Corrigendum: Glycemic Control Regimens in the Prevention of Surgical Site Infections: A Meta-Analysis of Randomized Clinical Trials
- 1Department of Nursing, The First People's Hospital of Longquanyi District, Chengdu, China
- 2Department of Internal Medicine, Yantai Qishan Hospital, Yantai, China
- 3Department of Science and Teaching, The First People's Hospital of Longquanyi District, Chengdu, China
- 4Department of Endocrinology, The First People's Hospital of Longquanyi District, Chengdu, China
- 5Department of Outpatient, China Medical University, Shenyang, China
- 6Department of OMFS, Regional Dental College, Guwahati, India
Background: Increased risk of surgical site infections (SSIs) caused by hyperglycemia makes it necessary to follow perioperative glucose lowering strategies to reduce postoperative complications. A meta-analysis was conducted to understand the efficacy of intensive vs. conventional blood glucose lowering regimens on the incidence of SSIs and hypoglycemia from various randomized controlled studies (RCTs).
Materials and Methods: A systematic literature review was conducted using MEDLINE and Central databases for RCTs that involved intensive (lower blood glucose target levels) vs. conventional (higher blood glucose target levels) strategies in patients undergoing various types of surgeries. The primary outcomes were SSIs or postoperative wound infections. Hypoglycemia and mortality outcomes were also studied. A random-effects model was used to calculate the pooled risk ratio (RR), and subgroup analyses were performed.
Results: A total of 29 RCTs were included in the meta-analysis with the information from 14,126 patients. A reduction in overall incidence of SSIs was found (RR 0.63, 0.50–0.80, p = 0.0002, I2= 56%). Subgroup analyses showed that intensive insulin regimens decreased the risk of SSIs in patients with diabetes, in cardiac and abdominal surgical procedures, and during the intraoperative and postoperative phases of surgery. However, the risk of hypoglycemia and mortality was increased in the intensive group compared to the conventional group.
Conclusion: The results of the meta-analysis provide support for the use of intensive insulin regimens during the perioperative phase for decreasing the incidence of SSIs in certain patient populations and surgical categories.
Introduction
Surgical site infections (SSIs) occur after an operative procedure and can range from superficial to deep wound infections. Global estimates of SSIs have ranged from 0.5 to 15% whereas studies in India have consistently shown higher rates from 23 to 38% (1). SSIs are a substantial cause of morbidity, prolonged hospitalization, hospital readmissions, and death and pose a considerable financial burden on healthcare systems (2, 3). Thus, the prevention and minimization of SSIs improve patient outcomes and reduce resource consumption (4, 5).
Strategies to reduce the risk of SSIs include interventions that can be delivered preoperatively, intraoperatively, or postoperatively. World Health Organization (WHO) and the Centers for Disease Control (CDC) have proposed guidelines recommending measures to prevent SSIs (6–8). Sterile procedures, maintaining patient homeostasis, wound closure interventions, and prophylactic antibiotics are commonly used to reduce the risk of SSIs (9).
Biological evidence demonstrates that diabetes can increase susceptibility to SSIs by compromising the immune system due to diminished leukocyte bactericidal activity and reduced chemotaxis and oxidative killing potential of neutrophils (10–12). Additionally, perioperative hyperglycemia or elevated blood glucose levels around the time of surgery are contributing factors to the risk of SSIs (13, 14). The perioperative period is the time surrounding a patient's surgical procedure and includes preoperative (before surgery), intraoperative (during surgery), and postoperative (after surgery) phases. Surgical stress causes the release of counter-regulatory catabolic hormones and inflammatory cytokines along with insulin tolerance and altered pancreatic β-cell function causing hyperinsulinemia and hyperglycemia (15–17). Based on the positive association of elevated blood glucose levels and SSIs, the CDC has recommended perioperative glycemic control and blood sugar levels <200 mg/dl in patients with and without diabetes for the prevention of SSIs (8). It is expected that intensive blood glucose lowering strategies will help in minimizing the risk of SSIs. However, low blood glucose levels are inherently associated with the risk of hypoglycemia, making it necessary to consider these adverse events.
Although several studies and meta-analysis on the benefits of perioperative glycemic control using intensive glucose control regimens (with stricter and lower blood glucose target levels) vs. conventional regimens (with higher blood glucose target levels) have been performed, there was no clear evidence in support of either of the treatments.
The objective of this paper is to synthesize current evidence from available randomized controlled trials evaluating the efficacy of intensive vs. conventional glucose control regimens in the prevention of SSIs in patients with and without diabetes. As lower blood glucose levels are associated with untoward outcomes like hypoglycemia, this meta-analysis also determines differences in hypoglycemia and mortality outcomes between intensive and conventional glucose control groups.
Materials and Methods
We followed the guidelines of preferred reporting items for systematic reviews and meta-analyses (PRISMA) normative recommendations in this study with the registration number SU#/IRB/2020/9342.
Search Strategy
A systematic literature search on MEDLINE (PubMed) and Cochrane Register of Controlled Trials (CENTRAL) was conducted in November 2021. No time limit was applied as several studies were published earlier than 1990. The following search terms were used in various combinations: surgical site infection, wound infection, SSI, postoperative, diabetes mellitus, hypoglycemia, insulin, blood glucose, and hypoglycemic agents. Additionally, a comprehensive list of search terms including Medical Subject Headings (MeSH) terms was applied. The titles and abstracts of studies that were potentially relevant were scanned, and the full text versions of the appropriate articles were read. Additional studies were identified by cross checking the reference lists of the relevant studies.
Study Selection or Inclusion/Exclusion Criteria
Randomized controlled studies (RCTs) and prospective randomized studies that compared intensive insulin regimen with tighter, stricter blood glucose control vs. conventional regimens were included across various surgical categories. All studies reporting SSIs or wound infections as outcomes were included irrespective of the definition of SSIs used and even if they were not the primary outcomes. Exclusion criteria were non-randomized studies, quasi-experimental studies, retrospective studies, and cohort studies.
Data Extraction and Quality Assessment
Following the identification of articles that met the inclusion criteria, data were extracted using a predefined data extraction form that included the following items: study author, publication year, surgery category, diabetes status of included patients, intervention, control, SSI data in each group, time of insulin administration, hypoglycemic events, and mortality.
The Cochrane Collaboration's risk of bias tool was used to assess the methodological quality of the included studies (18). This tool includes the following criteria: randomization, allocation concealment, blinding, and completeness of follow-up. The risk of bias for each item was graded as high, low, or unclear.
Quantitative Data Synthesis
Meta-analysis was performed using Review Manager (RevMan, Version 5. Copenhagen: The Nordic Cochrane Center, The Cochrane Collaboration, 2020). Absolute numbers of participants in each study developing a wound infection or SSI and the total number of participants in each group (intervention and control group) were used to calculate the risk ratio (RR) and the 95% confidence interval (CI). Meta-analyses were done using a random-effects model (the Mantel–Haenszel method), and heterogeneity in the included studies was evaluated using I2 statistic, with small heterogeneity for I2 values of 25%, moderate heterogeneity for I2 values of 25–50%, and high heterogeneity for I2 values >50% (19). Forest plots were constructed, and p < 0.05 was statistically significant. Subgroup analyses were also performed according to target blood glucose levels of intervention group, diabetes status of patients, timing of insulin administration, surgery type, and types of SSI.
