- 1Division of Plastic and Reconstructive Surgery, Department of Surgery, Yale New Haven Hospital, Yale School of Medicine, New Haven, CT, United States
- 2Craniologicum, Center for Craniomaxillofacial Surgery, Bern, Switzerland
- 3Medical Faculty, University of Bern, Bern, Switzerland
Facial implantology, a crucial facet of plastic and reconstructive surgery, focuses on optimizing implant materials for facial augmentation and reconstruction. This manuscript explores the use of Polyetheretherketone (PEEK) implants in craniofacial surgery, highlighting the challenges and advancements in this field. While PEEK offers mechanical resilience, durability, and compatibility with imaging modalities, its biologically inert nature hinders integration with the host tissue, which may lead to complications. In this systematic review, our aim was to assess the current state of knowledge regarding the clinical evaluation of Polyetheretherketone (PEEK) implants in facial implantology, with a focus on craniofacial augmentation and reconstruction in human studies. Additionally, we explore and discuss surface and structural modifications that may enhance bioreactivity and reduce complications in PEEK implants. A systematic review identified 32 articles detailing the use of PEEK Patient-Specific Implants (PSIs) in 194 patients for both reconstructive and aesthetic purposes. Complications, including infections and implant failures, were reported in 18% of cases, suggesting the need for improved implant materials. The discussion delves into the limitations of PEEK, prompting the exploration of surface and structural modifications to enhance its bioreactivity. Strategies, such as hydroxyapatite coating, titanium coating, and porous structures show promise in improving osseointegration and reducing complications. However, the literature review did not reveal reports of coated or modified PEEK in facial reconstructive or aesthetic surgery. In conclusion, although PEEK implants have been successfully used in craniofacial reconstruction, their biological inertness poses challenges. Surface modifications, particularly hydroxyapatite coatings, provide opportunities to promote osseointegration. Future research should focus on prospective long-term studies, especially in craniofacial surgery, to investigate the stability of uncoated PEEK implants and the potential benefits of surface modifications in clinical applications. Patient-specific PEEK implants hold promise for achieving durable craniofacial reconstruction and augmentation.
Introduction
Facial implantology is a scientific branch of plastic surgery that deals with outcome research and continued optimization of currently available implant materials for facial augmentation and reconstruction (1). Facial implants are part of a plastic surgeon's armamentarium to balance facial features, for example for aesthetic considerations and in patients with congenital or acquired mainly hard tissue asymmetries of the craniofacial skeleton. Aging-related predictable volume loss is a common reason for an acquired imbalance of facial features (2). Facial implants, often in combination with other orthognathic interventions, are frequently used to correct such facial asymmetries (3). Historically, autologous grafts were used, however, due to the unreliable nature of autologous grafts in terms of resorption (e.g., bone), donor site morbidity, prolonged operative times, limited availability, and difficult contouring, alloplastic materials have become standard of care for most elective augmentation cases in the adult patient. Alloplastic materials can be tailored to perfectly fit a patient's defect or augmentative needs via computer-assisted design and manufacturing processes to create patient-specific implants (PSI).
Various alloplastic materials, such as metals (e.g., titanium), polymers [silicone, porous polyethylene, polyether-ether ketone (PEEK)], and ceramics (e.g., Hydroxyapatite) can be used (4). However, all of these materials have associated disadvantages and the optimal facial implant has yet to be identified (4, 5). The ideal implant is chemically inert yet integrates with the host, can easily be contoured, is form-stable, and tolerates mechanical stress. In addition, resistance to infection or inflammatory reactions as well as the ability to osseointegrate are also important properties. PEEK implants have emerged as an excellent material for craniofacial augmentation and reconstruction. However, a major drawback of PEEK is its poor bioreactivity and bioinert nature. Thus, the material typically does not integrate with the host (i.e., poor osseointegration and low interfacial adhesion) causing a fibrous layer to form around the implant (6). The low bioreactivity of PEEK implants and the, therefore, often limited incorporation by the host have led to the development of modified PEEK implants. In the field of spinal surgery, the poor osseointegration and incorporation of the PEEK implant (cage) have resulted in fusion failures (7–10).
In this article, we investigate whether coated/modified PEEK implants have been scientifically evaluated for craniofacial augmentation and discuss material modifications that may further improve the clinical utility of this implant material.
Methods
A comprehensive and systematic literature review was conducted by the authors of this review on PubMed/MEDLINE, GoogleScholar, CENTRAL, and Web of Science from database inception to 1st December 2023 for studies investigating whether coated/modified PEEK implants have been clinically evaluated for facial implantology. The following search terms were entered: (PEEK OR Polyetheretherketone) AND (FACE OR FACIAL OR ZYGOMA OR FRONTAL OR MALAR OR MAXILLA OR MANDIBLE OR TEMPORAL). The search format has been adjusted to the appropriate syntax of the respective database. Non-human studies or articles in a language other than English were excluded. Studies looking at isolated cranioplasties were excluded. Additionally, the currently available literature was reviewed to identify all relevant information on PEEK and related coating strategies to enhance the bio-acceptance of PEEK implants, specifically focusing on craniofacial application of the implant material. This study was conducted in accordance with the Preferred Reporting Items for Systematic Reviews and Meta-Analyses (PRISMA) 2020 guidelines (11). This study should be viewed as a descriptive review, as we did not perform a quantitative meta-analysis due to the heterogeneity in outcome parameters. Two reviewers (M.K.-N. and L.K.) independently screened the titles and abstracts of the articles using Covidence (12). A subsequent full-text review was performed manually for abstracts that had been considered eligible. Any disagreements were discussed with a third reviewer (A.-F.S.) and resolved by consensus.
