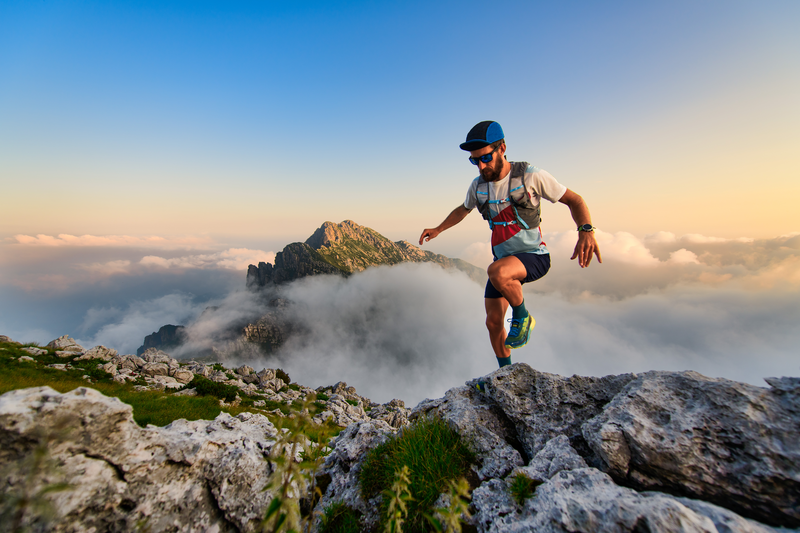
94% of researchers rate our articles as excellent or good
Learn more about the work of our research integrity team to safeguard the quality of each article we publish.
Find out more
SYSTEMATIC REVIEW article
Front. Surg. , 27 May 2022
Sec. Thoracic Surgery
Volume 9 - 2022 | https://doi.org/10.3389/fsurg.2022.914984
Background: Hypoxemia and fluctuations in respiratory mechanics parameters are common during one-lung ventilation (OLV) in thoracic surgery. Additionally, the incidence of postoperative pulmonary complications (PPCS) in thoracic surgery is higher than that in other surgeries. Previous studies have demonstrated that driving pressure-oriented ventilation can reduce both mortality in patients with acute respiratory distress syndrome (ARDS) and the incidence of PPCS in patients undergoing general anesthesia. Our aim was to determine whether driving pressure-oriented ventilation improves intraoperative physiology and outcomes in patients undergoing thoracic surgery.
Methods: We searched MEDLINE via PubMed, Embase, Cochrane, Web of Science, and ClinicalTrials.gov and performed a meta-analysis to compare the effects of driving pressure-oriented ventilation with other ventilation strategies on patients undergoing OLV. The primary outcome was the PaO2/FiO2 ratio (P/F ratio) during OLV. The secondary outcomes were the incidence of PPCS during follow-up, compliance of the respiratory system during OLV, and mean arterial pressure during OLV.
Results: This review included seven studies, with a total of 640 patients. The PaO2/FiO2 ratio was higher during OLV in the driving pressure-oriented ventilation group (mean difference [MD]: 44.96; 95% confidence interval [CI], 24.22–65.70.32; I2: 58%; P < 0.0001). The incidence of PPCS was lower (OR: 0.58; 95% CI, 0.34–0.99; I2: 0%; P = 0.04) and the compliance of the respiratory system was higher (MD: 6.15; 95% CI, 3.97–8.32; I2: 57%; P < 0.00001) in the driving pressure-oriented group during OLV. We did not find a significant difference in the mean arterial pressure between the two groups.
Conclusion: Driving pressure-oriented ventilation during OLV in patients undergoing thoracic surgery was associated with better perioperative oxygenation, fewer PPCS, and improved compliance of the respiratory system.
Systematic Review Registration: PROSPERO, identifier: CRD42021297063.
One-lung ventilation (OLV) has been widely used in thoracic surgery to isolate and protect the lungs (1). However, in the process of OLV, the lung on the non-operated side is still perfused, which causes intrapulmonary shunting (2). This, along with the lateral decubitus positioning of the patient and the intrathoracic pressure imbalance, causes an impairment in ventilation-perfusion matching, a decrease in the compliance of the respiratory system (CRS), and hemodynamic fluctuations (3), all of which may impair arterial oxygenation. Hypoxemia during OLV has always been the most difficult problem for anesthesiologists to overcome (4). These perioperative changes in physiological parameters, combined with mechanical ventilation-induced lung injury, result in a higher incidence of postoperative pulmonary complications (PPCS) in patients undergoing thoracic surgery than in those undergoing other types of surgery (5, 6). Therefore, the choice of intraoperative ventilation strategy is crucial.
