- 1Department of Mechanical and Industrial Engineering, Qatar University, Doha, Qatar
- 2Qatar Transportation and Traffic Safety Center (QTTSC), Qatar University, Doha, Qatar
- 3Department of Civil and Architectural Engineering, Qatar University, Doha, Qatar
- 4Engineering Management, Qatar University, Doha, Qatar
- 5Department of Professions and Professional Groups Studying, Russian Academy of Sciences, Moscow, Russia
Embarking on the World Cup journey with circular collaborative strategies can positively impact the environment and socioeconomic outcomes to prosper development at the center of sustainability. World Cup mega-events are set with overriding priorities in cutting down environmental footprints to accelerate sustainable development across the Fédération Internationale de Football Association movement to leave an enduring legacy post-event in global sports. This paper conducts the first of its kind comprehensive critical analysis on ecological quality in life cycle impact assessment for 2022 Fédération Internationale de Football Association World Cup modular container stadiums in Qatar. A “cradle-to-cradle” life cycle assessment, including the material and resource production, construction, operation, and end-of-life (EOL) phase, is analyzed in this study, taking the case of Ras Abu Aboud stadium. Ecoinvent v3.7.1 life cycle inventory database was used to quantify the ecosystem damage-related impacts. Two scenarios were considered for the operation phase: scenario 1 (single year of operation) and scenario 2 (30 years of operation). A sensitivity analysis was used to understand the extent of impact per category indicator subject to material quantity variations. The results showed that the planned circularity contributed to savings in the EOL phase of more than 4.26 × 107 species.year compared with 1.7 species.year across the overall life-cycle impacts. Several perspective-based circular and sharing economy scenarios were assessed to reveal the benefits of circular collaborative economy applications in leveraging possible ecological burdens before, during, and post-mega events in sustainable construction. This research acts as a backbone for future single-sport mega-events to attempt to transition to a carbon-neutral, fully sustainable event with an everlasting legacy.
Introduction
Background
Organizing the 2022 Fédération Internationale de Football Association (FIFA) World CupTM seals a historical landmark in the epic records of the oil-rich state of Qatar, with profound implications on mass tourism, culture, service provision, construction, and sustainable development (Qatar2022, 2020a). Sustainability has become the major concern in societies worldwide (Kucukvar et al., 2014; Alsarayreh et al., 2020; Kutty et al., 2020a), of mega-events accounting for several positive and negative impacts both locally and globally (Death, 2011). The threats triggered by infrastructure development and mass tourism in mega-events are the associated carbon, material, and ecological footprints that emanate into irreversible environmental (e.g., ecosystem damage and resource depletion) and social impacts (e.g., human health damage) (Ahmed and Pretorius, 2010). Acquiring an egalitarian balance between the infrastructure development standards and regional urban planning directives with global sustainable development agenda 2030 and FIFA World Cup regulations can often be challenging. A circular economy (CE), a paradigm well sough in contrast to the traditional “take, make, dispose” linear economic system, is an answer to bring in balance to the infrastructure development and the associated waste accumulation at multiple stages of the construction value chain (Ellen Macarthur Foundation, 2021). Circularity in the early planning phase of the value chain is required to support sustainable initiatives that guarantee reduced waste at the end of life, with prospects of reuse and recycle to result in zero waste (Onat et al., 2019). Focusing on reutilizing the EOL waste as raw materials for new construction projects can downsize the ecological footprints, a collaborative consumption initiative (Kutty et al., 2020a).
In an attempt to achieve an equitable balance between the socioeconomic outcomes and ecological damages inflicted by mega-events on the host and neighboring territories, the FIFA World Cup 2022 targets to reduce the global carbon and ecological footprint impact, adopting various measures at regional, national, and international levels (Qatar2022, 2020b). One of the propound attempts is the design and construction of modular stadiums (Manni et al., 2018; Al-Hamrani et al., 2021). The Ras Abu Aboud (RAA) Stadium is one of the prominent examples in this respect, with a unique architectural design that will completely be dismantled post-World Cup (Qatar2022, 2020c) to attain sustainable outcomes. However, the ecological impacts of World Cup sporting events have not been fully understood (MezaTalavera et al., 2019). Moreover, how the socioeconomic and cultural impacts can foster sustainable behaviors worldwide through collaborative practices needs to be further explored. Mega-events broadcast socioecological values and practices with carrying out the transition to sustainable behavior by showcasing innovative solutions, using supra-political levers to move toward sustainable living and consumption practices (Liang et al., 2016; Geeraert and Gauthier, 2018). Sharing and repairing activities can foster circular societal shifts at individual and community levels. However, to realize this potential, key actors need to be aware of associated risks and find ways to circumvent those while maximizing the benefits of sharing and repairing (MezaTalavera et al., 2019). On the other hand, concerns have been raised about, for example, public safety, privacy, and limited liability of sharing organizations (Mol and Zhang, 2011).
Integrating mega-sporting events with circular collaborative strategies can positively impact socioeconomic outcomes and prosper development at the center of sustainability (Manni et al., 2018). The state-of-the-art stadiums, demountable infrastructures, energy-efficient mass rapid transit systems, and innovative cooling technologies can support the transition of the host nation to an advanced sustainable circular collaborative economy, with a judicious recycle and reuse strategy pre- and post-event. Reimaging legacy during, before, and after mega-events through sustainable circular collaborative practices is a challenge that comes with impetus opportunities. These untapped opportunities must be untwined through critical analysis of the ecological damage points from the life cycle perspective of a cradle-to-cradle. In this regard, a “cradle-to-cradle” life cycle assessment to measure the midpoint environmental and endpoint ecosystem quality damage point is presented in this article. The reduced ownership and distributed utilization of assets to generate value through collaborative and circular initiatives are also discussed from a socioeconomic perspective in this paper, taking the case of the RAA Stadium, Qatar.
Literature Review
Life Cycle Influence Assessment
Life-cycle assessments (LCA) are methods to identify the existence or cradle-to-grave effect on creating, promoting, transportation, and delivering objects along the system cycle (Ekvall and Finnveden, 2001). The methodology considers structures and secret non-market movements of raw, intermediate product inputs and wastes and other mass and energy processing associated with the “product chain or method” (Ayres and Kneese, 1969). In some instances, the LCA procedure includes comparing a few options that are meant to have a comparable consumer service. The LCA is led to look for responses to questions such as: how to equate energy usage and possible damages of two separate production processes for the same product (Fischer-Kowalski and Weisz, 1999); what are the benefits of technical change; what are the relative impacts to the overall pollution from the various phases of this product life-cycle (Fischer-Kowalski and Haberl, 1993)?
The life cycle influence assessment (LCIA) transforms “stock” sources to simplified metrics. LCIA has two approaches: trouble-based (medium) and harm-based approach (endpoints) (Haas et al., 2005). In a problem-based approach, flows are assembled into ecological issues that fall under specific themes. The themes discussed in most previous LCIA studies include greenhouse impacts, degradation of natural resources, stratospheric depletion, rising temperatures, photochemical ozone production, ocean acidification, human toxicity, and marine toxicity (Haberl et al., 2004). These approaches seek to clarify the scope of several hundred flows to several fields of importance for the community (Weisz, 2006). The approaches that focus on damage often begin by categorizing a scheme's flow into separate ecological topics; however, the design was harmful to each environmental topic as per its impact on human and ecosystem health or damaged assets (Miyazaki et al., 2004; Tadeu et al., 2015). LCIA has been established as a knowledge and context-spreading method for life cycle inventory (LCI) data, relating mainly to power and size (Finnveden, 1997). It does not mean that the goods or devices analyzed have effects because LCI proves that such pollutants are related to some environmental issues or influence groups. However, it ensures that pollutants that lead to a stream of emissions equally known to apply to these environmental concerns or divisions are produced during the life cycle (Huberman and Pearlmutter, 2008). LCIA is thus used as a method to decide the degree to which a specific substance, method, component, or pollution can be related. Furthermore, the midpoint- and endpoint-oriented methods are discussed in Table 1 in detail.
Why Collaborative Circular Economy in Sustainable Construction?
By utilizing enormous quantities of natural resources, the construction industry leads to resource shortages and creates vast quantities of waste that lead to a substantial share of the environmental effects caused by the demand of an increasing world population. Many construction materials require vast quantities of energy and materials supplies (Kapsalis et al., 2019). Nevertheless, these products are either down cycled or scrap after construction. Subsequently, the construction industry can only use a limited proportion of the commercial benefit and strength of the building products (Eberhardt et al., 2019). To satisfy future requirements, the need for increased utilization of services would also increase alongside increasing human needs. By recirculating building materials, the concepts of circulatory economics can theoretically reduce to a minimum of the pending problems arising from the construction industry (Berg et al., 2018). Established mechanic technologies, for instance, may allow for the reassembly of materials and parts through reuse in corresponding construction projects, thus theoretically extending their service life (Elia et al., 2017).
The definition of collaborative circular economy (CCE) has recently been debated at different levels by various groups (Anastasiades et al., 2020). A collaborative economy will be a new owning economy as an “economic system of decentralized networks and marketplaces that unlocks the value of underused assets by matching needs and haves, in ways that bypass traditional middlemen” (Botsman and Rogers, 2011). In meta-review of the CCE concept, formed keys feature for collaborative platforms: (1) core business idea involves the value of unused or underutilized assets, (2) organizations involved in collaboration principles of transparency and authenticity, (3) the providers on the chain supply side should be “valued, respected and empowered,” for making consumers' lives environmentally, economically, and socially better, (4) all customers and providers should have benefited from goods and services access, and (5) CCE platform creating distributed marketplaces, decentralized networks with creating a sense of “collective accountability and mutual benefit” (Botsman and Rogers, 2011). To save energy and encourage the optimal utilization of resources, citing concerns over excessive resource usage in the building industry without respect for external consumption of resources, a paradigm change of the linear economy with sharing goals to the CE model is unavoidable (Adams et al., 2017). The successful move to sustainable construction may be encouraged by CCE being implemented in the construction sector. Despite an early stage of growth in the building industry, CCE's technological contribution in the building industry is rising significantly (Hossain et al., 2020). Moreover, the do-it-yourself methods emphasize the intentional use of digital technology to enhance consumer engagement and involvement in enterprises (Upadhyay et al., 2021a,b). The integration of various life cycle planning and development techniques for materials and grouch elements will provide a clear way to evaluate CCE's efficiency at the project design level and decrease the difficulty of using CCE for the building environment to make it easier for the various organizations in the supply chain to understand (Benachio et al., 2020). It will also help promote the alignment in the constructed environment of a more regularly accepted CCE concept, taking both short- and long-term priorities and benefits into account, fostering coordination among industry stakeholders in the diverse supply chains (Mao et al., 2018). Blockchain technology may aid the CE by lowering transaction costs, improving supply chain performance and interaction, ensuring human rights protection, improving health-care patient privacy and well-being, and lowering carbon emissions (Upadhyay et al., 2021c). Moreover, operational constraints in the multitier supply chain direct organizations' attention toward addressing their supply chain environmental issues (Jæger et al., 2021). Jraisat et al. (2021) discovered focal actor interaction mechanisms at triad levels, and the establishment of their dyads capacities leads to long-term supply chain sustainability (Upadhyay, 2020). Integration and synchronization of green initiatives with operational improvement measures have been highlighted as key aspects in the development of a sustainable green supply chain (Kumar et al., 2019; White et al., 2019).
