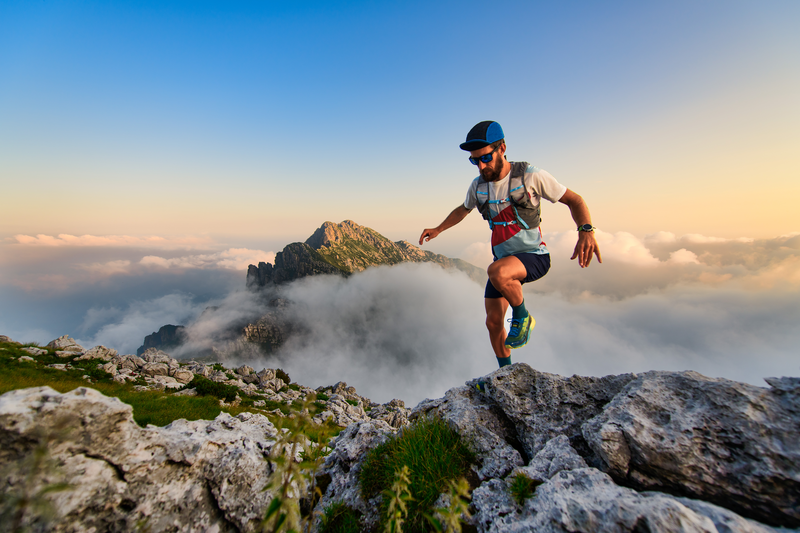
94% of researchers rate our articles as excellent or good
Learn more about the work of our research integrity team to safeguard the quality of each article we publish.
Find out more
REVIEW article
Front. Stroke , 14 June 2024
Sec. Mechanisms, Models, and Biomarkers of Stroke
Volume 3 - 2024 | https://doi.org/10.3389/fstro.2024.1382379
Ischemic stroke is a more common type of stroke and a leading cause of physical disability, cognitive decline, and death worldwide. Events occurring after an ischemic stroke episode determine the severity and outcomes. The NLR family pyrin domain containing 3 (NLRP3) inflammasome has emerged as a major contributor to the pathogenesis of ischemic stroke. Understanding its role in propagating ischemic injury is cardinal for therapeutic interventional research. In this review we summarize the current understanding of the underlying role of the NLRP3 inflammasome as well as highlight the current strides made in targeting the inflammasome as a modality to attenuate the effects of ischemic injury on brain tissue after a stroke event. We found that ischemic stroke initiates a cascade of complex intracellular processes beginning with oxidative stress that activates the nuclear factor kappa-light-chain-enhancer of activated B cells (NF-κB) consequentially activating the NLRP3 inflammasome. The NLRP3 inflammasome initiates inflammatory responses that exacerbate ischemic stroke. We have also briefly summarized the role of genetic susceptibility in stroke and its potential usage in clinical settings. Briefly, genetic mutations encoding the NLRP3 inflammasome are linked to stroke prognosis. A combination of advanced genetic testing and risk stratification based on sociodemographic, dietary, and lifestyle factors is encouraged for stroke prevention. IL-1β and IL-18 antagonists have been shown to inhibit the NLRP3 inflammasome consequently attenuating the adverse effects of ischemic stroke.
Stroke is the second leading cause of death and disability worldwide accounting for more than 5.5 million deaths each year with the bulk of the global stroke burden in low-and-middle-income (LMICs) (Lopez et al., 2006; Feigin et al., 2022). There are two major categories of stroke, ischemic and hemorrhagic. Ischemic stroke is characterized by occlusion of a blood vessel and supply to a part of the brain leading to loss of function (Donkor, 2018). Haemorrhagic stroke is characterized by rupture of a blood vessel in the brain (Donkor, 2018). In some cases, a transient ischemic attack (TIA) can occur and is characterized by blockage of blood flow to the brain occurring only for a short time (<5 min). Of the two major categories of shock, Ischemic shock is more prevalent (Johnston et al., 2009) and is projected to increase by 2030 (Pu et al., 2023).
The common risk factors for both ischemic and haemorrhagic stroke include but not limited to hypertension, cardiovascular diseases, diabetes mellitus, hypercholesterolemia, atrial fibrillation, smoking, alcohol consumption, old age, female sex, use of oral contraceptives, hyperuricemia, chronic bronchitis, periodontal disease, bacterial and viral infections, and poor dietary lifestyle including high salt intake, race and ethnicity, family history and genetic factors (Connor et al., 2007; Owolabi et al., 2009; Palm et al., 2009; O'Donnell et al., 2010; Li et al., 2012; Pu et al., 2023).
The underlying pathophysiology of ischemic stroke stems from the fact that occlusion of blood supply affects brain cell function resulting in hypoxia and hypoglycemia of the affected tissue leading to an infarction (Doyle et al., 2008; Donkor, 2018). In hemorrhagic stroke the hematoma causes an increase in intracranial pressure and the metabolic waste from the ruptured vessel including the formed clots and a complex interaction involving edema, inflammation and oxidative stress contribute to tissue injury and death of brain cells (Magid-Bernstein et al., 2022). In ischemic stroke, the NLR family pyrin domain containing 3 (NLRP3) inflammasome has been reported to play a critical role in contributing to the ischemic injury following blood vessel occlusion by increasing oxidative stress and the inflammatory response (Chen et al., 2019). However, the underlying mechanisms are not well elaborated. The risk and severity of Ischemic stroke and the role of the NLRP3 inflammasome are to a degree dependent on genetic polymorphisms in the genes encoding the NLRP3 inflammasome as well as specific single gene disorders associated primarily with the development of stroke, or polymorphisms resulting in disease conditions where stroke is a common manifestation. Understanding this genetic component is critical for specific therapeutic interventional modalities to prevent and reduce the risk of stroke. Moreover, identification of mutations encoding the NLRP3 inflammasome is important in understanding its role, phenotypic expression, and susceptibility to stroke.
In this review, we discuss in detail, the role of the NLRP3 inflammasome in the pathogenesis of ischemic stroke and later provide a brief current understanding of genetic susceptibility to ischemic stroke.
The main pathological mechanisms associated with cell injury and death in cerebral ischemic stroke are atherosclerosis, endothelial dysfunction, and neuroinflammation (Tuttolomondo et al., 2020). These mechanisms are mediated by several cellular pathological processes such as oxidative stress, inflammation, ATP depletion, mitochondrial damage, influx of Ca2+, defects in membrane permeability, and damage to DNA and intracellular proteins and organelles, all resulting in reversible and irreversible endothelial, glia, and neuronal cell injury depending on the nature and severity of the ischemia (Anrather and Iadecola, 2016).
Of the two main prominent cell types of the brain, glial cells and the neurons (Siletti et al., 2023), neurons are more susceptible to ischemic injury from cerebral ischemia (Won et al., 2022). Occlusion of a blood vessel in the brain leads to lack of oxygen and glucose required for major metabolic pathways such as the mitochondria oxidative phosphorylation that provide adenosine triphosphates (ATPs) needed by the cell (Ham and Raju, 2017; Huang J. et al., 2023). Reduced oxidative phosphorylation results in ATP depletion that has multiple pathological consequences for the brain cells (Guo et al., 2013; Clemente-Suárez et al., 2023). Lack of ATP causes a disruption of the Na+/K+ ATPase pump that leads to increased influx of Ca2+, water and sodium and increased efflux of K+ resulting in endoplasmic reticulum swelling, cellular swelling and loss of microvilli blebs (Gusarova et al., 2011; Miller and Zachary, 2017). Influx of Ca2+ via Ca2+ release-activated Ca2+ (CRAC) channels and release of Ca2+ from the endoplasmic reticulum during hypoxia is mainly mediated by the activation of AMP-activated kinase α1 (AMPK-α1) through Ca2+/calmodulin-dependent kinase β (CaMKKβ) and redistribution of the stromal interaction molecule 1 (STIM1) to the plasma membrane- endoplasmic reticulum junctions (Gusarova et al., 2011). Cellular swelling leads to cytotoxic edema and cell death (Brouns and De Deyn, 2009) (Figure 1). Suffice to say that these pathological events are common to all cells during ischemia, neurons and almost all brain cells are more susceptible to cell death and adverse outcomes following an ischemic stroke episode.
Figure 1. General cell death mechanisms in ischemic stroke. During an ischemic stroke when blood supply is cut off to an area of the brain, affected neurons go through a series of pathological changes leading to cell death by necrosis and elicit inflammatory responses that further exacerbate and accelerate injury. Lack of oxygen reduces oxidative phosphorylation (ATP production) in the mitochondria and lead to mitochondria dysfunction and oxidative stress that contributes to production of reactive oxygen species (ROS). Reduced ATP production leads to reduced protein synthesis, swelling of the endoplasmic reticulum and malfunction of the Na+/K+ ATPase pump. Reduced activity of the Na+/K+ ATPase pump results in increased Na+ and water retention in the cell causing swelling of intracellular organelles. ATP reduction or depletion leads to influx of calcium from outside the cell and its release from the ER mediated by AMP-activated kinase. Ca2+ activates protein kinase c (PKC). PKC activates the enzyme NADPH oxidase which increases activity and production of ROS. ROS activate the nuclear factor kappa-light-chain-enhancer of activated B cells (NF-κB) which activates the NLRP3 inflammasome and both contribute to the production of interleukin 1 beta (IL-1β) and IL-18 resulting in inflammatory responses. Cell death arising from these necrotic neurons also elicit an inflammatory response that recruits inflammatory cells, exacerbating cell injury. Created with BioRender.com.
The metabolic consequences of reduced ATP production results in increased anaerobic metabolism leading to increased lactic acid and clumping of nuclear chromatin (Miller and Zachary, 2017). Reduction in ATP also leads to detachment of ribosomes from the rough endoplasmic reticulum, consequentially leading to reduced protein synthesis, phospholipid synthesis and reacylation, membrane damage causing leakage of cellular contents that elicit an inflammatory response (Miller and Zachary, 2017; Almanza et al., 2019; Perkins and Allan, 2021). Ischemia is also associated with increased production of reactive oxygen species (ROS) due to deranged metabolic systems, and this in turn results in an oxidative burst by injured cells that are trying to survive (Olmez and Ozyurt, 2012; Rodrigo et al., 2013; Elsayed et al., 2020). ROS peroxidize intracellular proteins, lipids, and cellular organelles such as lysosomes that leak their digestive contents to the outside resulting in Ischemic cell death by necrosis (Alu et al., 2020; Ammendolia et al., 2021; He et al., 2023).
Several pro-inflammatory mediators such as IL1β, IL1α, TNF-α, leukotrienes, and chemokines including chemokine (C-X-C motif) ligand 4 (CXCL4), chemokine (C-C motif) ligand 5 (CCL5), CXCL7, CXCL8, chemokine (C-X3-C motif) ligand 1 (CX3CL1), adhesion molecules, proteases, prostanoids, inducible nitric oxide synthase (iNOS), cyclooxygenase 2 (COX-2), are heightened in stroke suggesting their pathological role (Puleo et al., 2022). The inflammatory response in ischemic stroke is responsible for the secondary injury that develops in a delayed manner (Brouns and De Deyn, 2009). The first step associated with inflammation in ischemic stroke is the activation of microglia and endothelial cells (Stoll, 2002) which is followed by infiltration of innate cells such as neutrophils, macrophages, natural killer cells and T lymphocytes that release cytokines, ROS and cytotoxic compounds responsible for further injury to ischemic cells (Wang et al., 2007; Fu et al., 2015). When endothelial cells are activated, they increase the expression of adhesion molecules such as E-selectin, P-selectin, vascular cell adhesion protein 1 (VCAM-1) and intercellular adhesion molecule 1 (ICAM-1) that lead to leukocyte rolling, recruitment, and finally, diapedesis through the vascular wall (Wang et al., 1994; Huang et al., 2000). Neutrophils are also attracted to the infarcted area during reperfusion via monocyte chemo-attractant protein-1 (MCP-1) secreted by activated glial cells and neurons (Geng et al., 2022). Reperfusion also activates a wide variety of cells like macrophages, neutrophils and natural killer cells that secret more pro-inflammatory cytokines and matrix metalloproteinases which disrupt the blood brain barrier exacerbating cerebral edema and activate iNOS and COX-2 which induce endothelial dysfunction leading to cell death (Yang et al., 2019; Geng et al., 2022; He et al., 2022).
