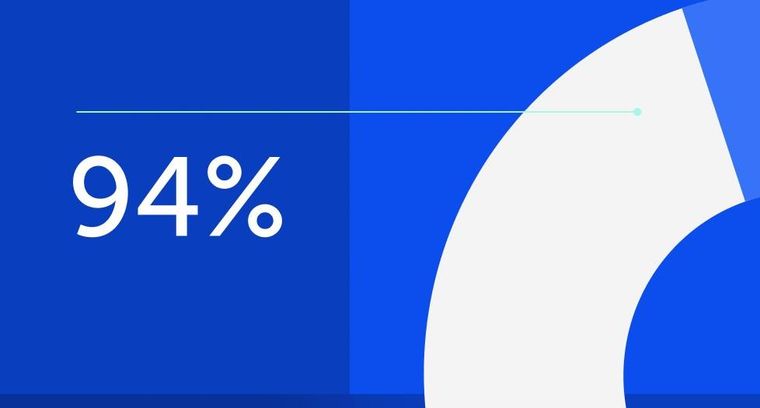
94% of researchers rate our articles as excellent or good
Learn more about the work of our research integrity team to safeguard the quality of each article we publish.
Find out more
SYSTEMATIC REVIEW article
Front. Surg., 16 August 2022
Sec. Orthopedic Surgery
Volume 9 - 2022 | https://doi.org/10.3389/fsurg.2022.911471
This article is part of the Research TopicDigitalisation and AI in Orthopedic Surgery and Rehabilitation 4.0View all 7 articles
Objective: The purpose of this study was to explore the safe and most effective initiation time for the functional recovery of patients with peri-ankle fractures after surgery.
Method: We searched electronic databases, including the Cochrane Library, Embase, PubMed and the reference lists of relevant articles published from inception to October 30, 2021. Two researchers independently performed literature screening and data extraction and evaluated the quality of the included literature using the Newcastle–Ottawa Scale. Network meta-analysis, including consistency testing, publication bias, and graphical plotting, was performed using Stata (v16.0).
Results: A total of 25 articles involving 1756 patients were included in this study. The results of the meta-analysis showed that functional exercise within 2 days after surgery may result in lower VAS scores compared to other techniques (P < 0.05). Functional exercise within 12 months may lead to higher AOFAS scores than that of other techniques (P < 0.05). The total postoperative complication rate, including deep vein thrombosis, showed no statistically significant differences between any two interventions (P > 0.05). The results of the surface under the cumulative ranking (SUCRA) showed that functional exercise within two days postoperatively may have the lowest VAS scores (SUCRA = 82.8%), functional exercise within 1 week postoperatively may have the lowest deep vein thrombosis rate (SUCRA = 66.8%), functional exercise within 10 days postoperatively may have the fewest total postoperative complication rate (SUCRA = 73.3%) and functional exercise within 12 months postoperatively may contribute to the highest AOFAS scores (SUCRA = 85.5%).
Conclusion: The results of this study suggest that initiation of rehabilitation within two days after surgery may be the best time to reduce postoperative pain; rehabilitation interventions within 10 days after surgery may be the optimal time for reducing the total postoperative complication rate, including deep vein thrombosis; and continued functional exercise within 12 months after surgery may steadily and ideally improve the function of the ankle joint.
Systematic Review Registration: doi: 10.37766/inplasy2021.12.0030, identifier: INPLASY2021120030
Peri-ankle fractures are the third most serious musculoskeletal fracture, with a mortality rate as high as 12% during the first year (1). In 2011, 174 cases per 100,000 persons were reported in Germany, accounting for approximately 60% of women aged over 50 years (2). It is predicted that caries of peri-ankle fractures will increase 3-fold by 2030 with the aging population and increasing participation in physical activities (3). The main cause of peri-ankle fracture is sprains and falls, followed by sports injuries. Furthermore, osteoporosis is an also an important cause in elderly individuals (4). The presence of osteoporosis, multiple serious comorbidities and functional independence make treatment a challenge.
