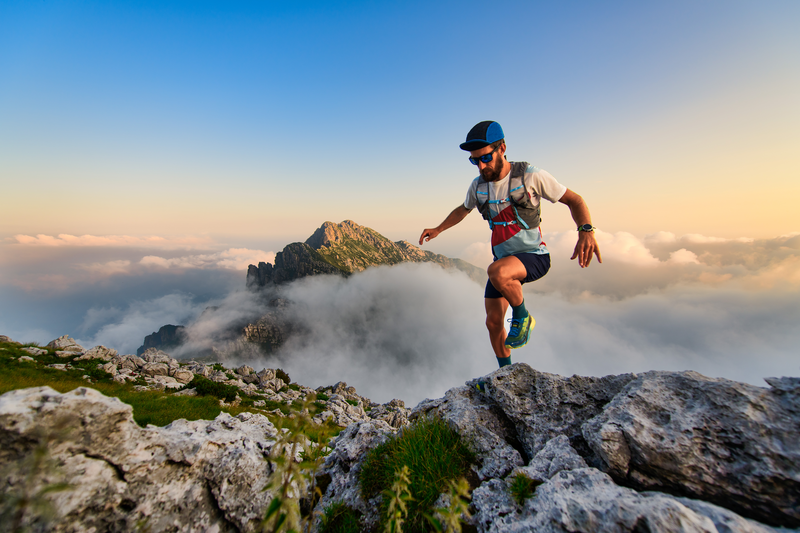
94% of researchers rate our articles as excellent or good
Learn more about the work of our research integrity team to safeguard the quality of each article we publish.
Find out more
REVIEW article
Front. Sustain. , 30 November 2022
Sec. Waste Management
Volume 3 - 2022 | https://doi.org/10.3389/frsus.2022.1007060
This article is part of the Research Topic The Sustainability Series: The Plastics Problem - Pathways towards Sustainable Solutions Against Plastic Pollution View all 13 articles
Plastic is a ubiquitous material that has caused major environmental impacts. Ecosystem damage from improperly disposed plastic waste is the most visible of these impacts; however, plastic also has less visible environmental impacts throughout its supply chain. At the same time, plastic is not unique in possessing severe, often invisible, environmental impacts that occur throughout its life cycle. Life cycle assessment (LCA) is a helpful tool can be used to contextualize the environmental impacts of plastic compared with alternative solutions or material substitutes. LCA can broaden our understanding of the environmental impacts of a product beyond what is the most obvious and visible, taking a comprehensive view that encompasses raw material extraction, manufacturing, transportation, use, and end-of-life. LCA can be used to target specific areas for improvement, understand and evaluate tradeoffs among different materials, and can be helpful to avoid environmental problem-shifting. This review provides an overview of the LCA process and describes the benefits and limitations of LCA methods as they pertain to plastic and plastic waste. This paper summarizes major trends that are observed in prior LCA studies, along with a discussion of how LCA can best be used to help resolve the plastics problem without causing other unintended issues. The life cycle perspective analyzes the environmental impact associated with a specific product, often comparing the environmental impacts of one alternative to another. An alternative perspective analyzes the aggregated environmental impacts of the entire plastic sector, analyzing the full scope and scale of plastics in the environment. Both perspectives provide meaningful data and insights, yet each provides an incomplete understanding of the plastics problem. The comparative LCA perspective and the aggregated environmental impact perspective can complement one another and lead to overall improved environmental outcomes when used in tandem. The discussion highlights that reduced consumption of the underlying need for plastic is the only way to ensure reduced environmental impacts, whereas interventions that promote material substitution and or incentivize shifts toward other kinds of consumption may result in unintended environmental consequences.
Life cycle assessment (LCA) is a tool to systematically evaluate the environmental impacts of products or systems (Vignon et al., 1992). It is often used to support design or policy decisions related to improving the sustainability of a product, to select among alternative materials, or identify consequences or tradeoffs that are not immediately obvious. Life cycle methods and datasets have evolved significantly over the past decades to improve overall data availability, robustness, and usefulness (Laurin, 2017); however, LCA practitioners widely acknowledge its imperfections and the need to continually improve the tool (Huijbregts et al., 2001; Reap et al., 2008; McKone et al., 2011; Hauschild et al., 2013; Bergerson et al., 2020).
Plastic, and plastic waste specifically, is an environmental issue that is studied by multiple intellectual communities, each with different research traditions and perspectives. Recently, there have been conversations in the scientific literature and popular press debating the extent and severity of the plastic issue. The debates include whether public perception of ecosystem risk corresponds to scientific evidence (Burton, 2017; Backhaus and Wagner, 2020; Völker et al., 2020; Catarino et al., 2021; Zhou et al., 2021), as well as the relative importance of plastic waste to other pressing environmental challenges (Stafford and Jones, 2019a; Ford et al., 2022). There have also been debates surrounding the potential effectiveness and impact of proposed solutions such as implementation of plastic bans (Lewis et al., 2010; Martinho et al., 2017; Wagner, 2017; Herberz et al., 2020; Macintosh et al., 2020; Völker et al., 2020; Meert et al., 2021; Gómez and Escobar, 2022; Huang and Woodward, 2022). Some researchers studying the impacts of plastic waste have critiqued life cycle thinking approaches for inappropriately analyzing the impact of plastic waste (Walker and McKay, 2021). While certain perspectives may never be fully resolved, this review seeks to provide insights on the usefulness and limitations of LCA to evaluate the plastic challenge and contrast the LCA perspective with an aggregated environmental perspective that focuses on the magnitude of total impact.
Specifically, this review seeks to (1) summarize major insights from the LCA community regarding the environmental impact of plastics and plastic waste; (2) discuss the limitations of LCA as it relates to plastic waste and identify where improvements can be made; and (3) discuss how different perspectives can lead to different conclusions and how to integrate these perspectives.
Plastic products are ubiquitous in society. The use of plastic has rapidly increased over the past decades due to relatively inexpensive production costs and a range of physical properties that have allowed technological advances across various industrial and product sectors (World Economic Forum, 2016). As with any material, plastics contribute to environmental impacts throughout their life cycle (APME, 2003): impacts associated with drilling for natural gas of which they are derived, manufacturing into the product or product pre-cursor, transportation, potential fugitive emissions during use, and end-of-life (Dormer et al., 2013). In addition to the emissions associated with energy use during all of these phases, plastics also contribute to resource depletion, consuming fossil fuel reserves through the conversion to plastics, even when recycling is available (Geyer et al., 2016; Zink and Geyer, 2019). Finally, plastics contribute to environmental impacts at their end-of-life, both in terms of waste management or recycling (Hou et al., 2018), or via leakage into ecosystems (Scagnetti and Lorenz, 2022).
As of 2015, plastics were responsible for 1,781 Mt CO2-eq throughout their entire life cycle, consisting of production (1,085 Mt), conversion (535 Mt) and end-of-life (161 Mt), which represents over 3% of overall global GHG emissions (Zheng and Suh, 2019). Although the majority of plastic is landfilled with a much smaller portion recycled, between 4.8 and 12.7 million Mt of plastic waste was estimated to enter the ocean from coastal countries in 2010 (Jambeck et al., 2015). In the United States, it is estimated that ~2% of plastic “leaks” into the environment, either through improper disposal or abrasion of materials during their normal use (i.e. tire wear, shedding from textiles) (Heller et al., 2020). Roughly 30% of plastics are considered durable, with an intended long-term use in construction, electrical, or consumer product use (Heller et al., 2020). Many plastics, particularly those used in the packaging sector, are designed for a single use with very short lifespans prior to end-of-life (Heller et al., 2020).
LCA is a tool that provides a framework for a comprehensive, systematic analysis of the environmental impacts of a product or process. LCA can be used to understand the full scope of impacts of a single product in its entirety or can be used to compare multiple products. It is used to evaluate all aspects of a product from raw feedstock extraction through its end-of life (i.e. cradle-to-grave) or can focus on specific portions of a supply chain, such as the environmental impacts before a product reaches the market (i.e. cradle-to-gate). Taking a holistic and systematic approach throughout the entire life cycle is critically important when trying to find solutions to specific environmental problems because there are numerous instances where the intended solution to one environmental problem caused a different kind of environmental issue (Davis and Thomas, 2006).
The major elements of an LCA are (1) goal and scope; (2) life cycle inventory; (3) life cycle impact assessment; (4) interpretation (Vignon et al., 1992). The goal and scope phase defines the research questions the assessment intends to answer, the environmental impacts that will be included, the data that will be collected, and the boundaries of the analysis. The life cycle inventory phase consists of data collection, verification, and sensitivity analysis. The life cycle impact assessment phase translates the material, energy, and emissions data compiled during the inventory phase into appropriate metrics to quantify the environmental impacts of interest. The interpretation phase discusses the overall results of the study, including helping to contextualize the results and highlighting the assumptions and limitations of the study. The phases of an LCA are often conducted iteratively to best tailor the assessment to its specific purpose.
