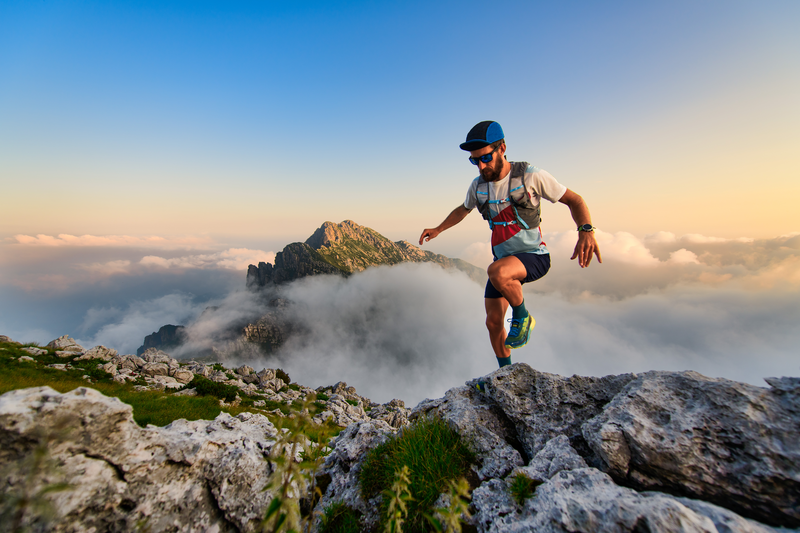
95% of researchers rate our articles as excellent or good
Learn more about the work of our research integrity team to safeguard the quality of each article we publish.
Find out more
MINI REVIEW article
Front. Surg. , 30 April 2018
Sec. Pediatric Orthopedics
Volume 5 - 2018 | https://doi.org/10.3389/fsurg.2018.00036
The incidence of anterior cruciate ligament (ACL) injuries in the pediatric population has risen in recent years. These injuries have historically presented a management dilemma in skeletally immature patients with open physes and significant growth remaining at time of injury. While those nearing skeletal maturity may be treated with traditional, transphyseal adult techniques, these same procedures risk iatrogenic damage to the growth plates and resultant growth disturbances in younger patients with open physes. Moreover, conservative management is non-optimal as significant instabilities of the knee remain. Despite the development of physeal-sparing reconstructive techniques for younger patients, there remains debate over which procedure may be most suitable on a patient to patient basis. Meanwhile, the drivers behind clinical and functional outcomes following ACL reconstruction remain poorly understood. Therefore, current strategies are not yet capable of optimizing surgical ACL reconstruction on an individualized basis with absolute confidence. Instead, aims to improve surgical treatment of ACL tears in skeletally immature patients will rely on additional approaches in the near future. Namely, finite element models have emerged as a tool to model complex knee joint biomechanics. The inclusion of several individualized variables such as bone age, three dimensional geometries around the knee joint, tunnel positioning, and graft tension collectively present a possible means of better understanding and even predicting how to enhance surgical decision-making. Such a tool would serve surgeons in optimizing ACL reconstruction in the skeletally immature individuals, in order to improve clinical outcomes as well as reduce the rate of post-operative complications.
Historically, anterior cruciate ligament (ACL) injuries were thought to be rare in the pediatric population. Instead, tibial eminence avulsion fractures were thought to be more common, as a result of the characteristically non-ossified bone found in adolescents (1). Recent studies however, indicate the incidence of ACL tears in the pediatric population has increased over the last 20 years, with the highest incidence occurring during high school years (2). Increased rates of ACL injuries are linked to both improved diagnostic techniques, as well as increased awareness within the medical community (3).
In the past, skeletally immature patients with ACL tears faced a management dilemma (4). In particular, the physes of the knee are of greatest anatomic concern due to the theoretical risk of physeal damage and growth disturbance. The distal femoral and proximal tibial physes contribute to 37 and 25% of overall limb length, respectively (5). As a result, pubertal status is of the utmost concern when evaluating surgical candidates’ risk of physeal disruption. In particular, sex differences between the age of typical skeletal maturity must be considered. In general, it is well-recognized females reach complete puberty including growth spurt and closure of the physes before their male counterparts. Skeletal maturity is reached at approximately age 14 years in girls, and age 16 in boys. Importantly, however, the amount of growth around the knee that remains after 12–13 years in girls and 14 years in boys is negligible (6). Therefore, until these ages are reached, surgeons must be aware of the growing physes when considering conservative versus operative management, and ultimately which ACL reconstruction strategy to utilize. Meanwhile, it must be kept in mind that each individual does not adhere to these guidelines, which means each case must be carefully analyzed in the setting of the particular patient and their growth status.
