- 1Department of Orthopaedics, Chinese PLA General Hospital, Beijing, China
- 2National Clinical Research Center for Orthopedics, Sports Medicine & Rehabilitation, Beijing, China
The risk of bisphosphonate (BP)-associated atypical femur fracture (AFF) has markedly increased over recent decades due to suppression of bone turnover, accumulation of structural micro-damage and reduction of bone remodeling consequent to long-term BP treatment. These medications further delay bone union and result in challenging clinical management. Teriparatide (TPTD), a synthetic human parathyroid hormone, exhibits unique anabolic effects and can increase bone remodeling and improve bone microarchitecture, further promoting fracture healing and reducing the rate of bone non-union. In this study, we briefly define AFF as well as the effects of BPs on AFFs, detailed the role of TPTD in AFF management and the latest clinical therapeutic findings. We have confirmed that TPTD positively promotes the healing of AFFs by reducing the time to bone union and likelihood of non-union. Thus, teriparatide therapy could be considered as an alternative treatment for AFFs, however, further research is required for the establishment of effective clinical guidelines of TPTD use in the management of AFF.
Background
As the mainstay of osteoporosis treatment, bisphosphonates (BPs) can significantly decrease the risk of hip and vertebral fractures in osteoporosis in that these medications effectively increase bone mass and enhance bone strength (1, 2). However, an increasing number of studies have suggested that AFFs are closely associated with BP therapy due to consequent suppression of bone turnover, reduced remodeling and ultimately impaired healing capacity in the setting of long-term treatment (>5 years) (3–6). Despite a low incidence of AFFs (3.0–9.8 per 100,000 person-years) (7), such fractures are often resistant to therapy, result in poor bone union and high implant failure rates due to hardened bone and complete medullary canal obliteration (8–10). The mean healing time of AFFs postoperatively was 7.3 months (2–31 months) reported in literature; nearly a tenth of patients required revision surgery due to bone non-union or implant failure and approximately half of patients conservatively managed for incomplete fractures were eventually treated surgically (11). Furthermore, younger patients (aged <65 years) have tended to be more affected as compared to the older population (aged>65 years) (12). AFFs thus not only represent a serious health issue and enormous economic burden for patients, but also present a challenging clinical situation to manage for orthopedic surgeons.
Although the guidelines on the treatment of AFF have not been established, clinical trials have suggested that optimal AFF treatment includes surgical (intramedullary nailing and plate) and medical therapy. Medical management of AFF was summarized in the original task force report (American Society for Bone and Mineral Research, ASBMR) in 2010 and updated in the second task force report in 2014 (13, 14). In addition to cessation of BPs and supplementation of adequate calcium and vitamin D, a parathyroid hormone analogue termed teriparatide (TPTD) is likely to promote fracture healing. TPTD, the only US FDA-approved anabolic bone agent, has been reported by numerous studies to stimulate bone formation and remodeling, thus accelerating typical fracture healing (15, 16). The application of TPTD in the treatment of AFFs has also been reported. Several descriptive analyses have revealed TPTD could improve osteal micro-architecture and reduce bone non-union, exerting mechanisms of action opposite to BPs (17–19). Although a number of animal and clinical studies have reported beneficial effects of TPTD on AFFs associated with long-term BP therapy (20–23), lacking of integrated, effective treatment guidelines for the management of BP-associated AFF remains an orthopedic dilemma. Here, we briefly define AFF as well as detail the pathogenic mechanisms of AFFs in the setting of long-term BP therapy, the role of TPTD in AFF management and the latest therapeutic advances in order to provide more effective guidelines for successful clinical AFF management.
Methods
A search of PUBMED, EMBASE, and the Cochrane Central Register of Controlled Trials databases was conducted from 2001 to 2021. The search strategy was developed using MeSH terms and keywords associated with terms relevant to “Atypical femur fracture”, “Atypical femur fractures”, “femur fracture”, “femur fractures”, “Subtrochanteric fractures”, “Subtrochanteric fracture”, “Bisphosphonate”, “Bisphosphonates”, “BPS”, “BP”, “Teriparatide”, “Teriparatides”, “TPTD” and “TPTDS” in various combinations. A total of 704 articles were searched and the results were screened using the following inclusion and exclusion criteria. Inclusion criteria were available for all articles. Exclusion criteria included: (1) Duplicate results were excluded. (2) Editorials, conference abstract and letters were excluded. Two authors independently extracted information from the articles, which they read and reviewed the titles and abstracts and then the full text of the retrieved articles. 82 articles were deemed appropriate for our study. In addition, These included 3 articles from a prospective study, 7 articles from retrospective case series, and 20 case reports. These articles were then analyzed in detail of clinical outcome of TPTD therapy for AFFs (Figure 1).
Results
Definition of AFF
Since the publication of the article suggesting an association between BPs and AFFs in 2005 (24), a growing number of case reports and series have revealed a high correlation between BP therapy and AFFs. Fractures were reported to be primarily located along the femoral diaphysis from just distal to the lesser trochanter to just proximal to the supracondylar flare (Figure 2) (13). These fractures were characterized as spontaneous and resulting from low-energy injury. They were usually not comminuted and fractured in the subtrochanteric area, or the proximal one-third of the femoral shaft. In order to clearly define AFF, the American Society for Bone and Mineral Research (ASBMR) established 5 major and 7 minor features of AFFs in the original 2010 task force report. All major features are required to satisfy the case definition of an AFF; none of the minor features are required, but occasionally some have been associated with such fractures. The 5 major features included: (1) fracture location anywhere along the femur from just distal to the lesser trochanter to just proximal to the supracondylar flare; (2) lack of association with trauma, or association with minimal trauma, as in a fall from a standing height or less; (3) transverse or short oblique fracture configuration; (4) non-comminuted nature; (5) fracture extension through both cortices and association with a medial spike; incomplete fractures involve only the lateral cortex. The 7 minor features included: (1) Localized periosteal reaction of the lateral cortex, often referred to in literature as beaking or flaring; (2) generalized cortical thickness increase of the diaphysis; (3) presence of prodromal symptoms such as dull or aching pain in the groin or thigh; (4) bilateral fractures and symptoms; (5) delayed healing; (6) comorbid conditions (e.g., vitamin D deficiency, RA, hypophosphatasia); (7) use of pharmaceutical agents (e.g., BPs, glucocorticoids, proton pump inhibitors). In order to standardize and improve diagnostic criteria, the definition of AFFs was revised in the second 2014 task force report. The major alterations were the establishment of clearly defined transverse or short oblique AFFs configurations, inclusion of minimal comminuted fractures, and the changing of the periosteal or endosteal lateral cortex stress reaction at the fracture site from minor to major categories(13, 14). Based on it, we classified AFFs into 5 types (Figure 3) according to their characteristics, providing further clarification.