Publication bias was assessed by a funnel plot in which the log RR for each study was plotted against its SE.
Results
Identification of Studies
A total of 2,362 records were identified by database searching of which 2,087 were screened by the title and abstract. Irrelevant records were removed (n = 1,851), and 236 RCTs were assessed for eligibility. However, 207 RCTs were excluded due to reasons such as inappropriate comparator groups, wound infections not reported as outcomes, an inappropriate trial design, and the lack of data. The process of selection is shown in Figure 1.
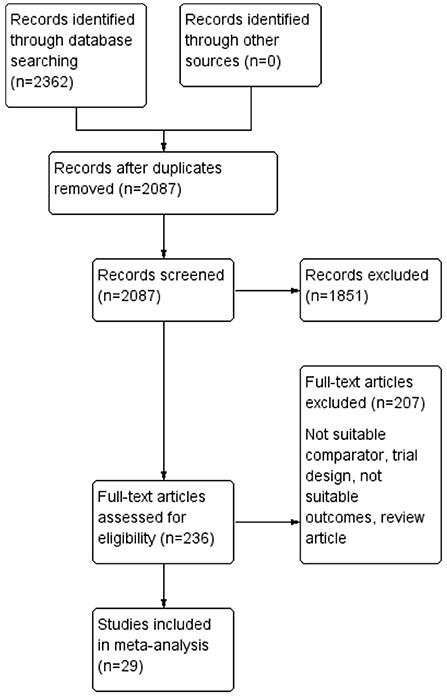
Figure 1. A flow chart for the identification and inclusion of studies in the meta-analysis according to Preferred Reporting Items for Systematic and Meta-Analyses (PRISMA).
Study Characteristics
In total, 29 RCTs totaling 14,126 participants met the inclusion criteria (intensive intervention group: 7,351 participants and control group: 6,775 participants). These RCTs involved a comparison of intensive blood glucose control to conventional blood glucose control or standard treatment regimens across various surgical categories in the perioperative period. All studies were randomized controlled trials or prospective randomized studies with sample sizes ranging from 26 to 6,025 participants. The studies included male and female participants undergoing various surgical procedures both with and without diabetes or mixed populations. All studies utilized insulin infusion in the intensive treatment group whereas insulin infusion and sliding-scale subcutaneous insulin administration were used in the conventional treatment group. SSIs were defined according to different criteria in the studies (Tables 1, 2). In the intensive treatment groups, blood glucose levels were mainly targeted between 80 and 120 mg/dl and liberal blood glucose values of <250 mg/dl were used in the conventional group.
Types of Surgery and Timing of Administration
Most studies were conducted on participants undergoing cardiac surgery (n = 15), neurosurgery (n = 3), and abdominal procedures (n = 4). In 5 studies, insulin was administered in the intraoperative phase, intraoperative and postoperative insulin administration was carried out in 15 studies, and in 7 studies insulin was administered postoperatively.
Bias Assessment
The results of the risk of bias evaluation are shown in Figure 2. Overall, there was a moderate to high risk of bias due to an unclear or a high risk related to randomization, blinding, and selective reporting domains.
The funnel plot was asymmetrical (Figure 3), indicating the possibility of publication bias.
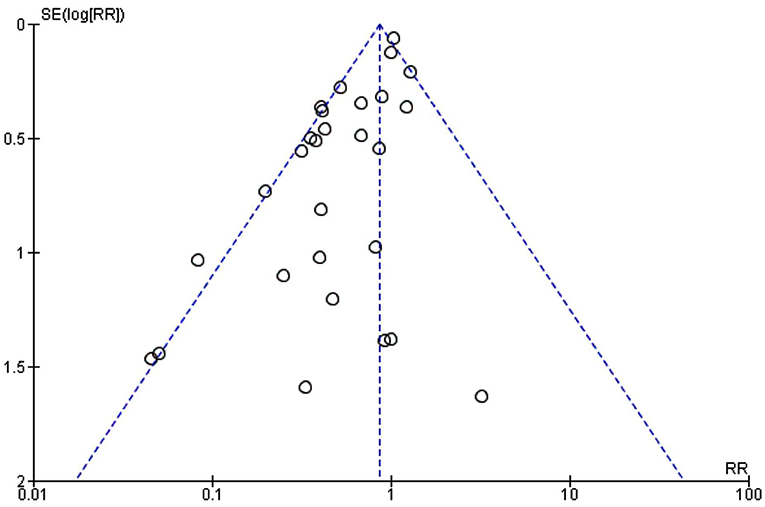
Figure 3. A funnel plot to assess publication bias in meta-analysis with the surgical site infection (SSI) outcome.
Surgical Site or Wound Infection Rates
The incidence of a SSI or wound infection in the included studies is shown in Table 3. The incidence of SSIs ranged from 0 to 32.3% in the intensive intervention group and from 0 to 66.7% in the conventional treatment group. The overall incidence of SSIs was 8.6% in the intensive intervention group and 10.7% in the conventional treatment group. Table 4 shows data for the types of SSI reported in the included studies.
Meta-Analysis Results
In addition, there was no significant difference between the subgroups indicating that the intensive regimen was preferred irrespective of the target blood glucose levels. Moderate to high heterogeneity could be attributed to the diabetes status of patients or the types of surgery (Figure 4).