The concept of driving pressure was first proposed in regards to patients with ARDS (defined according to the Berlin definition) (7). To minimize ventilator-induced lung injury, most studies scaled tidal volume (VT) by predicted body weight, making the VT facility more closely matched to the patient’s lung size (8, 9). However, in patients with ARDS, the CRS is lower, which significantly reduces the proportion of the lungs that can be ventilated (8, 9). Therefore, we hypothesized that, compared with using VT alone, attributing the VT to the CRS and using the quotient of the two as a predictor of functional lung size could be used to better predict the prognosis of patients with ARDS (7). Therefore, the driving pressure, which is defined as VT/CRS, can simply be calculated as the difference between the plateau pressure and positive end-expiratory pressure (PEEP) (7). Recently, several retrospective studies and systematic reviews have shown that the driving pressure is positively associated with the incidence of ventilator-induced lung injury, with low driving pressure shown to reduce mortality in patients with ARDS (7, 10, 11). Therefore, the relationship between driving pressure and PPCS may be more important than any other parameter (7, 11). Similarly, patients undergoing thoracic surgery with OLV tend to have a higher incidence of intraoperative hypoxemia and worse prognosis than patients with double-lung ventilation (DLV) because only the non-operated lung is ventilated. We hypothesized that such driving pressure-oriented ventilation, which minimizes the driving pressure during mechanical ventilation, would improve intraoperative physiological function and prognosis in patients with OLV.
Previous meta-analyses have demonstrated that a low driving pressure is associated with a lower incidence of PPCS in patients with DLV (11), and recent randomized controlled trials (RCTs) have demonstrated that driving pressure-oriented ventilation improves outcomes in patients with OLV (1). To date, no meta-analysis has demonstrated the applicability of such ventilation strategies in patients with OLV. For this study, RCTs on driving pressure-oriented ventilation in thoracic surgery were reviewed and a meta-analysis was performed to investigate whether driving pressure-oriented ventilation improves intraoperative physiological function and prognosis in patients undergoing OLV.
Ethical approval and patient consent were not required as this was a systematic review and meta-analysis of previously published studies. The study has been registered in PROSPERO. (http://www.crd.york.ac.uk/prospero), registration number: CRD42021297063.This systematic review and meta-analysis was conducted following the PRISMA guidelines. (Supplementary Table S1). (12) The following databases were searched for relevant research in back-to-back experiments by two authors (XL and WX) : MEDLINE via PubMed, Embase, Cochrane, Web of Science, and ClinicalTrials.gov from the first record to December 1, 2021. The search formula is as follows: (“one-lung ventilation”[Title/Abstract] OR “one lung ventilation”[Title/Abstract] OR “single lung ventilation”[Title/Abstract] OR “OLV”[Title/Abstract] OR “thoracic surgery”[Title/Abstract]) AND (“driving pressure”[Title/Abstract]). Disagreements were resolved by discussion with another author (YF).
The inclusion criteria for the study followed the following strategies: (1) Design: Results from our search strategy were limited to randomized controlled trials (RCTs) and human experiments. (2) Age and surgery: Adult (age >18 years) patients undergoing one-lung ventilation for thoracic surgery. (3) Interventions: RCTs using driving pressure-oriented ventilation or RCTs using other ventilation strategies but recording driving pressure during the study. (4) Eligible studies must report oxygenation index or partial pressure of oxygen and must report at least one of the following outcomes: compliance of the respiratory system, mean arterial pressure or incidence of postoperative pulmonary complications. The exclusion criteria for the study followed the following strategies: (1): case reports. (2): observational studies. (3): reviews. (4): Using a driving pressure-oriented ventilation strategy but not thoracic surgery. Two researchers screened all studies after excluding duplicate studies and screened references of included studies for additional relevant studies.
Primary outcomes: The PaO2/FiO2 ratio of patients during one-lung ventilation.
Secondary outcomes: (1) Incidence of postoperative pulmonary complications during follow-up. Postoperative pulmonary complications were assessed using the Melbourne Group Scale: chest x-ray findings of atelectasis or consolidation; raised white cell count [greater than 11.2 × 106/mL] or administration of respiratory antibiotics postoperatively, in addition to prophylactic antibiotics; temperature greater than 38°C; signs of infection on sputum microbiology; purulent sputum different from preoperative status; oxygen saturation less than 90% on room air; physician diagnosis of pneumonia; and prolonged intensive care unit stay [longer stay than 1 and 2 days for lung and esophagus surgery, respectively] or readmission to the intensive care unit. (13) (2) compliance of the respiratory system (CRS) during one-lung ventilation. (3) Mean arterial pressure (MAP) during one-lung ventilation.