LCA methods have become significantly more robust since their inception, with larger numbers of datasets becoming available from which to draw inventory data and increasing levels of sophistication and complexity. Nevertheless, some of the harshest critics of LCA methods are LCA practitioners themselves, with multiple formal and informal working groups seeking to improve challenges that persist in LCA's implementation (SETAC, 2001; Rosenbaum et al., 2008; Sonnemann and Valdivia, 2017; Bergerson et al., 2020). While the LCA method has specific requirements that are dictated by the International Organization for Standardization (ISO) (2006), much of the guidance within ISO allows a degree of flexibility to allow practitioners to make methodological choices that align with the goals of a particular study. This flexibility allows an LCA to be suited to a given purpose but can have a confounding effect where different LCAs of the same product produce seemingly different results. Much of the criticism levied at LCA tends to focus on one of three major issues: the boundaries of the analysis (Matthews and Small, 2000; Frijia et al., 2012; Choudhary et al., 2014; Kakadellis and Harris, 2020), data uncertainty and data quality (Huijbregts et al., 2001; SETAC, 2001; von Bahr and Steen, 2003; Lo et al., 2005; Tan et al., 2007; Hung., 2009; Mullins et al., 2011; de Kleine et al., 2014; Pernollet et al., 2017; Xue et al., 2017), and appropriately capturing the environmental impacts (Knoepfel, 1996; Notarnicola et al., 1998; Goedkoop and Spriensma, 2001; Bare et al., 2003; Rosenbaum et al., 2008; Hauschild et al., 2013; Ernstoff et al., 2019; Saling et al., 2020).
Defining the boundaries and functional unit of an LCA is one of the most critical decisions of the process and can greatly influence the overall results of a particular analysis (Deng and Williams, 2011; Frijia et al., 2012; Ng et al., 2013). While most LCA examine the full supply chain of a product “from cradle-to-grave” that include all stages of resource extraction, transportation, manufacturing, use, and disposal, there are valid reasons to truncate the analysis. For example, some LCA may focus on the “cradle-to-gate” boundary that do not include the consumer use or disposal phases in order to focus on impacts within the scope of a manufacturer's control. Similarly, different boundaries can be drawn associated with “attributional” and “consequential” impacts (Brander et al., 2009; Earles and Halog, 2011). An attributional boundary definition only includes the materials and energy that are directly associated with a given product. Meanwhile, a consequential boundary also includes estimation of indirect impacts that can result from changes that are induced by adoption of the product. In each of these cases, different boundary choices are likely to lead to different results (Bamber et al., 2020; Schaubroeck et al., 2021).
Lack of quality data is an issue frequently cited in the LCA literature (Miı̀ A I Canals et al., 2011; Hetherington et al., 2013; Sanju?n et al., 2013; Fernando Morales-Mendoza and Azzaro-Pantel, 2017). Data may not be available because the product is new and there is not a sufficient basis for data collection or there may be an inventory flow that is particularly difficult to measure, such as fugitive emissions. Fugitive emissions are unmeasured releases of an emission to the environment that occur outside of the designed flow of materials (Wanichpongpan and Gheewala, 2007; Brandt, 2011; Wang et al., 2018; Grubert and Brandt, 2019). Also termed “leakage”, fugitive emissions of plastic are the primary mechanism for plastic debris to enter aquatic environments and one of the current challenges of LCA for plastic products (Chitaka and von Blottnitz, 2021; Scagnetti and Lorenz, 2022). Marine litter often originates from communities where modern waste collection infrastructure is lacking or by inefficient capture of plastics by waste collection, leading to significant amount of plastic leakage in some contexts (Jambeck et al., 2015; Geyer et al., 2017).
Finally, much discussion has surrounded the methods that LCA practitioners use to characterize the environmental impacts of a product, which is particularly relevant for the case of plastic emissions73. The life cycle inventory phase of LCA collects raw data regarding energy and material inputs and emissions and wastes, but raw emissions data does not provide a full picture of actual environmental impact. The life cycle impact assessment phase is needed to translate the raw inventory data into a measure of environmental impact (Goedkoop and Spriensma, 2001; Norris et al., 2001; Jolliet et al., 2003; Bare and Gloria, 2006; Hauschild et al., 2013). A subset of the LCA research community has dedicated efforts to developing appropriate methods and tools to perform environmental impact assessment, characterizing and quantifying the environmental impacts associated with specific emissions and the causal linkages between emissions and impact (Jolliet et al., 2003; Landis and Theis, 2008; Rosenbaum et al., 2008; Hauschild et al., 2013; Speck et al., 2015; Huijbregts et al., 2017; Wenning et al., 2017). Life cycle impact assessment highlights that “environmental impact” is not a singular entity, but multiple categories of impact that affect the environment differently. LCA practitioners must select the environmental impact categories they will measure in the context of the study (van Hoof et al., 2014). Different studies may elect to focus on a subset of impact categories that are deemed the most relevant or for which data are known to be available. Common environmental impact categories include climate change, energy use, eutrophication, smog formation, ozone depletion, human toxicity, ecotoxicity, acidification, ozone depletion, and natural resource depletion (Bare, 2011). Additional impacts categories, such as methods to estimate the impacts of marine litter, are actively in the process of development and discussed in greater detail below.
There are methods to translate different kinds of emissions into a metric associated with a specific environmental impact category, which are known as midpoint indicators (Jolliet et al., 2004). For example, carbon dioxide, methane, and nitrous oxide are all greenhouse gas emissions, but nitrous oxide is much more powerful than carbon dioxide. Therefore, all greenhouse gas emissions have a conversion factor to translate each kind of emissions into a similar midpoint indicator, in this case, a global warming potential measured as mass of CO2-equivalents (IPCC, 2014). Endpoint indicators aggregate midpoint environmental impact categories into overall damage categories such as human health, biodiversity, and resource scarcity (Huijbregts et al., 2017).
When interpreting the results of an LCA, it is important to remember that all materials require energy and create emissions throughout their life cycle. All materials have some level of electricity-related and transport emissions, as well as environmental impacts that are specific to the material. Plastics are derived from fossil fuels and do not degrade easily, which lead to various ecological issues. Meanwhile, metals and glass require mining during their raw material extraction process and high temperatures during their manufacture. Pulp & paper production has significant aquatic loading of COD and BOD, whereas bio-based products (cotton, natural fibers, bioplastics) tend to consume large quantities of fresh water and land, while also contributing to aquatic nutrient and pesticide pollution. These tradeoffs are inherent and real and cannot be fully rectified by LCA or any other assessment tool. There is no manufactured material that is devoid of environmental impact; therefore, it is relatively rare to find comparative LCA where one alternative is better in all measured impact categories unless an alternative is able to reduce or eliminate consumption of materials. While plastic is certainly responsible for a host of environmental impacts including damage to marine life, LCA methods highlight that substituting alternative materials for plastic without an actual reduction in consumption are likely to create different environmental impacts elsewhere (Miller, 2020).
For proper interpretation of any LCA study, it is important to know what environmental impact categories are being measured and included in the study. Some impacts are relatively easily captured and have a direct, linear relationship with inventory emissions. This is the case with GHG emissions, where: (1) GHG emissions are relatively straightforward to estimate within the context of life cycle inventory data collection; (2) there are well established relationships between GHGs with different radiative properties to be able to translate into CO2 equivalents (i.e., methane is 25 stronger than CO2); (3) the relationship between emissions and impact can be reasonably approximated as linear (i.e., 100 kg CO2-eq has ten times greater impact than 10 kg CO2-eq). In addition, GHG emissions are not location specific; a kg of CO2 emitted in on geography has the same overall impact as a kg of CO2 emitted elsewhere. Even so, GHG emission inventories can be highly uncertain in specific contexts. For example, estimating the fugitive methane emissions from natural gas extraction are highly variable and are difficult to quantify without direct measurement (Howarth et al., 2011; Alvarez et al., 2012). Also, because CO2-eq are a midpoint impact category that are used as a proxy for overall damage, an LCA that reports climate emissions in terms of CO2-eq is generally not accounting for the full damage associated with CO2 emissions, including impacts of elevated GHG on oceans due to increased temperatures and acidification.
Environmental impacts not related to climate change tend to be more difficult to capture within LCA, due to both data availability and the nature of specific impacts. Although marine litter is a great example, it is not the only case where impacts are difficult to estimate from inventory results. For example, forest fragmentation that can be associated with some bio-based products has similar challenges (Seager et al., 2009). Similarly, quantifying noise pollution's impact to ecosystem and human health is difficult to quantify (Meyer et al., 2017). Fugitive emissions occur in many common pathways and are not often reported in LCA, for example coal dust that causes air pollution during rail transport or infrequent events such as coal ash or fracking fluid spills (Vengosh et al., 2009; Chen et al., 2017). Despite the challenges, there have been major efforts to improve characterization of a variety of environmental impacts beyond climate change (Knoepfel, 1996; Notarnicola et al., 1998; Goedkoop and Spriensma, 2001; Bare et al., 2003; Rosenbaum et al., 2008; Hauschild et al., 2013; Ernstoff et al., 2019; Saling et al., 2020), including efforts to create an impact indicator for marine litter.
