production is available throughout the world, the industrial expansion is limited to a few countries in Europe, Asia, and the United States due to the expensive production process (Arcaño et al., 2020). In the quest for an alternative method for producing xylitol, biotechnological methods have attracted the interest of researchers, which could address the above-mentioned limitations of the chemical method. Xylose bioconversion requires a simple fermentative approach and the overall energy consumption is relatively less (Chen, 2015). Microbial fermentation can be carried out under milder pressure and temperature compared to chemical methods. In the bioconversion process, organic waste can be extensively utilized and their environmental burden is reduced. Xylitol obtained through bioconversion can be used safely in food products as it does not have the risk of the presence of metal catalyst debris. The biotechnological process is safer and environmentally less polluting. Some inhibitors or impurities formed during hydrolysis of the biomass are either utilized or degraded partially by the microorganisms thus facilitating easier purification of the produced xylitol (Hernández-Pérez et al., 2019).
Consequently, the biotechnological method of producing xylitol has an added advantage in reducing production and purification costs and has the potential to replace chemical methods in terms of efficiency and sustainability. This is one of the main reasons behind the implementation of bioconversion methods of xylitol production by the leading manufacturers such as Thomson Biotech (China) and ZuChem (US) (Ravella et al., 2012). Figure 1 gives an overview of xylitol production strategy and its applications.
Unlike the chemical method of xylitol production, the biotechnological production of xylitol does not solely depend on purified xylose as a substrate. Bioconversion of xylitol can be done effectively by using various lignocellulosic biomass rich in hemicellulosic fraction as a carbon source (Chandel et al., 2018). The major portion of lignocellulosic biomass comprises cellulose, hemicellulose, and lignin while a small amount of pectin, ash, protein, and extractives are also present (Kumar et al., 2009). The cellulose bound to hemicellulose forms a matrix with lignin. Thus, formed lignocellulosic material functions as a protective complex for plants. Based on the species and age, different plant materials have a difference in the compositions of these constituents. Hemicellulose, which commonly makes up 25–35% is the second most abundant polymer of the lignocellulosic biomass, after cellulose. In some plants like water hyacinth, around 38–43% is made up of hemicellulose (Patel et al., 1993; Varanasi et al., 2018). Hemicellulose is a branched-chain heteropolymer made up of different sugars based on which it is classified as xylans (xylose and arabinose), mannans (mannose, galactose, glucose), and xyloglucans (xylose and glucose) (Naidu et al., 2018). Among these, xylan, which is comprised of β-D-xylopyranosyl units, makes up most of the hemicellulose fraction.
Lignocellulosic biomass that are rich in xylan content are widely used for xylitol production. Hemicellulose of hardwood (such as birch and oak) is found to have more xylose than softwood (Winkelhausen and Kuzmanova, 1998). In recent decades, wastes generated from agriculture or forestry are being employed to get value-added products such as xylitol. Sugarcane bagasse, corn stover, fruit pomaces, rice, and wheat straw, and sawdust are commonly investigated for xylitol bioproduction (de Albuquerque et al., 2014). The lignocellulosic biomass selection for industrial usage should be based on the presence of high xylan content, availability, and proximity to the industry.
The hemicellulosic fraction of the lignocellulosic biomass must be hydrolyzed to recover the pentose sugar, xylose, which is the main substrate for xylitol production. Compared to cellulose, it is easier to hydrolyze hemicellulose owing to its branched structure (Rafiqul and Sakinah, 2013). The yield of xylitol is influenced by the presence of by-products of hydrolysis and by the contamination of other fractions of the lignocellulose (Mussatto and Teixeira, 2010; Nair and Zhao, 2010). Pretreatment processes are included for efficient hydrolysis so that more xylose is accessible to the fermenting microorganisms and enzymes to produce a high xylitol yield. Pretreatment methods can be classified as (1) Physical methods—Microwave and Ultrasound methods; (2) Chemical methods—Dilute acid, ionic solvents, deep eutectic solvents, etc; (3) Physicochemical methods—Steam explosion, ammonia-based pretreatment; (4) Biological methods involving microorganisms like fungi (Kumar and Sharma, 2017). Table 1 compares different pretreatment methods with the respective xylitol yield.
Industrially, acid hydrolysis, particularly dilute-acid hydrolysis, is the most commonly employed method owing to its efficiency in hydrolyzing hemicellulose at a fast rate at less cost (Martin et al., 2013). Moraes et al. (2020) have obtained 99% extraction of xylose by pretreating the biomass with 1% sulphuric acid at 120°C. The use of nitric acid hydrolysis has been found to improve xylose extraction (Dalli et al., 2017b; Manaf et al., 2018; Shah et al., 2020). Acid pretreatment releases monomeric sugars from the hemicellulose thereby eliminating the need for a further hydrolysis step. Nevertheless, acid hydrolysis has the drawback of producing inhibitory by-products and it is corrosive to the reactor vessels as well. Furthermore, it hydrolyzes cellulose along with hemicellulose. Enzymatic hydrolysis, on the other hand, can produce xylose specifically and minimize the formation of by-products. In a study by Mardawati et al. (2018) comparing acid and enzymatic hydrolysis methods, it was observed that the specific growth rate of microbes and the xylitol yield were higher in the hydrolysate obtained by enzymes.
The hemicellulosic fraction can be solubilized by an eco-friendly method called auto-hydrolysis. This method, also known as the hydrothermal pretreatment method, uses just water to convert hemicellulose into oligomers from which monomeric sugars can be recovered using further enzymatic hydrolysis (Mardawati et al., 2020). Here, the biomass is treated at high pressure and temperature of around 200°C due to which the acetyl groups in the hemicellulose get hydrolyzed to release acids that break bonds between the carbohydrates and lignin. Baptista et al. (2018, 2020) have used the auto-hydrolysis method to pretreat corncob and have reported the highest titer of xylitol production using recombinant Saccharomyces cerevisiae.
Solvents such as ionic liquids and deep eutectic solvents (DES) are grabbing researchers' attention in recent years to pretreat hydrolysates (Behera et al., 2014; Liu et al., 2019). Although the physiochemical properties of these two solvents are alike, it has been observed that DESs are more advantageous owing to the cost and biocompatible nature. Ai et al. (2020) have obtained xylose and glucose yield of around 85%, employing DES-mediated extrusion for pretreating sorghum bagasse. The choice of the pretreatment method is purely based on the application. Combining two pretreatment methods can also be advantageous in obtaining a better yield of monomeric sugars (Kumar and Sharma, 2017).
Upon pretreatment and hydrolysis of biomass, apart from releasing monomeric sugars, many inhibitory compounds are produced. These compounds are derived from lignin or generated from the degradation of sugars, and they inhibit the fermentative microorganisms and enzymes. Furan compounds like furfural and hydroxymethylfurfural, phenolic compounds like vanillin and syringaldehyde, and acids such as formic acid, acetic acid, and levulinic acid are some of the common inhibitors formed in the hydrolysate of lignocellulosic biomass (Valdes et al., 2020; Bianchini et al., 2021). In the case of acid hydrolysis, inhibitors in the form of heavy metals can be produced due to corrosion of the equipment (Rehman et al., 2015). The type of inhibitors produced, and their concentration depends on the source of biomass and the treatment conditions used. The presence of phenolic compounds can alter the membrane integrity of the microorganisms. Damage to the DNA structure and enzyme activity can occur due to furans (Rao et al., 2016). The acids formed, affect the metabolism of xylose in yeast (Rafiqul et al., 2015a). The effect of inhibition depends on the concentration of the compounds, the fermentation conditions, and the ability of microorganisms to tolerate the inhibitory compound (Hernández-Pérez et al., 2019).
To obtain a better yield of xylitol from the xylose present in the hydrolysate, it is necessary to detoxify the hydrolysate and remove or inactivate the inhibitory compounds before fermentation. Neutralization, activated charcoal treatment, overliming, solvent extraction, ion-exchange resin treatment, and microbial detoxification are some of the commonly used detoxification methods. (Soares et al., 2016; Gupta et al., 2017; Kumar et al., 2018; Agarwal and Singh, 2019; Llano et al., 2021). Kumar et al. (2019) have reported the efficient removal of acetic acid and salts in the hydrolysate by employing a detoxification method involving activated charcoal followed by membrane filtration. However, the loss of sugar is a limitation of these methods. To minimize sugar loss while removing inhibitors, Dalli et al. (2017a) combined vacuum evaporation with solvent extraction and achieved 97 and 87.5% of furfural and acetic acid removal respectively.
Besides the research on the efficient and cost-effective detoxification methods, some researchers use adapted strains or recombinant strains of yeast to avoid a detoxification step (Santana et al., 2018). It has been reported in a study that a genetically engineered strain of Candida tropicalis has produced 0.98 gg−1 of xylitol from non-detoxified hydrolysate (Hong et al., 2016). The tolerance capacity of the microbial species used, the composition of the hydrolysate, the concentration, and the type of inhibitors present to decide the necessity for a detoxification step before fermentation. Also, a techno-economic analysis considering the cost of the detoxification method and the yield of xylitol should be taken into account.
Naturally, xylose is found in abundance in the hemicellulosic fraction of lignocellulosic biomass. Yet, there is a drawback in the industrial production of xylitol from xylose due to the lack of an economically viable availability of pure xylose (Cheng et al., 2014). Addressing this limitation, glucose might be used as a better and cheaper alternative for large-scale xylitol production. Early studies on the production of xylitol from glucose involved three steps. In the first step, D-glucose is converted to D-arabitol by Debaryomyces hansenii. Then D-arabitol is oxidized to D-xylulose by Acetobacter suboxydans. Finally, D-xylulose is converted to xylitol by C. guilliermondii var. soya. (Onishi and Suzuki, 1969). However, the yield of xylitol obtained is low, which limits the application of this method in large-scale production.