For continuous variables: P/F ratio, CRS, and MAP, due to the different time points of measurement, three experiments recorded the time points at which they were measured, and we performed subgroup analysis for the three outcomes.
Two authors (XL and WX) screened the titles and abstracts of initial search results, extracted data, and independently assessed the risk of bias. Get more information by directly asking the corresponding author in the relevant article if needed. Each randomized trial was assessed using the Cochrane Library’s RCT Risk of Bias tool, taking into account the following possible sources of bias. The methodological quality of the trial: random sequence generation; allocation concealment; blinding of participants and raters of lost-to-follow outcomes; incomplete outcome data; selective outcome reporting and other biases. And classify it as “low”, “high” or “unclear” risk. (Figure 2). Disagreements were resolved by discussion with another author (YF).
We performed meta-analyses using Review Manager software (RevMan version 5.4). The coefficient I2 was calculated to assess heterogeneity, which was defined as low (25%–49%), medium (50%–74%), and high (>75%) levels. A random-effects model was used for all analyses due to clinical methodological heterogeneity and other potential heterogeneities. Whenever there was significant heterogeneity, we performed a meta-analysis by omitting one study in turn to find potential sources of heterogeneity. Publication bias was due to the limited number of included studies (<10) and was not assessed. We calculated odds ratios (OR) using 95% CI for dichotomous variables and mean differences (MD) for continuous variables. When reporting continuous results as medians and interquartile ranges in some studies, we used the method described by McGrath et al. (14) to estimate the mean and standard deviation for data pooling for continuous variables. P < 0.05 was considered The difference is statistically significant.
Following the search strategy described above, we obtained 88 relevant articles from our initial search results and two relevant articles from manually reviewing the reference lists of the studies (Supplementary Table S2). Two authors (XL and WX) screened nine studies by reading the titles and abstracts and removing duplicate studies, non-randomized controlled trials, experimental reports, and reviews. After carefully reading the full text of the nine studies, two were excluded and only seven studies were included for full-text evaluation (1, 15–20). A total of 640 patients were included in these seven studies (Figure 1). All patients were adults with an American Society of Anesthesiologist physical status I-III undergoing OLV for thoracic surgery. The Cochrane Collaboration risk of bias tool (Figure 2) indicated that the risk of bias was low for most of the trials.
Table 1 shows the basic characteristics of the included studies. Since the RCTs of individualized PEEP we included all recorded intraoperative driving pressure, and the driving pressure of the experimental group was lower than that control group and this difference was statistically significant, we called “driving pressure-oriented”, that is, to decrease driving pressure during mechanical ventilation, so our study defines the experimental group of these studies as the driving pressure-oriented group. The reasons and details for our inclusion of these studies are provided in Supplementary Table S3.
Among the included studies, five reported PaO2/FiO2 ratio (15, 17–20) and two studies reported the PaO2 and FiO2 (FiO2 = 100% for both studies). (1, 16) In one study, the PaO2/FiO2 ratio was measured after 15 min of OLV (1), in another study, it was measured after 20 min of OLV (18), and in three studies, it was measured after 30 min of OLV (15, 16, 20). Two studies did not mention the specific time of measurement (17, 19). The PaO2/FiO2 ratio decreased in both groups during OLV compared with DLV in all the included studies. The PaO2/FiO2 ratio of the driving pressure-oriented group was higher during OLV (MD: 44.96; 95% CI, 24.22–65.70; I2: 58%; P < 0.0001) (Figure 3).
Three studies reported PPCS (1, 21, 22). All reports followed up on PPCS during the postoperative hospital stay, and the total number of patients with PPCS during postoperative follow-up was included in our analysis. A random effects model was applied, and the incidence of PPCS was found to be 30/224 (13.4%) in the driving pressure-oriented group and 46/226 (20.4%) in the control group. The incidence of PPCS in the driving pressure-oriented group was lower than that in the control group (OR: 0.58; 95% CI, 0.34–0.99; I2: 0%; P = 0.04) (Figure 4).
Figure 4. Summary data forest plot of the number of patients with postoperative pulmonary complications.
The CRS during OLV was reported in five studies (15–18, 20). The CRS was found to be higher in the driving pressure-oriented group than in the control group (MD: 6.15; 95% CI, 3.97–8.32; I2: 57%; P < 0.00001) (Figure 5). Five studies reported mean arterial pressure (MAP) during OLV (15–18, 20). We did not find a significant difference in the MAP between the two groups during OLV (MD: 0.51; 95% CI, −2.85–3.87; I2: 28%; P = 0.77) (Figure 6).