As previously mentioned, operative management restores stability of the knee but risks iatrogenic damage to the physis, which may ultimately lead to leg-length discrepancies and angular deformities of the lower extremity (7). Conversely, conservative management consisting of activity modification, rehabilitation exercises, and bracing avoids disruption of the physis, but has been associated with residual instability of the knee (8). These prolonged instabilities have been shown to lead to further complications and injuries, primarily meniscal tears and chondral degeneration (4). Therefore, the current consensus treatment for ACL tears in pediatric patients involves early surgical intervention restoring stability of the knee, which has now been associated with superior outcomes in comparison to conservative management (9).
However, ideal surgical techniques remain controversial due to insufficient data correlating these various techniques to clinical outcomes while taking into account the timing of surgery and rehabilitation regimes (8). The two primary surgical procedures in contention include transphyseal and physeal-sparing techniques. Anatomic, transphyseal reconstruction involves drilling tunnels though both the tibial and femoral physes, and is generally regarded with caution due to the risk of physeal disruption and growth disturbance. Physeal-sparing techniques, however, avoid drilling directly through the physis with modified all-epiphseal tunnels.
The tunnels used in transphyseal reconstructions account for little of the total cross sectional area of the physis. In addition, it has not been proven equivocally that drilling through the physis results in detectable valgus/varus deformities or growth disruption (2). Additionally, the question of which surgical procedure is optimal for each patient is complicated by variables such as the amount of remaining growth at time of surgery, and individual variations in geometry of the knee. Given advancements in finite element modeling capable of simulating biomechanical behavior of the knee joint (10), more sophisticated methods to optimize ACL reconstructions in skeletally immature patients on an individualized basis certainly seems an attainable goal in the relatively near future.
A summary of the following current surgical procedures is provided in Table 1. Previous studies have shown the ACL is composed of two main bundles consisting of the anteromedial (AM) and posterolateral (PL) constituents (15, 16). It has been determined the AM bundle assumes most of the strain in higher flexion angles, while the PL bundle takes up more strain in lower flexion angles (17). Together, the two bundles comprise the ACL, which prevents anterior tibial translation in addition to rotational stresses. Single bundle transtibial ACL reconstructions are often considered a traditional technique for restoring stability lost due to ACL rupture. While biomechanical studies have shown this technique can restore anterior-posterior stability, rotational kinematics are often not completely restored (18). Double bundle transtibial ACL reconstructions have been utilized to better restore stability and more normal knee kinematics (19). While this initially appears far more advantageous than the single bundle reconstructions, double bundle techniques are more technical procedures requiring longer operative times and more substantial bone loss. Overall, while some studies find double bundle reconstructions to provide better results, many other studies have failed to find significant differences in clinical outcomes (20). More recently, independent tunnel drilling for the femoral tunnel has been developed and accepted as a technique to more accurately replicate the anatomic femoral origin of the ACL (21).
As previously described, physeal-sparing techniques avoid disruption of the physes to decrease risk of growth abnormalities. In turn, this decreases the risk of leg length discrepancies and angular deformities. These procedures, however, are not without their disadvantages. Lawrence et al. describes an all-epiphyseal technique with a lateral femoral and oblique tibial tunnel, creating an acute angle the graft must traverse. Ultimately, this may lead to wear and unnecessary stress on the graft, increasing risk of re-rupture (12). To avoid this problem, an extraphyseal technique described by Janarv et al. places the graft in an “over-the-top” femoral position (13). While this technique avoids the graft being subjected to an acute angle, it is inherently non-anatomic and may not fully restore normal knee kinematics and stability.
Finally, partial physeal-sparing techniques (14) have been developed with the hopes of maintaining aspects of an anatomical reconstruction while minimizing risk of growth disturbance. In these procedures, a transphyseal tibial tunnel is drilled, but the femoral tunnel remains all-epiphyseal. As the femoral physis accounts for a larger proportion of limb growth, the avoidance of disrupting this physis is thought to minimize significant growth disturbances.
