- Department of Anesthesiology, Quanzhou First Hospital Affiliated to Fujian Medical University, Quanzhou, Fujian, China
Background: Single-lung ventilation (SLV) is a widely used procedure in thoracic surgery; however, it can lead to hypoxemia, which is attributed to intrapulmonary shunt and hypoxic pulmonary vasoconstriction. Stellate ganglion blockade (SGB) has shown protective effects during SLV in various pulmonary conditions. The objective of the study was to assess the clinical utility of ultrasound-guided SGB in patients undergoing thoracoscopic pulmonary lobectomy through a prospective clinical trial.
Methods: This prospective randomized controlled double-blind trial included 116 patients who underwent SLV. After exclusion, 88 patients were randomly assigned to either the SGB group (n = 40) or control group (n = 39), with the latter receiving no SGB. Hemodynamics using oxygenation index (OI) and the pulmonary shunt fraction (Qs/Qt), respiratory mechanics using dynamic lung compliance (Cdyn) and mean airway pressure (Pmean), and levels of pro-inflammatory factors (IL-6 and IL-8) were assessed as clinical outcomes after surgery.
Results: SLV induced upregulation of Pmean, Qs/Qt, and levels of IL-6 and IL-8 and downregulation of Cdyn and OI. Compared to the control group, the SGB group demonstrated significantly decreased Pmean, Qs/Qt, IL-6, and IL-8 and increased Cdyn and OI, suggesting the protective effects of SGB in patients who received SLV.
Conclusions: Ultrasound-guided SGB improves respiratory mechanics, hemodynamics, and inflammatory responses during SLV. Our findings suggest a protective role of SGB in reducing complications associated with SLV.
Clinical Trial Registration: The study was registered in the Chinese Clinical Trial Registry (#ChiCTR2200063210).
Introduction
Single-lung ventilation (SLV) is a widely adopted thoracoscopic technique shown to have promising efficacy in improving patient outcomes (1, 2). Despite this, hypoxemia resulting from poor oxygenation persists as a severe complication of SLV. Previous studies have shown that the collapse and loss of ventilation in the operated lung ultimately lead to a lack of oxygenation and blood return to the left heart. This imbalance of the ventilation/blood flow ratio and increased intrapulmonary shunt (3, 4) is associated with consequent hypoxemia and the initiation of lung self-regulation protection mechanisms, such as hypoxic pulmonary vasoconstriction (HPV) (5). Despite efforts to prevent the occurrence of intrapulmonary shunt and enhance arterial oxygenation during surgery, the incidence of intraoperative hypoxemia remains substantial, ranging from 9% to 27% (6, 7). Consequently, reducing intrapulmonary shunt and preventing hypoxemia during SLV remain critical needs in clinical practice.
The stellate ganglion, also known as the cervicothoracic ganglion, comprises sympathetic ganglia spanning from C3 to C7 and the initial thoracic sympathetic ganglion (8). Existing research concentrates on the protective role of stellate ganglion blockade (SGB) in the lungs—manifested through the inhibition of inflammatory responses, reduction of oxidative stress, suppression of cell apoptosis, regulation of pulmonary vascular tone, and maintenance of autonomic nervous system stability (9, 10). Noteworthy contributions by Liu et al. (11) highlighted the inhibition of pro-inflammatory factors TNF-a and IL-6 in severe trauma patients through SGB, thereby regulating early inflammatory reactions. In addition, SGB alleviates excessive peripheral vasoconstriction and rectifies abnormal hemodynamics by reinstating the balance between the sympathetic and vagus nerves (12). It is worth mentioning that unilateral SGB has been demonstrated to induce vasodilation in vessels innervated by postganglionic fibers, resulting in heightened blood flow velocity, increased blood flow, expanded vessel diameter, and a decrease in vascular resistance, thus theoretically impeding HPV (13). However, the effects of SGB on hypoxic pulmonary vasoconstriction and intrapulmonary shunt during SLV, as well as its potential to induce alterations in oxygen partial pressure, remain unclear.