Three studies recorded the PaO2/FiO2 ratio at 0.5 h of OLV, (15, 16, 20) and two studies recorded the PaO2/FiO2 ratio at 1 h of OLV. (15, 20) PaO2/FiO2 ratio was higher in the driving pressure-oriented group at 0.5 h.(MD: 35.64; 95% CI, 7.38–63.90; I2: 39%; P = 0.01) (Supplementary Figure S1). There was no significant difference compared with the control group at 1 h. (Supplementary Figure S2)
At 0.5 h and 1 h of OLV, the CRS of the driving pressure-oriented group was lower than the control group (MD: 4.63; 95% CI, 2.71–6.54; I2: 62%; P < 0.00001), (MD: 5.63; 95% CI, 3.69–7.57; I2: 0%; P < 0.00001) (Supplementary Figures S3, S4).
At 0.5 h and 1 h of OLV, we didn’t find a significant difference in MAP between the driving pressure-oriented group and the control group (Supplementary Figures S5, S6).
We detected a moderate degree of heterogeneity regarding our primary outcome and performed a sensitivity analysis to explore potential reasons for this. The heterogeneity was reduced to 55%, and the mean difference (MD) changed from 44.96 to 51.50 after excluding the study conducted by Zhang et al. (16) The heterogeneity was reduced to 50%, and the MD changed from 44.96 to 51.42 after excluding the study conducted by Park et al. (1) The heterogeneity was reduced to 0%, and the MD changed from 44.96 to 60.62 after excluding both studies (1, 16).
Funnel plots were used to evaluate the publication bias of the included studies. No evidence of publication bias for the primary outcome was suggested by visual inspection of the funnel plots. No significant publication bias was observed (Figure 7).
In this meta-analysis, we investigated and compared the effects of driving pressure-oriented ventilation with those of other ventilation strategies on intraoperative oxygenation, the CRS, MAP, and PPCS in patients undergoing OLV. Our main finding was that driving pressure-oriented ventilation improved the intraoperative PaO2/FiO2 ratio (MD: 44.96; 95% CI, 24.22–65.70; I2: 58%; P < 0.0001), reduced the incidence of PPCS (OR: 0.58; 95% CI, 0.34–0.99; I2: 0%; P = 0.04), improved the CRS during OLV (MD: 6.15; 95% CI, 3.97–8.32; I2: 57%; P < 0.00001), and did not significantly alter the MAP during OLV (MD: 0.51; 95% CI, −2.85–3.87; I2: 28%; P = 0.77).
The vast majority of patients receiving general anesthesia develop atelectasis (23), which impairs intraoperative oxygenation. In patients undergoing thoracic surgery, OLV is prone to intrapulmonary shunting, imbalance in ventilation-perfusion matching, and the proportion of lung that can be ventilated is significantly reduced. These factors ultimately lead to a substantially increased incidence of hypoxemia with OLV, so the choice of ventilation strategy for thoracic surgery is crucial. In recent years, lung-protective ventilation (LPV), which aims to use low VT and PEEP with the recruitment maneuver to prevent PPCs. (21, 22, 24, 25) However, low VT does not appear to be associated with lower PPCS (26–29). Moreover, individual characteristics, such as chest wall size and shape, abdominal contents, lung weight, and pleural pressure, vary from person to person, so a fixed PEEP may not be appropriate for everyone (30). Therefore, the definition of protective ventilation in thoracic surgery is still unclear. As we mentioned above, driving pressure can set the optimal VT and PEEP. (7). Our results show that patients’ intraoperative PaO2/FiO2 ratio and CRS with driving pressure-oriented ventilation were higher compared with other ventilation modalities. The results of the subgroup analysis showed that the PaO2/FiO2 ratio of the driving pressure group was still higher than that of the control group after 0.5 h of OLV. However, at 1 h of OLV, there was no significant difference in the PaO2/FiO2 ratio between the two groups. We speculate that this may be explained by the fact that the hypoxic pulmonary vasoconstriction response in the patients in the two groups took effect after 1 h of OLV, which reduces shunting and improves oxygenation, so in the study of Xu et al.(20). There was little difference in the PaO2/FiO2 ratio between the two groups (25). The subgroup analysis of the CRS showed that the driving pressure group had a higher CRS at both time points (0.5 h and 1 h), indicating that the effect of driving pressure on intraoperative respiratory mechanics was persistent over time. Therefore, driving pressure-oriented ventilation has a certain guiding effect on the setting of VT and PEEP during ventilation and may become the target of a new protective ventilation strategy (31).