Codagnone and Martens (2016) provide a good conceptual framework to map the collaborative economy, where they classified sharing platforms into (1) for-profit environmentally responsible companies in the one miming, production and recycling, reusing, and after-using chain, (2) non-profit activities for “true free sharing”—Couchsurfing, BeWelcome, (3) business-to-consumer—B2C, and (4) peer-to-peer—P2P categories. Many P2P platforms are owned and operated by companies, but the main actors are citizens (e.g., Uber, Airbnb, and Upwork). Theories of sustainable behavior have studied a complex of motivation, environmentally oriented actions, and values that reduce the burden on the environment, based on the principles of the theory of rational choice and behaviorism (Koger and Winter, 2011). There are Theory of Planned Behavior (Ajzen, 1991), the Norms Activation theory, which provides for behavior change through the rational rethinking of constructive and deconstructive practices for nature as a personal benefit for the individual (Schwartz, 1977), and the value–belief–norm model, which explores the balance between “egocentric” and “biospherical” values (Stern, 2005). Authors of these theories proposed that people always have a choice between constructive (saving nature) and destructive actions. This choice is determined by the time and resource costs, the efforts that need to be made to take environmental actions, and the determining factor here is what people consider rational for themselves (i.e., the values of an economy of unlimited growth or a green economy values, which will be the basis for the actions taken) (Kollmuss and Agyeman, 2002). Summarizing the meta-studies, it is possible to say that the higher the level of convenience of the technological infrastructure and the greater the control by institutions, as well as the higher the level of an individual's sense of personal responsibility for the actions performed, the more likely it is to practice ecological–sustainable practices and strengthened environmentally friendly norms of behavior are (Steg and Vlek, 2009; Chernovich, 2013). Mega-events act as an institution where it becomes possible to model from scratch a technologically convenient infrastructure that solves local social, environmental, and economic issues, creating new social and environmental practices throughout the life cycle through a system of sanctions and rewards affecting streamlining the process, ensuring proper levels of “comfort” characteristic for different categories of citizens.
The priority of using the CCE models gives opportunities for expanding and extending the life cycle. Policies for a CE have the potential to incentivize and change the behavior of both consumers and companies toward CE goals. Such networks enable partnerships and interactions across the value chain, and they can also be catalysts for change. CCE approach promotes equality in the provision of services both for participants with high economic and cultural capital and for the most disadvantaged social groups (Petropoulos, 2016; Nußholz, 2017).
Ecosystem Damage Assessment for Circular Economy Applications
Globally, habitats are at risk (Zink and Geyer, 2017; Onat et al., 2020; Al-Buenain et al., 2021). Damaged habitats are caused by the extinction of biodiversity within the environment, by degradation, and/or by the food web (Kutty et al., 2020b). Due to all animals living in dynamic, interdependent environments, removing or modifying one species or abiotic factors hold detrimental effects within the environment and for others (Murray et al., 2017). Industry requests for guidance in adopting sustainable growth policies have long been made (Park et al., 2010; Elhmoud and Kutty, 2021). The triple bottom line method prioritizes operational improvement, decreases greenhouse gas emissions, and enhances the social welfare of the general public (Laing et al., 2019). The CE is the latest effort to comprehend sustainable convergence between economic development and environmental well-being (Geng and Doberstein, 2008; Nuñez-Cacho et al., 2018). Digitalization might be extremely beneficial in the development of circular products that are both sustainable and circular. Furthermore, customers must be involved in the development of new, sustainable circular goods utilizing digitalization (Agrawal et al., 2021).
Determination of damage is a preliminary on-site measurement of an accident or natural disaster damage or failure. Damage evaluations document the extent, repair, reconstruction, or recovery of damage. The time taken for maintenance, replacement, and regeneration can also be measured (Itsubo et al., 2004). Human beings deliberately and unexpectedly change habitats and, consequently, impact human well-being [Sustainable Development Goals (SDG) 3] on the size of the environment. There is growing consensus that interventions (a) connect human behavior with probable changes in the environments and (b) link changes to consequent changes in human well-being are required for informed environmental management and policy (Ghisellini et al., 2016). Although the CE stresses the renovation of systems and resource cycling that can help make business models more competitive, it also encompasses the conflicts and boundaries (Huysveld et al., 2019). The CE opposes the produce–use–dispose paradigm and encourages resource reuse (Upadhyay et al., 2021d). This involves the lack of a social component, which restricts the ethical dimension and specific unforeseen implications of sustainable development (Saldarriaga-Hernandez et al., 2020). Social components, including social health (physical and psychological), depending on the environmental and economic conditions, the predominated balance of constructive sustainable social practices, values, and attitudes that save the environment and deconstructive in local society, determined quality of life and level of well-being. Well-being (expressed by the human development index of a country) levels off at a certain level of resource use (expressed by the material footprint of the country), showing that from a certain high level of well-being, additional resource consumption no longer improves the level of well-being, but the quality of the environment, solidarity, and safety made a contribution for the wellness and health (Tukker et al., 2014).
Evolutionary theories explain the desire for richness, a distinctive genetic program of behavior for human communities due to mining, accumulating, producing, and consuming necessary materials for societal survival. However, the societies whose values are based on the balanced cooperative values and lean production, services, carrying out sharing practices and benefits, creating a network of communications, developed faster in long-term wellness and richness perspective in comparing with societies that were built only on the values of growth, i.e., the accumulation of wealth and the consumption of resources. In other words, the higher the quality of CCE practices (environmental friendliness and sharing values), the higher the resource efficiency of the economy, and a lower burden on the environment, ecosystems are more resilient, the carrying capacity of the environment is higher, environmental crises are overcome faster, and less the level of social inequality (Buss, 2019). CCE helps secure global resource availability in recognizing values of the social well-being, preserve the ability of natural systems to deliver goods and services to society, create new green developing stimulus and technologies, new norms and institutions, encourages redesigning of policy and regulation, generate CCE value to society directly via stimulation of employment and benefits associated with boosting of the recovery, recycling and upgrading of valuable materials; creating new business models and eco-design systems that facilitate circularity, further developing clean and sustainable raw materials extraction and upgrading processes required to underpin healthy social systems (Jackson, 2009). This contributes to a modern concept of the CE as a monetary system that designs and manages both mechanism and performance to optimize ecological integrity and social health, such as scheduling, resources, sourcing, development, and reuse (Genovese et al., 2017).
The latest usage of energy and low-carbon development policies, which involve the introduction of the CE model, is motivated by challenges to balance industrial innovations, environmental and human health, and economic growth in China and elsewhere in the world (Prieto-Sandoval et al., 2018). A core topic of the CE principle is the determination of resources within a locked framework to ensure the use of natural resources while mitigating emissions or preventing limits on resources and maintaining economic development (Jun and Xiang, 2011; Sauvé et al., 2016). For example, to evaluate the effect of the Deepwater Horizon oil spillage on ecological resources, it is essential to determine how the ecosystem transition has occurred in the accident and how these changes have contributed to changes in ecosystem services provision and benefit (Atiku, 2020). To calculate such shifts, the disparity in ecosystem resource provision and value with vs. without must be calculated (Nakano et al., 2007). Moreover, circular practices support sustainability by helping businesses get the most out of limited resources and achieve zero waste goals. Better customer experience may also be obtained through service innovation that has a lower environmental effect (Upadhyay et al., 2019, 2020).
Secondly, how do improvements in ecological form and composition result in ecosystem service provision changes? The “ecological production functions,” as defined by the composition and operation of ecosystems, are used to characterize the provision of ecosystem services (Errington et al., 2018; Morseletto, 2020; Spanos et al., 2021). They can be used as a “transfer function” or model indicative of ecological dynamics to achieve an impact on ecosystem resources through ecosystem changes in ecosystem conditions (e.g., habitat loss and shrimp fishery yields). Environmental output functions could be used to forecast the evolving ecosystem structure and functioning of the distribution of different ecosystem services for all stakeholders participating in the economic system (Robertson et al., 2007; Winans et al., 2017).
State-of-the-Art and Objectives
Qatar's obligation to deliver FIFA World Cup™ 2022 as a carbon-neutral event has set standards for environmental leadership by managing the existing water and waste management practices, applying sustainable building standards, and implementing solutions with low CO2 emissions. Reducing an ecological burden from the carbon-gushing games requires CCE strategies that give way to new thinking on environmental effectiveness and economic efficiency to sustain legacy in global sports. To determine the environmental effect of a commodity in various ways, the LCA requires comprehensive techniques (Sleeswijk et al., 2008) to quantity impacts across various protected areas. There is a need for approaches to easily produce understandable and easy-to-understand outcomes for regular decisions in better understanding the impacts from a broader viewpoint. In light of the environmental problems posed by the consumer community, the study uses the ReciPe LCIA model, built as a method for resolving the burden inflicted on specific areas of protection. The ReciPe method is best regarded for its vast coverage of impact category indicators when compared with different methods such as EPS 2000, IMPACT (2002+), and Eco-indicator (99). This study is the first of its kind to understand the impact of modular stadium construction on the ecosystem from a life cycle perspective using the ReciPe method.
To continue, an issue associated with inventory items in LCA is the synergistic nature of some compounds. The synergistic effect of mixed compounds may increase the concern about the original compound or create a new compound(s) that is not captured in the inventory. Such synergistic compounds may have the potential to create combined impacts greater than those of the individual releases. This study ignores the synergistic compounds in the inventory, but due concern is given in recognizing the potential effects and other factors (e.g., antagonistic effects and assimilative capacity) when drawing conclusions based on the results of the ecological life cycle assessment conducted in the study. In addition, the fundamental terms used in ecological impact assessment arise confusions; for example, distinguishing between an inventory item and an impact is not always easy. Although the common practice is to account for the amount of solid waste materials produced by a system in inventory analysis, it is not common to account for the amount of natural habitat consumed to dispose of that solid waste. Accounting to a CE strategy, the amount of construction waste materials is considered as an input under the cradle-to-cradle assumption, while also classified as an impact on the ecosystem. Furthermore, most studies to date have just conducted life cycle assessments with supporting policy recommendations when it comes to understanding the impacts of green design infrastructures on the environment. None has yet focused on bringing CCE as a potential leverage source to minimize the impact on the ecosystem. This study brings out a perspective analysis from a CCE strategy for sustainable construction practices for World Cup mega-events to lessen the burden on the ecosystem. Despite best efforts, to green, the FIFA World Cup is challenging and requires critical analysis on possible burdens and leverage points to leave a long-lasting legacy. To this end, this paper aims to achieve the following objectives, namely:
a) Conduct a “cradle-to-cradle” life cycle assessment to quantify the midpoint environmental and endpoint ecosystem damage for the case of sustainable modular stadium designs using the ReciPe method considering the RAA Stadium as a case study.
b) Identify the most influencing impact category that inflicts possible damage to the ecosystem across each life cycle phase along with the material/activity that holds a significant impact across each impact indicator and the life cycle stage that inflicts most damage to the ecological diversity.
c) Investigate the extent of impact per category indicator subject to material quantity variations using a simulation-based sensitivity analysis.
d) Carry out a perspective analysis on how a circular collaborative economy can reduce possible ecological burdens before, during, and post-World Cup with possible alternative recommendations and strategies to achieve sustainable development.
Research Method
Method
The study adopts the conceptual framework developed by the United Nations Environment Programme (UNEP) and the Society of Environmental Toxicology and Chemistry guidelines for environmental life cycle assessment (E-LCA) to quantify the endpoint ecological quality for sustainable modular stadium designs taking the case of the RAA Stadium in the State of Qatar. The three-phase conceptual framework contains the following activities, namely:
1. Classification: the process of assignment and initial aggregation of life cycle inventory data to relatively homogeneous groupings of impacts (e.g., photochemical smog, lung disease, and fossil fuel depletion) within primary impact categories (e.g., ecosystem, human health, and natural resources).
2. Characterization: the qualitative and/or quantitative evaluation of potential impacts. The process of identifying impacts of concern (called assessment endpoints) and selecting actual or surrogate characteristics (called measurement endpoints) to describe the characterization of the impact involves using specific impact assessment models to develop impact descriptors.
3. Valuation: The explicit and collective process of assigning relative values and/or weights to impacts using informal or formal valuation methods.
The entire study process can be seen from the research flow diagram in Figure 1. Here, the first phase begins by identifying the goal and scope of the assessment and indicating the system boundaries and functional unit; the second phase is the life cycle inventory analysis, which involves data collection; the third phase is the LCIA, in which both the midpoint impact category indicators and the endpoint area of protection are selected; finally, the fourth phase covers the analysis and interpretation of results from the LCIA outputs.
Ras Abu Aboud Stadium: A Case Study
The RAA Stadium is the first of its kind sustainable modular stadium design in the World Cup history to host the 2022 carbon-neutral FIFA World Cup in Qatar's oil-rich state. Located on the waterfronts of West Bay with a seating capacity of 40,000, the RAA Stadium uses shipping containers and removable seats as modular blocks for construction that can be repurposed and dismantled after the mega-sporting event. These parts are intended to be used in community facilities such as hospitals and other projects, whether sports-related or not, both locally and abroad. From a sustainability perspective, the modular and prefabricated elements used in construction will seek to reduce the waste generated, carbon emissions, and the total amount of materials necessary for construction. Moreover, with the reuse of the seats, roof, and other parts of the stadium in developing countries, a positive legacy for Qatar will be established for years and even decades to come (FIFA, 2020).