In contrast to GHG emissions, marine litter associated with a single-use plastic application is (1) highly variable and difficult to measure (Malli et al., 2022); (2) the relationship between marine litter and physical ecosystem damage is complicated and the science surrounding environmental impact is still being debated (Salieri et al., 2021); (3) does not necessarily exhibit linear behavior between amount of discarded plastic and damage to ecosystems (Woods et al., 2016). A kg of plastic discarded in a coastal community with poor waste management infrastructure will have a different probability of ecosystem damage compared to a kg of plastic discarded in an inland community with well-developed management capabilities. In addition, estimating the probability that a given piece of discarded plastic will eventually cause ecosystem damage is difficult to measure directly. Finally, there is likely a non-linear relationship between marine litter and ecosystem damage. Marine litter can damage ecosystems in multiple ways, including physical damage associated with strangulation or ingestion of larger plastic debris, accumulation of microplastics in the digestive system, and toxicity associated with microplastic.
Multiple efforts to better estimate “plastic leakage” are ongoing to improve life cycle inventories and impact assessments of plastic materials. The Medellin Declaration on Marine Litter in 2017 established a commitment by the LCA community to provide evidence-based guidance for inclusion of plastic pollution in LCA, including both improved methods for calculating the fraction of plastics that are released into the environment and methods to estimate the resultant environmental damage (Sonnemann and Valdivia, 2017). The Marine Impacts in LCA working group was formed to help coordinate and disseminate efforts to reduce this gap in LCA methods (Boulay et al., 2021). Multiple efforts to integrate marine litter impacts alongside more long-standing impact categories are ongoing and vary in levels of complexity and robustness (Civancik-Uslu et al., 2019; Saling et al., 2020; Boulay et al., 2021; Lavoie et al., 2021; Stefanini et al., 2021; Woods et al., 2021; Corella-Puertas et al., 2022; Maga et al., 2022; Tang et al., 2022). The purpose of this review is not to discuss or debate different impact assessment metrics for plastic waste, but instead to highlight differences in scientific viewpoints that are likely to continue to occur in the use of LCA for evaluating the plastic challenge, irrespective of the development of suitable LCA impact metrics for marine litter.
Numerous LCA studies have evaluated the environmental impacts of plastic, which have been synthesized via a number of existing reviews (Al-Salem et al., 2009; Miandad et al., 2016; Deviatkin et al., 2019; Schwarz et al., 2019; Walker and Rothman, 2020; Alhazmi et al., 2021; Anshassi et al., 2021; Bishop et al., 2021; Davidson et al., 2021; Rodrigues Da Silva et al., 2021; Gómez and Escobar, 2022; Kan and Miller, 2022). It is not possible to make universal statements about the relative environmental impacts of different kinds of materials, since a material's physical properties may be more suitable for different functions, which in turn affects performance and subsequent environmental impact (Weidema et al., 2004). Nevertheless, some general trends and observations emerge in the context of the reviews.
As discussed above, “environmental impact” is not a single entity but multiple different kinds of environmental impact categories. Many comparative LCA uncover tradeoffs among different impact categories, often finding that there is not one product that is universally superior in all impact categories (Prado et al., 2022). Ultimately, the extent to which a decision-maker prioritizes different impact categories often guides the decision toward particular alternative. The extent to which a decision-maker values the tradeoffs uncovered in LCA is an inherently subjective process. Although though there are some methods available to help a decision maker better evaluate the tradeoffs, the fundamental issue of tradeoffs are not eliminated (Hertwich and Hammitt, 2001).
The overall plastic sector is responsible for significant global GHG emissions (Zheng and Suh, 2019); however, the climate impacts of an individual plastic product compared to an alternative using different materials is often lower on an LCA basis (Kouloumpis et al., 2020; Kelly and Dai, 2021). A recent cross-sector study found that plastics had lower GHG emissions than non-plastic alternatives in 13 of 14 cases, representing GHG savings from 10 to 90 percent (Helmcke et al., 2022). Meanwhile, multiple LCA studies on food containers show that plastic often exhibits a lower environmental impact relative to non-returnable glass and metal on a variety of environmental impact categories (Humbert et al., 2009; Saleh, 2016; Boesen et al., 2019). The results when comparing plastics to returnable glass, paperboard, and bio-based materials are mixed (Xie et al., 2011; Scipioni et al., 2013; Abejón et al., 2020). Although climate impact is often smaller for plastic, there are additional environmental impact categories to consider, including the impact on ecosystems.
The small number of studies that quantify marine litter or physical ecosystem damage have indicated that plastic tends to be the worst option with respect to that environmental impact category (Civancik-Uslu et al., 2019; Gao and Wan, 2022). This sets up a tradeoff where substituting plastic with another material such as glass may lead to reductions in marine litter at the expense of greater greenhouse gas emissions (Kouloumpis et al., 2020). That doesn't mean that the substitution shouldn't occur; but it is important for decision makers to be cognizant of that tradeoff. One study indicated that when included in a larger impact framework weighing multiple impact categories, marine litter does not change the overall rankings of products (Gao and Wan, 2022); however, the extent of the tradeoff will be different depending on the specific product system analyzed and prioritization of the impacts.
Different prioritizations of environmental impact categories can lead to different decisions. On one hand, those who value plastic pollution as the primary issue will likely prioritize any solution that reduces plastic pollution (Lavers et al., 2022). On the other side of the argument, those who prioritize climate change or another environmental impact may argue that alternative solutions should be promoted, even if they do not lead to a decrease in plastic production (Stafford and Jones, 2019a; Abejón et al., 2020). Some have argued that it is essential to develop solutions that do both–mitigate climate change and reduce the quantity of plastic waste (Stafford and Jones, 2019b; Miller, 2021; Ford et al., 2022). And certainly, there are some solutions–such as minimizing overall consumption–that can achieve win-win scenarios by getting at the root cause of many environmental issues simultaneously. Reduced consumption is often one of the only ways to reduce environmental impact across all environmental impact categories without resulting tradeoffs. Nevertheless, some level of material consumption will always need to occur. As LCA has consistently shown, it is relatively rare to find an option that reduces all environmental impacts at the same time. In these instances, the tradeoffs will be present and preferred “least damage” scenarios need to be chosen. Ignoring the tradeoffs that arise with a solitary focus on plastic waste reduction will likely lead to unintended consequences (Miller, 2020), and has the potential to distract from finding more holistic solutions. The following section describes some of the potential unintended consequences and how LCA is used to identify them.
Every LCA is suited to a particular purpose and system, so it is not possible to make universal assertions regarding the life cycle results of one material vs. another. One of the foundational requirements of LCA is to define a “functional unit” that can provide a comparison based on commensurate performance. Common measures of mass or volume are not always appropriate functional units. As an example, 1 kg of steel has very different physical properties when compared to 1 kg of a plastic polymer, which has very different physical properties to 1 kg of glass. Depending on the ultimate application and performance of these materials, the functional unit of a product may require more or less mass than other materials. Figure 1 demonstrates this concept, showing the relative number of 12 oz bottles that can be made from similar masses of glass and plastic and the subsequent difference of reporting GHG emissions on a functional unit basis. Even though the GHG emissions associated with producing 1kg of glass is lower than those associated with producing 1 kg of plastic (0.63 kg CO2-eq/kg glass vs. 2.3 kg CO2-eq/kg plastic), the impacts per product are lower for plastic because 1 kg of plastic produces 17 times the containers than a similar mass of glass.
Figure 1. 1 kg of glass and plastic to be used to produce 12 oz beverage containers, assumed to contain 10.3 g of PET for plastic and 180 g of glass and yielding 5.6 and 97 containers respectively. Assumes 2.3 kg CO2-eq/kg PET and 0.63 kg CO2-eq/kg glass.
LCA ensures that alternatives be compared on an appropriate functional unit basis, so as not to derive inappropriate conclusions. While a kg of polymer may generate more GHGs than a kg of glass, it is not an appropriate conclusion that glass has less climate impact than plastic. LCA methods highlight the importance of evaluating alternatives on a systematic basis.
LCA can also highlight potential unintended consequences of proposed alternatives. One specific proposed solution to plastic waste pollution is the implementation of plastic bag bans. A few recent LCA studies have evaluated the potential outcomes of bag bans, including a recent review that summarized the comparative impacts of reusable bags with single-use plastic bags (Gómez and Escobar, 2022). Although data is still relatively scarce, the review discusses the potential impact of plastic bag bans on consumer behavior, identifying three possible scenarios. First, fewer bags may be used overall as consumers forgo the need for any bag in certain circumstances. Second, single-use carrier bags of alternative materials (i.e., paper) may be used as a substitute. Finally, plastic bag bans may incentivize greater use of reusable carrier bags by customers. In all likelihood, any bag ban will result in some mixture of these outcomes (Martinho et al., 2017).
Decreased overall consumption of bags without any direct or indirect substitution will result in lower environmental impact. The other potential scenarios identified are more nuanced. The review found that substitution to single-use paper or other alternatives will result in greater global warming and ecotoxicity (Gómez and Escobar, 2022). In addition to direct changes associated with potential bag substitutes, there may be indirect changes to consumption patterns, such as greater consumer purchases of more durable garbage bags to compensate for the lack of single-use thin film plastic bags reused as waste bags (Martinho et al., 2017).
