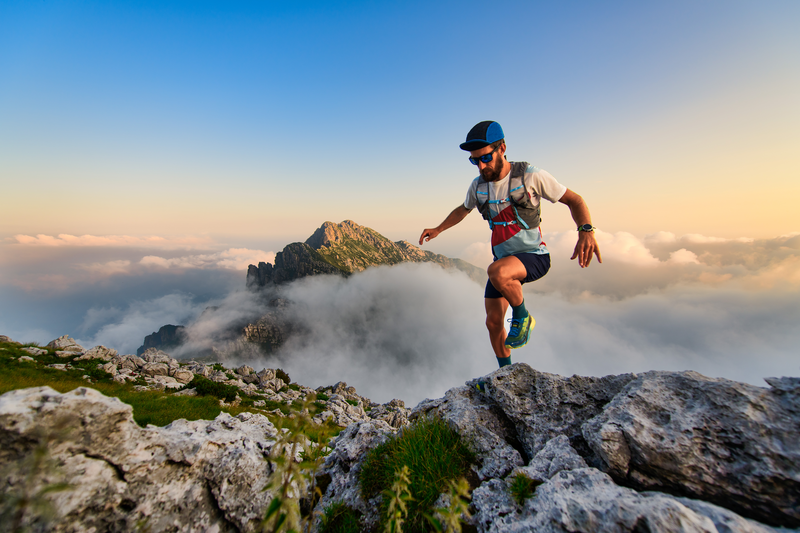
95% of researchers rate our articles as excellent or good
Learn more about the work of our research integrity team to safeguard the quality of each article we publish.
Find out more
SYSTEMATIC REVIEW article
Front. Surg. , 23 September 2022
Sec. Orthopedic Surgery
Volume 9 - 2022 | https://doi.org/10.3389/fsurg.2022.924753
This article is part of the Research Topic Optimization of Spine Surgery Outcomes in the Pre-, Peri-, and Postoperative Settings View all 18 articles
Objective: The purpose of this meta-analysis is to evaluate the effect of the application of platelet-rich plasma (PRP) in spinal fusion surgery on the fusion rate of the spine.
Methods: A comprehensive search of the PubMed, Embase, Cochrane Library, and Science Direct databases was conducted to identify randomized control trials (RCTs) or observational cohort studies that evaluated the efficacy and safety of PRP in spinal fusion. Data on final fusion rate, changes in the visual analog scale (VAS), estimated blood loss (EBL), and operative time was collected from the eligible studies for meta-analysis. Patients were divided into PRP and non-PRP groups according to whether PRP was used during the spinal fusion procedure.
Results: According to the selection criteria, 4 randomized controlled trials and 8 cohort studies with 833 patients and 918 levels were included. The outcomes indicated that PRP application is associated with a lower fusion rat (OR = 0.62, 95% CI: (0.43, 0.89), P = 0.009) at final follow-up (>24 months). Subgroup analysis showed a lower rate of spinal fusion in the PRP group compared to the non-PRP group (OR = 0.35, 95% CI: (0.21, 0.58), P < 0.001) when spinal fusion was assessed using only anterior-posterior radiographs. When the bone graft material was a combination of autologous bone + artificial bone, the spinal fusion rate was lower in the PRP group than in the non-PRP group (OR = 0.34, 95% CI: (0.16, 0.71), P = 0.004). The PRP and non-PRP groups showed no significant differences in VAS changes at the 24th postoperative month (WMD = 0.36, 95% CI: (−0.37, 1.09), P = 0.33); Application of PRP does not reduce the estimated blood loss (WMD = −86.03, 95% CI: (−188.23, 16.17), P = 0.10). In terms of operation time, using PRP does not prolong operation time (WMD = −3.74, 95% CI: (−20.53, 13.04), P = 0.66).
Conclusion: Compared with bone graft fusion alone, PRP cannot increase the rate of spinal fusion. Inappropriate methods of spinal fusion assessment or mixing PRP with artificial/allograft bone may have been responsible for the lower rate of spinal fusion in the PRP group.