An additional procedure, which has just recently been developed, includes a hybrid physeal-sparing technique for ACL reconstruction. This technique provides an anatomic reconstruction that minimizes the risk of physeal damage but is also reproducible and less technical than the aforementioned physeal-sparing techniques. Willson et al. posits this technique provides high success rates with low morbidity in adolescents nearing skeletal maturity (22).
In sum, the variety of surgical procedures each provide a set of advantages and disadvantages which must be considered in pediatric patients with ACL ruptures. When choosing which procedure is optimal for a particular patient, factors including skeletal age and 3D geometry of the knee both affect surgical outcome. In regard to skeletal age, a current consensus has been well-described in the literature (23). In pre-pubescent patients with open physes and a great deal of anticipated growth remaining, physeal-sparing techniques are considered the most appropriate approach. To contrast, in adolescents with closed or closing physes, adult transphyseal techniques are regarded most highly due to the relatively low risk of iatrogenic damage and growth disturbances. However, many patients fall somewhere in the middle of this spectrum, and uncertainties often arise in skeletally-immature patients.
As depicted in Table 2, a wide range of complications arises from both traditional transphyseal and physeal-sparing reconstructions. The main concern when performing ACL reconstructions has historically been disruption of the physes, leading to growth arrest. Ultimately, this disruption of growth leads to either leg length discrepancies or angular deformities of the leg (7). More recently, studies have suggested limb overgrowth leading to growth discrepancies may be underreported, and also pose a major clinical problem following ACL reconstruction in adolescents (30). Additional complications include re-rupture of the ACL graft, or even contralateral ACL tear. In fact, recent evidence suggests contralateral ACL tear may be more common than ACL graft rupture in patients following ACL reconstruction (31).
In response to these complications, further surgical intervention or other procedures such as percutaneous epiphysiodesis are often required. Additional procedures not only result in additional financial burden for patients and their families, but also require additional recovery time and further delay until return to normal activities. Moreover, few if any prevention or predictive measures are currently in place to minimize the risk of these adverse outcomes. In addition, patients experience a wide range of functional outcomes affecting their daily lives and ability to reengage in sports or similar activities. These outcomes are assessed via common knee scores such as the International Knee Documentation (IKDC) subjective evaluation and Lysholm knee scores (32). Ideally, surgeons would strive for preventative strategies to minimize the risk of post-operative complications and to maximize clinical knee scores representing functional outcomes. However, the many mechanisms contributing to complications have either not been elucidated or are not clearly understood.
For example, in a systematic review by Collins et al., (33) physeal-sparing techniques counter-intuitively accounted for 25% of angular deformities and 47% of limb length discrepancy cases. This is unexpected, as it has long been thought sparing the physis decreases the risk of growth abnormalities. Furthermore, Collins et al. discovered limb overgrowth accounted for 62% of cases involving limb length discrepancy. Historically, growth arrest due to disruption of the physis has been the main concern after ACL reconstruction. Therefore, it suggests growth abnormalities are most likely under reported in the current literature, in addition to a gross lack of understanding underlying the precise causes and mechanisms leading to growth abnormalities following ACL reconstruction.
Meanwhile, it has been shown the 3D geometry of the distal femoral condyles has significant effects on knee joint kinematics (34). Therefore, it is likely individual differences in condylar shape and contour affect post-operative outcomes, in addition to the aforementioned skeletal age. As will next be discussed, current surgical procedures fail to address individual differences in condylar geometry during the procedure. Ultimately, this may lead to differences in tunnel orientations, angles, and positions between patients, which further contribute to post-operative complications and outcomes.
Pena et al. have previously shown the angle of the femoral tunnel primarily affects tension of the graft, while the tibial tunnel significantly affects laxity and meniscal stresses (35). For example, tibial drill-guide angles between 55 and 65 degrees have been proposed as optimal angles for proper stress redistribution (36). Moreover, the graft tension is vitally important as enough tension is required to maintain stability, while a mechanism has been proposed where too much tension leads to physeal compression and ultimately damage (33). Furthermore, changes in tunnel positioning may take place in skeletally immature patients as growth around the knee potentially impacts reconstruction, which likely has direct impacts on in vivo graft tension as well. Of significance, a previous study has utilized multidector-row CT to demonstrate bone tunnel changes over time. Specifically, changes in angular geometry and actual movement of the articular outlets of tibial and femoral tunnels were measured at 1 year postoperatively compared to 3 weeks following the procedure (37). Therefore, it can be assumed tunnel position, angles, and draft tension cumulatively affect knee kinematics and functional outcomes.