Herein, this study aims to enroll patients undergoing thoracoscopic pulmonary lobectomy to employ ultrasound-guided SGB to observe its influence on intrapulmonary shunt and oxygenation during SLV, demonstrating the efficacy of this novel and practical approach to alleviate hypoxemia during SLV.
Method
Patients
Patients undergoing elective thoracoscopic pulmonary lobectomy at our hospital were intended to be included. The study was approved by Quanzhou First Hospital affiliated with Fujian Medical University. Informed consent was acquired from the patients. The study was registered in the Chinese Clinical Trial Registry (#ChiCTR2200063210).
Inclusion and exclusion criteria
The inclusion criteria in this research were as follows: (1) patients scheduled for thoracoscopic pulmonary lobectomy, (2) age between 18 and 75 years, and (3) ASA classification I–III. Patients were excluded if they met the following conditions: (1) FEV1/FVC <70%, (2) history of asthma or COPD, (3) acute pulmonary infections, or previous pulmonary surgery (4) receiving atropine and continuous vasoactive drug infusion.
Experimental grouping
The study was designed as a prospective randomized controlled double-blind trial, where participants were randomly assigned to either the SGB group or the control group. In the SGB group, the intervention involved ultrasound-guided right stellate ganglion blockade using 0.5% ropivacaine (7 ml) before anesthesia induction. The control group received standard anesthesia without SGB. Three anesthesiologists participated in the study: the first was responsible for pre-anesthetic stellate ganglion blockade and related preparations, the second for anesthesia induction and maintenance, and the third for clinical data collection and postoperative assessment. The latter two anesthesiologists were blinded to the group allocation.
Stellate ganglion blockade
Upon entering the operating room, routine monitoring measures, including electrocardiogram (ECG), heart rate (HR), blood pressure (BP), and pulse oximetry (SpO2) should be taken by connecting the monitor. Before the induction of general anesthesia, ultrasound-guided SGB was performed on the SGB group. The patients were positioned supine, and under ultrasound guidance, the needle was advanced from the anterior aspect of the neck to the superior aspect of the longus colli muscle at the level of the transverse process of C7.
Anesthesia protocol
Induction involved intravenous administration of fentanyl 0.4 μg/kg, propofol 1.5–2 mg/kg, and rocuronium 0.2 mg/kg. After endotracheal intubation, mechanical ventilation was initiated with maintenance of end-tidal carbon dioxide partial pressure between 35 and 45 mmHg. Anesthesia maintenance included continuous infusion of propofol and remifentanil to keep the BIS between 40 and 60. Fentanyl (5 μg) was administered 20 min before the end of surgery.
Risk analysis
The following lists items used for risk analysis: (1) hemodynamic management involved interventions for deviations from baseline values: when intraoperative mean arterial pressure (MAP) increased by >20% of the basal value or heart rate (HR) exceeded 100 beats/min, intravenous sufentanil 0.1 μg/kg was administered, along with a deepening of anesthesia. In cases of inadequate response, uradil 12.5 mg was intravenously injected. Conversely, if MAP decreased by >20% from the basal value, anesthesia depth was adjusted, and infusion rates were increased. Ephedrine hydrochloride (5–10 mg) was administered if needed. Continuous vasoactive drug infusion was initiated if hypotension persisted, leading to the exclusion of the subject from the study. (2) Bradycardia (<45 beats/minute) prompted observation for surgical-related factors to evaluate whether surgical operation pulls the viscera, with readiness to suspend surgical stimulation at any time. (3) Anesthesia depth was assessed and adjusted. (4) If correction remained insufficient, atropine (0.5 mg IV) was administered, with repeat doses as needed, resulting in exclusion from the study. (5) Intraoperative complications such as massive hemorrhage or anaphylactic shock, particularly those related to SGB, were promptly addressed through emergency resuscitation and subsequent exclusion from the study. (6) Intermediate open surgery, if required, led to the exclusion of the study subject. (7) Failure of ultrasound-guided stellate ganglion block resulted in the exclusion of the subject from the study.