Results
We identified 32 articles reporting data on the use of PEEK PSIs for reconstruction or aesthetic augmentation of the facial skeleton in 194 patients (Table 1, Supplementary Figure S1) (13–23, 25–39, 41–44).
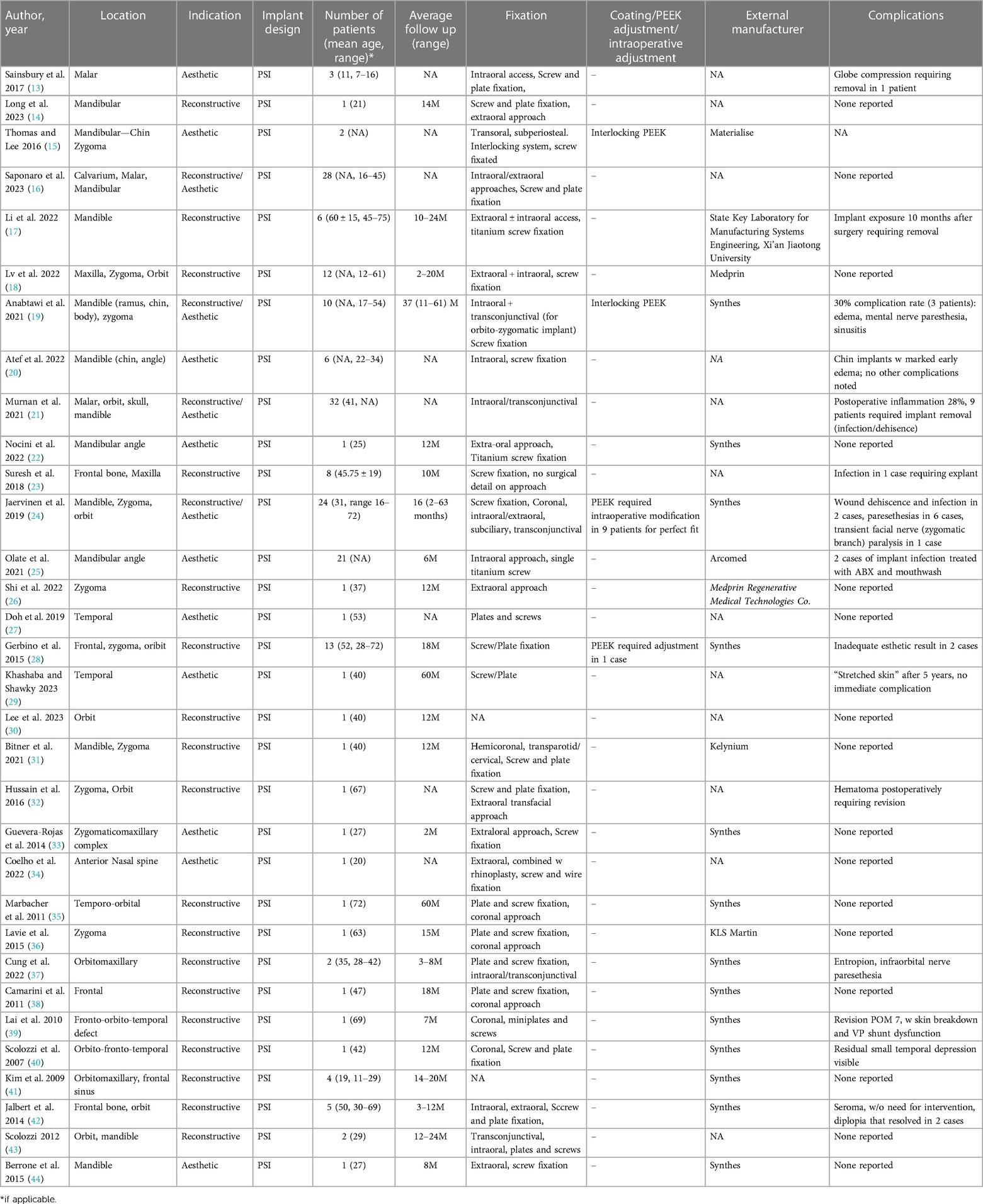
Table 1. Systematic review of the literature summarizing studies that report on PEEK implants used for reconstruction or augmentation of facial structures.
None of the articles described the use of coated PEEK implants or discussed surface modifications. The use of interlocking PEEK was documented in 2 cases. Intraoperative modifications were reported in 2 articles.