According to AO classification, displaced or/and dislocated unstable peri-ankle fractures are recommended to be treated with open reduction and internal fixation (ORIF) to achieve anatomical reconstruction (5). Regardless of whether the patient has conservative or operative treatment, a period of immobilization is essential (6). Ankle joint immobilization is carried out to protect surgical wounds, reduce pain after anesthesia, and minimize mechanical stress (7). The potential contribution of immobilization includes decreased muscle volume and loss of strength, reduced range of mobility, and the consequential influence of function (8). Many patients had good surgical reduction but failed to recover with normal ankle joint function due to the lack of effective postoperative functional exercise.
With the development of multidisciplinary team (MDT)-driven peri-operative care and rehabilitation, many doctors advocate early ankle exercises and weightbearing after surgery to accelerate functional rehabilitation (9). However, the initiation time of postoperative functional exercises and ankle range of motion remain unclear and controversial, which hinders their widespread implementation in the clinic.
An earlier systematic review compared the results of early ankle range of motion and weight-bearing with delayed exercises and concluded that early weightbearing postoperative regimens can improve the stiffness and range of movement of the ankle joint (10). However, there are no systematic reviews on the effects of different postoperative rehabilitation times or direct comparisons of different starting times. Network meta-analysis (NMA) allows for the indirect comparison of different interventions and the selection of the best timing (11). Herein, the purpose of this study was to explore the safe and most effective initiation time for functional recovery of patients with peri-ankle fractures using the NMA method. The study provides valuable information for future treatment and rehabilitation intervention of peri-ankle fractures.
This NMA strictly complied with the preferred reporting items for systematic review and meta-analysis (PRISMA) guidelines and Meta-Analyses (PRISMA) guidelines for NMA (12, 13). We have registered this systematic review with INPLASY (CRD2021120030) prospectively and report the results of an included prespecified outcome analysis.
The inclusion criteria were as follows: (1) randomized control trials (RCTs); (2) studies about peri-ankle fractures (fractures or dislocations which affected the ankle mortise and ankle joint stability); (3) the interventions were different functional exercise initiation times for the patients after surgery; and (4) extractable data reporting the total postoperative complication rate, incidence of deep vein thrombosis (DVT), AOFAS scores, and VAS scores of patients.
The exclusion criteria were as follows: (1) duplicate publications, original texts not found; (2) review categories, empirical summaries, case reports, conferences, and meta-analyses; (3) interventions not including the functional exercise initiation time and (4) contraindication for early weightbearing (eg, unstable osteosynthesis, open fracture, pilon fracture other significant comorbidities preventing early mobilization).
The PubMed, Cochrane Library, EMBASE and the reference lists of relevant published articles were systematically searched from inception to October 2021. The search strategies were “ankle” OR “distal tibia” OR “distal fibula” OR “talus” OR “medial malleolus” OR “lateral malleolus” OR “posterior malleolus” OR “unicondylar” OR “bimalleolar” OR “trimalleolar” AND “fracture” AND “rehabilitation” OR “training” OR “exercise” OR “weight bearing” AND “randomized” OR “RCT”.
The retrieved literature was imported into the literature management software. To screen the studies, two researchers independently performed the extraction of relevant information, and then the full text of the included studies was analyzed quantitatively according to the inclusion and exclusion criteria. Disagreements between the two researchers were resolved through discussion and negotiation by a third researcher. In addition, the investigators extracted data according to predesigned tables, including the study characteristics (author, year, country), patient characteristics (sample size, male/female, rehabilitation modality, type of fracture, time from injury to operation, intervention initiation time) and outcomes (AOFAS score, VAS score, total postoperative complication rate, DVT incidence, follow-up time).
The Cochrane Risk of Bias Assessment Tool was used to assess the quality of the included randomized controlled trials (14), which evaluates the risk of bias in six main areas (random sequence generation, allocation concealment, participant and outcome blinding, incomplete follow-up, selective reporting, and other biases), and the risk of bias distribution was plotted using Revman version 5.3 software.
