- Spine Center, Xin Hua Hospital, Affiliated to Shanghai Jiao Tong University School of Medicine, Shanghai, China
Mucopolysaccharidosis (MPS) is a progressive genetic disease that causes a deficiency in lysosomal enzymes, which play an important role in the degradation pathway of glycosaminoglycans. As a result of enzyme defects, mucopolysaccharides cannot be metabolized and thus accumulate. The cervical spine is one of the most commonly involved sites; thus, prompt surgical management before the onset of severe neurological deterioration is critical. However, because of the rarity of the disease, there is no standard treatment. In this review, we characterize the cervical spinal involvement in pediatric patients with MPS, describe the useful imaging technologies for diagnosis, and provide screening procedure for children with MPS. Surgical managements, including indications, surgical methods, possible difficulties, and solutions, are reviewed in detail.
Introduction
Mucopolysaccharidoses (MPSs) are a group of hereditary lysosomal storage diseases caused by a deficiency of an enzyme that plays an important role in the degradation pathway of glycosaminoglycans (GAGs). Except for Hunter syndrome (MPS type II), which has an X-linked recessive inheritance, all MPSs have autosomal recessive inheritance (1). The lack of enzymes leads to the storage of corresponding GAGs, which are considered to be the primary and direct cause of MPS (1). MPSs can be classified into seven types according to the type of the enzyme deficiency, and some of them can be further divided into subcategories (Table 1). In total, there are 12 different types and subtypes of MPSs, including the recently described mucopolysaccharidosis-plus syndrome (MPSPS). In MPSPS, heparan sulfate is stored, but there is no enzyme deficiency (2).
GAGs accumulating in the cells affect cellular processes such as cell adhesion and signaling, causing multiorgan and severe symptoms such as coarse facial features, cognitive retardation, hepatosplenomegaly, hernias, kyphoscoliosis, and corneal clouding (1). Skeletal involvements are the most common manifestations and have been reported in all subtypes of MPS except in MPS type IX. Most MPS types, especially types I and IV, have cervical spine involvement, presenting as an absence of the odontoid process, atlantoaxial dislocation, spinal canal stenosis and compression, and others. In the study of Remondino et al. (3), 43 of 52 patients with MPS had cervical diseases, and odontoid hypoplasia, along with subsequent atlantoaxial instability, was frequently observed in those patients. If it is not addressed, it will develop into myelopathy, which can be life-threatening (4).
Early diagnosis allows early intervention, thus improving the chances of a better outcome. At present, the diagnosis of MPS is relatively mature, including blood and urinary GAG tests, enzyme assays, and genetic tests (1). The development of imaging technology is of great importance in the diagnosis and preoperative evaluation of cervical involvement. Furthermore, close collaboration between clinicians and radiologists is essential (5). However, for those young patients, how to conduct screening and evaluation is still a problem to be solved.
Treatment of the etiology and the corresponding symptoms of MPS in the spine should be comprehensive, involving multiple disciplines. Etiological treatment mainly refers to the disease-specific treatment of MPS, including enzyme replacement therapy and hematopoietic stem cell transplantation, as well as new approaches, such as gene therapy, substrate reduction therapy, chaperone therapy, and combinations of these strategies. However, as MPS is a progressive disease and the lifespan of patients with MPS has increased with improvements in the medical treatment of MPS (6), and considering that the existing strategies cannot correct the pathological damage that has occurred, especially for bone damage, surgical intervention before the onset of serious consequences has become a last-resort, albeit a very effective, strategy.
Generally, the biggest threat to children with MPS may be damage to the heart and respiratory system. However, with the development of diagnostic and treatment technologies, MPS can be diagnosed and treated effectively at an early stage, which means that cardiopulmonary damage can be effectively controlled and the life expectancy can be prolonged. As existing treatments for bones and cognitive damage have limited effectiveness and cannot effectively prevent the progress of bone damage, and coupled with the prevalence of cervical involvement, early diagnosis of cervical involvement and prompt surgical intervention have become more important.
To improve outcomes in patients with MPS, this review characterizes the cervical spinal involvement and related factors, briefly describes the imaging tools for early diagnosis, provides a screening procedure for children with MPS, and discusses the possible surgical interventions for pediatric patients with MPS with cervical involvements.
Spinal involvements of MPS
Skeletal manifestations have been reported in all subtypes of MPS except in MPS type IX, and spinal manifestations are particularly common in the spectrum of skeletal disease in these patients (4). The degree of severity varies among different subtypes, which may be related to both the type and quantity of the accumulated GAG fragments. Even if they are of the same type, the severity also varies, which is considered to be the result of differences in the exact mutation site among patients (4).
Spinal cord compression in the cranial segment caused by MPS is the most important condition and usually needs surgical intervention. This condition can directly cause neurological damage, which may present as cervical pain, unsteady gait, frequent falls, progressive impairment of autonomous ambulation, and/or acute tetraplegia after even minor trauma (7). The main direct causes of spinal cord compression include atlantoaxial subluxation, thickening of the dura, and hypertrophy of the ligamentum flavum.