Systematic Review Registration: doi: 10.37766/inplasy2022.5.0055
Spinal fusion is an important method used to treat degenerative and traumatic diseases of the spine. Spinal non-fusion refers to the failure of bridging of adjacent vertebrae more than 1 year after surgery (1). Failure of spinal fusion will result in pseudoarthrosis, a common complication after spinal surgery. The formation of a pseudarthrosis often leads to loss of correction, recurrence of deformity, instability of the lumbar spine, low back pain with activity or weight bearing, or neurological symptoms (2). The prevalence of pseudarthrosis reported in the literature ranges from 0% to 56% (3). However, since many patients with pseudarthrosis remain asymptomatic, the true incidence may be underestimated by the literature. The use of bone graft extenders, such as bone morphogenetic proteins (BMPs) or platelet-rich plasma (PRP), has been considered to address this problem (4). Several studies (5–7) have shown that BMPs can improve spinal fusion rates. However, the possible side effects of BMPs, including inflammation, heterotopic bone formation, neck swelling, and radiculitis, have been reported (8, 9). In 2008, the FDA Public Health Notification published an alert regarding safety concerns for BMPs, which led to a gradual decline in their use (10). Therefore, an effective and safe method is needed to increase the rate of bone fusion after spinal fusion surgery.
Platelet activation can produce a variety of autologous growth factors (AFGs), such as platelet-derived growth factor (PDGF) and transforming growth factor-beta (TGF-b) (11). These growth factors promote mitogenesis in fibroblasts, osteoblasts, and mesenchymal cells by stimulating DNA synthesis, allowing them to proliferate and secrete more growth factors, and these cells then differentiate into osteoblasts. In addition, these growth factors also have a chemotactic effect on undifferentiated stem cells (12, 13). Therefore, high concentration of PRP in the fusion levels shows potential as an excellent osteoinductive agent or mitotic factor, which helps to promote bone fusion (14). At present, the technology of producing ultraconcentrated platelets has been produced and promoted as a result of its generated osteogenic action. However whether PRP promotes spinal fusion is inconclusive, even though many meta-analyses currently exist to resolve this controversy. For example, the pooled results of Saran et al. showed no difference in the final spinal fusion rate with the combination of PRP and autologous bone compared to autologous bone graft alone (15). The pooled results of Yolcu et al. (16) showed that the addition of PRP to the spinal fusion process decreased the final spinal fusion rate. Therefore, the objective of this meta-analysis is to re-evaluate the efficacy of PRP, which can aid in the decision-making process regarding the use of PRP in spinal fusion surgery.
The guidelines used for this systematic review and meta-analysis were the Preferred Reporting Items for Systematic Reviews and Meta-Analyses (PRISMA) (17). The protocol for this review was registered on the International Platform of Registered Systematic Review and Meta-analysis Protocols database with the registration number INPLASY202250055 and DOI number 10.37766/inplasy2022.5.0055.
A comprehensive literature search was performed through the following databases: PubMed, EMBASE, Cochrane Library, and ScienceDirect. We identified relevant articles published up to 1 May 2022 without language limitations. Studies were found using the following keywords: platelet-rich plasma, PRP, platelet gel, spinal fusion, bone inducer, and bone extenders. Two independent investigators screened eligible studies and reviewed references of the included studies to identify additional articles. When consensus could not be reached, a third reviewer was consulted.
The inclusion and exclusion criteria of studies followed PICOS principles. (1) Participants: Patients with spinal degenerative or traumatic diseases requiring spinal fusion treatment. (2) Interventions: Patients in whom the bone graft material used for spinal fusion is a mixture of grafted bone and PRP. (3) Comparisons: Patients whose bone graft material used for spinal fusion is bone graft alone. (4) Outcomes: Studies should include at least one of the following data: final spinal fusion rate, final changes of VAS, EBL, and operative time. (5) Study design: Observational studies and randomized control trials were eligible. Case reports, case series, commentaries, practice guidelines, systematic review,s an metaanalysiss were excluded.
The following data were extracted from the included studies: (1) study design: first author, country, publication time, and study type; (2) sample demographics: number of patients and fused levels, follow-up time, age, and sex; (3) fusion details: surgical procedure, bone graft material, imaging modalities for fusion assessment; (4) PRP preparation, formulation, and application methods; and (5) analysis variables: final spinal fusion rate (at least 24 months), final changes of VAS, EBL (excluding the amount of blood consumed for PRP preparation), and operative time. Successful spinal fusion is defined as the presence of bridging bone remodeling between the vertebral bodies or between the bilateral posterolateral intertransverse on static radiographs (anterior-posterior radiographs or CT) (18), or adjacent vertebrae translation <3 mm, angle <5° onflexion-extensionn radiographs (18).