The death of microglia and neuronal cells triggers an inflammatory response from surrounding immune cells via specific sensing mechanisms such as the inflammasome that will be discussed in more detail below.
The NLRP3 Inflammasome is a multimeric protein complex expressed mainly by innate immune cells and a variety of other cells such as microglia, lymphocytes, epithelial cells, osteoblasts, and neurons (Rada et al., 2014; Zahid et al., 2019). The NLRP3 Inflammasome contains three critical domains that regulate inflammatory signaling (Lebreton et al., 2018; Blevins et al., 2022). The three domains include the NLRP3 protein, the apoptosis-associated speck-like protein (ASC) which is an adaptor protein, and a procaspase-1, an effector enzyme (Zoete et al., 2014; Mamantopoulos et al., 2017). The NLRP3 protein is made up of a central nucleotide-binding and oligomerization domain (NOD or NACHT), amino-terminal pyrin domain (PYD) responsible for interacting with ASC and initiating inflammasome assembly, and a C-terminal leucine-rich repeat (LRR) domain (Franchi et al., 2009; Vajjhala et al., 2012). NLRP3 protein is a cytosolic pattern recognition receptor (PRR) that functions as a sensor protein which responds to endogenous stress-generated pathogen-associated molecular patterns (PAMPs) or damage-associated molecular patterns (DAMPs) (Kelley et al., 2019). When stimulated the sensor protein NLRP3 forms a supramolecular molecular complex referred to as “inflammasome” which then activates pro-caspase-1 (Fernandes-Alnemri et al., 2007). Pro-caspase-1 is activated to caspase-1 which in turn catalyzes the cleavage of pro-interleukin-1β (pro-IL-1β) and pro-IL-18 into active IL-1β and IL-18 (Manji et al., 2002). Caspase-1 also cleaves gasdermin D (GSDMD) which induces pyroptosis by forming pores in the plasma membrane of cells releasing DAMPs and eliciting an inflammatory response (Shi et al., 2015). IL-1β and IL-18 induce vasodilation, interferon-gamma (IFN-γ) production and recruitment of immune cells to injured tissues (Dinarello, 2009) (Figure 2). It is important to also highlight that absent-in-melanoma 2 (AIM2), a cytoplasmic sensor, can also interact with the PYD domain and when activated can process and contribute to the secretion of IL-1β and IL-18 (Kumari et al., 2020). AIM2 is also an inflammasome that particularly senses DNA (damaged from cell injury or health cell) in the cytosol and trigger the secretion of IL-1β and IL-18 (Kumari et al., 2020).
Figure 2. NLRP3 inflammasome activation in ischemic stroke. The inflammasome is activated by oxidant stress created by the occlusion. The oxidant stress from the mitochondria arises from a lack of oxygen and nutrients. An abnormal influx and inflow of ions from disrupted ion gated channels and NF-κB also activated the inflammasome complex which in turn activate caspase-1 leading to the activation of IL-1β and il-18. Gasdermin forms pores on the membrane promoting pyroptosis and subsequently, inflammation. The NF-κB pathway is activated via MyD88 signaling. TLR4, toll-like receptor 4; NLRP3, NLR family pyrin domain containing 3; ASC, apoptosis-associated speck-like protein; DAMPs, damage-associated molecular patterns; GSDMD, gasdermin D; NF-κB, nuclear factor kappa-light-chain-enhancer of activated B cells; MyD88, Myeloid differentiation primary response 88. Created with BioRender.com.
PRRs that are known to form inflammasomes include leucine-rich repeat (LRR)-containing proteins (NLR) family members NLRP1, NLRP2, NLRP3, NLRC4, NLRP6, NLRP7, NLRP12, IFI16, pyrin, AIM2, and nucleotide-binding oligomerization domain (NOD) (Elinav et al., 2011; Vladimer et al., 2012; Minkiewicz et al., 2013; Lamkanfi and Dixit, 2014; Sharma and Kanneganti, 2016). Among the variety of inflammasomes known to man, the NLRP3 inflammasome was not the first to be discovered but is the most studied and therefore a focus of this review (Bulté et al., 2023; Xu and Núñez, 2023). Although the NLRP3 inflammasome is central to immune responses against infectious agents, it has been reported to be activated and contribute to conditions that do not necessarily involve infections (Guo et al., 2015).
Generally, the activation of the NLRP3 inflammasome in cell injury requires two complementary signals; the first signal is mediated by nuclear factor kappa-light-chain-enhancer of activated B cells (NF-κB) and mitogen-activated protein kinase (MAPK) signaling pathways that serve to upregulate proteins of the NLRP3 inflammasome complex and the precursors of IL-1β and IL-18, and the second signal serves to assemble the NLRP3 inflammasome complex via activation of NLRP3 and ASC phosphorylation (Abais et al., 2015).
The NLRP3 inflammasome can be activated by a wide range of stimuli including ROS, ionic influx, mitochondrial and lysosomal damage among others (Hornung et al., 2008; Kelley et al., 2019). Events leading to potassium (K+) efflux and depletion of K+ have been reported to activate the NLRP3 inflammasome and lead to the activation of Ca2+ independent phospholipase A2, resulting in IL-1β maturation and release from macrophages and monocytes (Walev et al., 2000; Muñoz-Planillo et al., 2013). NLRP3 inflammasome activation is associated with increases in cytosolic Ca2+ concentrations suggesting that Ca2+ is critical for NLRP3 inflammasome activation (Weber and Schilling, 2014). Multiple stimuli including high intracellular ATP, nigericin, phospholipase C activation via G-protein-coupled receptors (GPCRs) trigger Ca2+ mobilization from extracellular stores via plasma membrane Ca2+ channels, the lumen of the endoplasmic reticulum and lysosomes (Murakami et al., 2012; Weber and Schilling, 2014). Na+ influx and Cl– efflux have also been implicated in NLRP3 inflammasome activation as the blockade of Na+ influx by gramicidin, K+-free medium, or nigericin and inhibition of Cl– efflux by 5-nitro-2-(3-phenylpropylamino) benzoic acid, 4,40-diisothiocyano-2,20-stilbenedisulfonic acid, mefenamic acid, flufenamic acid, and indanyloxyacetic acid 94, inhibits NLRP3 inflammasome activation and IL-1β secretion (Verhoef et al., 2005; Compan et al., 2012; Muñoz-Planillo et al., 2013; Daniels et al., 2016; Tang et al., 2017). ROS from NADPH oxidase, mitochondrial, lysosomes and other cellular organelles have also been reported to activate the NLRP3 inflammasome (Bauernfeind et al., 2011; Nakahira et al., 2011; Zhou et al., 2011).
The NLRP3 inflammasome is well expressed in the brain and spinal cord and during stroke, the member proteins of the NLRP3 inflammasome including ASC, caspase-1, IL-1β and IL-18 were upregulated in vitro and in vivo (Iadecola and Anrather, 2011; Yang-Wei Fann et al., 2013; Creagh, 2014; Zhao et al., 2021).
Ischemic stroke has been reported to induce activation of the NF-κB and MAPK signaling pathways in ischemic neurons in the brain promoting NLRP3 Inflammasome activation and subsequent neuronal cell death and brain injury (Fann et al., 2018). The activation of the NF-κB occurs following the priming signal where cellular debris and DAMPs from damaged parenchyma in ischemic stroke are recognized by PRRs (Kelley et al., 2019). PRRs then serve to activate NF-κB and NLRP3 inflammasome eliciting the transcription and translation of IL-18 and IL-1β levels (Kelley et al., 2019; Kumar, 2019). Two independent adaptor proteins are implicated in the NF-κB signaling pathway which is associated with NLRP3 inflammasome activation and induction of pro-inflammatory cytokines IL-18 and IL-1β. The Myeloid differentiation primary response 88 (MyD88) and TIR-domain-containing adapter-inducing interferon-β (TRIF) are two adaptor proteins that can initiate NLRP3 inflammasome activation separately via TLRs ligand interactions such as TLR4 (Duran et al., 2016; Zamyatina and Heine, 2020; Kim et al., 2023). Through the NF-κB-MyD88 dependent pathway, activation of TLR4 leads to the binding of MyD88 to the cytoplasmic portion of the TLR4 initiating the recruitment of interleukin-1 receptor-associated kinase 4 (IRAK-4) and IRAK-1 to form a complex (Puleo et al., 2022). IRAK-4 activates IRAK-1 by phosphorylation dependent mechanisms leading to its attachment to the tumor necrosis factor receptor associated factor 6 (TRAF6) and forming a quadruplet complex called myddosome (Puleo et al., 2022). IRAK-1 and TRAF-6 then activate IκB kinase-β (IKK -β) which leads to the degradation of IκB by means of phosphorylation causing the release and migration of NF-κB into the nucleus to elicit transcription and translation of pro-IL-1β and NLRP3 expression in priming the NLRP3-inflammasome for activation (Boaru et al., 2015; Zhong et al., 2016; Liu et al., 2022) (Figure 2). In the TRIF-dependent pathway, TRIF binds to the TLR4 together with tripartite interaction motif (TRIM) and activate trafficking kinesin-binding protein 1 (TAK-1) which then attaches to TRAF6 and initiates NLRP3-inflammasome activation via NF-κB in a similar fashion as the MyD88-dependent pathway (Kumar, 2019). The priming of NLRP3-inflammasome for activation is preceded by phosphorylation of ASC, and the deubiquitination and activation of NLRP3 which is mediated by a JAMM domain-containing Zn2+ metalloprotease called BRCC36 (Py et al., 2013). ASC then assembles with NLRP3 and procaspase-1 forming the NLRP3 inflammasome complex. Procaspase-1 is cleaved to caspase-1 which converts proinflammatory IL-1β and IL-18 into active IL-1β and IL-18 that promote neuroinflammatory damage in ischemic stroke (Liu et al., 2021).
The group by Fann et al. (2018) demonstrated that reducing activation of the NF-κB and MAPK pathways using intravenous immunoglobulins in ischemic stroke ameliorated cell death and injury by reducing the expression and activation of the NLRP1 and NLRP3 inflammasomes, and increasing expression of anti-apoptotic proteins, Bcl-2 and Bcl-xL in primary cortical neurons and/or cerebral tissue. Further, restoration of blood supply which is a key aspect in the treatment of stroke, results in cerebral ischemic-reperfusion injury mainly from the increased production of ischemia-induced production of ROS and activation of NLRP3 inflammasome that exacerbates injury and inflammation induced injury causing more brain cell damage and dysfunction, and brain edema (Wang et al., 2007; Minutoli et al., 2016).
