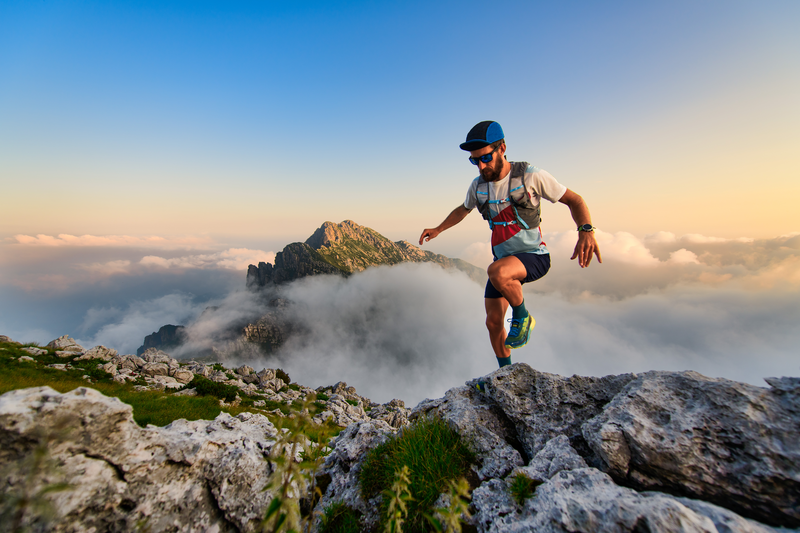
95% of researchers rate our articles as excellent or good
Learn more about the work of our research integrity team to safeguard the quality of each article we publish.
Find out more
ORIGINAL RESEARCH article
Front. Surg. , 10 June 2021
Sec. Cardiovascular Surgery
Volume 8 - 2021 | https://doi.org/10.3389/fsurg.2021.679273
Background: Prolonged mechanical ventilation (PMV) is common after cardiothoracic surgery, whereas the mechanical ventilation strategy after pulmonary endarterectomy (PEA) has not yet been reported. We aim to identify the incidence and risk factors for PMV and the relationship between PMV and short-term outcomes.
Methods: We studied a retrospective cohort of 171 who undergoing PEA surgery from 2014 to 2020. Cox regression with restricted cubic splines was performed to identify the cutoff value for PMV. The Least absolute shrinkage and selection operator regression and logistic regressions were applied to identify risk factors for PMV. The impacts of PMV on the short-term outcomes were evaluated.
Results: PMV was defined as the duration of mechanical ventilation exceeding 48 h. Independent risk factors for PMV included female sex (OR 2.911; 95% CI 1.303–6.501; P = 0.009), prolonged deep hypothermic circulatory arrest (DHCA) time (OR 1.027; 95% CI 1.002–1.053; P = 0.036), increased postoperative blood product use (OR 3.542; 95% CI 1.203–10.423; P = 0.022), elevated postoperative total bilirubin levels (OR 1.021; 95% CI 1.007–1.034; P = 0.002), increased preoperative pulmonary artery pressure (PAP) (OR 1.031; 95% CI 1.014–1.048; P < 0.001) and elongated postoperative right ventricular anteroposterior dimension (RVAD) (OR 1.119; 95% CI 1.026–1.221; P = 0.011). Patients with PMV had longer intensive care unit stays, higher incidences of postoperative complications, and higher in-hospital medical expenses.
Conclusions: Female sex, prolonged DHCA time, increased postoperative blood product use, elevated postoperative total bilirubin levels, increased preoperative PAP, and elongated postoperative RVAD were independent risk factors for PMV. Identification of risk factors associated with PMV in patients undergoing PEA may facilitate timely diagnosis and re-intervention for some of these modifiable factors to decrease ventilation time and improve patient outcomes.
Chronic thromboembolic pulmonary hypertension (CTEPH) is the main cause of type IV pulmonary hypertension (PH), which is defined as a mean pulmonary arterial pressure (mPAP) >25 mmHg with a pulmonary capillary wedge pressure (PCWP) <15 mmHg (1–3). The major pathological mechanism is elevated pulmonary vascular resistance (PVR) due to the obstruction of pulmonary embolism and incomplete thrombus resolution within the pulmonary arteries, which eventually contribute to progressive PH and right ventricular failure (4). The incidence of CTEPH in the general population and among acute pulmonary embolism survivors is 3–30 cases per million and 0.4–9.1%, respectively (2). Without appropriate treatment, the 3-year survival rate of patients with an mPAP >50 mmHg is not >10% (5). Pulmonary endarterectomy (PEA) remains the predominant guideline-recommended treatment for patients with CTEPH (6). The short-term and long-term outcomes of PEA are reasonable in experienced centers (7, 8), with in-hospital mortality not >5% (9).
Prolonged mechanical ventilation (PMV) after cardiothoracic surgery is associated with increased mortality and morbidity (10–14). For complex surgical procedures, such as cardiothoracic surgeries, the duration of postoperative mechanical ventilation is generally prolonged (14–16). The reported incidence of PMV after cardiothoracic surgeries varies from 2 (17) to 43 (18), according to different definitions of PMV and different patient selection with different surgery types. Numerous studies examined the independent risk factors for postoperative PMV in various cardiothoracic surgeries to identify modifiable factors (17, 19–21). Early identification and early prevention are central efforts to improving the prognosis of patients.