System Boundary and Functional Unit
The study adopts a “cradle-to-cradle” E-LCA to quantify the associated endpoint ecosystem damage across each impact category. Four life cycle phases were included in the study, namely, (a) raw material production phase, (b) construction phase, (c) operations phase, and (d) EOL phase. The first phase involves the manufacturing and production of construction materials, pipeline systems, openings, and finishing materials. This phase also involves the burden avoided by the recycled materials, which were used in the production process of several materials. The construction phase covers the total diesel consumed by heavy equipment and during the transportation processes of materials to the site and the freight transport of shipping containers through waterways.
Furthermore, the consumed electrical energy, the consumed water, solid wastes, and wastewater generation were covered in both the construction and the operation phases, knowing that the specified unit processes in the operation phase shown in Figure 2 were determined on an annual basis. Because the RAA Stadium is the first fully demountable stadium in the World Cup history, the EOL phase will cover some circularity and sharing economy scenarios for the reuse, repurpose, recycling, and refabrication of the stadium's components other purposes. The study considers the entire stadium area of 450,000 square-meter as the functional unit.
Inventory Data of Life Cycle
According to the requirement of the international standards, ISO 14.040 (International Organization for Standardization, 2006a) and ISO 14.044 (International Organization for Standardization, 2006b) series, the LCIA second phase in the LCA methodology, which contains the understanding and quantification of all physical energy and materials, flows input and output of the system. The actual data for the RAA Stadium for all the stages of the life cycle were obtained from the World Cup local organizing committee: Supreme Committee of Delivery and Legacy, State of Qatar. Life cycle inventory data are represented in Table 2. In the material production phase, the material quantities are measured in kilograms and are categorized into recycled and virgin (non-recycled) raw material amounts. Furthermore, the construction phase includes the data for energy consumption of electricity and water, fuel utilization, freight transportation, and waste generated. As for the operation phase, it is important to note that because the RAA Stadium is currently under construction, the operational data were estimated by the Supreme Committee based on the operation of Al-Janoub Stadium, Doha-Qatar, a stadium with a seating capacity of 40,000, equal to that of the RAA Stadium.
Life Cycle Impact Assessment
The LCIA transforms “stock” sources into simplified metrics. The study uses the ReCiPe 2008 impact assessment model for the endpoint area of protection “ecosystem quality (EQ).” The primary purpose of the ReCiPe process is to render a small number of predictor outcomes of the long list of life cycle inventories (Zanghelini et al., 2016). These metrics display the relative magnitude of a type of environmental effect. The endpoint impact assessment covers all the category indicators along the “cause–effect” chain from the LCI results to the respective area of protection (Park et al., 2016). The endpoint categories are divided into three groups based on their effect or damage inflicted on human health (HH), ecosystem diversity (ED), and resource availability (RA). The preliminary framework to characterize the measurement endpoints of specific inventory items to impacts was identified under the tier 5 type characterization model proposed in the 1992 SETAC Life-Cycle Impact analysis guide (see SETAC, 1993). Here, the site-specific ecological or human health-related information of the RAA Stadium is used to estimate the potential impacts of the inventory items. This study considers nine midpoint environmental impact category indicators, which are further converted into the endpoint area of protection: ecosystem diversity. The midpoint impact categories include climate change (CC), marine ecotoxicity (ME), terrestrial acidification (TA), urban land occupation (ULO), freshwater eutrophication (FEU), freshwater ecotoxicity (FET), terrestrial ecotoxicity (TE), agricultural land occupation (ALO), and natural land transformation (NLT).
The next stage in the LCA is to convert the LCI results to the ecosystem impact category so as to identify the possible impact on ecological quality. The life cycle impact assessment database Ecoinvent v3.7.1 developed by the Swiss Center was used for the analysis. The database offers LCI data covering building materials, renewable fibers, metals, chemicals, and energy (e.g., solar heat, wind power, and electricity) (Frischknecht et al., 2007). The study uses the ReCiPe midpoint (E, A) method, where the midpoint characterization factors are converted to the endpoint characterization factor using conversion factors obtained from the ReciPe 2008 handbook on LCIA (Goedkoop et al., 2008). In ReCiPe, each method (midpoint and endpoint) contains factors according to the three cultural perspectives. These perspectives, namely individualist (I), hierarchist (H), and egalitarian (E), represent a set of choices on issues such as time or expectations that proper management or future technology development can avoid future damages. The study uses the egalitarian cultural perspective, a long-term based on precautionary principle thinking, here the CCE thinking principle as a sustainable long-term solution to avoid damage.
The calculations to quantify the endpoint impact damage on ecological quality through an E-LCIA is as follows:
a) Choose the midpoint characterization factor (CFm) for each environmental impact category under the ReciPe midpoint (E, A) from the Ecoinvent v3.7.1 LCIA database for the selected activity.
b) Calculate the midpoint impact across each impact category (Mi,a,x) for quantity (Q) under each activity in the life cycle stage according to Equation 1
where,
Mi,a,x = the midpoint impact of ith impact indicator under the area of protection “a” using the uncertainty perspective “x.” Here, a and x represent ecosystem damage as the area of protection and egalitarian as the uncertainty perspective, respectively.
c) Evaluate the endpoint damage on the ecosystem across each impact category for Q quantity using the appropriate conversion factor (F) according to Equation 2
where,
Ei,a,x = endpoint impact of the ith impact indicator under the area of protection “a” using the uncertainty perspective “x.”
FM→E = midpoint to endpoint conversion factor obtained from ReciPe 2008 handbook on LCIA. Table 3 shows the ReCipe impact category indicators, conversion factors (from midpoint to endpoint), and units of conversion used in this study.
d) Calculate the overall endpoint damage across each life cycle stage following Equation 3
where,
E′j,a,x = endpoint impact of the jth life cycle phase under the area of protection “a” using the uncertainty perspective “x.”
n = number of environmental impact categories considered in the study.
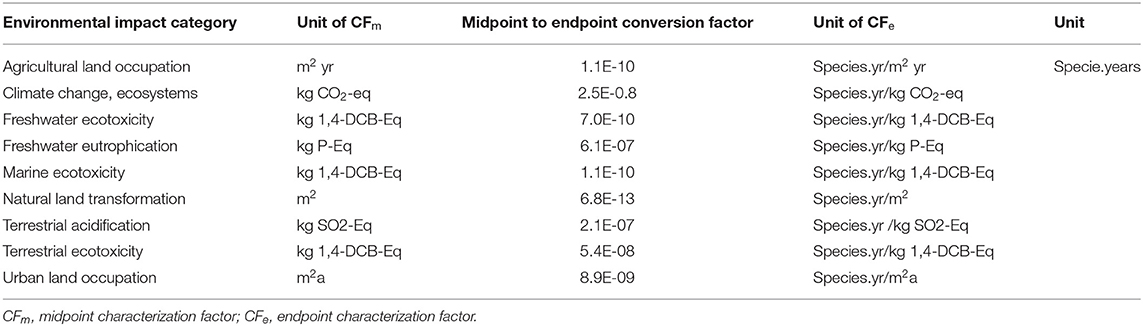
Table 3. ReCipe impact category indicators, conversion factors, and units of conversion used in this study.
Results and Discussion
Ecosystem Damage Assessment: A Life Cycle Approach
To find the ecosystem damage (in species.year) for sustainable modular stadiums taking the case of the RAA Stadium in Qatar, nine endpoint impact categories as stated in Life cycle impact assessment were assessed. These categories were studied through the stadium's life cycle stages: production, construction, operation, and EOL to identify the most influential life cycle stage and material/activity and to help drive conclusions and policy recommendations and highlight areas of improvement for reduced ecological impacts to the environment. Two scenarios were adopted for the life cycle analysis, enabling the comparison of possible savings in operations. Scenario 1 considers a circular approach with a single year of operation, after which the stadium would be dismantled post-FIFA 2022. Scenario 2 considers the standard approach with a 30-year operation, assuming that the RAA Stadium will remain for a life span of 30 years before decommissioning (Frawley and Adair, 2013).
Scenario 1 analysis revealed that the production phase is the most dominant, contributing 99.99% across all life cycle phases. This domination can be linked to the negative environmental impact of certain materials used within the stadium construction; detailed impact analysis for the materials used within RAA stadium will be investigated in the next section. Additionally, Figure 3A shows that CC and ME dominate all impacts categories across all the life cycle phases with a contribution ranging from 80 to 95%. The possible reasons could be due to greenhouse gas (GHG) emissions contributing to the CC impact category and possible acid rain, as ME is well related to the GHG emissions, or due to dumping harmful chemicals at the waterfronts during the life cycle.
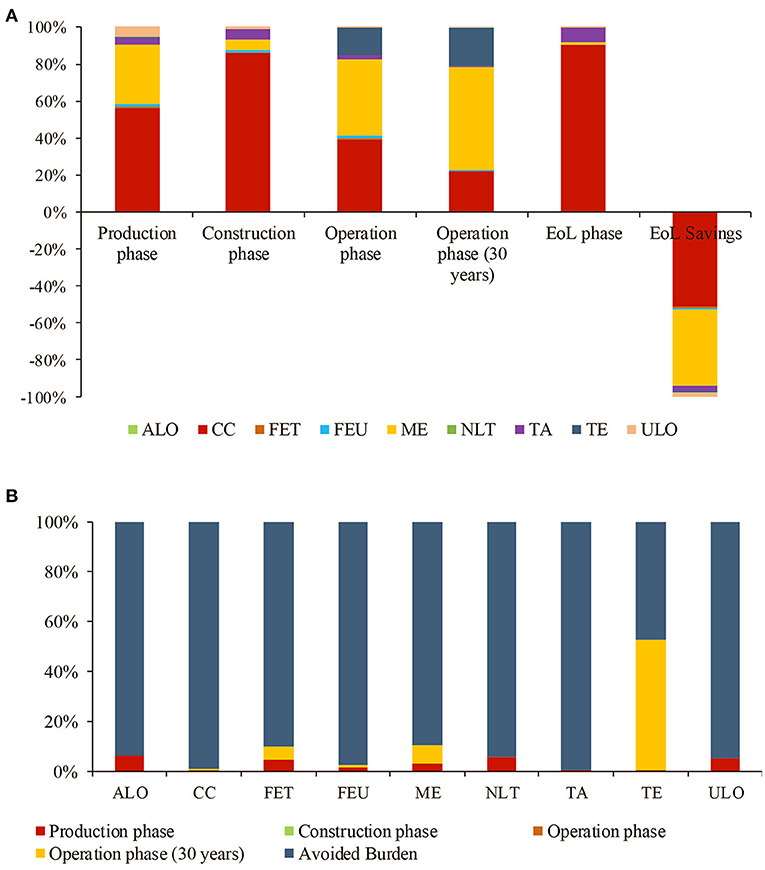
Figure 3. Percentage contribution of life cycle phases across each endpoint impact category. (A) Impact across each life cycle phase and the EOL savings. (B) Avoided burden against different life cycle phases.
On the other hand, Scenario 2 analysis revealed the dominance of both production and operation phases with the following comparative combinations of 99.78:0.21, 62.31:37.69, 46.63:53.40, 65.20:34.80, 27.04:72.96, 99.06:0.94, 82.04:17.96, 0.73:99.30, and 97.68:2.31% corresponding to the following impact categories ALO, CC, FET, FEU, ME, NLT, TA, TE, and ULO, respectively. The project's planned circularity contributed to saving at the EOL that reached more than 4.26 × 107 species.year compared with 1.7 species.year for the overall life cycle with a considerable burden avoided across the CC and ME impact categories, as shown in Figure 3A.
Additionally, using recycled materials within the stadium construction materials avoided more than 50% of the environmental burden across the overall impact categories (Figure 3B), which emphasizes the positive influence of using recycled materials during construction on the stadium's overall construction cost and the environment.
Further investigation conducted for the materials influencing each impact category across each life cycle phase revealed that concrete is the most influential material across the nine endpoint ecological impact categories, with a contribution ranging from 98.67 to 99.95%. Moreover, concrete has the highest avoided burden among all materials, as shown in Figure 4A. The remaining materials have a minor influence, and only the average metal product is significant among all the remaining 34 materials, contributing within a range from 0.02 to 1.26%.
