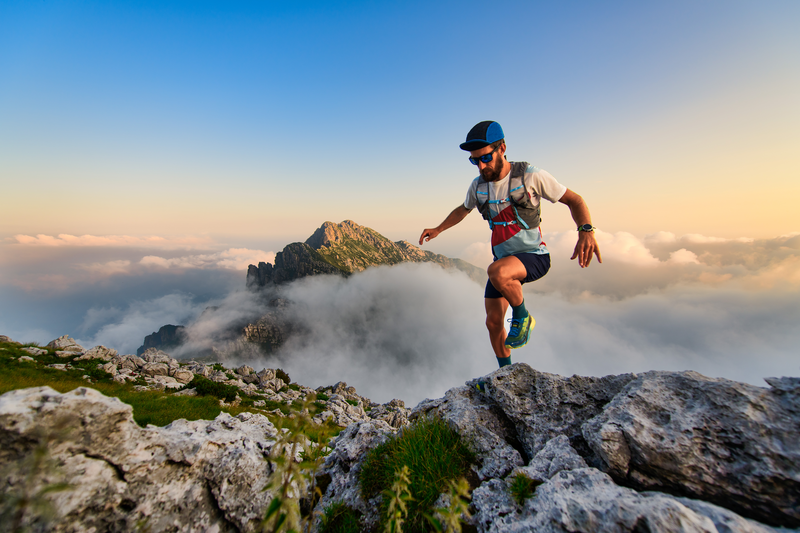
95% of researchers rate our articles as excellent or good
Learn more about the work of our research integrity team to safeguard the quality of each article we publish.
Find out more
ORIGINAL RESEARCH article
Front. Surg. , 14 September 2022
Sec. Orthopedic Surgery
Volume 9 - 2022 | https://doi.org/10.3389/fsurg.2022.965505
This article is part of the Research Topic Clinical Application of Medical Imaging for Functional Evaluation in Orthopaedics View all 21 articles
Objective: To investigate whether preoperative lateral anterior tibial subluxation (LATS) measured from magnetic resonance imaging (MRI) can influence tibial insertion and postoperative sagittal alignment after anterior cruciate ligament reconstructions (ACLRs).
Methods: 84 patients who underwent single-bundle ACLRs were retrospectively investigated. Among them, 39 patients (LATS of <6 mm) 23 patients (LATS of ≥6 mm and <10 mm) and 22 patients (excessive LATS of ≥10 mm) were defined as group 1, 2 and 3, respectively. LATS, the position of graft insertion into tibia as ratio of anterior-posterior width (AP ratio) and the sagittal graft angle (SGA) were postoperatively assessed from MRI at 2-year follow-up. Following linear regression analyses were employed.
Results: The group 3 exhibited the largest preoperative LATS and remained the most postoperative LATS. Moreover, the group 3 possessed the most posteriorly located tunnel insertion with the largest AP ratio and the most vertical graft orientation. Of all included patients, a moderate correlation was demonstrated between pre- and postoperative LATS (r = 0.635). A low correlation was observed between preoperative LATS and AP ratio (r = 0.300) and a moderate correlation was displayed between AP ratio and SGA (r = 0.656).
Conclusion: For ACL injuries with excessive LATS (≥10 mm), most posteriorly located tibial insertion was found out, and worse sagittal alignment containing high residual LATS was associated with more vertical graft orientation following ACLRs.
For single-bundle anterior cruciate ligament (ACL) reconstructions (ACLRs), a general consensus exists that restoring native ACL insertion site and graft orientation can restore knee kinematics, maintain knee stability, and decrease anteroposterior and rotatory instability (1, 2). Anterior tibial subluxation (ATS) in extension, also known as “resting pivoted position”, was radiological abnormal sagittal alignment between femur and tibia following the ACL injury (3). It was reported that lateral ATS (LATS) based on magnetic resonance imaging (MRI) could predict high-grade rotatory knee instability (4) and patients with LATS (≥6 mm) had much more cases of high-grade pivot shift (5).