Due to the characteristics of the PEA procedure, including a longer cardiopulmonary bypass (CPB) time with deep hypothermic circulatory arrest (DHCA) to achieve a bloodless visual field and direct intraoperative stretching of the pulmonary artery, the incidence of postoperative pulmonary complications is much higher than other cardiothoracic surgeries. The major complications after PEA are reperfusion pulmonary edema and pulmonary infection, which are not beneficial to the prognosis of patients (22). Therefore, the immediate identification of patients with a higher risk profile and the implementation of positive preventive and therapeutic strategies targeting pulmonary complications after PEA is particularly important. The incidence of PMV after PEA was not reported in previous studies. Therefore, the present study identified the incidence and potential risk factors for PMV after PEA and to clarify the relationship between the PMV and short-term outcomes.
From January 2014 to August 2020, patients undergoing PEA with CPB at Fuwai Hospital were consecutively included in this retrospective observational study. We excluded three patients who were not finally diagnosed with CTEPH at discharge and two patients who had incomplete clinical data. The final study population was 171 patients. All patients were given general anesthesia and postoperative targeted management according to the clinical practice in our hospital. The Research Ethics Committees of Fuwai Hospital approved this study.
All data were collected from the electronic medical record system, and two independent researchers were responsible for checking the data quality. Potential predictor variables were chosen based on the previous literature review, clinical judgment, and availability in the authors' hospital. Perioperative characteristics included age, sex, body mass index (BMI), blood type, University of California San Diego (UCSD) classification, New York Heart Association (NHYA) heart function classification, arterial partial pressure of oxygen (PaO2) and arterial partial pressure of carbon dioxide (PaCO2) inhaling room air on admission to the hospital, deep venous thrombosis history, acute pulmonary embolism history, acute thrombolysis history, antiphospholipid syndrome history, diabetes history, hyperlipidemia history, hypertension history, coronary artery disease (CAD) history, smoking history, concomitant cardiac operation, intraoperative sufentanil use, intraoperative etomidate use, intraoperative midazolam use, intraoperative dexmedetomidine use, intraoperative blood product use, intraoperative total amount of fluid, intraoperative fibrinogen use, intraoperative prothrombin use, intraoperative methylprednisolone use, intraoperative creatine phosphate sodium use, intraoperative ulinastatin use, intraoperative maximum vasoactive-inotropic score (VISmax), CPB time, aortic cross-clamp time, DHCA time, lowest rectal temperature during CPB, lowest nasopharyngeal temperature during CPB, intraoperative blood loss, postoperative blood product use within the first 72 h, and chest discharge within 72 h after surgery. The following preoperative and postoperative biomarkers were collected: white blood cell, monocyte, lymphocyte, neutrophil, hematocrit, platelet counts and hemoglobin levels; international normalized ratio (INR), prothrombin time (PT), activated partial thromboplastin time (APTT), erythrocyte sedimentation rate (ESR), total protein, albumin, alkaline phosphatase, aspartate aminotransferase, alanine aminotransferase, total bilirubin, serum creatinine, blood glucose, lactate dehydrogenase, creatine kinase and isoenzyme of creatine kinase-MB, and C-reactive protein (CRP) levels. The following preoperative and postoperative echocardiography and right heart catheterization variables were collected: left ventricle end-diastolic diameter, left ventricle ejection fraction, atrioventricular valve regurgitation, right ventricle anteroposterior dimension (RVAD), internal diameter of the major pulmonary artery, mPAP, PVR, cardiac output (CO) and cardiac output index (CI).
PMV was defined as the duration of postoperative mechanical ventilation exceeding the cutoff point. According to the results of the Cox regression model for predicting the postoperative length of hospital stay (p-LOHS), the cutoff value for PMV was chosen at which the hazard ratio of prolonged p-LOHS had the highest increasing trend. The VIS was calculated as dopamine (μg/kg/min) + dobutamine (μg/kg/min) + 10 × milrinone (μg/kg/min) + 50 × levosimendan (μg/kg/min) + 100 × epinephrine (μg/kg/min) + 100 × norepinephrine (g/kg/min) + 10,000 × vasopressin (U/kg/min) (23).
All patients underwent PEA with CPB. The surgical procedure and anesthesia management of PEA were demonstrated in detail in previous studies (22, 24). Briefly, the key to PEA is completing the bilateral endarterectomy of chronically thromboembolic pulmonary tissue via median sternotomy by CPB with intermittent DHCA. A circulatory arrest is inevitable to provide a bloodless surgical field, and deep hypothermia decreases the metabolism and oxygen demand of organs to play a protective role. Each duration of cardiac arrest is within the limit of 20 min, and the maintenance of proper cerebral oxygen saturation and selective cerebral perfusion (SACP) are of great importance to achieve effective cerebral protection.
All patients were transferred to the cardiac intensive care unit (ICU) after PEA. Postoperative extubation was performed when patients met the following standard institution protocols: spontaneous respiration, intact airway reflexes, manageable airway secretions, and hemodynamic stabilization. Postoperative ICU management was provided by a multidisciplinary team of surgeons, radiologists, cardiac anesthesiologists, intensivists, and nurses to provide highly specialized care for CTEPH patients.