Atlantoaxial subluxation is often the direct cause of cervical spinal cord compression, which can occur anteriorly, posteriorly, vertically, laterally, or in combinations (5). A pincer-like effect is caused by atlantoaxial subluxation between the posterior arch of the atlas and the axis, indenting the dorsal aspect of the spinal cord and causing further compression of the cord (5). For patients with MPS, the absence of the odontoid process is common and may be one of the main causes of atlantoaxial joint instability. Cervical spinal cord compression without dens hypoplasia is unusual, and only one such case has been reported (8). An unstable atlantoaxial joint further develops into atlantoaxial subluxation, which leads to spinal cord compression. Combined with relaxed ligaments, which is also common in patients with MPS, the risk of atlantoaxial subluxation greatly increases (4). This unstable situation poses a hidden, yet grave, danger and requires surgical intervention as soon as possible.
In addition, the thickened dura and hypertrophied ligamentum flavum are also important causes of compression of the spinal cord, which may be the result of the accumulation of GAGs. Different from C1-C2 instability, there is no ideal surgical method to address the problem of dura hypertrophy owing to its diffuse nature and important role in accommodating cerebrospinal fluid (CSF) (4).
Imaging techniques for early diagnosis
Skeletal and spinal manifestations are important clinical manifestations of MPS and may even be the first sign of MPS; for example, kyphosis is often the first sign of MPS type IV in early childhood despite a healthy appearance at birth (1, 9). Therefore, radiographic findings are essential for the diagnosis of MPS, especially for spinal diseases. Atlantoaxial dislocation and kyphosis are the most common spinal manifestations in MPS, which can be detected on roentgenography and three-dimensional computed tomography (3D CT) reconstruction. The presence of characteristic vertebral anomalies, such as a “beaked” vertebral body, provide clues for diagnosing MPS (9). Lateral plain-film flexion-extension studies are typically used to detect atlantoaxial instability, subluxation, and dislocation (5, 10), but their usefulness is limited in some patients with basilar invagination or enlarged mastoid processes, as the C1-C2 level is not clearly visible.
Magnetic resonance imaging (MRI) can visualize the spinal cord and spinal canal; thus, it is the most useful technology for determining whether there is a compression of the spinal cord and for monitoring nervous complication of MPS spinal involvement, which, in turn, helps us determine whether surgical intervention is needed (5). Spinal stenosis with loss of CSF flow on MRI suggests spinal cord compression. It is worth noting that the nervous deficit is not as severe as that suggested by imaging; thus, comprehensive consideration is needed to make an accurate judgment (Figure 1).
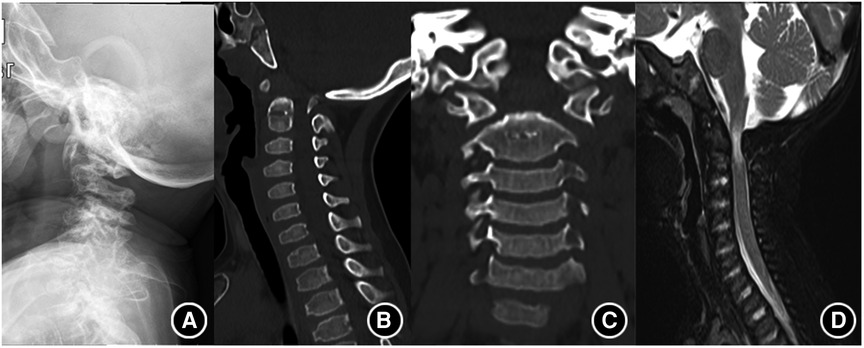
Figure 1. Typical imaging findings of MPS. (A) Shows atlantoaxial dislocation on roentgenography. (B,C) Shows the absence of odontoid process. (D) Shows the compression of the spinal cord.
The development of imaging technology has greatly helped in the diagnosis, but some problems remain. Although CT can clearly show the bony structure, it involves exposure to a large dose of radiation; thus, it should be carried out cautiously in young children. Meanwhile, most young patients find it difficult to tolerate the long examination time and loud noise during MRI examination. The usual practice is to sedate the child, but sedatives have substantial risks that cannot be ignored. To reduce the risk for young patients, we should try to minimize examination times. Anesthetization should be carried out by experienced anesthesiologists.