Inappropriate ventilation can exacerbate ventilator-induced lung injury and cause the release of a large number of inflammatory cytokines from lung endothelium and alveoli, which eventually leads to PPCS (32, 33). The occurrence of PPCS can delay patient recovery, increase the hospital length of stay and cost of hospitalization, and even lead to death. For many years, the use of LPV in thoracic surgery has been increasingly promoted to reduce the incidence of PPCS (21, 22, 24); however, changes in the VT and PEEP were not found to be associated with PPCS, or were found to be associated with PPCS only when they caused changes in the driving pressure (7). Our findings suggest that driving pressure-oriented ventilation reduces the incidence of PPCS in patients undergoing thoracic surgery, which is consistent with previous findings (1). Despite some heterogeneity in the type of surgery, the results of previous studies have demonstrated that driving pressure-oriented ventilation can reduce the incidence of PPCS and improve patient prognosis (1, 34). Since driving pressure is a new concept, this strategy is more widely used in patients with ARDS, and the number of RCTs that have focused on this aspect of thoracic surgery is limited. Although there may be some limitations in terms of predicting the incidence of PPCs, the results did not suggest high heterogeneity. Our findings may provide some guidance in decision-making regarding intraoperative ventilation strategies for thoracic surgeries.
Since the operated lung is collapsed during OLV, the pleural pressure will be unbalanced, which may have a certain impact on hemodynamics during ventilation. However, our MAP results showed no significant differences in the MAP during OLV between the two groups. Subgroup analyses also showed no significant differences between the groups, indicating that the change in driving pressure may only have an effect on lung compliance or may only affect the lung itself, while its effect on the pleural pressure may be comparable to other modes of ventilation. However, only a few studies were included in our analysis, especially in the subgroup analysis. In addition, differences in the amount of intraoperative fluid infusion, the use of vasoactive drugs, and the method of anesthesia used in the included studies will affect hemodynamic fluctuations; therefore, our findings should be interpreted with caution. More RCTs are needed to compare the effects of driving pressure on hemodynamics.
Based on the results of sensitivity analysis. We carefully analyzed the differences between the two studies (1, 16) and the other studies and concluded that the high heterogeneity may be due to the following: (1) the intraoperative blood gas analyses were conducted at different time points; (2) the number of patients included in the study by Park et al. was larger than that in the other studies. Although they were all patients with OLV, there may have been some differences in the baseline characteristics of the patients from other studies; (3) a protective ventilation strategy was adopted for the control group in Park et al.’s study (VT: 6–8 mL/kg IBW, PEEP = 5 cmH2O, combined with a certain amount of lung recruitment maneuvers), while in the other included studies, the control group basically adopted conventional ventilation strategies and protective ventilation strategies were not exclusively used; and 4) Zhang et al.’s study was designed to titrate PEEP to the best CRS and was not strictly driving pressure-oriented because the driving pressure of the experimental group was statistically significantly lower than that of the control group (P < 0.01). We thus regarded their experimental group as the driving pressure-oriented group. This difference in the ventilation mode may also help explain this heterogeneity.
Our study has some limitations. First, Our study included only seven RCTs with a total of 640 patients. It is difficult to draw Critical clinically significant conclusions from the small sample size. Second, not all of the trials included in the meta-analysis strictly titrated intraoperative ventilation parameters to the lowest driving pressure; rather, the driving pressure in the experimental group was statistically significantly lower than that in the control group. These experiments also used different ventilation settings, which may have had an impact on the results and degree of heterogeneity. Third, We initially decided to include RCTs and cohort studies on the PROSPERO protocol. However, the final search showed no relevant cohort studies, so we finally decided to include only RCTs. Furthermore, owing to the limited number of trials that reported the PPCS and met our inclusion criteria, we could only assess the patients’ intraoperative PaO2/FiO2 ratio as the primary outcome. So there were several deviations from our original PROSPERO protocol (title, outcomes, and inclusion criteria). Moreover, the intraoperative blood gas analysis was performed at different times for each experiment. Fourth, since only two studies recorded intraoperative shunt fraction, we were not able to assess shunt fraction even though this may have reflected intraoperative oxygenation better than the CRS. Finally, PPCS included postoperative lung injury, atelectasis, pulmonary infection, and barotrauma, among others, but only two studies provided subgroup data, so we did not perform a subgroup analysis of the PPCS. There are some more critical clinical outcomes - the ICU length of stay, hospital length of stay, and mortality. However, only one of our included studies recorded hospital length of stay (16), so we were unable to assess these more valuable clinical outcomes. For the continuous variables, since we considered that the results would be different depending on the time point, we performed a subgroup analysis. However, only three studies clearly recorded the monitoring time points of the continuous variables, which was insufficient. Therefore, the aggregate results of the subgroup analysis should be interpreted with caution.