The NLRP3 inflammasome contributes to brain cell injury through various mechanisms. Franke et al. (2021) demonstrated that the NLRP3 inflammasome is mainly expressed within ischemic neurons and drives inflammation in ischemia/reperfusion injury following transient middle cerebral artery occlusion in mice. In atherosclerosis, which is an underlying process that mediates ischemic stroke, NLRP3 is activated inside foam cells by ROS released by ruptured lysosomes leading to increased release of inflammatory cytokines such as IL-18 and IL-1β (Hua et al., 2013). IL-18 promotes necrosis of vascular smooth muscle cells releasing tissue metalloproteinases that destabilize plaque stability, increasing atherothrombotic and occlusion tendency (Zheng et al., 2013). Oxidized LDL that forms fatty streaks activates toll-like receptor type 12 or 4 (TLR-12/TLR-4) signaling that in turn activate downstream signal transduction molecules leading to the induction of myeloid differentiation primary response gene 88 (MyD88) which in turn leads to expression of NF-κB via interferon TIR-domain-containing adapter-inducing interferon-β (TRIF)-mediated mechanisms (Segovia et al., 2012; Tarallo et al., 2012). As discussed earlier, NF-κB promotes NLRP3 inflammasome activation and activation of pro- IL-1β via caspase-1 dependent mechanism to promote inflammation that further exacerbates ischemic injury (Segovia et al., 2012). IL-1β is a pleiotropic important cytokine involved in the activation of several proinflammatory signaling pathways in peripheral tissue including microglia and astrocytes in the brain during ischemic stroke (Mendiola and Cardona, 2018). IL-1β contributes to toxic cerebral edema and inflammation by disrupting the blood brain barrier and facilitating infiltration of immune cells in the central nervous system (Jagadapillai et al., 2022). In animal models, messenger ribonucleic acid (mRNA) levels of IL-1β are increased by 30 min post-cerebral ischemia and after a few hours IL-1β protein expression levels are also increased suggesting the pathological role of IL-1β in ischemic stroke (Buttini et al., 1994; Davies et al., 1999; Caso et al., 2007). This is evidenced by increased brain injury following administration of IL-1β and reduced infarct volume in IL-1β-deficient mice and mice administered with IL-1 receptor antagonist (IL-1ra) (Yamasaki et al., 1995; Boutin et al., 2001; Pradillo et al., 2012; Murray et al., 2015; Flygt et al., 2018; Ozen et al., 2020). One proposed mechanism mediating ischemic brain injury by IL-1 which is secreted by neutrophils is that IL-1 induces IL-17A in γδ T Cells and CXCL1 in Astrocytes to promote and amplify the post-stroke inflammatory response (Schädlich et al., 2022). CXCL1 is a specific chemokine attractant of neutrophils and IL-17A synergizes with TNF to enhance the expression of CXCL1 in astrocytes (Schädlich et al., 2022). Apart from increasing expression levels of CXCL1, systemic administration of IL-1β also increases macrophage inflammatory protein-2 (MIP-2) further enhancing neutrophil recruitment and infiltration and production of neutrophil-derived matrix metalloproteinase-9 (McColl et al., 2007, 2008).
Apart from IL-1β, activated microglia and astrocytes also secret TNF-α, transforming growth factor-β (TGF-β), interferon-β (IFN-β), MCP-1, stromal cell-derived factor 1 (SDF-1), macrophage inflammatory protein-1α (MIP-1α), and matrix metalloproteinases that disrupt the basal lamina of the blood brain barrier and gap junctions between endothelial cells increasing intravascular permeability, cell adhesion and diapedesis of recruited cells which then infiltrate the brain and cause more neuronal injury (Yang et al., 2019).
The early pathological processes resulting in inflammasome activation and induction of inflammation in ischemic stroke induces activation and infiltration of vascular smooth muscle cells, neutrophils, macrophages, lymphocytes, into the ischemic area, worsening tissue injury and inducing fibrotic changes (McDonald et al., 2010; Artlett, 2012).
Although development of drugs to target the NLRP3 inflammasome are underway, several therapeutic modalities do exist that can potentially be used to ameliorate ischemic injury in stroke (Feng et al., 2020; Franke et al., 2021). Animal studies have demonstrated the protective effect against neurological deterioration and infarct volume resulting from inhibition of the NLRP3 inflammasome after ischemic stroke (Deroide et al., 2013; Yang et al., 2014). Drugs that inhibit IL-1β such as the anti-IL-1β antibody canakinumab or antagonists of IL-1 receptor such as anakinra hormone or NLRP3 inhibitor such as atorvastatin are currently being studied and are known to reduce IL-1β levels by inhibiting activation of the NLRP3 inflammasome (McGonagle et al., 2007; Carné, 2011; Ridker et al., 2011, 2012; Satoh et al., 2014). Other drugs such as ritonavir, an inhibitor of caspase-1 and P2X7 receptor antagonists also reduce IL-1β and IL-18 levels (Stokes et al., 2006; Arulkumaran et al., 2011; López-Castejón and Pelegrín, 2012). Other drugs being studied that have potential to inhibit inflammasome activation and ameliorate ischemic stroke tissue injury include minocycline administrated 1 hour following reperfusion (Lu et al., 2016), IMM-H004 (Ai et al., 2019), progesterone and steroids 17b-estradiol (Lammerding et al., 2015; Zhang et al., 2017), Stachybotrys microspora triphenyl phenol-7 (SMTP-7) (Huang et al., 2018), MCC950 (Ismael et al., 2018), and other agents which have been described elsewhere (Feng et al., 2020).
However, due to the acute nature of the pathological changes occurring in ischemic stroke, timing, and dosage of these drugs remain unclear, requiring more studies (Gao et al., 2017).
Minimizing the inflammatory response after stroke is another modality of ameliorating the secondary adverse effects mediated by inflammatory cells. Use of anti-ICAM-1 antibody in transient focal cerebral ischemia model reduced cellular infiltrates into the brain leading to reduced ischemic brain damage (Zhang et al., 1994). However, clinical trials using anti-ICAM-1 antibody enlimomab was associated with further damage and adverse effects in human clinical trials (Furuya et al., 2001; Enlimomab Acute Stroke Trial Investigators, 2021). Other drugs such as IL-1RA antagonist, broad-spectrum tetracycline antibiotic minocycline, fingolimod an agonist of sphingosine-1-phosphate receptor, celecoxib (a selective inhibitor of cyclooxygenase 2), etanercept a TNFα inhibitor, and peroxisome proliferator-activated receptor gamma (PPAR-γ) agonists are being explored (Chu et al., 2004; Luo et al., 2006; Lampl et al., 2007; Fu et al., 2014; Lu et al., 2016; Lee et al., 2017; Duris et al., 2018).
Virtually all brain cells including neurons, astrocytes and microglia of both humans and rodents express the NLRP3 inflammasome but with variations in intensity and mechanisms (Chiarini et al., 2020; Pike et al., 2021). While the NLRP3 inflammasome is also expressed in neurons and astrocytes, the human microglia seems to predominate the expression (Lech et al., 2010; de Rivero Vaccari et al., 2014; Nyúl-Tóth et al., 2017; Pike et al., 2021; Sandhu and Kulka, 2021). As already discussed, activation of the NLRP3 inflammasome in human and rodent microglia is associated with inflammatory responses that exacerbate pathogenesis of injury including ischemic stroke injury. Pharmacological interventions that inhibit NLRP3 inflammasome activation appears to decrease this inflammatory response and attenuates further injury (de Rivero Vaccari et al., 2014). Although several therapeutics targeting inflammasomes are being investigated, there is scarcity of data to allow a comparison of these interventions with others used to treat ischemic stroke. Future studies to investigate this aspect are therefore warrantable.
Genetic mutations involving the NLRP3 inflammasome gene associated with stroke are still being studied and still new in the field. However, in a recent study by Kumar et al. (2022) conducted in an Indian population, they found that a susceptibility putative haplotype GTGTA within NLRP3 gene conferred a double risk of ischemic stroke in carriers and potentiated increased levels of c-reactive protein (CRP) and IL-1β in a dose-dependent manner.
In another study from China by Lv et al. (2020), they found that men who were carrying both caspase recruitment domain family member 8 (CARD8) rs2043211 AT and NLRP3 rs10754558 CG were seven times more likely to develop ischemic stroke compared to women. In another study among the Chinese Han population, the NLRP3 gene polymorphism rs4612666 increased susceptibility of large artery atherosclerosis (LAA) ischemic stroke by influencing plaque vulnerability (Cheng et al., 2018).
Several gene polymorphisms associated with susceptibility to ischemic stroke have been identified including the CYP11B1 gene (rs5283) and CYP4F2 gene (rs2108622, rs3093106, and rs3093105) in Chinese Han population (Liu and Duan, 2022; Huang K. et al., 2023). In addition, the diabetes risk variants (rs7578326 and rs12779790) and the susceptibility allele for myocardial infarction, the CDKN2A/B locus (rs2383207, 9p21) in the Religious Orders Study (ROS) and the Rush Memory and Aging Project (MAP) from the USA were also identified to increase the risk for ischemic stroke (Chou et al., 2013). Several other common variants in polygenic diseases are described elsewhere (Terni et al., 2014; Ekkert et al., 2022).
Genetic risk for ischemic stroke occurs through various mechanisms including single gene disorders associated primarily with the development of stroke and genetic mutations resulting in disorders where stroke is one of the manifestations. In addition, common genetic variants associated with the risk for stroke including polymorphisms that are associated with increasing the risk for those diseases that also increase the risk for stroke play a critical role in increasing susceptibility.
Small vessel diseases such as Cerebral Autosomal Dominant Arteriopathy with Subcortical Infarcts and Leukoencephalopathy (CADASIL) and Cerebral Autosomal Recessive Arteriopathy with Subcortical Infarcts and Leukoencephalopathy (CARASIL) are autosomal dominant and recessive diseases, respectively, associated with ischemic stroke and leukoencephalopathy (Boehme et al., 2017). CADASIL is associated with single gene mutation of the Notch3 gene encoding a transmembrane surface protein mainly expressed in vascular cells and plays a major role in cellular signaling pathways (Locatelli et al., 2020). CARASIL is associated with mutations in the high-temperature requirement-A (HtrA) serine peptidase-1 gene encoding an enzyme that plays a major role in defense against cellular stresses (Uemura et al., 2020; Grigaite et al., 2021).
The mutations in both Notch3 and HtrA serine peptidase-1 result in susceptibility to ischemia. Other single gene disorders that are associated with stroke include familial amyloid angiopathy and collagen 4 (COL4A1) mutations (Tan et al., 2019; Chojdak-Łukasiewicz et al., 2021). The underlying pathology associated with these diseases include rupture of small cortical vessels due to defects in collagen and deposition of amyloid plaques. Genetic disorders where ischemic stroke is a manifestation include Smooth Muscle Alpha-Actin (ACTA2) mutation associated disorders, sickle cell disease, Ehlers-Danlos Type 4, Marfan syndrome, fabry disease and Mitochondrial encephalopathy with lactic acid and stroke-like episodes (MELAS) (Boehme et al., 2017).
Heritability of ischemic stroke is lower for small vessel disease and higher and similar for cardioembolic and large vessel diseases (Bevan et al., 2012). Generally, family history and monozygotic twins have more than a 30% higher risk for ischemic stroke (Flossmann et al., 2004).