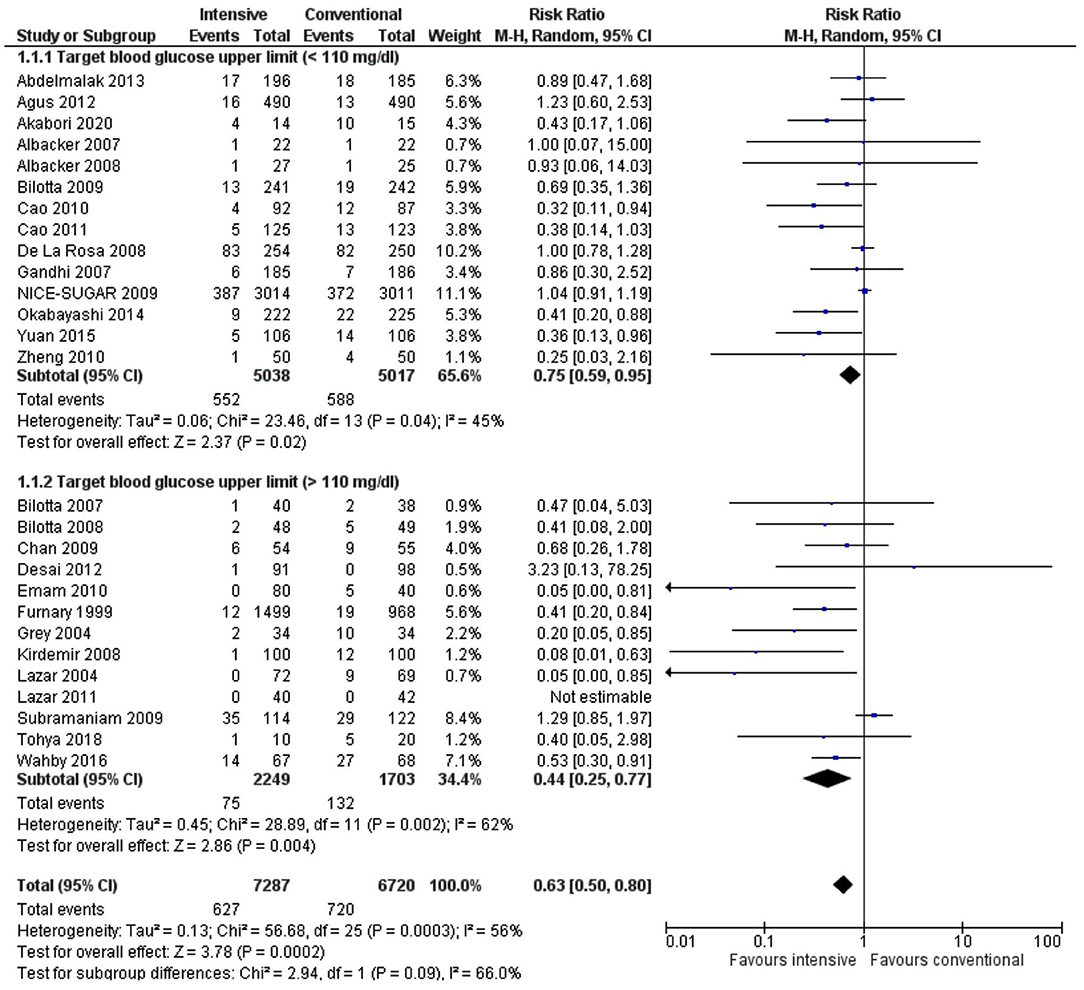
Figure 4. A forest plot for a subgroup analysis of target blood glucose level in the intervention group in studies using a random-effects model. Risk ratios (RRs) and 95% CIs are shown.
Stratification of the results by the patient population (diabetic, non-diabetic, or mixed) showed a significant decrease in the incidence of SSIs in favor of the intensive treatment group for the diabetic population only (RR 0.40, 0.28–0.56, p < 0.00001, I2 = 2%). In contrast, there was no significant difference in the SSI rate for studies, including non-diabetics (RR 0.61, 0.23–1.66, p = 0.33, I2 = 57%) or studies with both diabetic and non-diabetic patients (RR 0.93, 0.78–1.10, p = 0.38, I2= 16%) (Figure 5). The test for subgroup differences indicated a statistically significant subgroup difference (p < 0.0001), indicating that patient diabetic status does influence the response to the intensive glycemic control treatment. High heterogeneity (I2 = 57%) in the nondiabetic subgroup could be due to a low number of studies in this population and their inclusion in the analysis (n = 3).
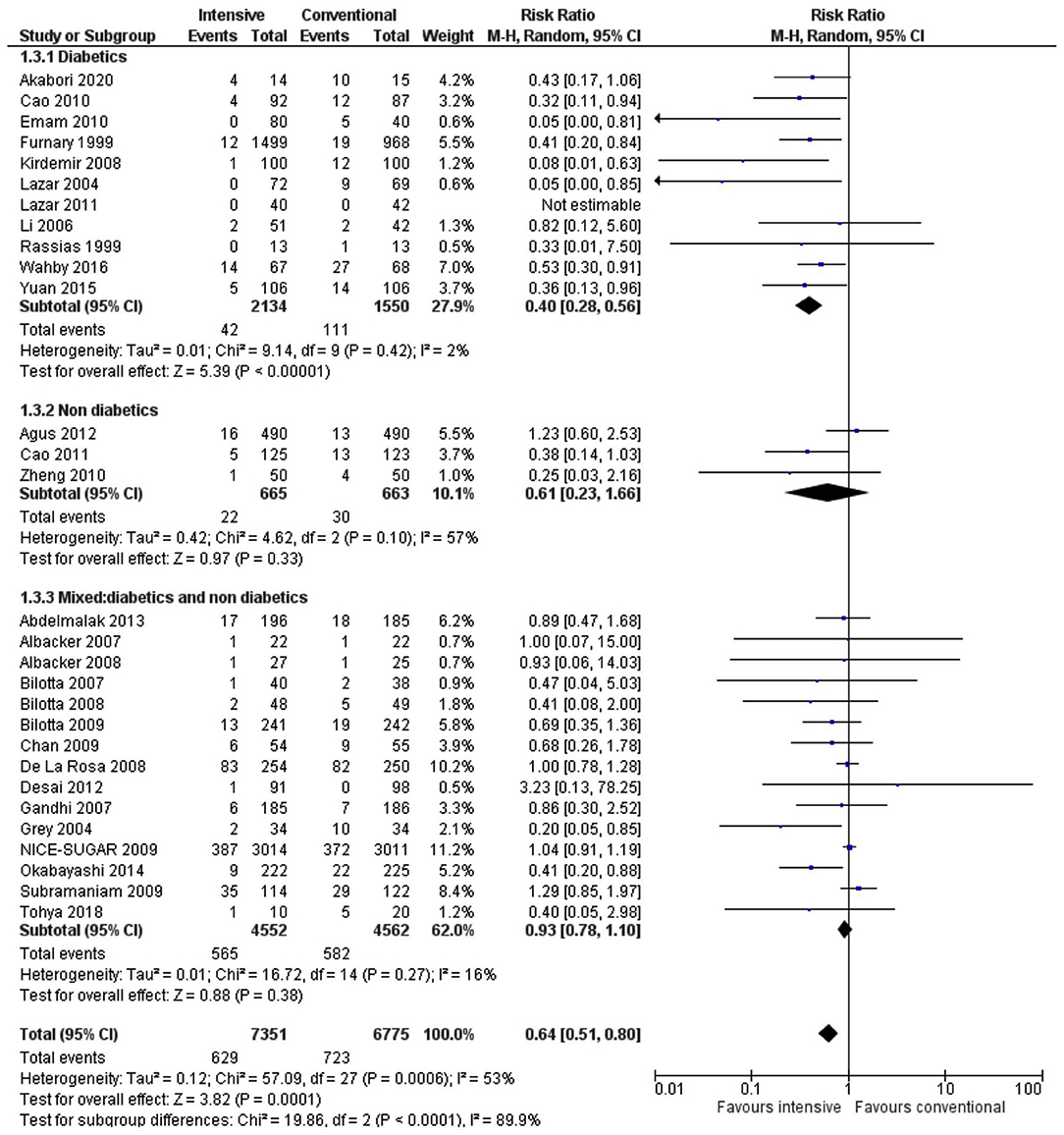
Figure 5. A forest plot for a subgroup analysis depending on the patient diabetes status in studies using a random-effects model. RRs and 95% CIs are shown.