Currently, a standard 7-mm-offset femoral tunnel guide is used to set the femoral guide pin in most conventional transphyseal ACL reconstructions. However, several studies such as Chung et al. (38) have suggested using a 10-mm-offset tunnel guide better enables the placement of a more anatomically positioned femoral tunnel which leads to greater stability, fewer complications, and better overall functional outcomes. This suggests very small differences in the positioning or placement of the guide pin have the potential to have tangible effects on clinical outcomes following ACL reconstructions. Meanwhile, it remains to be investigated how variation in individual distal femoral condyle 3D geometry and contour may affect positioning of the tunnel guide and guide pin. Based on Chung et al. suggesting even slight variation in the offset guide has important effects on tunnel positioning mimicking anatomical ACL placement, it is logical that small variations in distal femoral condyle geometry may affect tunnel angle and positioning as well. Although this remains to be investigated throughout the literature, anatomical variation in 3D geometry at the distal condyle likely effects tunnel placement, and in turn, has implications for vitally important clinical outcomes.
As previously illustrated, graft tension has important consequences on stability, functional outcomes, and complications such as graft rupture. However, the ideal graft tension which is determined during reconstruction remains a topic of contention within the surgical community. Most surgeons apply a “sufficient magnitude” of tension between 40 to 90 n to the graft at full extension (39). Kondo et al. illustrated how different tensioning strategies influenced functional outcomes such as knee flexion at 2 years of follow-up (40). Therefore, the great deal of variation in current graft tensioning protocols lends itself as a possible candidate to explain poorly understood differences in clinical outcomes. Additional factors compound the complexity of graft tension. For example, different graft types including patellar ligaments and hamstring tendons have been shown to exhibit different biomechanical properties such as strength and elasticity in long term follow-up studies (41).
Due to the ability of graft tension to affect functional outcomes, the need to better understand the many variables affecting tension after ACL reconstruction becomes imperative. Due to the fact that femoral condylar contour affects knee kinematics, it is plausible to conceive the 3D geometry has effects on long term graft tension. If these effects could be predicted through a computational model, graft tensioning protocols could be optimized to set initial graft tension more precisely to reduce post-surgical complications. Importantly, recent studies have validated the finite element modeling of such phenomena. Specifically, Pena et al. have demonstrated the plausibility of such modeling by using finite element analysis to study the effect of graft stiffness and graft tensioning on ACL reconstruction (42).
For decades, the biomechanics of the knee joint have been simulated by numerical means. Nonetheless, the intricate ability of the knee joint to provide both stability under high loads and simultaneous mobility continues to provide a challenging task to accurately model. Recently, modern non-linear, finite element models based on MRI have been shown to represent the knee with a “high degree of anatomical realism” (10). These models have since been developed to predict progressive aspects such degenerative pathology or surgical reconstruction. Most importantly, several studies have proven the ability of finite element analysis to model surgical repair and replacement strategies in relation to the knee (43–45). A review by Galbusera et al. highlights the progression and current utility of these models (10). Thus the simulation of the knee following surgical reconstruction is now achievable through the widespread validation of non-linear finite element packages and modern software with powerful capabilities to simulate the complex biomechanics of knee ligaments.
As previously illustrated, several factors pose the potential to be incorporated into finite element models to aid in the optimization of ACL reconstruction. For example, tunnel placement is of the foremost importance, but if and how it is affected by individual three-dimensional geometry of the knee would be a powerful modeling capability. Furthermore, skeletally immature patients pose the problem of growth around the knee taking place following reconstruction, which may resultantly alter tunnel positioning. Therefore, if bone age was to be incorporated into finite element models, the possibility of predicting tunnel position shifts could perhaps be anticipated to allow for optimal final tunnel location upon completion of growth around the knee. Finally, the many variables associated with graft tension such as tunnel angles, length, graft type, and tension set during the procedure, all have the potential to serve as valuable variables to better predict functional and clinical outcomes.