Continuous variables are shown as medians (interquartile ranges), and categorical variables are shown as counts (percentages). We used the non-parametric Mann–Whitney U-test to compare continuous variables, and the chi-squared test to compare categorical variables. Multivariate Cox regression with restricted cubic splines was performed to identify the cutoff value for PMV using the p-LOHS as the dependent variable, and the spline was plotted with three knots defined at the 5th, 50th, and 95th percentiles of the variable (25). Least absolute shrinkage and selection operator (LASSO) regression is the most popular and widely used regularized regression which was used to select variables by minimizing the potential collinearity and overfitting of variables with the help of an optimal lambda (λ) (26, 27). The most significant advantage of LASSO regression is that by penalizing all the coefficients of the variables, the coefficients of the relatively insignificant independent variables become zero and were thus excluded from the modeling. LASSO regression can treat all the variables simultaneously, rather than stepwise, which makes the modeling much more stable. LASSO regression is essential when a study has a relatively small sample size but many candidate variables, and it may help the selection of variables that are most important to include in a prediction model. Univariate and multivariate logistic regressions with the stepwise backward elimination method were used to identify risk factors for PMV. The area under the receiver operating characteristic curve (AU-ROC) was used to assess the discrimination of the logistic regression model, and the Hosmer–Lemeshow test was performed to evaluate its calibration. Two-sided P < 0.05 was considered significant. All statistical analyses were performed using Stata 14 software and R software (version 3.5.2).
There were 176 PEA surgeries performed at Fuwai Hospital between January 2014 and August 2020. After excluding three patients who were not finally diagnosed with CTEPH at discharge and two patients who had incomplete clinical data, the final study population contained 171 patients.
Of the 171 patients in this retrospective study, 61 patients (35.7%) were female, with a mean age of 46.79 ± 13.34 years. All patients were diagnosed with CTEPH. Six (3.5%) patients in our population had diabetes mellitus (DM), 28 (16.4%) patients had hypertension, 12 (7%) patients had CAD and 36 (21.1%) patients were smokers. The mean CPB and aortic cross-clamp times in the total study cohort were 224 ± 52 and 111 ± 43 min, respectively. DHCA was performed in all cases with a mean time of 35 ± 16 min. There was no difference in preoperative respiratory function between the PMV group and the non-PMV group.
We used multivariate Cox regression with the enter method to identify the short-term outcomes that may affect the duration of p-LOHS, and included extracorporeal membrane oxygenation treatment (ECMO), mechanical ventilation time, reintubation, reperfusion pulmonary edema, pulmonary hypertension crisis, pulmonary infection, pericardial effusion, postoperative arrhythmia, renal replacement treatment (RRT), chest drainage within 72 h after surgery, postoperative high-sensitivity C-reactive protein level, postoperative alanine aminotransferase level and postoperative aspartate aminotransferase level. In the Cox regression model, postoperative mechanical ventilation time, pulmonary hypertension crisis, pulmonary infection, and pericardial effusion were independent risk factors for prolonged p-LOHS. Then the restricted cubic spline Cox model was used to analyze the relationship between the mechanical ventilation time and the risk of prolonged p-LOHS. The log of the adjusted hazard ratios of mechanical ventilation time derived from the multivariate Cox model after adjustment of pulmonary hypertension crisis, pulmonary infection, and pericardial effusion was shown on the y-axis, and the mechanical ventilation time was shown on the x-axis. The 95% confidence intervals of the adjusted hazard ratios are represented by the gray area. The cutoff value for PMV was determined to be 48 h because patients with mechanical ventilation time >48 h had sharply increased risks of prolonged p-LOHS (Figure 1). The adjusted hazard ratio of PMV was 1.81 (1.3–2.5).
Figure 1. Cox regression model with restricted cubic splines of the relationship between the postoperative mechanical ventilation time and the risk of prolonged length of hospital stay. The log of the adjusted hazard ratios of mechanical ventilation time derived from the multivariate Cox model after adjustment of pulmonary hypertension crisis, pulmonary infection, and pericardial effusion is shown on the y-axis. The 95% confidence intervals of the adjusted hazard ratios are represented by the gray area. The risk function demonstrates an inflection point at 48 h.
According to the cutoff value we found, the incidence of postoperative PMV in our study group was 50.3% (n = 86). The mean ventilation time of the whole cohort was 98 h and the median was 49 h. Table 1 shows the demographic and perioperative variables of the two groups. There were significant differences between the two groups in preoperative cardiac status evaluated using an echocardiogram, preoperative PAP, preoperative PVR, preoperative total bilirubin, CPB time, and intraoperative VISmax.
A total of 102 variables were included in the LASSO regression, and 28 important variables were chosen at an ln λ of 8.4703. The LASSO regression is shown in Figure 2. Six of these 28 variables, including female sex, prolonged DHCA time, increased postoperative blood product use, elevated postoperative total bilirubin, increased preoperative PAP, and elongated postoperative RVAD were finally included in the logistic regression model and identified as independent risk factors for PMV (Table 2). The appropriateness of the logistic regression model was authorized by the Hosmer–Lemeshow goodness-of-fit statistic (p = 0.657). The area under the curve of ROC for PMV was 0.8341 (95% CI, 0.774–0.894) (Figure 3).