Additional modifiable and non-modifiable risk factors that may exacerbate stroke heritability and trigger stroke include age, female sex with parental history of stroke, (Schulz et al., 2004; Touzé and Rothwell, 2008), pollution, disorders where inflammation is the hallmark of pathogenesis, infectious diseases, cardiac atrial disorders, hypertension, diabetes, physical inactivity, hyperlipidemia, diet, alcohol consumption, apolipoprotein B to A1, race/ethnicity and smoking (Boehme et al., 2017).
Relating to age and sex, women have similar and sometimes higher risk for stroke at younger ages but during older ages, men have a slightly higher risk compared to women (Kapral et al., 2005). Regarding race and ethnicity, it is well established that the risk for stroke and stroke-related deaths is double in African Americans compared to their white counterparts (Kissela et al., 2004; Morgenstern et al., 2004; Zahuranec et al., 2006; Cruz-Flores et al., 2011; Ashley and Berry, 2020).
Common methods employed to discover genetic causes of ischemic stroke include candidate gene approaches, twin and family history studies, genome-wide association studies and next generation sequencing (Ekkert et al., 2022). Genetic susceptibility to ischemic stroke is more relevant when employing multilocus genetic risk scores and polygenic risk scores (polyGRS) because of the multifactorial etiological nature of ischemic stroke. However, due to the polygenic nature of ischemic stroke, the latter scoring technique also inferred from genome-wide association (GWA) studies but combining different subtypes related to susceptibilities to cardiometabolic and CVD risk such as arteriosclerosis, hypertension, hyperlipidemia is a better technique in estimating their cumulative contribution to stroke (Hachiya et al., 2017; Ekkert et al., 2022). A study by Hachiya et al. (2017) used this technique and found that use of polyGRS combined with a non-genetic risk model strengthened the predictive ability of ischemic stroke.
Thus, this method taken together with non-genetic risk factors for stroke is an emerging revolutionary concept that will soon gain ground in clinical practice. For now, this field is still growing with more studies required to advance the predictive technology of ischemic stroke in clinical practice to prevent cerebrovascular accidents and associated adverse outcomes.
Ischemic stroke accounts for most CVD deaths and remains an important area of constant study. The underlying role of the inflammasome in ischemic stroke and therapeutic targets is an ongoing field of study. Future research must be more focused on targeting signaling pathways involved in the activation of the NLRP3 Inflammasome to ameliorate the severity of brain injury due to ischemic stroke. However, more studies are required that will focus on preventive therapy and use of polyGRS combined with a non-genetic risk factor stratification to predict ischemic stroke more accurately and thus discover ways to delay or prevent ischemic stroke.
Clinical studies that are focused on identifying biomarkers of ischemic stroke by interrogating the components of the NLRP3 inflammasome are also warrantable. As reported previously, inflammasome proteins such as caspase-1, ASC in serum, ASC and IL-18 in cerebral spinal fluid (CSF) are potential predictive biomarkers of traumatic brain injury (Kerr et al., 2018). ASC protein levels were higher in patients with poorer outcomes following traumatic brain injury suggesting that inflammasome proteins could be used in clinical settings as diagnostic and predictive biomarkers (Kerr et al., 2018). Although this study by Kerr et al. (2018) was not focused primarily on ischemic stroke and it is unclear whether the NLRP3 inflammasome proteins were the ones being measured, future clinical studies could explore this in ischemic stroke patients with varying severity in outcomes to determine the diagnostic and prognostic value of using NLRP3 inflammasome proteins in ischemic stroke.
For therapeutic interventions, use of NLRP3 inhibitors is a promising area for future research. The Canakinumab Anti-inflammatory Thrombosis Outcomes Study (CANTOS) is one of those successful trials demonstrating that that inhibition of NLRP3 inflammasome is beneficial in ameliorating the adverse outcomes of cardio-cerebrovascular events (Ridker et al., 2011, 2012; Everett et al., 2020). The CANTOS trial was primarily based on inhibiting the production of IL-1β. Future trials could therefore target additional proteins in the NLRP3 inflammasome activation cascade and compare efficacy and superiority with Canakinumab. In clinical settings, prompt diagnosis, prognosis, and treatment modalities are critical for stroke patient outcomes. More research should therefore explore this area. However, determining the risk of a stroke in an individual who has never had a stroke or an individual with a high risk for recurrent stroke is more critical and important in alleviating the burden of stroke globally, but it is currently a big challenge.
Genetic testing may be beneficial in determining persons who are at risk for ischemic stroke. Although integrating genetic testing in clinical practice is a challenge, current advances in this area are promising. The use of genome-wide association studies, next generation sequencing or simply genetic risk scores and extended polygenic risk scores to estimate the cumulative contribution of genetic factors for stroke development and then combining this information with clinical information and known existing risk factors for stroke may be a better method to prevent stroke. Although there is no established gold standard method to determine stroke risk in clinical settings, there are several biomarkers and genetic markers available for aiding decision making in clinical practice. A recent detailed description of how genetic testing can be incorporated in clinical practice has been elaborated by Ekkert et al. (2022).
Although ischemic stroke is fatal for the brain and associated with morbidity and mortality, understanding the intracellular and molecular pathogenesis is crucial for management modalities. Ischemic stroke activates several intracellular pathways that contribute to more injury and promote more death of brain tissue. Among the inflammasomes known to man, the most studied is the NLRP3 Inflammasome. After a stroke event, the activation of the NLRP3 Inflammasome via the MyD88-IRAK/TRAF- NF-κB pathway is associated with more inflammation and worsening of brain injury and outcomes. Targeting the NLRP3 inflammasome has potential to reduce the infarct volume after ischemic stroke and attenuate brain injury consequently reducing morbidity and disability. More clinical studies are therefore required that focus on targeting different aspects of the NLRP3 inflammasome signaling cascade to prevent recruitment and infiltration of brain tissue with activated inflammatory cells. Although the NLRP3 inflammasome is the most studied in disease, the underlying mechanisms related to the NLRP3 Inflammasome activation and exacerbation of ischemic injury are still elusive and complex, involving multiple intracellular signaling pathways.
The outcomes of stroke events are usually adverse, therefore preventing ischemic stroke through use of a combination of advanced genetic testing, risk stratification based on sociodemographic, dietary, and lifestyle factors is encouraged.
SM: Conceptualization, Data curation, Funding acquisition, Methodology, Validation, Visualization, Writing – original draft, Writing – review & editing. AK: Supervision, Writing – original draft, Writing – review & editing.
The author(s) declare financial support was received for the research, authorship, and/or publication of this article. This work was supported by the Fogarty International Center and National Institute of Diabetes and Digestive and Kidney Diseases of the National Institutes of Health grants R01HL147818 and R01HL144941 (AK), 2D43TW009744 (SM), R21TW012635 (AK and SM) and the American Heart Association Award Number 24IVPHA1297559 https://doi.org/10.58275/AHA.24IVPHA1297559.pc.gr.193866 (AK and SK).
The authors declare that the research was conducted in the absence of any commercial or financial relationships that could be construed as a potential conflict of interest.
All claims expressed in this article are solely those of the authors and do not necessarily represent those of their affiliated organizations, or those of the publisher, the editors and the reviewers. Any product that may be evaluated in this article, or claim that may be made by its manufacturer, is not guaranteed or endorsed by the publisher.
The content is solely the responsibility of the authors and does not represent the official views of the National Institutes of Health and the American Heart Association.
Abais, J. M., Xia, M., Zhang, Y., Boini, K. M., and Li, P.-L. (2015). Redox regulation of NLRP3 inflammasomes: ROS as trigger or effector? Antioxidants Redox Sig. 22, 1111–1129. doi: 10.1089/ars.2014.5994
Ai, Q. D., Chen, C., Chu, S., Zhang, Z., Luo, Y., Guan, F., et al. (2019). IMM-H004 therapy for permanent focal ischemic cerebral injury via CKLF1/CCR4-mediated NLRP3 inflammasome activation. Transl. Res. 212, 36–53. doi: 10.1016/j.trsl.2019.05.007
Almanza, A., Carlesso, A., Chintha, C., Creedican, S., Doultsinos, D., Leuzzi, B., et al. (2019). Endoplasmic reticulum stress signalling – from basic mechanisms to clinical applications. The FEBS J. 286, 241–278. doi: 10.1111/febs.14608
Alu, A., Han, X., Ma, X., Wu, M., Wei, Y., Wei, X., et al. (2020). The role of lysosome in regulated necrosis. Acta Pharm. Sin. B 10, 1880–1903. doi: 10.1016/j.apsb.2020.07.003
Ammendolia, D. A., Bement, W. M., and Brumell, J. H. (2021). Plasma membrane integrity: implications for health and disease. BMC Biol. 19:71. doi: 10.1186/s12915-021-00972-y
Anrather, J., and Iadecola, C. (2016). Inflammation and stroke: an overview. Neurotherapeutics 13, 661–670. doi: 10.1007/s13311-016-0483-x
Artlett, C. M. (2012). The role of the NLRP3 inflammasome in fibrosis. Open Rheumatol. J. 6, 80–86. doi: 10.2174/1874312901206010080
Arulkumaran, N., Unwin, R. J., and Tam, F. W. (2011). A potential therapeutic role for P2X7 receptor (P2X7R) antagonists in the treatment of inflammatory diseases. Expert Opin. Investig. Drugs 20, 897–915. doi: 10.1517/13543784.2011.578068
Ashley, C., and Berry, S. D. (2020). The association between race and stroke prevalence in a patient cohort in Mississippi. Perspect Health Inf. Manag. 18:1i.