In order to control LATS and restore rotatory stability postoperatively, numerous researches focus on the location of femoral site during ACLRs. Compared with transtibial (TT) technique, anteromedial portal (AMP) technique can independently create more anatomical femoral tunnel rather than relying on tibial tunnel orientation (6, 7). However, less attention has been paid to the relationship between tibial tunnel insertion and postoperative knee stability. For achieving ideal tibial tunnel site, anatomic references such as a residual stump, anterior horn of lateral meniscus (AHLM), or medial tibial spine were used (8). But it was noteworthy that a significant locating error in tibial tunnel placement persisted using contemporary anatomic ACLR techniques (9), and improper placement of the tibial tunnel insertion could lead to potentially devastating consequences (10).
One study found that an anterior tibial tunnel site controlled Lachman and pivot-shift movements better than a relative posterior tibial footprint (11). Posteriorly placed tibial site might change graft orientation and vertical graft trajectory which could result in rotational knee laxity (12) with aggravating the incidence of early arthrosis (13). Nevertheless, the anterior footprint increased the risk of graft impingement in extension, particularly in positive pivot-shift cases (14). Given close relation between pivot shift and LATS, preoperative LATS raise concerns regarding tibial tunnel malpositioning under ACLRs. However, whether preoperative LATS can influence the tibial tunnel insertion and follow-up sagittal knee alignment has been scarcely investigated following single-bundle ACLRs. Preoperative LATS might be a clue for surgeons to predict postoperative sagittal alignment and knee stability.
The purpose of this study was to investigate whether preoperative LATS can influence the tibial insertion and analyze the sagittal knee alignment. It was hypothesized that (1) preoperative excessive LATS (≥10 mm) would be related to posterior tibial tunnel site, and (2) worse sagittal alignment with residual postoperative LATS in two-year follow-up visit.
From January 2017 to October 2019, 202 consecutive patients with noncontact ACL injuries who subsequently underwent primary single-bundle ACLRs in our department were retrospectively investigated. The exclusion criteria was set as follows: (1) partial ACL injury; (2) comitant PCL, medial collateral ligament, or posterolateral corner injury; (3) general joint laxity [more than 5 of 9 on Beighton score (15)] or significant knee hyperextension (>10°); (4) lost to a follow-up visit, follow-up time <2 years; (5) lack of available magnetic resonance imaging (MRI) data. Based on the exclusion criteria, 84 patients were left for subsequent allocation based on our Institutional Ethics Board.
All single-bundle ACLRs were performed by a single senior surgeon (HW) using 4-strand hamstring tendon autografts. Autografts have a diameter ranging from 8.0 to 9.0 mm. If 4-strand hamstring tendon autografts were less than 8.0 mm, allograft augmentation was conducted to achieve a diameter ranging from 8.0–9.0 mm. The femoral tunnel was independently drilled using the AMP technique with the knee in 120° of flexion, and the tibial tunnel placement was guided according to a combination of anatomic landmarks, with the ACL tibial stump being as the most commonly referenced landmark. The TightRope® suspensory fixation (Arthrex, USA) and Milagro® interference screw (DePuy Mitek, USA) were employed for femoral and tibial tunnel fixations, respectively.
All patients received the same rehabilitation protocol. During the first four weeks, a hinged knee brace was employed and unlocked to allow a passive range of motion from 0° to 90°, emphasizing early passive extension stretching. After four weeks postoperatively, partial weight-bearing was permitted. Full weight-bearing was not started until eight weeks following surgery. Patients were recommended to resume sporting activities at their pre-injury level nine months after surgery.
Preoperative data were individually extracted, including patient demographics [age, sex, body mass index (BMI), the period from injury to surgery, the follow-up time], MRI assessments of preoperative and follow-up LATS, the position of graft insertion into tibia as ratio of anterior-posterior width (AP ratio), the sagittal graft angle (SGA) and the meniscal tears during arthroscopic observation.