In patients undergoing thoracic surgery, driving pressure-oriented ventilation during OLV improves intraoperative oxygenation, reduces the incidence of PPCS, and improves CRS. However, more RCTs are necessary to confirm these findings.
The original contributions presented in the study are included in the article/Supplementary Material, further inquiries can be directed to the corresponding author/s.
X L, Y F, and J H designed and conceived the study, performed the statistical analysis, and drafted the manuscript. X L and Y F performed the systematic review, study selection, statistical analysis, and preparation of the article for publication. X L and W X contributed to data extraction and quality assessment. All authors contributed to the article and approved the submitted version.
This study was supported by Yunnan Provincial Science and Technology Department project (202101AY070001-133).
The Supplementary Material for this article can be found online at: https://www.frontiersin.org/articles/10.3389/fsurg.2022.914984/full#supplementary-material.
The authors declare that the research was conducted in the absence of any commercial or financial relationships that could be construed as a potential conflict of interest.
All claims expressed in this article are solely those of the authors and do not necessarily represent those of their affiliated organizations, or those of the publisher, the editors and the reviewers. Any product that may be evaluated in this article, or claim that may be made by its manufacturer, is not guaranteed or endorsed by the publisher.
1. Park M, Ahn HJ, Kim JA, Yang M, Heo BY, Choi JW, et al. Driving pressure during thoracic surgery: a randomized clinical trial. Anesthesiology. (2019) 130(3):385–93. doi: 10.1097/ALN.0000000000002600
2. Yoon S, Kim BR, Min SH, Lee J, Bahk JH, Seo JH. Repeated intermittent hypoxic stimuli to operative lung reduce hypoxemia during subsequent one-lung ventilation for thoracoscopic surgery: a randomized controlled trial. PLoS One. (2021) 16(4):e0249880. doi: 10.1371/journal.pone.0249880
3. Wang J, Fan SM, Zhang J. Epigallocatechin-3-gallate ameliorates lipopolysaccharide-induced acute lung injury by suppression of TLR4/NF-κB signaling activation. Braz J Med Biol Res. (2019) 52(7):e8092. doi: 10.1590/1414-431X20198092
4. Pardos PC, Garutti I, Piñeiro P, Olmedilla L, de la Gala F. Effects of ventilatory mode during one-lung ventilation on intraoperative and postoperative arterial oxygenation in thoracic surgery. J Cardiothorac Vasc Anesth. (2009) 23(6):770–4. doi: 10.1053/j.jvca.2009.06.002
5. Marret E, Cinotti R, Berard L, Piriou V, Jobard J, Barrucand B, et al. Protective ventilation during anaesthesia reduces major postoperative complications after lung cancer surgery: a double-blind randomised controlled trial. Eur J Anaesthesiol. (2018) 35(10):727–35. doi: 10.1097/EJA.0000000000000804
6. Yang M, Ahn HJ, Kim K, Kim JA, Yi CA, Kim MJ, et al. Does a protective ventilation strategy reduce the risk of pulmonary complications after lung cancer surgery?: a randomized controlled trial. Chest. (2011) 139(3):530–7. doi: 10.1378/chest.09-2293
7. Amato MB, Meade MO, Slutsky AS, Brochard L, Costa EL, Schoenfeld DA, et al. Driving pressure and survival in the acute respiratory distress syndrome. N Engl J Med. (2015) 372(8):747–55. doi: 10.1056/NEJMsa1410639
8. Terragni PP, Rosboch G, Tealdi A, Corno E, Menaldo E, Davini O, et al. Tidal hyperinflation during low tidal volume ventilation in acute respiratory distress syndrome. Am J Respir Crit Care Med. (2007) 175(2):160–6. doi: 10.1164/rccm.200607-915OC
9. Gattinoni L, Pesenti A. The concept of “baby lung”. Intensive Care Med. (2005) 31(6):776–84. doi: 10.1007/s00134-005-2627-z
10. Bellani G, Grassi A, Sosio S, Gatti S, Kavanagh BP, Pesenti A, et al. Driving pressure is associated with outcome during assisted ventilation in acute respiratory distress syndrome. Anesthesiology. (2019) 131(3):594–604. doi: 10.1097/ALN.0000000000002846