Bauernfeind, F., Bartok, E., Rieger, A., Franchi, L., Núñez, G., Hornung, V., et al. (2011). Cutting edge: reactive oxygen species inhibitors block priming, but not activation, of the NLRP3 inflammasome. The J. Immunol. 187, 613–617. doi: 10.4049/jimmunol.1100613
Bevan, S., Traylor, M., Adib-Samii, P., Malik, R., Paul, N. L. M., Jackson, C., et al. (2012). Genetic heritability of ischemic stroke and the contribution of previously reported candidate gene and genomewide associations. Stroke 43, 3161–3167. doi: 10.1161/STROKEAHA.112.665760
Blevins, H. M., Xu, Y., Biby, S., and Zhang, S. (2022). The NLRP3 inflammasome pathway: a review of mechanisms and inhibitors for the treatment of inflammatory diseases. Front. Aging Neurosci. 14:879021. doi: 10.3389/fnagi.2022.879021
Boaru, S. G., Borkham-Kamphorst, E., Van de Leur, E., Lehnen, E., Liedtke, C., Weiskirchen, R., et al. (2015). NLRP3 inflammasome expression is driven by NF-κB in cultured hepatocytes. Biochem. Biophys. Res. Commun. 458, 700–706. doi: 10.1016/j.bbrc.2015.02.029
Boehme, A. K., Esenwa, C., and Elkind, M. S. V. (2017). Stroke risk factors, genetics, and prevention. Circ. Res. 120, 472–495. doi: 10.1161/CIRCRESAHA.116.308398
Boutin, H., LeFeuvre, R. A., Horai, R., Asano, M., Iwakura, Y., and Rothwell, N. J. (2001). Role of IL-1α and IL-1β in ischemic brain damage. J. Neurosci. 21, 5528–5534. doi: 10.1523/JNEUROSCI.21-15-05528.2001
Brouns, R., and De Deyn, P. P. (2009). The complexity of neurobiological processes in acute ischemic stroke. Clin. Neurol. Neurosurg. 111, 483–495. doi: 10.1016/j.clineuro.2009.04.001
Bulté, D., Rigamonti, C., Romano, A., and Mortellaro, A. (2023). Inflammasomes: mechanisms of action and involvement in human diseases. Cells 12:1766. doi: 10.3390/cells12131766
Buttini, M., Sauter, A., and Boddeke, H. W. G. M. (1994). Induction of interleukin-1β mRNA after focal cerebral ischaemia in the rat. Mol. Brain Res. 23, 126–134. doi: 10.1016/0169-328X(94)90218-6
Carné, X. (2011). Canakinumab, a monoclonal antibody against IL-1β, with potential utility in different inflammatory processes. Med. Clin. 136, 34–37. doi: 10.1016/S0025-7753(11)70007-0
Caso, J. R., Moro, M. A., Lorenzo, P., Lizasoain, I., and Leza, J. C. (2007). Involvement of IL-1β in acute stress-induced worsening of cerebral ischaemia in rats. Eur. Neuropsychopharmacol. 17, 600–607. doi: 10.1016/j.euroneuro.2007.02.009
Chen, Y., Meng, J., Bi, F., Li, H., Chang, C., Ji, C., and Liu, W. (2019). NEK7 regulates NLRP3 inflammasome activation and neuroinflammation post-traumatic brain injury. Front. Mol. Neurosci. 12:202. doi: 10.3389/fnmol.2019.00202
Cheng, L., Yin, R., Yang, S., Pan, X., and Ma, A. (2018). Rs4612666 polymorphism of the NLRP3 gene is associated with the occurrence of large artery atherosclerotic ischemic strokes and microembolic signals. BioMed Res. Int. 2018:e6345805. doi: 10.1155/2018/6345805
Chiarini, A., Armato, U., Hu, P., and Dal Prà, I. (2020). Danger-sensing/patten recognition receptors and neuroinflammation in Alzheimer's disease. Int. J. Mol. Sci. 21:9036. doi: 10.3390/ijms21239036
Chojdak-Łukasiewicz, J., Dziadkowiak, E., and Budrewicz, S. (2021). Monogenic causes of strokes. Genes 12:1855. doi: 10.3390/genes12121855
Chou, S. H. Y., Shulman, J. M., Keenan, B. T., Secor, E. A., Buchman, A. S., Schneider, J., et al. (2013). Genetic susceptibility for ischemic infarction and arteriolosclerosis based on neuropathologic evaluations. Cereb. Dis. 36, 181–188. doi: 10.1159/000352054
Chu, K., Jeong, S. W., Jung, K. H., Han, S. Y., Lee, S. T., Kim, M., et al. (2004). Celecoxib induces functional recovery after intracerebral hemorrhage with reduction of brain edema and perihematomal cell death. J. Cereb. Blood Flow Metab. 24, 926–933. doi: 10.1097/01.WCB.0000130866.25040.7D
Clemente-Suárez, V. J., Redondo-Flórez, L., Beltrán-Velasco, A. I., and Ramos-Campo, D. J. (2023). Mitochondria and brain disease: a comprehensive review of pathological mechanisms and therapeutic opportunities. Biomedicines 11:2488. doi: 10.3390/biomedicines11092488
Compan, V., Baroja-Mazo, A., López-Castejón, G., Gomez, A. I., Martínez, C. M., Angosto, D., et al. (2012). Cell volume regulation modulates NLRP3 inflammasome activation. Immunity 37, 487–500. doi: 10.1016/j.immuni.2012.06.013
Connor, M. D., Walker, R., Modi, G., and Warlow, C. P. (2007). Burden of stroke in black populations in sub-Saharan Africa. The Lancet Neurol. 6, 269–278. doi: 10.1016/S1474-4422(07)70002-9
Creagh, E. M. (2014). Caspase crosstalk: integration of apoptotic and innate immune signalling pathways. Trends Immunol. 35, 631–640. doi: 10.1016/j.it.2014.10.004
Cruz-Flores, S., Rabinstein, A., Biller, J., Elkind, M. S. V., Griffith, P., Gorelick, P. B., et al. (2011). Racial-ethnic disparities in stroke care: the American experience: a statement for healthcare professionals from the American Heart Association/American Stroke Association. Stroke 42, 2091–2116. doi: 10.1161/STR.0b013e3182213e24
Daniels, M. J. D., Rivers-Auty, J., Schilling, T., Spencer, N. G., Watremez, W., Fasolino, V., et al. (2016). Fenamate NSAIDs inhibit the NLRP3 inflammasome and protect against Alzheimer's disease in rodent models. Nat. Commun. 7:12504. doi: 10.1038/ncomms12504
Davies, C. A., Loddick, S. A., Toulmond, S., Stroemer, R. P., Hunt, J., Rothwell, N. J., et al. (1999). The progression and topographic distribution of interleukin-1β expression after permanent middle cerebral artery occlusion in the rat. J. Cereb. Blood Flow Metab. 19, 87–98. doi: 10.1097/00004647-199901000-00010
de Rivero Vaccari, J. P., Dietrich, W. D., and Keane, R. W. (2014). Activation and regulation of cellular inflammasomes: gaps in our knowledge for central nervous system injury. J. Cereb. Blood Flow Metab. 34, 369–375. doi: 10.1038/jcbfm.2013.227
Deroide, N., Li, X., Lerouet, D., Vré, E. V., Baker, L., Harrison, J., et al. (2013). MFGE8 inhibits inflammasome-induced IL-1β production and limits postischemic cerebral injury. J. Clin. Invest. 123, 1176–1181. doi: 10.1172/JCI65167
Dinarello, C. A. (2009). Immunological and inflammatory functions of the interleukin-1 family. Ann. Rev. Immunolo. 27, 519–550. doi: 10.1146/annurev.immunol.021908.132612
Donkor, E. S. (2018). Stroke in the 21st century: a snapshot of the burden, epidemiology, and quality of life. Stroke Res. Treat 2018:3238165.
Doyle, K. P., Simon, R. P., and Stenzel-Poore, M. P. (2008). Mechanisms of ischemic brain damage. Neuropharmacology 55, 310–318. doi: 10.1016/j.neuropharm.2008.01.005
Duran, A., Valero, N., Mosquera, J., Delgado, L., Alvarez-Mon, M., Torres, M., et al. (2016). Role of the myeloid differentiation primary response (MYD88) and TIR-domain-containing adapter-inducing interferon-β (TRIF) pathways in dengue. Life Sci. 162, 33–40. doi: 10.1016/j.lfs.2016.08.023
Duris, K., Splichal, Z., and Jurajda, M. (2018). The role of inflammatory response in stroke associated programmed cell death. Curr. Neuropharmacol. 16, 1365–1374. doi: 10.2174/1570159X16666180222155833
Ekkert A. Šliachtenko A. Grigaite J. Burnyte B. Utkus A. Jatužis D.. (2022). Ischemic stroke genetics: What is new and how to apply it in clinical practice? Genes 13:48. doi: 10.3390/genes13010048
Elinav, E., Strowig, T., Kau, A. L., Henao-Mejia, J., Thaiss, C. A., Booth, C. J., et al. (2011). NLRP6 inflammasome regulates colonic microbial ecology and risk for colitis. Cell 145, 745–757. doi: 10.1016/j.cell.2011.04.022
Elsayed, W. M., Abdel-Gawad, E. H. A., Mesallam, D. I. A., and El-Serafy, T. S. (2020). The relationship between oxidative stress and acute ischemic stroke severity and functional outcome. The Egyptian J. Neurol. Psychiatr. Neurosurg. 56:74. doi: 10.1186/s41983-020-00206-y
Enlimomab Acute Stroke Trial Investigators (2021). Use of anti-ICAM-1 therapy in ischemic stroke: results of the enlimomab acute stroke trial. Neurology 57, 1428–1434. doi: 10.1212/WNL.57.8.1428
Everett, B. M., MacFadyen, J. G., Thuren, T., Libby, P., Glynn, R. J., Ridker, P. M., et al. (2020). Inhibition of interleukin-1β and reduction in atherothrombotic cardiovascular events in the CANTOS trial. J. Am. Coll. Cardiol. 76, 1660–1670. doi: 10.1016/j.jacc.2020.08.011
Fann, D. Y. W., Lim, Y. A., Cheng, Y. L., Lok, K. Z., Chunduri, P., Baik, S. H., et al. (2018). Evidence that NF-κB and MAPK signaling promotes NLRP inflammasome activation in neurons following ischemic stroke. Mol. Neurobiol. 55, 1082–1096. doi: 10.1007/s12035-017-0394-9
Feigin, V. L., Brainin, M., Norrving, B., Martins, S., Sacco, R. L., Hacke, W., et al. (2022). World stroke organization (WSO): global stroke fact sheet 2022. Int. J. Stroke 17, 18–29. doi: 10.1177/17474930211065917
Feng, Y. S., Tan, Z. X., Wang, M. M., Xing, Y., Dong, F., and Zhang, F. (2020). Inhibition of NLRP3 inflammasome: a prospective target for the treatment of ischemic stroke. Front. Cell. Neurosci. 14:155. doi: 10.3389/fncel.2020.00155
Fernandes-Alnemri, T., Wu, J., Yu, J. W., Datta, P., Miller, B., Jankowski, W., et al. (2007). The pyroptosome: a supramolecular assembly of ASC dimers mediating inflammatory cell death via caspase-1 activation. Cell Death Differ 14, 1590–1604. doi: 10.1038/sj.cdd.4402194
Flossmann, E., Schulz, U. G. R., and Rothwell, P. M. (2004). Systematic review of methods and results of studies of the genetic epidemiology of ischemic stroke. Stroke 35, 212–227. doi: 10.1161/01.STR.0000107187.84390.AA
Flygt, J., Ruscher, K., Norberg, A., Mir, A., Gram, H., Clausen, F., et al. (2018). Neutralization of interleukin-1β following diffuse traumatic brain injury in the mouse attenuates the loss of mature oligodendrocytes. J. Neurotr. 35, 2837–2849. doi: 10.1089/neu.2018.5660
Franchi, L., Warner, N., Viani, K., and Nuñez, G. (2009). Function of Nod-like receptors in microbial recognition and host defense. Immunol. Rev. 227, 106–128. doi: 10.1111/j.1600-065X.2008.00734.x
Franke, M., Bieber, M., Kraft, P., Weber, A. N. R., Stoll, G., Schuhmann, M. K., et al. (2021). The NLRP3 inflammasome drives inflammation in ischemia/reperfusion injury after transient middle cerebral artery occlusion in mice. Brain Behav. Immun. 92, 221–231. doi: 10.1016/j.bbi.2020.12.009
Fu, Y., Liu, Q., Anrather, J., and Shi, F. D. (2015). Immune interventions in stroke. Nat. Rev. Neurol. 11, 524–535. doi: 10.1038/nrneurol.2015.144
Fu, Y., Zhang, N., Ren, L., Yan, Y., Sun, N., Li, Y. J., et al. (2014). Impact of an immune modulator fingolimod on acute ischemic stroke. Proc. Natl. Acad. Sci. U. S. A. 111, 18315–18320. doi: 10.1073/pnas.1416166111
Furuya, K., Takeda, H., Azhar, S., McCarron, R. M., Chen, Y., Ruetzler, C. A., et al. (2001). Examination of several potential mechanisms for the negative outcome in a clinical stroke trial of enlimomab, a murine anti-human intercellular adhesion molecule-1 antibody: a bedside-to-bench study. Stroke 32, 2665–2674. doi: 10.1161/hs3211.098535
Gao, L., Dong, Q., Song, Z., Shen, F., Shi, J., Li, Y., et al. (2017). NLRP3 inflammasome: a promising target in ischemic stroke. Inflamm. Res. 66, 17–24. doi: 10.1007/s00011-016-0981-7
Geng, H., Chen, L., Tang, J., Chen, Y., and Wang, L. (2022). The Role of CCL2/CCR2 axis in cerebral ischemia-reperfusion injury and treatment: from animal experiments to clinical trials. Int J. Mol. Sci. 23:3485. doi: 10.3390/ijms23073485
Grigaite, J., Šiauryte, K., Audronyte, E., Preikšaitiene, E., Burnyte, B., Pranckevičiene, E., et al. (2021). Novel in-frame deletion in HTRA1 gene, responsible for stroke at a young age and dementia—a case study. Genes 12:1955. doi: 10.3390/genes12121955
Guo, C., Sun, L., Chen, X., and Zhang, D. (2013). Oxidative stress, mitochondrial damage and neurodegenerative diseases. Neural. Regen. Res. 8, 2003–2014.