The earliest article was published in 2007 by Scolozzi et al. reporting on the use of PEEK PSIs for the reconstruction of an orbito-fronto-temporal post-traumatic defect (40). The majority of articles (18/32; 56%) described the use of PEEK PSIs for reconstructive purposes (e.g., following tumor resection or for the reconstruction of posttraumatic defects), while 10 articles (31%) described primarily aesthetic indications (e.g., congenital deficiencies). Reconstruction/augmentation of the zygoma was described in 14 cases, mandible in 13 cases, orbit in 9 cases, frontal bone in 7, temporal bone in 3, and anterior nasal spine in 1.
In 4 articles, both reconstructive and aesthetic applications were described. The majority of articles were case reports (19/32; 60%). The age range was 7–75 years. Where available, follow-up time was recorded and found to be heterogeneous, ranging from 2 months to 5 years, with a calculated median of 12 months. Various complications in 36 patients (36/194; 18%) were reported, including globe compression requiring removal (1 patient), nerve paresthesia (10), infections/exposure requiring removal (12), facial nerve compression (1), dehiscence/infection not requiring removal (4), inadequate aesthetic result (3), hematoma/seroma (2), entropion (1), and diplopia not requiring removal (2). Details on the manufacturer were available in 20 cases, most of which used Synthes as their PEEK manufacturer (12).
Discussion
Polyetheretherketone (PEEK)
PEEK, a non-resorbable thermoplastic polymer, has become a well-known alloplastic material for cranial vault reconstruction and facial augmentation. PEEK has high mechanical resilience (elastic modulus comparable to cortical bone), is durable and retains its shape, features low thermal reactivity and bioreactivity, and is compatible with standard imaging modalities (i.e., MRI and CT). Additionally, PEEK implants allow intraoperative adjustments by any standard high-speed burrs and patient-individualized plate/screw fixation is easily possible.
However, due to its chemical composition, PEEK implants are biologically inert (7, 45). The high chemical stability is an advantage due to low toxicity, low thermal reactivity, and low peri-implant inflammatory reactions (45). A major drawback of PEEK is its poor bioreactivity and bioinert nature as well as its low antibacterial properties. Accordingly, the material typically fails to integrate with the host (poor osseointegration and low interfacial adhesion), leading to the formation of a fibrous layer around the implant (6). To date, long-term and large-scale studies specifically in the field of facial implantology are absent (Table 1). However, experience in other fields of orthopedic surgery may help guide implant design and selection in the field of facial implantology.
The low bioreactivity of PEEK implants and, hence, frequently limited incorporation by the host have prompted the development of modified PEEK implants (Figure 1). In the field of spinal surgery, the poor osseointegration and incorporation of the PEEK implant (cage) have caused fusion failures (7–10). Further studies in the area of craniofacial surgery revealed a total complication rate of nearly 16% with 9% implant failure in cranioplasties with PEEK (46). In another study, the infection rate was reported to be as high as 8% (24). PEEK implants may also favor biofilm formation and, thus, bacterial adhesion compared to alternative materials (47).
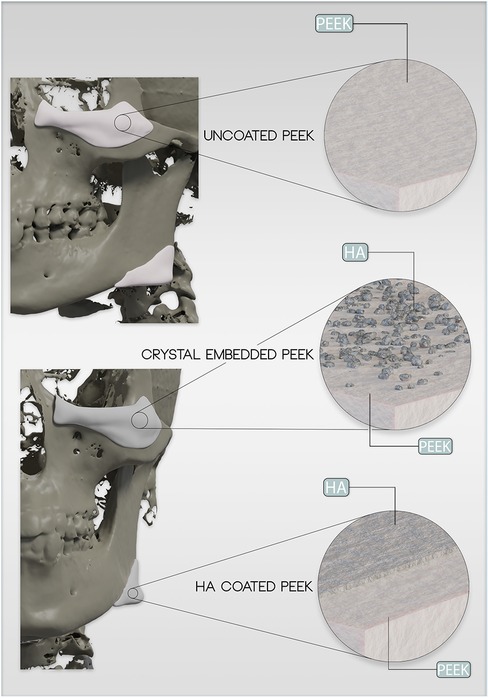
Figure 1. Example of zygomna and mandibular angle implant augmentation using patient-specific PEEK implants. Schematic demonstration of different PEEK-augmentation strategies using HaP embedding or surface coating. Embedded PEEK was designed to limit the possibility of damaging the HaP surface coat during the process of implant insertion.
To improve the characteristics of PEEK implants and the bioreactivity, integration, and ability of the host to control PEEK infections, a series of strategies have been employed which will be reviewed below (8, 48). Surface and structural modifications of existing implants have been described to improve osteoinductive and osteoconductive properties. For example, in spinal surgery, Ti-coated PEEK cages have been shown to offer improved osseointegration compared to uncoated PEEK (49). Our systematic review of the literature did not identify any reports of coated or modified PEEK application in facial reconstructive or aesthetic surgery. However to improve the characteristics of PEEK implants used for craniofacial augmentation and reconstruction, such strategies may need to be applied in order to further improve patient outcomes. Below we discuss some common straties used for ex situ PEEK modification.
