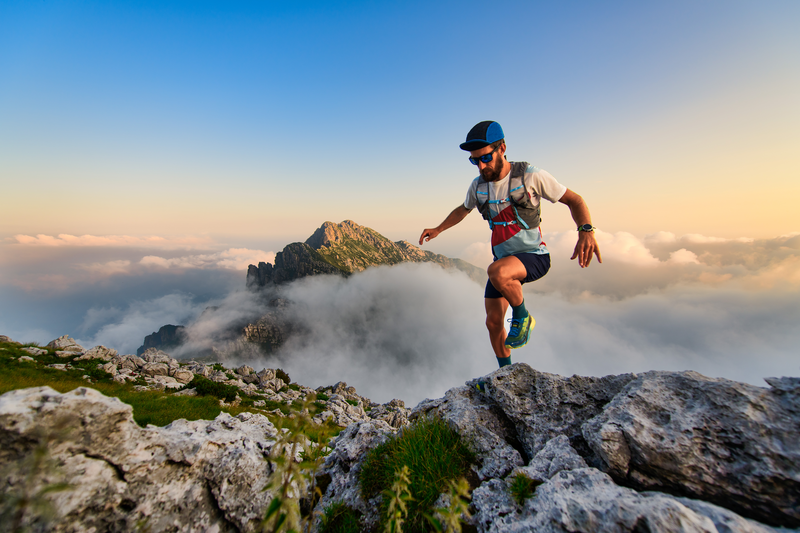
94% of researchers rate our articles as excellent or good
Learn more about the work of our research integrity team to safeguard the quality of each article we publish.
Find out more
ORIGINAL RESEARCH article
Front. Surg.
Sec. Neurosurgery
Volume 12 - 2025 | doi: 10.3389/fsurg.2025.1458572
This article is part of the Research Topic Advancing Evolution in Neuromodulation and Brain Mapping Surgery View all articles
The final, formatted version of the article will be published soon.
You have multiple emails registered with Frontiers:
Please enter your email address:
If you already have an account, please login
You don't have a Frontiers account ? You can register here
Epidural spinal cord stimulation (SCS) is a clinical neuromodulation technique that is commonly used to treat neuropathic pain, with patients typically undergoing a one-week percutaneous trial phase before permanent implantation. Traditional SCS involves stimulation of the thoracic spinal cord, but there has been substantial recent interest in cervical SCS to treat upper extremity pain, restore sensation from the missing hand after amputation, or restore motor function to paretic limbs in people with stroke and spinal cord injury. Because of the additional mobility of the neck, as compared to the trunk, lead migration can be a major challenge for cervical SCS, especially during the percutaneous trial phase. The objective of this study was to optimize the implantation procedure of cervical SCS leads to minimize lead migration and increase lead stability during SCS trials.In this study, four subjects underwent percutaneous placement of three SCS leads targeting the cervical spinal segments as part of a clinical trial aiming to restore sensation for people with upperlimb amputation. The leads were maintained for up to 29 days and weekly x-ray imaging was used to measure rostrocaudal and mediolateral lead migration based on bony landmarks.Lead migration was primarily confined to the rostrocaudal axis with the most migration occurring during the first week. Iterative improvements to the implantation procedure were implemented to increase lead stability across subjects. There was a decrease in lead migration for patients who had more rostral placement of the SCS leads. The average migration from the day of lead implant to lead removal was 29.7 mm for Subject 1 (lead placement ranging from T3-T4 to T1-T2), 41.9 mm for Subject 2 (T2-T3 to C7-T1), 1.9 mm for Subject 3 (T1-T2 to C7-T1), and 16.6 mm for Subject 4 (T1-T2 to C7-T1). We found that initial placement of spinal cord stimulator leads in the cervical epidural space as rostral as possible was critical to minimizing subsequent rostrocaudal lead migration.
Keywords: neuropathic pain, Spinal Cord Stimulation, lead migration, neuroprosthetics, cervical spine
Received: 02 Jul 2024; Accepted: 05 Mar 2025.
Copyright: © 2025 Finney, Levy, Chandrasekaran, Collinger, Boninger, Gaunt, Helm and Fisher. This is an open-access article distributed under the terms of the Creative Commons Attribution License (CC BY). The use, distribution or reproduction in other forums is permitted, provided the original author(s) or licensor are credited and that the original publication in this journal is cited, in accordance with accepted academic practice. No use, distribution or reproduction is permitted which does not comply with these terms.
* Correspondence:
Eric Helm, University of Pittsburgh, Pittsburgh, United States
Lee E Fisher, University of Pittsburgh, Pittsburgh, United States
Disclaimer: All claims expressed in this article are solely those of the authors and do not necessarily represent those of their affiliated organizations, or those of the publisher, the editors and the reviewers. Any product that may be evaluated in this article or claim that may be made by its manufacturer is not guaranteed or endorsed by the publisher.
Research integrity at Frontiers
Learn more about the work of our research integrity team to safeguard the quality of each article we publish.