A subgroup analysis showed that a decrease in the incidence of SSIs was statistically significant following intensive insulin treatment in cardiac surgery (RR 0.55, 0.36–0.85, p = 0.007, I2 = 28%) and abdominal surgery (RR 0.37, 0.23–0.61, p < 0.0001, I2 = 0%) but not in neurosurgery (RR 0.62, 0.34–1.14, p = 0.12, I2 = 0%). Furthermore, there was a significant influence of the type of surgery (p = 0.009) on the effect of the intensive insulin regimen (Figure 6).
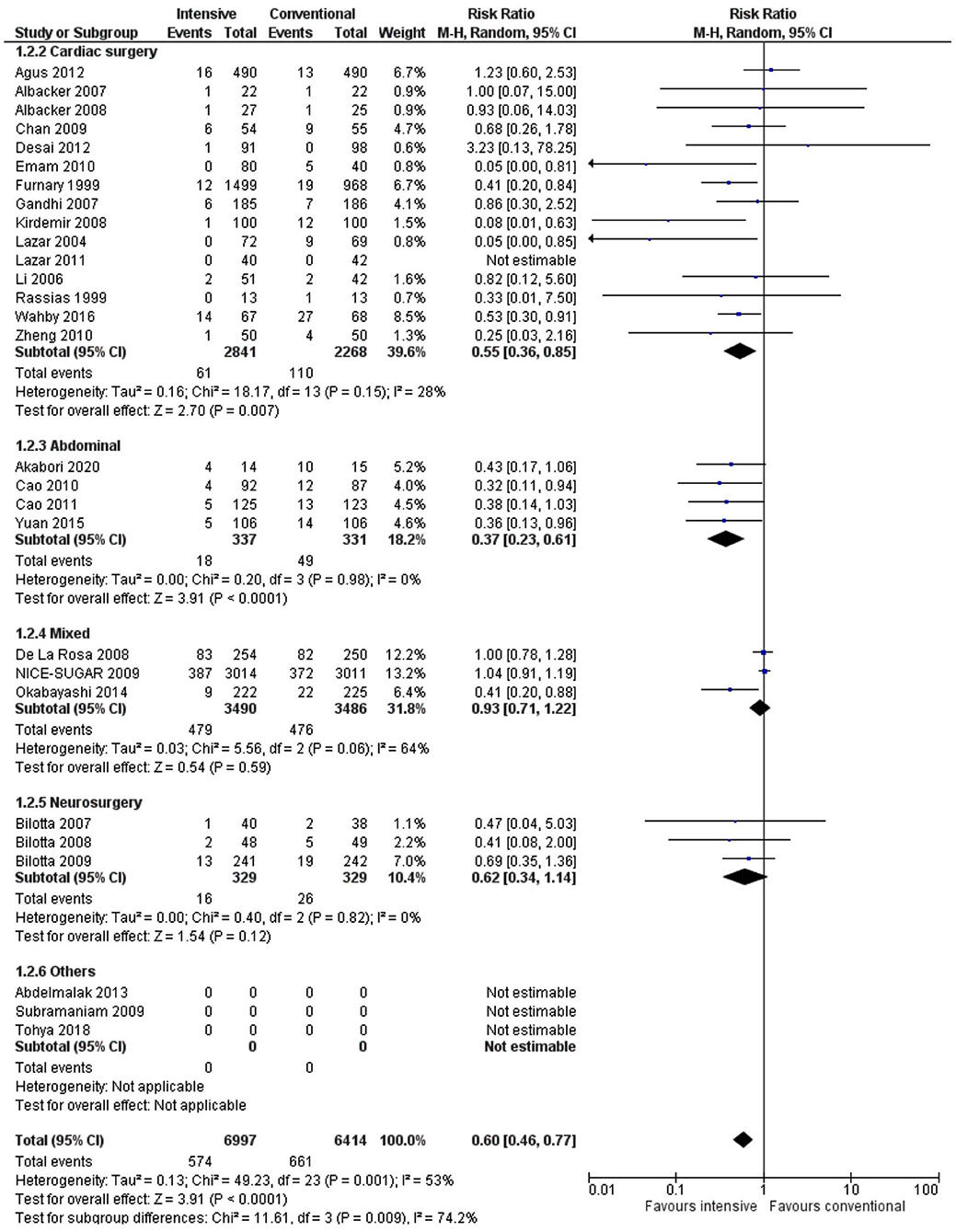
Figure 6. A forest plot for a subgroup analysis according to surgery type in studies using a random-effects model. RRs and 95% CIs are shown.
Intraoperative and postoperative insulin administration or only postoperative administration was associated with a significantly lower SSI risk (RR 0.64, 0.45–0.91, p = 0.01, I2 = 55% and RR 0.49, 0.30–0.80, p = 0.004, I2 = 35%) compared to when intensive treatment was done only during the intraoperative phase (RR 0.80, 0.51–1.25, p = 0.32, I2 = 37%) (Figure 7).