To convert glucose directly to xylitol, studies on the construction of genetically engineered microorganisms are being done. Povelainen and Miasnikov (2007) have expressed the xylitol-phosphate dehydrogenase gene in a strain of Bacillus subtilis that resulted in the production of xylitol from D-glucose with a yield of 23%. Cheng et al. (2014) have genetically engineered a Pichia pastoris strain with the xylose dehydrogenase gene and D-arabitol dehydrogenase gene from different microorganisms. This engineered strain converted glucose into xylitol in a single fermentation with a yield of 0.078 g xylitol/g glucose. Further, the authors concluded that the yield of D-arabitol could be improved if glucose is made to enter the pentose phosphate pathway and the cell NADPH demand is altered. The major issues to be addressed in the single fermentation process of xylitol production from D-glucose are (1) the yield and productivity of D-arabitol by genetically engineered strains is low and (2) the production of by-products such as other polyols have to be reduced as it may increase the osmotic stress of the cells (Qi et al., 2014). Thus, more studies on the screening of strains producing a better yield of D- arabitol and optimization of the conditions of fermentation are required to make the production of xylitol from D-glucose industrially feasible.
The biotechnological process of xylitol production is centered on the metabolism of xylose by microorganisms that can naturally utilize pentose sugars as a carbon source. Xylitol is produced by these microorganisms as an intermediate in the metabolic pathway of xylose (Narisetty et al., 2022). The first step in the metabolism of D-xylose occurring in yeast and fungi involves xylose reductase which reduces D-xylose to xylitol (Verduyn et al., 1985). The xylitol thus produced is secreted out of the cell or otherwise, it is oxidized by xylitol dehydrogenase to produce D-xylulose (Rizzi et al., 1989; Yablochkova et al., 2003). The secretion or oxidation of xylitol depends on the availability of cofactors. Xylose reductase requires NADPH or NADH and xylose dehydrogenase requires NADP or NAD for their activities. D-xylulose further gets phosphorylated by the action of xylulokinase and gets integrated with the non-oxidative route of the pentose phosphate pathway (Ravella et al., 2012) (Figure 2). In the process of xylitol bioconversion, continuous availability of NADPH is required so that there is limited oxidation of xylitol to xylulose. Numerous research works have been done to screen microbial strains that can efficiently produce xylitol (Hernández-Pérez et al., 2019; Narisetty et al., 2022).
Figure 2. Metabolic pathways involved in the assimilation of xylose, arabinose and glucose from the hemicellulosic hydrolysate by yeast.
In contrast, bacteria contain the enzyme xylose isomerase that can directly convert D-xylose into D-xylulose which further enters the pentose phosphate pathway after phosphorylation. However, there are some exceptions. Some of the earlier studies done in the 1970s have shown that a few bacterial strains belonging to Corynebacterium and Enterobacter species contain the enzyme xylose reductase and have the ability to accumulate xylitol (Yoshitake et al., 1973a,b). In a study by Izumori and Tuzaki (1988), Mycobacterium smegmatis was found to produce xylitol. Several bacterial cultures belonging to the species Cellulomonas, Corynebacterium, and Serratia were screened for xylitol production by Rangaswamy and Agblevor (2002) and it was observed that among those, Corynebacterium produced maximum xylitol yield. Recently it has been found that Pseudomonas putida can produce xylitol with a volumetric productivity of 0.98 g L−1 h−1 which is higher than other bacterial strains (Lugani and Sooch, 2020). Filamentous fungi such as Penicillium chrysogenum and Petromyces albertensis were first studied for xylitol production (Dahiya, 1991). In 2003, Sampaio et al. screened Aspergillus niger and 10 different strains of Penicillium and it was seen that Penicillium crustosum had the highest yield. However, bacteria and filamentous fungi do not favor xylitol production as much as yeast does. Table 2 shows xylitol bioproduction by different microorganisms.
Yeast is widely studied due to the high xylitol productivity and assimilation of xylose. The most common yeast that produces high xylitol yield includes Candida and Debaryomyces (Guo et al., 2006; Sampaio et al., 2008). In a recent study by Carneiro and Almeida (2019) involving 44 isolates, it was found that Meyerozyma species were the best utilizers of xylose and Wickerhamomyces anomalus produced high xylitol yield. Candida strains such as C. tropicalis and C. silvanorum are the most promising xylitol producers as they have been found to have the highest xylose reductase activity (Yablochkova et al., 2003). López-Linares et al. (2018) compared the production of xylitol by Debaryomyces hansenii and Candida guilliermondii and observed that C. guilliermondii showed high tolerance to inhibitors like furans and acids thus possessing the advantage of not requiring additional detoxification steps. Similarly, a strain of C.tropicalis was found to produce xylitol with high tolerance to acetic acid in the hydrolysate (Junior et al., 2019). Generally, the yield of xylitol is less if the hydrolysate is not detoxified. However, Prabhu et al. (2020a) obtained a high yield of xylitol using Pichia fermentans without detoxifying the hydrolysate. Oleaginous yeast such as Yarrowia lipolytica has also studied in the xylitol bioproduction (Prabhu et al., 2020b). Furthermore, xylitol production has been done by genetic manipulation of microorganisms such as Saccharomyces cerevisae and Escherichia coli which has been discussed in section Metabolic engineering for enhanced xylitol production .
Until now microorganisms are commonly used for most studies on xylitol production. However, limitations in product purity and stability and lower enzyme to substrate ratio have paved the way for research on xylose bioconversion through enzymatic method instead of using whole cells, to meet the demands on a large scale. In the microbial production of xylitol, xylose is required for cell growth and cell maintenance. Since the enzymatic method does not require such tedious process, 100% of xylose can be converted to xylitol (de Freitas Branco et al., 2011). The key enzyme acquiring industrial importance in converting xylose to xylitol is xylose reductase (XR) (Lugani et al., 2021). Kitpreechavanich et al. (1984) reported that 90% of xylose was enzymatically converted into xylitol by xylose reductase from Candida pelliculosa coupled with a methanogen oxidoreductase system for NADPH recycling. Other NADPH recycling systems including formate dehydrogenase and glucose dehydrogenase have also been studied (Xu et al., 2019). Yeast like Candida guilliermondii, Candida tenuis, Candida tropicalis which are the best xylitol producers are used to obtain XR enzyme (Häcker et al., 1999; Tomotani et al., 2009; Kim et al., 2019). Recently, hemicellulosic hydrolysates have been used for the enzymatic bioconversion process and high xylitol yield has been obtained (Rafiqul and Sakinah, 2015; Rafiqul et al., 2015a, 2021).
In addition to the advantage of the high yield of xylitol, the energy, water requirement, and time of incubation are comparatively lesser in the enzymatic bioconversion method (Rafiqul and Sakinah, 2013). However, the enzymatic bioconversion faces two main constraints which are (1) the high cost involved in the preparation of xylose reductase enzyme; (2) the requirement of a constant supply of NADPH. The development of stable XR and an effective system for cofactor regeneration are needed for improving enzymatic xylitol production (de Freitas Branco et al., 2012).
The pH suitable for yeast growth is generally acidic. Characterization of xylose reductase from a Candida tropicalis strain showed that the enzyme was active at a pH of 5 to 7 (Rafiqul et al., 2015b). The optimum pH for the production of xylitol by Candida strains has been reported to be 5 and 5.5 (Rudrangi and West, 2020; West, 2021). The production of xylitol by yeast generally employs a temperature of 30°C. However, depending on the species used, the optimum temperature range of xylitol producing yeast varies between 30 and 37°C. In a study employing Candida tropicalis for the production of xylitol, it was observed that there was a consumption of about 90% xylose at a temperature range of 29 and 34°C and there was a decrease in xylose consumption below 28°C and above 35°C (Tamburini et al., 2015). Sampaio et al. (2006a) investigated the effect of temperature and pH on xylitol production by a Debaryomyces hansenii strain and concluded that the optimum temperature and pH range was 30–35°C and 4–8 respectively. Nevertheless, some research employs microorganisms tolerant to higher temperatures. Zhang et al. (2014) have used a recombinant strain of Kluyveromyces marxianus that produced high productivity of 1.49 g L−1 h −1 xylitol from 100 g L−1 xylose at a temperature of 42°C. Temperature influences the microbial growth rate and the production of xylitol as the regulation of transport proteins involved in xylose sequestration and the enzyme activity depend on the temperature (Tamburini et al., 2015).
For obtaining a high yield of xylitol, the initial concentration of xylose must be high since xylose is necessary to induce the enzymes involved in xylitol production (Winkelhausen and Kuzmanova, 1998). To increase the initial concentration of xylose, hemicellulosic hydrolysates undergo a concentration step which will also possibly increase the inhibitor concentration present in the hydrolysate (Hernández-Pérez et al., 2019). Moreover, during batch fermentation, there might be substrate inhibition due to high initial substrate concentration. Thus, the initial xylose concentration has to be optimized such that there is a high xylitol yield and the performance of the microorganisms is not affected. It should be noted that the optimum initial xylose concentration depends on the type of hydrolysate and yeast strain used (Mussatto and Roberto, 2008). Apart from using a detoxification step, increasing the inoculum concentration could also help in reducing the effects of the inhibitory compounds in the hydrolysate (Felipe et al., 1997). To enhance the production of xylitol, co-substrates such as glucose and glycerol can be supplemented (Kogje and Ghosalkar, 2017; Ariyan and Uthandi, 2019). One of the key elements in the metabolism of D-xylose in yeast is oxygen. El-Baz et al. (2011) have reported that reduced aeration increases the xylitol production rate. In another study, it was reported that Pichia guilliermondii favored the production of xylitol at a very low volumetric oxygen transfer coefficient (kLa) of 0.075/h (Zou et al., 2010). Low kLa value favors xylitol production as an increase in kLa value will direct the metabolism toward cell growth rather than xylitol production (Martínez et al., 2000).
Batch fermentation in Erlenmeyer flasks or bioreactors is most commonly used for xylitol production (Mussatto and Roberto, 2003; López-Linares et al., 2018; Silva et al., 2020). A study by Shah et al. (2019) showed that batch fermentation of kenaf hydrolysate by a recombinant Escherichia coli gave a xylitol yield of 0.38 gg−1. Fed-batch fermentations can give better xylitol productivity as the concentration of substrate can be maintained constant. Li et al. (2012) carried out a two-stage fed-batch fermentation using Candida tropicalis and have reported that the xylitol productivity was 65.57% more than batch fermentation. Su et al. (2015) have reported the highest productivity of xylitol using E. coli by employing fed-batch fermentation. Recently, Ramirez and Escoto (2021) have implemented a genetic algorithm study to enhance xylose fermentation by a fed-batch process. A study by Martinez et al. (2003) describing the xylitol production by continuous fermentation of sugarcane hydrolysate by C. guilliermondii resulted in productivity of 0.68 gL−1h−1. Salgado et al. (2012) have employed a technique where two continuous stirred tank reactors were connected for the production of lactic acid and xylitol and this setup gave xylitol productivity of 0.218 gL−1h−1.