Two researchers independently assessed the risk of bias in randomized trials using the revised Cochrane Risk of Bias tool (RoB-2) (19) and the risk of bias in non-randomized trials using Risk of Bias In Non-Randomized Studies of Interventions (ROBINS-I) (20). Sensitivity analysis was performed by excluding a single study from each study and reanalyzing the data. Publication bias was detected by the Funnel diagram. Sensitivity analysis and publication bias analysis were implemented using RevMan 5.3.
The continuous data were calculated by weighted mean differences (WMDs) with 95% confidence intervals (CIs), and dichotomous variables were calculated by using odds ratios (ORs) with 95% confidence intervals (CIs). Statistical heterogeneity was calculated by using a chi-square test and I2 test. When I2 ≤ 50%, we performed a fixed-effect model for the meta-analysis. Otherwise, the random-effect model was performed. To investigate the impact of fusion assessment tools and the use of different bone grafting materials on spinal fusion rate, we also performed subgroup analysis. The meta-analysis was performed using RevMan 5.3 for Windows (Cochrane Collaboration, Oxford, UK). If the result of the meta-analysis was a probability of P < 0.05, it was statistically significant.
A total of 251 articles from PubMed, EMBASE, the Cochrane Library, and ScienceDirect were initially identified. PubMed (n = 132), EMBASE (n = 62), ScienceDirect (n = 53), and the Cochrane Library (n = 4). 231 studies were directly excluded by screening the titles and abstracts. 20 studies underwent a comprehensive full-text analysis. Finally, 12 studies met the inclusion criteria and were included in this meta-analysis. The flow diagram of the search strategy is summarized in Figure 1.
The eligible studies included 4 randomized control trials and 8 cohort studies. A total of 833 patients and 918 levels were involved in the 12 eligible studies. The PRP group included 364 patients and 401 levels, and the non-PRP group included 469 patients and 517 levels. The characteristics of the included studies are presented in Table 1. The imaging modalities and successful fusion or pseudarthrosis criteria for each study are shown in Table 2. The preparation methods, formulations, and usage of PRP are summarized in Table 3. The results of the quality evaluation of randomized controlled studies and non-randomized controlled studies are summarized in Tables 4, 5.
Table 4. The risk of bias in non-randomized trials using risk of bias in Non-randomized studies of interventions (ROBINS-I).
Table 5. The risk of bias in randomized trials using the revised cochrane risk of bias tool (RoB-2).
A total of 11 studies (21–31) reported final fusion rate in 851 levels (369 levels in the PRP group and 482 levels in the non-PRP group). The outcomes indicated that PRP application is associated with a lower fusion rate, and the difference was statistically significant (OR = 0.62, 95% CI: (0.43, 0.89), P = 0.009), as shown in Figure 2. Subgroup analysis showed a lower rate of spinal fusion in the PRP group compared to the non-PRP group (OR = 0.35, 95% CI: (0.21, 0.58), P < 0.001) when spinal fusion was assessed using only anterior-posterior radiographs (Figure 3). And when the bone graft material was a combination of autologous bone + artificial bone (Figure 4), the spinal fusion rate was lower in the PRP group than in the non-PRP group (OR = 0.34, 95% CI: (0.16, 0.71), P = 0.004).
Four studies (25, 30–32) compared the VAS changes between the PRP group and the non-PRP group at the 24th postoperative month. 70 patients were included in the PRP group, and 75 patients were included in the non-PRP group. A fixed-effect model was used for meta-analysis with I2 = 0%. The outcomes indicated that there was no statistically significant difference in VAS changes between the two groups (WMD = 0.36, 95% CI: (−0.37, 1.09), P = 0.33) (Figure 5).
Four studies (23–25, 30) were available to merge the analysis regarding EBL, including 79 patients in the PRP group and 214 patients in the non-PRP group. A random-effects model was used for meta-analysis with I2 = 65%. The merged data showed that there was no significant difference in EBL between the two groups (WMD = −86.03, 95% CI: (−188.23, 16.17), P = 0.10) (Figure 6).
Four studies (23–25, 30) compared the operation time between the PRP group and the non-PRP group. 79 patients were in the PRP group, and 214 patients were in the non-PRP group. The random-effect model was used for meta-analysis with I2 = 66%. The outcomes indicated that there was no significant difference in operative time between the two groups (WMD = −3.74, 95% CI: (−20.53, 13.04), P = 0.66) (Figure 7).
Sensitivity analysis was performed by excluding a single study of each study and reanalyzing the data. None of the research findings showed significant changes after that analysis. Funnel plots indicated there was minimal to no bias for all included studies (Figure 8).