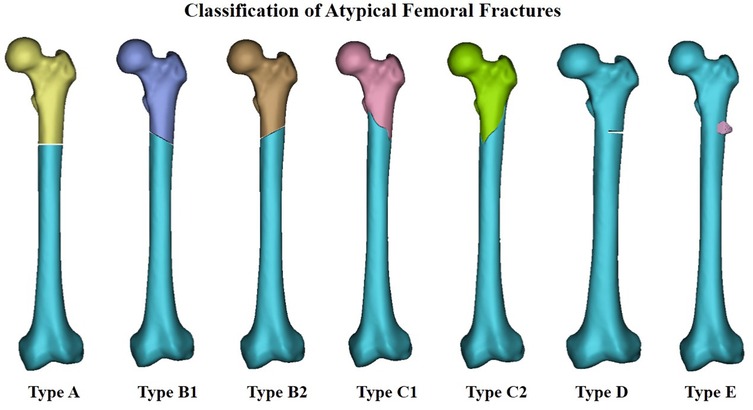
Figure 3. Classification of atypical femoral fractures; Type A implies transverse fracture, Type B implies short oblique fracture (which itself has2 subtypes), Type C implies spiral fracture associated with a medial spike (which itself has 2 subtypes), Type D implies incomplete fractures involving only the lateral cortex, Type E implies localized periosteal reaction in the lateral cortex.
BP influences on AFFs
BP, consisting of P-C-P bonds, not only imbues strong affinity for hydroxyapatite (HAP) binding and in turn prevents both HAP crystal growth and dissolution, but also directly or indirectly inhibits osteoclast-mediated resorption and remodeling of bone (25–28). Via these pathways, BP can prevent bone loss, reduce bone turnover and increase overall mineralization (29), and widely used in the management of osteoprosis, pagets disease of the bone and osteocarcinomas. Throughout treatment, BPs are incorporated into newly formed bone and persist there for long time. In addition, a continuous decrease in chronic bone turnover likely leads to greater bone fragility (30). The research had proved that the risk of atypical femur fracture increased with longer duration of BP use and rapidly decreased after BP discontinuation. In addition, Asians had a higher risk than Whites.
Table 1 details how BP treatment predisposes to AFFs development. Due to the high affinity of BP to HAP binding and long-term effects on bone resorption, a marked decrease in bone remodeling delays prompt micro-fracture healing (31). As micro-fractures accumulate, a greater stress reaction results. This is the reason x-ray imaging reveals these fractures to exhibit focal lateral cortical thickening, or transverse, short oblique fracture lines as the stress reaction develops. Moreover, BPs decrease both crystal formation and dissolution, thereby narrowing crystal distribution without affecting mean crystal size and increasing overall mineralization (32). As bone turnover is reduced, BPs prevent maturation of collagen and increase levels of advanced glycation end products (33). Due to alteration in the properties of the HAP and bone matrix, bone tissue homogenizes and is less able to reduce local stress. This enhances energy distribution that makes the bone more brittle and predisposes to fracture (34). Studies have confirmed BP to directly suppress vasculogenesis and retard the remodeling of calcified cartilage calluses into mature bone during fracture healing (35, 36). AFFs healing is thus typically prolonged and may even result in bone non-union. Appropriate treatment strategies are essential for successful AFFs management, and TPTD, with its unique bone-forming effects, is likely a good choice.
The role of teriparatide in AFFs
TPTD has mainly been used to treat osteoporosis in postmenopausal women and older men since 2002 after its approval by the FDA for this purpose (37). It is manufactured using a genetically modified strain of E. coli and consists of a 1–34 N-terminal amino acid sequence of the intact human parathyroid hormone while maintaining whole parathyroid hormone (PTH) biological activities (38). The current recommended dose is 20 µg once daily by subcutaneous injection in a sustained treatment cycle for no greater than 2 years. Numerous studies have reported TPTD to directly activate osteoblast via binding to their PTH receptors, thus increasing osteoblast genesis and reducing osteoblast apoptosis (39). TPTD increases production of growth factors and cytokines, such as IGF-1 and TGF-β, resulting in pre-osteoblast recruitment from marrow stromal cells (40, 41). TPTD also positively affects cell-signaling pathways, such as IHH, Wnt/β-catenin and AC/cAMP-PKA and promotes osteoblast proliferation and differentiation (42–44). TPTD was furthermore confirmed to reduce sclerostin, an inhibitor of bone formation in both rodents and humans (45, 46). TPTD thus possesses significant advantages in the promotion of bone formation and is considered a good choice for the treatment of fractures (47, 48).