To increase the fermentation rate and reuse the cells, immobilization of cells is being preferred. Immobilization can be done by entrapment in beads or gels using carriers like sodium alginate, polyvinyl alcohol polyacrylamide agarose gel, κ-carrageenan, and gelatin (Yewale et al., 2016). Abd Rahman et al. (2020) have implemented immobilization of recombinant E. coli cells on multi-walled carbon nanotubes to enhance xylitol production. Recently, carbon fiber treated with Fenton reagent has been found to improve immobilization efficiency which resulted in improved xylitol production (Wang et al., 2021). Production of xylitol using immobilized cells can be carried out using different modes of operations such as batch, continuous, and fed-batch fermentation (Pérez-Bibbins et al., 2015).
The viability of the bioproduction of xylitol depends on the downstream processing and recovery of xylitol from the fermentation broth. The nature of the product such as its size, the fermentation broth's concentration, and the presence of impurities like sugars, other sugar alcohols, media constituents, and inhibitory compounds in the broth decide the type of recovery process to be used (Martínez et al., 2015). The most common method employed for xylitol purification is crystallization. However, the broth must be clarified before the crystallization step to produce pure crystals. Clarification of the fermented media is usually done by using ion exchange resins, activated charcoal, liquid-liquid extraction, and membrane separation (Sampaio et al., 2006b; Canilha et al., 2008a; Misra et al., 2011). Faneer et al. (2017) have enhanced the purity of xylitol to 92% using polyethersulfone nanofiltration membrane which was efficient in removing other impurities like arabinose as well. After concentrating the broth using ion exchange resins, a two-stage crystallization process was performed by Martínez et al., by which up to 94% pure xylitol crystals were obtained (Martínez et al., 2007). In recent years, there is a search for greener methods for downstream processing that will be eco-friendly and cost-efficient as well. Faneer et al. (2018) have employed a pressure filtration membrane to obtain a high concentration of xylitol. Junior and Rocha (2021) have developed a purification process for xylitol using protic ionic liquids which gave a crystal yield of 70% with 85% purity.
Many wild and engineered strains are explored for increased xylitol production. Some of the wild potent microorganisms with inbuilt xylitol producing capacity are Candida sp., Pichia sp., Debaryomyces sp., Mycobacterium sp., Gluconobacter sp., and Corynebacterium sp. (Guo et al., 2006). To enhance xylitol production, genetic engineering techniques such as the introduction of genes coding for the key enzymes, disruption of hindering genes, or overexpression of existing gene is adopted (Johan et al., 1991; Handumrongkul et al., 1998). Table 3 shows some of the important engineered microorganisms with enhanced xylitol production. Key enzymes for xylitol production such as xylose reductase and xylose isomerase are isolated from potent fungi and are incorporated into microorganisms using genetic engineering techniques.
Guirimand et al. (2019) showed that engineered Saccharomyces cerevisiae with cell surface display of Aspergillus aculeatus β-glucosidase (bgl1), Trichoderma reesei endoglucanase II (egl2), Aspergillus oryzae β-xylosidase A (xyl A) and cytosolic Scheffersomyces stipitis xylose reductase (Xyl1) results in enhanced xylitol production with 44% conversion rate (xylitol yield-3.7 gL−1) after 96 hours. Su et al. (2015) improved xylitol production in E. coli by blocking xylose catabolism and xylitol phosphorylation. Xylose catabolism is blocked by deleting xylulose kinase (xylB) and xylose isomerase (xylA) genes and phosphorylation of the xylitol produced is blocked by disrupting fructose phosphotransferase system (ptsF) resulting in increased production of xylitol (xylitol yield of 172.4 gL−1 is achieved after 110 h of batch fermentation).
Kim et al. (2015) engineered E. coli for increased xylitol production by introducing the following changes in the gene structure: (1) Deletion of arabinose transcriptional regulator (araC); (2) Intergenic mutation in xylose isomerase (xylA) and Orotate phosphoribosyl transferase (pyrE); (3) Missense mutation in Arabinose-proton symporter (araE) and Putative undecaprenyl-diphosphatase (ybjG). The resulting mutant strain E. coli GX20 showed rapid conversion of xylose to xylitol even in the presence of glucose in the medium. Zha et al. (2013) engineered Saccharomyces cerevisiae with xylose reductase (xyl1) from Scheffersomyces stipitis and β-glucosidase (gh1-1) and cellodextrin transporter (cdt-1) from Neurospora crassa resulting in increased xylitol production by 85.7% when co-fermented with cellobiose and xylose compared to co-fermentation with glucose and xylose. Oh et al. (2013) engineered Saccharomyces cerevisiae with xylose reductase (xyl1) from Scheffersomyces (Pichia) stipitis and β-glucosidase (gh1-1) and cellodextrin transporter (cdt-1) from Neurospora crassa, increased overall xylitol productivity by 40% when co-fermented with cellobiose and xylose.
One of the largest commercially produced sugar alcohols is xylitol. In the 1970s, a Finnish company commercialized xylitol production (Honkala et al., 1999). DuPont, an American-based company is a highly noted world's largest producer of xylitol from renewable resources such as hardwoods and maize as the feedstocks and commercialize xylitol under the trade name xivia (Ravella et al., 2012). Xylitol is considered to be one of the major value-added chemicals that can be produced from biomass (Werpy and Petersen, 2004). By 2020, the market value of xylitol was 921 million USD globally and it is projected to become 1.37 billion USD in 5 years [International Market Analysis Research Consulting Group (IMARC), 2020]. Xylitol has been certified to be safe for consumption by the US Food and Drug Association (Food Drug Administration, 2006). Nearly 70% of xylitol production is used in the production of confectionery items and chewing gums (Ahuja et al., 2020). In the bioproduction of xylitol, techno-economic analysis and assessment of environmental impact must be done at every step of the production process. This will ensure that the biomass is valorized sustainably with minimal waste production. To achieve a circular bio-based economy, every component of biomass has to be utilized to produce a wide range of products like fuels, chemicals, and energy. Integrating xylitol production with other products makes the bioconversion process industrially feasible (Morales-Rodriguez et al., 2016; Medina et al., 2018).
For the success of a biorefinery, it is crucial to understand the environmental impact through a life cycle assessment. Hafyan et al. (2019) have studied the environmental impact, hazard potential, and techno-economic aspects to select the optimum capacity for producing xylitol. Dasgupta et al. (2021) have estimated the material and energy balance and carbon dioxide emission levels in the bioproduction of xylitol using corncob. It has been reported that the energy requirement and greenhouse gas emission can be reduced by employing heat integration between processes.
Currently, more than 35 nations have endorsed the utilization of xylitol in food sources, drugs, and oral wellbeing items, chiefly in chewing gums, toothpaste, syrups, and candy preparations (Barathikannan and Agastian, 2016). Routine xylitol utilization might be characterized as everyday utilization of 5–7 g of xylitol no less than three times each day. The suggested portion for dental caries avoidance is 6–10 g/d but it varies from one individual to another. According to many studies, the maximum dosage of xylitol for children is 45 g/d and for adults is 100 g/d and the acceptable dosage is 40 g/d (Nayak et al., 2014).
Long-term use of conventional white sugar in food and food products leads to many complications such as diabetes, inflammatory diseases, gingivitis, obesity, cardiovascular problems, metabolic syndrome, and dental caries. People suffering from obesity, find it too difficult to lose weight. Weight gain is directly linked to an increase in cholesterol level in the blood and lipid storage and cause many cardiovascular diseases. Replacing white sugar with xylitol helps in stabilizing blood sugar levels and decreasing overall lipid storage (Islam and Indrajit, 2012). It contributes to weight reduction and indirectly prevents the onset of cardiovascular disease. The diet of nursing women and pregnant ladies with gestational diabetes can be replaced by food items containing xylitol to prevent the impact of diabetes (Yamagata et al., 1969). When consumed, xylitol is digested into carbon dioxide and water, requiring no insulin for metabolism and having no effect on blood glucose levels. Compared to glucose only 20–30% of consumed xylitol is absorbed in the upper gastrointestinal tract and then it is non-actively transported through the intestinal tract.
Using xylitol as a sweetness enhancer in food preparation improves certain properties of food such as taste, color, longevity, and texture (Benahmed et al., 2020). Xylitol is extensively used in the manufacturing of chewing gums, chocolate, hard candies, wafer fillings, chocolate, pastilles, pectin jellies, ice cream fillings, and other sweets. Xylitol possesses some of the properties such as remineralization, moisture retention, non-fermentability, microbial stability, high solubility, and prebiotic effect (Mäkinen, 2000). Xylitol provides flexibility, a pleasing and cooling effect to the confectioneries. In the manufacturing of xylitol-based chewing gums, the cooling effect is promoted by the endothermic property (34.8 cal/g) of xylitol. As it does not undergo milliard reaction, xylitol will not char on heating and because of this property, it gives unique taste and color to the food items. It is used to sweeten the flour which is used for making bread, rusks, and cakes. Crystalline xylitol is used as a sanding material in the preparation of sweets and confectioneries. It is also used in the process of protein extraction as a stabilizing agent as it prevents the denaturation of protein. This property of xylitol is used to increase the shelf life of food products and so xylitol is highly recognized as a food preservative. In the food industry, xylitol is used as a stabilizer, moisturizer, antioxidant, and cryoprotectant.
Xylitol is markedly used in the manufacturing of toothpaste and mouth rinses for people with gum problems and sensitive gums due to its anti-cariogenic and tooth rehardening property (Janket et al., 2019). Streptococcus mutans and Helicobacter pylori are the oral pathogenic bacteria causing tooth decay, xerostomia, plaque formation, gingival inflammation, and erosion of teeth. These bacteria metabolize sugar residues present in the mouth and feed on them. Xylitol cannot be metabolized by these bacteria and so brushing teeth with toothpaste containing xylitol prevents dental caries. Due to the endothermic property of xylitol, toothpaste and mouthwashes give a cooling and refreshing effect. Xylitol sweetened night medicines after brushing are highly recommended for children (Feigal et al., 1981). Xylitol shows well-defined anti-bacterial properties and inhibits the growth of microorganisms. It also prevents the attachment of these microorganisms to the teeth surface and reduces their corrosive activity by reducing acid production potential (Nayak et al., 2014).