Statistical analysis
Comparative analyses between the control group and SGB group were conducted using independent t-test or Mann–Whitney test for continuous variables and chi-square tests or Fisher's exact test for categorical variables. Continuous variables collected at multiple time points, including hemodynamic parameters, pulmonary dynamics, oxygenation indices, and inflammatory factors, underwent two-way ANOVA analysis with a Bonferroni post hoc test to assess within-group and between-group variations over time. The significance level was set at p < 0.05.
Results
Overview of patient recruitment and group assignment
We evaluated 116 patients undergoing elective thoracoscopic pulmonary lobectomy at our hospital, with 19 patients not meeting the inclusion criteria and 9 refusing to participate (Figure 1). The eligible 88 patients were randomly divided into the SGB group and the control group. Each group received respective interventions. In the control group, two cases were excluded during surgery due to hemodynamic reasons, one case due to bradycardia, one case of intraoperative complications, and one case converted to open chest surgery. Finally, 39 cases were included in the analysis. In the SGB group, one case was excluded due to hemodynamic reasons, one case due to bradycardia, one case of SGB failure, and one case converted to open chest surgery. In the end, 40 cases were included in the analysis. In addition, comparing the baseline data of the two groups, there were no differences in terms of gender, age, surgical side, ASA classification, surgery duration, SLV, and pharmaceutical interventions (additional sufentanil use, uradil use, and ephedrine hydrochloride use) (Table 1).
Evaluation of respiratory mechanics
Hemodynamic parameters were further analyzed through the mean arterial pressure and heart rate of the two groups of patients at five time points: before induction of anesthesia (T1), before single-lung ventilation (T2), 30 min of single-lung ventilation (T3), 1 h of single-lung ventilation (T4), and 30 min after extubation (T5). Our data showed that the patients of the two groups had a decrease in blood pressure and heart rate during anesthesia and single-lung ventilation, and then recovered later on, but there was no significant difference between the two groups at each time point (Table 2). To evaluate how SGB affects respiratory mechanics, we employed the measurement of dynamic lung compliance (Cdyn, Figure 2A) and mean airway pressure (Pmean, Figure 2B). In the control group, with the prolongation of time from T2 to T4 and T5, Cdyn significantly decreased at T4 (p < 0.001), while Pmean significantly increased at T4 (p < 0.0001) and T5 (p < 0.001). We further compared the changes in Cdyn and Pmean values of groups control and SGB group at T2, T4, and T5 time points, respectively. The SGB group caused a significant increase of Cdyn at T4 (p < 0.01) and T5 (p < 0.05) compared with the control group; however, a significant decrease in Pmean at T4 (p < 0.05) and T5 (p < 0.05). To sum up, SLV leads to a decrease in dynamic lung compliance and an increase in mean airway pressure, and the treatment of SGB weakens its impact.
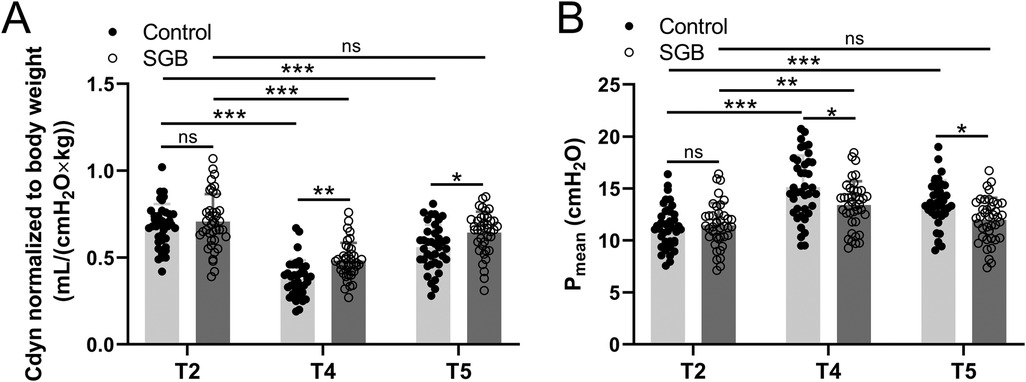
Figure 2. Comparisons of dynamic lung compliance (Cdyn) normalized to body weight (A) and mean airway pressure (Pmean) (B) between the two groups at the time before single-lung ventilation (T2), 1 h since single-lung ventilation (T4), and 30 min after extubation (T5). Data were presented as mean ± SD showing all the data points. n = 40 in the SGB group and n = 39 in the control group. *p < 0.05, **p < 0.01, ***p < 0.001 and ns means no significance.