Fractures are understood to undergo primary and secondary healing. Primary healing or direct bone healing is characterized by fractured bone cortex ends directly uniting and results in restoration of anatomy and rigid internal fixation. Secondary healing, or indirect bone healing, is similar to embryonic bone formation and includes intramembranous and endochondral ossification via callus formation. Fractures typically heal via secondary healing which the processes involved are indeed complex (49). A better physiological environment decreases chances of delayed union or non-union occurring. In the setting of osteoporosis, poor osteoblast function and inappropriate callus maturity, coupled with the effects of BPs, result in frequent complications of bone injury. However, TPTD, exerting anabolic effects as opposed to BP (Table 1) has been confirmed to significantly redistribute BPs within fracture sites in some studies, even reduce BP content with prolonged use of this drug (50). Moreover, because of anabolism leading to increased bone turnover and remodeling, TPTD not only replaces fully mineralized bone matrix with newly synthesized and less completely mineralized matrix but also decreases production of glycated collagen while increasing expression of new type II and X collagen (51, 52). Such alterations in mineralized bone and matrix result in preferable osseous heterogeneity. Furthermore, fracture callus size, density and volume become significantly increased (51). Interestingly, intermittent TPTD administration was found to promote osteoblast genesis and decrease adipogenesis at the site of cancellous bone, resulting in better bone union (53). Dobnig et al. (54) found that changes in iliac crest microdamage in previously treatment-naive patients managed with TPTD, or in patients switched from alendronate to TPTD, entailed decreased crack density (Cr.Dn), crack surface density (Cr.S.Dn) and crack length (Cr.Le) when compared to patients previously treated with alendronate after 24 months of TPTD administration. These findings demonstrate that TPTD reduces accumulation of bone microdamage in postmenopausal women previously treated with alendronate. Similarly, Paul et al. (21) studied 15 patients referred to The Colorado Center for Bone Research (CCBR) after they suffered BP-associated AFF using quantitative iliac crest histomorphometry both before and after 12 months of TPTD (20 µg SQ/day). Results revealed that discontinuation of BPs and administration of TPTD was associated with improvement in bone turnover and an increase in all 3 dynamic histomorphometric parameters; including bone formation, mineralized surface, and mineral apposition. Although a number of studies have verified the beneficial effects of TPTD on AFF healing, the mechanism of TPTD action in the setting of AFFs remains unknown. Based on prior research, TPTD has unique potential in the treatment of AFFs. Also a large number of clinical evidence has additionally found that TPTD therapy can be considered as an alternative treatment for AFF.
Clinical outcome of TPTD therapy for AFFs
The management of BP-associated AFFs varies due to AFF type (i.e., stress reaction; stress fracture; incomplete or complete subtrochanteric or femoral shaft fracture). On account of high rates of poor union and surgical difficulties, neither conservative nor operative management are capable of achieving good treatment outcomes. TPTD supplementation, however, reducing the time to bone union and likelihood of non-union, positively affects AFF healing (55, 56). Several case reports as well as retrospective (Table 2) and prospective studies (Table 3) have demonstrated the efficacy of TPTD in the treatment of AFFs (17–19, 21, 57–82).
Gomberg et al. (68) initially reported TPTD use in AFF management. The patient was a postmenopausal woman treated with a BP for 13 years. Obvious reduction of edema, pain and appearance of faint cortical bridging at fracture sites after 6 months of continuous TPTD treatment was noted. Then, a number of case reports or series subsequently found TPTD use for AFF treatments could reduce periosteal reaction and promote microdamage repair in the setting of conservative or surgical treatment. In addition to pain reduction and minimization of dysfunction, alkaline phosphatase levels in AFF patients who suffered hypophosphatasia were effectively restored with TPTD administration (74). Treatment of TPTD is usually started for diagnosis or after surgery. The dose is usually 20 µg/d and the treatment cycle ranges from 3 to 24 months. Calcium and vitamin D were often used in combination. It is worth emphasizing that TPTD combined with stable fixation results in satisfactory AFF outcomes in most cases. Almost all patients had clinical outcomes of bone healing, and the healing time was between 1 and 6 months.
Saleh et al. (57) first retrospectively investigated 10 patients that suffered a total of 14 incomplete AFFs. 5 of 14 fractures did not manifest with a radiolucent fracture line and were healed conservatively with TPTD. Radiolucent fracture lines across thickened cortices were noted in 9 fractures. Two of these fractures responded to 3 months of conservative therapy with TPTD and were found to have completely healed on radiography. Six of these fractures chose surgical prophylaxis after 3 months of conservative management, whereas 1 patient underwent surgical prophylaxis without a trial of conservative management. Miyakoshi et al. (58) retrospectively reviewed the medical records of 45 consecutive AFFs in 34 Japanese patients who had been treated with oral BPs for osteoporosis prior to their AFFs and followed for a period of 12 months. A total of 37 complete or incomplete AFFs were treated surgically and 8 incomplete AFFs were treated conservatively. Patients were non-randomly divided into non-TPTD (n = 24) and TPTD (n = 21) groups. Results revealed the healing time of all surgically-treated AFFs to have been significantly shorter in the TPTD group and the frequency of delayed healing or non-union to have been significantly lower in the TPTD group as well. Although similar findings were noted in surgically treated complete AFFs, sub-analyses of incomplete AFFs treated conservatively revealed no significant differences between groups. Hence, TPTD was found to markedly accelerate fracture healing postoperatively that would otherwise have been delayed. Chiang et al.'s (63) prospective study involved 14 patients that consecutively presented with AFFs over a course of 2 years. Of these, 5 were offered TPTD therapy while the remaining 9 were not due to contraindications. The 5 patients who had undergone TPTD therapy were found to have a 2–3 folds increase in bone remodeling markers and more rapid fracture healing. Of the 9 patients managed conservatively or surgically, 7 suffered poor fracture healing with ongoing pain, 1 sustained a contralateral atypical fracture and 1 experienced fracture union only after 1 year. Similar results were reported in Yeh's (60) study, 13 patients who suffered AFFs were all treated with intramedullary fixation, 6 patients underwent TPTD treatment initially for at least 6 months while others did not. The mean time to bone union was 4.4 months in the TPTD treatment group and 6.2 months in the non-TPTD treatment group. The means of the modified Harris Hip Score and Numerical Rating Scale were significantly better in the TPTD group at 6 months postoperatively. TPTD was thus confirmed to greatly assist in fracture healing, hip function recovery, and pain relief. A randomized pilot clinical trial confirmed immediate therapy with TPTD to be superior for fracture healing in the setting of AFFs compared to a 6-month delay in TPTD therapy and effectively prevented a decrease in bone density (65).