Surface and structural modifications
Bioceramic coatings
HaP is a bioceramic material that consists of calcium and phosphate and presents the natural inorganic component of human bone (50). HaP based implants are known to promote superior cellular attachment and integration (osteoconduction, osseointegration) compared to other alloplastic implants (51). Thus, HaP-derived implants are more likely to be incorporated by the recipient when compared to for example titanium or silicone implants. In a study using HaP for facial augmentation, implant volume stability of 99.7% was shown at 2 year follow up (52).
Hence, HaP is considered an excellent material for surface coating (or embedding) of PEEK implants (Figure 1). Hydroxyapatite (HaP) coating or embedding has been studied to improve the bioactivity of PEEK implants. In general, HaP is the most commonly used surface modification for PEEK implants used for spinal surgery (8).
In vivo experiments have demonstrated improved osseointegration of HaP-coated PEEK implants (53). In an in vivo pre-clinical model, HaP-coated PEEK implants were reported to regenerate a larger bone volume on the implant surface and provide superior bone-to-implant contact (53).
Johannson et al. demonstrated that spin-coated PEEK with HaP implanted into rabbit femur/tibia was able to increase removal torque and improve contact between PEEK and native bone (54). In another study, Hahn et al. documented that aerosol-deposited HaP on PEEK enhanced bioreactivity both in vivo and in vitro (55). In vivo studies using a rabbit tibia defect model indicated that HaP coating improved the bone-to-implant contact interface (55). Clinically, HaP-coated PEEK cages were used for anterior cervical spine fusion and demonstrated higher fusion rates compared to uncoated PEEK implants (56).
Other materials that were proposed for PEEK coating are HaP/SiO2 (silicon dioxide) which showed improved osseointegration (57). Other proposed bioceramic coatings include zirconium-dioxide (ZrO2), which is widely used in dental implantology, offering excellent biocompatibility. It, therefore, may also represent a promising option for coating PEEK implants (58). In this context it is worth mentioning that ZrO2-coated dental implants were found to improve the bone-to-implant contact ratio when compared to uncoated implants (59). In addition, ZrO2 exhibits antibacterial properties and promotes osseointegration (8).
Titanium-coated PEEK
Titanium implants are commonly used in craniofacial surgery, for example for orbital reconstruction. Most plating systems are made from titanium due to its high biocompatibility, excellent compatibility with imaging modalities, mechanical strength, and corrosion resistance.
In pre-clinical studies, in vitro co-culturing of 3D-printed Ti-coated PEEK implants with pre-osteoblasts led to improved attachment, proliferation, and differentiation on Ti-coated PEEK samples (60). In an in vivo model, these findings were confirmed, with evidence of improved biocompatibility and bone regeneration on Ti-coated PEEK implants compared to uncoated PEEK implants (60).
In the setting of spine surgery, titanium-coated PEEK cages have shown improved bony fusion rates compared to standard PEEK cages, suggesting improved stability and integration of the implant (56, 61).
Structural modification compared to smooth surface PEEK
Limited osseointegration may be attributed to the fact that PEEK implants are typically smooth surface implants (6). Porous PEEK with 300–400 um porous features (created by melt extrusion technique) was developed with the intent of facilitating bony ingrowth similar to porous polyethylene implants (62). In vivo pre-clinical experiments in a rat model revealed bony ingrowth into the implant with improved osseointegration compared to smooth surface PEEKs. Notably, porous PEEK retained the majority of the solid version's mechanical strength (about 74% of the fatigue resistance of smooth, non-porous, PEEK implants).
An interesting variation are 3D-printed PEEK-hydroxyapatite microporous prints (average pore size of 280 um) which offer improved in vitro cellular attachment and proliferation compared to standard PEEK, although a loss of tensile strength with an increase in HaP component and porosity was seen (48, 63).
Torstrick et al. investigated the effect of titanium-coated PEEK (roughened titanium surface) and porous PEEK implants in comparison to smooth PEEK: porous PEEK implants exhibited the best osteoinductive and osseointegrative properties when compared to smooth and titanium-coated PEEK implants (6).
Nanostructured PEEK implants are a novel biotechnological advancement with the aim of further promoting PEEK implant bioreactivity and osseointegration (8). Nanostructures involve specific topographic modifications, including the creation of pores and pillars on the surface of PEEK implants. Zheng et al. reported that fine surface structure modification can influence cellular behavior and may help promote osseointegration of modified PEEK implants (8).
Conclusion
PEEK implants have successfully been used for craniofacial reconstruction. However, reporting on the outcome is highly heterogeneous with short follow-up time. Additionally, we saw a relatively high complication rate (18%) which may be reflective of the high number of complex case reports that focus on challenging cases. Experience with PEEK implants in other specialties suggests that the biologically inert nature of PEEK implants and the resulting poor integration may have significant downsides. Long-term studies on PEEK implants in craniofacial surgery are warranted to investigate the stability of uncoated PEEK implants.