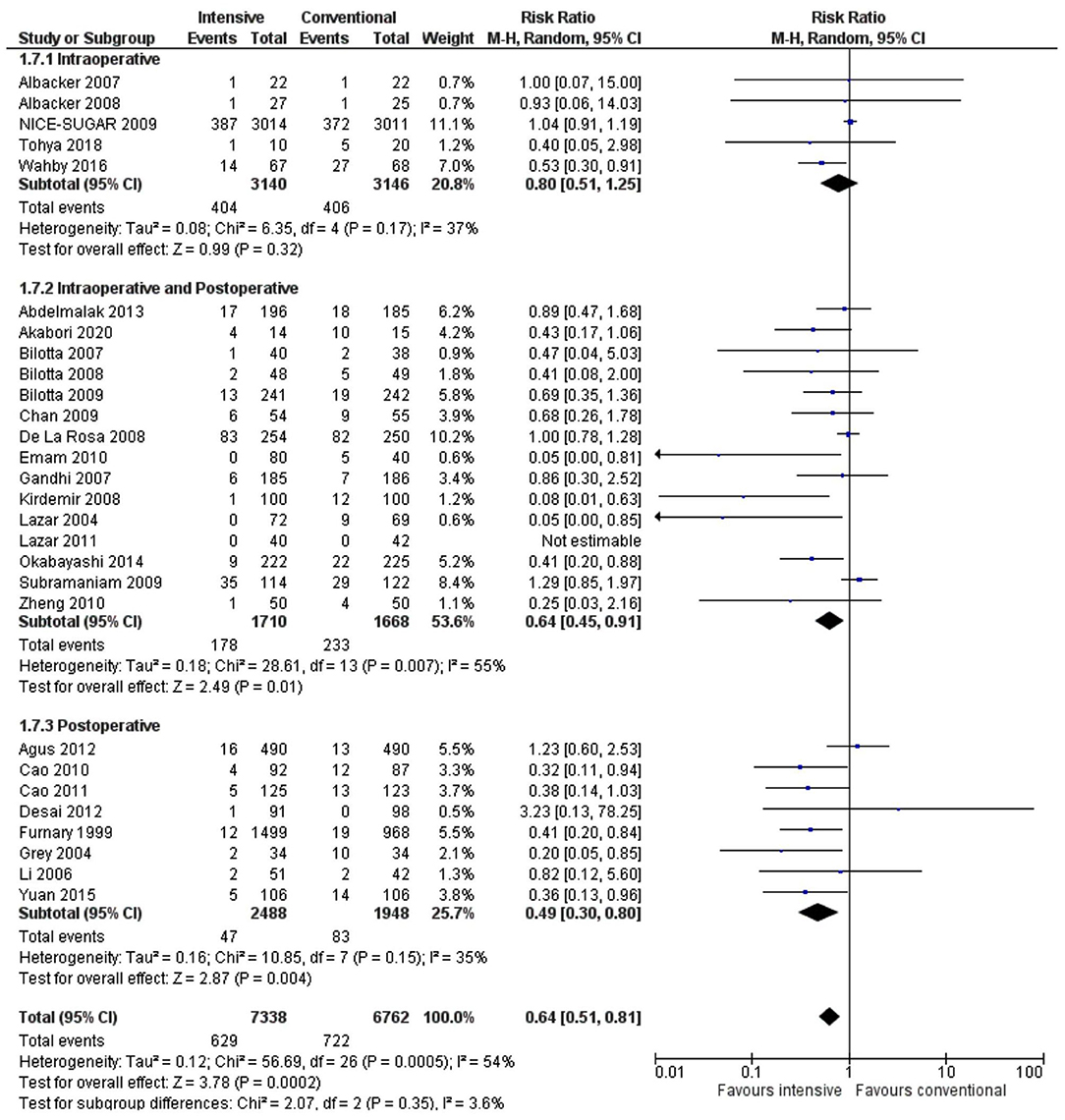
Figure 7. A forest plot for a subgroup analysis according to timing of insulin administration in studies using a random-effects model. RRs and 95% CIs are shown.
The risk of hypoglycemia and mortality was significantly higher in the intensive treatment compared to the conventional group (RR 3.90, 1.78–8.51, p = 0.0006, I2 = 99% and RR 1.10, 1.01–1.19, p = 0.02, I2 = 0%) (Figures 8, 9). High heterogeneity for the hypoglycemia outcome could be due to various surgical procedures and mixed patient populations as well as an inconsistent definition for hypoglycemia.
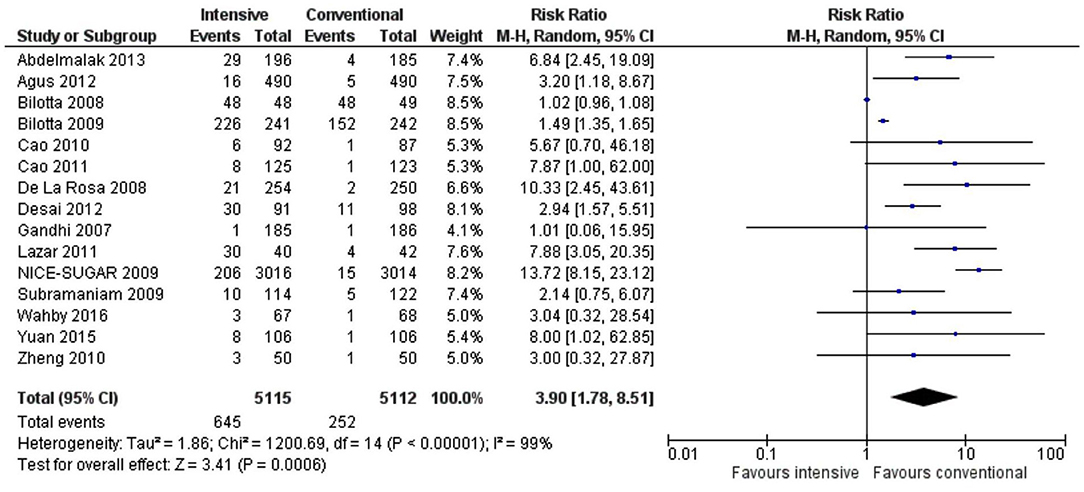
Figure 8. A forest plot of hypoglycemic events for studies using a random-effects model. RRs and 95% CIs are shown.
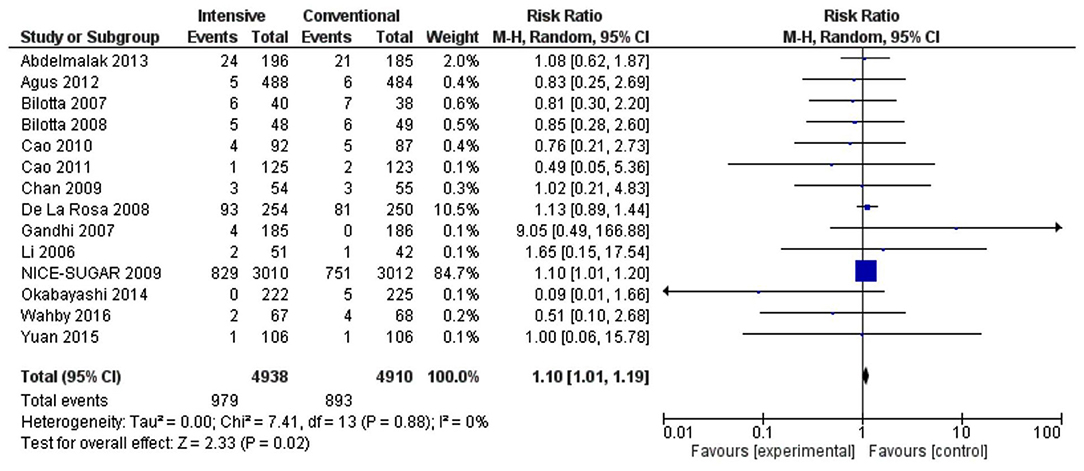
Figure 9. Forest plot of mortality outcome for studies using a random-effects model. RRs and 95% CIs are shown. Experimental: intensive, control: conventional.
There was no significant difference in the incidence of superficial or deep SSIs between intensive and conventional groups (superficial SSI RR 0.56, 0.14–2.22, p = 0.41, I2 = 0% and deep SSI RR 0.86, 0.51–1.45, p = 0.45, I2 = 0%).
Discussion
The present study provides current and valuable information on the efficacy of intensive blood glucose lowering strategies in decreasing the incidence of SSIs across various surgical categories and in different patient populations with regard to the diabetes status. In this meta-analysis, most studies utilized intensive insulin treatment for targeting blood glucose levels 70–120 mg/dl in the intraoperative and postoperative, and only postoperative phases of surgery.