Although TPTD was found to initially promote rapid healing of AFF, the development of new, contralateral AFFs highlights that TPTD does not indefinitely protect against future AFF occurrence. TPTD may thus be limited in its ability to reverse skeletal side effects of long term BP therapy. Anti-resorptive therapies have also been implicated in the development of contralateral AFFs in the setting of adjunctive strontium ranelate therapy (77). Moreover, Watts et al. (64) conducted a prospective and open-label study involving 14 AFF patients who were previously treated with BPs, examining changes in bone mineral density, trabecular bone score, bone turnover markers, and fracture healing, as well as quantitative histomorphometry, after a 24-month TPTD treatment period. At 24 months, 6 patients were found to have healed fractures, 3 to have partially healed fractures, 2 with unchanged fractures and 1 with nonunion. However, in a patient who suffered 2 successive fractures, the fracture that occurred prior to TPTD treatment was reported as healed while the fracture that occurred during TPTD treatment was found to be only partially healed. No significant effects of TPTD on hip bone mineral density, mineralizing surface to bone surface ratio, or trabecular bone score were noted. In addition, no consistent effect on fracture healing was noted, implying that sole use of TPTD is likely not reliable for rapid healing of AFFs. TPTD should, however, be understood as exerting beneficial effects on AFF healing. A greater amount of high level of evidence, in particular randomized controlled trials, should be performed to further confirm the efficacy of TPTD in AFF management.
Discussion
Long-term application of BPs, affecting bone mass and resulting in AFF, made the treatment of AFFs difficult. Due to its unique biological effects, TPTD has been demonstrated in basic experiments to reverse the effect of BP on bone and promote the healing of AFFs, and has achieved good results in clinical application. Based on the articles we reviewed, it is not difficult to find that TPTD has certain advantages in promoting the healing of complete or incomplete AFFs, especially in combination with strong internal fixation and medical supplement with calcium, cholecalciferol and vitamin D.
Based on the existing clinical evidences, especially the prospective and RCT studies, it provides certain clinical basis for the TPTD treatment of AFFs. Hence, we have developed a preliminary diagnosis and treatment process based on the latest clinical findings to assist AFF treatment. Firstly, regular imaging examinations (MRI is recommended) are recommended for patients who have been using BPs for a long time (especially those who have been using BPs for more than 5 years), regardless of whether they have symptoms of thigh pain, for the purpose of early detection and treatment. Secondly, BPs therapy should be stopped immediately after AFF diagnosis, and conservative or surgical treatment should be given according to fracture type. Thirdly, it is recommended to apply TPTD (20 µg/day, 12–24 months) immediately after diagnosis for patients receiving conservative treatment and patients receiving surgery, and calcium, cholecalciferol and vitamin D should be combined. Fourthly, surgical intervention is recommended as soon as possible for patients with ineffective conservative treatment, and preventive surgical treatment is also recommended for patients with initial symptoms or incomplete fractures. Fifth, regular monitoring of blood calcium after the application of TPTD is recommended, and the treatment cycle depends on the time of fracture healing condition, and it is recommended that the application should not exceed 24 months. Replacement of other drugs such as Denosumab is recommended after the cessation of TPTD treatment. We hope that AFFs can be easily treated through the above treatment process.
But so far, even though AFF has been studied a lot, it still has some shortcomings. In general, the mechanism by which TPTD promotes AFF healing is unclear. In addition, we found that most of the current clinical studies were case reports and retrospective studies with low level of evidence. The specific time of TPTD initiation, dose and cycle of application, and the difference between conservative and surgical treatment were not clear. Therefore, more high-level clinical studies are needed to confirm the efficacy of TPTD in treating AFFs.
Conclusion
BP-associated AFFs present numerous difficulties to both clinicians and patients. An effective treatment is thus urgently needed. Basic and clinical research has revealed TPTD to exert a unique anabolic effect in AFF healing and underscores its importance as an alternative treatment for this condition. Further research, however, is required for the establishment of effective clinical guidelines of TPTD use in the management of AFFs.
Author contributions
JG initial concept, data collection and analysis, initial draft and edited the paper. XL data collection and analysis, initial draft and edited the paper. XW participated in data collection and analysis. XL participated in data collection and analysis. JL helped to draft the manuscript, reviewed and edited the paper. ML helped to draft the manuscript, reviewed and edited the paper. All authors contributed to the article and approved the submitted version.
Funding
This work was supported by grants from the PLA Major Project (AWS17J004), the National Natural Science Foundation of China (82002317), Sub-topic of Key Basic Research Project of Army Basic Strengthening Program (2020-JCJQ-ZD-264-*) and Military Training Injury Prevention and Treatment Project (20XLS30).
Conflict of interest
The authors declare that the research was conducted in the absence of any commercial or financial relationships that could be construed as a potential conflict of interest.
Publisher's note
All claims expressed in this article are solely those of the authors and do not necessarily represent those of their affiliated organizations, or those of the publisher, the editors and the reviewers. Any product that may be evaluated in this article, or claim that may be made by its manufacturer, is not guaranteed or endorsed by the publisher.