Guo, H., Callaway, J. B., and Ting, J. P. Y. (2015). Inflammasomes: mechanism of action, role in disease, and therapeutics. Nat. Med. 21, 677–687. doi: 10.1038/nm.3893
Gusarova, G. A., Trejo, H. E., Dada, L. A., Briva, A., Welch, L. C., Hamanaka, R. B., et al. (2011). Hypoxia leads to Na,K-ATPase downregulation via Ca2+ release-activated Ca2+ channels and AMPK activation?. Mol. Cell Biol. 31, 3546–3556. doi: 10.1128/MCB.05114-11
Hachiya, T., Kamatani, Y., Takahashi, A., Hata, J., Furukawa, R., Shiwa, Y., et al. (2017). Genetic predisposition to ischemic stroke. Stroke 48, 253–258. doi: 10.1161/STROKEAHA.116.014506
Ham, P. B., and Raju, R. (2017). Mitochondrial function in hypoxic ischemic injury and influence of aging. Prog. Neurobiol. 157, 92–116. doi: 10.1016/j.pneurobio.2016.06.006
He, T., Yang, G. Y., and Zhang, Z. (2022). Crosstalk of astrocytes and other cells during ischemic stroke. Life 12:910. doi: 10.3390/life12060910
He, X., Li, X., Tian, W., Li, C., Li, P., Zhao, J., et al. (2023). Role of redox-mediated lysosomal dysfunction and therapeutic strategies. Biomed. Pharmacother. 165:115121. doi: 10.1016/j.biopha.2023.115121
Hornung, V., Bauernfeind, F., Halle, A., Samstad, E. O., Kono, H., Rock, K. L., et al. (2008). Silica crystals and aluminum salts activate the NALP3 inflammasome through phagosomal destabilization. Nat. Immunol. 9, 847–856. doi: 10.1038/ni.1631
Hua, K. F., Yang, S. M., Kao, T. Y., Chang, J. M., Chen, H. L., Tsai, Y. J., et al. (2013). Osthole mitigates progressive IgA nephropathy by inhibiting reactive oxygen species generation and NF-κB/NLRP3 pathway. PLoS ONE 8:e77794. doi: 10.1371/journal.pone.0077794
Huang, J., Chen, L., Yao, Z., Sun, X., Tong, X., Dong, S., et al. (2023). The role of mitochondrial dynamics in cerebral ischemia-reperfusion injury. Biomed. Pharmacother. 162:114671. doi: 10.1016/j.biopha.2023.114671
Huang, J., Choudhri, T. F., Winfree, C. J., McTaggart, R. A., Kiss, S., Mocco, J., et al. (2000). Postischemic cerebrovascular E-selectin expression mediates tissue injury in murine stroke. Stroke 31, 3047–3053. doi: 10.1161/01.STR.31.12.3047
Huang, K., Ma, T., Li, Q., Zhou, Y., Qin, T., Zhong, Z., et al. (2023). Genetic variants of CYP4F2 associated with ischemic stroke susceptibility in the han population from southern China. PGPM 16, 599–607. doi: 10.2147/PGPM.S413632
Huang, Y., Ohta, Y., Shang, J., Morihara, R., Nakano, Y., Fukui, Y., et al. (2018). Antineuroinflammatory effect of SMTP-7 in ischemic mice. J. Stroke Cerebrovas. Dis. 27, 3084–3094. doi: 10.1016/j.jstrokecerebrovasdis.2018.06.039
Iadecola, C., and Anrather, J. (2011). The immunology of stroke: from mechanisms to translation. Nat. Med. 17, 796–808. doi: 10.1038/nm.2399
Ismael, S., Zhao, L., Nasoohi, S., and Ishrat, T. (2018). Inhibition of the NLRP3-inflammasome as a potential approach for neuroprotection after stroke. Sci. Rep. 8:5971. doi: 10.1038/s41598-018-24350-x
Jagadapillai, R., Qiu, X., Ojha, K., Li, Z., El-Baz, A., Zou, S., et al. (2022). Potential cross talk between autism risk genes and neurovascular molecules: a pilot study on impact of blood brain barrier integrity. Cells 11:2211. doi: 10.3390/cells11142211
Johnston, S. C., Mendis, S., and Mathers, C. D. (2009). Global variation in stroke burden and mortality: estimates from monitoring, surveillance, and modelling. The Lancet Neurol. 8, 345–354. doi: 10.1016/S1474-4422(09)70023-7
Kapral, M. K., Fang, J., Hill, M. D., Silver, F., Richards, J., Jaigobin, C., et al. (2005). Investigators of the registry of the canadian stroke network. Sex differences in stroke care and outcomes: results from the registry of the canadian stroke network. Stroke 36, 809–814. doi: 10.1161/01.STR.0000157662.09551.e5
Kelley, N., Jeltema, D., Duan, Y., and He, Y. (2019). The NLRP3 inflammasome: an overview of mechanisms of activation and regulation. Int. J. Mol. Sci. 20:3328. doi: 10.3390/ijms20133328
Kerr, N., Lee, S. W., Perez-Barcena, J., Crespi, C., Ibañez, J., Bullock, M. R., et al. (2018). Inflammasome proteins as biomarkers of traumatic brain injury. PLoS ONE 13:e0210128. doi: 10.1371/journal.pone.0210128
Kim, H. J., Kim, H., Lee, J. H., and Hwangbo, C. (2023). Toll-like receptor 4 (TLR4): new insight immune and aging. Immun. Ageing 20:67. doi: 10.1186/s12979-023-00383-3
Kissela, B., Schneider, A., Kleindorfer, D., Khoury, J., Miller, R., Alwell, K., et al. (2004). Stroke in a biracial population: the excess burden of stroke among blacks. Stroke 35, 426–431. doi: 10.1161/01.STR.0000110982.74967.39
Kumar, N., Kaur, M., Singh, G., Valecha, S., Khinda, R., Di Napoli, M., et al. (2022). A susceptibility putative haplotype within NLRP3 inflammasome gene influences ischaemic stroke risk in the population of Punjab, India. Int. J. Immunogenet. 49, 260–270. doi: 10.1111/iji.12589
Kumar, V. (2019). Toll-like receptors in the pathogenesis of neuroinflammation. J. Neuroimmunol. 332, 16–30. doi: 10.1016/j.jneuroim.2019.03.012
Kumari, P., Russo, A. J., Shivcharan, S., and Rathinam, V. A. (2020). AIM2 in health and disease: inflammasome and beyond. Immunol. Rev. 297, 83–95. doi: 10.1111/imr.12903
Lamkanfi, M., and Dixit, V. M. (2014). Mechanisms and functions of inflammasomes. Cell 157, 1013–1022. doi: 10.1016/j.cell.2014.04.007
Lammerding, L., Slowik, A., Johann, S., Beyer, C., and Zendedel, A. (2015). Poststroke inflammasome expression and regulation in the peri-infarct area by gonadal steroids after transient focal ischemia in the rat brain. Neuroendocrinology 103, 460–475. doi: 10.1159/000439435
Lampl, Y., Boaz, M., Gilad, R., Lorberboym, M., Dabby, R., Rapoport, A., et al. (2007). Minocycline treatment in acute stroke: an open-label, evaluator-blinded study. Neurology 69, 1404–1410. doi: 10.1212/01.wnl.0000277487.04281.db
Lebreton, F., Berishvili, E., Parnaud, G., Rouget, C., Bosco, D., Berney, T., et al. (2018). NLRP3 inflammasome is expressed and regulated in human islets. Cell Death Dis. 9, 1–10. doi: 10.1038/s41419-018-0764-x
Lech, M., Avila-Ferrufino, A., Skuginna, V., Susanti, H. E., and Anders, H.-J.. (2010). Quantitative expression of RIG-like helicase, NOD-like receptor and inflammasome-related mRNAs in humans and mice. Int. Immunol. 22, 717–728. doi: 10.1093/intimm/dxq058
Lee, J. H., Kam, E. H., Kim, J. M., Kim, S. Y., Kim, E. J., Cheon, S. Y., et al. (2017). Intranasal administration of interleukin-1 receptor antagonist in a transient focal cerebral ischemia rat model. Biomol. Ther. 25, 149–157. doi: 10.4062/biomolther.2016.050
Li, X., Cai, X., Bian, P., and Hu, L. (2012). High salt intake and stroke: meta-analysis of the epidemiologic evidence. CNS Neurosci. Ther. 18, 691–701. doi: 10.1111/j.1755-5949.2012.00355.x
Liu, D., Zhong, Z., and Karin, M. (2022). NF-κB: a double-edged sword controlling inflammation. Biomedicines 10:1250. doi: 10.3390/biomedicines10061250
Liu, G., and Duan, Y. (2022). CYP11B1 gene polymorphisms and susceptibility to ischemic stroke in a Chinese Han population. Front. Neurosci. 16:1030551. doi: 10.3389/fnins.2022.1030551
Liu, J., Zheng, J., Xu, Y., Cao, W., Wang, J., Wang, B., et al. (2021). Enriched environment attenuates pyroptosis to improve functional recovery after cerebral ischemia/reperfusion injury. Front. Aging Neurosci. 13:717644. doi: 10.3389/fnagi.2021.717644
Locatelli, M., Padovani, A., and Pezzini, A. (2020). Pathophysiological mechanisms and potential therapeutic targets in cerebral autosomal dominant arteriopathy with subcortical infarcts and leukoencephalopathy (CADASIL). Front. Pharmacol. 11:321. doi: 10.3389/fphar.2020.00321
Lopez, A. D., Mathers, C. D., Ezzati, M., Jamison, D. T., and Murray, C. J. (2006). Global and regional burden of disease and risk factors, 2001: systematic analysis of population health data. The Lancet 367, 1747–1757. doi: 10.1016/S0140-6736(06)68770-9
López-Castejón, G., and Pelegrín, P. (2012). Current status of inflammasome blockers as anti-inflammatory drugs. Exp. Opin. Investig. Drugs 21, 995–1007. doi: 10.1517/13543784.2012.690032
Lu, Y., Xiao, G., and Luo, W. (2016). Minocycline suppresses NLRP3 inflammasome activation in experimental ischemic stroke. Neuroimmunomodulation 23, 230–238. doi: 10.1159/000452172
Luo, Y., Yin, W., Signore, A. P., Zhang, F., Hong, Z., Wang, S., et al. (2006). Neuroprotection against focal ischemic brain injury by the peroxisome proliferator-activated receptor-gamma agonist rosiglitazone. J. Neurochem. 97, 435–448. doi: 10.1111/j.1471-4159.2006.03758.x
Lv, J., Jiang, X., Zhang, J., Peng, X., and Lin, H. (2020). Combined polymorphisms in genes encoding the inflammasome components NLRP3 and CARD8 confer risk of ischemic stroke in men. J. Stroke Cereb. Dis. 29:104874. doi: 10.1016/j.jstrokecerebrovasdis.2020.104874
Magid-Bernstein, J., Girard, R., Polster, S., Srinath, A., Romanos, S., Awad, I. A., et al. (2022). Cerebral hemorrhage: pathophysiology, treatment, and future directions. Circ. Res. 130, 1204–1229. doi: 10.1161/CIRCRESAHA.121.319949
Mamantopoulos, M., Ronchi, F., Hauwermeiren, F. V., Vieira-Silva, S., Yilmaz, B., Martens, L., et al. (2017). Nlrp6- and ASC-dependent inflammasomes do not shape the commensal gut microbiota composition. Immunity 47, 339–348. doi: 10.1016/j.immuni.2017.07.011
Manji, G. A., Wang, L., Geddes, B. J., Brown, M., Merriam, S., Al-Garawi, A., et al. (2002). PYPAF1, a PYRIN-containing Apaf1-like protein that assembles with ASC and regulates activation of NF-κB *. J. Biol. Chem. 277, 11570–11575. doi: 10.1074/jbc.M112208200