NMA was performed using Stata (v16.0) software. Comparisons between the different starting times were represented by network plots, with lines between the points indicating direct comparisons and the thickness of the lines indicating the amount of comparison between the two intervention times (15). Local inconsistency tests for direct and indirect comparisons were performed using nodal splits, and P < 0.05 was considered to be a local inconsistency. Relative risk (RR) was used for count data, and weighted mean difference (WMD) or standardized standard deviation (SMD) and its 95% confidence interval (CI) were used for measurement data. Intervention efficacy was estimated as the probability of ranking by surface under the cumulative ranking (SUCRA). Publication bias and small sample effects among the included studies were assessed by funnel plots (16). The risk of bias of the included studies was analyzed using Revman, where green, yellow and red in the images represent low risk of bias, unclear and high risk of bias, respectively.
The detailed article searching and study selection process is listed in Figure 1. After systematic search, a total of 1,607 articles were obtained, and 930 articles were obtained after deweighting by Endnote X9 software. Seventy-five articles were obtained after reading the titles and abstracts and excluding irrelevant studies, noncontrolled experimental studies, conferences, abstracts, meta-analyses, etc. For the remaining articles, three articles with noncompliant outcome indicators, two articles with incomplete data reporting and seven articles were excluded due being considered low-quality articles after reading the full text, and 25 articles with a total of 1,756 patients were finally included. Table 1 lists the basic information of all the included studies.
Figure 1. Flow chart of the literature screening process RCT, randomized controlled trial; E, experimental group; C, control group; NA, Not available; lauge-hansen Se, lauge-hansen supination external rotation type; lauge-hansen Pe, lauge-hansen pronation- eversion; RT, rehabilitation training; WB, weight bearing AOFAS scores②VAS scores③total postoperative complication rates④DVT rates.
In Figure 2, the quality of the 25 articles was good overall, with red indicating high risk, yellow indicating unclear risk, and green indicating low risk in the bias distribution graph. Twenty-five studies were adequately randomized, 6 reported allocation concealment, and five reported blinding of outcome assessment. Thirteen studies did not report blinding of the participants and personnel. All other risks of bias were low.
The network evidence plots involving 14 intervention times in this NMA, with respect to the AOFAS scores, VAS scores, total postoperative complication rate, and DVT rates, are shown in Figure 3A–D. Lines between two connected points indicate direct comparisons, and unconnected points indicate indirect comparisons via NMA. The width of the line represents the number of data sets from the included studies, while the size of the nodes shows the total sample size from A to N.
Figure 3. Reticulated evidence diagram of different rehabilitation intervention times after the operation. A: 1 day postoperatively, B: 2 days postoperatively, C: 1 week postoperatively, D: 8 days postoperatively, E: 10 days postoperatively, F: 2 weeks postoperatively, G: 3 weeks postoperatively, H: 4 weeks postoperatively, I: 6 weeks postoperatively, J: 9 weeks postoperatively, K: 12 weeks postoperatively, L: 6 months postoperatively, M: 12 months postoperatively, N: 24 months postoperatively. (A) Network evidence for AOFAS scores; (B) Network evidence for VAS scores; (C) Network evidence for total postoperative complication rates; (D) Network evidence for DVT rates.
There were 14 studies reporting AOFAS scores with intervention times including A, B, F, I, J, K, L, M and N. There was a closed ring between the interventions. There were direct and indirect comparisons between the interventions. The consistency test results showed P > 0.05. Therefore, the statistical analysis could be performed directly under the consistency model. The results of the NMA showed that the AOFAS scores were lower in B than in L, M and N, lower in F than in K, L, M and N, and lower in I than in L and M. The differences were statistically significant (P < 0.05); the remaining comparisons between the two intervention times were not statistically significant (P < 0.05) (Table 2).
There were 10 studies reporting the VAS scores for interventions including A, B, F, G, I, K, L, M and N. The results of the reticulated meta-analysis showed that the VAS scores of B were lower than those of F, G, I, K, L, M and N. The differences were all statistically significant (P < 0.05) (Table 3).
Nineteen studies reported the total postoperative complication rate of the interventions including A, B, C, D, E, F, G, H and I. There was a closed ring between the interventions. There were direct and indirect comparisons between the interventions, and the results of the consistency test showed (P > 0.05). Therefore, statistical analysis could be performed directly under the consistency model. The results of NMA showed that there were no statistically significant comparisons between any two interventions (P > 0.05) (Table 4).