Streptococcus pneumoniae and Haemophilus influenzae are the common otopathogens causing ear infections such as acute otitis media. Xylitol with its anti-bacterial and anti-inflammatory potential reduces upper respiratory tract and middle ear infections by preventing the growth of the bacteria. Chewing gums and syrup containing xylitol have been displayed to ensure from the middle ear infection in children (Uhari et al., 1996; Vernacchio et al., 2014). Xylitol is used in the manufacturing of capsules and is added in oral drug preparations such as syrups, tonics, and vitamin formulations to increase palatability.
Some reports showed that the consumption of xylitol can reduce constipation, and for some people, it induces some side effects such as irritable bowel syndrome, diarrhea, and nephrolithiasis. Many cases have reported the toxic effect of xylitol on dogs. However, according to the animal poison control center of American society, xylitol is considered safe for other mammals like humans and cats, except for dogs [Peterson, 2013; American Association of Poison Control Centers (AAPCC), 2021].
Xylitol is a commercially successful, high market value artificial sweetener, produced by the biological action of microorganisms on the pentose sugar xylose. Low calorific value, insulin-independent metabolism, anti-cariogenic property, remineralization property of xylitol is highly exploited in food and pharmaceutical industries. Commercially many food and candy items, confectionery, pharmaceutical products are produced by replacing sucrose with xylitol. Due to the high demand for xylitol, the global production rate had been increased tremendously. The chemical method of xylitol production is cost-ineffective and not environmentally safe and the enzymatic method of production is time-consuming. The production of xylose-rich hemicellulosic hydrolysate is another snag in the large-scale production of xylitol. These difficulties can be overcome by the microbial method of xylitol production. Various genetic engineering strategies to modulate key enzymes such as xylose reductase, xylose isomerase, xylulokinase for enhanced xylitol yield and optimizing fermentative parameters based on kinetics studies, modeling and simulation need to be employed for large scale production of xylitol. Recent advances in integrated biorefinery approach, biomass conversion innovations, and metabolic engineering techniques would disclose new fortuity for economical and eco-friendly production of xylitol to meet the growing demand.
DU and RK has equally contributed in drafting the manuscript with relevant information. VK involved in critical inputs in framing the manuscript outline. SJ is responsible for coordinating the team, framing the outline, correction, and final drafting of the manuscript. All authors contributed to the article and approved the submitted version.
The authors declare that the research was conducted in the absence of any commercial or financial relationships that could be construed as a potential conflict of interest.
All claims expressed in this article are solely those of the authors and do not necessarily represent those of their affiliated organizations, or those of the publisher, the editors and the reviewers. Any product that may be evaluated in this article, or claim that may be made by its manufacturer, is not guaranteed or endorsed by the publisher.
Abd Rahman, N. H., Jahim, J. M., Munaim, M. S., Rahman, R. A., Fuzi, S. F., and Illias, R. M. (2020). Immobilization of recombinant Escherichia coli on ulti-walled carbon nanotubes for xylitol production. Enzyme Microb. Technol. 135:109495. doi: 10.1016/j.enzmictec.2019.109495
Agarwal, B., and Singh, L. K. (2019). “Sugar and Sugar Alcohols: Xylitol,” in High Value Fermentation Products: Human Health, ed. S. Saran, V. Babu, A. Chuabey (Wiley online library), 285–307.
Ahuja, V., Macho, M., Ewe, D., Singh, M., Saha, S., and Saurav, K. (2020). Biological and pharmacological potential of xylitol: a molecular insight of unique metabolism. Foods. 9,1592. doi: 10.3390/foods9111592
Ai, B., Li, W., Woomer, J., Li, M., Pu, Y., Sheng, Z., et al. (2020). Natural deep eutectic solvent mediated extrusion for continuous high-solid pretreatment of lignocellulosic biomass. Green Chem. 22, 6372–6383. doi: 10.1039/D0GC01560A
Akinterinwa, O., and Cirino, P. C. (2008). Heterologous expression of D-xylulokinase from Pichia stipitis enables high levels of xylitol production by engineered Escherichia coli growing on xylose. Metab. Eng. 11, 48–55. doi: 10.1016/j.ymben.2008.07.006
American Association of Poison Control Centers (AAPCC) (2021). Available online at: https://www.aapcc.org/ (accessed November 20, 2021).
Arcaño, Y. D., García, O. D., Mandelli, D., Carvalho, W. A., and Pontes, L. A. (2020). Xylitol: A review on the progress and challenges of its production by chemical route. Catal. Today. 344, 2–14. doi: 10.1016/j.cattod.2018.07.060
Ariyan, M., and Uthandi, S (2019). Xylitol Production by Xylose Reductase over producing Recombinant Escherichia coli M15. Madras Agric. J. 106, 247. doi: 10.29321/MAJ.2019.000247
Audemar, M., Ramdani, W., Junhui, T., Raluca Ifrim, A., Ungureanu, A., Jérôme, F., et al. (2020). Selective hydrogenation of xylose to xylitol over Co/SiO2 catalysts. ChemCatChem. 12, 1973-1978. doi: 10.1002/cctc.201901981
Baptista, S. L., Carvalho, L. C., Romaní, A., and Domingues, L. (2020). Development of a sustainable bioprocess based on green technologies for xylitol production from corn cob. Ind. Crops. Prod. 156:112867. doi: 10.1016/j.indcrop.2020.112867
Baptista, S. L., Cunha, J. T., Romaní, A., and Domingues, L. (2018). Xylitol production from lignocellulosic whole slurry corn cob by engineered industrial Saccharomyces cerevisiae PE-2. Bioresour. Technol. 267, 481–491. doi: 10.1016/j.biortech.2018.07.068
Barathikannan, K., and Agastian, P. (2016). “Xylitol: Production, optimization and industrial application.” Int. J. Curr. Microbiol. Appl. Sci. 5, 324–339. doi: 10.20546/ijcmas.2016.509.036
Baudel, H. M., Abreu, C. D., and Zaror, C. Z. (2005). Xylitol production via catalytic hydrogenation of sugarcane bagasse dissolving pulp liquid effluents over Ru/C catalyst. J. Chem. Technol. Biotechnol. 80, 230–233. doi: 10.1002/jctb.1155
Behera, S., Arora, R., Nandhagopal, N., and Kumar, S. (2014). Importance of chemical pretreatment for bioconversion of lignocellulosic biomass. Renew. Sustain. Energy Rev. 36, 91–106. doi: 10.1016/j.rser.2014.04.047
Benahmed, A. G., Gasmi, A., Arshad, M., Shanaida, M., Lysiuk, R., Peana, M., et al. (2020). Health benefits of xylitol. Appl. Microbiol. Biotechnol. 104, 7225–7237. doi: 10.1007/s00253-020-10708-7
Bhattacharya, A., Ganguly, A., Sadhukhan, A. K., and Chatterjee, P. K. (2018). Investigations on the effect of driving parameters for xylitol production from water hyacinth biomass. Indian J. Biotechnol. 17, 272–283. doi: 10.7904/2068-4738-VII(14)-13
Bianchini, I. D., Sene, L., da Cunha, M. A., and Felipe, M. D. (2021). Short-term Adaptation Strategy Improved Xylitol Production by Candida guilliermondii on Sugarcane Bagasse Hemicellulosic Hydrolysate. Bioenergy Res. 17, 1–3. doi: 10.1007/s12155-021-10324-x
Canilha, L., Carvalho, W., Felipe, M. D. G. A., de Almeida e Silva, J. B. (2008b). Xylitol production from wheat straw hemicellulosic hydrolysate: hydrolysate detoxification and carbon source used for inoculum preparation. Braz. J. Microbiol. 39, 333–336. doi: 10.1590/S1517-83822008000200025
Canilha, L., Carvalho, W., Giulietti, M., Felipe, M. D. G. A., de Almeida e Silva, J. B. (2008a). Clarification of a wheat straw-derived medium with ion-exchange resins for xylitol crystallization. J. Chem. Technol. Biotechnol. 83, 715–721. doi: 10.1002/jctb.1861
Carneiro, C. V., and Almeida, J. R. (2019). Xylitol production: identification and comparison of new producing yeasts. Microorganisms. 7, 484. doi: 10.3390/microorganisms7110484
Chandel, A. K., Antunes, F. A., Terán-Hilares, R., Cota, J., Ellil,ä, S., Silveira, M. H., et al. (2018). “Bioconversion of hemicellulose into ethanol and value-added products: commercialization, trends, and future opportunities,” in Advances in sugarcane biorefinery, eds. A. K. Chandel and M. H. Silveira (Elsevier). 97–134.
Chen, H. (2015). “Lignocellulose biorefinery product engineering,” in Lignocellulose Biorefinery Engineering, ed. H. Chan (Cambridge; Woodhead Publishing Limited), 125–165.