Figure 2. (A) Selection of tuning parameter (lambda [λ]) in the least absolute shrinkage and selection operator (LASSO) model via 10-fold cross-validation. The optimal ln (λ) = 8.4703, was chosen based on minimum criteria. (B) LASSO coefficients produced by the LASSO logistic regression analysis. Twenty-eight variables (total 102 variables) with non-zero coefficients were chosen in the LASSO logistic regression model based on the optimal ln λ-value and were chosen as the most important variables after considering the correlation among candidate variables.
Figure 3. The area under the receiver operating characteristic curve (AU-ROC) for prolonged mechanical ventilation.
The 90-day mortality was 1.8% (3/171). There was no 90-day mortality difference (p = 0.246) between PMV patients and non-PMV patients, but all three deaths occurred in the PMV group. Of the 171 patients, 86 (50.3%) required PMV. Twelve patients (14%) in the PMV group required tracheotomy, and no patients in the non-PMV group required tracheotomy. Compared to patients without PMV, patients with PMV were associated with more blood transfusions (30.2 vs. 15.3%, p = 0.028), a higher rate of delirium (17.4 vs. 5.9%, p = 0.011), reperfusion pulmonary edema (9.3 vs. 1.2%, p = 0.047), pulmonary infection (51.2 vs. 24.7%, p < 0.001) and RRT (7 vs. 0%, p = 0.029), prolonged length of ICU stay (LOIS) (4 (3–5) days vs. 6 (5–10) days, p < 0.001), and increased in-hospital medical expenses (22.15 [18.59–25.89] $1,000 vs. 27.38 [22.38–38.01] $1,000, p < 0.001). Table 3 shows the short-term outcomes.
Our study defined PMV as the duration of mechanical ventilation after PEA longer than 48 h, the incidence of which was 50.3% (n = 86). Although there was no difference in 90-days mortality between the two groups, all three deaths occurred in the PMV group. PMV was significantly associated with prolonged LOIS, higher rates of postoperative complications, and higher in-hospital medical expenses. Compared to the non-PMV group, the PMV group had an in-hospital costs increase of $5,230. Female sex, prolonged DHCA time, increased postoperative blood product use, elevated postoperative total bilirubin levels, increased preoperative PAP, and elongated postoperative ADRV were independent risk factors for PMV.
PMV has been variously defined in previous studies as exceeding 24 h (28), 48 h (21), 72 h (17), 7 days (18), or 21 days (29) of mechanical ventilation in accordance with previous clinical guidelines, clinical experience or the 90th percentile of the duration of mechanical ventilation for the cohort. We tried to use statistical methods to find the cutoff where the hazard ratio of p-LOHS increased steeply, which was 48 h. The PMV incidence (50.3%) in our study was significantly higher than previous studies for various cardiothoracic surgeries, which may be due to the following reasons. One major reason may be the differences in PMV definitions. Sharma et al. (12) defined PMV as exceeding 48 h. Although their incidence of PMV after cardiac surgery was only 6%, they excluded relatively severe procedures, such as ventricular assist device insertion or heart transplantation. Papathanasiou et al. (18) from Germany identified an incidence of 43% in patients after left ventricular assist device implantation, and their definition of PMV was longer than 7 days. However, the baseline cardiopulmonary dysfunction was relatively severe in our cohort, which directly contributed to postoperative PMV.
The present study used the multiple logistic regression model to identify that female sex, prolonged DHCA time, increased postoperative blood product use, elevated postoperative total bilirubin levels, increased preoperative PAP and elongated postoperative ADRV were independent risk factors for PMV. Notably, several variables that were often considered risk factors for PMV after cardiothoracic surgeries were not identified as risk factors in this study, such as advanced age, increased BMI, low ejection fraction, and emergent surgery (12, 14, 30). The reason may be the characteristics of CTEPH patients. Patients with CTEPH are generally of younger ages, and their BMI distribution is narrow. CTEPH primarily involves the right heart system (1), and the early impact on ejection is relatively small. Moreover, there was no emergent surgery in this study cohort.
The relationship between female sex and mechanical ventilation time after cardiothoracic surgery is controversial (31, 32). Our study found that female sex was a risk factor for PMV after PEA, and female patients had a 2.9-fold increased incidence of PMV. Our findings are supported by the results of many previous studies that showed that female patients had longer mechanical ventilation times and worse outcomes than male patients after cardiac surgeries (28, 33). The exact reason for the sex differences is not known. We hypothesize that it may be related to the hormonal and biological differences between males and females. Butterworth et al. (34) demonstrated that female patients tended to have more opioid exposure after cardiac surgery, which may result in a higher incidence of opioid-induced respiratory depression.
Prolonged DHCA time was the strongest predictor, with a 3.5-fold increased incidence of PMV in our study. Prior studies support this finding (11, 35). Although DHCA provides a bloodless field of vision for the dissection plane and protection for important organs, such as the brain, with the reduction of metabolic demands and oxygen consumption, hypothermia itself has several potential damaging side effects. Previous studies reported that deep hypothermic temperatures may lead to hypothermia-induced coagulation dysfunction, increased systemic inflammatory response, increased capillary permeability, microvasculature endothelial dysfunction, and increased risk of organ dysfunction, such as renal and respiratory failure (36). An increasing number of surgeons recently chose moderate hypothermic circulation in aortic arch surgery because it avoids the adverse effects of hypothermia and decreases CPB times by reducing the cooling and rewarming time (37–39). Prashanth et al. (39) reported that moderate hypothermic circulatory arrest (MHCA) with SACP was safe, with satisfactory neurological outcomes, without increasing mortality and morbidity. Because DHCA time was a modifiable risk factor in this study, we hypothesized that MHCA would be beneficial to the recovery of self-help ventilation compared to DHCA.