In pre-clinical studies testing surface modifications, hydroxyapatite has been demonstrated to improve the integration of PEEK implants. Future research is essential to analyze the benefit of PEEK surface coating for clinical applicability—especially in craniofacial surgery. Patient-specific PEEK implants represent a unique opportunity to achieve long-lasting desired reconstruction and augmentation of the craniofacial skeleton.
Author contributions
MK-N: Conceptualization, Data curation, Formal Analysis, Funding acquisition, Investigation, Methodology, Project administration, Resources, Software, Supervision, Validation, Visualization, Writing – original draft, Writing – review & editing. LK: Conceptualization, Investigation, Software, Writing – review & editing. SK: Formal Analysis, Validation, Writing – review & editing. CD: Investigation, Software, Visualization, Writing – review & editing. A-FS: Conceptualization, Data curation, Investigation, Methodology, Software, Supervision, Writing – original draft, Writing – review & editing.
Funding
The author(s) declare that no financial support was received for the research, authorship, and/or publication of this article.
Conflict of interest
The authors declare that the research was conducted in the absence of any commercial or financial relationships that could be construed as a potential conflict of interest.
Publisher's note
All claims expressed in this article are solely those of the authors and do not necessarily represent those of their affiliated organizations, or those of the publisher, the editors and the reviewers. Any product that may be evaluated in this article, or claim that may be made by its manufacturer, is not guaranteed or endorsed by the publisher.
Supplementary material
The Supplementary Material for this article can be found online at: https://www.frontiersin.org/articles/10.3389/fsurg.2024.1351749/full#supplementary-material
Supplementary Figure S1
Prisma flow diagram of the herein presented systematic review.
References
1. Kauke-Navarro M, Safi A-F. Balancing beauty and science: a review of facial implant materials in craniofacial surgery. Front Surg. (2024) 11:1348140. doi: 10.3389/fsurg.2024.1348140
2. Wong C-H, Mendelson B. Newer understanding of specific anatomic targets in the aging face as applied to injectables: aging changes in the craniofacial Skeleton and facial ligaments. Plast Reconstr Surg. (2015) 136:44S–8S. doi: 10.1097/PRS.0000000000001752
3. Rojas YA, Sinnott C, Colasante C, Samas J, Reish RG. Facial implants: controversies and criticism. A comprehensive review of the current literature. Plast Reconstr Surgery. (2018) 142:991–9. doi: 10.1097/PRS.0000000000004765
4. Rubin PJ, Yaremchuk MJ. Complications and toxicities of implantable biomaterials used in facial reconstructive and aesthetic surgery: a comprehensive review of the literature. Plast Reconstr Surg. (1997) 100:1336–53. doi: 10.1097/00006534-199710000-00043
6. Torstrick FB, Lin ASP, Potter D, Safranski DL, Sulchek TA, Gall K, et al. Porous PEEK improves the bone-implant interface compared to plasma-sprayed titanium coating on PEEK. Biomaterials. (2018) 185:106–16. doi: 10.1016/j.biomaterials.2018.09.009
7. Yuan B, Cheng Q, Zhao R, Zhu X, Yang X, Yang X, et al. Comparison of osteointegration property between PEKK and PEEK: effects of surface structure and chemistry. Biomaterials. (2018) 170:116–26. doi: 10.1016/j.biomaterials.2018.04.014
8. Zheng Z, Liu P, Zhang X, Xin J, Wang Y, Zou X, et al. Strategies to improve bioactive and antibacterial properties of polyetheretherketone (PEEK) for use as orthopedic implants. Mater Today Bio. (2022) 16:100402. doi: 10.1016/j.mtbio.2022.100402
9. Yang JJ, Yu CH, Chang B-S, Yeom JS, Lee JH, Lee C-K. Subsidence and nonunion after anterior cervical interbody fusion using a stand-alone polyetheretherketone (PEEK) cage. Clin Orthop Surg. (2011) 3:16. doi: 10.4055/cios.2011.3.1.16
10. Kim PD, Baron EM, Levesque M. Extrusion of expandable stacked interbody device for lumbar fusion: case report of a complication. Spine. (2012) 37:E1155–8. doi: 10.1097/BRS.0b013e318257f14d
11. Page MJ, McKenzie JE, Bossuyt PM, Boutron I, Hoffmann TC, Mulrow CD, et al. The PRISMA 2020 statement: an updated guideline for reporting systematic reviews. Syst Rev. (2021) 10:89. doi: 10.1186/s13643-021-01626-4