All selected patients were supine and scanned using a 1.5-T MRI (Discovery 750, GE Medical Systems) with knee extension in the neutral position on the admission day before ACLR. The images contained sagittal, coronal, and axial sections with T1- and T2-weighted phases. For LATS measurement, the protocol for LATS was according to the method previously described by Tanaka et al (16).
Briefly, a best-fit circle tangent to the lateral posterior femoral condyle border was first drawn on the sagittal section of the most medial edge of the fibula. Along the posterior margin of the circle, a straight line was depicted vertically to the tibial plateau. After that, another parallel line was drawn across the posterior cortex of tibia. The distance between these two lines was identified as LATS. They were performed on both preoperative (Figure 1A) and follow-up MRIs (Figure 1B).
Figure 1. For MRI measurement (A) preoperative and (B) postoperative LATS, the slice showing the most medial edge of the fibula was chosen. Following that, a best-fit circle tangent to the lateral posterior femoral condyle border was first drawn. Along the posterior margin of this circle, a straight line was depicted vertical to the tibial plateau, and another parallel line (dotted line) was drawn across the posterior cortex of the tibia. The distance between these two lines was defined as the amount of LATS. (C) AP ratio was defined as the distance between the anterior tibial margin to the center of tibial graft insertion/the tibia's total AP distance (b/a). A line vertical to the tibia's axis (dotted line) was drawn from the anterior tibial cortex to the posteriormost part of posterior intercondylar margin to confirm the tibia's total AP distance. (D) SGA was defined as the angle between the tangent to the graft and a line perpendicular to the tibia's long axis (dotted line). LATS, lateral anterior tibial subluxation. AP ratio, anteroposterior ratio; SGA, sagittal graft angle.
For tibial tunnel insertion, a line vertical to the tibia's axis was drawn from the anterior tibial cortex to the posteriormost part of posterior intercondylar margin to confirm the tibia's total AP distance. The AP ratio was defined as the distance between anterior tibial margin to the center of tibial graft insertion/the tibia's total AP distance (Figure 1C). As to graft orientation, SGA was defined as the angle between the tangent to the graft and a line perpendicular to the tibia's long axis (Figure 1D) (17, 18).
Two investigators separately measured and recorded the MRI data. Additionally, an independent investigator performed all MRI remeasurements on each selected patient twice within two weeks. The intraclass correlation coefficients (ICCs) were calculated to determine the inter- and intra-observer reliability of measurement by randomly choosing 30 patients (10 from each group). The details regarding inter- and intra-observer ICCs (with 95% confidence intervals) are given in Table 1.
A previous cadaveric study indicated that 6 mm of LATS was necessary to produce a pivot shift and 10 mm of LATS was the mean amount of subluxation in grade I pivot shift (19). Therefore, preoperative LATS was classified into three grade in the present study: <6 mm for low grade, ≥ 6 mm and <10 mm for high grade, ≥ 10 mm for excessive grade.
Statistical analyses were conducted using SPSS software (version 20.0, IBM Corp., USA). Individual variables were tested for normality. Descriptive statistics were calculated for patient demographic data, preoperative and follow-up LATS, SGA, AP ratio and meniscal tears. The Student t-test was used to evaluate continuous variables. The Fisher exact test or the Pearson chi-square test was used to compare categorical variables. Linear regression analyses were performed to test the relationship between pre- and postoperative LATS, AP ratio, respectively. The correlation between AP ratio and SGA was also analyzed. Correlation strength was defined as follows: r value of 0.1–0.3 = weak; 0.3–0.5 = low; 0.5–0.7 = moderate and over 0.7 = high (20). The significant difference was set as p < 0.05. For determining the power of this study, the software G*Power 3.1 (Universität Düsseldorf, Germany) was used to performed post hoc power analysis (21). An effect size was calculated based on the pre- and postoperative LATS. With the underlying effect size and α of 0.05, a power of 1.0 was obtained.