Evaluation of hemodynamics
We also assessed the changes in hemodynamics brought about by SGB. The pulmonary shunt fraction (Qs/Qt) and oxygenation index (OI) were monitored, and arterial blood and central venous blood were sampled at five time points (T1, T2–T6) for blood gas analysis to calculate the intrapulmonary shunt rate. In the control group, we measured Qs/Qt, and it reached a peak at T4 then decreased in subsequent measurements and at 24 h postoperatively (T6) almost returned to the same level as T1. However, the SGB group had significantly lower Qs/Qt values at T3 (p < 0.001), T4 (p < 0.001), and T5 (p < 0.01) compared to the control group (Figure 3A). Furtherly, from T3 to T6, OI value showed a tendency to decrease compared to T1, whereas the SGB group significantly increased OI at T3 (p < 0.05), T4 (p < 0.001), and T5 (p < 0.05) compared to the control group (Figure 3B). Our results suggested that SGB reversed the elevated Qs/Qt and reduced OI caused by SLV.
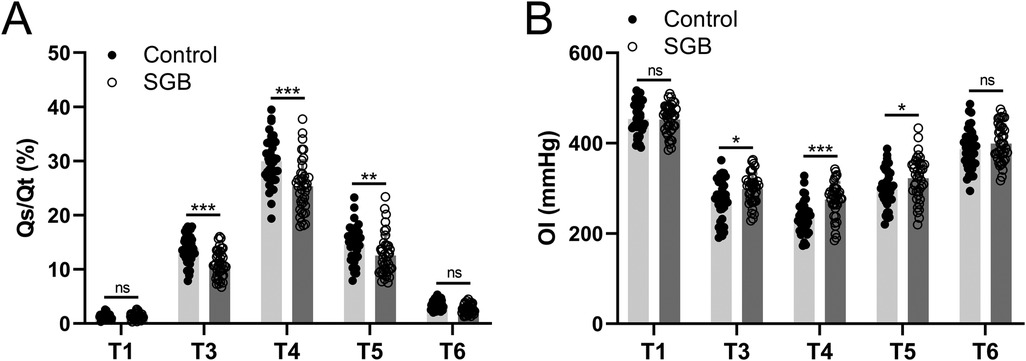
Figure 3. Comparisons of the intrapulmonary shunt (Qs/Qt, A) and oxygenation index (OI, B) between the two groups at the time before anesthetic induction (T1), 30 min since single-lung ventilation (T3), 1 h since single-lung ventilation (T4), 30 min after extubation (T5), and 24 h after surgery (T6). . OI = PaO2/FiO2. Data were presented as mean ± SD showing all the data points. n = 40 in the SGB group and n = 39 in the control group. *p < 0.05, **p < 0.01, ***p < 0.001 and ns means no significance.