The present study found that CPB time was not an independent risk factor for PMV after PEA, which was controversial in previous studies (12, 31). Matthew et al. (11) examined risk factors for prolonged postoperative respiratory support in patients undergoing thoracic aortic surgery and found that CPB time was not associated with the need for prolonged postoperative respiratory support. Maria et al. (18) recently examined predictors of prolonged ventilation in patients who received left ventricular assist device implantation and did not find an association between CPB time and increased risk of PMV. However, the CPB time was a predictor for PMV after cardiac surgery in Sharma et al. (12) and Lei et al. (31), and they believed that prolonged CPB time had adverse effects on pulmonary function by inducing a systemic inflammatory response, producing oxygen free radicals, and activating polymorphonuclear neutrophils. This finding needs further confirmation in future studies.
Similar to previous studies, our study showed that postoperative blood product transfusion was an independent risk factor for PMV. Previous studies found that patients requiring blood product transfusion had increased pulmonary complications, adverse postoperative events, and hospitalization-related costs compared to non-transfusion recipients (11, 40, 41). An analysis of nearly 17,000 patients undergoing CABG surgery showed that the transfusion of even one unit of red blood cells had an adverse impact on the patient prognosis, such as increasing the risk of morbidity and mortality (42). Our findings further emphasized the importance of strengthening blood conservation methods and improving guidelines for blood product transfusion.
In addition, increased preoperative PAP and elongated postoperative RVAD were independent risk factors for postoperative PMV. They contributed to a 1.031- and 1.119-fold increase of postoperative PMV, respectively. The higher the preoperative PH is, the more serious the patient's condition is indicated before the operation, and the longer it takes patients to recover after surgery. Susanna et al. (43) found that preoperative mPAP <16 mmHg is strongly associated with morbidity and mortality after Bidirectional Cavopulmonary Connection, while PVR is not. Patients in the high preoperative mPAP group experienced the longest mechanical ventilation time and p-LOHS, the highest risk of postoperative arrhythmias and need for supplemental oxygen at discharge. Ruchit et al. (44) found that decreasing the patients' mPAP preoperatively can lead to potential benefits for postoperative early surgical outcomes like shortened duration of mechanical ventilation, reduced length of ICU stay and p-LOHS. In turn, elongated postoperative RVAD suggests poorer recovery of right ventricular function after surgery, resulting in patients requiring more recovery time. Several molecular and cellular mechanisms have been proposed in the development of acute RVD secondary to PH (45). RV wall tension increase leads to cardiomyocyte stress and injury secondary to ischemia, substrate depletion, and mitochondrial energy metabolism impairment. Feasible treatment measures may include: (1) General measures include avoiding increased atrioventricular load, reducing atrioventricular contractility and optimizing atrioventricular preload, applying “atrioventricular protective” ventilation strategies to maintain sinus rhythm and atrioventricular synchronization; (2) Medicine therapy, inotropic and vascular activity support (46).
This study is the first study to demonstrate the scientific definition, incidence, and risk factors for PMV in CTEPH patients after PEA. Our findings have multiple implications for perioperative management in CTEPH patients and provide future research directions on pulmonary complications after PEA. Although some risk factors in the present study were not modifiable, including female sex, increased preoperative PAP, elongated postoperative RVAD, and elevated postoperative total bilirubin levels, these parameters may be used to identify patients with a high risk for PMV after PEA. The avoidance or targeted therapy of the modifiable factors, which included decreasing DHCA time and the implementation of a conservative blood transfusion protocol, may help reduce the incidence of PMV, shorten the LOIS and p-LOHS, and reduce unnecessary hospitalization costs. Because MHCA is safe and has satisfactory neurological outcomes, MHCA instead of DHCA may be a better strategy to reduce the risk of PMV in CTEPH patients undergoing PEA.
The present study has several limitations. First, this study is a retrospective, single-institution study involving only PEA, and the application of some conclusions may be limited. With the possibility of missing other important factors that may be related to the mechanical ventilation weaning outcome, such as pre- or post-operative fluid balance, CT and MRI data, the risk model can only be applied within the variables that were included in this study. Second, due to the relatively aggressive definition of PMV in this study, the incidence of PMV and the short-term outcomes of CTEPH patients after PEA may have been overestimated. We have not clarified the underlying etiology of PMV. Third, because of the small sample size, the internal and external validations of the prediction ability were not performed. A large-scale prospective study is needed in the future to derive an exact predictive model of PMV after PEA.