The clinical significance of a predictive computational model capable of optimizing surgical outcomes are robust and far-reaching. Such an algorithm would not only allow surgeons to plan individualized procedures with unprecedented insight and ability, but would significantly increase functional outcomes while minimizing the risk of complications. With a greater understanding and ability to predict the effects of individual 3D geometry of the knee joint on tunnel positioning and graft tension, surgical techniques could be adjusted for individuals using pre-operative MRI data. Such a powerful tool may additionally be able to shorten surgical times based on pre-operative planning, reduce recovery time, and shorten rehabilitation regimens as patients experienced optimized reconstructions allowing for fast and complication-free recoveries.
The usefulness and validity of 3D-imaging of the knee was first established years ago by utilizing CT evaluation of tunnel placement (46). More recently, several studies have begun to highlight the utility of finite element modeling. For example, finite element analysis has been utilized to investigate the effects of tunnel orientation and stress distribution in ACL grafts (47), as well as to investigate the optimization of graft placement in ACL reconstruction (48). These studies provide early evidence of finite-element analysis aiding in the optimization of ACL reconstruction. The main limitation to these models is obviously the potential cost, which would potentially pose a barrier to widespread use on an individualized basis. Nonetheless, the development of select, cornerstone models in well-designed studies may be enough to glean useful information that can be applied to individual cases.
Within the pediatric population, ACL injuries are becoming increasingly common. Current consensus suggests early reconstruction restores joint stability and is associated with superior long-term outcomes in comparison to solely conservative management. While physeal-sparing techniques have been developed for younger patients with significant growth remaining, these procedures have not eliminated the risks of post-operative complications and still pose distinct disadvantages. Therefore, in an effort to optimize ACL reconstruction in skeletally immature patients, the development and incorporation of finite element models poses a possible future direction. These models may potentially guide surgeons in choosing surgical techniques by including several individualized variables to allow optimal tunnel positioning, graft tension, and resulting biomechanics of the knee. Through these methods, the goal to continually strive to improve outcomes and reduce complications may be achieved.
This study was reviewed and approved by the applicable IRB/ECs with respect to scientific content and compliance with applicable research and human subjects regulations.
All authors have met the criteria for authorship. They have made substantial contribution, have read and approved of the final submitted work, as well as agree to be accountable for all aspects of the work ensuring questions related to accuracy or integrity are appropriately investigated and resolved.
The authors declare that the research was conducted in the absence of any commercial or financial relationships that could be construed as a potential conflict of interest.
ACL, anterior cruciate ligament; AM, Anteromedial; PL, Posterolateral.
1. Kocher MS, Garg S, Micheli LJ, Beck NA, Lawrence JTR, Nordin JD. Physeal sparing reconstruction of the anterior cruciate ligament in skeletally immature prepubescent children and adolescents. J Bone Joint Surg Am (2005) 87(11):2371–9. doi: 10.2106/JBJS.D.02802
2. Beck NA, Lawrence JTR, Nordin JD, Defor TA, Tompkins M. ACL tears in school-aged children and Adolescents over 20 years. Pediatrics (2017) 139(3):e20161877. doi: 10.1542/peds.2016-1877
3. Adirim TA, Cheng TL. Overview of injuries in the young athlete. Sports Med (2003) 33(1):75–81. doi: 10.2165/00007256-200333010-00006
4. Mohtadi N, Grant J. Managing anterior cruciate ligament deficiency in the skeletally immature individual: a systematic review of the literature. Clin J Sport Med (2006) 16(6):457–64. doi: 10.1097/01.jsm.0000248844.39498.1f
5. Fabricant PD, Kocher MS. Anterior cruciate ligament injuries in children and adolescents. Orthop Clin North Am (2016) 47(4):777–88. doi: 10.1016/j.ocl.2016.05.004
6. Kelly PM, Diméglio A. Lower-limb growth: how predictable are predictions? J Child Orthop (2008) 2(6):407–15. doi: 10.1007/s11832-008-0119-8