Unlike a previous meta-analysis on a similar topic, the current meta-analysis attempts to categorize different subgroups and determine the efficacy of intensive insulin treatment by a subgroup, thus attempting to determine where tight glucose control is most likely to be beneficial. The utilization of intensive glucose control protocols in diabetic patient populations, cardiac, and abdominal surgical procedures, and during the intraoperative and postoperative and only the postoperative phase of surgery were shown to be associated with a decreased risk of SSIs compared to when conventional strategies were used. Furthermore, a decrease in the incidence of SSIs was observed irrespective of whether target blood glucose levels during intensive treatment were <110 or >110 mg/dl. Kao et al. (13) concluded that studies comparing intensive and conventional glycemic control regimens could not be meta-analyzed due to heterogeneity in patient populations, the type of surgery, mortality risk, timing of administration, the route of administration, and target blood glucose levels. A recent meta-analysis in 2017 by de Vries et al. included 15 RCTs and showed that stricter blood glucose target levels of <150 mg/dl using reduced SSIs with an inherent risk of hypoglycemic events (49). This meta-analysis includes more studies and provides pooled effect estimates for hypoglycemic events and adverse events such as mortality.
Despite the beneficial effects of lowering SSI rates, the current meta-analysis showed a higher risk of mortality caused by intensive insulin therapy. However, it is important to note most of this data came from the Normoglycemia in Intensive Care Evaluation-Survival Using Glucose Algorithm Regulation (NICE-SUGAR) study which included critically ill patients and in which the blood glucose target levels in the intensive insulin group were comparatively lower than other studies (81–108 mg/dl) (41). Similar results were seen in the study by Gandhi (35) wherein the achieved blood glucose levels were much lower. However, increased hypoglycemic events were seen in almost all studies that reported hypoglycemia as an outcome. High heterogeneity seen in the meta-analysis of the results for hypoglycemia can be attributed to differences in definitions of hypoglycemia used between the included trials, which were either the number of patients experiencing at least one hypoglycemic episode or the percentage of glucose measurements below a cut-off value. Increased monitoring of glucose levels in the intensive groups vs. conventional groups could have also resulted in measurement bias and increase in hypoglycemic events. Therefore, these results should be interpreted with caution and may not provide an exact estimate. Furthermore, hypoglycemic episodes in most studies were asymptomatic and the reduction in SSIs probably outweighs the risk of hypoglycemia, thereby supporting the use of intensive treatment. With regard to oral surgery, Tohya et al. (45) investigated the effects of intraoperative glycemic control by glucose–insulin infusion to achieve a target blood glucose level of 80–120 mg/dl in patients with major oral or maxillofacial surgeries compared to the infusion of Ringer's lactate solution alone. This study did not find a significant difference in the incidence of SSIs between the groups (p = 0.31) but is limited by a small sample size (n = 30) (45).
Although there was no significant difference between the rates of SSIs in intensive and conventional glucose control groups when stratified by the types of SSI, the small number of studies used to obtain the results should be considered during interpretation. Additionally, the types of SSI (superficial or deep) was reported only in case of cardiac surgeries. Most studies reported the incidence of overall SSIs or wound infections.
Limitations
Although this meta-analysis provides insights into which cases intensive insulin treatment may be beneficial, there are some limitations. The definition of SSIs and follow-up times differed among studies, which can potentially affect the results. Several other studies were found during the literature search process that involved a comparison of intensive with conventional glucose control regimens, which reported infections as outcomes. However, the lack of data on SSIs or wound infections resulted in these studies being excluded from the analysis. Details on postoperative nutritional protocols and antibiotic or other concomitant medication use were not reported in most studies, which can confound the results. Most studies included in the meta-analysis were performed in patients undergoing cardiac surgery and those reporting ICU stay, which limits generalizability of the results to a wider patient population. In most cases, the achieved blood glucose levels are associated with the outcomes of SSIs and hypoglycemia and not the target level or route of administration, which makes it essential to report actual achieved levels in all the studies. Another important aspect that needs to be considered when evaluating perioperative glucose control for the reduction of SSIs is the safety and feasibility of administering insulin as this can increase healthcare costs and require skilled staff. The risk of bias was moderate to high in several domains due to unclear risks making it difficult to ascertain trial quality, which is another challenge.
Conclusion
Regardless of possible limitations, the present meta-analysis indicates that perioperative glucose control using an intensive regimen is beneficial in decreasing the incidence of SSIs in some patient populations, and surgeries depending on the timing of administration and should be considered. The use of intensive glucose lowering strategies should be carried out with regard to current surgical practices and procedures and regarding the patient status with adequate safety monitoring.
Data Availability Statement
The original contributions presented in the study are included in the article/supplementary material, further inquiries can be directed to the corresponding author/s.
Ethics Statement
All procedures performed in the study were in accordance with the institutional and/or national research committee's standards and with the 1964 Helsinki declaration and its later amendments or comparable ethical standards.
Author Contributions
JL and QL derived a concept and designed this study. YH analyzed data and drafting of this manuscript. SZ collected the data and helped in data analysis. XB and SR: proofreading and final editing along with guarantor of the manuscript. All authors read and approved the final version of the manuscript.
Funding
Scientific research topics of Sichuan Institute of Health Information (2019040) Construction and Application of Intelligent Learning Adverse Event Reporting System Based on Clinical Knowledge Base.
Conflict of Interest
The authors declare that the research was conducted in the absence of any commercial or financial relationships that could be construed as a potential conflict of interest.
Publisher's Note
All claims expressed in this article are solely those of the authors and do not necessarily represent those of their affiliated organizations, or those of the publisher, the editors and the reviewers. Any product that may be evaluated in this article, or claim that may be made by its manufacturer, is not guaranteed or endorsed by the publisher.