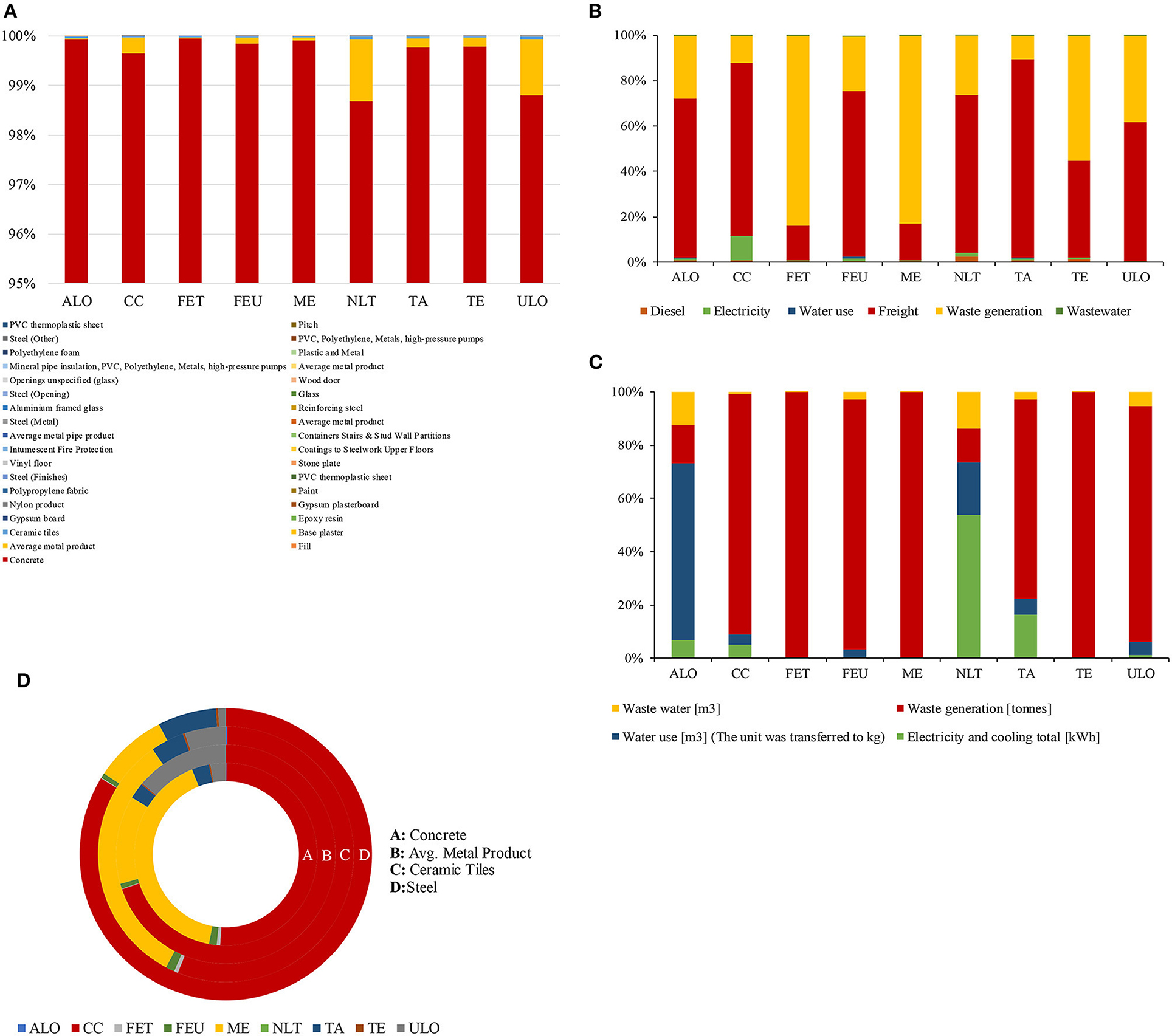
Figure 4. (A) Material contribution in the resource production phase. (B) Contribution of activities in the construction phase. (C) Contribution of activities in the operations phase. (D) Contribution of materials across each impact category per phase.
On the other hand, the two primary causative activities among the impact categories are freight and waste generation in the construction phase, as shown in Figure 4B. Freight contributes significantly across the CC impact category with an endpoint value of 7.97 × 10−1 species.year and a contribution of 76.22%. GHG emissions play a role in impacting CC due to the standard shipping methods that rely on non-renewable energy sources, as most of the construction materials are imported from outside the State of Qatar. Freight's influence on FET and TA ranges between 15.17 and 87.60%, respectively. The second-ranked influencer for the construction phase is the waste generation with a value of 1.28 × 10−1 species.year for CC (12.26%); due to the lack of a complete recycling process for all the construction waste, most of the construction waste ends up in landfills. Waste generation contributes ~15.17 and 87.60% for FET and TA, respectively. The remaining activities hold a negligible impact of <1%.
Furthermore, all the activities contribute differently across each impact category for the operation phase. The activities contributing across each impact category is as follows: “water used” across ALO (with a contribution of 66.29%), waste generation impacting CC, FET, FEU, ME, TA, TE, and ULO (with contributions of 90.35, 99.73, 93.97, 99.78, 74.74, 99.97, and 88.50%, respectively), and “electricity and cooling” impacting NLT (with a contribution of 53.57%), as shown in Figure 4C. However, the highest value of impact was for the “waste generation” across the CC impact category with a value of 1.17 × 10−2 species.year; the reasons match the one's highlighted in the operation phase.
Finally, in the EOL phase, we focus on net savings. The dominating material is again concrete with a significant saving across CC, for a value of 2.06 × 107 species.year. The savings range from a value of 98.67 to 99.95% for NLT and FET, respectively. The most savings were for the impact category CC, 51 to 84% for concrete and steel, followed by the impact category ME with a range of saving from 8 to 41% for steel and concrete, as indicated in Figure 4D. These substantial EOL savings are due to possible reuse/recycle strategies instead of completely using the virgin concrete structures.
Sensitivity Analysis
The level of sensitivity for each environmental indicator across all the life cycle stages subject to possible variations in the quantity of the material used was identified through a sensitivity analysis. Tableau v2021.1 software was used to conduct the sensitivity study. Under a probabilistic scenario, each material quantity across the respective life cycle stage that contributed significantly to the ecosystem damage endpoint was chosen as the sensitivity analysis's input parameters. In the production phase, the respective quantities for concrete, average metal product, ceramic tiles, and steel were subject to a simultaneous increase (+) or decreased (-) in quantities by ±10, ±30, and ±50%. All the other material quantities were kept constant due to their negligible contribution to the ecosystem damage endpoint as identified through the LCIA analysis. Varying these materials will not bring any significant change in the sensitivity study and may even bias the analysis results. A tornado chart shows the impact of the set input parameters on the response variables. The bars in the tornado chart show the change in the response variable (environmental impact category). The impact category at the top has the biggest effect on the response variable, whereas the one at the bottom has the least effect. Results showing the sensitivity of each environmental impact category to changes in the quantity of materials in the material production phase can be seen in Table 4. The sensitivity analysis results (see Figure 5) reveal CC as the impact category most sensitive to changes in material quantities in the raw material production phase. ME is the second most sensitive impact category followed by TA, ULO, FEU, FET, TE, ALO, and NLT as the least sensitive environmental impact category to possible volumetric changes in quantity.
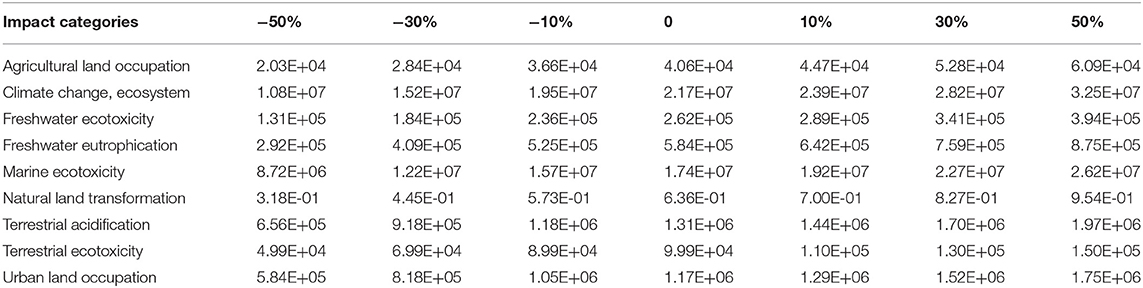
Table 4. Sensitivity analysis results for quantitative variations across each endpoint impact category for production phase (in species.yr).
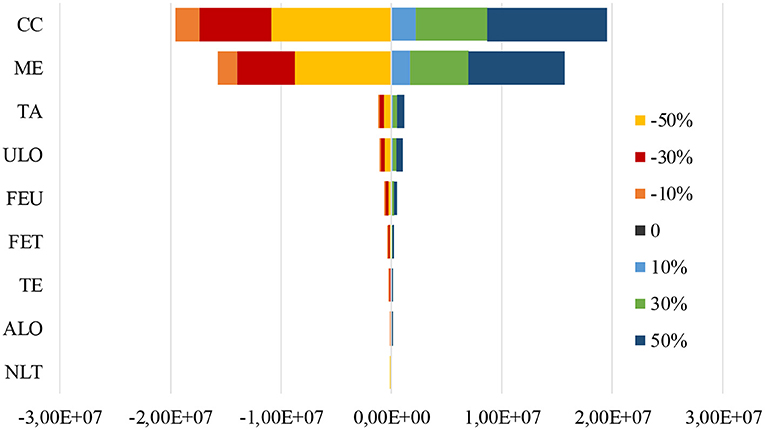
Figure 5. Results of sensitivity analysis across environmental impact categories due to change in material quantity in production phase.
The sensitivity analysis results across the construction and operation stages for each impact category to changes in the material quantity as depicted in Tables 5, 6, respectively. In the construction phase, freight (tkm) and waste (kg) were subject to quantitative changes by ±10, ±30, and ±50%. The other materials were kept constant. It was observed that CC is most sensitive to volumetric changes in the construction phase (Figure 6). The second most sensitive impact group inflicting damage to the ecosystem quality with possible changes in material quantity in the construction phase is the ME, followed by TA, ULO, FEU, FET, TE, ALO, and NLT (in decreasing order of sensitivity). When considering the operations phase, climate change is still the most sensitive impact category subject to quantitative variations followed by natural land transformation, marine ecotoxicity, terrestrial ecotoxicity, terrestrial acidification, freshwater eutrophication, urban land occupation and freshwater ecotoxicity, and agricultural land occupation. In this phase, the analysis was carried out by varying electricity and cooling (kWh) and waste generation (tons) quantities while keeping other inventory items constant. As explained earlier, these elements resulted in inflicting significant damage to the ecosystem endpoint across each impact category, as observed from the LCIA. Results of sensitivity analysis across environmental impact categories due to change in item volume in the operations phase can be seen from the tornado diagram in Figure 7.
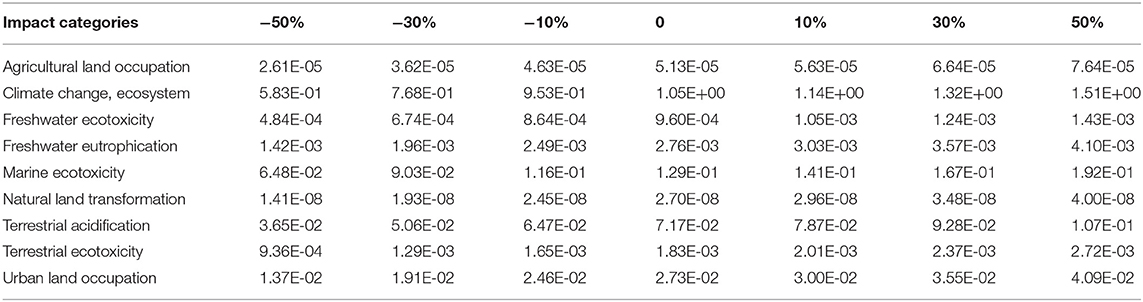
Table 5. Sensitivity analysis results for quantitative variations across each endpoint impact category for construction phase (in species.yr).