39 patients who has low LATS (<6 mm) were selected as group 1 and left 45 patients were subsequently divided into two groups: high LATS (≥6 mm and <10 mm) (group 2, n = 23) and excessive LATS of ≥ 10 mm (group 3, n = 22) (Figure 2).
Table 2 compared the preoperative characteristics of patients with different grades of LATS. The group 3 with excessive LATS (≥10 mm) exhibited significantly larger LATS than the group 2 and the group 1 (10.6 (10.0–11.1) mm vs. 7.0 (6.6–7.3) mm, p < 0.001; 10.6 (10.0–11.1) mm vs. 2.4 (1.8–3.1) mm, p < 0.001), respectively. Moreover, the group 3 (LATS ≥ 10 mm) contained more cases of high-grade (Grade 2/3) pivot shift than those in the group 1 ((LATS <6 mm) with significance (p = 0.019). However, no differences were found in age, sex, BMI, period from injury to surgery and lateral meniscus tear among three groups.
For postoperative follow-ups (Table 3), postoperative LATS of all three groups were reduced after ACLRs. Whereas, the group 3 remained more residual LATS than the group 2 and the group 1 (6.5 (5.2–7.9) mm vs. 3.8 (2.7–4.9) mm, p = 0.002; 6.5 (5.2–7.9) mm vs. 2.1 (1.3–2.9) mm, p < 0.001), respectively.
In terms of the tibial tunnel insertion, the group 3 had a posteriorly located tibial tunnel site with the AP ratio of 44.1 (41.9–46.3)%. Moreover, its exhibited more vertical sagittal graft orientation with larger SGA [58.3 (55.8–60.8)°] than those of other two groups. However, the comparison between the group 1 and the group 2 revealed no significant difference on AP ratio [40.9 (39.3–42.6)% vs. 41.2 (39.6–42.9)%, p = 0.804] or SGA [53.4 (51.4–55.4) ° vs. 54.0 (52.2–55.9) °, p = 0.670, Table 3].
In all included patients (n = 84), a moderate correlation was shown between preoperative and postoperative LATS [Figure 3A; r = 0.635 (0.487–0.748), p < 0.001]. For tibial insertion analysis, there was a low correlation between preoperative LATS and AP ratio [Figure 3B; r = 0.300 (0.090–0.482), p = 0.006]. Regarding the follow-up sagittal graft orientation, moderate correlation with statistical significance was demonstrated between AP ratio and SGA [Figure 3C; r = 0.656 (0.514–0.763), p < 0.001].
Figure 3. (A) A moderate correlation was found between preoperative and postoperative LATS [r = 0.635 (0.487–0.748), p < 0.001]. (B) A low correlation was found between preoperative LATS and AP ratio [r = 0.300 (0.090–0.482), p = 0.006]. (C) It was demonstrated that SGA was moderately correlated with AP ratio [r = 0.656 (0.514–0.763); p < 0.001]. LATS, lateral anterior tibial subluxation; AP ratio, anteroposterior ratio; SGA, sagittal graft angle.
The current study's findings indicated that for single-bundle ACLRs, ACL injuries with preoperative excessive LATS (≥10 mm) was related to increased posterior tibial tunnel insertion with worse sagittal alignment (postoperative LATS of >6 mm) and more vertical graft orientation at two-year follow-up time.
Several researches have reported that preoperative LATS was found to be a risk factor for predicting the grade of pivot shift in acute ACL injuries (4, 5), and preoperative pivot shift was found to be a risk factor for residual pivot shift following ACLRs (22, 23). Song et al. demonstrated that excessive preoperative ATS for both lateral and medial sides (>10 mm) could not be reduced with anatomic ACLRs with high residual LATS (>6 mm) (24), which was in accordance with our results [6.5 (5.2–7.9) mm].