The incidence (50.3%) of PMV, which was defined using the cutoff value (48 h), after PEA in this study was significantly higher than other surgeries. This finding was likely because of the different definitions of PMV and different degrees of baseline cardiopulmonary dysfunction. Multivariate analysis revealed that female sex, prolonged DHCA time, increased postoperative blood product use, elevated postoperative total bilirubin levels, increased preoperative PAP and elongated postoperative RVAD were independent risk factors for PMV. Compared to the non-PMV group, the PMV group had prolonged LOIS, higher rates of postoperative complications, and higher in-hospital medical expenses. The identification of risk factors associated with PMV in CTEPH patients undergoing PEA may facilitate timely diagnosis and reinterventions for some of these modifiable factors to achieve the goals of decreasing ventilation time and improving patient outcomes.
The raw data supporting the conclusions of this article will be made available by the authors, without undue reservation.
GW and CZ proposed the idea of this investigation. SS, JL, ZF, and LY were responsible for the collection of data and material. CZ helped with the statistical analysis and wrote the manuscript. GW helped to revise the manuscript. All authors contributed extensively to the work presented in this paper, read, and approved the final manuscript.
This work was supported by the National Natural Science Foundation of China (Grant No. 81970344).
The authors declare that the research was conducted in the absence of any commercial or financial relationships that could be construed as a potential conflict of interest.
We thank Dr. Yinan Li of the Department of Anesthesiology, Fuwai Hospital in Beijing for her contribution to the statistical and manuscript revising support.
The Supplementary Material for this article can be found online at: https://www.frontiersin.org/articles/10.3389/fsurg.2021.679273/full#supplementary-material
1. Kim NH, Delcroix M, Jais X, Madani MM, Matsubara H, Mayer E, et al. Chronic thromboembolic pulmonary hypertension. Eur Respir J. (2019) 53:1801915. doi: 10.1183/13993003.01915-2018
2. Lang IM, Pesavento R, Bonderman D, Yuan JX. Risk factors and basic mechanisms of chronic thromboembolic pulmonary hypertension: a current understanding. Eur Respir J. (2013) 41:462–8. doi: 10.1183/09031936.00049312
3. Simonneau G, Montani D, Celermajer DS, Denton CP, Gatzoulis MA, Krowka M, et al. Haemodynamic definitions and updated clinical classification of pulmonary hypertension. Eur Respir J. (2019) 53:1801913. doi: 10.1183/13993003.01913-2018
4. Lang IM, Madani M. Update on chronic thromboembolic pulmonary hypertension. Circulation. (2014) 130:508–18. doi: 10.1161/circulationaha.114.009309
5. Riedel M, Stanek V, Widimsky J, Prerovsky I. Longterm follow-up of patients with pulmonary thromboembolism. Late prognosis and evolution of hemodynamic and respiratory data. Chest. (1982) 81:151–8. doi: 10.1378/chest.81.2.151
6. Galiè N, Humbert M, Vachiery JL, Gibbs S, Lang I, Torbicki A, et al. 2015 ESC/ERS guidelines for the diagnosis and treatment of pulmonary hypertension: the joint task force for the diagnosis and treatment of pulmonary hypertension of the European Society of Cardiology (ESC) and the European Respiratory Society (ERS): endorsed by: association for European Paediatric and Congenital Cardiology (AEPC), International Society for Heart and Lung Transplantation (ISHLT). Eur Respir J. (2015) 46:903–75. doi: 10.1183/13993003.01032-2015
7. Cannon JE, Su L, Kiely DG, Page K, Toshner M, Swietlik E, et al. Dynamic risk stratification of patient long-term outcome after pulmonary endarterectomy: results from the United Kingdom National Cohort. Circulation. (2016) 133:1761–71. doi: 10.1161/circulationaha.115.019470
8. Delcroix M, Lang I, Pepke-Zaba J, Jansa P, D'Armini AM, Snijder R, et al. Long-term outcome of patients with chronic thromboembolic pulmonary hypertension: results from an international prospective registry. Circulation. (2016) 133:859–71. doi: 10.1161/circulationaha.115.016522
9. Gustavo A, Heresi MD, Nancy B. Chronic thromboembolic pulmonary hypertension. Am J Resp Crit Care. (2018) 197:P5–6. doi: 10.1164/rccm.1974P5
10. Nabozny MJ, Barnato AE, Rathouz PJ, Havlena JA, Kind AJ, Ehlenbach WJ, et al. Trajectories and prognosis of older patients who have prolonged mechanical ventilation after high-risk surgery. Crit Care Med. (2016) 44:1091–7. doi: 10.1097/ccm.0000000000001618
11. Schechter MA, Shah AA, Englum BR, Williams JB, Ganapathi AM, Davies JD, et al. Prolonged postoperative respiratory support after proximal thoracic aortic surgery: is deep hypothermic circulatory arrest a risk factor? J Crit Care. (2016) 31:125–9. doi: 10.1016/j.jcrc.2015.10.021