Eight studies reported the incidence of DVT and interventions including A, B, C, D, E, F, G, H and I. The results of NMA showed that there were no statistically significant comparisons between any two interventions (P > 0.05) (Table 5).
A total of 14 interventions were included in this study. The AOFAS score, VAS score, total postoperative complication rate, and DVT rate were ranked under the 14 interventions. The results of the AOFAS score probability ranking showed that M (85.5%) > N (79.6%) > L (75.4%) > (58.6%) > J (57.1%) > A (48.2%) > F (17.7%) > I (16.5%) > B (11.5%), suggesting that M may be the intervention time that resulted in the highest AOFAS score in patients. The results of the VAS score probability ranking showed that B (82.8%) > N (75.3%) > M (66.8%) > L (55.8%) > I (49.3%) > K (36.4%) > G (35.8%) > F (30.6%) > A (17.3%), suggesting that B may be the intervention time that results in the lowest VAS score in patients. The results of the postoperative complication probability ranking showed that E (73.3%) > D (68.8%) > C (67.9%) > F (66%) > H (53.7%) > A (42.4%) > I (35.8%) > B (31.6%) > G (10.5%), suggesting that E may be the intervention time that allows patients to have the lowest total postoperative complication rate. The results of the probability ranking of the incidence of DVT showed that C (66.8%) > E (66%) > A (63.3%) > B (47.6%) > F (40.3%) > H (39%) > G (27.1%), suggesting that C may be the intervention time that resulted in the lowest incidence of DVT in patients.
The node-splitting method of inconsistency of our results and its Bayesian p value showed good agreement with the confidence intervals crossed with the blank values in all loops (P > 0.05), and there were no significant differences between the direct and indirect effects in the network meta-analysis.
Funnel plots were constructed to analyze publication bias in the included studies (17). However, the scattered distribution of points with incomplete symmetry suggests that there may be some publication bias, and the scattered distribution at the bottom of the funnel plot for each study indicator suggests a small sample effect (Figure 4A–D). Therefore, these results should be interpreted with caution.
Figure 4. The funnel plot of outcome measures. (A) Funnel plot for the AOFAS scores; (B) Funnel plot for the VAS scores; (C) Funnel plot of the total postoperative complication rates; (D) Funnel plot of the DVT rates.
The results of this NMA demonstrate our hypothesis that early rehabilitative exercise in the postoperative period was superior to delayed interventions. Rehabilitative exercise, which begins at postoperative day two, is superior for decreasing the VAS score; rehabilitative exercise that begins within 1 week postoperatively may be optimal for reducing the incidence of postoperative complications when it is initiated within 10 days, in order to avoid DVT; and staying in functional rehabilitation exercises for 12 months after surgery may be beneficial for obtaining the best AOFAS score. Overall, based on our confidence interval and ranking results, we conclude that the initiation of rehabilitation on postoperative day 2 may be the best way to improve postoperative pain; that rehabilitation interventions within 10 days after surgery may be optimal for reducing the total incidence of postoperative complications, including DVT; and that continued functional exercise for 12 months after surgery may steadily improve the function of the ankle joint.
Although there is consensus on early rehabilitation with brace protection, the decisions about which postoperative phase (e.g., immediate postoperative period, after wound healing, after scab formation, after fracture healing) to use rehabilitation interventions for patients with peri-ankle fractures vary from surgeon to surgeon. In a 2-part trial performed by Ahl (18, 19), comparing early range of motion (orthosis) and delayed weight bearing, early range of motion and early weight bearing, late range of motion (cast) and delayed weight bearing, or late range of motion and early weight bearing for ankle surgery a trend towards significantly improved functional limb outcomes was found in the early weight bearing, early range of motion group of patients, early motion group that reached statistical significance within 12 months. This study echoes our experimental results perfectly.
For peri-ankle fractures, postoperative functional exercises (including progressive weight bearing, clinical pathway exercises, emerging functional devices, and visual feedback balance exercises) the standard weight-bearing protocol includes 10 to 12 weeks of non–weight bearing followed by 4 to 6 weeks of progressive weight bearing whereby in the first week patients bear weight at 25% of their body weight and increase the amount of weight bearing by 25% each week until they are able to bear their full weight.