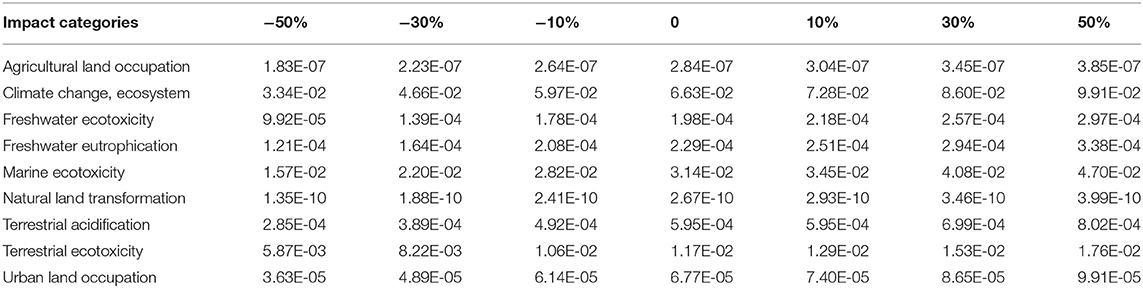
Table 6. Sensitivity analysis results for quantitative variations across each endpoint impact category for operations phase (in species.yr).
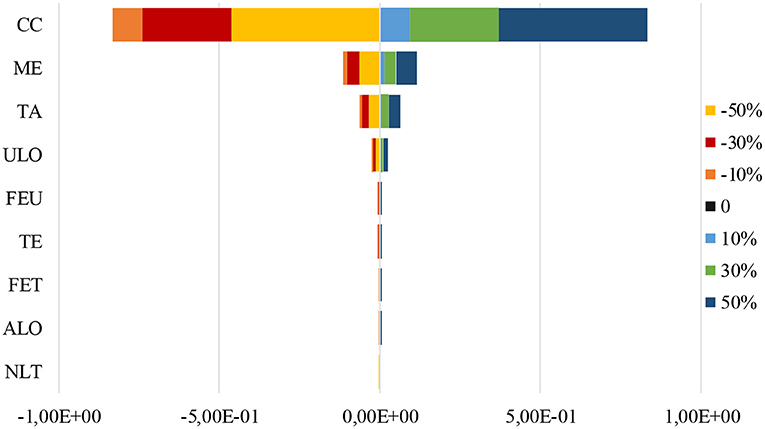
Figure 6. Sensitivity analysis results across environmental influence categories due to changes in item volume in the construction phase.
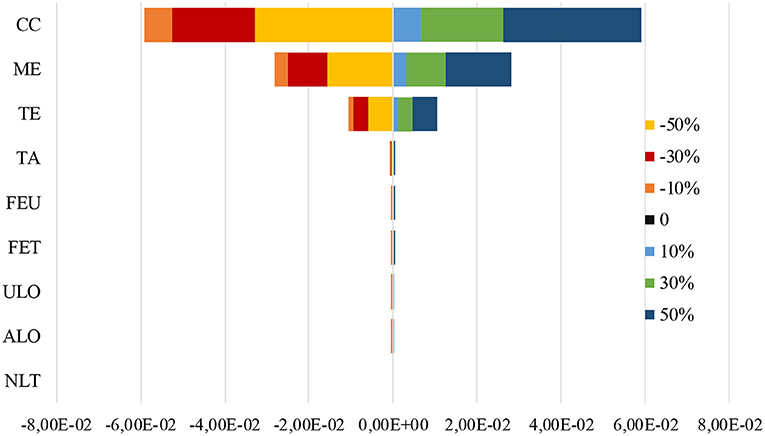
Figure 7. Sensitivity analysis results across environmental influence categories due to change in item volume in operations phase.
Rethinking Ecological Quality Through CCE: A Perspective Analysis
An EOL management from a CCE perspective besides the waste treatment alternative as argued in Ecosystem damage assessment: a life cycle approach is analyzed considering before, during, and post-event scenarios. Facilitating the football pitches laid out in the stadiums of Qatar as training grounds for various football associations in parts of Asia and the Middle East can help enhance the sportive spirit partnership (SDG: 17) goals of Qatar, a shift toward sharing economy goals. Such readily available training grounds can help many football nations to take advantage of the existing infrastructures to power up their level of excellence in football. Subtropical humid climates often hold a negative impact on sporting events. Countries such as India and China, which have magnificent infrastructures in place for mega-events, often face difficulty scrolling up the list of host cities during bids due to their adverse humid climate, which can bring overheating concerns to the players. Transferring the innovative temperature control technology used in the FIFA 2022 World CupTM stadiums to various such national league member states in Asia-pacific and Africa can help improve players' performance and the game as a whole. This supports the United Nations global technology transfer initiative and strengthens the transboundary partnership goals of UN SDGs (SDG: 17).
Reusing shipping containers reduces the burden on the environment, with a considerable reduction of 29% in CO2 when shared and circulated within the economy (Gilbert et al., 2017; Kucukvar et al., 2021). This concept falls under the umbrella term CCE. For instance, repurposing shipping containers to “container gardens and greenhouses” helps in balancing the emissions generated during the event (SDG: 13) with reducing the cost and materials involved in constructing new greenhouse structures (SDG: 12). This ensures proper utilization of resources through sharing practices. A similar approach can be utilized by repurposing, refurbishing, and recycling the used shipping containers to bring circularity and sharing economy practices into action to maintain post-event legacy and sustainability. Further applications of shipping containers post-event mapped with corresponding SDGs is presented in Figure 8. As in many sports events, preventive actions to reduce waste and waste controlling and measuring play a significant role as a sustainability advantage (Rajan and Booth, 2016; Kutty and Abdalla, 2020). Atchariyasopon (2017) concluded that <15% of waste generated during sporting events made it to the recycling process. The rest of the commingled solid waste went to landfills and generated unnecessary GHG emissions. Therefore, the implementation of circular solid waste management in mega-sports events has to follow the waste management strategy of sorting, collecting, recycling, composting, energy recovering, treating, and disposing.
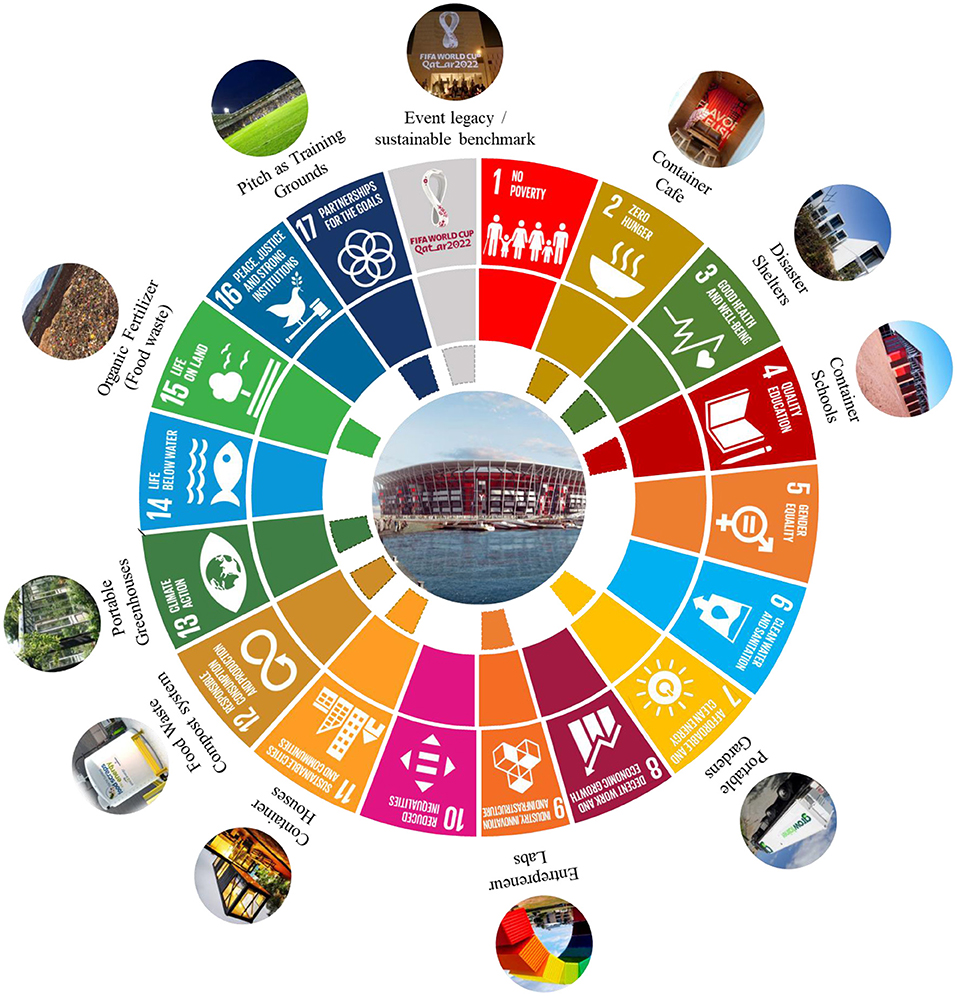
Figure 8. Benefits of modular stadium design to leverage ecological burden, aligned to UN SDGs and LOC agenda.
Participating and encouraging fans' in-stadium schemes for recycling can be challenging. Fans' engagement, attention, and awareness need operational efforts to reduce an environmental footprint, reduce stadium's waste, and an ecosystem damage reduction strategy through a participatory approach. Marketing campaigns and a video promoted on social media broadcasted on big screens during the event can be the most effective ways to introduce the home crew. This enables knowledge sharing through crowdsourced platforms to harmonize sustainability in the FIFA initiative. The seats from modular stadiums like the RAA Stadium can be 100% recycled, of which 10% have already been recovered from the ocean for its current state design (SDG: 14). This will enhance the CE's value by using reused plastic from the hometown's waste to manufacture new seating platforms. Hence, adopting strategic waste management and recycling that, a CE initiative, would support the host nation in maintaining post-event legacy and meeting sustainability targets and goals.
Reusing wastewater and water conservation lead to significant environmental benefits, developing from decreases in wastewater discharge and diversion impacts on environmental water quality (SDG: 6, SDG: 7, SDG: 14). To bring out a balance to the anticipated ecological deterioration during the event, Qatar can implement policies to improve the efficiency of water, lessen the consumption per capita, and maximize recycling of treated industrial and sewage water for district cooling services, construction works, landscaping, and garden irrigation. Hence, adopting strategic wastewater management and circularity schemes can reduce the burden on the environment. Similarly, the CO2 emissions in building materials (embodied CO2) developed by burning fossil fuels result in significant GHG emissions (~50%) (Primasetra, 2019). Therefore, the host nation needs to decrease the influences associated with embodied CO2 emissions; one way is to use reused and eco-friendly materials for construction due to their low embodied energy. The materials used in modular stadiums can be reused once dismantled for several other building purposes with the State of Qatar. This creates a shared use of resources and a possible resource allocation. Reusing material is a part of designing a sustainable stadium that can significantly impact the environment. The material used in this process shall be scheduled at the beginning of the design phase, which can be found in the electrical fitting, flooring, finishes, etc. of the stadium (SDG: 11, SDG: 12). Planning is essential to maintain sustainability long after the event.
Approximately 60 tons of waste by game can be produced. However, through proper recycling and composting systems, more than 95% of this waste will be removed from landfills. The recyclable or compostable materials can be utilized based on extensive selection and agreement with stadium vendors, which is the best way to accomplish this task (Aquino and Nawari, 2015). For example, the cyclopean concrete (CYC) methodology can be utilized, incorporating excavated boulders from the site within the concrete mix for under-raft foundation casting for future stadium designs. In comparison with the traditional concrete casting approach, this approach reduces the environmental and economic burdens. The study concluded that GHG emissions were reduced by ~32% by adapting the CYC technique in Al- Jonoub Stadium in Qatar. Therefore, the CYC approach achieves the vital low-cost and unconventional material from waste crops existing in Qatar, enhancing the lesser environmental influence than the traditional concrete casting (Al-Hamrani et al., 2021). Thus, understanding and rethinking ecological quality through possibilities of the circular collaborative economy can considerably reduce the ecosystem damage to achieve sustainable development goals for mega-event hosts long after the event.