References
1. Arora A, Bharadwaj P, Chaturvedi H, Chowbey P, Gupta S, Leaper D, et al. A review of prevention of surgical site infections in Indian hospitals based on global guidelines for the prevention of surgical site infection. JPSIC. (2018) 6:1–12. doi: 10.4103/jpsic.jpsic_29_17
3. Norman G, Atkinson RA, Smith TA, Rowlands C, Rithalia AD, Crosbie EJ, et al. Intracavity lavage and wound irrigation for prevention of surgical site infection. Cochr Database Syst Rev. (2017) 10:CD012234. doi: 10.1002/14651858.CD012234.pub2
4. Dimick JB, Chen SL, Taheri PA, Henderson WG, Khuri SF, Campbell DA. Hospital costs associated with surgical complications: a report from the private-sector national surgical quality improvement program. J Am Coll Surg. (2004) 19:531–7. doi: 10.1016/j.jamcollsurg.2004.05.276
5. Astagneau P, Rioux C, Golliot F, Brücker G, INCISO Network Study Group. Morbidity and mortality associated with surgical site infections: results from the 1997-1999 INCISO survellaince. J Hosp Infect. (2001) 48:267–74. doi: 10.1053/jhin.2001.1003
6. Allegranzi B, Bischoff P, de Jonge S, Kubilay NZ, Zayed B, Gomes SM, et al. New WHO recommendations on preoperative measures for surgical site infection prevention: an evidence-based global perspective. Lancet. (2016) 3099:1–12. doi: 10.1016/S1473-3099(16)30398-X
7. Allegranzi B, Zayed B, Bischoff P, Kubilay NZ, de Jonge S, de Vries F, et al. New WHO recommendations on intraoperative and postoperative measures for surgical site infection prevention: an evidence-based global perspective. Lancet. (2016) 3099:1–16. doi: 10.1016/S1473-3099(16)30402-9
8. Berrios-Torres SI, Umscheid CA, Bratzler DW, Leas B, Stone EC, Kelz RR, et al. Centers for Disease Control and Prevention Guideline for the prevention of surgical site infection. JAMA Surg. (2017) 152:784–91. doi: 10.1001/jamasurg.2017.0904
9. Liu Z, Dumville JC, Norman G, Westby MJ, Blazeby J, McFarlane E, et al. Intraoperative interventions for preventing surgical site infection: an overview of Cochrane Reviews. Cochrane Datab Syst Rev. (2018) 2:CD012653. doi: 10.1002/14651858.CD012653.pub2
10. Jeon CY, Furuya EY, Berman MF, Larson EL. The role of pre-operative and post-operative glucose control in surgical-site infections and mortality. PLOS ONE. (2012) 7:e45616. doi: 10.1371/journal.pone.0045616
11. Delamaire M, Maugendre D, Moreno M, Le Goff MC, Allannic H, Genetet B. Impaired leucocyte functions in diabetic patients. Diabet Med. (1997) 14:29–34. doi: 10.1002/(SICI)1096-9136(199701)14:1<29::AID-DIA300>3.0.CO;2-V
12. Rayfield EJ, Ault MJ, Keusch GT, Brothers MJ, Nechemias C, Smith H. Infection and diabetes: the case for glucose control. Am J Med. (1982) 72:439–50. doi: 10.1016/0002-9343(82)90511-3
13. Kao LS, Meeks D, Moyer VA, Lally KP. Peri-operative glycemic control regimens for preventing surgical site infections in adults. Cochrane Database Syst Rev. (2010) 3:CD006806. doi: 10.1002/14651858.CD006806.pub2
14. Estrada CA, Young JA, Nifong LW, Chitwood WR Jr. Outcomes and perioperative hyperglycemia in patients with or without diabetes mellitus undergoing coronary artery bypass grafting. Ann Thoracic Surg. (2003) 75:1392–9. doi: 10.1016/S0003-4975(02)04997-4
15. Qadan M, Weller EB, Gardner SA, Maldonado C, Fry DE, Polk HC Jr. Glucose and surgical sepsis: a study of underlying immunologic mechanisms. J Am Coll Surg. (2010) 210:966–74. doi: 10.1016/j.jamcollsurg.2010.02.001
16. Dagogo-Jack S, Alberti KGMM. Management of diabetes mellitus in surgical patients. Diabetes Spectr. (2002) 15:44–8. doi: 10.2337/diaspect.15.1.44
17. Esposito K, Nappo F, Marfella R, Guigliano G, Guigliano F, Ciotola M, et al. Inflammatory cytokine concentrations are acutely increased by hyperglycemia in humans: role of oxidative stress. Circulation. (2002) 106:2067–72. doi: 10.1161/01.CIR.0000034509.14906.AE
18. Higgins JPT, Altman DG, Gøtzsche PC, Jüni P, Moher D, Oxman AD, et al. The Cochrane Collaboration's tool for assessing risk of bias in randomised trials. BMJ. (2011) 343:d5928. doi: 10.1136/bmj.d5928
19. Higgins JPT, Thompson SG, Deeks JJ, Altman D. Measuring inconsistency in meta-analyses. BMJ. (2003) 327:557–56. doi: 10.1136/bmj.327.7414.557
20. Abdelmalak BB, Bonilla A, Mascha EJ, Maheshwari A, Tang WHW, You J, et al. Dexamethasone, light anaesthesia, and tight glucose control (DeLiT) randomized controlled trial. Br J Anaesthesia. (2013) 111:209–21. doi: 10.1093/bja/aet050
21. Agus MS, Steil GM, Wypij D, Costello JM, Laussen PC, Langer M, et al. Tight glycemic control versus standard care after pediatric cardiac surgery. N Engl J Med. (2012) 367:1208–19. doi: 10.1056/NEJMoa1206044
22. Akabori H, Tani M, Kitamura N, Maehira H, Imashuku Y, Tsujita Y, et al. Perioperative tight glycemic control using artificial pancreas decreases infectious complications via suppression of inflammatory cytokines in patients who underwent pancreaticoduodenectomy: a prospective, non-randomized clinical trial. Am J Surg. (2020) 220:365–71. doi: 10.1016/j.amjsurg.2019.12.008
23. Albacker TB, Carvalho G, Schricker T, Lachapelle K. Myocardial protection during elective coronary artery bypass grafting using high-dose insulin therapy. Ann Thorac Surg. (2007) 84:1920–7. doi: 10.1016/j.athoracsur.2007.07.001
24. Albacker T, Carvalho G, Schricker T, Lachapelle K. High-dose insulin therapy attenuates systemic inflammatory response in coronary artery bypass grafting patients. Ann Thorac Surg. (2008) 86:20–8. doi: 10.1016/j.athoracsur.2008.03.046
25. Bilotta F, Spinelli A, Giovannini F, Doronzio A, Delfini R, Rosa G. The effect of intensive insulin therapy on infection rate, vasospasm, neurologic outcome, and mortality in neurointensive care unit after intracranial aneurysm clipping in patients with acute subarachnoid hemorrhage: a randomized prospective pilot trial. J Neurosurg Anesthesiol. (2007) 19:156–60. doi: 10.1097/ANA.0b013e3180338e69
26. Bilotta F, Caramia R, Cernak I, Paoloni FP, Doronzio A, Cuzzone V, et al. Intensive insulin therapy after severe traumatic brain injury: a randomized clinical trial. Neurocrit Care. (2008) 9:159–66. doi: 10.1007/s12028-008-9084-9
27. Bilotta F, Caramia R, Paoloni FP, Delfini R, Rosa G. Safety and efficacy of intensive insulin therapy in critical neurosurgical patients. Anesthesiology. (2009) 110:611–9. doi: 10.1097/ALN.0b013e318198004b