A portion of clinicians advocate primary postoperative braking and rehabilitation exercises after wound healing, and they are mainly concerned about the risk of postoperative wound infection. However, this standpoint quickly contradicted that the number of infections was similar in the immediate postoperative and delayed groups (20). Thomas (21) found that early ankle range of motion, with or without early weight bearing, improved range of motion and functional scores at 9 to 12 weeks after treatment. At 1 year, there was no difference between the groups. Despite similar long-term functional outcomes, early motion has been associated with a higher rate of superficial and deep wound complications. The wound complications (e.g., infections and delayed healing) are minimally associated with immediate ankle motion. In most cases, however, patients are fearful of weight-bearing immediately after surgical fixation. Patients' psychological expectations of painful weight-bearing starting within 1 week after surgery are more difficult to manage than in the 4th week after surgery (22), and the bedside physician's education and rehabilitation instructions are particularly important at this time. A biofeedback training study may solve the question of whether 24 h of training complied with touch-down weight-bearing instructions is an effective way to train patients and has wide potential clinical applicability (23).
There is also some concern that weight bearing and activity without waiting for the primary bone callus to form may cause the fracture to displace (24). Laarhoven et al. scholars (25, 26) reported a randomised trial of patients with fractures of the ankle of AO types A, B and C and compared two regimes of postoperative management after internal fixation. They found through long-term follow-up that immediate postoperative weight bearing has not been shown to result in loss of reduction, hardware failure, or revision surgery for osteolysis. Conversely, maintaining some micromovement of the fracture fragment with firm internal fixation may facilitate the healing process (27). Controlled axial loading of the fracture site usually results in a larger volume of healing tissue and a faster healing time compared to no loading or excessive early loading. It has also been suggested that the syndesmotic screws should be removed prior to rehabilitation, because the effect of the syndesmotic screws on ankle mobility may affect the outcome of early rehabilitation training (28). However, there is evidence that removal of the screws does not enhance the ankle range of motion, so whether the screws are removed is also not a criterion for postoperative weight bearing (29). In addition, none of the studies on peri-fractures after fixation allowed unrestricted weight-bearing, generally allowing 20–25 kg of protected weight-bearing. Gul et al. (25) reported on patients with operated Weber A/B/C fractures who were weight-bearing immediately after surgical stabilisation without protective immobilisation treatment for ankle fractures. Their outcomes were better compared to controls who received postoperative plaster immobilisation and non-weight bearing treatment. Patients with early weight-bearing group were found to have significantly less time in hospital, return to work. Under the circumstance of poor fixation or associated with osteoporosis, weight-bearing or exercise needs to be delayed (30). Therefore, the weight-bearing status should be determined by the patient's clinical fracture stability rather than adhering to convention.
Postoperative weight-bearing not only stimulates fracture healing but also nonweight-bearing can be detrimental (31). The consequences of 10 days of bed rest in healthy adults can result in a significant decrease in lower extremity strength, explosive power and aerobic capacity, and reduced physical activity (32). Older adults who undergo postoperative braking are at increased risk for DVT and pulmonary embolism (PE), pneumonia, cardiovascular disease, stressors, and infections (33). According to our findings, these complications (e.g., DVT, PE, etc.) can be minimized by limiting protected functional exercise for 10 days postoperatively.
Although the ultimate goal of surgery is to achieve good joint function, the end of surgery does not mean the end of treatment. Therefore, early postoperative functional exercise should be promoted to all patients with peri-ankle fractures (especially elderly patients) to reduce the restriction on their daily activities, thus shortening the length of hospital stay and returning to independent living as soon as possible (34). Peri-ankle fracture could cause truncal movement asymmetry in the vertical direction accompanied by slower walking cadence and smaller step lengths (35). No studies have been conducted on proprioceptive deficits after peri-ankle fractures and their effect on the rehabilitation process. From our point of view, functional training should be continued for 12 months after surgery to restore proprioception and improve ankle function steadily. Therefore, surgeons and patients should pay attention to the continuity of postoperative rehabilitation exercise.