Evaluation of inflammatory response
The SLV-induced inflammatory response was measured by the expression of inflammatory factors IL-6 and IL-8 in patients' peripheral blood at T2, T6, and 72 h postoperatively (T7) in Figure 4A. A significant upregulation of IL-6 was observed at T6 (p < 0.001) and T7 (p < 0.05) compared to T2 in the control group, but after treatment with SGB, it significantly downregulated at T6 (p < 0.001) and T7 (p < 0.05) comparing to the control group. Similar results were observed from the expression level of IL-8, i.e., significant upregulations at T6 (p < 0.001) and T7 (p < 0.001) compared to T2 in the control group, but after applying SGB, a significant downregulation of IL-8 was detected at T6 (p < 0.01) compared to the control group (Figure 4B). The expressions of IL-6 and IL-8 in this study indicate that SLV-related upregulations were suppressed by SGB.
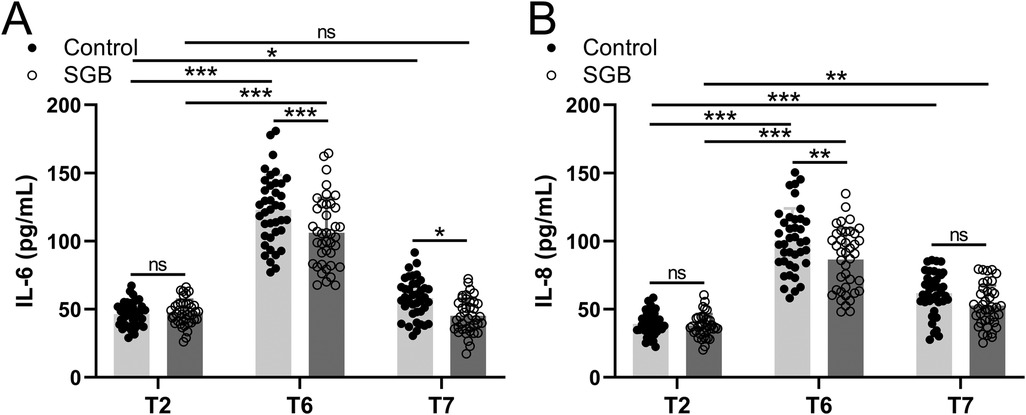
Figure 4. Comparisons of serum IL-6 (A) and IL-8 (B) between the two groups at the time before single-lung ventilation (T2), 24 h after surgery (T6), and 72 h after surgery (T7). Data were presented as mean ± SD showing all the data points. n = 40 in the SGB group and n = 39 in the control group. *p < 0.05, **p < 0.01, ***p < 0.001 and ns means no significance.
Discussion
This study aimed to address a critical clinical gap related to hypoxemia during SLV in patients undergoing thoracoscopic pulmonary lobectomy. Despite the widespread adoption of SLV in thoracic surgery, hypoxemia remains a significant complication, contributing to increased morbidity and mortality (14). HPV is a self-regulatory protective mechanism in the hypoxic state of the pulmonary circulation. HPV begins immediately after SLV treatment, after which it rises as the partial pressure of alveolar oxygen (PO2) decreases, and it has been shown that general anesthetics affect HPV, indicating choosing the right type of anesthesia intraoperatively is critical to the success of SLV (15). Furthermore, the mechanism that leads to hypoxemia is because of the persistent challenge of managing intrapulmonary shunt and optimizing oxygenation during SLV (16), implying an urgent need for alternative approaches to enhance oxygenation and mitigate complications during SLV (17, 18).
Limitations of previous studies include the suboptimal efficacy of existing interventions in addressing the multifaceted nature of hypoxemia during SLV, and efforts have been devoted to improving instruments such as optimizing ventilators and exploring different ventilation modes. The stellate ganglion, renowned for its ability to regulate inflammatory responses and maintain the stability of the autonomic nervous system, posed a distinctive target for intervention. SGB offered a novel intervention for improving respiratory and hemodynamic parameters during SLV (19), and the protective effects of SGB in various pulmonary conditions have been shown, making it a promising avenue for exploring its applications in SLV (20, 21). Moreover, the foundation of this study lay in the potential vasodilatory effects of SGB, which could result in heightened blood flow and reduced vascular resistance in innervated vessels (22). But to our best knowledge, we first observed improvement in dynamic lung compliance and reduction in mean airway pressure in the SGB group during SLV may indicate a potential benefit of SGB in reducing the impact of ventilation on pulmonary function.