Owing to the severity and inevitability of cervical spinal cord involvement in patients with MPS, we believe that the following screening procedures are necessary for children with MPS with or without obvious related clinical manifestations, as some patients with MPS (especially type IV) may appear healthy at birth and spinal abnormalities only appear in early childhood (Figure 2). First, cervical anterior, lateral, and dynamic lateral flexion-extension radiographs should be performed routinely to detect cervical deformity and joint instability in the early stage. In view of the potential damage of CT and MRI to young patients, if a child has no positive radiographic findings or related symptoms and a negative neurological examination, further radiological examination is not necessary for the time being, but regular follow-up is needed. If there are positive findings, related clinical symptoms, or neurological signs, furtherMRI examinations are recommended to accurately determine the existence and severity of spinal canal stenosis, and spinal cord compression and to determine whether there are surgical indications. As MRI, including active dynamic flexion and extension scans, is the most useful technique to detect spinal cord compression, it was recommended to be carried out every year (11). CT was suggested to be reserved for children who was considered for surgical procedure (7, 11). If there are surgical indications, CT angiography is also recommended before the operation plan is made to determine the location of blood vessels at the surgical site, which is especially important for patients with developmental malformations. We believe that this screening procedures is helpful for the early diagnosis of cervical lesions in children with MPS, and can provide some reference for spinal surgeons.
Management of spinal involvements
Because of the low incidence of MPS, no unified consensus on surgical indications exists, and applicable literature is scarce (related studies are summarized in Table 2). Although some studies believe that surgical interventions do not halt neurological progression in most preoperatively clinically symptomatic patients, surgical intervention at an early age is still advocated because early intervention before clinical symptoms is of vital importance for long-term neurological preservation (10). With the development of imaging technologies, attempts on a more nuanced approach rely on a combination of clinical examination and radiology, particularly on MRI (5, 11). Unfortunately, there seem to be no convincing research results so far. Based on our analysis and summary of existing literature, combined with our clinical experience, we recommend the following indications for surgical intervention: increasing cervical cord compression in MRI, with or without notable myelopathy; evidence of instability on cervical dynamic lateral flexion and extension radiography; progressive clinical neurological signs with seemingly non-progressive radiological changes (10). For children with definite absence of odontoid process, surgical intervention should also be carried out promptly, because this condition has the potential danger of atlantoaxial dislocation and further nerve damage.
The biggest threat to children with MPS may be damage to the heart and respiratory system, which also means great risk of anesthesia. Therefore, adequate preoperative preparation, strict intraoperative monitoring, and postoperative management are very important. Multidisciplinary consultations should be conducted before operation, including anesthesiology, intensive care medicine, pediatric cardiovascular medicine, pediatric respiratory medicine, metabolic and genetic diseases, and spinal surgeons. The assessment of cardiopulmonary function and risk of anesthesia are particularly important. The intensive care unit should be fully prepared before the operation. At the same time, electrophysiological detection equipment should be prepared for intraoperative monitoring, and 3D printed models should be prepared to facilitate surgeons' accurate understanding of complex structures during operation. Fine-cut 3D reconstructed preoperative studies should be carried out to make the most suitable choice among the different surgical options (7). Anesthesia should be performed by an experienced anesthesiologist, and fiberoptic intubation may be useful, considering the GAG-induced tracheal deformities. A right atrial central venous catheter is placed sometimes, as the risk of dangerous cardiac events is increased in patients with MPS (12). Intensive care should be obligatory, owing to the increased risk of pulmonary insufficiency (12).
In view of the rarity of the disease, the choice of surgery is also controversial. A study published in 2017 advocated that, for patients without craniocervical instability, stand-alone craniocervical decompression is feasible and osteosynthesis is not necessary (12). Decompression surgery without prophylactic osteosynthesis reduces procedure time, iatrogenic trauma, and hospital time. The overall mortality in their case series is lower than that in the applicable literature, but their rate of respiratory-related complication is higher (5). In the study of Krenzlin et al. (12), although the first stand-alone decompression surgery yielded good postoperative results, the reoperation rate was high (60% in type I, 40% in type IVA, and 50% in type VI).
Clinically relevant restenosis, which was believed to be caused by underlying MPS, was the main reason for readmission and re-surgery. GAG deposits in connective tissues and dura mater result in increased rigidity, counteracting the anatomical misalignment and anticipated hypermobility. This balance is the basis for stand-alone craniocervical decompression, but with the development of medical treatment in recent years, the medical treatment for the etiology of MPS can be carried out synchronously with the operation at an early age, the deposition of GAG is slowed down, and the structural damage caused by the surgery cannot be compensated quickly, which leads to increased potential risk of instability. Giussani et al. (7) believed that removal of the posterior ring of C1, hypertrophied ligamentum flavum, and occipitoatlantal membrane in posterior decompression surgery inevitably aggravates craniovertebral junction instability and may expose patients to acute post-traumatic myelopathy after even a minor trauma in flexion. Besides, in the study of stand-alone surgery, the average age of those 15 patients was approximately 15 years (i.e., they were old enough for reoperation). However, with the development of diagnostic technology, these patients are being diagnosed at a younger age (i.e., at ∼1 or 2 years), during which surgery is difficult and reoperation seems impossible. Therefore, it is important to perform early internal fixation to stabilize the spine and minimize the possibility of re-surgery.