As previously stated, sufficient coverage of the native ACL footprint is critical for successful restoration of normal knee kinematics (25), but whether the LATS can influence the tibial insertion needs to be investigated. Several studies revealed that tibial site was located at an average AP ratio of 38.5% to 40.7% (26, 27), which was consistent with our results from the group 1 and group 2. However, the group 3 with excessive LATS contained a larger AP ratio indicating that the tibial insertion was posteriorly placed and a low correlation between preoperative LATS and AP ratio (r = 0.300). Therefore, preoperative excessive LATS may be a factor for posteriorly locating tibial insertion.
It was found that a significant positioning error in tibial tunnel placement posterior to the native ACL footprint remains with by using contemporary surgical technique for anatomic ACLRs (9). For ACL injuries with excessive LATS, the subluxated relationship between tibia and femur may mislead surgeons` observation and judgement and aggravate the positioning error. Furthermore, excessive LATS (≥10 mm) could give rise to the concern of possible notch impingement and loss of extension. Consequently, relative posterior tibial tunnel placement might be a compromise on graft impingement that would weaken the knee stability. And for graft orientation, our results find that the group 3 exhibited the most vertical graft angle and moderate correlation was shown between the AP ratio and SGA (r = 0.656, p < 0.001) for all cases. Therefore, the angle of graft orientation might be increased due to posteriorly placed tibial tunnel site, which resulted in worse control of AP subluxation and tibial rotation that were previously reported (28, 29).
Surgical techniques for eliminating LATS in ACL injuries remain controversial. One study reported that a single-bundle ACLR was sufficient to restore ATS and tibial rotation (30), and another research demonstrated that an anatomic ACLR covering the central 2/3 of native ACL footprint could restore knee stability with preoperative Grade-3 pivot shift (31). Unfortunately, our results about postoperative LATS in the excessive group appeared to contradict the above conclusions. Anterolateral ligament (ALL) were reported to be crucial to control LATS as they were secondary stabilizers after ACL injuries to maintain anterolateral stability (22). It is noticeable that several authors (32, 33) recommended that ALL reconstruction or augmentation combined with ACLR could reduce ATS for initially restoring tibiofemoral alignment. Further studies focusing on the potential effect of the ALL reconstruction to the placement of tibia tunnel insertion are required.
We acknowledge that this study contains the following limitations: first, although ATS was 2-dimensionally evaluated using MRI with standard protocol, the measurements may be confounded by leg rotation and knee flexion variations. Second, no immediate postoperative MRI scans were conducted on included patients. After ACLR, the sagittal tibiofemoral alignment was possibly restored to normal at time 0 and then deteriorated over time.
For ACL injuries with excessive LATS (≥10 mm), most posteriorly located tibial insertion was found out, and worse sagittal alignment containing high postoperative LATS was associated with more perpendicular graft orientation following single-bundle ACLRs.
The original contributions presented in the study are included in the article/Supplementary Material, further inquiries can be directed to the corresponding author/s.
The studies involving human participants were reviewed and approved by Second Affiliated Hospital, School of Medicine, Zhejiang University . Written informed consent for participation was not required for this study in accordance with the national legislation and the institutional requirements.
AL, XY prepared original materials and wrote the manuscript. CL and WY made statistics. ZX and SY made commentary and revision. All authors have read and approved the manuscript. HW planned the study project and made supervision. All authors contributed to the article and approved the submitted version.
The authors declare that the research was conducted in the absence of any commercial or financial relationships that could be construed as a potential conflict of interest.
All claims expressed in this article are solely those of the authors and do not necessarily represent those of their affiliated organizations, or those of the publisher, the editors and the reviewers. Any product that may be evaluated in this article, or claim that may be made by its manufacturer, is not guaranteed or endorsed by the publisher.