In addition, following CCE strategies can aid in harmonizing several other socioeconomic and environmental initiatives with SDGs to foster the event legacy and avoid possible ecosystem damage, namely:
1. SDG 1: Involving exclusive social groups of the population, waste pickers, or association of waste pickers for working in FIFA events (during and post-event).
2. SDG 6: Setting norms on water saving and water cleaning, reusing wastewater, and water conservation or facilitating boxes with free water in recyclable cups.
3. SDG 8: Seasonal and regular green jobs in circularity, it is any job or profession that directly includes or indirectly supports one of the strategies of the circular and CCE economy and climate change prevention. There are three types of circular green works: main, auxiliary, and indirectly related to the support in FIFA on every stage of LCA chains in waste and resource management, marketing, engineering, building, transport, etc.
4. SDG 10: Environmental and social justice for all groups of stakeholders, equal rights to affordable and quality resources and services, including in-process CCE system service.
5. SDG 16: Creating new social, environmental, and economic structures and institutions under sustainability and circularity.
6. SDG 17: Creating and strengthening of collaboration chains with NGOs, scientific sector, public–private partnership, and customers in CCE on all levels of the life cycle stages, promoting event legacy—historical and cultural capital of mega-events with environmentally friendly value creation.
Concluding Remarks and Future Works
The research quantified the ecosystem damage-related impacts to better understand the ecological quality in life cycle impact assessment for modular container stadiums taking the case of the RAA Stadium in the State of Qatar using a cradle-to-cradle LCA approach. The study presented EOL management through an impact quantification method along with a perspective analysis through the lens of the circular collaborative economy in aligning the FIFA World Cup 2022 initiatives with UN SDGs and reducing the ecological burden during, before, and post-event. A sensitivity analysis was carried out to investigate the extent of impact per category indicator subject to material quantity variations. E-LCA results highlighted the contributing life cycle phase and material/activity for each impact category. Four main life cycle phases were considered for the assessment: production, construction, operation, and EOL phase. For the operations phase, two scenarios were considered: 1 year of operation, after which the stadium would be dismantled, and 30 years of operation, to compare the impact of a long-term operation on the different impact categories. The results of the study are summarized in Table 7.
The results of the sensitivity analysis revealed climate change as the most sensitive impact category for possible parametric variations. Considering the post-event scenario, the authors suggest alternatives for the future application of shipping containers apart from the current target to use the containers overseas for sports complex facilities such as constructing container restaurants, schools, retail stores, coffee shops, students housing, commercial offices, art galleries, portable toilets, indoor garden area, sedan garages, cold storages, medical facilities, libraries and warehouses, swimming pool and mobile jacuzzies, transportable greenhouses, and container movie theaters, which have a visually pleasing aesthetics and are cost-effective. In addition, the containers can be reused to build compact shipping container homes and lodgings that can provide characterful eco-friendly living spaces for tourists that visit Qatar post-2022. Several peer-to-peer rental platforms such as Airbnb most seek such compact container mansions to preserve the badge of sustainability while delivering services. This can help Qatar maintain a post-2022 legacy and help Qatar generate a new market for sharing economy and eco-friendly tourism, a sustainable slant for development.
Alternatively, the authors suggest using sustainable and green element substitutes while constructing similar demountable infrastructures in the future. The authors recommend considering the reuse of the material options from the beginning of the design phase. Using green steel on roofs reduces energy consumption, which eventually reduces GHG emissions because it helps to reduce the heat island effect, which can assist in lowering the indoor temperature by up to 5°F and resulting in a possible energy savings of up to 5%. Another example of upgrading the lighting fixtures of efficient LED lighting (when possible) offers instant paybacks in cost vs. benefits that are estimated at $32,000/year per fixture. Installation of glass-reinforced plastic as a waste management system in lieu of concrete or steel material will reduce a huge amount of CO2 and reduce the amount of GHG emissions. Moreover, window glass and frame material have significant impact levels on environmental performance, which must be considered during the design phase. Therefore, utilizing glass-reinforced plastic window frames as substituents to the traditional glass window can improve the energy performance and potential energy at the product life cycle, thermal performance, and structural performance (Katar, 2017).
The presented research act as a roadmap for developing economies that target to address sustainability when hosting future World Cup mega-events; however, the research study was conducted during the construction phase of the RAA Stadium; therefore, there is plenty of assumptions made regarding the operational phase data provided by the World Cup 2022 hosting committee. However, this limitation can pave the way for further research in this selected area of knowledge by conducting a post-World Cup impact assessment study. The authors recommend using “life cycle sustainability assessment (LCSA),” extending the study to include social impacts such as human health and quality of life through a social-life cycle assessment, life cycle cost approach to understand the economic sustainability in constructing modular stadiums for carbon-neutral mega-events and resource depletion damage using an E-LCA approach. It is also suggested that when a close relation to the ecological objectives is necessary, the ecological scarcity approach is better used. The EPS approach can also give politicians and reform advocates useful guidance. Finally, it has been concluded that ReCiPe's strongest asset is that it helps product analysis to consider diverse philosophies. The current study has focused on evaluating the ecological quality of using container stadiums for hosting mega-sporting events. The CCE strategies presented for the EOL phase can be extended in a more detailed study that would quantify the benefits and costs of each alternative, which would, in return, help in better decision-making. Also, applications of more advanced multi-criteria decision techniques would further assist decision-makers in choosing the best alternatives while incorporating green design practices and possible retrofits.
Data Availability Statement
The original contributions presented in the study are included in the article, further inquiries can be directed to the corresponding author/s.
Author Contributions
MK: conceptualization, method, formal analysis, and supervision. NO: method, analysis, and writing. AK: methods, formal analysis, visualization, and writing. NA: writing, editing, and reviewing. NA-A: writing and visualization. AN: writing literature review and formal analysis. All authors contributed to the article and approved the submitted version.
Conflict of Interest
The authors declare that the research was conducted in the absence of any commercial or financial relationships that could be construed as a potential conflict of interest.
Publisher's Note
All claims expressed in this article are solely those of the authors and do not necessarily represent those of their affiliated organizations, or those of the publisher, the editors and the reviewers. Any product that may be evaluated in this article, or claim that may be made by its manufacturer, is not guaranteed or endorsed by the publisher.
References
Adams, K. T., Osmani, M., Thorpe, T., and Thornback, J. (2017). “Circular economy in construction: current awareness, challenges, and enablers,” in Proceedings of the Institution of Civil Engineers-Waste and Resource Management (Thomas Telford Ltd.).
Agrawal, R., Wankhede, V. A., Kumar, A., Upadhyay, A., and Garza-Reyes, J. A. (2021). Nexus of circular economy and sustainable business performance in the era of digitalization. Int. J. Product. Perform. Manage. 2021, 1–27. doi: 10.1108/IJPPM-12-2020-0676
Ahmed, F., and Pretorius, L. (2010). Mega-events and environmental impacts: The 2010 FIFA World Cup in South Africa. Alternation 17, 274–296.
Ajzen, I. (1991). The theory of planned behavior. Organ. Behav. Hum. Decis. Process. 50, 179–211. doi: 10.1016/0749-5978(91)90020-T
Al-Buenain, A., Al-Muhannadi, S., Falamarzi, M., Kutty, A. A., Kucukvar, M., and Onat, N. C. (2021). The adoption of electric vehicles in qatar can contribute to net carbon emission reduction but requires strong government incentives. Vehicles 3, 618–635. doi: 10.3390/vehicles3030037
Al-Hamrani, A., Kim, D., Kucukvar, M., and Onat, N. C. (2021). Circular economy application for a Green Stadium construction towards sustainable FIFA world cup Qatar 2022™. Environ. Impact Assess. Rev. 87:106543. doi: 10.1016/j.eiar.2020.106543
Alsarayreh, M. M., AlSuwaidi, M. F., Sharif, R. A., and Kutty, A. A. (2020). “The factors affecting CO2 emission in the european union countries: a statistical approach to sustainability across the food industry,” in The 2020 IEEE 7th International Conference on Industrial Engineering and Applications (ICIEA) (IEEE). doi: 10.1109/ICIEA49774.2020.9102066
Anastasiades, K., Blom, J., Buyle, M., and Audenaert, A. (2020). Translating the circular economy to bridge construction: Lessons learned from a critical literature review. Renewa. Sustain. Energy Rev. 117:109522. doi: 10.1016/j.rser.2019.109522
Aquino, I., and Nawari, N. O. (2015). Sustainable design strategies for sport stadia. Suburban Sustainab. 3:3. doi: 10.5038/2164-0866.3.1.1020
Ardente, F., Beccali, M., Cellura, M., and Mistretta, M. (2008). Building energy performance: an LCA case study of kenaf-fibers insulation board. Energy Build. 40, 1–10. doi: 10.1016/j.enbuild.2006.12.009
Atchariyasopon, K. (2017). Sustainable solid waste management in sports events: a case study of football matches in Thailand. J. Population Soc. Stud. 25, 69–81. Retrieved from: https://so03.tci-thaijo.org/index.php/jpss/article/view/102290
Atiku, S. O. (2020). Knowledge Management for the Circular Economy. Handbook of Research on Entrepreneurship Development and Opportunities in Circular Economy. Namibia: Namibia University of Science and Technology. doi: 10.4018/978-1-7998-5116-5.ch027
Ayres, R. U., and Kneese, A. V. (1969). Production, consumption, and externalities. Am. Econ. Rev. 59, 282–297.
Bare, J. C. (2002). TRACI: The tool for the reduction and assessment of chemical and other environmental impacts. J. Indus. Ecol. 6, 49–78. doi: 10.1162/108819802766269539
Bellekom, S., Potting, J., and Benders, R. (2006). Feasibility of applying site-dependent impact assessment of acidification in LCA (8 pp). Int. J. Life Cycle Assessment 11, 417–424. doi: 10.1065/lca2005.08.221
Benachio, G. L. F., Freitas, M. D. C. D., and Tavares, S. F. (2020). Circular economy in the construction industry: A systematic literature review. J. Clean. Prod. 260:121046. doi: 10.1016/j.jclepro.2020.121046
Berg, A., Antikainen, R., Hartikainen, E., Kauppi, S., Kautto, P., Lazarevic, D., et al. (2018). Circular Economy for Sustainable Development. Reports of the Finnish Environment Institute 26/2018, Finnish Environment Institute: Programme for Sustainable Circular Economy. Available online at: http://hdl.handle.net/10138/251516
Botsman, R., and Rogers, R. (2011). What's Mine Is Yours: How Collaborative Consumption is Changing the Way We Live. London: HarperCollins Business.
Bovea, M. D., and Powell, J. C. (2016). Developments in life cycle assessment are applied to evaluate the environmental performance of construction and demolition wastes. Waste Manage. 50, 151–172. doi: 10.1016/j.wasman.2016.01.036
Bribian, I. Z., Uson, A. A., and Scarpellini, S. (2009). Life cycle assessment in buildings: State-of-the-art and simplified LCA methodology as a complement for building certification. Build. Environ. 44, 2510–2520. doi: 10.1016/j.buildenv.2009.05.001
Buss, D. M. (2019). Evolutionary Psychology: The New Science of the Mind (6th ed.). New York, NY: Routledge.
Chernovich, E. (2013). Technology and Proenvironmental Behavior in Urban Households: How Technologies Mediate Domestic Routines. Available online at: https://www.hse.ru/data/2013/10/09/1280336516/18STI2013.pdf (assessed October 2, 2021).
Codagnone, C., and Martens, B. (2016). Scoping the Sharing Economy: Origins, Definitions, Impact and Regulatory Issues, JRC Science for Policy Report. Luxembourg: Publications Office of the European Union. doi: 10.2139/ssrn.2783662
De Gracia, A., Rincón, L., Castell, A., Jiménez, M., Boer, D., Medrano, M., et al. (2010). Life cycle assessment of the inclusion of phase change materials (PCM) in experimental buildings. Energy Build. 42, 1517–1523. doi: 10.1016/j.enbuild.2010.03.022
De Haes, H. A. U., Heijungs, R., Suh, S., and Huppes, G. (2004). Three strategies to overcome the limitations of life-cycle assessment. J. Indus. Ecol. 8, 19–32. doi: 10.1162/1088198042442351
Death, C. (2011). “Greening” the 2010 FIFA World Cup: Environmental sustainability and the mega-event in South Africa. J. Environ. Policy Plann. 13, 99–117. doi: 10.1080/1523908X.2011.572656
Eberhardt, L. C. M., Birgisdottir, H., and Birkved, M. (2019). “Potential of circular economy in sustainable buildings,” in IOP Conference Series: Materials Science and Engineering (IOP Publishing). doi: 10.1088/1757-899X/471/9/092051
Ekvall, T., and Finnveden, G. (2001). Allocation in ISO 14041-a critical review. J. Clean. Prod. 9, 197–208. doi: 10.1016/S0959-6526(00)00052-4
Elhmoud, E. R., and Kutty, A. A. (2021). “Sustainability assessment in aviation industry: a mini-review on the tools, models and methods of assessment,” in Proceedings of the 2nd African International Conference on Industrial Engineering and Operations Management Harare (Harare).