7. Kaeding CC, Flanigan D, Donaldson C. Surgical techniques and outcomes after anterior cruciate ligament reconstruction in preadolescent patients. Arthroscopy (2010) 26(11):1530–8. doi: 10.1016/j.arthro.2010.04.065
8. Al-Hadithy N, Dodds AL, Akhtar KS, Gupte CM. Current concepts of the management of anterior cruciate ligament injuries in children. Bone Joint J (2013) 95-B(11):1562–9. doi: 10.1302/0301-620X.95B11.31778
9. Davis DL, Chen L, Ehinger M. A study of epiphyses in the young prepubescent knee using magnetic resonance imaging: evaluation of parameters for anterior cruciate ligament reconstruction. Orthop J Sports Med (2014) 2(4):2325967114530090. doi: 10.1177/2325967114530090
10. Galbusera F, Freutel M, Dürselen L, D'Aiuto M, Croce D, Villa T, et al. Material models and properties in the finite element analysis of knee ligaments: a literature review. Front Bioeng Biotechnol (2014) 2:54. doi: 10.3389/fbioe.2014.00054
11. van Eck CF, Lesniak BP, Schreiber VM, Fu FH. Anatomic single- and double-bundle anterior cruciate ligament reconstruction flowchart. Arthroscopy (2010) 26(2):258–68. doi: 10.1016/j.arthro.2009.07.027
12. Lawrence JT, Bowers AL, Belding J, Cody SR, Ganley TJ. All-epiphyseal anterior cruciate ligament reconstruction in skeletally immature patients. Clin Orthop Relat Res (2010) 468(7):1971–7. doi: 10.1007/s11999-010-1255-2
13. Janarv PM, Nyström A, Werner S, Hirsch G. Anterior cruciate ligament injuries in skeletally immature patients. J Pediatr Orthop (1996) 16(5):673–7.
14. Lipscomb AB, Anderson AF. Tears of the anterior cruciate ligament in adolescents. J Bone Joint Surg Am (1986) 68(1):19–28. doi: 10.2106/00004623-198668010-00004
15. Girgis FG, Marshall JL, Monajem A. The cruciate ligaments of the knee joint. Anatomical, functional and experimental analysis. Clin Orthop Relat Res (1975) 106(106):216–31.
16. Yasuda K, van Eck CF, Hoshino Y, Fu FH, Tashman S. Anatomic single- and double-bundle anterior cruciate ligament reconstruction, part 1: Basic science. Am J Sports Med (2011) 39(8):1789–800. doi: 10.1177/0363546511402659
17. Sakane M, Fox RJ, Woo SL, Livesay GA, Li G, Fu FH. In situ forces in the anterior cruciate ligament and its bundles in response to anterior tibial loads. J Orthop Res (1997) 15(2):285–93. doi: 10.1002/jor.1100150219
18. Fu FH, Jordan SS. The lateral intercondylar ridge--a key to anatomic anterior cruciate ligament reconstruction. J Bone Joint Surg Am (2007) 89(10):2103–4. doi: 10.2106/JBJS.G.00851
19. Abebe ES, Moorman CT, Dziedzic TS, Spritzer CE, Cothran RL, Taylor DC, et al. Femoral tunnel placement during anterior cruciate ligament reconstruction: an in vivo imaging analysis comparing transtibial and 2-incision tibial tunnel-independent techniques. Am J Sports Med (2009) 37(10):1904–11. doi: 10.1177/0363546509340768
20. Xu M, Gao S, Zeng C, Han R, Sun J, Li H, et al. Outcomes of anterior cruciate ligament reconstruction using single-bundle versus double-bundle technique: meta-analysis of 19 randomized controlled trials. Arthroscopy (2013) 29(2):357–65. doi: 10.1016/j.arthro.2012.08.024
21. Giron F, Cuomo P, Edwards A, Bull AM, Amis AA, Aglietti P. Double-bundle "anatomic" anterior cruciate ligament reconstruction: a cadaveric study of tunnel positioning with a transtibial technique. Arthroscopy (2007) 23(1):7–13. doi: 10.1016/j.arthro.2006.08.008
22. Willson RG, Kostyun RO, Milewski MD, Nissen CW. Anterior cruciate ligament reconstruction in skeletally immature patients: early results using a hybrid physeal-sparing technique. Orthop J Sports Med (2018) 6(2):2325967118755330:232596711875533. doi: 10.1177/2325967118755330
23. Lang PJ, Sugimoto D, Micheli LJ. Prevention, treatment, and rehabilitation of anterior cruciate ligament injuries in children. Open Access J Sports Med (2017) 8:133–41. doi: 10.2147/OAJSM.S133940
24. Kumar S, Ahearne D, Hunt DM. Transphyseal anterior cruciate ligament reconstruction in the skeletally immature: follow-up to a minimum of sixteen years of age. J Bone Joint Surg Am (2013) 95(1):e1. doi: 10.2106/JBJS.K.01707
25. Kocher MS, Smith JT, Zoric BJ, Lee B, Micheli LJ. Transphyseal anterior cruciate ligament reconstruction in skeletally immature pubescent adolescents. J Bone Joint Surg Am (2007) 89(12):2632–9. doi: 10.2106/JBJS.F.01560
26. Edwards PH, Grana WA. Anterior cruciate ligament reconstruction in the immature athlete: long-term results of intra-articular reconstruction. Am J Knee Surg (2001) 14(4):232–7.