Fusion rate is considered one of the most important factors in evaluating the clinical efficacy of PRP in lumbar fusion. The clinical use of PRP in promoting spinal fusion is currently controversial. Weiner et al. (27) reasoned that PRP must have an inhibitory effect on osseointegration because PRP may interfere with the generation and function of the bone morphogenetic protein. Similar to Weiner, Castro, et al. (23) examined 22 patients who received TLIF with PRP and compared them to 62 patients who did not get PRP. They discovered no significant changes in the final lumbar fusion between the two groups, hence they did not recommend PRP for clinical use. Unfortunately, the reasons why PRP shows negative effects in clinical applications are inconclusive, and one possible explanation is that only anteroposterior radiographs are used to assess the fusion status of the spine. According to Jordan et al.'s systematic review of evaluation methods for lumbar and cervical spine fusion (3), CT and flexion-extension radiographs are the two preferred imaging modalities for determining the diagnosis of pseudarthrosis. In his description, anteroposterior radiographs had only a 43% to 82% probability of correlation with surgical exploration, with a high rate of false-negative, making them relatively insensitive in the diagnosis of pseudarthrosis (33–37). For this reason, This meta-analysis performed a subgroup analysis based on the imaging modalities used to assess spinal fusion and re-evaluated the ability of PRP promoting spinal fusion. Considering the type of bone graft (autologous, artificial, or allogeneic) may affect the activity of PRP. We also performed a subgroup analysis according to the type of grafted bone. The analysis showed that the combination of autologous bone + artificial bone may decrease the rate of spinal fusion. We all know that autologous bone has excellent osteoconductivity, osteoinductivity and osteogenesis (38). However, artificial bone or allogeneic bone is not biologically active. The implanted autologous bone provides the microporous scaffold structure required for bone growth and has good vascular growth ability, and also provides a large amount of cytokines to promote the activation of osteoblasts, making it the most ideal bone grafting material for spinal fusion (39). Our analysis suggests that the rate of spinal fusion decreased when the artificial bone is mixed with the graft material. One possible explanation is that all PRPs contain high concentrations of leukocytes (according to the PRP production process) and the potential immune response triggered by this may inhibit spinal fusion. However, this requires basic experiments to further validate. Although the subgroup analysis did not reveal that the combination of allogeneic bone + PRP inhibited spinal fusion, such result is questionable due to the small sample size (only one study).
In 2006, the concept of leukocyte-rich PRP was introduced by Everts et al. (40). Therefore, PRP can be broadly classified into two types according to the number of leukocytes contained: leukocyte-poor PRP (LP-PRP) and leukocyte-rich PRP (LR-PRP). unfortunately, none of the included literature mentioned whether the prepared PRP contained leukocytes and the concentration of leukocytes. However, the PRP preparation process was the same for all studies, i.e., the use of gravity centrifugation techniques and equipment to separate the sediment brown-yellow layer from blood units containing platelets and leukocytes, and further concentrate the brown-yellow layer (40). All of the preparation processes did not de-leukocyte the brown-yellow layer, and therefore it can be concluded that the all included studies used LR-PRP. Increased leukocyte (neutrophil) increase levels of pro-inflammatory cytokines, including interleukin-1β (IL-1β) and tumor necrosis factor-α (TNF-α), produce destructive proteases, induce an inflammatory environment, counteract the beneficial effects of growth factors, inhibit extracellular matrix secretion and promote degradation of bone and chondrocytes, impair the ability of differentiated stem cells to differentiate toward bone and cartilage, and eventually leads to degeneration of bone and cartilage tissues and aggravates the pain and swelling response (41). In contrast, the use of LP-PRP effectively reduces the concentration of inflammatory factors, decreasing the inflammatory response and accelerating tissue regeneration. A meta-analysis by Riboh et al. (42) comparing LP-PRP and LR-PRP in the treatment of knee osteoarthritis found that LP-PRP injection significantly improved the Western Ontario and McMaster Universities Osteoarthritis Index (WOMAC) and International Knee Documentation Committee (IKDC) subjective scores compared with LR-PRP or placebo. Also, the application of P-PRP had a lower incidence of adverse reactions compared to LR-PRP. However, there are no clinical trials assessing the efficacy of LP-PRP in promoting spinal fusion or other bone healing. Considering that currently, LR-PRP has not shown any benefit in promoting spinal fusion, LP-PRP could be a potential breakthrough.