Our results also detected the effect of SGB on increasing oxygenation and reducing intrapulmonary shunt, unlike anesthesia methods such as isoflurane (4). It is also worth noting that during SLV, ischemia, and reperfusion of lung tissue, repeated atrophic re-expansion and exposure to stimuli such as surgical operation and excessive stretching of lung tissues are applied, resulting in the promotion of the release of lung-derived inflammatory substances and aggravation of systemic inflammatory response. In this study, SGB also took a part in pulmonary protection through the downregulation of IL-6 and IL-8, which were induced by employing SLV.
These findings may pave the way for a novel, targeted intervention that addresses the complexities of hypoxemia during SLV, ultimately improving patient outcomes in thoracic surgery. The demonstrated improvements in pulmonary dynamics and inflammatory response in our study warrant further exploration in larger cohorts and varied clinical scenarios. Future studies should focus on patient stratification, refine the timing of interventions, and explore the long-term implications of SGB in thoracic surgery. While the study did not reveal a decisive impact on these parameters, the small variations observed may be indicative of a potential role for SGB in specific subsets of patients or under certain conditions. Additionally, the selected time points for data collection may not capture the full spectrum of changes induced by SGB during and after surgery. Our study supports the utilization of SGB in various diseases that involve SLV (23–26).
Conclusions
Ultrasound-guided SGB could be a favorable intervention for mitigating SLV-induced alterations in HPV, intrapulmonary shunt dynamics, oxygen partial pressure, and inflammation.
Data availability statement
The original contributions presented in the study are included in the article/Supplementary Material, further inquiries can be directed to the corresponding author.
Ethics statement
The studies involving humans were approved by Quanzhou First Hospital affiliated with Fujian Medical University. The studies were conducted in accordance with the local legislation and institutional requirements. The participants provided their written informed consent to participate in this study. Written informed consent was obtained from the individual(s) for the publication of any potentially identifiable images or data included in this article.
Author contributions
GZ: Data curation, Validation, Writing – original draft, Writing – review & editing. CS: Data curation, Validation, Writing – original draft, Writing – review & editing. ZK: Data curation, Funding acquisition, Resources, Supervision, Validation, Visualization, Writing – original draft, Writing – review & editing. JZ: Data curation, Validation, Writing – original draft, Writing – review & editing. SL: Data curation, Validation, Writing – original draft, Writing – review & editing.
Funding
The authors declare financial support was received for the research, authorship, and/or publication of this article. The study was supported by the Startup Fund for Scientific Research, Fujian Medical University (Grant number: 2020QH1268) and Quanzhou City Science and Technology Program of China (2021N058S).
Conflict of interest
The authors declare that the research was conducted in the absence of any commercial or financial relationships that could be construed as a potential conflict of interest.
Publisher's note
All claims expressed in this article are solely those of the authors and do not necessarily represent those of their affiliated organizations, or those of the publisher, the editors and the reviewers. Any product that may be evaluated in this article, or claim that may be made by its manufacturer, is not guaranteed or endorsed by the publisher.