For reusable bags, consumers need to reuse the reusable bags a sufficient number of times in order to realize an environmental benefit over the single-use item to account for the greater material intensity that causes its durability. The number of reuses that are required for a reusable bag to “break even” with its single-use plastic counterpart is highly variable and depends on the type of material used, the environmental impact category in question, and a variety of other assumptions (Lewis et al., 2010). While not specifically focused on carrier bags, a number of LCA studies have highlighted the importance of consumer reuse to demonstrate environmental benefits of reusables over single-use plastic (Woods and Bakshi, 2014; Potting and van der Harst, 2015; Blanca-Alcubilla et al., 2020; Fetner et al., 2021). Certain reusable items may never break even with single-use plastic on certain impact categories, due to the impacts of washing the reusable alternative being greater than the single-use item (Fetner et al., 2021).
There are also numerous case studies in the LCA literature dedicated to the use of plastic in food packaging and potential tradeoffs that can arise between packaging and food loss (Silvenius et al., 2014; Wohner et al., 2019). One of the most difficult aspects of LCA of food packaging is to appropriately capture the performance of packaging materials with respect to food waste (Heard et al., 2019; Heller et al., 2019; Kan and Miller, 2022). With unique physical properties to be able to product preservation, plastics can often improve the shelf life of specific foods better than other packaging alternatives, and food production can be environmentally intensive, particularly in the context of meat or dairy. Therefore, in addition to merely analyzing the environmental impacts of the packaging, an appropriately conducted LCA should also examine any change in performance.
When considering the plastic waste problem, there are generally two perspectives that are used to categorize the extent and severity of environmental impacts of plastic. The life cycle perspective analyzes the environmental impact associated with a specific product, often comparing one product alternative to another and assessing which has lower impact (Abejón et al., 2020; Helmcke et al., 2022). An alternative perspective analyzes the environmental impacts of the plastic sector in aggregate, analyzing the full scope and scale of plastics in the environment (Jambeck et al., 2015; World Economic Forum, 2016; Geyer et al., 2017). Both perspectives provide meaningful data and insights, yet each provides an incomplete understanding of the plastics problem. These differing perspectives may exacerbate some of the divisions in discussions surrounding solutions to plastic waste. When evaluating only comparative impacts, it may be easy to overlook the bigger picture and the aggregated impacts of the plastics industry (Walker and McKay, 2021). When evaluating only the aggregate impacts of plastic, it may be easy to overlook other environmental issues that may be created by well-intended solutions (Miller, 2021). Understanding and integrating these perspectives may be necessary to come up with satisfactory solutions to plastic waste challenges.
Researchers working specifically on the issue of plastic pollution tend to focus on the aggregate current and future impacts of plastics on aquatic systems (Jambeck et al., 2015; Verma et al., 2016; Kubowicz and Booth, 2017; Schnurr et al., 2018; Kosior and Crescenzi, 2020; Saling et al., 2020; Walker and McKay, 2021). Researchers who focus on the aggregate impact of plastic highlight the magnitude of environmental impacts for which the plastic sector is responsible. As earlier discussed, plastic is ubiquitous and the industry is responsible for major environmental impact given the size of the industry (Jambeck et al., 2015; Zheng and Suh, 2019). Anticipated exponential growth in the plastics industry will only exacerbate the impacts of plastic across a range of environmental impact categories. As the transportation and electricity industries undergo large-scale transitions to lower carbon futures, the proportion of climate emissions associated with the plastic sector is expected to increase significantly (World Economic Forum, 2016). This aggregate perspective highlights the overall damage for which the plastic sector is responsible and underscores the need to reduce the impacts of the industry. At the same time, the aggregate perspective does not usually account for the potential consequences that may occur as a result of material substitution away from plastic (Miller, 2020). Focusing only on reducing the aggregate impact of plastics without also assessing the potential consequences of increases in alternative materials may cause a different suite of environmental issues. It may be easy to develop simple heuristics that imply any reduction of plastic will result in environmental improvement. While reduction in plastic use will reduce the specific environmental impact of marine litter, the overall system is complicated and requires a more holistic approach (Gao and Wan, 2022). When elimination of plastic results in actual reduction of consumption, there is likely an environmental benefit (Ford et al., 2022). When reducing plastic increases the consumption of another material, there are likely tradeoffs that will occur (Lindh et al., 2016). When material substitutes or indirect consequences occur as a result of plastic elimination (i.e., increased food waste), the aggregate impact on the environment is not guaranteed to be favorable (Silvenius et al., 2014; Heller et al., 2019).
In contrast to the aggregate approach, LCA practitioners tend to focus on the environmental impacts of plastic in comparison to other alternatives rather than the aggregate impacts of plastic. Most LCA compare the impacts of plastic relative to other alternatives to perform a similar function. This comparative perspective is useful to help inform design decisions about a specific product, answering questions such as “Is it better to make this cup out of metal or plastic?” (Millet et al., 2007). Comparative LCA provide insights into the material(s) that produce the fewest environmental impacts for a given product function and identify tradeoffs among environmental impact categories, such as marine litter and GHG emissions (Hertwich and Hammitt, 2001; Gao and Wan, 2022). LCA can also offer insights into how to resolve potential tradeoffs or where to focus efforts to result in the greatest improvement potential across emission categories. At the same time, the life cycle perspective does explicitly discuss the aggregate impact of individual product choices. The comparative life cycle perspective can identify an alternative with fewer environmental impacts; however, that alternative may still cause a great deal of environmental damage. Just because one alternative is “better” in a given impact category does not actually mean that it is “good” (McDonough and Braungart, 2002; Schnitzer and Ulgiati, 2007). Therefore, LCA information that indicates that a preferred alternative can be potentially misleading or misinterpreted, where the actual environmental impact of plastics is not fully considered.
In order to find effective solutions to the plastic problem, it will be essential to understand both the aggregated impacts of the entire plastic economy and the comparative impacts of plastic relative to other materials. Neither of the aggregate or relative perspective is better or worse; both can be useful. Ideally, they complement one another. One major question to ask should be, “If it's better, will it matter?” This involves understanding the overall size of a product's market and the aggregate scale of environmental impact (Bergerson et al., 2020). A 2% reduction in emissions associated with something that is responsible for 10% of the aggregate impact is going to have greater effectiveness than something with a 10% reduction of 1% of the aggregate impact.
To summarize, LCA has the potential to make valuable contributions to the overall plastic debate by helping to identify potential unintended consequences of proposed solutions that could cause environmental problem shifting. While an imperfect tool, LCA can be used to place the effects of environmental policy and design decisions into context and provide rigorous and systematic analysis. LCA will never be able to rectify systemic tradeoffs among different materials, such as the marine litter pollution of single-use plastic compared to the additional BOD/COD loading from paper. Nevertheless, LCA can quantify the impacts of individual materials and help provide a framework to discuss those tradeoffs.
Certain LCA studies may point out tradeoffs associated with material substitution, particularly since relative to other materials, plastics tend to consume relatively low amounts of energy, which tends to translate to lower overall emissions. On an aggregate basis, the GHG emissions associated with plastics are large; but the relative GHG emissions of individual products tend to be quite small. Although LCA may be able to identify alternatives with fewer environmental impact, the aggregate impacts of plastic use cannot and should not be ignored. Just because a product alternative has fewer environmental impacts does not actually mean that the alternative is actually sustainable.
The comparative LCA perspective and the aggregated environmental impact perspective can complement one another and lead to overall improved environmental outcomes when used in tandem. While material substitution may not necessarily lead to improved outcomes, there are ways to reduce both marine litter and GHG emissions simultaneously. The plastic waste issue highlights the need to focus on reduced overall consumption rather than material substitution at a similar level of consumption. Interventions that lead to a true reduction of consumption will reduce overall environmental impact. Merely shifting away from plastic as a priority area of focus without understanding the full consequences of a material substitution has the potential to create environmental problem shifting and not achieve sustainable outcomes.
SM was responsible for compiling and writing the manuscript.
The author declares that the research was conducted in the absence of any commercial or financial relationships that could be construed as a potential conflict of interest.
All claims expressed in this article are solely those of the authors and do not necessarily represent those of their affiliated organizations, or those of the publisher, the editors and the reviewers. Any product that may be evaluated in this article, or claim that may be made by its manufacturer, is not guaranteed or endorsed by the publisher.
Abejón, R., Bala, A., Vázquez-Rowe, I., Aldaco, R., and Fullana-i-Palmer, P. (2020). When plastic packaging should be preferred: Life cycle analysis of packages for fruit and vegetable distribution in the Spanish peninsular market. Resour. Conserv. Recycl. 155, 104666. doi: 10.1016/j.resconrec.2019.104666
Alhazmi, H., Almansour, F. H., and Aldhafeeri, Z. (2021). Plastic waste management: a review of existing life cycle assessment studies. Sustainability. 13, 5340. doi: 10.3390/su13105340
Al-Salem, S. M., Lettieri, P., and Baeyens, J. (2009). Recycling and recovery routes of plastic solid waste (PSW): a review. Waste Manag. 29. doi: 10.1016/j.wasman.2009.06.004
Alvarez, R. A., Pacala, S. W., Winebrake, J. J., Chameides, W. L., and Hamburg, S. P. (2012). Greater focus needed on methane leakage from natural gas infrastructure. Proc. Nat. Acad. Sci. 109, 6435–6440. doi: 10.1073/pnas.1202407109
Anshassi, M., Sackles, H., and Townsend, T. G. (2021). A review of LCA assumptions impacting whether landfilling or incineration results in less greenhouse gas emissions. Resour Conserv Recycl. 174, 105810. doi: 10.1016/j.resconrec.2021.105810
APME (2003). Eco-Profiles of the European plastics industry: Polyolefins. Brussels: Association of Plastics Manufacturers in Europe, I Boustead.