Occipitocervical fusion is the most commonly used surgical strategy, because it is believed to be difficult to establish satisfactory stability in patients with ligamentous laxity if only C1-C2 fusion is performed (9, 13–15). Besides, as surgical intervention is recommended at an early age in such cases, the small size of these young patients is the main challenge for spinal surgeons when establishing stability via C1-C2 fusion alone because there is no dedicated cervical pediatric instrumentation available and even the smallest one for an adult is too big compared with the smaller vertebral dimensions. This makes it more difficult to put the screws into the correct position, especially for those with developmental deformity of the cervical vertebra (9, 14). However, in our center, we still prefer C1-C2 fusion because we try to preserve the child's cervical spine flexion-extension capacity as much as possible. Reducing unnecessary disabilities are of great importance in improving the children's quality of life and integrating them into society. Besides, C1-C2 fusion can also make the surgery possible for patients who cannot afford expensive treatments. Based on our experience and study of the literature, C1-C2 fusion is feasible and appropriate even for young children, especially with the help of new technologies such as 3D printing and intraoperative 3D image-based navigation system. Our center has successfully performed several cases of C1-C2 fusion operations for children with MPS type IVA, and all of them have achieved satisfactory prognosis. The youngest patient was only 2 years old, and the clinical symptoms associated with cervical spinal cord compression improved remarkably after the operation; however, other problems occurred in the thoracolumbar segment a few months later.
Posterior cervical C1-C2 fusion is an important, yet difficult and risky, procedure in spinal surgery, and effective internal fixation techniques reduce the risk of the operation and improve the fusion rate. In 1910, Mixter and Osgood (16) first reported the technique of C1-C2 stabilization by tying the odontoid with silk to treat a chronic non-healing odontoid fracture. Although the silk was replaced by wires and various modifications to the technique have been made in subsequent years, the disadvantages of the sublaminar wire technology are obvious: first, the spinal cord can be easily be injured during the passing of two separate sublaminar wires under both C1 and C2 laminae (17); second, the fixation offers poor rotational stability; and third, the early micro-movement reduces the fusion rate. Besides, the C1 posterior arch and C2 lamina/spinous processes should be intact (17). The use of interlaminar clamps and transarticular screw fixation of C1-C2 were first introduced in 1975 and 1979, which improved biomechanical stability and fusion rate (18–21). Goel and Laheri (22) used C1 lateral mass–C2 pars screw construct connected by posterior cervical plates to achieve posterior fixation, and Harms and Melcher (23) then modified the Goel technique. They used C1 lateral mass screws and C2 pedicle screws connected by rods to achieve rigid fixation, which provided great stability and fixation rate and reduced injury to the nerve root and vertebral artery. The Harms technique has been widely accepted by spine surgeons worldwide and is considered the gold standard (24, 25). For patients with MPS, the rate of structural variation of the cervical vertebrae is very high, and the standard Harms technique may not be achieved; thus, some alternatives are needed. The C1 “pedicle” screw fixation technique provides higher pull-out strength and avoidance of the neurovascular elements, but the risk of injury to the vertebral artery is higher than that of C1 lateral mass screws, and fracture may occur in the posterior arch. The C1 posterior arch crossing screw technique can be used as an alternative for failed conventional atlantoaxial screw placement or failed screw placement. C2 intralaminar screw fixation provided a salvage technique in cases of failed C2 pedicle screw placement or instances of high-riding vertebral artery (26, 27). Because of considerable anatomic variability, none of the previously described techniques allow absolute safety, and there are some hybrid constructs of those techniques to address complex clinical situations (17).
Cervical pedicle screws (CPSs) and rods offer greater stability than other techniques, but the risk of serious complications, such as injury to the vertebral artery, spinal cord, and nerve roots, remains. Computer-assisted surgery serves as an effective tool in improving accuracy. Preoperative and postoperative CT data do not match because they were obtained at different positions. Intraoperative 3D image-based navigation can reduce the discrepancy and facilitate safe and accurate insertion (28–32). The O-arm is an intraoperation image system that allows high-definition 3D navigation and thus facilitates a more accurate, convenient, and quick insertion of the screws. The O-arm-based navigation can reduce CPS malposition but cannot completely prevent it. As the position of the cervical structure can easily change, especially in young patients whose cervical structure is very small, the discrepancy of alignment between 3D image and CPS insertion reduces the accuracy. In our practice, we use the O-arm-based navigation system to determine insertion points and explore insertion paths using a micro-grinding drill. According to our practice, 3D printing may make this difficult and dangerous operation easier and safer, especially for patients with severe deformities that make placement of screws difficult.
With the in-depth understanding of MPS, advances in related monitoring tools, and progress in anesthetic techniques, the surgery for MPS has become safer than ever; thus, we advocate early decompression, posterior fixation and fusion of C1-C2 with CPS, and bone graft for children with MPS. Based on the experience of our center, we believe this is the most beneficial surgical management patients with MPS with for cervical involvement. (Typical case is presented in Figure 3).