In theory, platelet activation increases the inflammatory cascade, which may have a relieving effect on inflammatory pain. Activated platelets release many anti-inflammatory mediators that can reduce inflammation and pain. In a prospective randomized controlled trial (43), researchers evaluated the effect of applying PRP on postoperative pain reduction and functional recovery in patients who underwent open subacromial decompression, and they found patients treated with PRP demonstrated reduced visual analog scales of pain, significantly less use of pain medication, and greater shoulder range of motion compared to control patients.
Although our study did not find additional pain-relieving effects of PRP, a larger sample size is needed for further verification. Applying PRP may reduce EBL, as platelet activation is a key link in blood coagulation (25). However, our results showed that PRP can not reduce EBL. One possible explanation is that bone grafting is often the last step of spinal fusion, and most intraoperative blood loss occurs before bone grafting. It is worth noting that our estimated blood loss refers to intraoperative and postoperative blood loss and does not include the amount of blood required for PRP preparation. According to the gravity centrifugation two-step method of PRP preparation, approximately 450 ml of blood is required to make PRP; however, the concentrated red blood cell layer produced during the preparation process is perfused into the patient, so we cannot obtain the exact amount of blood loss due to PRP preparation. Given that PRP does not reduce intraoperative and postoperative blood loss, it is reasonable to believe that the total blood loss in patients in the PRP group was greater than that in the non-PRP group.
Our meta-analysis also shows that the use of PRP does not prolong the operation time (P = 0.66). As reported in the literature (23), anesthesia and operating room times were significantly prolonged for patients who received PRP. However, in most cases, the preparation of the PRP and its mixing with the autologous bone graft can be performed by the operating room technician, while the surgeon and his assistant can concentrate on preparing the fusion bed. therefore, the PRP preparation process does not waste too much time during the surgery.
We admit that the current research has limitations. To begin, while some relevant trials have been published, the number of participants in some groups was modest, and some of the research were not RCTs. Second, various confounders, such as drug use, disease history, smoking history, and primary disease were not taken into account, which adds to some heterogeneous of the pooled result, but the sensitivity analysis suggested the robustness of the results. Furthermore, there was variability in terms of follow-up time among the included study. However, a minimum follow-up period of 12 months is sufficient to observe the efficacy of PRP in spinal fusion.
Compared with bone graft fusion alone, PRP cannot increase the rate of spinal fusion. Inappropriate methods of spinal fusion assessment or mixing PRP with artificial/allograft bone may have been responsible for the lower rate of spinal fusion in the PRP group.
The original contributions presented in the study are included in the article/Supplementary Material, further inquiries can be directed to the corresponding author/s.
HY and TS proposed research topics and collected literatures; HY and BY drafted the manuscript; ZZ, QT and YJ corrected the English All authors reviewed this article. All authors contributed to the article and approved the submitted version.
This work was supported by Tianjin Key Medical Discipline (Specialty) Construction Project (YJYXZDXK-064B).
The authors declare that the research was conducted in the absence of any commercial or financial relationships that could be construed as a potential conflict of interest.
All claims expressed in this article are solely those of the authors and do not necessarily represent those of their affiliated organizations, or those of the publisher, the editors and the reviewers. Any product that may be evaluated in this article, or claim that may be made by its manufacturer, is not guaranteed or endorsed by the publisher.