11. Neto AS, Hemmes SN, Barbas CS, Beiderlinden M, Fernandez-Bustamante A, Futier E, et al. Association between driving pressure and development of postoperative pulmonary complications in patients undergoing mechanical ventilation for general anaesthesia: a meta-analysis of individual patient data. Lancet Respir Med. (2016) 4(4):272–80. doi: 10.1016/S2213-2600(16)00057-6
12. Page MJ, McKenzie JE, Bossuyt PM, Boutron I, Hoffmann TC, Mulrow CD, et al. The PRISMA 2020 statement: an updated guideline for reporting systematic reviews. BMJ. (2021) 372:n71. doi: 10.1136/bmj.n71
13. Agostini P, Cieslik H, Rathinam S, Bishay E, Kalkat MS, Rajesh PB, et al. Postoperative pulmonary complications following thoracic surgery: are there any modifiable risk factors? Thorax. (2010) 65(9):815–8. doi: 10.1136/thx.2009.123083
14. McGrath S, Zhao X, Steele R, Thombs BD, Benedetti A, Depression Screening Data (DEPRESSD) Collaboration. Estimating the sample mean and standard deviation from commonly reported quantiles in meta-analysis. Stat Methods Med Res. (2020): 29(9):2520–37. doi: 10.1177/0962280219889080
15. Liu K, Huang C, Xu M, Wu J, Frerichs I, Moeller K, et al. PEEP guided by electrical impedance tomography during one-lung ventilation in elderly patients undergoing thoracoscopic surgery. Ann Transl Med. (2019) 7(23):757. doi: 10.21037/atm.2019.11.95
16. Zhang Y, Zhang M, Wang X, Shang G, Dong Y. Individualized positive end-expiratory pressure in patients undergoing thoracoscopic lobectomy: a randomized controlled trial. Braz J Anesthesiol. (2021) 71(5):565–71. doi: 10.1016/j.bjane.2021.04.001
17. Spadaro S, Grasso S, Karbing DS, Fogagnolo A, Contoli M, Bollini G, et al. Physiologic evaluation of ventilation perfusion mismatch and respiratory mechanics at different positive end-expiratory pressure in patients undergoing protective one-lung ventilation. Anesthesiology. (2018) 128(3):531–8. doi: 10.1097/ALN.0000000000002011
18. Rauseo M, Mirabella L, Grasso S, Cotoia A, Spadaro S, D’Antini D, et al. Peep titration based on the open lung approach during one lung ventilation in thoracic surgery: a physiological study. BMC Anesthesiol. (2018) 18(1):156. doi: 10.1186/s12871-018-0624-3
19. Spadaro S, Grasso S, Karbing DS, Santoro G, Cavallesco G, Maniscalco P, et al. Physiological effects of two driving pressure-based methods to set positive end-expiratory pressure during one lung ventilation. J Clin Monit Comput. (2021) 35(5):1149–57. doi: 10.1007/s10877-020-00582-z
20. Xu D, Wei W, Chen L, Li S, Lian M. Effects of different positive end-expiratory pressure titrating strategies on oxygenation and respiratory mechanics during one- lung ventilation: a randomized controlled trial. Ann Palliat Med. (2021) 10(2):1133–44. doi: 10.21037/apm-19-441
21. Liu Z, Liu X, Huang Y, Zhao J. Intraoperative mechanical ventilation strategies in patients undergoing one-lung ventilation: a meta-analysis. Springerplus. (2016) 5(1):1251. doi: 10.1186/s40064-016-2867-0
22. Okahara S, Shimizu K, Suzuki S, Ishii K, Morimatsu H. Associations between intraoperative ventilator settings during one-lung ventilation and postoperative pulmonary complications: a prospective observational study. BMC Anesthesiol. (2018) 18(1):13. doi: 10.1186/s12871-018-0476-x
23. Lundquist H, Hedenstierna G, Strandberg A, Tokics L, Brismar B. CT-assessment of dependent lung densities in man during general anaesthesia. Acta Radiol. (1995) 36(6):626–32. doi: 10.1186/s12871-018-0476-x
24. Liu J, Meng Z, Lv R, Zhang Y, Wang G, Xie J. Effect of intraoperative lung-protective mechanical ventilation on pulmonary oxygenation function and postoperative pulmonary complications after laparoscopic radical gastrectomy. Braz J Med Biol Res. (2019) 52(6):e8523. doi: 10.1590/1414-431(20198523
25. Tao T, Bo L, Chen F, Xie Q, Zou Y, Hu B, et al. Effect of protective ventilation on postoperative pulmonary complications in patients undergoing general anaesthesia: a meta-analysis of randomised controlled trials. BMJ Open. (2014) 4(6):e005208. doi: 10.1136/bmjopen-2014-005208