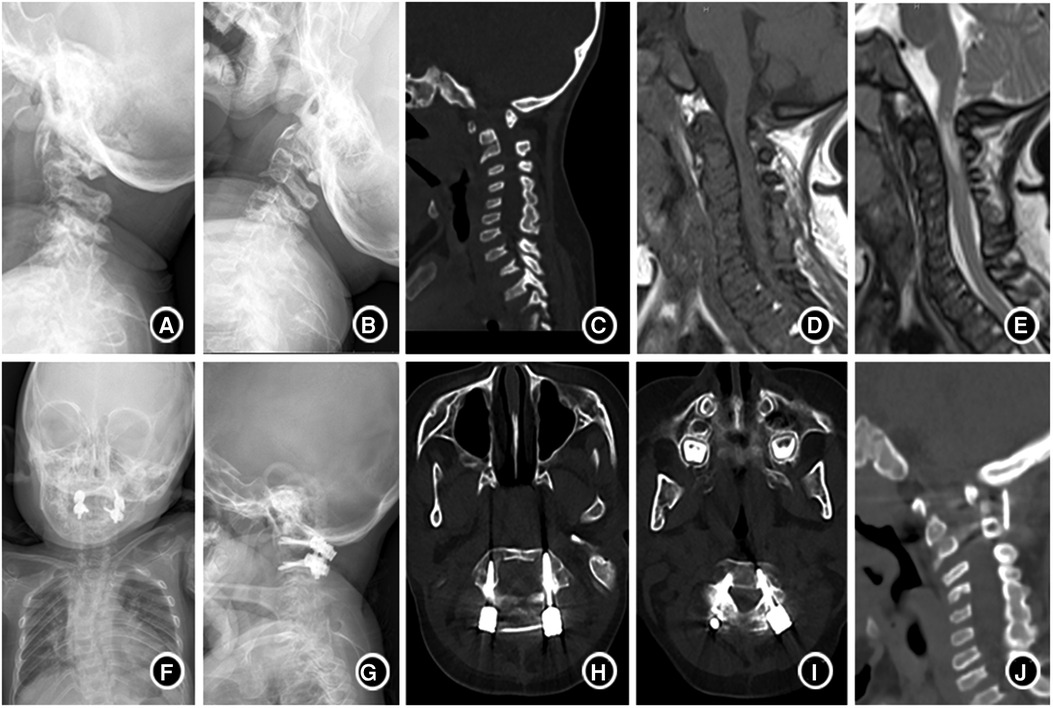
Figure 3. A 4-year-old boy with type IVA mucopolysaccharide disease (A,B). Lateral plain-film flexion-extension studies before the surgery showed uneven cervical bone density, flattened vertebra, atlantoaxial instability. (C) CT suggested atlantoaxial subluxation and odontoid process absence; (D,E) MRI indicated spinal canal stenosis, high cervical spinal cord compression and edema; (F,G) showed that the anatomical position of atlantoaxial returned to normal half a year after operation. (H–J). CT showed that the screw position was good, and the bone graft was fused with the atlantoaxial vertebra 6 months after operation.
Two main types of bone grafts are available for fusion: autogenous and allogenous. Allograft technologies were most commonly used to eliminate donor-site morbidity and complications related to autogenous bone graft. A study in 2017 compared structural allograft and autograft for instrumented atlantoaxial fusions in a series of 32 pediatric patients (33). In this study, the outcomes and fusion rates were similar regardless of whether an autograft or allograft was used; fusion time was increased when using allograft technologies, but blood loss was decreased and donor-site morbidity was avoided. However, as more clinical cases were studied and further long-term follow-up were conducted, fusion failure when using allograft has been observed. As reoperation of pediatric patients with failed fusion can be challenging, we believe that autograft should be the first priority to increase the success rate as much as possible. Available autograft bones include the iliac crest, ribs, and external plate of the skull. The anatomical features of the skull make it a good site for harvesting autogenous bones, which reduces injury to other sites. For C1-C2 fusion, the external plate of the skull should be the first choice. However, the skull plate cannot be used when occipitocervical fusion is needed, as the skull cannot be nailed without the outer plate. In a young child, the iliac crest is very thin and cartilage-based, which limits its application, and harvesting of the ribs can do a lot of damage.
In addition, osteoporosis is a common condition in patients with MPS. The term “dysostosis multiplex” is used to describe the abnormalities of MPS diseases. Osteoporosis has also been described in animal models of MPS. GAGs accumulate in all cells related to bone formation and remodeling in animal models of MPS, interfering with the normal formation of mineralized cartilage septa, which is required for osteoblasts and osteoclasts in the formation of new bones. Besides, the risk of poor bone mineralization of patients with MPS increases with malnutrition and reduction of physical activities caused by pain or exercise intolerance (34, 35). The pathophysiological basis of osteoporosis in patients with MPS is not completely understood. Considering the possibility of osteoporosis and the smaller bone structure of young patients with MPS, external fixation with brace for 3 to 6 months is needed to reduce internal fixation failure and thus avoid reoperation. Anti-osteoporosis therapy may be effective for postoperative recovery.