12. Kellermeyer L, Harnke B, Knight S. Covidence and rayyan. JMLA. (2018) 106:166–79. doi: 10.5195/jmla.2018.513
13. Sainsbury DCG, George A, Forrest CR, Phillips JH. Bilateral malar reconstruction using patient-specific polyether ether ketone implants in treacher–collins syndrome patients with absent zygomas. J Craniofac Surgery. (2017) 28:515–7. doi: 10.1097/SCS.0000000000003351
14. Long J, Zhang J, Kang J, Fan Y, Zhang Z, Shi J, et al. Customed 3D-printed polyetheretherketone (PEEK) implant for secondary salvage reconstruction of mandibular defects: case report and literature review. J Craniofac Surgery. (2023) 34:2460–3. doi: 10.1097/SCS.0000000000009420
15. Thomas M, Lee NJ. Interlocking polyetheretherketone implant. Int J Oral Maxillofac Surg. (2016) 45:969–70. doi: 10.1016/j.ijom.2016.01.019
16. Saponaro G, Todaro M, Barbera G, Scivoletto G, Foresta E, Gasparini G, et al. Patient-specific facial implants in polyetheretherketone and their stability: a preliminary study. Ann Plast Surg. (2023) 90:564–7. doi: 10.1097/SAP.0000000000003527
17. Li Y, Li Z, Tian L, Li D, Lu B, Shi C, et al. Clinical application of 3D-printed PEEK implants for repairing mandibular defects. J Craniomaxillofac Surgery. (2022) 50:621–6. doi: 10.1016/j.jcms.2022.06.002
18. Lv M, Yang X, Gvetadze SR, Gupta A, Li J, Sun J. Accurate reconstruction of bone defects in orbital–maxillary–zygomatic (OMZ) complex with polyetheretherketone (PEEK). J Plast Reconstr Aesthet Surg. (2022) 75:1750–7. doi: 10.1016/j.bjps.2021.11.101
19. Anabtawi M, Thomas M, Lee NJ. The use of interlocking polyetheretherketone (PEEK) patient-specific facial implants in the treatment of facial deformities. A retrospective review of ten patients. J Oral Maxillofac Surg. (2021) 79:1145.e1–.e9. doi: 10.1016/j.joms.2020.12.009
20. Atef M, Mounir M, Shawky M, Mounir S, Gibaly A. Polyetheretherketone patient-specific implants (PPSI) for the reconstruction of two different mandibular contour deformities. Oral Maxillofac Surg. (2022) 26:299–309. doi: 10.1007/s10006-021-00984-6
21. Murnan EJ, Christensen BJ. Risk factors for postoperative inflammatory complications after maxillofacial reconstruction using polyether-ether-ketone implants. J Oral Maxillofac Surg. (2021) 79:696.e1–e7. doi: 10.1016/j.joms.2020.09.039
22. Nocini R, D’Agostino A, Trevisiol L, Favero V. Mandibular recontouring with polyetheretherketone (PEEK) patient-specific implants. BMJ Case Rep. (2022) 15:e248826. doi: 10.1136/bcr-2022-248826
23. Suresh V, Anolik R, Powers D. The utility of polyether-ether-ketone implants adjacent to Sinus cavities after craniofacial trauma. J Oral Maxillofac Surg. (2018) 76:2361–9. doi: 10.1016/j.joms.2018.05.002
24. Järvinen S, Suojanen J, Kormi E, Wilkman T, Kiukkonen A, Leikola J, et al. The use of patient specific polyetheretherketone implants for reconstruction of maxillofacial deformities. Journal of Cranio-Maxillofacial Surgery. (2019) 47:1072–6. doi: 10.1016/j.jcms.2019.03.018
25. Olate S, Uribe F, Huentequeo-Molina C, Goulart DR, Sigua-Rodriguez EA, Alister JP. Mandibular angle contouring using porous polyethylene stock or PEEK-based patient specific implants. A critical analysis. J Craniofac Surgery. (2021) 32:242–6. doi: 10.1097/SCS.0000000000006926
26. Shi H, Yin X, Hu Y. Solitary neurofibroma of the zygoma: three-dimensional virtual resection and patient-specific polyetheretherketone implant reconstruction. J Craniofac Surgery. (2022) 33:e781–3. doi: 10.1097/SCS.0000000000008526
27. Doh G, Eo S, Hong KY. Temporal hollowing augmentation with polyetheretherketone patient-specific implant. J Craniofac Surgery. (2019) 30:2131–3. doi: 10.1097/SCS.0000000000005629
28. Gerbino G, Zavattero E, Zenga F, Bianchi FA, Garzino-Demo P, Berrone S. Primary and secondary reconstruction of complex craniofacial defects using polyetheretherketone custom-made implants. J Craniomaxillofac Surgery. (2015) 43:1356–63. doi: 10.1016/j.jcms.2015.06.043
29. Khashaba M, Shawky M. Patient-specific PEEK implant for treatment of temporal myositis ossificans (five years follow-up): a case report. J Stomatol Oral Maxillofac Surgery. (2023) 124:101593. doi: 10.1016/j.jormas.2023.101593
30. Lee J, Choi YJ, Rha EY. Orbital reconstruction using a polyetheretherketone patient-specific implant after removal of a mucocele developing after orbital fracture repair. J Craniofac Surgery. (2023) 34:2321–2. doi: 10.1097/SCS.0000000000009636