Chen, X., Jiang, Z. H., Chen, S., and Qin, W. (2010). Microbial and bioconversion production of D-xylitol and its detection and application. Int. J. Biol. Sci. 6, 834–844. doi: 10.7150/ijbs.6.834
Cheng, H., Lv, J., Wang, H., Wang, B., Li, Z., and Deng, Z. (2014). Genetically engineered Pichia pastoris yeast for conversion of glucose to xylitol by a single-fermentation process. Appl. Microbiol. Biotechnol. 98, 3539–3552. doi: 10.1007/s00253-013-5501-x
Chin, J. W., and Cirino, P. C. (2011). Improved NADPH supply for xylitol production by engineered Escherichia coli with glycolytic mutations. Biotechnol. Prog. 27, 333–341. doi: 10.1002/btpr.559
Cirino, P. C., Chin, J. W., and Ingram, L. O. (2006). Engineering Escherichia coli for xylitol production from glucose-xylose mixtures. Biotechnol. Bioeng. 95, 1167–1176. doi: 10.1002/bit.21082
Dahiya, J. S. (1991). Xylitol production by Petromyces albertensis grown on medium containing D-xylose. Can. J. Microbiol. 37, 14–18. doi: 10.1139/m91-003
Dalli, S. S., Da Silva, S. S., Uprety, B. K., and Rakshit, S. K. (2017a). Enhanced production of xylitol from poplar wood hydrolysates through a sustainable process using immobilized new strain Candida tropicalis UFMG BX 12-a. Appl. Biochem. Biotechnol. 182, 1053–1064. doi: 10.1007/s12010-016-2381-4
Dalli, S. S., Patel, M., and Rakshit, S. K. (2017b). Development and evaluation of poplar hemicellulose prehydrolysate upstream processes for the enhanced fermentative production of xylitol. Biomass. Bioenergy. 105, 402–410. doi: 10.1016/j.biombioe.2017.08.001
Dasgupta, D., Sidana, A., Ghosh, P., Sharma, T., Singh, J., Prabhune, A., et al. (2021). Energy and life cycle impact assessment for xylitol production from corncob. J. Clean. Prod. 278, 123217. doi: 10.1016/j.jclepro.2020.123217
de Albuquerque, T. L., da Silva Jr, I. J., de Macedo, G. R., and Rocha, M. V. (2014). Biotechnological production of xylitol from lignocellulosic wastes: a review. Process Biochem. 49, 1779–1789. doi: 10.1016/j.procbio.2014.07.010
de Albuquerque, T. L., Gomes, S. D., Marques, J. E. Jr„ da Silva, I. J. Jr., and Rocha, M. V. (2015). Xylitol production from cashew apple bagasse by Kluyveromyces marxianus CCA510. Catal. Today. 255, 33–40. doi: 10.1016/j.cattod.2014.10.054
de Freitas Branco, R., Chandel, A. K., and da Silva, S. S. (2012). “Enzymatic production of xylitol: current status and future perspectives,” in D-Xylitol, eds. S. S. Da Silva and A. K. Chandel (Berlin: Springer), 193–204.
de Freitas Branco, R., dos Santos, J. C., and da Silva, S. S. (2011). A novel use for sugarcane bagasse hemicellulosic fraction: xylitol enzymatic production. Biomass. Bioenergy. 35, 3241–3246. doi: 10.1016/j.biombioe.2011.02.014
El-Baz, A. F., Shetaia, Y. M., and Elkhouli, R. R. (2011). Xylitol production by Candida tropicalis under different statistically optimized growth conditions. Afr. J. Biotechnol. 10, 15353–15363. doi: 10.5897/AJB10.1575
Espinoza-Acosta, J. L. (2020). Biotechnological production of xylitol from agricultural waste. Biotecnia. 22, 126–134. doi: 10.18633/biotecnia.v22i1.1160
Faneer, K. A., Rohani, R., and Mohammad, A. W. (2018). Influence of pluronic addition on polyethersulfone membrane for xylitol recovery from biomass fermentation solution. J. Clean. Prod. 171, 995–1005. doi: 10.1016/j.jclepro.2017.10.075
Faneer, K. A., Rohani, R., Mohammad, A. W., and Ba-Abbad, M. M. (2017). Evaluation of the operating parameters for the separation of xylitol from a mixed sugar solution by using a polyethersulfone nanofiltration membrane. Korean J. Chem. Eng. 34, 2944–2957. doi: 10.1007/s11814-017-0186-y
Feigal, R. J., Jensen, M, E., and Mensing, C. A. (1981). Dental caries potential of liquid medications. Pediatr. 68, 416–419. doi: 10.1542/peds.68.3.416
Felipe, M. G., Vitolo, M., Mancilha, I. M., and Silva, S. S. (1997). Environmental parameters affecting xylitol production from sugar cane bagasse hemicellulosic hydrolyzate by Candida guilliermondii. J. Ind. Microbiol. Biotechnol. 18, 251–254. doi: 10.1038/sj.jim.2900374
Food Drug Administration (2006). Food Additive Status List. Available online at: https://www.fda.gov/food/food-additives-petitions/food-additive-status-list, (accessed October 10, 2021).
Guirimand, G., Inokuma, K., Bamba, T., Matsuda, M., Morita, K., Sasaki, K., et al. (2019). Cell-surface display technology and metabolic engineering of Saccharomyces cerevisiae for enhancing xylitol production from woody biomass. Green Chem. 21, 1795–1808. doi: 10.1039/C8GC03864C
Guo, C., Zhao, C., He, P., Lu, D., Shen, A., and Jiang, N. (2006). Screening and characterization of yeasts for xylitol production. J. Appl. Microbiol. 101, 1096–1104. doi: 10.1111/j.1365-2672.2006.02994.x
Gupta, R., Gautam, S., Shukla, R., and Kuhad, R. C. (2017). Study of charcoal detoxification of acid hydrolysate from corncob and its fermentation to xylitol. J. Environ. Chem. Eng. 5, 4573–4582. doi: 10.1016/j.jece.2017.07.073
Häcker, B., Habenicht, A., Kiess, M., and Mattes, R. (1999). Xylose utilisation: cloning and characterisation of the xylose reductase from Candida tenuis. Biol. Chem. 380, 1395–1403. doi: 10.1515/BC.1999.179
Hafyan, R., Bhullar, L., Putra, Z., Bilad, M. R., Wirzal, M. D., and Nordin, N. A. (2019). “Sustainability assessment of xylitol production from empty fruit bunch,” in MATEC Web of Conferences, (EDP Sciences) p. 06018.
Handumrongkul, C., Ma, D. P., and Silva, J. L. (1998). Cloning and expression of Candida guilliermondii xylose reductase gene (xyl1) in Pichia pastoris. Appl. Microbiol. Biotechnol. 49, 399–404. doi: 10.1007/s002530051189
Hernández-Pérez, A. F., de Arruda, P. V., Sene, L., da Silva, S. S., Kumar Chandel, A., and de Almeida Felipe, M. D. (2019). Xylitol bioproduction: state-of-the-art, industrial paradigm shift, and opportunities for integrated biorefineries. Crit. Rev. Biotechnol. 39, 924–943. doi: 10.1080/07388551.2019.1640658
Hong, E., Kim, J., Rhie, S., Ha, S. J., Kim, J., and Ryu, Y. (2016). Optimization of dilute sulfuric acid pretreatment of corn stover for enhanced xylose recovery and xylitol production. Biotechnol. Bioprocess Eng. 21, 612–619. doi: 10.1007/s12257-016-0483-z
Honkala, S., Honkala, E., Tynjäl,ä, J., and Kannas, L. (1999). Use of xylitol chewing gum among Finnish schoolchildren. Acta Odontol. Scand. 57, 306–309. doi: 10.1080/000163599428526
International Market Analysis Research Consulting Group (IMARC) (2020). Xylitol Market: Global Industry Trends, Share, Size, Growth, Opportunity and Forecast 2021-2026. Available online at: https://www.imarcgroup.com/xylitol-market (accessed October 16, 2021).
Islam, M. S., and Indrajit, M. (2012). Effects of xylitol on blood glucose, glucose tolerance, serum insulin and lipid profile in a type 2 diabetes model of rats. Ann. Nutr. Metab. 61, 57–64. doi: 10.1159/000338440
Izumori, K., and Tuzaki, K. (1988). Production of xylitol from D-xylulose by Mycobacterium smegmatis. J. Ferment. Technol. 66, 33–36. doi: 10.1016/0385-6380(88)90126-4
Janakiram, C., Kumar, C. D., and Joseph, J. (2017). Xylitol in preventing dental caries: A systematic review and meta-analyses. J. Nat. Sci. Biol. Med. 8, 16–21. doi: 10.4103/0976-9668.198344
Janket, S. J., Jaspreet, B., Isaac, P., Leland, K. A., and Jukka, H. M. (2019). Oral and systemic effects of xylitol consumption. Caries Res. 53, 491–501. doi: 10.1159/000499194
Jeon, W. Y., Yoon, B. H., Ko, B. S., Shim, W., Y., and Kim, J. H. (2012). Xylitol production is increased by expression of codon-optimized Neurospora crassa xylose reductase gene in Candida tropicalis. Bioprocess Biosyst. Eng. 35, 191–198. doi: 10.1007/s00449-011-0618-8
Johan, H., Walfridsson, M., Airaksinen, U., Ojamo, H., Hägerdal, B. H., et al. (1991). Xylitol production by recombinant Saccharomyces cerevisiae. Bio/technology 9, 1090–1095. doi: 10.1038/nbt1191-1090
Junior, J. E., and Rocha, M. V. (2021). Development of a purification process via crystallization of xylitol produced for bioprocess using a hemicellulosic hydrolysate from the cashew apple bagasse as feedstock. Bioprocess Biosyst. Eng. 44, 713–725. doi: 10.1007/s00449-020-02480-9
Junior, W. G. M., Pacheco, T. F., Trichez, D., Almeida, J. R., and Gonçalves, S. B. (2019). Xylitol production on sugarcane biomass hydrolysate by newly identified Candida tropicalis JA2 strain. Yeast. 36, 349–361. doi: 10.1002/yea.3394
Kalhorinia, S., Goli, J. K., Yadav, K. S., Naseeruddin, S., and Rao, L. V. (2014). Xylitol production from water hyacinth (Eichhornia crassipes) by Candida tropicalis Y-27405. Biosci. Biotechnol. Res. Asia 11, 427–434. doi: 10.13005/bbra/1291
Khankal, R., Chin, J. W., and Cirino, P. C. (2008). Role of xylose transporters in xylitol production from engineered Escherichia coli. J. Biotechnol. 134, 246–252. doi: 10.1016/j.jbiotec.2008.02.003
Kim, S., Lee, J., and Sung, B. H. (2019). Isolation and characterization of the stress-tolerant Candida tropicalis YHJ1 and evaluation of its xylose reductase for xylitol production from acid pre-treatment wastewater. Front. Bioeng. Biotechnol. 7:138. doi: 10.3389/fbioe.2019.00138
Kim, S. M., Choi, B. Y., Ryu, Y. S., Jung, S. H., Park, J. M., Kim, G. H., et al. (2015). Simultaneous utilization of glucose and xylose via novel mechanisms in engineered Escherichia coli. Metab. Eng. 30, 141–148. doi: 10.1016/j.ymben.2015.05.002