Conclusions
The compression of the cervical spinal cord due to various reasons is the main life-threatening factor in children with MPS. Advances in imaging technology, especially MRI, enable us to detect abnormalities of the spine and spinal cord as soon as possible and perform surgical intervention before neurological deterioration and loss of function. Although the number of cases is limited, decompression, autogenous bone fusion, and internal fixation with screws seem to be the best treatment options for children with MPS at present. With the help of various preoperative high-resolution reconstruction techniques, intraoperative 3D image-based navigation system, and 3D printing technology, C1-C2 fixation is feasible and safe in most cases, which preserves the flexion-extension capacity of the cervical spine as much as possible. In view of the high incidence of spinal diseases in children with MPS, we recommend that once MPS is diagnosed, relevant tests should be carried out as soon as possible to rule out cervical vertebra–related diseases.
Author contributions
H-TL, Y-HZ, and F-CZ contributed to conception and design of the study. Z-HL organized the database. F-CZ performed the statistical analysis. H-TL and Q-QZ wrote the first draft of the manuscript. Y-HZ, Z-HL, JS, and JS wrote sections of the manuscript. All authors contributed to the article and approved the submitted version.
Funding
This study was supported by grants from the Shanghai Municipal Health Commission (No. 201840061).
Conflict of interest
The authors declare that the research was conducted in the absence of any commercial or financial relationships that could be construed as a potential conflict of interest.
Publisher's note
All claims expressed in this article are solely those of the authors and do not necessarily represent those of their affiliated organizations, or those of the publisher, the editors and the reviewers. Any product that may be evaluated in this article, or claim that may be made by its manufacturer, is not guaranteed or endorsed by the publisher.
References
1. Zhou J, Lin J, Leung WT, Wang L. A basic understanding of mucopolysaccharidosis: incidence, clinical features, diagnosis, and management. IntrActable Rare Dis Res. (2020) 9(1):1–9. doi: 10.5582/irdr.2020.01011
2. Brusius-Facchin AC, Rojas Malaga D, Leistner-Segal S, Giugliani R. Recent advances in molecular testing to improve early diagnosis in children with mucopolysaccharidoses. Expert Rev Mol Diagn. (2018) 18(10):855–66. doi: 10.1080/14737159.2018.1523722
3. Remondino RG, Tello CA, Noel M, Wilson AF, Galaretto E, Bersusky E, et al. Clinical manifestations and surgical management of spinal lesions in patients with mucopolysaccharidosis: a report of 52 cases. Spine Deform. (2019) 7(2):298–303. doi: 10.1016/j.jspd.2018.07.005
4. Peck SH, Casal ML, Malhotra NR, Ficicioglu C, Smith LJ. Pathogenesis and treatment of spine disease in the mucopolysaccharidoses. Mol Genet Metab. (2016) 118(4):232–43. doi: 10.1016/j.ymgme.2016.06.002
5. Solanki GA, Martin KW, Theroux MC, Lampe C, White KK, Shediac R, et al. Spinal involvement in mucopolysaccharidosis IVA (morquio-brailsford or morquio a syndrome): presentation, diagnosis and management. J Inherit Metab Dis. (2013) 36(2):339–55. doi: 10.1007/s10545-013-9586-2
6. Williams N, Cundy PJ, Eastwod DM. Surgical management of thoracolumbar kyphosis in patients with mucopolysaccharidosis: a systematic review. Spine. (2017) 42(23):1817–25. doi: 10.1097/brs.0000000000002242
7. Giussani C, Guida L, Canonico F, Sganzerla EP. Cerebral and occipito-atlanto-axial involvement in mucopolysaccharidosis patients: clinical, radiological, and neurosurgical features. Ital J Pediatr. (2018) 44(Suppl 2):119. doi: 10.1186/s13052-018-0558-x
8. Shukla D, Arvind S, Devi BI. Myelopathy in a dwarf: a case of Morquio's Syndrome without odontoid hypoplasia. Neurol India. (2011) 59(1):126–7. doi: 10.4103/0028-3886.76861
9. Crostelli M, Mazza O, Mariani M, Mascello D, Iorio C. Spine challenges in mucopolysaccharidosis. Int Orthop. (2019) 43(1):159–67. doi: 10.1007/s00264-018-4143-0