31. Bitner BF, Wang B, Tjoa T. Mandibular chondrosarcoma reconstructed using custom implant and radial forearm free flap. J Craniofac Surg. (2021) 32:e405–7. doi: 10.1097/SCS.0000000000007137
32. Hussain RN, Clark M, Berry-Brincat A. The use of a polyetheretherketone (PEEK) implant to reconstruct the midface region. Ophthal Plast Reconstr Surgery. (2016) 32:e151–3. doi: 10.1097/IOP.0000000000000345
33. Guevara-Rojas G, Figl M, Schicho K, Seemann R, Traxler H, Vacariu A, et al. Patient-specific polyetheretherketone facial implants in a computer-aided planning workflow. J Oral Maxillofac Surg. (2014) 72:1801–12. doi: 10.1016/j.joms.2014.02.013
34. Coelho DDC, Zille DR, Lima J, Grillo R. Nasal spine implant to correct nasal asymetry and to enable nose correction accompanying orthognathic surgery. J Stomatol Oral Maxillofac Surgery. (2022) 123:491–3. doi: 10.1016/j.jormas.2022.02.016
35. Marbacher S, Andereggen L, Fandino J, Lukes A. Combined bone and soft-tissue augmentation surgery in temporo-orbital contour reconstruction. J Craniofac Surgery. (2011) 22:266–8. doi: 10.1097/SCS.0b013e3181f7b781
36. Lavie J, Stalder MW, St. Hilaire H. Virtual resection and subsequent design of a patient-specific alloplastic implant in the preoperative planning and surgical treatment of a venous malformation of the zygoma. J Craniofac Surgery. (2015) 26:e641–3. doi: 10.1097/SCS.0000000000002042
37. Cung T-D, Hu S, Govindaraj S, Elahi E. Preservation of infraorbital nerve in orbital floor and maxillary defect reconstruction with patient-specific three-dimensional implant: a case report. Ophthal Plast Reconstr Surgery. (2022) 38:e136–41. doi: 10.1097/IOP.0000000000002180
38. Camarini ET, Tomeh JK, Dias RR, Da Silva EJ. Reconstruction of frontal bone using specific implant polyether-ether-ketone. J Craniofac Surgery. (2011) 22:2205–7. doi: 10.1097/SCS.0b013e3182326f2c
39. Lai JB, Sittitavornwong S, Waite PD. Computer-assisted designed and computer-assisted manufactured polyetheretherketone prosthesis for complex fronto-orbito-temporal defect. J Oral Maxillofac Surg. (2011) 69:1175–80. doi: 10.1016/j.joms.2010.05.034
40. Scolozzi P, Martinez A, Jaques B. Complex orbito-fronto-temporal reconstruction using computer-designed PEEK implant. J Craniofac Surgery. (2007) 18:224–8. doi: 10.1097/01.scs.0000249359.56417.7e
41. Kim MM, Boahene KDO, Byrne PJ. Use of customized polyetheretherketone (PEEK) implants in the reconstruction of complex maxillofacial defects. Arch Facial Plast Surg. (2009) 11:53–7. doi: 10.1001/archfaci.11.1.53
42. Jalbert F, Boetto S, Nadon F, Lauwers F, Schmidt E, Lopez R. One-step primary reconstruction for complex craniofacial resection with PEEK custom-made implants. J Craniomaxillofac Surg. (2014) 42:141–8. doi: 10.1016/j.jcms.2013.04.001
43. Scolozzi P. Maxillofacial reconstruction using polyetheretherketone patient-specific implants by “mirroring” computational planning. Aesth Plast Surg. (2012) 36:660–5. doi: 10.1007/s00266-011-9853-2
44. Berrone M, Aldiano C, Pentenero M, Berrone S. Correction of a mandibular asymmetry after fibula reconstruction using a custom-made polyetheretherketone (PEEK) onlay after implant supported occlusal rehabilitation. Acta Otorhinolaryngol Ital. (2015) 35:285–8. PMID: 26824216.26824216
45. Ma R, Tang T. Current strategies to improve the bioactivity of PEEK. Int J Mol Sci. (2014) 15:5426–45. doi: 10.3390/ijms15045426
46. Punchak M, Chung LK, Lagman C, Bui TT, Lazareff J, Rezzadeh K, et al. Outcomes following polyetheretherketone (PEEK) cranioplasty: systematic review and meta-analysis. J Clin Neurosci. (2017) 41:30–5. doi: 10.1016/j.jocn.2017.03.028
47. Sarfraz S, Mäntynen P-H, Laurila M, Rossi S, Leikola J, Kaakinen M, et al. Comparison of Titanium and PEEK medical plastic implant materials for their bacterial biofilm formation properties. Polymers (Basel). (2022) 14:3862. doi: 10.3390/polym14183862
48. Wei Z, Zhang Z, Zhu W, Weng X. Polyetheretherketone development in bone tissue engineering and orthopedic surgery. Front Bioeng Biotechnol. (2023) 11:1207277. doi: 10.3389/fbioe.2023.1207277
49. Kashii M, Kitaguchi K, Makino T, Kaito T. Comparison in the same intervertebral space between titanium-coated and uncoated PEEK cages in lumbar interbody fusion surgery. J Orthop Sci. (2020) 25:565–70. doi: 10.1016/j.jos.2019.07.004