1. Zaffagnini S, Signorelli C, Bonanzinga T, Roberti Di Sarsina T, Grassi A, Budeyri A, et al. Technical variables of ACL surgical reconstruction: effect on post-operative static laxity and clinical implication. Knee Surg Sports Traumatol Arthrosc. (2016) 24:3496–506. doi: 10.1007/s00167-016-4320-x
2. Zantop T, Herbort M, Raschke MJ, Fu FH, Petersen W. The role of the anteromedial and posterolateral bundles of the anterior cruciate ligament in anterior tibial translation and internal rotation. Am J Sports Med. (2007) 35:223–7. doi: 10.1177/0363546506294571
3. Almekinders LC, De Castro D. Fixed tibial subluxation after successful anterior cruciate ligament reconstruction. Am J Sports Med. (2001) 29:280–3. doi: 10.1177/03635465010290030301
4. Lian J, Novaretti JV, Sheean AJ, Patel NK, Whaley S, Popchak A, et al. Static lateral tibial plateau subluxation predicts high-grade rotatory knee laxity in anterior cruciate ligament–deficient knees. Am J Sports Med. (2019) 47:277–84. doi: 10.1177/0363546518812435
5. Song GY, Zhang H, Zhang J, Liu X, Xue Z, Qian Y, et al. Greater static anterior tibial subluxation of the lateral compartment after an acute anterior cruciate ligament injury is associated with an increased posterior tibial slope. Am J Sports Med. (2018) 46:1617–23. doi: 10.1177/0363546518760580
6. Liu A, Sun M, Ma C, Chen Y, Xue X, Guo P, et al. Clinical outcomes of transtibial versus anteromedial drilling techniques to prepare the femoral tunnel during anterior cruciate ligament reconstruction. Knee Surg Sports Traumatol Arthrosc. (2017) 25:2751–9. doi: 10.1007/s00167-015-3672-y
7. Youm YS, Cho SD, Lee SH, Youn CH. Modified transtibial versus anteromedial portal technique in anatomic single-bundle anterior cruciate ligament reconstruction: comparison of femoral tunnel position and clinical results. Am J Sports Med. (2014) 42:2941–7. doi: 10.1177/0363546514551922
8. Kassam AM, Tillotson L, Schranz PJ, Mandalia VI. The lateral meniscus as a guide to anatomical tibial tunnel placement during anterior cruciate ligament reconstruction. Open Orthop J. (2015) 9:542–7. doi: 10.2174/1874325001509010542
9. Pedneault C, Laverdière C, Hart A, Boily M, Burman M, Martineau PA. Evaluating the accuracy of tibial tunnel placement after anatomic single-bundle anterior cruciate ligament reconstruction. Am J Sports Med. (2019) 47:3187–94. doi: 10.1177/0363546519873633
10. Hosseini A, Lodhia P, Van de Velde SK, Asnis PD, Zarins B, Gill TJ, et al. Tunnel position and graft orientation in failed anterior cruciate ligament reconstruction: a clinical and imaging analysis. Int Orthop. (2012) 36:845–52. doi: 10.1007/s00264-011-1333-4
11. Bedi A, Maak T, Musahl V, Citak M, O'Loughlin PF, Choi D, et al. Effect of tibial tunnel position on stability of the knee after anterior cruciate ligament reconstruction: is the tibial tunnel position most important? Am J Sports Med. (2011) 39:366–73. doi: 10.1177/0363546510388157
12. Lee MC, Seong SC, Lee S, Chang CB, Park YK, Jo H, et al. Vertical femoral tunnel placement results in rotational knee laxity after anterior cruciate ligament reconstruction. Arthroscopy. (2007) 23:771–8. doi: 10.1016/j.arthro.2007.04.016
13. Scanlan SF, Blazek K, Chaudhari AMW, Safran MR, Andriacchi TP. Graft orientation influences the knee flexion moment during walking in patients with Anterior cruciate ligament reconstruction. Am J Sports Med. (2009) 37:2173–8. doi: 10.1177/0363546509339574
14. Zuiderbaan HA, Khamaisy S, Nawabi DH, Thein R, Nguyen JT, Lipman JD, et al. Notchplasty in anterior cruciate ligament reconstruction in the setting of passive anterior tibial subluxation. Knee. (2014) 21:1160–5. doi: 10.1016/j.knee.2014.08.011