Backhaus, T., and Wagner, M. (2020). Microplastics in the environment: much ado about nothing? A Debate. Global Challenges. 4, 1900022. doi: 10.1002/gch2.201900022
Bamber, N., Turner, I., Arulnathan, V., Li, Y., Zargar Ershadi, S., Smart, A., et al. (2020). Comparing sources and analysis of uncertainty in consequential and attributional life cycle assessment: review of current practice and recommendations. Int. J. Life Cycle Assess. 25, 168–180. doi: 10.1007/s11367-019-01663-1
Bare, J., Norris, G. A., Pennington, D. W., and McKone, T. (2003). TRACI The Tool for the reduction and assessment of chemical and other environmental impacts. J. Ind. Ecol. 6, 49–78. doi: 10.1162/108819802766269539
Bare, J. C. (2011). TRACI 2.0 - The tool for the reduction and assessment of chemical and other environmental impacts. Clean Technol. Environ. Policy. 13, 687–696. doi: 10.1007/s10098-010-0338-9
Bare, J. C., and Gloria, T. P. (2006). Critical analysis of the mathematical relationships and comprehensiveness of life cycle impact assessment approaches. Environ. Sci. Technol. 40, 1104–1113 doi: 10.1021/es051639b
Bergerson, J. A., Brandt, A., Cresko, J., Carbajales-Dale, M., MacLean, H. L., Matthews, H. S., et al. (2020). Life cycle assessment of emerging technologies: evaluation techniques at different stages of market and technical maturity. J. Ind. Ecol. 24, 11–25. doi: 10.1111/jiec.12954
Bishop, G., Styles, D., and Lens, P. N. L. (2021). Environmental performance comparison of bioplastics and petrochemical plastics: A review of life cycle assessment (LCA) methodological decisions. Resour. Conserv. Recycl. 168, 105451. doi: 10.1016/j.resconrec.2021.105451
Blanca-Alcubilla, G., Bala, A., de Castro, N., Colom,é, R., and Fullana-i-Palmer, P. (2020). Is the reusable tableware the best option? Analysis of the aviation catering sector with a life cycle approach. Sci. Total Envir. 708, 135121. doi: 10.1016/j.scitotenv.2019.135121
Boesen, S., Bey, N., and Niero, M. (2019). Environmental sustainability of liquid food packaging: Is there a gap between Danish consumers' perception and learnings from life cycle assessment? J. Clean. Prod. 210, 1193–1206. doi: 10.1016/j.jclepro.2018.11.055
Boulay, A.-M., Verones, F., and Vázquez-Rowe, I. (2021). Marine plastics in LCA: current status and MarILCA's contributions. Int. J. Life Cycle Assess. 26, 2105–2108. doi: 10.1007/s11367-021-01975-1
Brander, M., Tipper, R., Hutchison, C., Davis, G., and Press, E. (2009). Consequential and Attributional Approaches to LCA: a Guide to Policy Makers with Specific Reference to Greenhouse Gas LCA of Biofuels. Edinburgh: Ecometrica.
Brandt, A. R. (2011). Variability and uncertainty in life cycle assessment models for greenhouse gas emissions from canadian oil sands production. Environ. Sci. Technol. 46, 1253–1261. doi: 10.1021/es202312p
Burton, G. A. (2017). Stressor exposures determine risk: so, why do fellow scientists continue to focus on superficial microplastics risk? Environ. Sci. Technol. 51, 13515–13516. doi: 10.1021/acs.est.7b05463
Catarino, A. I., Kramm, J., Völker, C., Henry, T. B., and Everaert, G. (2021). Risk posed by microplastics: Scientific evidence and public perception. Curr. Opin. Green Sustain. Chem. 29, 100467. doi: 10.1016/j.cogsc.2021.100467
Chen, L., Miller, S. A., and Ellis, B. R. (2017). Comparative human toxicity impact of electricity produced from shale gas and coal. Environ. Sci. Technol. 51, 13018–13027. doi: 10.1021/acs.est.7b03546
Chitaka, T. Y., and von Blottnitz, H. (2021). Development of a method for estimating product-specific leakage propensity and its inclusion into the life cycle management of plastic products. Int. J. Life Cycle Assess. 26, 1431–1438. doi: 10.1007/s11367-021-01905-1
Choudhary, S., Liang, S., Cai, H., Keoleian, G. A., Miller, S. A., Kelly, J., et al. (2014). Reference and functional unit can change bioenergy pathway choices. Int. J. Life Cycle Assessm. 19, 796–805. doi: 10.1007/s11367-013-0692-z
Civancik-Uslu, D., Puig, R., Hauschild, M., and Fullana-i-Palmer, P. (2019). Life cycle assessment of carrier bags and development of a littering indicator. Sci. Total Envir. 685, 621–630. doi: 10.1016/j.scitotenv.2019.05.372
Corella-Puertas, E., Guieu, P., Aufoujal, A., Bulle, C., and Boulay, A.-M. (2022). Development of simplified characterization factors for the assessment of expanded polystyrene and tire wear microplastic emissions applied in a food container life cycle assessment. J. Ind. Ecol. 1–13. doi: 10.1111/jiec.13269
Davidson, M. G., Furlong, R. A., and McManus, M. C. (2021). Developments in the life cycle assessment of chemical recycling of plastic waste – a review. J. Clean. Prod. 293, 126163. doi: 10.1016/j.jclepro.2021.126163
Davis, J. M., and Thomas, V. M. (2006). Systematic approach to evaluating trade-offs among fuel options: the lessons of MTBE. Ann. N. Y. Acad. Sci. 1076, 498–515 doi: 10.1196/annals.1371.068
de Kleine, R. D., Keoleian, G. A., Miller, S. A., Burnham, A., and Sullivan, J. L. (2014). Impact of updated material production data in the GREET life cycle model. J. Ind. Ecol. 18, 356–365. doi: 10.1111/jiec.12132
Deng, L., and Williams, E. D. (2011). Functionality versus “typical product” measures of technological progress. J. Ind. Ecol. 15, 108–121 doi: 10.1111/j.1530-9290.2010.00306.x
Deviatkin, I., Khan, M., Ernst, E., and Horttanainen, M. (2019). Wooden and plastic pallets: a review of life cycle assessment (LCA) studies. Sustainability. 11, 5750. doi: 10.3390/su11205750
Dormer, A., Finn, D. P., Ward, P., and Cullen, J. (2013). Carbon footprint analysis in plastics manufacturing. J. Clean. Prod. 51, 133–141. doi: 10.1016/j.jclepro.2013.01.014
Earles, J. M., and Halog, A. (2011). Consequential life cycle assessment: a review. Int. J. Life Cycle Assess. 16, 445–453. doi: 10.1007/s11367-011-0275-9
Ernstoff, A., Niero, M., Muncke, J., Trier, X., Rosenbaum, R. K., Hauschild, M., et al. (2019). Challenges of including human exposure to chemicals in food packaging as a new exposure pathway in life cycle impact assessment. Int. J. Life Cycle Assess. 24, 543–552. doi: 10.1007/s11367-018-1569-y
Fernando Morales-Mendoza, L., and Azzaro-Pantel, C. (2017). Bridging LCA data gaps by use of process simulation for energy generation. Clean Technol Environ Policy. 19, 1535–1546. doi: 10.1007/s10098-017-1349-6
Fetner, H., Shelie, ·, Miller, A., and Benetto, E. (2021). Environmental payback periods of reusable alternatives to single-use plastic kitchenware products. Int. J. Life Cycle Assess. 26, 1521–1537. doi: 10.1007/s11367-021-01946-6
Ford, H. v, Jones, N. H., Davies, A. J., Godley, B. J., Jambeck, J. R., Napper, I. E., et al. (2022). The fundamental links between climate change and marine plastic pollution. Sci. Total Environ. 806, 150392. doi: 10.1016/j.scitotenv.2021.150392
Frijia, S., Guhathakurta, S., and Williams, E. (2012). Functional unit, technological dynamics, and scaling properties for the life cycle energy of residences. Environ. Sci. Technol. 46, 1782–1788. doi: 10.1021/es202202q
Gao, A. L., and Wan, Y. (2022). Life cycle assessment of environmental impact of disposable drinking straws: a trade-off analysis with marine litter in the United States. Sci. Total Environ. 817, 153016. doi: 10.1016/j.scitotenv.2022.153016
Geyer, R., Jambeck, J. R., and Law, K. L. (2017). Production, use, and fate of all plastics ever made. Sci. Adv. 3. doi: 10.1126/sciadv.1700782
Geyer, R., Kuczenski, B., Zink, T., and Henderson, A. (2016). Common misconceptions about recycling. J. Ind. Ecol. 20, 1010–1017. doi: 10.1111/jiec.12355
Goedkoop, M., and Spriensma, R. (2001). The Eco-indicator 99: A damage oriented method for Life Cycle Impact Assessment. Amersfoort: PRe product ecology consultants.
