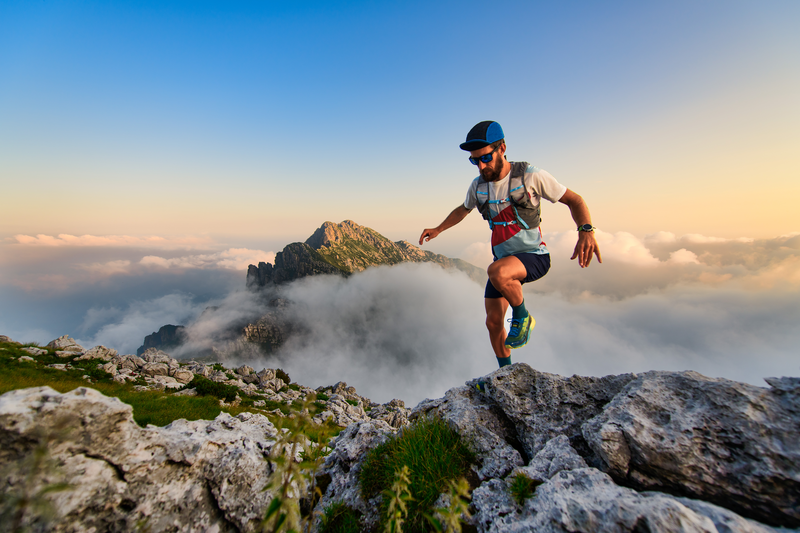
94% of researchers rate our articles as excellent or good
Learn more about the work of our research integrity team to safeguard the quality of each article we publish.
Find out more
REVIEW article
Front. Surg.
Sec. Orthopedic Surgery
Volume 12 - 2025 | doi: 10.3389/fsurg.2025.1461319
The final, formatted version of the article will be published soon.
You have multiple emails registered with Frontiers:
Please enter your email address:
If you already have an account, please login
You don't have a Frontiers account ? You can register here
The objective of the bibliometric analysis was to quantify and identify the current status and trends of laser bone ablation research from 1979 to 2023.The Web of Science (WOS) core collection database was used to search for articles on laser bone ablation published from 1979 to 2023. The collected data were then imported into Microsoft Excel, VOSviewer, and CiteSpace for detailed analysis and visualization.Results: A total of 383 articles were included for analysis. The United States made the most significant contributions to the field in terms of both quantity and quality.Moreover, Cattin, Philippe C emerged as the author with the highest number of publications, while the University of Basel stood out as the institution with the greatest publication output. Lasers in Surgery and Medicine emerged as not only the journal with the most publications but also held considerable influence within its domain.Prominent keywords that surfaced frequently included "ablation," "er:yag laser," and "bone."The annual number of publications in the field of laser bone ablation is showing an overall upward trend. Research on laser bone ablation primarily focuses on investigating the parameters of this technique, as well as its application in treating bone tumors, performing laser stapes surgery, and various applications of laser bone ablation.The laser osteotomy, laser ablation of bone tumors, animal experiments, and the interaction with biological tissues during laser bone ablation are expected to be the focal areas and future directions in this field.
Keywords: :laser, Bone Ablation, bibliometrics analysis, visual analysis, Citespace, VOSviewer
Received: 08 Jul 2024; Accepted: 24 Feb 2025.
Copyright: © 2025 Zhang, Liu, Zhang, Shen, Han, Li, Zhao and Wang. This is an open-access article distributed under the terms of the Creative Commons Attribution License (CC BY). The use, distribution or reproduction in other forums is permitted, provided the original author(s) or licensor are credited and that the original publication in this journal is cited, in accordance with accepted academic practice. No use, distribution or reproduction is permitted which does not comply with these terms.
* Correspondence:
Junqiang Wang, Peking University, Beijing, 100871, Beijing Municipality, China
Disclaimer: All claims expressed in this article are solely those of the authors and do not necessarily represent those of their affiliated organizations, or those of the publisher, the editors and the reviewers. Any product that may be evaluated in this article or claim that may be made by its manufacturer is not guaranteed or endorsed by the publisher.
Research integrity at Frontiers
Learn more about the work of our research integrity team to safeguard the quality of each article we publish.