Elia, V., Gnoni, M. G., and Tornese, F. (2017). Measuring circular economy strategies through index methods: A critical analysis. J. Clean. Prod. 142, 2741–2751. doi: 10.1016/j.jclepro.2016.10.196
Ellen Macarthur Foundation (2021). Design and the Circular Economy. Available online at: https://ellenmacarthurfoundation.org/articles/design-and-the-circular-economy (accessed October 2, 2021).
Errington, I., King, C. K., Wilkins, D., Spedding, T., and Hose, G. C. (2018). Ecosystem effects and the management of petroleum-contaminated soils on subantarctic islands. Chemosphere 194, 200–210. doi: 10.1016/j.chemosphere.2017.11.157
FIFA (2020). First Sustainability Progress Report. FIFA World Cup 2022™. Available online at: https://digitalhub.fifa.com/m/4710ffeccb27c535/original/qlsdbl7ipsax0ndjqyup-pdf.pdf (accessed October 22, 2021).
Finnveden, G. (1997). Valuation methods within LCA-Where are the values? Int. J. Life Cycle Assessment 2, 163–169. doi: 10.1007/BF02978812
Finnveden, G., Hauschild, M. Z., Ekvall, T., Guinée, J., Heijungs, R., Hellweg, S., et al. (2009). Recent developments in life cycle assessment. J. Environ. Manage. 91, 1–21. doi: 10.1016/j.jenvman.2009.06.018
Fischer-Kowalski, M., and Haberl, H. (1993). Metabolism and colonization. Modes of production and the physical exchange between societies and nature. Innovation 6, 415–442. doi: 10.1080/13511610.1993.9968370
Fischer-Kowalski, M., and Weisz, H. (1999). Society as a hybrid between material and symbolic realms: Toward a theoretical framework of society-nature interaction. Adv. Human Ecol. 8, 215–252.
Frawley, S., and Adair, D. (2013). Managing the Olympics (1st ed.). London: Palgrave Macmillan. doi: 10.1057/9780230389588
Frischknecht, R., Jungbluth, N., Althaus, H.-J., Doka, G., Heck, T., Hellweg, S., et al. (2007). Overview and Methodology. Dübendorf: Ecoinvent report No. 1. Swiss Centre for Life Cycle Inventories.
Gebreslassie, B. H., Guillén-Gosálbez, G., Jiménez, L., and Boer, D. (2009). Design of environmentally conscious absorption cooling systems via multi-objective optimization and life cycle assessment. Appl. Energy 86, 1712–1722. doi: 10.1016/j.apenergy.2008.11.019
Geeraert, A., and Gauthier, R. (2018). Out-of-control Olympics: Why the IOC is unable to ensure an environmentally sustainable Olympic Games. J. Environ. Policy Plann. 20, 16–30. doi: 10.1080/1523908X.2017.1302322
Geng, Y., and Doberstein, B. (2008). Developing the circular economy in China: Challenges and opportunities for achieving leapfrog development'. Int. J. Sustain. Dev. World Ecol. 15, 231–239. doi: 10.3843/SusDev.15.3:6
Genovese, A., Acquaye, A. A., Figueroa, A., and Koh, S. L. (2017). Sustainable supply chain management and the transition towards a circular economy: Evidence and some applications. Omega 66, 344–357. doi: 10.1016/j.omega.2015.05.015
Gernuks, M., Buchgeister, J., and Schebek, L. (2007). Assessment of environmental aspects and determination of environmental targets within environmental management systems (EMS)-development of a procedure for Volkswagen. J. Clean. Prod. 15, 1063–1075. doi: 10.1016/j.jclepro.2006.06.003
Ghisellini, P., Cialani, C., and Ulgiati, S. (2016). A review on circular economy: the expected transition to a balanced interplay of environmental and economic systems. J. Clean. Prod. 114, 11–32. doi: 10.1016/j.jclepro.2015.09.007
Gilbert, P., Wilson, P., Walsh, C., and Hodgson, P. (2017). The role of material efficiency to reduce CO2 emissions during ship manufacture: a life cycle approach. Marine Policy 75, 227–237. doi: 10.1016/j.marpol.2016.04.003
Goedkoop, M., Heijungs, R., Huijbregts, M., Schryver, A., Struijs, J., and Zelm, R. (2008). ReCiPE 2008: A Life Cycle Impact Assessment Method which Comprises Harmonised Category Indicators at the Midpoint and the Endpoint Level, 1st Edn. Report 1: Characterization. Available online at: https://www.leidenuniv.nl/cml/ssp/publications/recipe_characterisation.pdf
Haas, W., Hertwich, E., Hubacek, K., Korytarova, K., Ornetzeder, M., and Weisz, H. (2005). The Environmental Impacts of Consumption: Research Methods and Driving Forces. IIASA Interim Report. Laxenburg: IIASA.
Haberl, H., Fischer-Kowalski, M., Krausmann, F., Weisz, H., and Winiwarter, V. (2004). Progress towards sustainability? What the conceptual framework of material and energy flow accounting (MEFA) can offer. Land Use Policy 21, 199–213. doi: 10.1016/j.landusepol.2003.10.013
Hossain, M. U., Ng, S. T., Antwi-Afari, P., and Amor, B. (2020). Circular economy and the construction industry: Existing trends, challenges and prospective framework for sustainable construction. Renew. Sustain. Energy Rev. 130:109948. doi: 10.1016/j.rser.2020.109948
Huberman, N., and Pearlmutter, D. (2008). A life-cycle energy analysis of building materials in the Negev desert. Energy Build. 40, 837–848. doi: 10.1016/j.enbuild.2007.06.002
Huysveld, S., Hubo, S., Ragaert, K., and Dewulf, J. (2019). Advancing circular economy benefit indicators and application on open-loop recycling of mixed and contaminated plastic waste fractions. J. Clean. Prod. 211, 1–13. doi: 10.1016/j.jclepro.2018.11.110
International Organization for Standardization (2006a). Environmental Management Life Cycle Assessment Principles and Frameworks.
International Organization for Standardization (2006b). ISO 14044:2006(en) Environmental Management - Life Cycle Assessment- Requirements and Guidelines. Available Online at: https://www.iso.org/obp/ui/#iso:std:iso:14044:ed-1:v1:en (accessed July 27, 2021).
Itsubo, N., Sakagami, M., Washida, T., Kokubu, K., and Inaba, A. (2004). Weighting across safeguard subjects for LCIA through the application of conjoint analysis. Int. J. Life Cycle Assessment 9, 196–205. doi: 10.1007/BF02994194
Jæger, B., Menebo, M. M., and Upadhyay, A. (2021). Identification of environmental supply chain bottlenecks: a case study of the Ethiopian healthcare supply chain. Manage. Environ. Q. 32:6. doi: 10.1108/MEQ-12-2019-0277
Jackson, T. (2009). Prosperity without growth. The transition to a sustainable economy. Sustain. Dev. Commission 2009:288. doi: 10.4324/9781849774338
Jolliet, O., Müller-Wenk, R., Bare, J., Brent, A., Goedkoop, M., Heijungs, R., et al. (2004). The LCIA midpoint-damage framework of the UNEP/SETAC life cycle initiative. Int. J. Life Cycle Assessment 9, 394–404. doi: 10.1007/BF02979083
Jraisat, L., Upadhyay, A., Ghalia, T., Jresseit, M., Kumar, V., and Sarpong, D. (2021). Triads in sustainable supply-chain perspective: why is a collaboration mechanism needed? Int. J. Product. Res. 2021, 1–17. doi: 10.1080/00207543.2021.1936263
Jun, H., and Xiang, H. (2011). The development of the circular economy is a fundamental way to achieve sustainable agriculture development in China. Energy Proc. 5, 1530–1534. doi: 10.1016/j.egypro.2011.03.262
Kapsalis, V. C., Kyriakopoulos, G. L., and Aravossis, K. G. (2019). Investigation of ecosystem services and circular economy interactions under an inter-organizational framework. Energies 12:1734. doi: 10.3390/en12091734
Katar, I. M. (2017). Evaluate state-of-the-art carbon fibers' composites (CFC) as finishing materials in building construction. Int. J. Appl. Eng. Res. 12, 13826–13833.
Koger, S. M., and Winter, D. D. (2011). The Psychology of Environmental Problems: Psychology for Sustainability. doi: 10.4324/9780203847978
Kollmuss, A., and Agyeman, J. (2002). Mind the gap: why do people act environmentally and what are the barriers to pro-environmental behavior? Environ. Educ. Res. 8, 239–260. doi: 10.1080/13504620220145401
Kucukvar, M., Egilmez, G., and Tatari, O. (2014). Sustainability assessment of US final consumption and investments: triple-bottom-line input-output analysis, Journal of cleaner production. J. Clean. Product. 81. 234–243. doi: 10.1016/j.jclepro.2014.06.033
Kucukvar, M., Kutty, A. A., Al-Hamrani, A., Kim, D., Nofal, N., Onat, N. C., et al. (2021). How circular design can contribute to social sustainability and legacy of the FIFA World Cup Qatar 2022™? The case of innovative shipping container stadium. Environ. Impact Assessment Rev. 91:106665. doi: 10.1016/j.eiar.2021.106665
Kumar, N., Brint, A., Shi, E., Upadhyay, A., and Ruan, X. (2019). Integrating sustainable supply chain practices with operational performance: an exploratory study of Chinese SMEs. Product. Plann. Control 30, 464–478. doi: 10.1080/09537287.2018.1501816
Kutty, A. A., and Abdalla, G. M. (2020). “Tools and techniques for food security and sustainability related assessments: a focus on the data and food waste management system,” in Proceedings of the 5th NA Conference on Industrial Engineering and Operations Management (Detroit, MI).
Kutty, A. A., Abdella, G. M., Kucukvar, M., Onat, N. C., and Bulu, M. (2020a). A system thinking approach for harmonizing smart and sustainable city initiatives with United Nations sustainable development goals. Sustain. Dev. 28, 1347–1365. doi: 10.1002/sd.2088
Kutty, A. A., Yetiskin, Z., Abraham, M. M., Nooh, M. A., Kucukvar, M., and Abdella, G. M. (2020b). “An empirical assessment on the transportation sustainability indicators and their impact on economic productivity,” in Proceedings of the 5th North American International Conference on Industrial Engineering and Operations Management (Michigan: IEOM).
Laing, T., Upadhyay, A., Mohan, S., and Subramanian, N. (2019). Environmental improvement initiatives in the coal mining industry: maximisation of the triple bottom line. Product. Plann. Control 30, 426–436. doi: 10.1080/09537287.2018.1501813
Liang, Y. W., Wang, C. H., Tsaur, S. H., Yen, C. H., and Tu, J. H. (2016). Mega-event and urban sustainable development. Int. J. Event Festival Manage. 7, 152–171. doi: 10.1108/IJEFM-05-2016-0033
Manni, M., Coccia, V., Nicolini, A., Marseglia, G., and Petrozzi, A. (2018). Towards zero energy stadiums: The case study of the Dacia arena in Udine, Italy. Energies 11:2396. doi: 10.3390/en11092396
Mao, J., Li, C., Pei, Y., and Xu, L. (2018). Circular Economy and Sustainable Development Enterprises. Singapore: Springer. doi: 10.1007/978-981-10-8524-6
Menoufi, K. A. I. (2011). Life Cycle Analysis and Life Cycle Impact Assessment Methodologies: State of the Art. Lleida: Universitat de Lleida. Available online at: http://hdl.handle.net/10459.1/45831
MezaTalavera, A., Al-Ghamdi, S. G., and Koc, M. (2019). Sustainability in mega-events: Beyond Qatar 2022. Sustainability 11:6407. doi: 10.3390/su11226407
Miyazaki, N., Siegenthaler, C., Schoenbaum, T., and Azuma, K. (2004). Calculation of eco factors for Japan: method for environmental accounting based on the ecoscarcity principle (Japan Environmental Policy Priorities Index). Soc. Sci. Res. Institute Monograph Series 7, 1–54.