References
1. Black DM, Delmas PD, Eastell R, Reid IR, Boonen S, Cauley JA, et al. Once-yearly zoledronic acid for treatment of postmenopausal osteoporosis. N Engl J Med. (2007) 356:1809–22. doi: 10.1056/NEJMoa067312
2. Reinsdorf S, Habermann B, Hochmuth K, Kurth AA. Bisphosphonates in osteoporosis therapy. Standards and perspectives. Orthopade. (2007) 36:110–7. doi: 10.1007/s00132-006-1035-6
3. Capeci CM, Tejwani NC. Bilateral low-energy simultaneous or sequential femoral fractures in patients on long-term alendronate therapy. J Bone Joint Surg Am. (2009) 91:2556–61. doi: 10.2106/jbjs.h.01774
4. Park-Wyllie LY, Mamdani MM, Juurlink DN, Hawker GA, Gunraj N, Austin PC, et al. Bisphosphonate use and the risk of subtrochanteric or femoral shaft fractures in older women. JAMA. (2011) 305:783–9. doi: 10.1001/jama.2011.190
5. Geada N, Mafra I, Barroso R, Franco J. Atypical femoral fractures and bisphosphonates treatment: is it a risk factor. Acta Med Port. (2014) 27:704–9. doi: 10.20344/amp.5118
6. Schilcher J, Koeppen V, Aspenberg P, Michaëlsson K. Risk of atypical femoral fracture during and after bisphosphonate use. N Engl J Med. (2014) 371:974–6. doi: 10.1056/NEJMc1403799
7. Abrahamsen B. Older women who use bisphosphonate for longer than 5 years may have increased odds of a subtrochanteric or femoral shaft fracture, but absolute risk is low. Evid Based Med. (2011) 16:168–9. doi: 10.1136/ebm.2011.000013
8. Weil YA, Rivkin G, Safran O, Liebergall M, Foldes AJ. The outcome of surgically treated femur fractures associated with long-term bisphosphonate use. J Trauma. (2011) 71:186–90. doi: 10.1097/TA.0b013e31821957e3
9. Egol KA, Park JH, Rosenberg ZS, Peck V, Tejwani NC. Healing delayed but generally reliable after bisphosphonate-associated complete femur fractures treated with IM nails. Clin Orthop Relat Res. (2014) 472:2728–34. doi: 10.1007/s11999-013-2963-1
10. Chun-Man M, Man-Hong C, Wah-Bong W. Surgical difficulties and complications in the treatment of bisphosphonate-related proximal femur fractures. J Orthop. (2015) 19:83–8. doi: 10.1016/j.jotr.2014.06.002
11. Koh A, Guerado E, Giannoudis PV. Atypical femoral fractures related to bisphosphonate treatment: issues and controversies related to their surgical management. Bone Joint J. (2017) 99-b:295–302. doi: 10.1302/0301-620x.99b3.bjj-2016-0276.r2
12. Khow KS, Shibu P, Yu SC, Chehade MJ, Visvanathan R. Epidemiology and postoperative outcomes of atypical femoral fractures in older adults: a systematic review. J Nutr Health Aging. (2017) 21:83–91. doi: 10.1007/s12603-015-0652-3
13. Shane E, Burr D, Ebeling PR, Abrahamsen B, Adler RA, Brown TD, et al. Atypical subtrochanteric and diaphyseal femoral fractures: report of a task force of the American Society for Bone and Mineral Research. J Bone Miner Res. (2010) 25:2267–94. doi: 10.1002/jbmr.253
14. Shane E, Burr D, Abrahamsen B, Adler RA, Brown TD, Cheung AM, et al. Atypical subtrochanteric and diaphyseal femoral fractures: second report of a task force of the American Society for Bone and Mineral Research. J Bone Miner Res. (2014) 29:1–23. doi: 10.1002/jbmr.1998
15. Haas AV, LeBoff MS. Osteoanabolic agents for osteoporosis. J Endocr Soc. (2018) 2:922–32. doi: 10.1210/js.2018-00118
16. Anagnostis P, Gkekas NK, Potoupnis M, Kenanidis E, Tsiridis E, Goulis DG. New therapeutic targets for osteoporosis. Maturitas. (2019) 120:1–6. doi: 10.1016/j.maturitas.2018.11.010
17. Carvalho NN, Voss LA, Almeida MO, Salgado CL, Bandeira F. Atypical femoral fractures during prolonged use of bisphosphonates: short-term responses to strontium ranelate and teriparatide. J Clin Endocrinol Metab. (2011) 96:2675–80. doi: 10.1210/jc.2011-0593
18. Fukuda F, Kurinomaru N, Hijioka A. Weekly teriparatide for delayed unions of atypical subtrochanteric femur fractures. Biol Ther. (2014) 4:73–9. doi: 10.1007/s13554-014-0013-5
19. Mastaglia SR, Aguilar G, Oliveri B. Teriparatide for the rapid resolution of delayed healing of atypical fractures associated with long-term bisphosphonate use. Eur J Rheumatol. (2016) 3:87–90. doi: 10.5152/eurjrheum.2015.0010
20. Gopal GK, Tam KL, Krishnan SP, Maddern IL. Bisphosphonate-associated atypical subtrochanteric femur fractures in the older patient. N Z Med J. (2014) 127:81–5.24548959
21. Miller PD, McCarthy EF. Bisphosphonate-associated atypical sub-trochanteric femur fractures: paired bone biopsy quantitative histomorphometry before and after teriparatide administration. Semin Arthritis Rheum. (2015) 44:477–82. doi: 10.1016/j.semarthrit.2014.09.005
22. Collinge C, Favela J. Use of teriparatide in osteoporotic fracture patients. Injury. (2016) 47(Suppl 1):S36–8. doi: 10.1016/s0020-1383(16)30009-2
23. Kim JT, Jeong HJ, Lee SJ, Kim HJ, Yoo JJ. Adjuvant teriparatide therapy for surgical treatment of femoral fractures; does it work. Hip Pelvis. (2016) 28:148–56. doi: 10.5371/hp.2016.28.3.148