15. Redler LH, Dennis ER, Mayer GM, Kalbian IL, Nguyen JT, Shubin Stein BE, et al. Does ligamentous laxity protect against chondral and osteochondral injuries in patients with patellofemoral instability? Orthop J Sports Med. (2022) 10:23259671221107609. doi: 10.1177/23259671221107609
16. Tanaka MJ, Jones KJ, Gargiulo AM, Delos D, Wickiewicz TL, Potter HG, et al. Passive anterior tibial subluxation in anterior cruciate ligament-deficient knees. Am J Sports Med. (2013) 41:2347–52. doi: 10.1177/0363546513498995
17. Stäubli HU, Rauschning W. Tibial attachment area of the anterior cruciate ligament in the extended knee position. Anatomy and cryosections in vitro complemented by magnetic resonance arthrography in vivo. Knee Surg Sports Traumatol Arthrosc. (1994) 2:138–46. doi: 10.1007/BF01467915
18. Konarski A, Strang M, Jain N. The natural orientation of the Anterior Cruciate Ligament compared to the tibial plateau on magnetic resonance imaging scans. J Orthop. (2020) 22:422–6. doi: 10.1016/j.jor.2020.09.010
19. Bedi A, Musahl V, Lane C, Citak M, Warren RF, Pearle AD. Lateral compartment translation predicts the grade of pivot shift: a cadaveric and clinical analysis. Knee Surg Sports Traumatol Arthrosc. (2010) 18:1269–76. doi: 10.1007/s00167-010-1160-y
20. Scheffel PT, Henninger HB, Burks RT. Relationship of the intercondylar roof and the tibial footprint of the ACL: implications for ACL reconstruction. Am J Sports Med. (2013) 41:396–401. doi: 10.1177/0363546512467955
21. Zhang Z, Zhang H, Song G, Wang X, Zhang J, Zheng T, et al. A high-grade J sign is more likely to yield higher postoperative patellar laxity and residual maltracking in patients with recurrent patellar dislocation treated with derotational distal femoral osteotomy. Am J Sports Med. (2020) 48:117–27. doi: 10.1177/0363546519884669
22. Ueki H, Nakagawa Y, Ohara T, Watanabe T, Horie M, Katagiri H, et al. Risk factors for residual pivot shift after anterior cruciate ligament reconstruction: data from the MAKS group. Knee Surg Sports Traumatol Arthrosc. (2018) 26:3724–30. doi: 10.1007/s00167-018-5005-4
23. McDonald LS, van der List JP, Jones KJ, Zuiderbaan HA, Nguyen JT, Potter HG, et al. Passive anterior tibial subluxation in the setting of anterior cruciate ligament injuries. Am J Sports Med. (2017) 45:1537–46. doi: 10.1177/0363546516688673
24. Song GY, Zhang H, Zhang J, Zhang ZJ, Zheng T, Feng H. Excessive preoperative anterior tibial subluxation in extension is associated with Inferior knee stability after anatomic anterior cruciate ligament reconstruction. Am J Sports Med. (2020) 48:573–80. doi: 10.1177/0363546519900158
25. Araujo PH, Asai S, Pinto M, Protta T, Middleton K, Linde-Rosen M, et al. ACL graft position affects in situ graft force following ACL reconstruction. J Bone Joint Surg Am. (2015) 97:1767–73. doi: 10.2106/JBJS.N.00539
26. Lee JK, Lee S, Seong SC, Lee MC. Anatomy of the anterior cruciate ligament insertion sites: comparison of plain radiography and three-dimensional computed tomographic imaging to anatomic dissection. Knee Surg Sports Traumatol Arthrosc. (2015) 23:2297–305. doi: 10.1007/s00167-014-3041-2
27. Parkinson B, Gogna R, Robb C, Thompson P, Spalding T. Anatomic ACL reconstruction: the normal central tibial footprint position and a standardised technique for measuring tibial tunnel location on 3D CT. Knee Surg Sports Traumatol Arthrosc. (2017) 25:1568–75. doi: 10.1007/s00167-015-3683-8