McColl, B. W., Rothwell, N. J., and Allan, S. M. (2007). Systemic inflammatory stimulus potentiates the acute phase and CXC chemokine responses to experimental stroke and exacerbates brain damage via interleukin-1- and neutrophil-dependent mechanisms. J. Neurosci. 27, 4403–4412. doi: 10.1523/JNEUROSCI.5376-06.2007
McColl, B. W., Rothwell, N. J., and Allan, S. M. (2008). Systemic inflammation alters the kinetics of cerebrovascular tight junction disruption after experimental stroke in mice. J. Neurosci. 28, 9451–9462. doi: 10.1523/JNEUROSCI.2674-08.2008
McDonald, B., Pittman, K., Menezes, G. B., Hirota, S. A., Slaba, I., Waterhouse, C. C. M., et al. (2010). Intravascular danger signals guide neutrophils to sites of sterile inflammation. Science 330, 362–366. doi: 10.1126/science.1195491
McGonagle, D., Tan, A. L., Shankaranarayana, S., Madden, J., Emery, P., McDermott, M. F., et al. (2007). Management of treatment resistant inflammation of acute on chronic tophaceous gout with anakinra. Ann. Rheum. Dis. 66, 1683–1684. doi: 10.1136/ard.2007.073759
Mendiola, A. S., and Cardona, A. E. (2018). The IL-1β phenomena in neuroinflammatory diseases. J. Neural Transm. 125, 781–795. doi: 10.1007/s00702-017-1732-9
Miller, M. A., and Zachary, J. F. (2017). Mechanisms and morphology of cellular injury, adaptation, and death. Pathol. Basis Vet. Dis. 2:1. doi: 10.1016/B978-0-323-35775-3.00001-1
Minkiewicz, J., de Rivero Vaccari, J. P., and Keane, R. W. (2013). Human astrocytes express a novel NLRP2 inflammasome. Glia 61, 1113–1121. doi: 10.1002/glia.22499
Minutoli, L., Puzzolo, D., Rinaldi, M., Irrera, N., Marini, H., Arcoraci, V., et al. (2016). ROS-mediated NLRP3 inflammasome activation in brain, heart, kidney, and testis ischemia/reperfusion injury. Oxidative Med. Cellular Long. 2016:e2183026. doi: 10.1155/2016/2183026
Morgenstern, L. B., Smith, M. A., Lisabeth, L. D., Risser, J. M. H., Uchino, K., Garcia, N., et al. (2004). Excess stroke in Mexican Americans compared with non-hispanic whites: the brain attack surveillance in corpus christi project. Am. J. Epidemiol. 160, 376–383. doi: 10.1093/aje/kwh225
Muñoz-Planillo, R., Kuffa, P., Martínez-Colón, G., Smith, B. L., Rajendiran, T. M., Núñez, G., et al. (2013). K+ efflux is the common trigger of NLRP3 inflammasome activation by bacterial toxins and particulate matter. Immunity 38, 1142–1153. doi: 10.1016/j.immuni.2013.05.016
Murakami, T., Ockinger, J., Yu, J., Byles, V., McColl, A., Hofer, A. M., et al. (2012). Critical role for calcium mobilization in activation of the NLRP3 inflammasome. Proc. Nat. Acad. Sci. 109, 11282–11287. doi: 10.1073/pnas.1117765109
Murray, K. N., Parry-Jones, A. R., and Allan, S. M. (2015). Interleukin-1 and acute brain injury. Front. Cell Neurosci. 9:8. doi: 10.3389/fncel.2015.00018
Nakahira, K., Haspel, J. A., Rathinam, V. A. K., Lee, S. J., Dolinay, T., Lam, H. C., et al. (2011). Autophagy proteins regulate innate immune responses by inhibiting the release of mitochondrial DNA mediated by the NALP3 inflammasome. Nat. Immunol. 12, 222–230. doi: 10.1038/ni.1980
Nyúl-Tóth, Á., Kozma, M., Nagyoszi, P., Nagy, K., Fazakas, C., Haskó, J., et al. (2017). Expression of pattern recognition receptors and activation of the non-canonical inflammasome pathway in brain pericytes. Brain Behav. Immun. 64, 220–231. doi: 10.1016/j.bbi.2017.04.010
O'Donnell, M. J., Xavier, D., Liu, L., Zhang, H., Chin, S. L., Rao-Melacini, P., et al. (2010). Risk factors for ischaemic and intracerebral haemorrhagic stroke in 22 countries (the INTERSTROKE study): a case-control study. The Lancet 376, 112–123. doi: 10.1016/S0140-6736(10)60834-3
Olmez, I., and Ozyurt, H. (2012). Reactive oxygen species and ischemic cerebrovascular disease. Neurochem. Int. 60, 208–212. doi: 10.1016/j.neuint.2011.11.009
Owolabi, M. O., Ugoya, S., and Platz, T. (2009). Racial disparity in stroke risk factors: the Berlin–Ibadan experience; a retrospective study. Acta Neurol. Scand. 119, 81–87. doi: 10.1111/j.1600-0404.2008.01077.x
Ozen, I., Ruscher, K., Nilsson, R., Flygt, J., Clausen, F., Marklund, N., et al. (2020). Interleukin-1 beta neutralization attenuates traumatic brain injury-induced microglia activation and neuronal changes in the globus pallidus. Int. J. Mol. Sci. 21:387. doi: 10.3390/ijms21020387
Palm, F., Urbanek, C., and Grau, A. (2009). Infection, its treatment and the risk for stroke. Curr. Vasc. Pharmacol. 7, 146–152. doi: 10.2174/157016109787455707
Perkins, H. T., and Allan, V. (2021). Intertwined and finely balanced: endoplasmic reticulum morphology, dynamics, function, and diseases. Cells 10:2341. doi: 10.3390/cells10092341
Pike, A. F., Varanita, T., Herrebout, M. A. C., Plug, B. C., Kole, J., Musters, R. J. P., et al. (2021). α-Synuclein evokes NLRP3 inflammasome-mediated IL-1β secretion from primary human microglia. Glia 69, 1413–1428. doi: 10.1002/glia.23970
Pradillo, J. M., Denes, A., Greenhalgh, A. D., Boutin, H., Drake, C., McColl, B. W., et al. (2012). Delayed administration of interleukin-1 receptor antagonist reduces ischemic brain damage and inflammation in comorbid rats. J. Cereb. Blood Flow Metab. 32, 1810–1819. doi: 10.1038/jcbfm.2012.101
Pu, L., Wang, L., Zhang, R., Zhao, T., Jiang, Y., Han, L., et al. (2023). Projected global trends in ischemic stroke incidence, deaths and disability-adjusted life years from 2020 to 2030. Stroke 54, 1330–1339. doi: 10.1161/STROKEAHA.122.040073
Puleo, M. G., Miceli, S., Di Chiara, T., Pizzo, G. M., Della Corte, V., Simonetta, I., et al. (2022). Molecular mechanisms of inflammasome in ischemic stroke pathogenesis. Pharmaceuticals 15:1168. doi: 10.3390/ph15101168
Py, B. F., Kim, M. S., Vakifahmetoglu-Norberg, H., and Yuan, J. (2013). Deubiquitination of NLRP3 by BRCC3 critically regulates inflammasome activity. Mol. Cell 49, 331–338. doi: 10.1016/j.molcel.2012.11.009
Rada, B., Park, J. J., Sil, P., Geiszt, M., and Leto, T. L. (2014). NLRP3 inflammasome activation and interleukin-1β release in macrophages require calcium but are independent of calcium-activated NADPH oxidases. Inflamm. Res. 63, 821–830. doi: 10.1007/s00011-014-0756-y
Ridker, P. M., Howard, C. P., Walter, V., Everett, B., Libby, P., Hensen, J., et al. (2012). Effects of interleukin-1β inhibition with canakinumab on hemoglobin A1c, lipids, C-reactive protein, interleukin-6, and fibrinogen: a phase IIb randomized, placebo-controlled trial. Circulation 126, 2739–2748. doi: 10.1161/CIRCULATIONAHA.112.122556
Ridker, P. M., Thuren, T., Zalewski, A., and Libby, P. (2011). Interleukin-1β inhibition and the prevention of recurrent cardiovascular events: rationale and design of the Canakinumab Anti-inflammatory Thrombosis Outcomes Study (CANTOS). Am. Heart J. 162, 597–605. doi: 10.1016/j.ahj.2011.06.012
Rodrigo, R., Fernández-Gajardo, R., Gutiérrez, R., Matamala, J. M., Carrasco, R., Miranda-Merchak, A., et al. (2013). Oxidative stress and pathophysiology of ischemic stroke: novel therapeutic opportunities. CNS Neurol. Disord. Drug Targets 12, 698–714. doi: 10.2174/1871527311312050015
Sandhu, J. K., and Kulka, M. (2021). Decoding mast cell-microglia communication in neurodegenerative diseases. Int. J. Mol. Sci. 22:1093. doi: 10.3390/ijms22031093
Satoh, M., Tabuchi, T., Itoh, T., and Nakamura, M. (2014). NLRP3 inflammasome activation in coronary artery disease: results from prospective and randomized study of treatment with atorvastatin or rosuvastatin. Clin. Sci. 126, 233–241. doi: 10.1042/CS20130043
Schädlich, I. S., Vienhues, J. H., Jander, A., Piepke, M., Magnus, T., Lambertsen, K. L., et al. (2022). Interleukin-1 mediates ischemic brain injury via induction of IL-17A in γδ T cells and CXCL1 in astrocytes. Neuromol. Med. 24, 437–451. doi: 10.1007/s12017-022-08709-y
Schulz, U. G. R., Flossmann, E., and Rothwell, P. M. (2004). Heritability of ischemic stroke in relation to age, vascular risk factors, and subtypes of incident stroke in population-based studies. Stroke 35, 819–824. doi: 10.1161/01.STR.0000121646.23955.0f
Segovia, J., Sabbah, A., Mgbemena, V., Tsai, S.-Y., Chang, T.-H., Berton, M. T., et al. (2012). TLR2/MyD88/NF-κB pathway, reactive oxygen species, potassium efflux activates NLRP3/ASC inflammasome during respiratory syncytial virus infection. PLoS ONE 7:e29695. doi: 10.1371/journal.pone.0029695
Sharma, D., and Kanneganti, T. D. (2016). The cell biology of inflammasomes: Mechanisms of inflammasome activation and regulation. J. Cell Biol. 213, 617–629. doi: 10.1083/jcb.201602089
Shi, J., Zhao, Y., Wang, K., Shi, X., Wang, Y., Huang, H., et al. (2015). Cleavage of GSDMD by inflammatory caspases determines pyroptotic cell death. Nature 526, 660–665. doi: 10.1038/nature15514
Siletti, K., Hodge, R., Mossi Albiach, A., Lee, K. W., Ding, S. L., Hu, L., et al. (2023). Transcriptomic diversity of cell types across the adult human brain. Science (2023) 382:eadd7046. doi: 10.1126/science.add7046
Stokes, L., Jiang, L. H., Alcaraz, L., Bent, J., Bowers, K., Fagura, M., et al. (2006). Characterization of a selective and potent antagonist of human P2X(7) receptors, AZ11645373. Br. J. Pharmacol. 149, 880–887. doi: 10.1038/sj.bjp.0706933
Stoll, G. (2002). Inflammatory cytokines in the nervous system: multifunctional mediators in autoimmunity and cerebral ischemia. Rev. Neurol. 158, 887–891.