24. Odvina CV, Zerwekh JE, Rao DS, Maalouf N, Gottschalk FA, Pak CY. Severely suppressed bone turnover: a potential complication of alendronate therapy. J Clin Endocrinol Metab. (2005) 90:1294–301. doi: 10.1210/jc.2004-0952
25. Frith JC, Mönkkönen J, Auriola S, Mönkkönen H, Rogers MJ. The molecular mechanism of action of the antiresorptive and antiinflammatory drug clodronate: evidence for the formation in vivo of a metabolite that inhibits bone resorption and causes osteoclast and macrophage apoptosis. Arthritis Rheum. (2001) 44:2201–10. doi: 10.1002/1529-0131(200109)44:9%3C2201::aid-art374%3E3.0.co;2-e
26. Lawson MA, Xia Z, Barnett BL, Triffitt JT, Phipps RJ, Dunford JE, et al. Differences between bisphosphonates in binding affinities for hydroxyapatite. J Biomed Mater Res B Appl Biomater. (2010) 92:149–55. doi: 10.1002/jbm.b.31500
27. Nancollas GH, Tang R, Phipps RJ, Henneman Z, Gulde S, Wu W, et al. Novel insights into actions of bisphosphonates on bone: differences in interactions with hydroxyapatite. Bone. (2006) 38:617–27. doi: 10.1016/j.bone.2005.05.003
28. Rogers MJ, Crockett JC, Coxon FP, Mönkkönen J. Biochemical and molecular mechanisms of action of bisphosphonates. Bone. (2011) 49:34–41. doi: 10.1016/j.bone.2010.11.008
29. Fratzl P, Roschger P, Fratzl-Zelman N, Paschalis EP, Phipps R, Klaushofer K. Evidence that treatment with risedronate in women with postmenopausal osteoporosis affects bone mineralization and bone volume. Calcif Tissue Int. (2007) 81:73–80. doi: 10.1007/s00223-007-9039-8
30. Durchschlag E, Paschalis EP, Zoehrer R, Roschger P, Fratzl P, Recker R, et al. Bone material properties in trabecular bone from human iliac crest biopsies after 3- and 5-year treatment with risedronate. J Bone Miner Res. (2006) 21:1581–90. doi: 10.1359/jbmr.060701
31. Karim L, Vashishth D. Role of trabecular microarchitecture in the formation, accumulation, and morphology of microdamage in human cancellous bone. J Orthop Res. (2011) 29:1739–44. doi: 10.1002/jor.21448
32. Adele B. Bone mineral crystal size. Osteoporos Int. (2003) 14(Suppl 5):S16–S20; discussion S20–1. doi: 10.1007/s00198-003-1468-2
33. Saito M, Marumo K. [Roles of collagen enzymatic and advanced glycation end products associated crosslinking as a determinant of bone quality]. Nihon Rinsho. (2011) 69:1189–97.21774356
34. Donnelly E, Meredith DS, Nguyen JT, Gladnick BP, Rebolledo BJ, Shaffer AD, et al. Reduced cortical bone compositional heterogeneity with bisphosphonate treatment in postmenopausal women with intertrochanteric and subtrochanteric fractures. J Bone Miner Res. (2012) 27:672–8. doi: 10.1002/jbmr.560
35. Einhorn TA. Can an anti-fracture agent heal fractures? Clin Cases Miner Bone Metab. (2010) 7:11–4.22461283
36. Ziebart T, Pabst A, Klein MO, Kämmerer P, Gauss L, Brüllmann D, et al. Bisphosphonates: restrictions for vasculogenesis and angiogenesis: inhibition of cell function of endothelial progenitor cells and mature endothelial cells in vitro. Clin Oral Investig. (2011) 15:105–11. doi: 10.1007/s00784-009-0365-2
37. Neer RM, Arnaud CD, Zanchetta JR, Prince R, Gaich GA, Reginster JY, et al. Effect of parathyroid hormone (1-34) on fractures and bone mineral density in postmenopausal women with osteoporosis. N Engl J Med. (2001) 344:1434–41. doi: 10.1056/nejm200105103441904
38. Kraenzlin ME, Meier C. Parathyroid hormone analogues in the treatment of osteoporosis. Nat Rev Endocrinol. (2011) 7:647–56. doi: 10.1038/nrendo.2011.108
39. Hanley DA, Watson PH, Hodsman AB, Dempster DW. Chapter 78 - pharmacological mechanisms of therapeutics: parathyroid hormone A2. In: Bilezikian JP, Raisz LG, Martin TJ, editors. Principles of bone biology. 3rd ed. Amsterdam, London: Elsevier (2008). p. 1659–95. doi: 10.1016/B978-0-12-373884-4.00085-9
40. Hock JM, Gera I. Effects of continuous and intermittent administration and inhibition of resorption on the anabolic response of bone to parathyroid hormone. J Bone Miner Res. (1992) 7:65–72. doi: 10.1002/jbmr.5650070110
41. Papapoulos SE. Anabolic bone therapies in 2014: new bone-forming treatments for osteoporosis. Nat Rev Endocrinol. (2015) 11:69–70. doi: 10.1038/nrendo.2014.214
42. Friedl G, Turner RT, Evans GL, Dobnig H. Intermittent parathyroid hormone (PTH) treatment and age-dependent effects on rat cancellous bone and mineral metabolism. J Orthop Res. (2007) 25:1454–64. doi: 10.1002/jor.20433
43. Canalis E. Update in new anabolic therapies for osteoporosis. J Clin Endocrinol Metab. (2010) 95:1496–504. doi: 10.1210/jc.2009-2677
44. Esbrit P, Alcaraz MJ. Current perspectives on parathyroid hormone (PTH) and PTH-related protein (PTHrP) as bone anabolic therapies. Biochem Pharmacol. (2013) 85:1417–23. doi: 10.1016/j.bcp.2013.03.002
45. Canalis E, Giustina A, Bilezikian JP. Mechanisms of anabolic therapies for osteoporosis. N Engl J Med. (2007) 357:905–16. doi: 10.1056/NEJMra067395
46. Jilka RL. Molecular and cellular mechanisms of the anabolic effect of intermittent PTH. Bone. (2007) 40:1434–46. doi: 10.1016/j.bone.2007.03.017