28. Webster KE, Wotherspoon S, Feller JA, McClelland JA. The effect of anterior cruciate ligament graft orientation on rotational knee kinematics. Knee Surg Sports Traumatol Arthrosc. (2013) 21:2113–20. doi: 10.1007/s00167-012-2310-1
29. Zampeli F, Ntoulia A, Giotis D, Tsiaras VA, Argyropoulou M, Pappas E, et al. Correlation between anterior cruciate ligament graft obliquity and tibial rotation during dynamic pivoting activities in patients with anatomic anterior cruciate ligament reconstruction: an in vivo examination. Arthroscopy. (2012) 28:234–46. doi: 10.1016/j.arthro.2011.08.285
30. Zampeli F, Terzidis I, Espregueira-Mendes J, Georgoulis JD, Bernard M, Pappas E, et al. Restoring tibiofemoral alignment during ACL reconstruction results in better knee biomechanics. Knee Surg Sports Traumatol Arthrosc. (2018) 26:1367–74. doi: 10.1007/s00167-017-4742-0
31. Noyes FR, Huser LE, Levy MS. The effect of an ACL reconstruction in controlling rotational knee stability in knees with intact and physiologic laxity of secondary restraints as defined by tibiofemoral compartment translations and graft forces. J Bone Joint Surg Am. (2018) 100:586–97. doi: 10.2106/JBJS.16.01412
32. Littlefield CP, Belk JW, Houck DA, Kraeutler MJ, LaPrade RF, Chahla J, et al. The anterolateral ligament of the knee: an updated systematic review of anatomy, biomechanics, and clinical outcomes. Arthroscopy. (2021) 37:1654–66. doi: 10.1016/j.arthro.2020.12.190
33. Marom N, Ouanezar H, Jahandar H, Zayyad ZA, Fraychineaud T, Hurwit D, et al. Lateral extra-articular tenodesis reduces anterior cruciate ligament graft force and anterior tibial translation in response to applied pivoting and anterior drawer loads. Am J Sports Med. (2020) 48:3183–93. doi: 10.1177/0363546520959322
Keywords: lateral anterior tibial subluxation, tibial tunnel insertion, graft orientation, anterior cruciate ligament, magnetic resonance imaging
Citation: Liu A, Ye X, Li C, Yang W, Yan S, Xin Z and Wu H (2022) Preoperative excessive lateral anterior tibial subluxation is related to posterior tibial tunnel insertion with worse sagittal alignment after anterior cruciate ligament reconstructions. Front. Surg. 9:965505. doi: 10.3389/fsurg.2022.965505
Received: 9 June 2022; Accepted: 31 August 2022;
Published: 14 September 2022.
Edited by:
Tsung-Yuan Tsai, Shanghai Jiao Tong University, ChinaReviewed by:
Qian Kun Ni, Southwest Sports Medicine Center, China© 2022 Liu, Ye, Li, Yang, Yan, Xin and Wu. This is an open-access article distributed under the terms of the Creative Commons Attribution License (CC BY). The use, distribution or reproduction in other forums is permitted, provided the original author(s) and the copyright owner(s) are credited and that the original publication in this journal is cited, in accordance with accepted academic practice. No use, distribution or reproduction is permitted which does not comply with these terms.
*Correspondence: Haobo Wu MjUwNTAxNEB6anUuZWR1LmNu Zengfeng Xin b3N4aW56ZkB6anUuZWR1LmNu
†These authors have contributed equally to this work
Specialty Section: This article was submitted to Orthopaedic Surgery, a section of the journal Frontiers in Surgery
Disclaimer: All claims expressed in this article are solely those of the authors and do not necessarily represent those of their affiliated organizations, or those of the publisher, the editors and the reviewers. Any product that may be evaluated in this article or claim that may be made by its manufacturer is not guaranteed or endorsed by the publisher.
Research integrity at Frontiers
Learn more about the work of our research integrity team to safeguard the quality of each article we publish.