Gómez, I. D. L., and Escobar, A. S. (2022). The dilemma of plastic bags and their substitutes: a review on LCA studies. Sustain. Prod. Consum. 30, 107–116. doi: 10.1016/j.spc.2021.11.021
Grubert, E. A., and Brandt, A. R. (2019). Three considerations for modeling natural gas system methane emissions in life cycle assessment. J. Clean. Prod. 222, 760–767. doi: 10.1016/j.jclepro.2019.03.096
Hauschild, M. Z., Goedkoop, M., Guinée, J., Heijungs, R., Huijbregts, M., Jolliet, O., et al. (2013). Identifying best existing practice for characterization modeling in life cycle impact assessment. Int. J. Life Cycle Assess. 18, 683–697. doi: 10.1007/s11367-012-0489-5
Heard, B. R., Bandekar, M., Vassar, B., and Miller, S. A. (2019). Comparison of life cycle environmental impacts from meal kits and grocery store meals. Resour. Conserv Recycl. 147, 189–200. doi: 10.1016/j.resconrec.2019.04.008
Heller, M. C., Mazor, M. H., and Keoleian, G. A. (2020). Plastics in the US: toward a material flow characterization of production, markets and end of life. Envir. Res. Lett. 15, 094034. doi: 10.1088/1748-9326/ab9e1e
Heller, M. C., Selke, S. E. M., and Keoleian, G. A. (2019). Mapping the influence of food waste in food packaging environmental performance assessments. J. Ind. Ecol. 23, 480–495. doi: 10.1111/jiec.12743
Helmcke, S., Hundertmark, T., Musso, C., Ong, W. J., Oxgaard, J., and Wallach, J. (2022). Climate impact of plastics. Vienna: McKinsey & Company.
Herberz, T., Barlow, C. Y., and Finkbeiner, M. (2020). Sustainability assessment of a single-use plastics ban. Sustainability. 12, 3746 doi: 10.3390/su12093746
Hertwich, E. G., and Hammitt, J. K. (2001). A decision-analytic framework for impact assessment part i: LCA and decision analysis. Int. J. Life Cycle Assess. 6, 5–12. doi: 10.1007/BF02977588
Hetherington, A. C., Li, A., Owen, B., Griffiths, G., and Mcmanus, M. C. (2013). Use of LCA as a development tool within early research: challenges and issues across different sectors. Int. J. Life Cycle Assess. 19, 130–143. doi: 10.1007/s11367-013-0627-8
Hou, P., Xu, Y., Taiebat, M., Lastoskie, C., Miller, S. A., and Xu, M. (2018). Life cycle assessment of end-of-life treatments for plastic film waste. J. Clean. Prod. 201, 1052–1060. doi: 10.1016/j.jclepro.2018.07.278
Howarth, R. W., Santoro, R., and Ingraffea, A. (2011). Methane and the greenhouse-gas footprint of natural gas from shale formations. Clim. Change. 106, 679–690 doi: 10.1007/s10584-011-0061-5
Huang, Y.-K., and Woodward, R. T. (2022). Spillover effects of grocery bag legislation: evidence of bag bans and bag fees. Environ Resour Econ (Dordr). 81, 711–741. doi: 10.1007/s10640-022-00646-5
Huijbregts, M. A. J., Norris, G. A., Bretz, R., Ciroth, A., Maurice, B., Bahr, B., et al. (2001). Framework for modeling data uncertainty in life cycle inventories. Int. J. Life Cycle Assess. 6, 127–132. doi: 10.1007/BF02978728
Huijbregts, M. A. J., Steinmann, Z. J. N., Elshout, P. M. F., Stam, G., Verones, F., Vieira, M., et al. (2017). ReCiPe2016: a harmonised life cycle impact assessment method at midpoint and endpoint level. Int. J. Life Cycle Assess. 22, 138–147. doi: 10.1007/s11367-016-1246-y
Humbert, S., Rossi, V., Margni, M., Jolliet, O., and Loerincik, Y. (2009). Life cycle assessment of two baby food packaging alternatives: glass jars vs. plastic pots. Int. J. Life Cycle Assess. 14, 95–106. doi: 10.1007/s11367-008-0052-6
Hung. (2009). Quantifying system uncertainty of life cycle assessment based on Monte Carlo simulation. Int. J. Life Cycle Assess. 14, 19–27 doi: 10.1007/s11367-008-0034-8
International Organization for Standardization (ISO) (2006). 14040 Environmental Management - Life cycle assessment. Prinicples and framework. Geneva, Switzerland: International Organization for Standardization (ISO)
IPCC (2014). Climate Change 2014: Synthesis Report. Contribution of Working Groups I, II, and III to the Fifth Assessment Report of the Intergovernmental Panel on Climate Change. Geneva, Switzerland.
Jambeck, J. R., Geyer, R., Wilcox, C., Siegler, T. R., Perryman, M., Andrady, A., et al. (2015). Plastic waste inputs from land into the ocean. Science (1979). 347, 768–771. doi: 10.1126/science.1260352
Jolliet, O., Margni, M., Charles, R., Humbert, S., Payet, J., Rebitzer, G., et al. (2003). IMPACT 2002+: a new life cycle impact assessment methodology. Int. J. Life Cycle Assess. 8, 324–330 doi: 10.1007/BF02978505
Jolliet, O., Miiller-Wenk, R., Bare, J., Brent, A., Goedkoop, M., Heijungs, R., et al. (2004). The LCIA Midpoint-damage Framework of the UNEP/SETAC Life Cycle Initiative. Int. J. Life Cycle Assess. 9, 394–404.
Kakadellis, S., and Harris, Z. M. (2020). Don't scrap the waste: the need for broader system boundaries in bioplastic food packaging life-cycle assessment – a critical review. J. Clean. Prod. 274, 122831. doi: 10.1016/j.jclepro.2020.122831
Kan, M., and Miller, S. A. (2022). Environmental impacts of plastic packaging of food products. Resour. Conserv. Recycl. 180, 106156. doi: 10.1016/j.resconrec.2022.106156
Kelly, J. C., and Dai, Q. (2021). “Life-Cycle Analysis of Vehicle Lightweighting: A Review BT - Electric, Hybrid, and Fuel Cell Vehicles,” in, Elgowainy, A. (New York, NY: Springer New York) p. 91–104. doi: 10.1007/978-1-0716-1492-1_1080
Knoepfel, I. H. (1996). A framework for environmental impact assessment of long-distance energy transport systems. Energy (Oxford). 21, 693–702 doi: 10.1016/0360-5442(95)00131-X
Kosior, E., and Crescenzi, I. (2020). Chapter 16 - Solutions to the plastic waste problem on land and in the oceans, Letcher, R. (Academic Press) p. 415–446. doi: 10.1016/B978-0-12-817880-5.00016-5
Kouloumpis, V., Pell, R. S., Correa-Cano, M. E., and Yan, X. (2020a). Potential trade-offs between eliminating plastics and mitigating climate change: An LCA perspective on Polyethylene Terephthalate (PET) bottles in Cornwall. Sci. Total Environ. 727, 138681. doi: 10.1016/j.scitotenv.2020.138681
Kubowicz, S., and Booth, A. M. (2017). Biodegradability of plastics: challenges and misconceptions. Environ. Sci. Technol. 51, 12058–12060. doi: 10.1021/acs.est.7b04051
Landis, A. E., and Theis, T. L. (2008). “Comparison of life cycle impact assessment tools in the case of biofuels,” in 2008 IEEE International Symposium on Electronics and the Environment (IEEE), 1–7. doi: 10.1109/ISEE.2008.4562869
Laurin, L. (2017). Overview of LCA—history, concept, and methodology. Encyclopedia of Sustainable Technol. 217–222. doi: 10.1016/B978-0-12-409548-9.10058-2
Lavers, J. L., Bond, A. L., and Rolsky, C. (2022). Far from a distraction: plastic pollution and the planetary emergency. Biol. Conserv. 272, 109655. doi: 10.1016/j.biocon.2022.109655
Lavoie, J., Boulay, A.-M., and Bulle, C. (2021). Aquatic micro- and nano-plastics in life cycle assessment: Development of an effect factor for the quantification of their physical impact on biota. J Ind Ecol. 1–13. doi: 10.1111/jiec.13140
Lewis, H., Verghese, K., and Fitzpatrick, L. (2010). Evaluating the sustainability impacts of packaging: the plastic carry bag dilemma. Packag. Technol. Sci. 23, 145–160. doi: 10.1002/pts.886
Lindh, H., Olsson, A., and Williams, H. (2016). Consumer perceptions of food packaging: contributing to or counteracting environmentally sustainable development? Packag. Technol. Sci. 29, 3–23. doi: 10.1002/pts.2184
Lo, S.-C., Ma, H., and Lo, S.-L. (2005). Quantifying and reducing uncertainty in life cycle assessment using the Bayesian Monte Carlo method. Sci. Total Envir. 340, 23–33 doi: 10.1016/j.scitotenv.2004.08.020
Macintosh, A., Simpson, A., Neeman, T., and Dickson, K. (2020). Plastic bag bans: lessons from the Australian Capital Territory. Resour. Conserv. Recycl. 154, 104638. doi: 10.1016/j.resconrec.2019.104638
Maga, D., Galafton, C., Blömer, J., Thonemann, N., Özdamar, A., and Bertling, J. (2022). Methodology to address potential impacts of plastic emissions in life cycle assessment. Int. J. Life Cycle Assess. 27, 469–491. doi: 10.1007/s11367-022-02040-1
Malli, A., Corella-Puertas, E., Hajjar, C., and Boulay, A.-M. (2022). Transport mechanisms and fate of microplastics in estuarine compartments: a review. Mar. Pollut. Bull. 177, 113553. doi: 10.1016/j.marpolbul.2022.113553
Martinho, G., Balaia, N., and Pires, A. (2017). The Portuguese plastic carrier bag tax: the effects on consumers' behavior. Waste Manag. 61, 3–12. doi: 10.1016/j.wasman.2017.01.023
Matthews, H. S., and Small, M. J. (2000). Extending the boundaries of life-cycle assessment through environmental economic input-output models. J Ind Ecol 4, 7–10 doi: 10.1162/108819800300106357
McDonough, W., and Braungart, M. (2002). “Chapter 2: ‘Why Being “Less Bad” Is No Good,”' in Cradle to Cradle (New York: North Point Press).