47. Choi HS, Park SY, Kim YM, Kim SH, Kim KM, Chung YS. Medical treatment of severe osteoporosis including new concept of advanced severe osteoporosis. Osteoporos Sarcopenia. (2016) 2:13–9. doi: 10.1016/j.afos.2016.02.003
48. Huang TW, Chuang PY, Lin SJ, Lee CY, Huang KC, Shih HN, et al. Teriparatide improves fracture healing and early functional recovery in treatment of osteoporotic intertrochanteric fractures. Medicine (Baltimore). (2016) 95:e3626. doi: 10.1097/md.0000000000003626
49. Webb JCJ, Tricker J. A review of fracture healing. Curr Orthop. (2000) 14:457–63. doi: 10.1054/cuor.2000.0145
50. Murphy CM, Schindeler A, Cantrill LC, Mikulec K, Peacock L, Little DG. PTH(1-34) treatment increases bisphosphonate turnover in fracture repair in rats. J Bone Miner Res. (2015) 30:1022–9. doi: 10.1002/jbmr.2424
51. Kakar S, Einhorn TA, Vora S, Miara LJ, Hon G, Wigner NA, et al. Enhanced chondrogenesis and Wnt signaling in PTH-treated fractures. J Bone Miner Res. (2007) 22:1903–12. doi: 10.1359/jbmr.070724
52. Jobke B, Muche B, Burghardt AJ, Semler J, Link TM, Majumdar S. Teriparatide in bisphosphonate-resistant osteoporosis: microarchitectural changes and clinical results after 6 and 18 months. Calcif Tissue Int. (2011) 89:130–9. doi: 10.1007/s00223-011-9500-6
53. Nozaka K, Miyakoshi N, Kasukawa Y, Maekawa S, Noguchi H, Shimada Y. Intermittent administration of human parathyroid hormone enhances bone formation and union at the site of cancellous bone osteotomy in normal and ovariectomized rats. Bone. (2008) 42:90–7. doi: 10.1016/j.bone.2007.08.041
54. Dobnig H, Stepan JJ, Burr DB, Li J, Michalská D, Sipos A, et al. Teriparatide reduces bone microdamage accumulation in postmenopausal women previously treated with alendronate. J Bone Miner Res. (2009) 24:1998–2006. doi: 10.1359/jbmr.090527
55. Pietrogrande L, Raimondo E. Teriparatide in the treatment of non-unions: scientific and clinical evidences. Injury. (2013) 44(Suppl 1):S54–7. doi: 10.1016/s0020-1383(13)70013-5
56. Borges JL, Freitas A, Bilezikian JP. Accelerated fracture healing with teriparatide. Arq Bras Endocrinol Metabol. (2013) 57:153–6. doi: 10.1590/s0004-27302013000200010
57. Saleh A, Hegde VV, Potty AG, Schneider R, Cornell CN, Lane JM. Management strategy for symptomatic bisphosphonate-associated incomplete atypical femoral fractures. Hss j. (2012) 8:103–10. doi: 10.1007/s11420-012-9275-y
58. Miyakoshi N, Aizawa T, Sasaki S, Ando S, Maekawa S, Aonuma H, et al. Healing of bisphosphonate-associated atypical femoral fractures in patients with osteoporosis: a comparison between treatment with and without teriparatide. J Bone Miner Metab. (2015) 33:553–9. doi: 10.1007/s00774-014-0617-3
59. Alwahhabi BK, Alsuwaine BA. Long-term use of bisphosphonates in osteoporosis. Saudi Med J. (2017) 38:604–8. doi: 10.15537/smj.2017.6.19793
60. Yeh WL, Su CY, Chang CW, Chen CH, Fu TS, Chen LH, et al. Surgical outcome of atypical subtrochanteric and femoral fracture related to bisphosphonates use in osteoporotic patients with or without teriparatide treatment. BMC Musculoskelet Disord. (2017) 18:527. doi: 10.1186/s12891-017-1878-5
61. Tsuchie H, Miyakoshi N, Iba K, Kasukawa Y, Nozaka K, Dohke T, et al. The effects of teriparatide on acceleration of bone healing following atypical femoral fracture: comparison between daily and weekly administration. Osteoporos Int. (2018) 29:2659–65. doi: 10.1007/s00198-018-4658-7
62. Tsuchie H, Miyakoshi N, Kasukawa Y, Nozaka K, Saito K, Kinoshita H, et al. Evaluation of factors affecting the occurrence of second atypical fracture after bone union of the first atypical fracture. Bone. (2021) 143:115671. doi: 10.1016/j.bone.2020.115671
63. Chiang CY, Zebaze RM, Ghasem-Zadeh A, Iuliano-Burns S, Hardidge A, Seeman E. Teriparatide improves bone quality and healing of atypical femoral fractures associated with bisphosphonate therapy. Bone. (2013) 52:360–5. doi: 10.1016/j.bone.2012.10.006
64. Watts NB, Aggers D, McCarthy EF, Savage T, Martinez S, Patterson R, et al. Responses to treatment with teriparatide in patients with atypical femur fractures previously treated with bisphosphonates. J Bone Miner Res. (2017) 32:1027–33. doi: 10.1002/jbmr.3081
65. Greenspan SL, Vujevich K, Britton C, Herradura A, Gruen G, Tarkin I, et al. Teriparatide for treatment of patients with bisphosphonate-associated atypical fracture of the femur. Osteoporos Int. (2018) 29:501–6. doi: 10.1007/s00198-017-4286-7
66. Huang HT, Kang L, Huang PJ, Fu YC, Lin SY, Hsieh CH, et al. Successful teriparatide treatment of atypical fracture after long-term use of alendronate without surgical procedure in a postmenopausal woman: a case report. Menopause. (2012) 19:1360–3. doi: 10.1097/gme.0b013e318260143d
67. Holm J, Eiken P, Hyldstrup L, Jensen JE. Atypical femoral fracture in an osteogenesis imperfecta patient successfully treated with teriparatide. Endocr Pract. (2014) 20:e187–90. doi: 10.4158/ep14141.cr