28. Cao S-G, Ren J-A, Shen B, Chen D, Zhou Y-B, Li J-S. Intensive versus conventional insulin therapy in type 2 diabetes patients undergoing D2 gastrectomy for gastric cancer: a randomized controlled trial. World J Surg. (2011) 35:85–92. doi: 10.1007/s00268-010-0797-5
29. Cao S, Zhou Y, Chen D, Niu Z, Wang D, Lv L, et al. Intensive versus conventional insulin therapy in nondiabetic patients receiving parenteral nutrition after D2 gastrectomy for gastric cancer: a randomized controlled trial. J Gastrointest Surg. (2011) 15:1961–8. doi: 10.1007/s11605-011-1654-z
30. Chan RP, Galas FR, Hajjar LA, Bello CN, Piccioni MA, Auler JO. Intensive perioperative glucose control does not improve outcomes of patients submitted to open-heart surgery: a randomized controlled trial. Clinics. (2009) 64:51–60. doi: 10.1590/S1807-59322009000100010
31. De La Rosa GD, Donado JH, Restrepo AH, Quintero AM, González LG, Saldarriaga NE, et al. Strict glycaemic control in patients hospitalised in a mixed medical and surgical intensive care unit: a randomised clinical trial. Critical Care. (2008) 12:R120. doi: 10.1186/cc7017
32. Desai SP, Henry LL, Holmes SD, Hunt SL, Martin CT, Hebsur S, et al. Strict versus liberal target range for perioperative glucose in patients undergoing coronary artery bypass grafting: a prospective randomized controlled trial. J Thoracic Cardiovas Surg. (2012) 143:318–25. doi: 10.1016/j.jtcvs.2011.10.070
33. Emam IA, Allan A, Eskander K, Dhanraj K, Farag E-S, El-Kadi Y, et al. Our experience of controlling diabetes in the peri-operative period of patients who underwent cardiac surgery. Diab Res Clin Prac. (2010) 88:242–6. doi: 10.1016/j.diabres.2010.03.002
34. Furnary AP, Zerr KJ, Grunkemeier GL, Starr A. Continuous intravenous insulin infusion reduces the incidence of deep sternal wound infection in diabetic patients after cardiac surgical procedures. Ann Thorac Surg. (1999) 67:352–62. doi: 10.1016/S0003-4975(99)00014-4
35. Gandhi GY, Nuttall GA, Abel MD, Mullany CJ, Schaff HV, O'Brein PC, et al. Intensive intraoperative insulin therapy versus conventional glucose management during cardiac surgery. Ann Int Med. (2007) 146:233. doi: 10.7326/0003-4819-146-4-200702200-00002
36. Grey NJ, Perdrizet GA. Reduction of nosocomial infections in the surgical intensive-care unit by strict glycemic control. Endocr Pract. (2004) 10(Suppl):46–52. doi: 10.4158/EP.10.S2.46
37. Kirdemir P, Yildirim V, Kiris I, Kuralay E, Ibrisim E, Ozal E, et al. Does continuous insulin therapy reduce postoperative supraventricular tachycardia incidence after coronary artery bypass operations in diabetic patients? J Cardiothorac Vasc Anesth. (2008) 22:383–7. doi: 10.1053/j.jvca.2007.09.015
38. Lazar HL, Chipkin SR, Fitzgerald CA, Bao Y, Cabral H, Apstein CS. Tight glycemic control in diabetic coronary artery bypass graft patients improves perioperative outcomes and decreases recurrent ischemic events. Circulation. (2004) 109:1497–502. doi: 10.1161/01.CIR.0000121747.71054.79
39. Lazar HL, McDonnell MM, Chipkin S, Fitzgerald C, Bliss C, Cabral H. Effects of aggressive versus moderate glycemic control on clinical outcomes in diabetic coronary artery bypass graft patients. Ann Surg. (2011) 254:458–64. doi: 10.1097/SLA.0b013e31822c5d78
40. Li JY, Sun S, Wu SJ. Continuous insulin infusion improves postoperative glucose control in patients with diabetes mellitus undergoing coronary artery bypass surgery. Tex Heart Inst J. (2006) 33:445–51.
41. NICE-SUGAR Study Investigators. Intensive versus conventional glucose control in critically ill patients. N Engl J Med. (2009) 360:1283–97. doi: 10.1056/NEJMoa0810625
42. Okabayashi T, Shima Y, Sumiyoshi T, Kozuki A, Tokumaru T, Iiyama T, et al. Intensive versus intermediate glucose control in surgical intensive care unit patients. Diab Care. (2014) 37:1516–24. doi: 10.2337/dc13-1771
43. Rassias AJ, Marrin CAS, Arruda J, Whalen PK, Beach M, Yeager MP. Insulin infusion improves neutrophil function in diabetic cardiac surgery patients. Anesth Analg. (1999) 88:1011–6. doi: 10.1213/00000539-199905000-00008
44. Subramaniam B, Panzica PJ, Novack V, Mahmood F, Matyal R, Mitchell JD, et al. Continuous perioperative insulin infusion decreases major cardiovascular events in patients undergoing vascular surgery. Anesth. (2009) 110:970–7. doi: 10.1097/ALN.0b013e3181a1005b
45. Tohya A, Kohjitani A, Ohno S, Yamashita K, Manabe Y, Sugimura M. Effects of glucose-insulin infusion during major oral and maxillofacial surgery on postoperative complications and outcomes. JA Clin Rep. (2018) 4:9. doi: 10.1186/s40981-018-0148-3
46. Wahby EA, Elnasr MM, Eissa MI, Mahmoud SM. Perioperative glycemic control in diabetic patients undergoing coronary artery bypass graft surgery. J Egyptian Soc CardioThorac Surg. (2016) 24:143–9. doi: 10.1016/j.jescts.2016.05.007
47. Yuan J, Liu T, Zhang X, Si Y, Ye Y, Zhao C, et al. Intensive versus conventional glycemic control in patients with diabetes during enteral nutrition after gastrectomy. J Gastroinst Surg. (2015) 19:1553–8. doi: 10.1007/s11605-015-2871-7
48. Zheng R, Gu C, Wang Y, Yang Z, Dou K, Wang J, et al. Impacts of intensive insulin therapy in patients undergoing heart valve replacement. Heart Surg Forum. (2010) 13:E292–298. doi: 10.1532/HSF98.20101017
Keywords: surgical site infections, glycemic control, laparoscopic surgeries, neurosurgeries, general surgery
Citation: Lai J, Li Q, He Y, Zou S, Bai X and Rastogi S (2022) Glycemic Control Regimens in the Prevention of Surgical Site Infections: A Meta-Analysis of Randomized Clinical Trials. Front. Surg. 9:855409. doi: 10.3389/fsurg.2022.855409
Received: 30 January 2022; Accepted: 18 February 2022;
Published: 25 March 2022.
Edited by:
Mahesh C. Misra, All India Institute of Medical Sciences, IndiaReviewed by:
Mohit Kumar Joshi, All India Institute of Medical Sciences, IndiaAnurag Srivastava, BALCO Medical Centre, India
Copyright © 2022 Lai, Li, He, Zou, Bai and Rastogi. This is an open-access article distributed under the terms of the Creative Commons Attribution License (CC BY). The use, distribution or reproduction in other forums is permitted, provided the original author(s) and the copyright owner(s) are credited and that the original publication in this journal is cited, in accordance with accepted academic practice. No use, distribution or reproduction is permitted which does not comply with these terms.
*Correspondence: Xiaodong Bai, YmFpeGlhb2RvbmcxNDcmI3gwMDA0MDtnbWFpbC5jb20=
†These authors have contributed equally to this work and share first authorship