To our knowledge, this is the first study to explore this aspect using an NMA. However, some limitations that need to be considered may affect the conclusions drawn from this study, such as selection bias due to language limitations, differences in intervention duration, and inadequate reporting of adverse event data. Nevertheless, we believe that the results of this study will help identify the optimal time point for postoperative rehabilitation interventions for peri-ankle fractures and provide further recommendations or guidance for clinical practice.
We conclude that initiation of rehabilitation on postoperative day 2 may be the best way to improve postoperative pain; that rehabilitation interventions within 10 days after surgery may be optimal for reducing the incidence of postoperative complications, including DVT; and that continued functional exercise for 12 months after surgery may steadily improve ankle joint function. Based on our results, we emphasize the importance of postoperative rehabilitation exercise and suggest the following: first, the appropriate rehabilitation time and protocol should be selected according to the patient's own condition in clinical practice; second, future researchers should make direct comparisons between different rehabilitation times (e.g., within 2 days, 7 days, 7 to 10 days, or other times after surgery); and third, the long-term effects of different start times, such as follow-up surveys at 3, 6, and 12 months after leaving the hospital, should be studied.
The original contributions presented in the study are included in the article/Suplementary Material, further inquiries can be directed to the corresponding author/s.
KZ: study design, data collections, and writing. SD: data collections, data analysis, and writing. WW: study design and review. All authors contributed to the article and approved the submitted version. KZ and SD have contributed equally to this work.
The authors declare that the research was conducted in the absence of any commercial or financial relationships that could be construed as a potential conflict of interest.
All claims expressed in this article are solely those of the authors and do not necessarily represent those of their affiliated organizations, or those of the publisher, the editors and the reviewers. Any product that may be evaluated in this article, or claim that may be made by its manufacturer, is not guaranteed or endorsed by the publisher.
1. Pearce O, Al-Hourani K, Kelly M. Ankle fractures in the elderly: current concepts. Injury. (2020) 51:2740–7. doi: 10.1016/j.injury.2020.10.093.33153712
2. Goost H, Wimmer MD, Barg A, Kabir K, Valderrabano V, Burger C. Fractures of the ankle joint: investigation and treatment options. Dtsch Arztebl Int. (2014) 111:377–88. doi: 10.3238/arztebl.2014.0377.24939377
3. Kannus P, Palvanen M, Niemi S, Parkkari J, Järvinen M. Stabilizing incidence of low-trauma ankle fractures in elderly people Finnish statistics in 1970–2006 and prediction for the future. Bone. (2008) 43:340–2. doi: 10.1016/j.bone.2008.04.015.18538645
4. Lee KM, Chung CY, Kwon SS, Won SH, Lee SY, Chung MK, et al. Ankle fractures have features of an osteoporotic fracture. Osteoporos Int. (2013) 24:2819–25. doi: 10.1007/s00198-013-2394-6.23681087
5. Lee S, Lin J, Hamid KS, Bohl DD. Deltoid ligament rupture in ankle fracture: diagnosis and management. J Am Acad Orthop Surg. (2019) 27:e648–58. doi: 10.5435/JAAOS-D-18-00198.30475279
6. Backx EMP, Hangelbroek R, Snijders T, Verscheijden M-L, Verdijk LB, de Groot LCPGM, et al. Creatine loading does not preserve muscle mass or strength during leg immobilization in healthy, young males: a randomized controlled trial. Sports Med. (2017) 47:1661–71. doi: 10.1007/s40279-016-0670-2.28054322
7. Early ankle movement versus immobilization in the postoperative management of ankle fracture in adults: a systematic review and meta-analysis – PubMed. https://pubmed.ncbi.nlm.nih.gov/25098197/ (Accessed March 10, 2022).