10. Broomfield A, Zuberi K, Mercer J, Moss G, Finnegan N, Hensman P, et al. Outcomes from 18 years of cervical spine surgery in MPS IVA: a single centre's Experience. Child Care Health Dev's Nerv Syst: ChNS. (2018) 34(9):1705–16. doi: 10.1007/s00381-018-3823-9
11. Charrow J, Alden TD, Breathnach CA, Frawley GP, Hendriksz CJ, Link B, et al. Diagnostic evaluation, monitoring, and perioperative management of spinal cord compression in patients with Morquio syndrome. Mol Genet Metab. (2015) 114(1):11–8. doi: 10.1016/j.ymgme.2014.10.010
12. Krenzlin H, Ta-Chih T, Lampe C, Lampe C, Knuf M, Horn P, et al. Stand-alone craniocervical decompression is feasible in children with mucopolysaccharidosis type I, IVA, and VI. Spine J. (2018) 18(8):1455–9. doi: 10.1016/j.spinee.2018.04.002
13. Rajinda P, Towiwat S, Chirappapha P. Comparison of outcomes after atlantoaxial fusion with C1 lateral mass-C2 pedicle screws and C1-C2 transarticular screws. Eur Spine J. (2017) 26(4):1064–72. doi: 10.1007/s00586-016-4829-1
14. Moon E, Lee S, Chong S, Park JH. Atlantoaxial instability treated with free-hand C1-C2 fusion in a child with Morquio syndrome. Child Care Health Dev's Nerv Syst: ChNS. (2020) 36(8):1785–9. doi: 10.1007/s00381-020-04561-2
15. Williams N, Narducci A, Eastwood DM, Cleary M, Thompson D. An evidence-based approach to the management of children with morquio a syndrome presenting with craniocervical pathology. Spine. (2018) 43(24):E1443–e1453. doi: 10.1097/brs.0000000000002743
16. Mixter SJ, Osgood RB. IV. Traumatic lesions of the atlas and axis. Ann Surg. (1910) 51(2):193–207. doi: 10.1097/00000658-191002000-00004
17. Chen Q, Brahimaj BC, Khanna R, Kerolus MG, Tan LA, David BT, et al. Posterior atlantoaxial fusion: a comprehensive review of surgical techniques and relevant vascular anomalies. J Spine Surg. (2020) 6(1):164–80. doi: 10.21037/jss.2020.03.05
18. Farey ID, Nadkarni S, Smith N. Modified Gallie technique versus transarticular screw fixation in C1-C2 fusion. Clin Orthop Relat Res. (1999) 359:126–35. doi: 10.1097/00003086-199902000-00013
19. Stillerman CB, Wilson JA. Atlanto-axial stabilization with posterior transarticular screw fixation: technical description and report of 22 cases. Neurosurgery. (1993) 32(6):948–54. discussion 954-5. doi: 10.1227/00006123-199306000-00011
20. Taggard DA, Kraut MA, Clark CR, Traynelis VC. Case-control study comparing the efficacy of surgical techniques for C1-C2 arthrodesis. J Spinal Disord Tech. (2004) 17(3):189–94. doi: 10.1097/00024720-200406000-00005
21. Neo M, Matsushita M, Iwashita Y, Yasuda T, Sakamoto T, Nakamura T. Atlantoaxial transarticular screw fixation for a high-riding vertebral artery. Spine. (2003) 28(7):666–70. doi: 10.1097/01.Brs.0000051919.14927.57
22. Goel A, Laheri V. Plate and screw fixation for atlanto-axial subluxation. Acta Neurochir. (1994) 129(1-2):47–53. doi: 10.1007/bf01400872
23. Harms J, Melcher RP. Posterior C1-C2 fusion with polyaxial screw and rod fixation. Spine. (2001) 26(22):2467–71. doi: 10.1097/00007632-200111150-00014
24. Elliott RE, Tanweer O, Boah A, Smith ML, Frempong-Boadu A. Comparison of safety and stability of C-2 pars and pedicle screws for atlantoaxial fusion: meta-analysis and review of the literature. J Neurosurg Spine. (2012) 17(6):577–93. doi: 10.3171/2012.9.Spine111021
25. Elliott RE, Tanweer O, Boah A, Morsi A, Ma T, Smith ML, et al. Atlantoaxial fusion with screw-rod constructs: meta-analysis and review of literature. World Neurosurg. (2014) 81(2):411–21. doi: 10.1016/j.wneu.2012.03.013
26. Lehman RA Jr., Dmitriev AE, Helgeson MD, Sasso RC, Kuklo TR, Riew KD. Salvage of C2 pedicle and pars screws using the intralaminar technique: a biomechanical analysis. Spine. (2008) 33(9):960–5. doi: 10.1097/BRS.0b013e31816c915b
27. Dorward IG, Wright NM. Seven years of experience with C2 translaminar screw fixation: clinical series and review of the literature. Neurosurgery. (2011) 68(6):1491–9. discussion 1499. doi: 10.1227/NEU.0b013e318212a4d7