27. Chotel F, Henry J, Seil R, Chouteau J, Moyen B, Bérard J. Growth disturbances without growth arrest after ACL reconstruction in children. Knee Surg Sports Traumatol Arthrosc (2010) 18(11):1496–500. doi: 10.1007/s00167-010-1069-5
28. Shifflett GD, Green DW, Widmann RF, Marx RG. Growth Arrest Following ACL Reconstruction With Hamstring Autograft in Skeletally Immature Patients: A Review of 4 Cases. J Pediatr Orthop (2016) 36(4):355–61. doi: 10.1097/BPO.0000000000000466
29. Kocher MS, Garg S, Micheli LJ. Physeal sparing reconstruction of the anterior cruciate ligament in skeletally immature prepubescent children and adolescents. Surgical technique. J Bone Joint Surg Am (2006) 88(Suppl 1 Pt 2):283–93. doi: 10.2106/00004623-200609001-00012
30. Koch PP, Fucentese SF, Blatter SC. Complications after epiphyseal reconstruction of the anterior cruciate ligament in prepubescent children. Knee Surg Sports Traumatol Arthrosc (2016) 24(9):2736–40. doi: 10.1007/s00167-014-3396-4
31. Magnussen RA, Meschbach NT, Kaeding CC, Wright RW, Spindler KP. ACL Graft and Contralateral ACL Tear Risk within Ten Years Following Reconstruction: A Systematic Review. JBJS Rev (2015) 3(1):1. doi: 10.2106/JBJS.RVW.N.00052
32. Collins NJ, Misra D, Felson DT, Crossley KM, Roos EM. Measures of knee function: International Knee Documentation Committee (IKDC) Subjective Knee Evaluation Form, Knee Injury and Osteoarthritis Outcome Score (KOOS), Knee Injury and Osteoarthritis Outcome Score Physical Function Short Form (KOOS-PS), Knee Outcome Survey Activities of Daily Living Scale (KOS-ADL), Lysholm Knee Scoring Scale, Oxford Knee Score (OKS), Western Ontario and McMaster Universities Osteoarthritis Index (WOMAC), Activity Rating Scale (ARS), and Tegner Activity Score (TAS). Arthritis Care Res (Hoboken). (2011) 63(Suppl 11):S208–28. doi: 10.1002/acr.20632
33. Collins MJ, Arns TA, Leroux T, Black A, Mascarenhas R, Bach BR, et al. Growth Abnormalities Following Anterior Cruciate Ligament Reconstruction in the Skeletally Immature Patient: A Systematic Review. Arthroscopy (2016) 32(8):1714–23. doi: 10.1016/j.arthro.2016.02.025
34. Siebold R, Axe J, Irrgang JJ, Li K, Li K, Tashman S, , et al. A computerized analysis of femoral condyle radii in ACL intact and contralateral ACL reconstructed knees using 3D CT. Knee Surg Sports Traumatol Arthrosc (2010) 18(1):26–31. doi: 10.1007/s00167-009-0969-8
35. Peña E, Calvo B, Martinez MA, Palanca D, Doblaré M. Influence of the tunnel angle in ACL reconstructions on the biomechanics of the knee joint. Clin Biomech (2006) 21(5):508–16. doi: 10.1016/j.clinbiomech.2005.12.013
36. Yao J, Wen CY, Zhang M, Cheung JT, Yan C, Chiu KY, et al. Effect of tibial drill-guide angle on the mechanical environment at bone tunnel aperture after anatomic single-bundle anterior cruciate ligament reconstruction. Int Orthop (2014) 38(5):973–81. doi: 10.1007/s00264-014-2290-5
37. Araki D, Kuroda R, Matsumoto T, Nagamune K, Matsushita T, Hoshino Y, et al. Three-dimensional analysis of bone tunnel changes after anatomic double-bundle anterior cruciate ligament reconstruction using multidetector-row computed tomography. Am J Sports Med (2014) 42(9):2234–41. doi: 10.1177/0363546514540274
38. Chung JY, Ha CW, Lee DH, Park YG, Park YB, Awe SI. Anatomic placement of the femoral tunnel by a modified transtibial technique using a large-offset femoral tunnel guide: A cadaveric study. Knee (2016) 23(4):659–65. doi: 10.1016/j.knee.2015.10.001