References
1. Santos RS, Gan J, Ohara CJ, Daly B, Ebright MI, Desimone M, et al. Microwave ablation of lung tissue: impact of single-lung ventilation on ablation size. Ann Thorac Surg. (2010) 90:1116–9. doi: 10.1016/j.athoracsur.2010.05.068
2. Daiko H, Fujita T, Ohgara T, Yamazaki N, Fujii S, Ohno Y, et al. Minimally invasive hybrid surgery combined with endoscopic and thoracoscopic approaches for submucosal tumor originating from thoracic esophagus. World J Surg Oncol. (2015) 13:40. doi: 10.1186/s12957-015-0452-6
3. Lohser J, Slinger P. Lung injury after one-lung ventilation: a review of the pathophysiologic mechanisms affecting the ventilated and the collapsed lung. Anesth Analg. (2015) 121:302–18. doi: 10.1213/ANE.0000000000000808
4. Zheng X, Lv Z, Yin K, Peng M. Effects of epidural anesthesia combined with inhalation anesthesia or intravenous anesthesia on intrapulmonary shunt and oxygenation in patients undergoing long term single lung ventilation. Pak J Med Sci. (2018) 34:799–803. doi: 10.12669/pjms.344.14585
5. Licker M, Hagerman A, Jeleff A, Schorer R, Ellenberger C. The hypoxic pulmonary vasoconstriction: from physiology to clinical application in thoracic surgery. Saudi J Anaesth. (2021) 15:250–63. doi: 10.4103/sja.sja_1216_20
6. Ju NY, Gao H, Huang W, Niu FF, Lan WX, Li F, et al. Therapeutic effect of inhaled budesonide (pulmicort(R) turbuhaler) on the inflammatory response to one-lung ventilation. Anaesthesia. (2014) 69:14–23. doi: 10.1111/anae.12479
7. Zhang Y, Escher AR Jr, Cohen JB, Liu J. Anesthetic management of right single-lung ventilation in a patient with anomalous left superior pulmonary venous return for left pulmonary lobectomy. Cureus. (2019) 11:5780. doi: 10.7759/cureus.5780
8. Lipov E, Gluncic V, Lukic IK, Candido K. How does stellate ganglion block alleviate immunologically-linked disorders? Med Hypotheses. (2020) 144:110000. doi: 10.1016/j.mehy.2020.110000
9. Chen Y, Xie Y, Xue Y, Wang B, Jin X. Effects of ultrasound-guided stellate ganglion block on autonomic nervous function during CO2-pneumoperitoneum: a randomized double-blind control trial. J Clin Anesth. (2016) 32:255–61. doi: 10.1016/j.jclinane.2016.03.019
10. Yin M, Li ZH, Wang C, Li Y, Zhang H, Du HB, et al. Stellate ganglion blockade repairs intestinal mucosal barrier through suppression of endoplasmic Reticulum stress following hemorrhagic shock. Int J Med Sci. (2020) 17:2147–54. doi: 10.7150/ijms.47662
11. Liu MH, Tian J, Su YP, Wang T, Xiang Q, Wen L. Cervical sympathetic block regulates early systemic inflammatory response in severe trauma patients. Med Sci Monit. (2013) 19:194–201. doi: 10.12659/MSM.883833
12. Deng JJ, Zhang CL, Liu DW, Huang T, Xu J, Liu QY, et al. Treatment of stellate ganglion block in diseases: its role and application prospect. World J Clin Cases. (2023) 11:2160–7. doi: 10.12998/wjcc.v11.i10.2160
13. Samagh N, Panda NB, Gupta V, Bharti N, Tripathi M, Bhagat H, et al. Impact of stellate ganglion block in the management of cerebral vasospasm: a prospective interventional study. Neurol India. (2022) 70:289–95. doi: 10.4103/0028-3886.338735
14. Bernasconi F, Piccioni F. One-lung ventilation for thoracic surgery: current perspectives. Tumori. (2017) 103:495–503. doi: 10.5301/tj.5000638
15. Lumb AB, Slinger P. Hypoxic pulmonary vasoconstriction: physiology and anesthetic implications. Anesthesiology. (2015) 122:932–46. doi: 10.1097/ALN.0000000000000569
16. Li XQ, Tan WF, Wang J, Fang B, Ma H. The effects of thoracic epidural analgesia on oxygenation and pulmonary shunt fraction during one-lung ventilation: an meta-analysis. BMC Anesthesiol. (2015) 15:166. doi: 10.1186/s12871-015-0142-5