McKone, W. W., Berck, P., Auffhammer, M., and Lipman, T. N. (2011). Grand challenges for life-cycle assessment of biofuels. Environ. Sci. Technol. 45, 1751–1756 doi: 10.1021/es103579c
Meert, J., Izzo, A., and Atkinson, J. D. (2021). Impact of plastic bag bans on retail return polyethylene film recycling contamination rates and speciation. Waste Manag. 135, 234–242. doi: 10.1016/j.wasman.2021.08.043
Meyer, R., Benetto, E., Igos, E., and Lavandier, C. (2017). Analysis of the different techniques to include noise damage in life cycle assessment. A case study for car tires. Int. J. Life Cycle Assess. 22, 744–757. doi: 10.1007/s11367-016-1188-4
Miì A I Canals, L., Azapagic, A., Doka, G., Jefferies, D., King, H., Mutel, C., et al. (2011). Approaches for addressing life cycle assessment data gaps for bio-based products. Ind. Ecol. 15, 707–725. doi: 10.1111/j.1530-9290.2011.00369.x
Miandad, R., Barakat, M. A., Aburiazaiza, A. S., Rehan, M., and Nizami, A. S. (2016). Catalytic pyrolysis of plastic waste: a review. Process Saf Environ. 102. doi: 10.1016/j.psep.2016.06.022
Miller, S. A. (2020). Five misperceptions surrounding the environmental impacts of single-use plastic. Environ. Sci. Technol. 54, 14143–14151. doi: 10.1021/acs.est.0c05295
Miller, S. A. (2021). Response to comment on “five misperceptions surrounding the environmental impacts of single-use plastic.” Environ Sci Technol. 55, 1341–1342. doi: 10.1021/acs.est.0c08671
Millet, D., Bistagnino, L., Lanzavecchia, C., Camous, R., and Poldma, T. (2007). Does the potential of the use of LCA match the design team needs? J. Cleaner Production 16, 1958–1970 doi: 10.1016/j.jclepro.2005.07.016
Mullins, K. A., Griffin, W. M., and Matthews, H. S. (2011). Policy implications of uncertainty in modeled life-cycle greenhouse gas emissions of biofuels. Environ. Sci. Technol. 45, 132–138. doi: 10.1021/es1024993
Ng, F. S.-F., Muthu, S. S., Li, Y., and Hui, P. C.-L. (2013). A critical review on life cycle assessment studies of diapers. Crit. Rev. Environ. Sci. Technol. 43, 1795–1822. doi: 10.1080/10643389.2012.671746
Norris, G. A., Basson, L., and Seppala, J. (2001). Decision analysis frameworks for life-cycle impact assessment. J Ind Ecol 5, 45–68 doi: 10.1162/10881980160084033
Notarnicola, B., Huppes, G., and van den Berg, N. W. (1998). Evaluating options in LCA: the emergence of conflicting paradigms for impact assessment and evaluation. Int. J. Life Cycle Assess. 3, 289–300 doi: 10.1007/BF02979839
Pernollet, F., Coelho, C. R. v, and van der Werf, H. M. G. (2017). Methods to simplify diet and food life cycle inventories: Accuracy versus data-collection resources. J. Clean. Prod. 140, 410–420. doi: 10.1016/j.jclepro.2016.06.111
Potting, J., and van der Harst, E. (2015). Facility arrangements and the environmental performance of disposable and reusable cups. Int. J. Life Cycle Assess. 20, 1143–1154. doi: 10.1007/s11367-015-0914-7
Prado, V., Seager, T. P., and Guglielmi, G. (2022). Challenges and risks when communicating comparative LCA results to management. Int. J. Life Cycle Assess. doi: 10.1007/s11367-022-02090-5
Reap, J., Roman, F., Duncan, S., and Bras, B. (2008). A survey of unresolved problems in life cycle assessment. Int. J. Life Cycle Assess. 13. doi: 10.1007/s11367-008-0009-9
Rodrigues Da Silva, T., Rangel Garcez De Azevedo, A., Cecchin, D., Teixeira Marvila, M., Amran, M., Fediuk, R., et al. (2021). Materials application of plastic wastes in construction materials: a review using the concept of life-cycle assessment in the context of recent research for future. Perspectives. 14, 3549. doi: 10.3390/ma14133549
Rosenbaum, R., Bachmann, T., Gold, L., Huijbregts, M., Jolliet, O., Juraske, R., et al. (2008). USEtox—the UNEP-SETAC toxicity model: recommended characterisation factors for human toxicity and freshwater ecotoxicity in life cycle impact assessment. Int. J. Life Cycle Assess. 13, 532–546 doi: 10.1007/s11367-008-0038-4
Saleh, Y. (2016). Comparative life cycle assessment of beverages packages in Palestine. J. Clean. Prod. 131, 28–42. doi: 10.1016/j.jclepro.2016.05.080
Salieri, B., Stoudmann, N., Hischier, R., Som, C., and Nowack, B. (2021). How relevant are direct emissions of microplastics into freshwater from an LCA perspective? Sustainability. 13. doi: 10.3390/su13179922
Saling, P., Gyuzeleva, L., Wittstock, K., Wessolowski, V., and Griesshammer, R. (2020). Life cycle impact assessment of microplastics as one component of marine plastic debris. Int. J. Life Cycle Assess. 25, 2008–2026. doi: 10.1007/s11367-020-01802-z
Sanju?n, N. S., Stoessel, F., and Hellweg, S. (2013). Closing data gaps for LCA of food products: estimating the energy demand of food processing. Environ. Sci. Technol. 48, 1132–1140. doi: 10.1021/es4033716
Scagnetti, C., and Lorenz, M. (2022). Plastic leakage of packaging in life cycle assessment – a theoretical framework. E3S Web Conf. 349. doi: 10.1051/e3sconf/202234903002
Schaubroeck, T., Schaubroeck, S., Heijungs, R., Zamagni, A., Brandão, M., Benetto, E., et al. (2021). Attributional and consequential life cycle assessment: definitions, conceptual characteristics and modelling restrictions. Sustainability. 13, 7386. doi: 10.3390/su13137386
Schnitzer, H., and Ulgiati, S. (2007). Less bad is not good enough: approaching zero emissions techniques and systems. J. Clean. Prod. 15, 1185–1189. doi: 10.1016/j.jclepro.2006.08.001
Schnurr, R. E. J., Alboiu, V., Chaudhary, M., Corbett, R. A., Quanz, M. E., Sankar, K., et al. (2018). Reducing marine pollution from single-use plastics (SUPs): a review. Mar. Pollut. Bull. 137, 157–171. doi: 10.1016/j.marpolbul.2018.10.001
Schwarz, A. E., Ligthart, T. N., Boukris, E., and van Harmelen, T. (2019). Sources, transport, and accumulation of different types of plastic litter in aquatic environments: a review study. Mar. Pollut. Bull. 143, 92–100. doi: 10.1016/j.marpolbul.2019.04.029
Scipioni, A., Niero, M., Mazzi, A., Manzardo, A., and Piubello, S. (2013). Significance of the use of non-renewable fossil CED as proxy indicator for screening LCA in the beverage packaging sector. Int. J. Life Cycle Assess. 18, 673–682. doi: 10.1007/s11367-012-0484-x
Seager, T. P., Miller, S. A., and Kohn, J. (2009). “Land use and geospatial aspects in life cycle assessment of renewable energy,” in 2009 IEEE International Symposium on Sustainable Systems and Technology, ISSST'09 in Cooperation with 2009 IEEE International Symposium on Technology and Society. ISTAS. doi: 10.1109/ISSST.2009.5156724
SETAC (2001). SETAC-Europe LCA Working grouop “data availability and data quality.” Int. J. Life Cycle Assess. 6, 127 doi: 10.1007/BF02978727
Silvenius, F., Grönman, K., Katajajuuri, J.-M., Soukka, R., Koivupuro, H.-K., and Virtanen, Y. (2014). The role of household food waste in comparing environmental impacts of packaging alternatives. Packag. Technol. Sci. 27, 277–292. doi: 10.1002/pts.2032
Sonnemann, G., and Valdivia, S. (2017). Medellin declaration on marine litter in life cycle assessment and management. Int. J. Life Cycle Assess. 22, 1637–1639. doi: 10.1007/s11367-017-1382-z
Speck, R., Selke, S., Auras, R., and Fitzsimmons, J. (2015). Life cycle assessment software selection can impact results. Methods, Tools Software. 20, 18–28. doi: 10.1111/jiec.12245
Stafford, R., and Jones, P. J. S. (2019a). Viewpoint – ocean plastic pollution: a convenient but distracting truth? Mar Policy. 103, 187–191. doi: 10.1016/j.marpol.2019.02.003
Stafford, R., and Jones, P. J. S. (2019b). We should not separate out environmental issues, but the current approach to plastic pollution can be a distraction from meaningful action. A response to Avery-Gomm et al. Mar Policy 107, 103585. doi: 10.1016/j.marpol.2019.103585
Stefanini, R., Borghesi, G., Ronzano, A., and Vignali, G. (2021). Plastic or glass: a new environmental assessment with a marine litter indicator for the comparison of pasteurized milk bottles. Int. J. Life Cycle Assess. 26, 767–784. doi: 10.1007/s11367-020-01804-x
Tan, R. R., Briones, L. M. A., and Culaba, A. B. (2007). Fuzzy data reconciliation in reacting and non-reacting process data for life cycle inventory analysis. J. Clean. Prod. 15, 944–949 doi: 10.1016/j.jclepro.2005.09.001
Tang, Y., Mankaa, R. N., and Traverso, M. (2022). An effect factor approach for quantifying the impact of plastic additives on aquatic biota in life cycle assessment. Int. J. Life Cycle Assess. 27, 564–572. doi: 10.1007/s11367-022-02046-9
van Hoof, G., Vieira, M., Gausman, M., and Weisbrod, A. (2014). Policies and support in relation to lca Indicator selection in life cycle assessment to enable decision making: issues and solutions. Int. J. Life Cycle Assess. 18, 1568–1580.