1. Raizman NM, O'Brien JR, Poehling-Monaghan KL, Yu WD. Pseudarthrosis of the spine. J Am Acad Orthop Surg. (2009) 17(8):494–503. doi: 10.5435/00124635-200908000-00003
2. Shen J, Wang Q, Wang Y, Min N, Wang L, Wang F, et al. Comparison between fusion and non-fusion surgery for lumbar spinal stenosis: a meta-analysis. Adv Ther. (2021) 38(3):1404–14. doi: 10.1007/s12325-020-01604-7
3. Gruskay JA, Webb ML, Grauer JN. Methods of evaluating lumbar and cervical fusion. Spine J. (2014) 14(3):531–9. doi: 10.1016/j.spinee.2013.07.459
4. Knighton DR, Hunt TK, Thakral KK, Goodson WH 3rd. Role of platelets and fibrin in the healing sequence: an in vivo study of angiogenesis and collagen synthesis. Ann Surg. (1982) 196(4):379–88. doi: 10.1097/00000658-198210000-00001
5. Burkus JK, Sandhu HS, Gornet MF, Longley MC. Use of rhBMP-2 in combination with structural cortical allografts: clinical and radiographic outcomes in anterior lumbar spinal surgery. J Bone Joint Surg Am. (2005) 87(6):1205–12. doi: 10.2106/jbjs.D.02532
6. Haid RJ, Branch CJ, Alexander JT, Burkus JK. Posterior lumbar interbody fusion using recombinant human bone morphogenetic protein type 2 with cylindrical interbody cages. Spine J. (2004) 4(5):527–38; discussion 38–9. doi: 10.1016/j.spinee.2004.03.025
7. Vaccaro AR, Anderson DG, Patel T, Fischgrund J, Truumees E, Herkowitz HN, et al. Comparison of OP-1 Putty (rhBMP-7) to iliac crest autograft for posterolateral lumbar arthrodesis: a minimum 2-year follow-up pilot study. Spine. (2005) 30(24):2709–16. doi: 10.1097/01.brs.0000190812.08447.ba
8. McClellan JW, Mulconrey DS, Forbes RJ, Fullmer N. Vertebral bone resorption after transforaminal lumbar interbody fusion with bone morphogenetic protein (rhBMP-2). J Spinal Disord Tech. (2006) 19(7):483–6. doi: 10.1097/01.bsd.0000211231.83716.4b
9. Shields LB, Raque GH, Glassman SD, Campbell M, Vitaz T, Harpring J, et al. Adverse effects associated with high-dose recombinant human bone morphogenetic protein-2 use in anterior cervical spine fusion. Spine. (2006) 31(5):542–7. doi: 10.1097/01.brs.0000201424.27509.72
10. Makino T, Tsukazaki H, Ukon Y, Tateiwa D, Yoshikawa H, Kaito T. The biological enhancement of spinal fusion for spinal degenerative disease. Int J Mol Sci. (2018) 19(8). doi: 10.3390/ijms19082430
11. Slater M, Patava J, Kingham K, Mason RS. Involvement of platelets in stimulating osteogenic activity. J Orthop Res. (1995) 13(5):655–63. doi: 10.1002/jor.1100130504
12. Centrella M, McCarthy TL, Canalis E. Platelet-derived growth factor enhances deoxyribonucleic acid and collagen synthesis in osteoblast-enriched cultures from fetal rat parietal bone. Endocrinology. (1989) 125(1):13–9. doi: 10.1210/endo-125-1-13
13. Marx RE, Carlson ER, Eichstaedt RM, Schimmele SR, Strauss JE, Georgeff KR. Platelet-rich plasma: growth factor enhancement for bone grafts. Oral Surg Oral Med Oral Pathol Oral Radiol Endod. (1998) 85(6):638–46. doi: 10.1016/S1079-2104(98)90029-4
14. Tullberg T, Brandt B, Rydberg J, Fritzell P. Fusion rate after posterior lumbar interbody fusion with carbon fiber implant: 1-year follow-up of 51 patients. Eur Spine J. (1996) 5(3):178–82. doi: 10.1007/BF00395510
15. Manini DR, Shega FD, Guo C, Wang Y. Role of platelet-rich plasma in spinal fusion surgery: systematic review and meta-analysis. Adv Orthop. (2020) 2020:8361798. doi: 10.1155/2020/8361798
16. Yolcu YU, Wahood W, Eissa AT, Alvi MA, Freedman BA, Elder BD, et al. The impact of platelet-rich plasma on postoperative outcomes after spinal fusion: a systematic review and meta-analysis. J Neurosurg Spine. (2020):1–8. doi: 10.3171/2020.3.Spine2046
17. Shamseer L, Moher D, Clarke M, Ghersi D, Liberati A, Petticrew M, et al. Preferred reporting items for systematic review and meta-analysis protocols (PRISMA-P) 2015: elaboration and explanation. Br Med J. (2015) 350:g7647. doi: 10.1136/bmj.g7647
18. Choudhri TF, Mummaneni PV, Dhall SS, Eck JC, Groff MW, Ghogawala Z, et al. Guideline update for the performance of fusion procedures for degenerative disease of the lumbar spine. Part 4: radiographic assessment of fusion status. J Neurosurg Spine. (2014) 21(1):23–30. doi: 10.3171/2014.4.SPINE14267