Mol, A. P. J., and Zhang, L. (2011). Olympic Games, Mega-Events and Civil Societies: Globalization, Environment Resistance. Basingstoke: Palgrave Macmillan
Morseletto, P. (2020). Restorative and regenerative: Exploring the concepts in the circular economy. J. Indust. Ecol. 24, 763–773. doi: 10.1111/jiec.12987
Murray, A., Skene, K., and Haynes, K. (2017). The circular economy: an interdisciplinary exploration of the concept and application in a global context. J. Business Ethics 140, 369–380. doi: 10.1007/s10551-015-2693-2
Nakano, K., Nakaniwa, C., Kabeya, T., Iguchi, T., and Aoki, R. (2007). Current activities of the life cycle assessment Society of Japan. Int. J. Life Cycle Assessment 12:546. doi: 10.1065/lca2007.10.360
Norris, G. A. (2002). Impact characterization in the tool for the reduction and assessment of chemical and other environmental impacts: Methods for acidification, eutrophication, and ozone formation. J. Indus. Ecol. 6, 79–101. doi: 10.1162/108819802766269548
Nuñez-Cacho, P., Górecki, J., Molina-Moreno, V., and Corpas-Iglesias, F. A. (2018). What gets measured gets done: Development of a circular economy measurement scale for building industry. Sustainability 10:2340. doi: 10.3390/su10072340
Nußholz, J. L. K. (2017). Circular business models: defining a concept and framing an emerging researchfield. Sustainability 9:1810. doi: 10.3390/su9101810
Onat, N., Kucukvar, M., and Afshar, S. (2019). Eco-efficiency of electric vehicles in the United States: A life cycle assessment based principal component analysis. J. Clean. Prod. 212, 515–526. doi: 10.1016/j.jclepro.2018.12.058
Onat, N. C., Abdella, G. M., Kucukvar, M., Kutty, A. A., Al-Nuaimi, M., Kumbaroglu, G., et al. (2020). How eco-efficient are electric vehicles across Europe? A regionalized life cycle assessment-based eco-efficiency analysis. Sustainable Dev. 29, 941–956. doi: 10.1002/sd.2186
Park, J., Sarkis, J., and Wu, Z. (2010). Creating an integrated business and environmental value within the context of China's circular economy and ecological modernization. J. Clean. Prod. 18, 1494–1501. doi: 10.1016/j.jclepro.2010.06.001
Park, Y. S., Egilmez, G., and Kucukvar, M. (2016). Emergy and endpoint impact assessment of agricultural and food production in the United States: A supply chain-linked ecologically-based life cycle assessment. Ecol. Indic. 62, 117–137. doi: 10.1016/j.ecolind.2015.11.045
Pennington, D. W., Potting, J., Finnveden, G., Lindeijer, E., Jolliet, O., Rydberg, T., et al. (2004). Life cycle assessment Part 2: Current impact assessment practice. Environ. Int. 30, 721–739. doi: 10.1016/j.envint.2003.12.009
Petropoulos, G. (2016). An Economic Review on the Collaborative Economy. Directorate General for Internal Policies. European Union.
Prieto-Sandoval, V., Jaca, C., and Ormazabal, M. (2018). Towards a consensus on the circular economy. J. Clean. Prod. 179, 605–615. doi: 10.1016/j.jclepro.2017.12.224
Primasetra, A. (2019). Implementation of re-used shipping containers for green architecture (Case Study: ITSB Creative Hub-Cikarang). KnE Soc. Sci. 2019, 67–82. doi: 10.18502/kss.v3i21.4959
Qatar2022 (2020a). Experts Discuss Qatar's Goal of Hosting the First Carbon-Neutral FIFA World Cup™ | See You In 2022. See You In 2022. Available online at: https://www.qatar2022.qa/en/news/experts-discuss-qatars-goal-of-hosting-the-first-carbon-neutral-fifa-world-cup (accessed April 20, 2021).
Qatar2022 (2020b). FIFA World Cup 2022™ First Sustainability Progress Report published | See You In 2022. See You In 2022. Available online at: https://www.qatar2022.qa/en/news/fifa-world-cup-first-sustainability-progress-report-published (accessed April 15, 2021).
Qatar2022 (2020c). Turning Old Into New: Al Rayyan Venue's Sustainability Experience | See You In 2022. See You In 2022. Available online at: https://www.qatar2022.qa/en/news/turning-old-into-new-al-rayyan-venues-sustainability-experience (accessed March 25, 2021).
Rajan, J., and Booth, A. (2016). Sustainability and waste management of the 2015 Prince George Canada Winter Games. Int. J. Sustain. Dev. Plann. 11, 255–262. doi: 10.2495/SDP-V11-N3-255-262
Robertson, S. J., McGill, W. B., Massicotte, H. B., and Rutherford, P. M. (2007). Petroleum hydrocarbon contamination in boreal forest soils: a mycorrhizal ecosystems perspective. Biol. Rev. 82, 213–240. doi: 10.1111/j.1469-185X.2007.00012.x
Saldarriaga-Hernandez, S., Hernandez-Vargas, G., Iqbal, H. M., Barcelo, D., and Parra-Saldívar, R. (2020). Bioremediation potential of Sargassum sp. biomass to tackle pollution in coastal ecosystems: Circular economy approach. Sci. Total Environ. 715:136978. doi: 10.1016/j.scitotenv.2020.136978
Sauvé, S., Bernard, S., and Sloan, P. (2016). Environmental sciences, sustainable development, and circular economy: Alternative concepts for trans-disciplinary research. Environ. Dev. 17, 48–56. doi: 10.1016/j.envdev.2015.09.002
Schwartz, S. H. (1977). Normative influences on altruism. Adv. Exp. Soc. Psychol. 10, 221–279. doi: 10.1016/S0065-2601(08)60358-5
SETAC (1993). A Conceptual Framework for Life-Cycle Impact Assessment. Society of Environmental Toxicology and Chemistry and SETAC Foundation for Environmental Education. Pensacola, FL.
Sleeswijk, A. W., van Oers, L. F., Guinée, J. B., Struijs, J., and Huijbregts, M. A. (2008). Normalisation in product life cycle assessment: An LCA of the global and European economic systems in the year 2000. Sci. Total Environ. 390, 227–240. doi: 10.1016/j.scitotenv.2007.09.040
Spanos, I., Kucukvar, M., Bell, T. C., Elnimah, A., Hamdan, H., Al Meer, B., et al. (2021). How FIFA World Cup 2022™ can meet the carbon neutral commitments and the United Nations 2030 Agenda for Sustainable Development?: Reflections from the tree nursery project in Qatar. Sustainable Dev. 1–24. doi: 10.1002/sd.2239
Steg, L., and Vlek, C. A. J. (2009). Encouraging pro-environmental behaviour: An integrative review and research agenda. J. Environ. Psychol. 29, 309–317. doi: 10.1016/j.jenvp.2008.10.004
Stern, P. C. (2005). Understanding individuals' environmentally significant behavior. Environ. Law Report. 35, 10785–10790.
Tadeu, S., Rodrigues, C., Tadeu, A., Freire, F., and Simões, N. (2015). Energy retrofit of historic buildings: Environmental assessment of cost-optimal solutions. J. Build. Eng. 4, 167–176. doi: 10.1016/j.jobe.2015.09.009
Tillman, A. M. (2000). Significance of decision-making for LCA methodology. Environ. Impact Assess. Rev. 20, 113–123. doi: 10.1016/S0195-9255(99)00035-9
Tukker, A., Bulavskaya, T., Giljum, S., de Koning, A., Lutter, S., Simas, M., et al. (2014). The Global Resource Footprint of Nations. Carbon, Water, Land and Materials Embodied in Trade and Final Consumption Calculated With EXIOBASE 2.1. Leiden/Delft/Trondheim. Technical report EU Commission.
Upadhyay, A. (2020). Antecedents of green supply chain practices in developing economies. Manage. Environ. Q. 32:6. doi: 10.1108/MEQ-12-2019-0274
Upadhyay, A., Akter, S., Adams, L., Kumar, V., and Varma, N. (2019). Investigating “circular business models” in the manufacturing and service sectors. J. Manufact. Technol. Manage. 30, 590–606. doi: 10.1108/JMTM-02-2018-0063
Upadhyay, A., Ayodele, J. O., Kumar, A., and Garza-Reyes, J. A. (2020). A review of challenges and opportunities of blockchain adoption for operational excellence in the UK automotive industry. J. Global Operat. Strategic Sourc. 14, 7–60. doi: 10.1108/JGOSS-05-2020-0024
Upadhyay, A., Kumar, A., and Akter, S. (2021d). An analysis of UK retailers' initiatives towards circular economy transition and policy-driven directions. Clean Technol. Environ. Policy 2021, 1–9. doi: 10.1007/s10098-020-02004-9
Upadhyay, A., Kumar, A., Kumar, V., and Alzaben, A. (2021a). A novel business strategies framework of do-it-yourself practices in logistics to minimise environmental waste and improve performance. Business Strategy Environ. 1–11. doi: 10.1002/bse.2846
Upadhyay, A., Laing, T., Kumar, V., and Dora, M. (2021b). Exploring barriers and drivers to the implementation of circular economy practices in the mining industry. Resources Policy 72:102037. doi: 10.1016/j.resourpol.2021.102037
Upadhyay, A., Mukhuty, S., Kumar, V., and Kazancoglu, Y. (2021c). Blockchain technology and the circular economy: Implications for sustainability and social responsibility. J. Clean. Product. 293, 126–130. doi: 10.1016/j.jclepro.2021.126130
Weisz, H. (2006). Accounting for Raw Material Equivalents of Traded Goods: A Comparison of Input-Output Approaches in Physical, Monetary, and Mixed Units. IFF, Abt. Soziale Ökologie.
White, G. R., Cicmil, S., Upadhyay, A., Subramanian, N., Kumar, V., and Dwivedi, A. (2019). The soft side of knowledge transfer partnerships between universities and small to medium enterprises: an exploratory study to understand process improvement. Product. Plann. Control 30, 907–918. doi: 10.1080/09537287.2019.1582098
Winans, K., Kendall, A., and Deng, H. (2017). The history and current applications of the circular economy concept. Renew. Sustain. Energy Rev. 68, 825–833. doi: 10.1016/j.rser.2016.09.123
Zanghelini, G. M., de Souza Junior, H. R., Kulay, L., Cherubini, E., Ribeiro, P. T., and Soares, S. R. (2016). A bibliometric overview of Brazilian LCA research. Int. J. Life Cycle Assessment 21, 1759–1775. doi: 10.1007/s11367-016-1132-7
Keywords: collaborative circular economy, FIFA world cup, life cycle assessment, sharing economy, sustainability, sustainable development
Citation: Kucukvar M, Kutty AA, Onat NC, Al Jurf N, Al-Abdulmalek N, Naser A and Ermolaeva Y (2021) How Can Collaborative Circular Economy Practices in Modular Construction Help Fédération Internationale de Football Association World Cup Qatar 2022 to Achieve Its Quest for Sustainable Development and Ecological Systems? Front. Sustain. 2:758174. doi: 10.3389/frsus.2021.758174
Received: 13 August 2021; Accepted: 06 October 2021;
Published: 18 November 2021.
Edited by:
Pedro Núñez-Cacho, University of Jaén, SpainReviewed by:
Srikanta Routroy, Birla Institute of Technology and Science, IndiaArvind Upadhyay, University of Brighton, United Kingdom
Copyright © 2021 Kucukvar, Kutty, Onat, Al Jurf, Al-Abdulmalek, Naser and Ermolaeva. This is an open-access article distributed under the terms of the Creative Commons Attribution License (CC BY). The use, distribution or reproduction in other forums is permitted, provided the original author(s) and the copyright owner(s) are credited and that the original publication in this journal is cited, in accordance with accepted academic practice. No use, distribution or reproduction is permitted which does not comply with these terms.
*Correspondence: Murat Kucukvar, mkucukvar@qu.edu.qa