17. Verhage RJ, Boone J, Rijkers GT, Cromheecke GJ, Kroese AC, Weijs TJ, et al. Reduced local immune response with continuous positive airway pressure during one-lung ventilation for oesophagectomy. Br J Anaesth. (2014) 112:920–8. doi: 10.1093/bja/aet476
18. Baumgardner JE, Hedenstierna G. Ventilation/perfusion distributions revisited. Curr Opin Anaesthesiol. (2016) 29:2–7. doi: 10.1097/ACO.0000000000000269
19. Wittwer ED, Radosevich MA, Ritter M, Cha YM. Stellate ganglion blockade for refractory ventricular arrhythmias: implications of ultrasound-guided technique and review of the evidence. J Cardiothorac Vasc Anesth. (2020) 34:2245–52. doi: 10.1053/j.jvca.2019.12.015
20. Guo JR, Guo W, Jin XJ, Yu J, Jin BW, Xu F, et al. Effects of stellate ganglionic block on hemodynamic changes and intrapulmonary shunt in perioperative patients with esophageal cancer. Eur Rev Med Pharmacol Sci. (2014) 18:3864–9.25555877
21. Li XF, Jin L, Yang JM, Luo QS, Liu HM, Yu H. Effect of ventilation mode on postoperative pulmonary complications following lung resection surgery: a randomised controlled trial. Anaesthesia. (2022) 77:1219–27. doi: 10.1111/anae.15848
22. Hu N, Wu Y, Chen BZ, Han JF, Zhou MT. Protective effect of stellate ganglion block on delayed cerebral vasospasm in an experimental rat model of subarachnoid hemorrhage. Brain Res. (2014) 1585:63–71. doi: 10.1016/j.brainres.2014.08.012
23. Kucukbingoz C, Tepe O, Ozmen C, Ozbek HT, Deniz A. Can stellate ganglion blockage be an alternative treatment for refractory ventricular arrhythmias: case series. Medicine (Baltimore). (2023) 102:e34135. doi: 10.1097/MD.0000000000034135
24. Lopez-Millan Infantes JM, Coca-Gamito C, Camara-Faraig A, Diaz-Infante E, Garcia-Rubira JC. Stellate ganglion block for the management of electrical storm: an observational study. Rev Esp Anestesiol Reanim (Engl Ed). (2024) 71(1):1–7. doi: 10.1016/j.redare.2023.01.004
25. Luo D, Su Y, Pang Y. Effects of ultrasound-guided stellate ganglion block on postoperative sore throat and postoperative sleep disturbance after lumbar spine surgery: a randomized controlled trial. BMC Anesthesiol. (2023) 23:343. doi: 10.1186/s12871-023-02301-y
Keywords: ultrasound-guided stellate ganglion blockade, single-lung ventilation, intrapulmonary shunt, oxygenation, thoracoscopic pulmonary lobectomy
Citation: Zhu G, Su C, Kang Z, Zeng J and Li S (2024) Effects of ultrasound-guided stellate ganglion block on intrapulmonary shunt and oxygenation in patients with single-lung ventilation. Front. Surg. 11:1438146. doi: 10.3389/fsurg.2024.1438146
Received: 31 May 2024; Accepted: 9 December 2024;
Published: 23 December 2024.
Edited by:
Gianni Angelini, University of Bristol, United KingdomReviewed by:
Thomas E. Sharp III, University of South Florida, United StatesLeonidas Papastavrou, Athens Medical Center, Greece
Yener Aydin, Atatürk University, Turkey
Copyright: © 2024 Zhu, Su, Kang, Zeng and Li. This is an open-access article distributed under the terms of the Creative Commons Attribution License (CC BY). The use, distribution or reproduction in other forums is permitted, provided the original author(s) and the copyright owner(s) are credited and that the original publication in this journal is cited, in accordance with accepted academic practice. No use, distribution or reproduction is permitted which does not comply with these terms.
*Correspondence: Zhenming Kang, a2FuZ3poZW5taW5nQGZqbXUuZWR1LmNu
†These authors have contributed equally to this work