19. Hung HY, Hung WL, Shih CL, Chen CY. Drug-induced liver injury by glecaprevir/pibrentasvir treatment for chronic hepatitis C infection: a systematic review and meta-analysis. Ann Med. (2022) 54(1):108–20. doi: 10.1080/07853890.2021.2012589
20. Cheng HY, French CE, Salam AP, Dawson S, McAleenan A, McGuinness LA, et al. Lack of evidence for ribavirin treatment of Lassa fever in systematic review of published and unpublished studies(1). Emerg Infect Dis. (2022) 28(8):1559–68. doi: 10.3201/eid2808.211787
21. Acebal-Cortina G, Suárez-Suárez MA, García-Menéndez C, Moro-Barrero L, Iglesias-Colao R, Torres-Pérez A. Evaluation of autologous platelet concentrate for intertransverse lumbar fusion. Eur Spine J. (2011) 20(Suppl 3):361–6. doi: 10.1007/s00586-011-1904-5
22. Carreon LY, Glassman SD, Anekstein Y, Puno RM. Platelet gel (AGF) fails to increase fusion rates in instrumented posterolateral fusions. Spine. (2005) 30(9):E243–6; discussion E7. doi: 10.1097/01.brs.0000160846.85397.44
23. Castro FJ. Role of activated growth factors in lumbar spinal fusions. J Spinal Disord Tech. (2004) 17(5):380–4. doi: 10.1097/01.bsd.0000110342.54707.19
24. Hee HT, Majd ME, Holt RT, Myers L. Do autologous growth factors enhance transforaminal lumbar interbody fusion? Eur Spine J. (2003) 12(4):400–7. doi: 10.1007/s00586-003-0548-5
25. Jenis LG, Banco RJ, Kwon B. A prospective study of autologous growth factors (AGF) in lumbar interbody fusion. Spine J. (2006) 6(1):14–20. doi: 10.1016/j.spinee.2005.08.014
26. Tsai CH, Hsu HC, Chen YJ, Lin MJ, Chen HT. Using the growth factors-enriched platelet glue in spinal fusion and its efficiency. J Spinal Disord Tech. (2009) 22(4):246–50. doi: 10.1097/BSD.0b013e3181753ae2
27. Weiner BK, Walker M. Efficacy of autologous growth factors in lumbar intertransverse fusions. Spine. (2003) 28(17):1968–70; discussion 71. doi: 10.1097/01.BRS.0000083141.02027.48
28. Hartmann EK, Heintel T, Morrison RH, Weckbach A. Influence of platelet-rich plasma on the anterior fusion in spinal injuries: a qualitative and quantitative analysis using computer tomography. Arch Orthop Trauma Surg. (2010) 130(7):909–14. doi: 10.1007/s00402-009-1015-5
29. Feiz-Erfan I, Harrigan M, Sonntag VK, Harrington TR. Effect of autologous platelet gel on early and late graft fusion in anterior cervical spine surgery. J Neurosurg Spine. (2007) 7(5):496–502. doi: 10.3171/SPI-07/11/496
30. Sys J, Weyler J, Van Der Zijden T, Parizel P, Michielsen J. Platelet-rich plasma in mono-segmental posterior lumbar interbody fusion. Eur Spine J. (2011) 20(10):1650–7. doi: 10.1007/s00586-011-1897-0
31. Kubota G, Kamoda H, Orita S, Inage K, Ito M, Yamashita M, et al. Efficacy of platelet-rich plasma for bone fusion in transforaminal lumbar interbody fusion. Asian Spine J. (2018) 12(1):112–8. doi: 10.4184/asj.2018.12.1.112
32. Kubota G, Kamoda H, Orita S, Yamauchi K, Sakuma Y, Oikawa Y, et al. Platelet-rich plasma enhances bone union in posterolateral lumbar fusion: a prospective randomized controlled trial. Spine J. (2019) 19(2):e34–40. doi: 10.1016/j.spinee.2017.07.167
33. Sugiyama S, Wullschleger M, Wilson K, Williams R, Goss B. Reliability of clinical measurement for assessing spinal fusion: an experimental sheep study. Spine. (2012) 37(9):763–8. doi: 10.1097/BRS.0b013e31822ffa05
34. Albert TJ, Pinto M, Denis F. Management of symptomatic lumbar pseudarthrosis with anteroposterior fusion. A functional and radiographic outcome study. Spine. (2000) 25(1):123–9; discussion 30. doi: 10.1097/00007632-200001010-00021
35. Buchowski JM, Liu G, Bunmaprasert T, Rose PS, Riew KD. Anterior cervical fusion assessment: surgical exploration versus radiographic evaluation. Spine. (2008) 33(11):1185–91. doi: 10.1097/BRS.0b013e318171927c
36. Brodsky AE, Kovalsky ES, Khalil MA. Correlation of radiologic assessment of lumbar spine fusions with surgical exploration. Spine. (1991) 16(6 Suppl):S261–5. doi: 10.1097/00007632-199106001-00017