Vengosh, A., Dwyer, G. S., Hsu-Kim, H., Amrika, D.eonarine, Bergin, M., and Kravchenko, J. (2009). Survey of the potential environmental and health impacts in the immediate aftermath of the coal ash spill in Kingston, Tennessee. Environ. Sci. Technol. 43, 6326–6333 doi: 10.1021/es900714p
Verma, R., Vinoda, K. S., Papireddy, M., and Gowda, A. N. S. (2016). Toxic pollutants from plastic waste—a review. Procedi Environ Sci 35. doi: 10.1016/j.proenv.2016.07.069
Vignon, B. W., Tolle, D. A., Cornaby, B. W., Latham, H. C., Harrison, C. L., Boguski, T. L., et al. (1992). Life-Cycle Assessment: Inventory Guidelines and Principles. Cincinnati: US Environmental Protection Agency, Battelle and Franklin Associates Ltd
Völker, C., Kramm, J., and Wagner, M. (2020). On the Creation of risk: framing of microplastics risks in science and media. Global Challeng. 4, 1900010. doi: 10.1002/gch2.201900010
von Bahr, B., and Steen, B. (2003). Reducing epistemological uncertainty in life cycle inventory. J Clean Prod. 12, 369-388. doi: 10.1016/S0959-6526(02)00197-X
Wagner, T. P. (2017). Reducing single-use plastic shopping bags in the USA. Waste Manag.70, 3–12. doi: 10.1016/j.wasman.2017.09.003
Walker, S., and Rothman, R. (2020). Life cycle assessment of bio-based and fossil-based plastic: a review. J. Clean. Prod. 261, 121158. doi: 10.1016/j.jclepro.2020.121158
Walker, T., and McKay, D. (2021). Comment on “five misperceptions surrounding the environmental impacts of single-use plastic). Environ. Sci. Technol. 55, 1339–1340 doi: 10.1021/acs.est.0c07842
Wang, P., Li, W., and Kara, S. (2018). Dynamic life cycle quantification of metallic elements and their circularity, efficiency, and leakages. J. Clean. Prod. 174, 1492–1502. doi: 10.1016/j.jclepro.2017.11.032
Wanichpongpan, W., and Gheewala, S. H. (2007). Life cycle assessment as a decision support tool for landfill gas-to energy projects. J. Clean. Prod. 15, 1819–1826. doi: 10.1016/j.jclepro.2006.06.008
Weidema, B., Wenzel, H., Petersen, C., and Hansen, K. (2004). The product, functional unit and reference flows in LCA. Environmental News 70, 1–46
Wenning, R. J., Apitz, S. E., Kapustka, L., and Seager, T. (2017). The need for resilience in environmental impact assessment. Integr. Environ. Assess. Manag. 13. doi: 10.1002/ieam.1972
Wohner, B., Pauer, E., Heinrich, V., and Tacker, M. (2019). Packaging-Related Food Losses and Waste: An Overview of Drivers and Issues. Sustainability. 11. doi: 10.3390/su11010264
Woods, J. S., Veltman, K., Huijbregts, M. A. J., Verones, F., and Hertwich, E. G. (2016). Towards a meaningful assessment of marine ecological impacts in life cycle assessment (LCA). Environ Int. 89–90, 48–61. doi: 10.1016/j.envint.2015.12.033
Woods, J. S., Verones, F., Jolliet, O., Vázquez-Rowe, I., and Boulay, A.-M. (2021). A framework for the assessment of marine litter impacts in life cycle impact assessment. Ecol. Indic. 129, 107918. doi: 10.1016/j.ecolind.2021.107918
Woods, L., and Bakshi, B. R. (2014). Reusable vs. disposable cups revisited: guidance in life cycle comparisons addressing scenario, model, and parameter uncertainties for the US consumer. Int J Life Cycle Assess 19, 931–940. doi: 10.1007/s11367-013-0697-7
World Economic Forum Ellen MacArthur Foundation, and McKinsey and Company. (2016). The New Plastics Economy - Rethinking the future of plastics. Cowes.
Xie, M., Li, L., Qiao, Q., Sun, Q., and Sun, T. (2011). A comparative study on milk packaging using life cycle assessment: from PA-PE-Al laminate and polyethylene in China. J. Clean. Prod. 19, 2100–2106. doi: 10.1016/j.jclepro.2011.06.022
Xue, L., Liu, G., Parfitt, J., Liu, X., van Herpen, E., Stenmarck, A., et al. (2017). Missing food, missing data? A critical review of global food losses and food waste data. Environ Sci Technol, acs.est.7b00401. doi: 10.1021/acs.est.7b00401
Zheng, J., and Suh, S. (2019). Strategies to reduce the global carbon footprint of plastics. Nat. Clim. Chang. 9, 374–378. doi: 10.1038/s41558-019-0459-z
Zhou, A., Zhang, Y., Xie, S., Chen, Y., Li, X., Wang, J., et al. (2021). Microplastics and their potential effects on the aquaculture systems: a critical review. Rev Aquac 13, 719–733. doi: 10.1111/raq.12496
Keywords: marine litter, comparative LCA, circular economy, plastic bag bans, waste management, reduced consumption
Citation: Miller SA (2022) The capabilities and deficiencies of life cycle assessment to address the plastic problem. Front. Sustain. 3:1007060. doi: 10.3389/frsus.2022.1007060
Received: 29 July 2022; Accepted: 14 November 2022;
Published: 30 November 2022.
Edited by:
Oihane C. Basurko, Technology Center Expert in Marine and Food Innovation (AZTI), SpainReviewed by:
Anne-Marie Boulay, Polytechnique Montréal, CanadaCopyright © 2022 Miller. This is an open-access article distributed under the terms of the Creative Commons Attribution License (CC BY). The use, distribution or reproduction in other forums is permitted, provided the original author(s) and the copyright owner(s) are credited and that the original publication in this journal is cited, in accordance with accepted academic practice. No use, distribution or reproduction is permitted which does not comply with these terms.
*Correspondence: Shelie A. Miller, c2hlbGllbUB1bWljaC5lZHU=
Disclaimer: All claims expressed in this article are solely those of the authors and do not necessarily represent those of their affiliated organizations, or those of the publisher, the editors and the reviewers. Any product that may be evaluated in this article or claim that may be made by its manufacturer is not guaranteed or endorsed by the publisher.
Research integrity at Frontiers
Learn more about the work of our research integrity team to safeguard the quality of each article we publish.