8. Psatha M, Wu Z, Gammie FM, Ratkevicius A, Wackerhage H, Lee JH, et al. A longitudinal MRI study of muscle atrophy during lower leg immobilization following ankle fracture. J Magn Reson Imaging. (2012) 35:686–95. doi: 10.1002/jmri.22864.22045592
9. Latham NK, Harris BA, Bean JF, Heeren T, Goodyear C, Zawacki S, et al. Effect of a home-based exercise program on functional recovery following rehabilitation after hip fracture: a randomized clinical trial. JAMA. (2014) 311:700–8. doi: 10.1001/jama.2014.469.24549550
10. Lin C-WC, Donkers NAJ, Refshauge KM, Beckenkamp PR, Khera K, Moseley AM. Rehabilitation for ankle fractures in adults. Cochrane Database Syst Rev. (2012) 11:CD005595. doi: 10.1002/14651858.CD005595.pub3.23152232
11. Rouse B, Chaimani A, Li T. Network meta-analysis: an introduction for clinicians. Intern Emerg Med. (2017) 12:103–11. doi: 10.1007/s11739-016-1583-7.27913917
12. Cornell JE. The PRISMA extension for network meta-analysis: bringing clarity and guidance to the reporting of systematic reviews incorporating network meta-analyses. Ann Intern Med. (2015) 162:797–8. doi: 10.7326/M15-0930.26030637
13. Hutton B, Salanti G, Caldwell DM, Chaimani A, Schmid CH, Cameron C, et al. The PRISMA extension statement for reporting of systematic reviews incorporating network meta-analyses of health care interventions: checklist and explanations. Ann Intern Med. (2015) 162:777–84. doi: 10.7326/M14-2385.26030634
14. Higgins JPT, Altman DG, Gøtzsche PC, Jüni P, Moher D, Oxman AD, et al. The cochrane collaboration’s tool for assessing risk of bias in randomised trials. Br Med J. (2011) 343:d5928. doi: 10.1136/bmj.d5928.
15. Lu G, Ades AE. Combination of direct and indirect evidence in mixed treatment comparisons. Statist Med. (2004) 23:3105–24. doi: 10.1002/sim.1875.
16. Salanti G, Ades AE, Ioannidis JPA. Graphical methods and numerical summaries for presenting results from multiple-treatment meta-analysis: an overview and tutorial. J Clin Epidemiol. (2011) 64:163–71. doi: 10.1016/j.jclinepi.2010.03.016.20688472
17. Chaimani A, Higgins JPT, Mavridis D, Spyridonos P, Salanti G. Graphical tools for network meta-analysis in STATA. PLoS One. (2013) 8:e76654. doi: 10.1371/journal.pone.0076654.24098547
18. Finsen V, Saetermo R, Kibsgaard L, Farran K, Engebretsen L, Bolz KD, et al. Early postoperative weight-bearing and muscle activity in patients who have a fracture of the ankle. J Bone Joint Surg Am. (1989) 71:23–7. doi: 10.2106/00004623-198971010-00005
19. Ahl T, Dalén N, Holmberg S, Selvik G. Early weight bearing of displaced ankle fractures. Acta Orthop Scand. (1987) 58:535–8. doi: 10.3109/17453678709146394.3425284
20. Farsetti P, Caterini R, Potenza V, De Luna V, De Maio F, Ippolito E. Immediate continuous passive motion after internal fixation of an ankle fracture. J Orthop Traumatol. (2009) 10:63–9. doi: 10.1007/s10195-009-0048-4.19484357
21. Thomas G, Whalley H, Modi C. Early mobilization of operatively fixed ankle fractures: a systematic review. Foot Ankle Int. (2009) 30:666–74. doi: 10.3113/FAI.2009.0666.19589314
22. Kokonyei G, Galambos A, Edes AE, Kocsel N, Szabo E, Pap D, et al. Anticipation and violated expectation of pain are influenced by trait rumination: an fMRI study. Cogn Affect Behav Neurosci. (2019) 19:56–72. doi: 10.3758/s13415-018-0644-y.30251186
23. Hustedt JW, Blizzard DJ, Baumgaertner MR, Leslie MP, Grauer JN. Lower-extremity weight-bearing compliance is maintained over time after biofeedback training. Orthopedics. (2012) 35:e1644–1648. doi: 10.3928/01477447-20121023-24.23127458
24. Doherty C, Bleakley C, Delahunt E, Holden S. Treatment and prevention of acute and recurrent ankle sprain: an overview of systematic reviews with meta-analysis. Br J Sports Med. (2017) 51:113–25. doi: 10.1136/bjsports-2016-096178.28053200
25. Gul A, Batra S, Mehmood S, Gillham N. Immediate unprotected weight-bearing of operatively treated ankle fractures. Acta Orthop Belg. (2007) 73:360–5.17715727