12. Sharma V, Rao V, Manlhiot C, Boruvka A, Fremes S, Wasowicz M. A derived and validated score to predict prolonged mechanical ventilation in patients undergoing cardiac surgery. J Thorac Cardiovasc Surg. (2017) 153:108–15. doi: 10.1016/j.jtcvs.2016.08.020
13. Vagheggini G, Vlad EP, Mazzoleni S, Bortolotti U, Guarracino F, Ambrosino N. Outcomes for difficult-to-wean subjects after cardiac surgery. Respiratory care. (2015) 60:56–62. doi: 10.4187/respcare.03315
14. Thanavaro J, Taylor J, Vitt L, Guignon MS, Thanavaro S. Predictors and outcomes of postoperative respiratory failure after cardiac surgery. J Eval Clin Pract. (2020) 26:1490–7. doi: 10.1111/jep.13334
15. Anastasian ZH, Gaudet JG, Levitt LC, Mergeche JL, Heyer EJ, Berman MF. Factors that correlate with the decision to delay extubation after multilevel prone spine surgery. J Neurosurg Anesth. (2014) 26:167–71. doi: 10.1097/ana.0000000000000028
16. Flegler S, Paro FM. Factors associated with intubation time and ICU stay after CABG. Braz J Cardiov Surg. (2015) 30:631–5. doi: 10.5935/1678-9741.20150074
17. Saleh HZ, Shaw M, Al-Rawi O, Yates J, Pullan DM, Chalmers JA, et al. Outcomes and predictors of prolonged ventilation in patients undergoing elective coronary surgery. Interact Cardiov Th. (2012) 15:51–6. doi: 10.1093/icvts/ivs076
18. Papathanasiou M, Mincu RI, Lortz J, Horacek M, Koch A, Pizanis N, et al. Prolonged mechanical ventilation after left ventricular assist device implantation: risk factors and clinical implications. ESC heart failure. (2019) 6:545–51. doi: 10.1002/ehf2.12428
19. Bartz RR, Ferreira RG, Schroder JN, Davies J, Liu WW, Camara A, et al. Prolonged pulmonary support after cardiac surgery: incidence, risk factors and outcomes: a retrospective cohort study. J Crit Care. (2015) 30:940–4. doi: 10.1016/j.jcrc.2015.04.125
20. Gumus F, Polat A, Yektas A, Totoz T, Bagci M, Erentug V, et al. Prolonged mechanical ventilation after cabg: risk factor analysis. J Cardiothor Vasc An. (2015) 29:52–8. doi: 10.1053/j.jvca.2014.09.002
21. Hsu H, Lai HC, Liu TJ. Factors causing prolonged mechanical ventilation and peri-operative morbidity after robot-assisted coronary artery bypass graft surgery. Heart vessels. (2019) 34:44–51. doi: 10.1007/s00380-018-1221-6
22. Madani M, Mayer E, Fadel E, Jenkins DP. Pulmonary endarterectomy. Patient selection, technical challenges, and outcomes. Ann Am Thorac Soc. (2016) 13:S240–7. doi: 10.1513/AnnalsATS.201601-014AS
23. Koponen T, Karttunen J, Musialowicz T, Pietiläinen L, Uusaro A, Lahtinen P. Vasoactive-inotropic score and the prediction of morbidity and mortality after cardiac surgery. Brit J Anaesth. (2019) 122:42836. doi: 10.1016/j.bja.2018.12.019
24. Manecke GR Jr. Anesthesia for pulmonary endarterectomy. Semin Thorac Cardiov. (2006) 18:236–42. doi: 10.1053/j.semtcvs.2006.09.005
25. Durrleman S, Simon R. Flexible regression models with cubic splines. Stat Med. (1989) 8:551–61. doi: 10.1002/sim.4780080504
26. Tibshirani R. Regression shrinkage and selection via the Lasso. J R Stat Soc Ser B Methodol. (1996) 58:267–88.
27. Heinze G, Wallisch C, Dunkler D. Variable selection–a review and recommendations for the practicing statistician. Biometrical J. (2018) 60:431–49. doi: 10.1002/bimj.201700067
28. Fernandez-Zamora MD, Gordillo-Brenes A, Banderas-Bravo E, Arboleda-Sánchez JA, Hinojosa-Pérez R, Aguilar-Alonso E, et al. Prolonged mechanical ventilation as a predictor of mortality after cardiac surgery. Resp Care. (2018) 63:550–7. doi: 10.4187/respcare.04915
29. Hadem J, Gottlieb J, Seifert D, Fegbeutel C, Sommer W, Greer M, et al. Prolonged mechanical ventilation after lung transplantation-a single-center study. Am J Transplant. (2016) 16:1579–87. doi: 10.1111/ajt.13632
30. Bailey ML, Richter SM, Mullany DV, Tesar PJ, Fraser JF. Risk factors and survival in patients with respiratory failure after cardiac operations. Ann Thorac Surg. (2011) 92:1573–9. doi: 10.1016/j.athoracsur.2011.04.019
31. Lei Q, Chen L, Zhang Y, Fang N, Cheng W, Li L. Predictors of prolonged mechanical ventilation after aortic arch surgery with deep hypothermic circulatory arrest plus antegrade selective cerebral perfusion. J Cardiothor Vasc An. (2009) 23:495–500. doi: 10.1053/j.jvca.2008.09.006
32. Serrano N, García C, Villegas J, Huidobro S, Henry CC, Santacreu R, et al. Prolonged intubation rates after coronary artery bypass surgery and ICU risk stratification score. Chest. (2005) 128:595–601. doi: 10.1378/chest.128.2.595
33. Chung J, Stevens LM, Ouzounian M, El-Hamamsy I, Bouhout I, Dagenais F, et al. Sex-related differences in patients undergoing thoracic aortic surgery. Circulation. (2019) 139:1177–84. doi: 10.1161/circulationaha.118.035805