Kitpreechavanich, V., Hayashi, M., Nishio, N., and Nagai, S. (1984). Conversion of D-xylose into xylitol by xylose reductase from Candida pelliculosa coupled with the oxidoreductase system of methanogen strain HU. Biotechnol. Lett. 6, 651–656. doi: 10.1007/BF00133831
Kogje, A. B., and Ghosalkar, A. (2017). Xylitol production by genetically modified industrial strain of Saccharomyces cerevisiae using glycerol as co-substrate. J. Ind. Microbiol. Biotechnol. 44, 961–971. doi: 10.1007/s10295-017-1914-3
Kumar, A. K., and Sharma, S. (2017). Recent updates on different methods of pretreatment of lignocellulosic feedstocks: a review. Bioresour. Bioprocess. 4, 1–9. doi: 10.1186/s40643-017-0137-9
Kumar, P., Barrett, D. M., Delwiche, M. J., and Stroeve, P. (2009). Methods for pretreatment of lignocellulosic biomass for efficient hydrolysis and biofuel production. Ind. Eng. Chem. Res. 48, 3713–3729. doi: 10.1021/ie801542g
Kumar, S., Dheeran, P., Singh, S. P., Mishra, I. M., and Adhikari, D. K. (2015). Bioprocessing of bagasse hydrolysate for ethanol and xylitol production using thermotolerant yeast. Bioprocess Biosyst. Eng. 38, 39–47. doi: 10.1007/s00449-014-1241-2
Kumar, V., Krishania, M., Sandhu, P. P., Ahluwalia, V., Gnansounou, E., and Sangwan, R. S. (2018). Efficient detoxification of corn cob hydrolysate with ion-exchange resins for enhanced xylitol production by Candida tropicalis MTCC 6192. Bioresour. Technol. 251,416–419. doi: 10.1016/j.biortech.2017.11.039
Kumar, V., Sandhu, P. P., Ahluwalia, V., Mishra, B. B., and Yadav, S. K. (2019). Improved upstream processing for detoxification and recovery of xylitol produced from corncob. Bioresour. Technol. 291, 121931. doi: 10.1016/j.biortech.2019.121931
Kuyper, M., Hartog, M. M. P., Toirkens, M. J., Almering, M. J. H., Winkler, A. A., Dijken, J. P. V., et al. (2005). Metabolic engineering of a xylose-isomerase-expressing Saccharomyces cerevisiae strain for rapid anaerobic xylose fermentation. FEMS Yeast Res. 5, 399–409. doi: 10.1016/j.femsyr.2004.09.010
Kwon, S. G., Park, S. W., and Oh, D. K. (2006). Increase of xylitol productivity by cell-recycle fermentation of Candida tropicalis using submerged membrane bioreactor. J. Biosci. Bioeng. 101, 13–18. doi: 10.1263/jbb.101.13
Lee, J. K., Koo, B. S., and Kim, S. Y. (2003). Cloning and characterization of the xyl1 gene, encoding an NADH-preferring xylose reductase from Candida parapsilosis, and its functional expression in Candida tropicalis. Appl. Environ. Microbiol. 69, 6179–6188. doi: 10.1128/AEM.69.10.6179-6188.2003
Li, M., Meng, X., Diao, E., and Du, F. (2012). Xylitol production by Candida tropicalis from corn cob hemicellulose hydrolysate in a two-stage fed-batch fermentation process. J. Chem. Technol. Biotechnol. 87, 387–392. doi: 10.1002/jctb.2732
Liu, Y., Zheng, J., Xiao, J., He, X., Zhang, K., Yuan, S., et al. (2019). Enhanced enzymatic hydrolysis and lignin extraction of wheat straw by triethylbenzyl ammonium chloride/lactic acid-based deep eutectic solvent pretreatment. ACS omega. 4,19829–19839. doi: 10.1021/acsomega.9b02709
Llano, T., Rueda, C., Dosal, E., Andrés, A., and Coz, A. (2021). Multi-criteria analysis of detoxification alternatives: Techno-economic and socio-environmental assessment. Biomass. Bioenergy. 154, 106274. doi: 10.1016/j.biombioe.2021.106274
López-Linares, J. C., Romero, I., Cara, C., Castro, E., and Mussatto, S. I. (2018). Xylitol production by Debaryomyces hansenii and Candida guilliermondii from rapeseed straw hemicellulosic hydrolysate. Bioresour. Technol. 247, 736–743. doi: 10.1016/j.biortech.2017.09.139
López-Linares, J. C., Ruiz, E., Romero, I., Castro, E., and Manzanares, P. (2020). Xylitol production from exhausted olive pomace by Candida boidinii. Appl. Sci. 10, 6966. doi: 10.3390/app10196966
Lugani, Y., Puri, M., and Sooch, B. S. (2021). Recent insights, applications and prospects of xylose reductase: a futuristic enzyme for xylitol production. Eur. Food Res. Technol. 247, 921–946. doi: 10.1007/s00217-020-03674-x
Lugani, Y., and Sooch, B. S. (2020). Fermentative production of xylitol from a newly isolated xylose reductase producing Pseudomonas putida BSX-46. LWT. 134, 109988. doi: 10.1016/j.lwt.2020.109988
Mäkinen, K. K. (2000). The rocky road of xylitol to its clinical application. J. Dent. Res. 79, 1352–1355. doi: 10.1177/00220345000790060101
Manaf, S. F., Jahim, J. M., Harun, S., and Luthfi, A. A. (2018). Fractionation of oil palm fronds (OPF) hemicellulose using dilute nitric acid for fermentative production of xylitol. Ind. Crops. Prod. 115, 6–15. doi: 10.1016/j.indcrop.2018.01.067
Mardawati, E., Andoyo, R., Syukra, K. A., Kresnowati, M. T., and Bindar, Y. (2018). “Production of xylitol from corn cob hydrolysate through acid and enzymatic hydrolysis by yeast,” in IOP Conference Series: Earth and Environmental Science 1 (Bogor: IOP Publishing). 012019. doi: 10.1088/1755-1315/141/1/012019
Mardawati, E., Maharani, N., Wira, D. W., Harahap, B. M., Yuliana, T., and Sukarminah, E. (2020). xylitol production from oil palm empty fruit bunches (OPEFB) via simultaneous enzymatic hydrolysis and fermentation process. J. Ind. Inf. Technol. Agricult. 2, 25064. doi: 10.24198/jiita.v2i1.25064
Martin, J. F., Sánchez, S., and Cuevas, M. (2013). Evaluation of the effect of the dilute acid hydrolysis on sugars release from olive prunings. Renew. Energy. 51, 382–387 doi: 10.1016/j.renene.2012.10.002
Martínez, E. A., Canettieri, E. V., Bispo, J. A., Giulietti, M., De Almeida e Silva, J. B., and Converti, A. (2015). Strategies for xylitol purification and crystallization: a review. Sep. Sci. Technol. 50, 2087–2098. doi: 10.1080/01496395.2015.1009115
Martínez, E. A., e Silva, J. B., Giulietti, M., and Solenzal, A. I. (2007). Downstream process for xylitol produced from fermented hydrolysate. Enzyme Microb. Technol. 40, 1193–1198. doi: 10.1016/j.enzmictec.2006.09.003
Martinez, E. A., Silva, S. S., e Silva, J. B., Solenzal, A. I., and Felipe, M. G. (2003). The influence of pH and dilution rate on continuous production of xylitol from sugarcane bagasse hemicellulosic hydrolysate by C. guilliermondii. Process Biochem. 38, 1677–1683. doi: 10.1016/S0032-9592(02)00244-3
Martínez, E. A., Silva, S. S., and Felipe, M. G. (2000). Effect of the oxygen transfer coefficient on xylitol production from sugarcane bagasse hydrolysate by continuous stirred-tank reactor fermentation. Appl. Biochem. Biotechnol. 84, 633–641. doi: 10.1385/ABAB:84-86:1-9:633
Mathew, A. K., Abraham, A., Mallapureddy, K. K., and Sukumaran, R. K. (2018). “Lignocellulosic biorefinery wastes, or resources,” in Waste Biorefinery, eds. T. Bhaskar, A. Pandey, S. V. Mohan, D. J. Lee. (Elsevier), 267–297.
Medina, J. D., Woiciechowski, A. L., Zandona Filho, A., Brar, S. K., Magalhaes Junior, A. I., and Soccol, C. R. (2018). Energetic and economic analysis of ethanol, xylitol and lignin production using oil palm empty fruit bunches from a Brazilian factory. J. Clean. Prod. 195, 44–55. doi: 10.1016/j.jclepro.2018.05.189
Mishra, D. K., Dabbawala, A. A., and Hwang, J. S. (2013). Ruthenium nanoparticles supported on zeolite Y as an efficient catalyst for selective hydrogenation of xylose to xylitol. J. Mol. Catal. A. Chem. 376, 63–70. doi: 10.1016/j.molcata.2013.04.011
Misra, S., Gupta, P., Raghuwanshi, S., Dutt, K., and Saxena, R. K. (2011). Comparative study on different strategies involved for xylitol purification from culture media fermented by Candida tropicalis. Sep. Sci. Technol. 78, 266–273. doi: 10.1016/j.seppur.2011.02.018
Moraes, E. D. J. C., Silva, D. D. V., Dussán, K. J., Tesche, L. Z., de Almeida Silva, J. B., Rai, M., et al. (2020). Xylitol-sweetener production from barley straw: optimization of acid hydrolysis condition with the energy consumption simulation. Waste Biomass Valorization. 11, 1837–1849. doi: 10.1007/s12649-018-0501-9
Morales-Rodriguez, R., Perez-Cisneros, E. S., Jose, A., and Rodriguez-Gomez, D. (2016). Evaluation of biorefinery configurations through a dynamic model-based platform: Integrated operation for bioethanol and xylitol co-production from lignocellulose. Renew. Energy. 89, 135–143. doi: 10.1016/j.renene.2015.12.019
Mussatto, S. I. (2012). “Application of xylitol in food formulations and benefits for health,” in D-Xylitol, eds. S. S. Da Silva and A. K. Chandel (Berlin: Springer), 309–323.
Mussatto, S. I., and Roberto, I. C. (2003). Xylitol production from high xylose concentration: evaluation of the fermentation in bioreactor under different stirring rates. J. Appl. Microbiol. 95, 331–337. doi: 10.1046/j.1365-2672.2003.01990.x
Mussatto, S. I., and Roberto, I. C. (2008). Establishment of the optimum initial xylose concentration and nutritional supplementation of brewer's spent grain hydrolysate for xylitol production by Candida guilliermondii. Process Biochem. 43, 540–546. doi: 10.1016/j.procbio.2008.01.013
Mussatto, S. I., and Teixeira, J. A. (2010). “Lignocellulose as raw material in fermentation processes,” in Current Research, Technology and Education Topics in Applied Microbiology and Microbial Biotechnology, ed. Méndez-Vilas, A., (Formatex Research Center), 897–907.