37. Kant AP, Daum WJ, Dean SM, Uchida T. Evaluation of lumbar spine fusion. Plain radiographs versus direct surgical exploration and observation. Spine. (1995) 20(21):2313–7. doi: 10.1097/00007632-199511000-00009
38. Gerstl JVE, Rendon LF, Burke SM, Doucette J, Mekary RA, Smith TR. Complications and cosmetic outcomes of materials used in cranioplasty following decompressive craniectomy-a systematic review, pairwise meta-analysis, and network meta-analysis. Acta Neurochir. (2022) doi: 10.1007/s00701-022-05251-5
39. Frietsch JJ, Miethke J, Linke P, Crodel CC, Schnetzke U, Scholl S, et al. Treosulfan plus fludarabine versus TEAM as conditioning treatment before autologous stem cell transplantation for B-cell non-hodgkin lymphoma. Bone Marrow Transplant. (2022) 57(7):1164–70. doi: 10.1038/s41409-022-01701-x
40. Everts P, Onishi K, Jayaram P, Lana JF, Mautner K. Platelet-Rich plasma: new performance understandings and therapeutic considerations in 2020. Int J Mol Sci. (2020) 21(20). doi: 10.3390/ijms21207794
41. Niemiec P, Szyluk K, Jarosz A, Iwanicki T, Balcerzyk A. Effectiveness of platelet-rich plasma for lateral epicondylitis: a systematic review and meta-analysis based on achievement of minimal clinically important difference. Orthop J Sports Med. (2022) 10(4):23259671221086920. doi: 10.1177/23259671221086920
42. Riboh JC, Saltzman BM, Yanke AB, Fortier L, Cole BJ. Effect of Leukocyte concentration on the efficacy of platelet-rich plasma in the treatment of knee osteoarthritis. Am J Sports Med. (2016) 44(3):792–800. doi: 10.1177/0363546515580787
43. Everts PA, Devilee RJ, Brown Mahoney C, van Erp A, Oosterbos CJ, Stellenboom M, et al. Exogenous application of platelet-leukocyte gel during open subacromial decompression contributes to improved patient outcome. A Prospective Randomized Double-Blind Study. Eur Surg Res. (2008) 40(2):203–10. doi: 10.1159/000110862
Keywords: fusion rate, platelet-rich plasma (PRP), autologous growth factors, spinal surgery, spinal fusion
Citation: Yu H, Zhou Z, Yu B, Sun T, Tang Q and Jia Y (2022) The efficacy of platelet-rich plasma applicated in spinal fusion surgery: A meta-analysis. Front. Surg. 9:924753. doi: 10.3389/fsurg.2022.924753
Received: 20 April 2022; Accepted: 30 August 2022;
Published: 23 September 2022.
Edited by:
Sravisht Iyer, Hospital for Special Surgery, United StatesReviewed by:
Hiroshi Noguchi, University of Tsukuba, Japan© 2022 Yu, Zhou, Yu, Sun, Tang and Jia. This is an open-access article distributed under the terms of the Creative Commons Attribution License (CC BY). The use, distribution or reproduction in other forums is permitted, provided the original author(s) and the copyright owner(s) are credited and that the original publication in this journal is cited, in accordance with accepted academic practice. No use, distribution or reproduction is permitted which does not comply with these terms.
*Correspondence: Bin Yu cm15eXl1YmluQDE2My5jb20=
†These authors share first authorship.
Specialty Section: This article was submitted to Orthopedic Surgery, a section of the journal Frontiers in Surgery
Abbreviations PRP, platelet-rich plasma; RCTs, randomized control trials; VAS, visual analog scale; EBL, estimated blood loss; BMPs, bone morphogenetic proteins; AFGs, autologous growth factors; PDGF, platelet-derived growth factor; TGF-b, transforming growth factor-beta; WMDs, weighted mean differences; ORs, odds ratios; CIs, confidence intervals.
Disclaimer: All claims expressed in this article are solely those of the authors and do not necessarily represent those of their affiliated organizations, or those of the publisher, the editors and the reviewers. Any product that may be evaluated in this article or claim that may be made by its manufacturer is not guaranteed or endorsed by the publisher.
Research integrity at Frontiers
Learn more about the work of our research integrity team to safeguard the quality of each article we publish.