26. van Laarhoven CJ, Meeuwis JD, van der Werken C. Postoperative treatment of internally fixed ankle fractures: a prospective randomised study. J Bone Joint Surg Br. (1996) 78:395–9. doi: 10.1302/0301-620X.78B3.0780395
27. Wei M, Wei Y, Liu Y. Effects of early weightbearing on microfracture treatment of osteochondral lesions of talus with subchondral bone defects. CURR MED SCI. (2019) 39:88–93. doi: 10.1007/s11596-019-2004-8.30868496
28. Olerud C. The effect of the syndesmotic screw on the extension capacity of the ankle joint. Arch Orthop Trauma Surg. (1985) 104:299–302. doi: 10.1007/BF00435946.4091635
29. Briceno J, Wusu T, Kaiser P, Cronin P, Leblanc A, Miller C, et al. Effect of syndesmotic implant removal on dorsiflexion. Foot Ankle Int. (2019) 40:499–505. doi: 10.1177/1071100718818572.30654661
30. Schlundt C, El Khassawna T, Serra A, Dienelt A, Wendler S, Schell H, et al. Macrophages in bone fracture healing: their essential role in endochondral ossification. Bone. (2018) 106:78–89. doi: 10.1016/j.bone.2015.10.019.26529389
31. Sandmann ME, Shoeman JA, Thompson LV. The fiber-type-specific effect of inactivity and intermittent weight-bearing on the gastrocnemius muscle of 30-month-old rats. Arch Phys Med Rehabil. (1998) 79:658–62. doi: 10.1016/s0003-9993(98)90040-5.9630145
32. Mortreux M, Ko FC, Riveros D, Bouxsein ML, Rutkove SB. Longitudinal time course of muscle impairments during partial weight-bearing in rats. NPJ Microgravity. (2019) 5:20. doi: 10.1038/s41526-019-0080-5.31453318
33. Barfod KW, Hansen MS, Hölmich P, Kristensen MT, Troelsen A. Efficacy of early controlled motion of the ankle compared with immobilisation in non-operative treatment of patients with an acute Achilles tendon rupture: an assessor-blinded, randomised controlled trial. Br J Sports Med. (2020) 54:719–24. doi: 10.1136/bjsports-2019-100709.31597624
34. Jansen H, Jordan M, Frey S, Hölscher-Doht S, Meffert R, Heintel T. Active controlled motion in early rehabilitation improves outcome after ankle fractures: a randomized controlled trial. Clin Rehabil. (2018) 32:312–8. doi: 10.1177/0269215517724192.28805066
Keywords: peri-ankle fractures, rehabilitative exercise, meta-analysis, postoperative functional recovery, periarticular fractures
Citation: Zhao K, Dong S and Wang W (2022) When is the optimum time for the initiation of early rehabilitative exercise on the postoperative functional recovery of peri-ankle fractures? A network meta-analysis. Front. Surg. 9:911471. doi: 10.3389/fsurg.2022.911471
Received: 2 April 2022; Accepted: 29 July 2022;
Published: 16 August 2022.
Edited by:
Matthew Smuck, Stanford University, United StatesReviewed by:
Tsung-Li Lin, China Medical University Hospital, Taiwan© 2022 Zhao, dong and wang. This is an open-access article distributed under the terms of the Creative Commons Attribution License (CC BY). The use, distribution or reproduction in other forums is permitted, provided the original author(s) and the copyright owner(s) are credited and that the original publication in this journal is cited, in accordance with accepted academic practice. No use, distribution or reproduction is permitted which does not comply with these terms.
*Correspondence: Wei Wang aGJ6eXd3QGhvdG1haWwuY29t
†These authors have contributed equally to this work
Specialty Section: This article was submitted to Orthopedic Surgery, a section of the journal Frontiers in Surgery
Disclaimer: All claims expressed in this article are solely those of the authors and do not necessarily represent those of their affiliated organizations, or those of the publisher, the editors and the reviewers. Any product that may be evaluated in this article or claim that may be made by its manufacturer is not guaranteed or endorsed by the publisher.
Research integrity at Frontiers
Learn more about the work of our research integrity team to safeguard the quality of each article we publish.