Naidu, D. S., Hlangothi, S. P., and John, M. J. (2018). Bio-based products from xylan: A review. Carbohydr. Polym. 179, 28–41. doi: 10.1016/j.carbpol.2017.09.064
Nair, N. U., and Zhao, H. (2010). Selective reduction of xylose to xylitol from a mixture of hemicellulosic sugars. Metab. Eng. 12, 462–468. doi: 10.1016/j.ymben.2010.04.005
Narisetty, V., Cox, R., Bommareddy, R. R., Agrawal, D., Ahmad, E., Pant, K. K., et al. (2022). Valorisation of xylose to renewable fuels and chemicals, an essential step in augmenting the commercial viability of lignocellulosic biorefineries. Sustain. Energy Fuels. 6, 29–65. doi: 10.1039/D1SE00927C
Nayak, P. A., Nayak, U. A, and Khandelwal, V. (2014). The effect of xylitol on dental caries and oral flora. Clin. Cosmet. Investig. Dent. 6, 89–94. doi: 10.2147/CCIDE.S55761
Nyyssöl,ä, A., Pihlajaniemi, A., Palva, A., Weymarn, N. V, and Leisola, M. (2005). Production of xylitol from D-xylose by recombinant Lactococcus lactis. J. Biotechnol. 118, 55–66. doi: 10.1016/j.jbiotec.2005.03.014
Oh, E. J., Ha, S. J., Kim, S. R., Lee, W. H., Galazka, J. M., Cate, J. H. D., et al. (2013). Enhanced xylitol production through simultaneous co-utilization of cellobiose and xylose by engineered Saccharomyces cerevisiae. Metab. Eng. 15, 226–234. doi: 10.1016/j.ymben.2012.09.003
Onishi, H., and Suzuki, T. (1969). Microbial production of xylitol from glucose. Appl. Microbiol. 18, 1031–1035. doi: 10.1128/am.18.6.1031-1035.1969
Patel, V., Desai, M., and Madamwar, D. (1993). Thermochemical pretreatment of water hyacinth for improved biomethanation. Appl. Biochem. Biotechnol. 42, 67–74. doi: 10.1007/BF02788902
Pérez-Bibbins, B., Torrado-Agrasar, A., Salgado, J. M., Mussatto, S. I., and Domínguez, J. M. (2015). Xylitol production in immobilized cultures: a recent review. Crit. Rev. Biotechnol. 36, 691–704. doi: 10.3109/07388551.2015.1004660
Peterson, M. E. (2013). Xylitol. Topics in Compan. An Med. 28, 18–20. doi: 10.1053/j.tcam.2013.03.008
Ping, Y., Ling, H., Song, G., and Ge, J. (2013). Xylitol production from non-detoxified corncob hemicellulose acid hydrolysate by Candida tropicalis. Biochem Eng J. 75, 86–91. doi: 10.1016/j.bej.2013.03.022
Povelainen, M., and Miasnikov, A. N. (2007). Production of xylitol by metabolically engineered strains of Bacillus subtilis. J. Biotechnol. 128, 24–31. doi: 10.1016/j.jbiotec.2006.09.008
Prabhu, A. A., Bosakornranut, E., Amraoui, Y., Agrawal, D., Coulon, F., Vivekanand, V., et al. (2020a). Enhanced xylitol production using non-detoxified xylose rich pre-hydrolysate from sugarcane bagasse by newly isolated Pichia fermentans. Biotechnol. Biofuels. 13, 1–5. doi: 10.1186/s13068-020-01845-2
Prabhu, A. A., Thomas, D. J., Ledesma-Amaro, R., Leeke, G. A., Medina, A., Verheecke-Vaessen, C., et al. (2020b). Biovalorisation of crude glycerol and xylose into xylitol by oleaginous yeast Yarrowia lipolytica. Microb. Cell Factories. 19, 1–8. doi: 10.1186/s12934-020-01378-1
Pratter, S. M., Eixelsberger, T., and Nidetzky, B. (2015). Systematic strain construction and process development: Xylitol production by Saccharomyces cerevisiae expressing Candida tenuis xylose reductase in wild-type or mutant form. Bioresour. Technol. 198, 732–738. doi: 10.1016/j.biortech.2015.09.046
Qi, X., Wang, X., Lin, J., Zhu, J., Luo, Y., Deng, W., et al. (2014). Microbial and enzymatic process for xylitol production from D-glucose. Curr. Org. Chem. 18, 3131–3135. doi: 10.2174/138527281824150119095755
Rafiqul, I. S., Mimi Sakinah, A. M., and Zularisam, A. W. (2021). Improvement of enzymatic bioxylitol production from sawdust hemicellulose: optimization of parameters. Prep. Biochem. Biotechnol. 2, 1–11. doi: 10.1080/10826068.2021.1897840
Rafiqul, I. S., and Sakinah, A. M. (2013). Processes for the production of xylitol—a review. Food Rev. Int. 29, 127–156. doi: 10.1080/87559129.2012.714434
Rafiqul, I. S., and Sakinah, A. M. (2015). Biochemical properties of xylose reductase prepared from adapted strain of Candida tropicalis. Appl. Biochem. Biotechnol. 175, 387–399. doi: 10.1007/s12010-014-1269-4
Rafiqul, I. S., Sakinah, A. M., and Zularisam, A. W. (2015a). Enzymatic production of bioxylitol from sawdust hydrolysate: screening of process parameters. Appl. Biochem. Biotechnol. 176, 1071–1083. doi: 10.1007/s12010-015-1630-2
Rafiqul, I. S., Sakinah, A. M., and Zularisam, A. W. (2015b). Inhibition by toxic compounds in the hemicellulosic hydrolysates on the activity of xylose reductase from Candida tropicalis. Biotechnol. Lett. 37, 191–196. doi: 10.1007/s10529-014-1672-5
Ramirez, O. S., and Escoto, H. H. (2021). Enhancement of xylitol production by fed-batch policy designed through stochastic search. Chem. Eng. Trans. 86, 1009–1014.
Rangaswamy, S., and Agblevor, F. A. (2002). Screening of facultative anaerobic bacteria utilizing D-xylose for xylitol production. Appl. Microbiol. Biotechnol. 60, 88–93. doi: 10.1007/s00253-002-1067-8
Rao, L. V., Goli, J. K., Gentela, J., and Koti, S. (2016). Bioconversion of lignocellulosic biomass to xylitol: an overview. Bioresour. Technol. 213, 299–310. doi: 10.1016/j.biortech.2016.04.092
Ravella, S. R., Gallagher, J., Fish, S., and Prakasham, R. S. (2012). “Overview on commercial production of xylitol, economic analysis and market trends,” in D-Xylitol, eds. S. S. Da Silva and A. K. Chandel (Berlin, Springer), 291–306.
Rehman, S., Murtaza, M. A., and Mushtaq, Z. (2016). “Xylitol as sweetener,” in Sweeteners, Reference Series in Phytochemistry, eds J. M. Merillon and K. Ramawat (Cham: Springer), 1–21. doi: 10.1007/978-3-319-26478-3_30-1
Rehman, S. U., Mushtaq, Z., Zahoor, T., Jamil, A., and Murtaza, M. A. (2013). Xylitol: A review on bioproduction, application, health benefits, and related safety issues. Crit. Rev. Food Sci. Nutr. 55, 1514–1528. doi: 10.1080/10408398.2012.702288
Rehman, S. U., Mushtaq, Z., Zahoor, T., Jamil, A., and Murtaza, M. A. (2015). Xylitol: a review on bioproduction, application, health benefits, and related safety issues. Crit. Rev. Food Sci. Nutr. 55, 1514–1528. doi: 10.1080/1408398.2012.702288
Rizzi, M., Harwart, K., Erlemann, P., Bui-Thanh, N. A., and Dellweg, H. (1989). Purification and properties of the NAD+-xylitol-dehydrogenase from the yeast Pichia stipitis. J. Ferment. Bioeng. 67, 20–24. doi: 10.1016/0922-338X(89)90080-9
Rodrigues, R. C. L. B.Kenealy, W. R., et al. (2011). Xylitol production from DEO hydrolysate of corn stover by Pichia stipitis YS-30. J Ind Microbiol Biotechnol. 38, 1649–1655. doi: 10.1007/s10295-011-0953-4
Rudrangi, S. S., and West, T. P. (2020). Effect of pH on xylitol production by Candida species from a prairie cordgrass hydrolysate. Z. Naturforsch. C. 75, 489–493. doi: 10.1515/znc-2020-0140
Salgado, J. M., Rodríguez, N., Cortés, S., and Domínguez, J. M. (2012). Coupling two sizes of CSTR-type bioreactors for sequential lactic acid and xylitol production from hemicellulosic hydrolysates of vineshoot trimmings. N. Biotechnol. 29, 421–427. doi: 10.1016/j.nbt.2011.07.003
Sampaio, F. C., Chaves-Alves, V. M., Converti, A., Passos, F. M., and Coelho, J. L. (2008). Influence of cultivation conditions on xylose-to-xylitol bioconversion by a new isolate of Debaryomyces hansenii. Bioresour. Technol. 99, 502–508. doi: 10.1016/j.biortech.2007.01.017
Sampaio, F. C., De Moraes, C. A., De Faveri, D., Perego, P., Converti, A., and Passos, F. M. (2006a). Influence of temperature and pH on xylitol production from xylose by Debaryomyces hansenii UFV-170. Process Biochem. 41, 675–681. doi: 10.1016/j.procbio.2005.08.019
Sampaio, F. C., Passos, F. M., Passos, F. J., De Faveri, D., Perego, P., and Converti, A. (2006b). Xylitol crystallization from culture media fermented by yeasts. Chem. Eng. Process.: Process Intensif. 45, 1041–1046 doi: 10.1016/j.cep.2006.03.012
Sampaio, F. C., Silveira, W. B., Chaves-Alves, V. M., Passos, F. M., and Coelho, J. L. (2003). Screening of filamentous fungi for production of xylitol from D-xylose. Brazilian J. Microbiol. 34, 325–328. doi: 10.1590/S1517-83822003000400007
Santana, N. B., Dias, J. C., Rezende, R. P., Franco, M., Oliveira, L. K., and Souza, L. O. (2018). Production of xylitol and bio-detoxification of cocoa pod husk hemicellulose hydrolysate by Candida boidinii XM02G. PLoS ONE. 13, e0195206 doi: 10.1371/journal.pone.0195206
Sasaki, M., Jojima, T., Inui, M., and Yukawa, H. (2010). Xylitol production by recombinant Corynebacterium glutamicum under oxygen deprivation. Appl. Microbiol. Biotechnol. 86, 1057–1066. doi: 10.1007/s00253-009-2372-2