26. Colquhoun DA, Leis AM, Shanks AM, Mathis MR, Naik BI, Durieux ME, et al. A lower tidal volume regimen during one-lung ventilation for lung resection surgery is not associated with reduced postoperative pulmonary complications. Anesthesiology. (2021) 134(4):562–76. doi: 10.1097/ALN.0000000000003729
27. Karalapillai D, Weinberg L, Peyton P, Ellard L, Hu R, Pearce B, et al. Effect of intraoperative low tidal volume vs conventional tidal volume on postoperative pulmonary complications in patients undergoing major surgery: a randomized clinical trial. JAMA. (2020) 324(9):848–58. doi: 10.1001/jama.2020.12866
28. Blank RS, Colquhoun DA, Durieux ME, Kozower BD, McMurry TL, Bender SP, et al. Management of one-lung ventilation: impact of tidal volume on complications after thoracic surgery. Anesthesiology. (2016) 124(6):1286–95. doi: 10.1097/ALN.0000000000001100
29. Writing Group for the PReVENT Investigators, Simonis FD, Serpa Neto A, Binnekade JM, Braber A, Bruin KCM, et al. Effect of a low vs intermediate tidal volume strategy on ventilator-free days in intensive care unit patients without ARDS: a randomized clinical trial. JAMA. (2018) 320(18):1872–80. doi: 10.1001/jama.2018.14280
30. Li P, Kang X, Miao M, Zhang J. Individualized positive end-expiratory pressure (PEEP) during one-lung ventilation for prevention of postoperative pulmonary complications in patients undergoing thoracic surgery: a meta-analysis. Medicine (Baltimore). (2021) 100(28):e26638. doi: 10.1097/MD.0000000000026638
31. Rusca M, Proietti S, Schnyder P, Frascarolo P, Hedenstierna G, Spahn DR, et al. Prevention of atelectasis formation during induction of general anesthesia. Anesth Analg. (2003) 97(6):1835–9. doi: 10.1213/01.ANE.0000087042.02266.F6
32. Beitler JR, Malhotra A, Thompson BT. Ventilator-induced lung injury. Clin Chest Med. (2016) 37(4):633–46. doi: 10.1016/j.ccm.2016.07.004
33. de la Gala F, Piñeiro P, Garutti I, Reyes A, Olmedilla L, Cruz P, et al. Systemic and alveolar inflammatory response in the dependent and nondependent lung in patients undergoing lung resection surgery: a prospective observational study. Eur J Anaesthesiol. (2015) 32(12):872–80. doi: 10.1097/EJA.0000000000000233
Keywords: driving pressure, lung-protective ventilation, one-lung ventilation (OLV), oxygenation, postoperative pulmonary complications (PPCs)
Citation: Li X, Xue W, Zhang Q, Zhu Y, Fang Y and Huang J (2022) Effect of Driving Pressure-Oriented Ventilation on Patients Undergoing One-Lung Ventilation During Thoracic Surgery: A Systematic Review and Meta-Analysis. Front. Surg. 9:914984. doi: 10.3389/fsurg.2022.914984
Received: 7 April 2022; Accepted: 12 May 2022;
Published: 27 May 2022.
Edited by:
Davide Tosi, IRCCS Ca 'Granda Foundation Maggiore Policlinico Hospital, ItalyReviewed by:
Ryan Ruiyang Ling, Yong Loo Lin School of Medicine, National University of Singapore, SingaporeCopyright © 2022 Li, Xue, Zhang, Zhu, Fang and Huang. This is an open-access article distributed under the terms of the Creative Commons Attribution License (CC BY). The use, distribution or reproduction in other forums is permitted, provided the original author(s) and the copyright owner(s) are credited and that the original publication in this journal is cited, in accordance with accepted academic practice. No use, distribution or reproduction is permitted which does not comply with these terms.
*Correspondence: Yu Fang ZmFuZ3l1QHlkeXkuY24= Jie Huang eWR5eWhqQDE2My5jb20=
†These authors have contributed equally to this work
Specialty section: This article was submitted to Thoracic Surgery, a section of the journal Frontiers in Surgery
Disclaimer: All claims expressed in this article are solely those of the authors and do not necessarily represent those of their affiliated organizations, or those of the publisher, the editors and the reviewers. Any product that may be evaluated in this article or claim that may be made by its manufacturer is not guaranteed or endorsed by the publisher.
Research integrity at Frontiers
Learn more about the work of our research integrity team to safeguard the quality of each article we publish.