50. Gosain AK, Song L, Riordan P, Amarante MT, Nagy PG, Wilson CR, et al. A 1-year study of osteoinduction in hydroxyapatite-derived biomaterials in an adult sheep model: part I. Plast Reconstr Surg. (2002) 109:619–30. doi: 10.1097/00006534-200202000-00032
51. Ramesh N, Moratti SC, Dias GJ. Hydroxyapatite–polymer biocomposites for bone regeneration: a review of current trends. J Biomed Mater Res. (2018) 106:2046–57. doi: 10.1002/jbm.b.33950
52. Mendelson BC, Jacobson SR, Lavoipierre AM, Huggins RJ. The fate of porous hydroxyapatite granules used in facial skeletal augmentation. Aesthetic Plast Surg. (2010) 34:455–61. doi: 10.1007/s00266-010-9473-2
53. Durham JW, Montelongo SA, Ong JL, Guda T, Allen MJ, Rabiei A. Hydroxyapatite coating on PEEK implants: biomechanical and histological study in a rabbit model. Mater Sci Eng C Mater Biol Appl. (2016) 68:723–31. doi: 10.1016/j.msec.2016.06.049
54. Johansson P, Jimbo R, Kozai Y, Sakurai T, Kjellin P, Currie F, et al. Nanosized hydroxyapatite coating on PEEK implants enhances early bone formation: a histological and three-dimensional investigation in rabbit bone. Materials (Basel). (2015) 8:3815–30. doi: 10.3390/ma8073815
55. Hahn B-D, Park D-S, Choi J-J, Ryu J, Yoon W-H, Choi J-H, et al. Osteoconductive hydroxyapatite coated PEEK for spinal fusion surgery. Appl Surf Sci. (2013) 283:6–11. doi: 10.1016/j.apsusc.2013.05.073
56. Zhu C, He M, Mao L, Li T, Zhang L, Liu L, et al. Titanium-interlayer mediated hydroxyapatite coating on polyetheretherketone: a prospective study in patients with single-level cervical degenerative disc disease. J Transl Med. (2021) 19:14. doi: 10.1186/s12967-020-02688-z
57. Frankenberger T, Graw CL, Engel N, Gerber T, Frerich B, Dau M. Sustainable surface modification of polyetheretherketone (PEEK) implants by hydroxyapatite/silica coating—an in vivo animal study. Materials (Basel). (2021) 14:4589. doi: 10.3390/ma14164589
58. Li J, Qian S, Ning C, Liu X. rBMSC and bacterial responses to isoelastic carbon fiber-reinforced poly(ether-ether-ketone) modified by zirconium implantation. J Mater Chem B. (2016) 4:96–104. doi: 10.1039/C5TB01784J
59. Sollazzo V, Pezzetti F, Scarano A, Piattelli A, Bignozzi C, Massari L, et al. Zirconium oxide coating improves implant osseointegration in vivo. Dent Mater. (2008) 24:357–61. doi: 10.1016/j.dental.2007.06.003
60. Jung H-D, Jang T-S, Lee JE, Park SJ, Son Y, Park S-H. Enhanced bioactivity of titanium-coated polyetheretherketone implants created by a high-temperature 3D printing process. Biofabrication. (2019) 11:045014. doi: 10.1088/1758-5090/ab376b
61. Hasegawa T, Ushirozako H, Shigeto E, Ohba T, Oba H, Mukaiyama K, et al. The titanium-coated PEEK cage maintains better bone fusion with the endplate than the PEEK cage 6 months after PLIF surgery: a multicenter, prospective, randomized study. Spine. (2020) 45:E892–902. doi: 10.1097/BRS.0000000000003464
62. Evans NT, Torstrick FB, Lee CSD, Dupont KM, Safranski DL, Chang WA, et al. High-strength, surface-porous polyether-ether-ketone for load-bearing orthopedic implants. Acta Biomater. (2015) 13:159–67. doi: 10.1016/j.actbio.2014.11.030
Keywords: PEEK, polyether ether ketone (PEEK), implant, face, craniofacial
Citation: Kauke-Navarro M, Knoedler L, Knoedler S, Deniz C and Safi A-F (2024) Surface modification of PEEK implants for craniofacial reconstruction and aesthetic augmentation—fiction or reality? Front. Surg. 11:1351749. doi: 10.3389/fsurg.2024.1351749
Received: 7 December 2023; Accepted: 5 February 2024;
Published: 28 February 2024.
Edited by:
Maria Grazia Raucci, Institute of Polymers, Composites and Biomaterials, National Research Council (IPCB-CNR), ItalyReviewed by:
Farah Asa'ad, University of Gothenburg, SwedenJanosch Schoon, University Medicine Greifswald, Germany
© 2024 Kauke-Navarro, Knoedler, Knoedler, Deniz and Safi. This is an open-access article distributed under the terms of the Creative Commons Attribution License (CC BY). The use, distribution or reproduction in other forums is permitted, provided the original author(s) and the copyright owner(s) are credited and that the original publication in this journal is cited, in accordance with accepted academic practice. No use, distribution or reproduction is permitted which does not comply with these terms.
*Correspondence: Ali-Farid Safi YWxpLWZhcmlkLnNhZmlAZmFjdWx0eS51bmliZS5jaA==