Shah, S. S., Luthfi, A. A., Jahim, J. M., Harun, S., and Low, K. O. (2020). An improvement in fermentability of acid-hydrolysed hemicellulose from kenaf stem for xylitol production. Int. J. Food Eng. 9, 4080. doi: 10.1515/ijfe-2019-0230
Shah, S. S., Luthfi, A. A., Low, K. O., Harun, S., Manaf, S. F., Illias, R. M., et al. (2019). Preparation of kenaf stem hemicellulosic hydrolysate and its fermentability in microbial production of xylitol by Escherichia coli BL21. Sci. Rep. 9, 1–3. doi: 10.1038/s41598-019-40807-z
Silva, D. D., Dussán, K. J., Idarraga, A., Grangeiro, L., Silva, S. S., Cardona, C. A., et al. (2020). Production and purification of xylitol by Scheffersomyces amazonenses via sugarcane hemicellulosic hydrolysate. Biofuel. Bioprod. Biorefin. 14, 344–356. doi: 10.1002/bbb.2085
Sindhu, R., Binod, P., Pandey, A., Madhavan, A., Alphonsa, J. A., Vivek, N., et al. (2017). Water hyacinth a potential source for value addition: an overview. Bioresour. technol. 230, 152–162. doi: 10.1016/j.biortech.2017.01.035
Soares, L. C., Chandel, A. K., Pagnocca, F. C., Gaikwad, S. C., Rai, M., and da Silva, S. S. (2016). Screening of yeasts for selection of potential strains and their utilization for in situ microbial detoxification (ISMD) of sugarcane bagasse hemicellulosic hydrolysate. Indian J. Microbiol. 56, 172–181. doi: 10.1007/s12088-016-0573-9
Su, B., Wu, M., Zhang, Z., Lin, J., and Yang, L. (2015). Efficient production of xylitol from hemicellulosic hydrolysate using engineered Escherichia coli. Metab. Eng. 31, 112–122. doi: 10.1016/j.ymben.2015.07.003
Tada, K., Horiuchi, J. I., Kanno, T., and Kobayashi, M. (2004). Microbial xylitol production from corn cobs using Candida magnoliae. J. Biosci. Bioeng. 98, 228–230. doi: 10.1016/S1389-1723(04)00273-7
Tamburini, E., Costa, S., Marchetti, M. G., and Pedrini, P. (2015). Optimized production of xylitol from xylose using a hyper-acidophilic Candida tropicalis. Biomolecules. 5, 1979–1989. doi: 10.3390/biom5031979
Tiefenbacher, F, . (ed.). (2017). “Technology of Main Ingredients—Sweeteners and Lipids,” in The Technology of Wafers and Waffles: Operational Aspects, (Academic Press), 123–225. doi: 10.1016/B978-0-12-809438-9.00003-X
Tomotani, E. J., Arruda, P. V., Vitolo, M., and Felipe, M. D. (2009). Obtaining partial purified xylose reductase from Candida guilliermondii. Braz. J. Microbiol. 40, 631–635. doi: 10.1590/S1517-83822009000300027
Traff, K. L., Cordero, R. R. O., Zyl, W., H, V., and Hagerdal, B. H. (2001). Deletion of the GRE3 aldose reductase gene and its influence on xylose metabolism in recombinant strains of Saccharomyces cerevisiae expressing the xylA and XKS1 genes. Appl. Environ. Microbiol. 67, 5668–5674. doi: 10.1128/AEM.67.12.5668-5674.2001
Uhari, M., Kontiokari, T., Koskela, M., and Niemela, M. (1996). Xylitol chewing gum in prevention of acute otitis media: double blind randomised trial. BMJ. 313, 1180–1183. doi: 10.1136/bmj.313.7066.1180
Valdes, G., Mendonça, R. T., and Aggelis, G. (2020). Lignocellulosic biomass as a substrate for oleaginous microorganisms: a review. Appl. Sci. 10, 7698. doi: 10.3390/app10217698
Varanasi, J. L., Kumari, S., and Das, D. (2018). Improvement of energy recovery from water hyacinth by using integrated system. Int. J. Hydrog. Energy 43, 1303–1318. doi: 10.1016/j.ijhydene.2017.11.110
Verduyn, C., Van Kleef, R., Frank, J., Schreuder, H., Van Dijken, J. P., and Scheffers, W. A. (1985). Properties of the NAD (P) H-dependent xylose reductase from the xylose-fermenting yeast Pichia stipitis. Biochem. J. 226, 669–677 doi: 10.1042/bj2260669
Vernacchio, L., Corwin, M. J., Vezina, R. M., Pelton, S. I., Feldman, H. A., Beasley, T. C., et al. (2014). Xylitol syrup for the prevention of acute otitis media. Pediatr. 133, 289–295. doi: 10.1542/peds.2013-2373
Wang, L., Shen, Y., Zhang, Y., Wei, Q., Liang, Y., Tian, H., et al. (2021). A novel surface treatment of carbon fiber with Fenton reagent oxidization for improved cells immobilization and xylitol fermentation. Microporous. Mesoporous. Mater. 325, 111318. doi: 10.1016/j.micromeso.2021.111318
Wang, W., Ling, H., and Zhao, H. (2015). Steam explosion pretreatment of corn straw on xylose recovery and xylitol production using hydrolysate without detoxification. Process Biochem. 50, 1623–1628. doi: 10.1016/j.procbio.2015.06.001
Werpy, T., and Petersen, G. (2004). Top value added chemicals from biomass: volume I–results of screening for potential candidates from sugars and synthesis gas. National Renewable Energy Lab. p. 76. doi: 10.2172/15008859
West, T. P. (2021). Xylitol Production by Candida Species from Hydrolysates of Agricultural Residues and Grasses. Ferment. 7, 243. doi: 10.3390/fermentation7040243
Winkelhausen, E., and Kuzmanova, S. (1998). Microbial conversion of D-xylose to xylitol. J. Ferment. Bioeng. 86, 1–4. doi: 10.1016/S0922-338X(98)80026-3
Xu, Y., Chi, P., Bilal, M., and Cheng, H. (2019). Biosynthetic strategies to produce xylitol: an economical venture. Appl. Microbiol. Biotechnol. 103, 5143–5160. doi: 10.1007/s00253-019-09881-1
Yablochkova, E. N., Bolotnikova, O. I., Mikhailova, N. P., Nemova, N. N., and Ginak, A. I. (2003). The activity of xylose reductase and xylitol dehydrogenase in yeasts. Microbiology. 72, 414–417. doi: 10.1023/A:1025032404238
Yadav, M., Mishra, D. K., and Hwang, J. S. (2012). Catalytic hydrogenation of xylose to xylitol using ruthenium catalyst on NiO modified TiO2 support. Appl. Catal. A: Gen. 425, 110–116. doi: 10.1016/j.apcata.2012.03.007
Yamagata, S., Goto, Y., Ohneda, A., Anzai, M., Kawashima, S., Kikuchi, J., et al. (1969). “Clinical Application of Xylitol in Diabetes”, in International Symposium on Metabolism, Physiology, and Clinical Use of Pentoses and Pentitols, (Berlin, Heidelberg: Springer), 316–325.
Yewale, T., Panchwagh, S., Rajagopalan, S., Dhamole, P. B., and Jain, R. (2016). Enhanced xylitol production using immobilized Candida tropicalis with non-detoxified corn cob hemicellulosic hydrolysate. Biotech. 6, 75. doi: 10.1007/s13205-016-0388-8
Yoshitake, J., Ishizaki, H., Shimamura, M., and Imai, T. (1973a). Xylitol production by an Enterobacter species. Agr Biol Chem. 37, 2261–2267 doi: 10.1080/00021369.1973.10861002
Yoshitake, J., Shimamura, M., and Imai, T. (1973b). Xylitol production by a Corynebacterium species. Agr Biol Chem. 37, 2251–2259. doi: 10.1080/00021369.1973.10861001
Zha, J., Li, B. Z., Shen, M. H., Hu, M. L., Song, H., and Yuan, Y. J. (2013). Optimization of CDT-1 and XYL1 expression for balanced co-production of ethanol and xylitol from cellobiose and xylose by engineered Saccharomyces cerevisiae. PLoS One. 8:e68317. doi: 10.1371/journal.pone.0068317
Zhang, J., Zhang, B., Wang, D., Gao, X., and Hong, J. (2014). Xylitol production at high temperature by engineered Kluyveromyces marxianus. Bioresour. Technol. 152, 192–201. doi: 10.1016/j.biortech.2013.10.109
Keywords: bioproduction, hemicellulose valorization, lignocellulosic biomass, xylitol, xylose
Citation: Umai D, Kayalvizhi R, Kumar V and Jacob S (2022) Xylitol: Bioproduction and Applications-A Review. Front. Sustain. 3:826190. doi: 10.3389/frsus.2022.826190
Received: 30 November 2021; Accepted: 17 January 2022;
Published: 15 February 2022.
Edited by:
Ruchi Agrawal, TERI Gram, IndiaReviewed by:
Ajar Nath Yadav, Eternal University, IndiaCopyright © 2022 Umai, Kayalvizhi, Kumar and Jacob. This is an open-access article distributed under the terms of the Creative Commons Attribution License (CC BY). The use, distribution or reproduction in other forums is permitted, provided the original author(s) and the copyright owner(s) are credited and that the original publication in this journal is cited, in accordance with accepted academic practice. No use, distribution or reproduction is permitted which does not comply with these terms.
*Correspondence: Samuel Jacob, c2FtdWVsamJAc3JtaXN0LmVkdS5pbg==
Disclaimer: All claims expressed in this article are solely those of the authors and do not necessarily represent those of their affiliated organizations, or those of the publisher, the editors and the reviewers. Any product that may be evaluated in this article or claim that may be made by its manufacturer is not guaranteed or endorsed by the publisher.
Research integrity at Frontiers
Learn more about the work of our research integrity team to safeguard the quality of each article we publish.