34. Butterworth J, James R, Prielipp R, Cerese J, Livingston J, Burnett D. Female gender associates with increased duration of intubation and length of stay after coronary artery surgery. cabg clinical benchmarking database participants. Anesthesiology. (2000) 92:414–24. doi: 10.1097/00000542-200002000-00023
35. Luo HY, Hu KJ, Zhou JY, Wang CS. Analysis of the risk factors of postoperative respiratory dysfunction of type a aortic dissection and lung protection. Perfusion. (2009) 24:199–202. doi: 10.1177/0267659109346671
36. Xie L, Xu Y, Huang G, Ye M, Hu X, Shu S, et al. MHCA with SACP versus DHCA in pediatric aortic arch surgery: a comparative study. Sci Rep-UK. (2020) 10:4439. doi: 10.1038/s41598-020-61428-x
37. Wu Y, Xiao L, Yang T, Wang L, Chen X. Aortic arch reconstruction: deep and moderate hypothermic circulatory arrest with selective antegrade cerebral perfusion. Perfusion. (2017) 32:389–93. doi: 10.1177/0267659116688423
38. Leshnower BG, Myung RJ, Kilgo PD, Vassiliades TA, Vega JD, Thourani VH, et al. Moderate hypothermia and unilateral selective antegrade cerebral perfusion: a contemporary cerebral protection strategy for aortic arch surgery. Ann Thorac Surg. (2010) 90:547–54. doi: 10.1016/j.athoracsur.2010.03.118
39. Vallabhajosyula P, Jassar AS, Menon RS, Komlo C, Gutsche J, Desai ND, et al. Moderate versus deep hypothermic circulatory arrest for elective aortic transverse hemiarch reconstruction. Ann Thorac Surg. (2015) 99:1511–7. doi: 10.1016/j.athoracsur.2014.12.067
40. LaPar DJ, Crosby IK, Ailawadi G, Ad N, Choi E, Spiess BD, et al. Blood product conservation is associated with improved outcomes and reduced costs after cardiac surgery. J Thorac Cardiov Sur. (2013) 145:796–803. doi: 10.1016/j.jtcvs.2012.12.041
41. Preventza O, Coselli JS, Garcia A, Akvan S, Kashyap S, Simpson KH, et al. Aortic root surgery with circulatory arrest: predictors of prolonged postoperative hospital stay. J Thorac Cardiov Sur. (2017) 153:511–8. doi: 10.1016/j.jtcvs.2016.10.090
42. Paone G, Likosky DS, Brewer R, Theurer PF, Bell GF, Cogan CM, et al. Transfusion of 1 and 2 units of red blood cells is associated with increased morbidity and mortality. Ann Thorac Surg. (2014) 97:87–93. doi: 10.1016/j.athoracsur.2013.07.020
43. Tran S, Sullivan PM, Cleveland J, Kumar SR, Takao C. Elevated pulmonary artery pressure, not pulmonary vascular resistance, is an independent predictor of short-term morbidity following bidirectional cavopulmonary connection. Pediatric Cardiol. (2018) 39:1572–80. doi: 10.1007/s00246-018-1932-6
44. Patel R, Thingnam SKS, Mishra AK, Rohit MK, Upadhyay V, Halder V, et al. Benefits of perioperative sildenafil therapy in children with a ventricular septal defect with pulmonary artery hypertension on early surgical outcomes. J Card Surg. (2020) 35:3302–9. doi: 10.1111/jocs.15030
45. Bogaard HJ, Abe K, Vonk Noordegraaf A, Voelkel NF. The right ventricle under pressure: cellular and molecular mechanisms of right-heart failure in pulmonary hypertension. Chest. (2009) 135:794–804. doi: 10.1378/chest.08-0492
Keywords: prolonged mechanical ventilation, chronic thromboembolic pulmonary hypertension, pulmonary endarterectomy, risk factors, outcome
Citation: Zhang C, Yang L, Shi S, Fang Z, Li J and Wang G (2021) Risk Factors for Prolonged Mechanical Ventilation After Pulmonary Endarterectomy: 7 Years' Experience From an Experienced Hospital in China. Front. Surg. 8:679273. doi: 10.3389/fsurg.2021.679273
Received: 11 March 2021; Accepted: 17 May 2021;
Published: 10 June 2021.
Edited by:
Antonio Miceli, Istituto Clinico Sant'Ambrogio, ItalyReviewed by:
Andrea Montisci, Ospedale Civile di Brescia, ItalyCopyright © 2021 Zhang, Yang, Shi, Fang, Li and Wang. This is an open-access article distributed under the terms of the Creative Commons Attribution License (CC BY). The use, distribution or reproduction in other forums is permitted, provided the original author(s) and the copyright owner(s) are credited and that the original publication in this journal is cited, in accordance with accepted academic practice. No use, distribution or reproduction is permitted which does not comply with these terms.
*Correspondence: Guyan Wang, Z3V5YW53YW5nMjAwNkAxNjMuY29t
Disclaimer: All claims expressed in this article are solely those of the authors and do not necessarily represent those of their affiliated organizations, or those of the publisher, the editors and the reviewers. Any product that may be evaluated in this article or claim that may be made by its manufacturer is not guaranteed or endorsed by the publisher.
Research integrity at Frontiers
Learn more about the work of our research integrity team to safeguard the quality of each article we publish.