Tan, R. Y. Y., Traylor, M., Megy, K., Duarte, D., Deevi, S. V. V., Shamardina, O., et al. (2019). How common are single gene mutations as a cause for lacunar stroke? Neurology 93, e2007–e2020. doi: 10.1212/WNL.0000000000008544
Tang, T., Lang, X., Xu, C., Wang, X., Gong, T., Yang, Y., et al. (2017). CLICs-dependent chloride efflux is an essential and proximal upstream event for NLRP3 inflammasome activation. Nat. Commun. 8:202. doi: 10.1038/s41467-017-00227-x
Tarallo, V., Hirano, Y., Gelfand, B. D., Dridi, S., Kerur, N., Kim, Y., et al. (2012). DICER1 loss and Alu RNA induce age-related macular degeneration via the NLRP3 inflammasome and MyD88. Cell 149, 847–859. doi: 10.1016/j.cell.2012.03.036
Terni, E., Giannini, N., Brondi, M., Montano, V., Bonuccelli, U., Mancuso, M., et al. (2014). Genetics of ischaemic stroke in young adults. BBA Clin. 3, 96–106. doi: 10.1016/j.bbacli.2014.12.004
Touzé, E., and Rothwell, P. M. (2008). Sex differences in heritability of ischemic stroke: a systematic review and meta-analysis. Stroke 39, 16–23. doi: 10.1161/STROKEAHA.107.484618
Tuttolomondo, A., Puleo, M. G., Velardo, M. C., Corpora, F., Daidone, M., Pinto, A., et al. (2020). Molecular biology of atherosclerotic ischemic strokes. Int. J. Mol. Sci. 21:9372. doi: 10.3390/ijms21249372
Uemura, M., Nozaki, H., Kato, T., Koyama, A., Sakai, N., Ando, S., et al. (2020). HTRA1-related cerebral small vessel disease: a review of the literature. Front. Neurol. 11:545. doi: 10.3389/fneur.2020.00545
Vajjhala, P. R., Mirams, R. E., and Hill, J. M. (2012). Multiple binding sites on the pyrin domain of ASC protein allow self-association and interaction with NLRP3 protein *. J. Biol. Chem. 287, 41732–41743. doi: 10.1074/jbc.M112.381228
Verhoef, P. A., Kertesy, S. B., Lundberg, K., Kahlenberg, J. M., and Dubyak, G. R. (2005). Inhibitory effects of chloride on the activation of caspase-1, IL-1beta secretion, and cytolysis by the P2X7 receptor. J. Immunol. 175, 7623–7634. doi: 10.4049/jimmunol.175.11.7623
Vladimer, G. I., Weng, D., Paquette, S. W. M., Vanaja, S. K., Rathinam, V. A. K., Aune, M. H., et al. (2012). The NLRP12 inflammasome recognizes yersinia pestis. Immunity 37, 96–107. doi: 10.1016/j.immuni.2012.07.006
Walev, I., Klein, J., Husmann, M., Valeva, A., Strauch, S., Wirtz, H., et al. (2000). Potassium regulates IL-1β processing via calcium-independent phospholipase A21. The J. Immunol. 164, 5120–5124. doi: 10.4049/jimmunol.164.10.5120
Wang, Q., Tang, X. N., and Yenari, M. A. (2007). The inflammatory response in stroke. J. Neuroimmunol. 184, 53–68. doi: 10.1016/j.jneuroim.2006.11.014
Wang, X., Siren, A. L., Liu, Y., Yue, T. L., Barone, F. C., Feuerstein, G. Z., et al. (1994). Upregulation of intercellular adhesion molecule 1 (ICAM-1) on brain microvascular endothelial cells in rat ischemic cortex. Brain Res. Mol. Brain Res. 26, 61–68. doi: 10.1016/0169-328X(94)90074-4
Weber, K., and Schilling, J. D. (2014). Lysosomes integrate metabolic-inflammatory cross-talk in primary macrophage inflammasome activation *. J. Biol. Chem. 289, 9158–9171. doi: 10.1074/jbc.M113.531202
Won, W., Choi, H. J., Yoo, J- Y., Kim, D., Kim, T. Y., Ju, Y., et al. (2022). Inhibiting peripheral and central MAO-B ameliorates joint inflammation and cognitive impairment in rheumatoid arthritis. Exp. Mol. Med. 54, 1188–1200. doi: 10.1038/s12276-022-00830-z
Xu, J., and Núñez, G. (2023). The NLRP3 inflammasome: activation and regulation. Trends Biochem. Sci. 48, 331–344. doi: 10.1016/j.tibs.2022.10.002
Yamasaki, Y., Matsuura, N., Shozuhara, H., Onodera, H., Itoyama, Y., Kogure, K., et al. (1995). Interleukin-1 as a pathogenetic mediator of ischemic brain damage in rats. Stroke 26, 676–681. doi: 10.1161/01.STR.26.4.676
Yang, C., Hawkins, K. E., Doré, S., and Candelario-Jalil, E. (2019). Neuroinflammatory mechanisms of blood-brain barrier damage in ischemic stroke. Am. J. Physiol. Cell Physiol. 316, C135–C153. doi: 10.1152/ajpcell.00136.2018
Yang, F., Wang, Z., Wei, X., Han, H., Meng, X., Zhang, Y., et al. (2014). NLRP3 deficiency ameliorates neurovascular damage in experimental ischemic stroke. J. Cereb. Blood Flow Metab 34, 660–667. doi: 10.1038/jcbfm.2013.242
Yang-Wei Fann, D., Lee, S. Y., Manzanero, S., Tang, S. C., Gelderblom, M., Chunduri, P., et al. (2013). Intravenous immunoglobulin suppresses NLRP1 and NLRP3 inflammasome-mediated neuronal death in ischemic stroke. Cell Death Dis. 4, e790. doi: 10.1038/cddis.2013.326
Zahid, A., Li, B., Kombe, A. J. K., Jin, T., and Tao, J. (2019). Pharmacological inhibitors of the NLRP3 inflammasome. Front. Immunol. 10:2538. doi: 10.3389/fimmu.2019.02538
Zahuranec, D. B., Brown, D. L., Lisabeth, L. D., Gonzales, N. R., Longwell, P. J., Eden, S. V., et al. (2006). Differences in intracerebral hemorrhage between Mexican Americans and non-Hispanic whites. Neurology 66, 30–34. doi: 10.1212/01.wnl.0000191402.41914.d2
Zamyatina, A., and Heine, H. (2020). Lipopolysaccharide recognition in the crossroads of TLR4 and caspase-4/11 mediated inflammatory pathways. Front. Immunol. 11:585146. doi: 10.3389/fimmu.2020.585146
Zhang, R. L., Chopp, M., Li, Y., Zaloga, C., Jiang, N., Jones, M. L., et al. (1994). Anti-ICAM-1 antibody reduces ischemic cell damage after transient middle cerebral artery occlusion in the rat. Neurology 44, 1747–1751. doi: 10.1212/WNL.44.9.1747
Zhang, S., Jiang, L., Che, F., Lu, Y., Xie, Z., Wang, H., et al. (2017). Arctigenin attenuates ischemic stroke via SIRT1-dependent inhibition of NLRP3 inflammasome. Biochem. Biophys. Res. Commun. 493, 821–826. doi: 10.1016/j.bbrc.2017.08.062
Zhao, F., Li, W., Ruan, S., Yan, F., Gao, Y., Li, M., et al. (2021). Relationship between NLRP3 activation level of inflammasome and the change of cognitive functions in patients with acute ischemic stroke. Chin. J. Behav. Med. Brain Sci. 2021, 515–521.
Zheng, F., Xing, S., Gong, Z., and Xing, Q. (2013). NLRP3 inflammasomes show high expression in aorta of patients with atherosclerosis. Heart Lung Circ. 22, 746–750. doi: 10.1016/j.hlc.2013.01.012
Zhong, Z., Umemura, A., Sanchez-Lopez, E., Liang, S., Shalapour, S., Wong, J., et al. (2016). NF-κB Restricts inflammasome activation via elimination of damaged mitochondria. Cell 164, 896–910. doi: 10.1016/j.cell.2015.12.057
Zhou, R., Yazdi, A. S., Menu, P., and Tschopp, J. A. (2011). role for mitochondria in NLRP3 inflammasome activation. Nature 469, 221–225. doi: 10.1038/nature09663
Keywords: NLRP3, inflammasome, ischemic stroke, genetic susceptibility, cardiovascular disease, brain, caspase-1
Citation: Masenga SK and Kirabo A (2024) The NLRP3 inflammasome in ischemic stroke. Front. Stroke 3:1382379. doi: 10.3389/fstro.2024.1382379
Received: 05 February 2024; Accepted: 20 May 2024;
Published: 14 June 2024.
Edited by:
Penélope Aguilera, Manuel Velasco Suárez National Institute of Neurology and Neurosurgery, MexicoReviewed by:
Guofang Shen, Beckman Research Institute, City of Hope, United StatesCopyright © 2024 Masenga and Kirabo. This is an open-access article distributed under the terms of the Creative Commons Attribution License (CC BY). The use, distribution or reproduction in other forums is permitted, provided the original author(s) and the copyright owner(s) are credited and that the original publication in this journal is cited, in accordance with accepted academic practice. No use, distribution or reproduction is permitted which does not comply with these terms.
*Correspondence: Sepiso K. Masenga, c2VwaXNvbWFzZW5nYUBnbWFpbC5jb20=; Annet Kirabo, QW5uZXQuS2lyYWJvQHZ1bWMub3Jn
Disclaimer: All claims expressed in this article are solely those of the authors and do not necessarily represent those of their affiliated organizations, or those of the publisher, the editors and the reviewers. Any product that may be evaluated in this article or claim that may be made by its manufacturer is not guaranteed or endorsed by the publisher.
Research integrity at Frontiers
Learn more about the work of our research integrity team to safeguard the quality of each article we publish.