68. Gomberg SJ, Wustrack RL, Napoli N, Arnaud CD, Black DM. Teriparatide, vitamin D, and calcium healed bilateral subtrochanteric stress fractures in a postmenopausal woman with a 13-year history of continuous alendronate therapy. J Clin Endocrinol Metab. (2011) 96:1627–32. doi: 10.1210/jc.2010-2520
69. Zhang HY, Weng HL, Li M, Zhang J. Different surgical outcomes in a patient with bilateral atypical femoral fracture related to bisphosphonate use with or without teriparatide treatment. Osteoporos Int. (2019) 30:2349–54. doi: 10.1007/s00198-019-05131-2
70. Uppin R, Gupta S, Prakash S. A case report of bisphosphonate-induced bilateral osteoporotic subtrochanteric fracture femurii: review of literature. J Orthop Case Rep. (2016) 6:31–4. doi: 10.13107/jocr.2250-0685.558
71. Tsuchie H, Miyakoshi N, Nishi T, Abe H, Segawa T, Shimada Y. Combined effect of a locking plate and teriparatide for incomplete atypical femoral fracture: two case reports of curved femurs. Case Rep Orthop. (2015) 2015:213614. doi: 10.1155/2015/213614
72. Tarazona-Santabalbina FJ, Aguilella-Fernández L. Bisphosphonate long-term treatment related bilateral subtrochanteric femoral fracture. Can teriparatide be useful? Aging Clin Exp Res. (2013) 25:605–9. doi: 10.1007/s40520-013-0137-3
73. Tan J, Sano H, Poole K. Antiresorptive-associated spontaneous fractures of both tibiae, followed by an atypical femur fracture during the sequential treatment with alendronate, denosumab then teriparatide. BMJ Case Rep. (2019) 12:e229366. doi: 10.1136/bcr-2019-229366
74. Righetti M, Wach J, Desmarchelier R, Coury F. Teriparatide treatment in an adult patient with hypophosphatasia exposed to bisphosphonate and revealed by bilateral atypical fractures. Joint Bone Spine. (2018) 85:365–7. doi: 10.1016/j.jbspin.2017.12.001
75. Ramchand SK, Chiang CY, Zebaze RM, Seeman E. Recurrence of bilateral atypical femoral fractures associated with the sequential use of teriparatide and denosumab: a case report. Osteoporos Int. (2016) 27:821–5. doi: 10.1007/s00198-015-3354-0
76. Ramani A, Wagner R. Periprosthetic femoral stress fracture after bisphosphonate treatment resulting in nonunion successfully treated with teriparatide: a case report. JBJS Case Connect. (2016) 6:e37. doi: 10.2106/jbjs.cc.15.00172
77. Nguyen HH, Milat F, Ebeling PR. A new contralateral atypical femoral fracture despite sequential therapy with teriparatide and strontium ranelate. Bone Rep. (2017) 6:34–7. doi: 10.1016/j.bonr.2017.01.002
78. Lampropoulou-Adamidou K, Tournis S, Balanika A, Antoniou I, Stathopoulos IP, Baltas C, et al. Sequential treatment with teriparatide and strontium ranelate in a postmenopausal woman with atypical femoral fractures after long-term bisphosphonate administration. Hormones (Athens). (2013) 12:591–7. doi: 10.14310/horm.2002.1448
79. Blum L, Cummings K, Goulet JA, Perdue AM, Mauffrey C, Hake ME. Atypical femur fractures in patients receiving bisphosphonate therapy: etiology and management. Eur J Orthop Surg Traumatol. (2016) 26:371–7. doi: 10.1007/s00590-016-1742-6
80. Asano Y, Tajiri K, Yagishita S, Nakanishi H, Ishii T. Bilateral atypical ulnar fractures occurring after long-term treatment with bisphosphonate for 7 years and with teriparatide for 2 years: a case report. Osteoporos Int. (2020) 31:2473–6. doi: 10.1007/s00198-020-05618-3
81. Takahashi Y, Hatashita S, Shinden Y, Ito M, Kaneuchi Y, Hakozaki M, et al. Periprosthetic fracture resembling atypical femoral fracture after fixation with retrograde intramedullary nail in elderly women: a report of two cases. In Vivo. (2021) 35:1837–42. doi: 10.21873/invivo.12445
Keywords: teriparatide, atypical femoral fractures, bisphosphonate, subtrochanteric fracture, therapy
Citation: Gao J, Liu X, Wu X, Li X, Liu J and Li M (2023) A brief review and clinical evidences of teriparatide therapy for atypical femoral fractures associated with long-term bisphosphonate treatment. Front. Surg. 9:1063170. doi: 10.3389/fsurg.2022.1063170
Received: 6 October 2022; Accepted: 7 November 2022;
Published: 6 January 2023.
Edited by:
Paphon Sa-ngasoongsong, Mahidol University, ThailandReviewed by:
Junlin Zhou, Capital Medical University, ChinaFei Wang, Third Hospital of Hebei Medical University, China
© 2023 Gao, Liu, Wu, Li, Liu and Li. This is an open-access article distributed under the terms of the Creative Commons Attribution License (CC BY). The use, distribution or reproduction in other forums is permitted, provided the original author(s) and the copyright owner(s) are credited and that the original publication in this journal is cited, in accordance with accepted academic practice. No use, distribution or reproduction is permitted which does not comply with these terms.
*Correspondence: Ming Li bGltaW5nODkxMjE1QDE2My5jb20= Jianheng Liu amlhbmhlbmdsaXVAMTI2LmNvbQ==
†These authors share first authorship
Specialty Section: This article was submitted to Orthopedic Surgery, a section of the journal Frontiers in Surgery