28. Ishikawa Y, Kanemura T, Yoshida G, Matsumoto A, Ito Z, Tauchi R, et al. Intraoperative, full-rotation, three-dimensional image (O-arm)-based navigation system for cervical pedicle screw insertion. J Neurosurg Spine. (2011) 15(5):472–8. doi: 10.3171/2011.6.Spine10809
29. Hott JS, Deshmukh VR, Klopfenstein JD, Sonntag VK, Dickman CA, Spetzler RF, et al. Intraoperative Iso-C C-arm navigation in craniospinal surgery: the first 60 cases. Neurosurgery. (2004) 54(5):1131–6. discussion 1136-7. doi: 10.1227/01.neu.0000119755.71141.13
30. Ito Y, Sugimoto Y, Tomioka M, Hasegawa Y, Nakago K, Yagata Y. Clinical accuracy of 3D fluoroscopy-assisted cervical pedicle screw insertion. J Neurosurg Spine. (2008) 9(5):450–3. doi: 10.3171/spi.2008.9.11.450
31. Rajasekaran S, Vidyadhara S, Ramesh P, Shetty AP. Randomized clinical study to compare the accuracy of navigated and non-navigated thoracic pedicle screws in deformity correction surgeries. Spine. (2007) 32(2):E56–64. doi: 10.1097/01.brs.0000252094.64857.ab
32. Rajasekaran S, Vidyadhara S, Shetty AP. Iso-C3D fluoroscopy-based navigation in direct pedicle screw fixation of Hangman fracture: a case report. J Spinal Disord Tech. (2007) 20(8):616–9. doi: 10.1097/BSD.0b013e318074f978
33. Zhang YH, Shen L, Shao J, Chou D, Song J, Zhang J. Structural allograft versus autograft for instrumented atlantoaxial fusions in pediatric patients: radiologic and clinical outcomes in series of 32 patients. World Neurosurg. (2017) 105:549–56. doi: 10.1016/j.wneu.2017.06.048
34. Lin HY, Shih SC, Chuang CK, Chen MR, Niu DM, Lin SP. Assessment of bone mineral density by dual energy x-ray absorptiometry in patients with mucopolysaccharidoses. Orphanet J Rare Dis. (2013) 8:71. doi: 10.1186/1750-1172-8-71
35. Fung EB, Johnson JA, Madden J, Kim T, Harmatz P. Bone density assessment in patients with mucopolysaccharidosis: a preliminary report from patients with MPS II and VI. J Pediatr Rehabil Med. (2010) 3(1):13–23. doi: 10.3233/PRM-2010-0105
36. White KK, Steinman S, Mubarak SJ. Cervical stenosis and spastic quadriparesis in Morquio disease (MPS IV). A case report with twenty-six-year follow-up. J Bone Joint Surg Am. (2009) 91(2):438–42. doi: 10.2106/JBJS.H.00148
37. Houten JK, Kinon MD, Goodrich JT. Morquio's syndrome and craniocervical instability. Pediatr Neurosurg. (2011) 47(3):238–40. doi: 10.1159/000334310
38. Ain MC, Chaichana KL, Schkrohowsky JG. Retrospective study of cervical arthrodesis in patients with various types of skeletal dysplasia. Spine (Phila Pa 1976). (2006) 31(6):E169–74. doi: 10.1097/01.brs.0000202758.61848.61
Keywords: mucopolysaccharidoses, cervical spine, surgical managements, occipitocervical fusion, atlantoaxial fusion
Citation: Liu H, Song J, Zhou F, Liang Z, Zhang Q, Zhang Y and Shao J (2023) Cervical spine involvement in pediatric mucopolysaccharidosis patients: Clinical features, early diagnosis, and surgical management. Front. Surg. 9:1059567. doi: 10.3389/fsurg.2022.1059567
Received: 1 October 2022; Accepted: 22 November 2022;
Published: 6 January 2023.
Edited by:
Fuxin Wei, Sun Yat-sen University, ChinaReviewed by:
Ym Xi, The Affiliated Hospital of Qingdao University, ChinaCinthia Castro Do Nascimento
© 2023 Liu, Song, Zhou, Liang, Zhang, Zhang and Shao. This is an open-access article distributed under the terms of the Creative Commons Attribution License (CC BY). The use, distribution or reproduction in other forums is permitted, provided the original author(s) and the copyright owner(s) are credited and that the original publication in this journal is cited, in accordance with accepted academic practice. No use, distribution or reproduction is permitted which does not comply with these terms.
*Correspondence: Yue-Hui ZhangenloYW5keTgwMzBAMTI2LmNvbQ== Jiang Shao c2hhb2ppYW5nQHhpbmh1YW1lZC5jb20uY24=
†These authors have contributed equally to this work
Specialty Section: This article was submitted to Orthopedic Surgery, a section of the journal Frontiers in Surgery
Abbreviation MPS, mucopolysaccharidosis; CT, computed tomography; MRI, magnetic resonance imaging; CT, computed tomographic angiography; 3D, three-dimensional.