39. Cunningham R, West JR, Greis PE, Burks RT. A survey of the tension applied to a doubled hamstring tendon graft for reconstruction of the anterior cruciate ligament. Arthroscopy (2002) 18(9):983–8. doi: 10.1053/jars.2002.36102
40. Kondo E, Yasuda K, Kitamura N, Onodera J, Yokota M, Yagi T, et al. Effects of initial graft tension on clinical outcome after anatomic double-bundle anterior cruciate ligament reconstruction: comparison of two graft tension protocols. BMC Musculoskelet Disord (2016) 17:65. doi: 10.1186/s12891-016-0909-y
41. Biuk E, Zelic Z, Rapan S, Curic G, Biuk D, Radic R. Analysis of biomechanical properties of patellar ligament graft and quadruple hamstring tendon graft. Injury (2015) 46(Suppl 6):S14–17. doi.
42. Pena E, Martinez MA, Calvo B, Palanca D, Doblare M. A finite element simulation of the effect of graft stiffness and graft tensioning in ACL reconstruction. Clin Biomech (2005) 20(6):636–44.
43. Yoon KH, Kim YH, Ha JH, Kim K, Park WM. Biomechanical evaluation of double bundle augmentation of posterior cruciate ligament using finite element analysis. Clin. Biomech. (2010) 25:1042–6.
44. Fitzpatrick CK, Komistek RD, Rullkoetter PJ. Developing simulations to reproduce in vivo fluoroscopy kinematics in total knee replacement patients. J Biomech (2014) 47(10):2398–405. doi: 10.1016/j.jbiomech.2014.04.024
45. Innocenti B, Bilgen ÖF, Labey L, van Lenthe GH, Sloten JV, Catani F. Load sharing and ligament strains in balanced, overstuffed and understuffed UKA. A validated finite element analysis. J Arthroplasty (2014) 29(7):1491–8. doi: 10.1016/j.arth.2014.01.020
46. Forsythe B, Kopf S, Wong AK, et al. The location of femoral and tibial tunnels in anatomic double-bundle anterior cruciate ligament reconstruction analyzed by three-dimensional computed tomography models. J Bone Joint Surg Am (2010) 92(6):1418–26.
Keywords: anterior cruciate ligament, injury, reconstruction, adolescents, modeling
Citation: Richter DJ, Lyon R, Van Valin S and Liu X (2018). Current Strategies and Future Directions to Optimize ACL Reconstruction in Adolescent Patients. Front. Surg. 5:36. doi: 10.3389/fsurg.2018.00036
Received: 07 October 2017; Accepted: 19 April 2018;
Published: 30 April 2018
Edited by:
Klane K. White, University of Washington, United StatesReviewed by:
Dominick Tuason, Pediatric Orthopaedic Associates of New Jersey, United StatesCopyright © 2018 Richter, Lyon, Van Valin and Liu. This is an open-access article distributed under the terms of the Creative Commons Attribution License (CC BY). The use, distribution or reproduction in other forums is permitted, provided the original author(s) and the copyright owner are credited and that the original publication in this journal is cited, in accordance with accepted academic practice. No use, distribution or reproduction is permitted which does not comply with these terms.
*Correspondence: Xue-Cheng Liu, eGxpdUBjaHcub3Jn
Disclaimer: All claims expressed in this article are solely those of the authors and do not necessarily represent those of their affiliated organizations, or those of the publisher, the editors and the reviewers. Any product that may be evaluated in this article or claim that may be made by its manufacturer is not guaranteed or endorsed by the publisher.
Research integrity at Frontiers
Learn more about the work of our research integrity team to safeguard the quality of each article we publish.